- School of Rural and Environmental Studies, Ecology and Territory Department, Pontificia Universidad Javeriana, Bogotá, Colombia
The study of tadpole assemblages allows inferring habitat availability and using their occupation as a means of proxy for the effective reproduction of the species, contributing to complementary information for the study of their adult forms. Environmental variables, represented by abiotic variables, vegetation structure, matrix management, and landscape elements, affect species having reproductive modes associated with oviposition and development in bodies of water. In the Orinoco region, most amphibians have complex life cycles and deposit their eggs in highly dynamic lentic bodies of water. Therefore, it is important to know how larval assemblages change over short periods of water accumulation and their relationship with environmental variables. Fieldwork was conducted during 9 weeks of larval sampling, from the beginning of the rainy season. We evaluate changes in anuran assemblages associated with water accumulation in five temporary water bodies of anthropogenic (road or pasture) and natural (savanna or gallery forest) origin. Twenty environmental variables were evaluated and measured in the center of each water body. Of these, nine landscape variables were measured only once during the study. The other eleven variables, representing management practices, physicochemical and structural characteristics of the water bodies, were measured weekly during the 3 months of sampling. We explored differences in the structure and diversity of larval-stage anuran assemblages using statistical tests suitable for small sample sizes (i.e., permutational multivariate analysis of variance PERMANOVA and the distance-based linear modeling DistLM). Of the 14 species found, two species had remarkedly high abundances from which Rhinella humboldti (19% of the total tadpole abundance) was a generalist inhabiting the natural and anthropogenic water bodies, while Leptodactylus insularum (18% of the total tadpole abundance) was a specialist at a natural pond in the savanna. The natural water bodies contained the highest number of species (between 10 and 12) and a total abundance of larvae (between 847 and 485 individuals). In contrast, the anthropogenic water body tracks generated by tractors were only occupied by two species with 50 individuals in total, while the water body generated by the trampling of cattle in pastures had three species with 474 individuals. These three species that inhabited the anthropogenic puddles were also found in the natural ponds and none of the eight species of hylids inhabited the puddles. In each field trip, all the tadpoles were collected from the sampled bodies of water. However, a week later, we found that each of the water bodies had been recolonized by four species (Leptodactylus fuscus, Leptodactylus fragilis, Elachistocleis ovalis, and R. humbolti). The variables with the highest explanatory power on the variation of anuran assemblage structure throughout all the water bodies were height of plants, number of cattle, distance to the nearest native forest edge, distance to an anthropic lentic body of water, distance to a natural lentic body of water, and pH. The bodies of water immersed in the natural cover were more diverse and had a greater degree of spatial and temporal species turnover. Our study calls for the importance of understanding the turnover of larval stage anurans over short periods, associated with water accumulation, in highly dynamic systems such as natural ponds and anthropogenic puddles. The importance of species traits and local processes is also highlighted, from environmental variables to human management activities, in the conservation of amphibian assemblages.
Introduction
The Orinoco region has been transformed by livestock, crops, and mining exploitation for more than 50 years (Etter et al., 2008; Angarita-Sierra, 2014). In these landscapes, the vegetation structure and environmental heterogeneity shape the amphibian assemblages that inhabit productive systems (Caceres-Andrade and Urbina-Cardona, 2009) as well as the seasonality of flood pulses (Angarita-Sierra, 2014; Angarita-Sierra, 2021). Anurans with complex life cycles require a body of water to lay their eggs and allow their tadpoles to develop without parental care, which allows them to colonize anthropogenic cover types (Souza et al., 2008; Suazo Oruño, 2009; Costa et al., 2016).
The anurans that successfully disperse through anthropogenic matrices possess high phenotypic plasticity in the face of abiotic variations (Figueroa et al., 2009; Eterovick et al., 2015). However, the distance between natural forest cover and aquatic habitats is a limitation for effective colonization, which is reflected in the composition of anuran assemblages at the larval stage (Wells, 2007; Souza et al., 2008; Costa et al., 2016; Ouchi-Melo et al., 2017). During dispersal to and from aquatic breeding sites, adult anurans and newly metamorphosed juveniles face increased dehydration, predation, and exposure to contaminants (Crump, 2015). In that sense, anurans that reproduce in water bodies are affected by habitat fragmentation due to the disconnection between the vegetation cover that adults inhabit and the water bodies where they oviposit (Becker et al., 2007, Becker et al., 2010; Costa et al., 2016).
Depending on the reproductive mode, anurans must tolerate the different biotic and abiotic conditions found in the transformed landscapes (Caceres-Andrade and Urbina-Cardona, 2009; Cortés-Gómez et al., 2013; D’Amen et al., 2017; Isaacs Cubides and Urbina Cardona, 2011). Species that reproduce by depositing their eggs in lentic temporary bodies of water have larval stages adapted for rapid growth, followed by the terrestrial stage adapted for dispersal (Wilbur, 1987; McDiarmid & Altig, 1999; Wells, 2007; Figueroa et al., 2009). Adult anurans colonize and select oviposition sites and larval development sites using microhabitat characteristics based on abiotic features, food availability, and predation risk (Wells, 2007). Tadpoles’ survival depends on the hydroperiod, physical and chemical characteristics of the habitats, the availability of resources over time, the composition and density of predators, and the traits that allow them to adapt to highly dynamic environments such as lentic and seasonal bodies of water (McDiarmid & Altig, 1999; Wells, 2007).
In some parts of the Orinoco, anurans preferentially use temporary water bodies to deposit their eggs (Angarita-Sierra, 2014; Angarita-Sierra, 2021). Species that lay eggs in lentic bodies of water immersed in productive systems may experience truncated breeding seasons with shorter hydroperiods and run the risk of ponds drying out before the tadpoles can metamorphose (Guayara-Barragán & Bernal, 2012; Crump, 2015). Thus, breeding seasons may vary from one species to another, depending on both the phenology of the species, the size and age of the female, and postural characteristics (e.g., eggs deposited in water, bubble nest, foam nest, embedded in the dorsum of aquatic females, or carried by adults) and the responses of populations to habitat fragmentation (Wells, 2007). However, it is unknown the effect of anthropogenic matrix management and distance to different landscape elements on the anuran’s ability to colonize bodies of water in tropical flooded savanna (Eterovick et al., 2015; Costa et al., 2016; Pelinson et al., 2016).
In our study area (in the department of Casanare) 46 species of anurans have been reported, of which 28 breeds in lentic bodies of water (Pedroza-Banda et al., 2014). Also, the natural savannas have the greatest abundance and richness of anurans in the region (Blanco-Torres et al., 2017), but they are highly transformed by land-use changes for cattle ranching (Etter et al., 2008; Angarita-Sierra, 2014; Angarita-Sierra, 2021). We evaluated larval stage anuran assemblages inhabiting different bodies of water with the following objectives: 1) to determine the variation in species diversity between natural ponds (immersed in savannas or gallery forest) and anthropogenic puddles (immersed on roads or pastures); 2) to characterize temporal changes in environmental variables between bodies of water shaped by aquatic physicochemical variables, vegetation structure, land use management practices, and distance to landscape elements; and 3) to determine the relationship between changes in species diversity and the environmental variables.
We hypothesized that the anuran assemblages in natural bodies of water will have greater species richness and larval abundance than those inhabiting anthropogenic puddles. The diversity of anurans inhabiting our natural pools will be more influenced by the size of the water body and its depth, as opposed to anthropogenic puddles which will mainly depend on the temperature of the water. We expect that regardless of the origin (natural or anthropogenic) of the water body, the distance of the water bodies to the nearest native forest edge, and the vegetation structure, which allows high temporal stability in the physicochemical variables of the water, they will also influence the larval stage anuran assemblages. Temporal stability in canopy cover and other abiotic variables at natural ponds will allow for low species exchange between ponds, showing great differences in anuran diversity that inhabit highly temporal and dynamic anthropogenic puddles.
Materials and Methods
Study Area
Fieldwork was carried out in the flooded savannas of the La Moravia farm (05°21′31″N 072°13′16″W, 214 masl) in the municipality of Yopal, department of Casanare in the Orinoco region of Colombia. The climate is very humid, and the annual temperature ranges between 26°C and 32°C. Rainfall is seasonal with a dry period between November and March and a wet season from May to October. The highest precipitation is registered in July with 206.4 mm of rain, and the mean annual rainfall is 2476.3 mm (Lasso et al., 2014; 2010).
The study area comprises different natural vegetation formations such as flooded and seasonally flooded savannas, high plain savannas, sandy savannas, and gallery forests (Rodriguéz et al., 2011; Rangel, 2015). Those natural vegetation types have been transformed after anthropogenic intervention from which selective logging and grazing have the greatest impact at the landscape level (Etter et al., 2008). Currently, the predominant vegetation is the natural savanna composed of grasses, especially the Poaceae and Cyperaceae in association with some dispersed woody plants (Armenteras et al., 2005). As a flat landscape, the microtopography shapes the formation of water bodies such as temporal natural ponds and anthropogenic puddles. We selected five unconnected temporal water bodies with different environmental variables (e.g., types of substrates, depths, and amount of vegetation around the water surface) from which three were natural ponds (immersed in natural savanna or gallery forest) and two anthropogenic puddles (immersed in road or pasture; Supplementary Material S1).
Tadpole Sampling
Data were collected during the rainy season of 2008 (from May to July). The fieldwork began with the first rainfall, at the beginning of the wet season, so with each passing week, there was more accumulated water in the surveyed ponds and puddles. During the first surveyed week, all those bodies of water exhibited a similar size; therefore, the permanent sampling site was established in the center of each water body. Tadpole surveys were conducted between 9:00 and 16:00 h using small dip nets in the water column and amongst the aquatic vegetation. We sampled random microhabitats distributed throughout the area of each lentic water body, using a 30 cm × 20 cm net with a wire mesh of 3 mm2. On each body of water, we made a total of 15 sweeps during two hours, and between each pass, we waited five minutes to allow for the tadpoles to leave their shelters (Heyer et al., 1994). These sweeps were carried out along the vertical column of water, to detect larvae with different habits (neustonic, nectonic, and benthic). Due to water transparency, it was possible to visualize tadpoles on the water body substrate. For the puddle in the pasture, the surveys were conducted from 11:00 to avoid the effects of cattle muddying and making tadpole detectability difficult since one of the livestock management practices is to move the animals from one pasture to another, in the early hours of the morning.
All the tadpoles were euthanized using chlorobutanol and fixed in 10% buffered formalin for 2 weeks and preserved in a 1:1 mixture of 10% buffered formalin and water (Angulo et al., 2006). All tadpoles were determined to species using specialized taxonomic keys (Lynch, 2006; Lynch and Suárez Mayorga, 2011). Collected tadpoles were deposited in the herpetological collection of the Instituto de Ciencias Naturales (ICN) of the Universidad Nacional de Colombia. All larvae from each water body were collected during each week of sampling because the collected material was originally used in anuran taxonomy studies (Camacho-Rozo & Camacho-Reyes, 2010; Lynch and Suárez Mayorga, 2011).
Microhabitat Measurements
Twenty environmental variables were measured at the center of each body of water, from which nine were only measured once during the study (Table 1): Elevation (masl), distances to nearest bodies of water (to lentic and lotic anthropic bodies of water, as well as to stream and river), distance to the nearest natural forest edge, distance to the nearest road, distance to the nearest paddock with cattle, and distance to the nearest house, were measured with a GPS from the center of each of the sampling sites. Distances to forest edges and water bodies represent habitat supplementation opportunities for the species, while distances to pastures, roads, and houses are a proxy for threats such as habitat contamination, trampling, and predation by domestic species (e.g., cats).
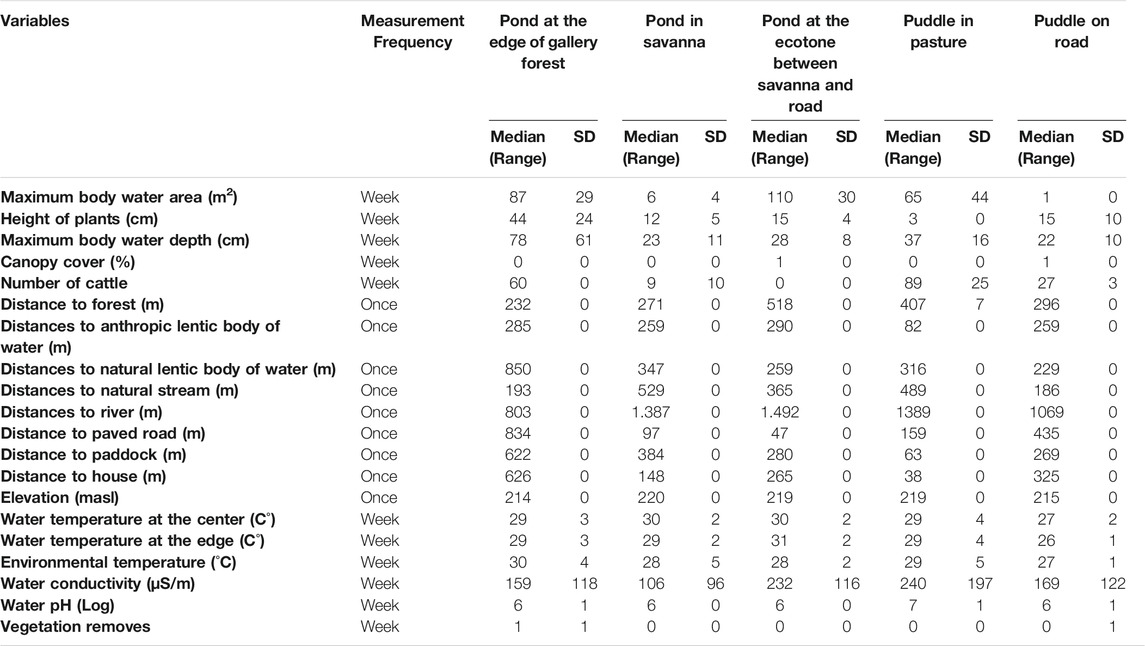
TABLE 1. Structural and environmental characterization of five bodies of water in tropical flooded savanna at the Yopal Municipality (Casanare Department, Colombia).
The other eleven variables were measured weekly, during the 3 months of sampling as follows (Table 1): temperature of the water at the center and the edge of the water body (C°), environmental temperature (°C), water conductivity, and water pH, assessed with specialized equipment for environmental and underwater measurements brand ®OAKTON Waterproof pH/CON 450 m. Canopy cover over the body of water was visually measured each week and reported as a percentage. The mean height of plants (cm) was measured with a meter from the floor to the maximum height of the emergent vegetation inside the body of water. Maximum depth (cm) of each body of water and the maximum body water area (m2) were measured from the center of each sampling site. The number of cattle and the management practice of vegetation removal (native grasses and shrubs) were measured as the degree of intensity of human activities.
Data Analysis
In the statistical analyses, the survey of each pond and puddle in each sampling week was treated as an independent sampling unit. In this regard, we used all n = 36 observations for each variable as we expected that all microhabitat measurements (measured once or weekly) influenced temporal and spatial exchange on larval stage anurans. We determined the completeness of inventory by comparing the observed number of species with different richness estimators. Each estimator was calculated with 9,999 permutations (Ugland et al., 2003) on each surveyed water body, using the program PRIMER v6.1.14 and PERMANOVA add on v1.0.4 (Clarke et al., 2008). We calculated Jacknife 1 and 2 as they assumed environmental heterogeneity along with the samples (Magurran, 2004), so we considered it suitable to estimate species richness in the entirety of the surveyed bodies of water. Chao 1 and 2 were used to estimate species richness for each pond and puddle, as it assumed habitat homogeneity (Magurran, 2004). Finally, Bootstrap was calculated for each pond and puddle, and all surveyed bodies of water due to its robustness for estimating the richness of assemblages with rare species (Magurran, 2004).
We determined the similarity of anuran species between bodies of water with the complementarity index (Colwell & Coddington, 1994; Magurran, 2004). This index relates the number of species in site A, with the number of species in site B and the number of common species between A and B. The values obtained from the complementarity analysis range from zero-percent dissimilarity (that is both sites were identical in assemblage composition), up to one when the assemblages from both sites were completely different, so they are considered as highly complementary (Colwell & Coddington, 1994).
We explored changes in anuran assemblage structure, considering species composition and abundance, on each of the five bodies of water during the nine surveyed weeks using a heat map (Somerfield & Clarke, 2013) that represents the Whittaker association index (1952) for each species surveyed in the ponds and puddles. Differences in anuran tadpole assemblage structure were tested, based on a square-root-transformed matrix of species abundances, and using a permutational multivariate analysis of variance (PERMANOVA) of a Bray-Curtis similarity matrix, a type III partial sums of squares and 9.999 permutations of the residuals under a reduced model (Anderson and Ter Braak, 2003). The experimental design of the mixed model had three factors: habitat origin (fixed factor with two levels: natural vs anthropogenic); a body of water (random factor with five levels) nested within habitat origin (fixed factor with two levels: natural and anthropogenic); and periods of water accumulation (fixed factor with three levels: May, June, and July). Significant factors and their interactions were analyzed using an a posteriori pair-wise comparison with the t-statistic and 9.999 permutations. To visualize the effects detected by the PERMANOVA in anuran tadpole assemblage structure, we performed a principal coordinate analysis (PCoA), an unconstrained ordination of multivariate data of the Bray Curtis similarity matrix (Anderson & Willis, 2003). In the PCoA, the arrow vector orientation and length represent the association, direction, and strength between the species with the ordination axis, so we visualized those species with Pearson correlation values with more than 0.5 with the two first ordination axes.
Differences in the richness and total abundance of larvae were also evaluated using a PERMANOVA under the same experimental design as above but based on Euclidean distance matrices. All analyses were conducted in PRIMER v 7 & PERMANOVA add-on software (Clarke et al., 2008; Somerfield & Clarke, 2013).
Spearman correlation coefficients were used to determine linear relationships among micro-habitat variables and to identify a subset of non-correlated variables for posterior analyses (Supplementary Material S2). We identified the best candidate models that relate tadpole assemblage structure, species richness, and total abundances to the environmental variables found in the bodies of water by running distance-based linear modeling (DistLM subroutine; Anderson et al., 2008). Using the BEST selection procedure we selected the more adjusted models, per number of variables, based on the corrected Akaike’s information criterion for small sample size (AICc; Mcardle & Anderson, 2001), from the exploration of all possible combinations of non-collinear predictor variables. The BEST routine allows measuring how closely related are two sets of multivariate data, by calculating a rank correlation coefficient between all the predictor variables (from a Euclidean distance matrix) with a Bray Curtis similarity matrix, matching this multivariate environmental structure to the multivariate species assemblage pattern. The BEST routine allows us to identify those environmental predictor variables which better explain the multivariate pattern in the assemblage structure (Clarke & Gorley, 2015). In this sense, the BEST procedure is ideal and powerful for ecological correlational studies (Anderson, 2007; Clarke & Gorley, 2015) since it avoids the problems arising from classical stepwise procedures (Johnson & Omland, 2004; Goodenough et al., 2012). Additionally, we conducted a marginal test for the variables contained in the best-fitted model in which the Pseudo-F statistic is a direct multivariate analog of Fisher’s F ratio in the traditional regression (Anderson et al., 2008), and additionally reported the proportion of the variance explained by each variable. We carried out a distance-based redundancy analysis (dbRDA subroutine) as a linear combination of explanatory variables of the best-fitted model on anuran tadpole assemblage structure (Legendre & Andersson, 1999). Redundancy is then considered as the proportion of multivariate variability explained by the predictor variables (Mcardle & Anderson, 2001). Through the visual inspection of the ordination performed in dbRDA it is possible to identify the direction and strength of the association between each predictor (environmental) variable and the axes of ordination represented by their orientation and length. In this sense, these vectors can be interpreted as the effect of each of the environmental variables on the constrained ordination showing the strength of the relationships between each predictor variable and the dbRDA axes (Anderson et al., 2008).
Once the predictor variables of the best-fitted model were identified, the changes in the abundances of each species along the environmental variables with the highest proportion of explained variance were determined. Coherent groups of species with statistically indistinguishable patterns of response to each of the environmental variables (but significantly different between coherent groups) were identified from the Type 3 SIMPROF test with 9,999 Monte Carlo simulations of the similarity profiles (Somerfield & Clarke, 2013). This routine allowed us to explore, through the Pi statistic, to what extent the similarity data of species abundances along the gradient in the predictor variable (the observed profile) deviated from the null distribution of the simulated similarity profiles (Clarke et al., 2008). All analyses were conducted using the PRIMER v6.1.14 & PERMANOVA add-on v1.0.4 software (Clarke et al., 2008).
Results
Larval Stage Anuran assemblage’ Diversity
After 120 people/hours of sampling effort, we collected 2416 tadpoles belonging to 14 species (Table 2). The two dominant species in the studied bodies of water were Rhinella humboldti (with 458 individuals, representing 19% of the total tadpole abundance) and Leptodactylus insularum (with 442 individuals; 18% of the total tadpole abundance) (Table 2).
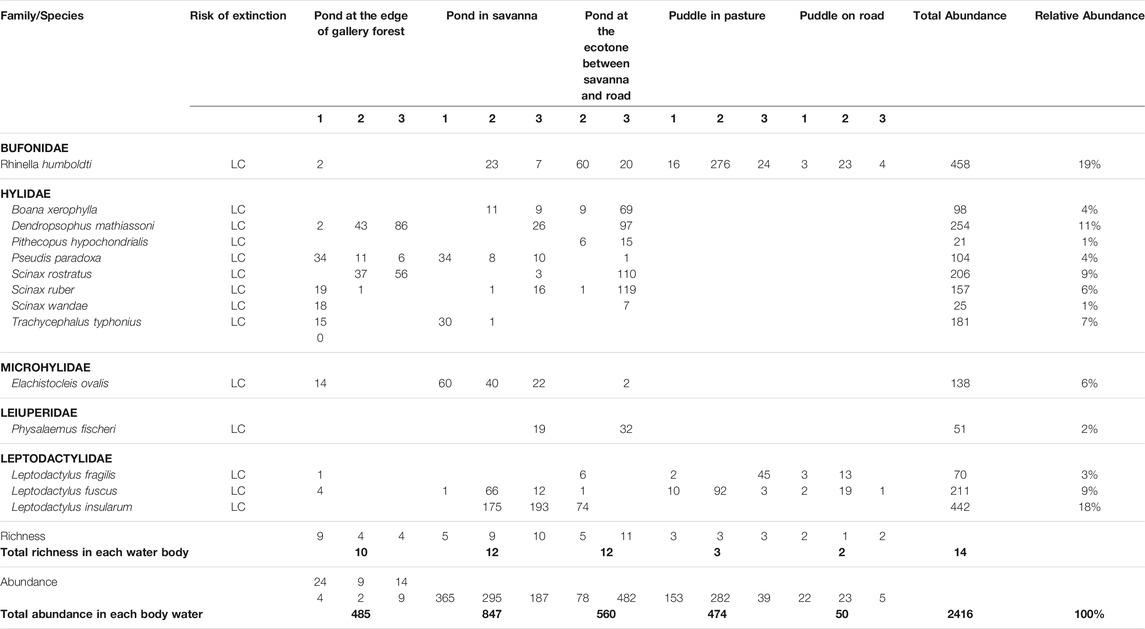
TABLE 2. Composition, richness, and abundance of anurans in larval developmental stage found in five bodies of water in tropical flooded savanna of the Yopal Municipality (Casanare Department, Colombia). On the second row, under the name of the type of each body of water, the numbers represent the surveyed month, in which 1 is the beginning of the rainfall seasons and 3 is the month with the highest precipitation; representing a temporal dynamic of water accumulation in the bodies of water.
The completeness of the inventory for all sampled water bodies fluctuated between 99.4 and 100% (Supplementary Material S3), showing asymptotic species accumulation curves (Figures 1A,B); so, it is assumed that the 14 species found, adequately represented the assemblages of anurans that oviposited in ponds and puddles (Table 2). The observed number of species in each water bodies accounted for 90–100% of the estimated species richness, but ponds had between 4 and 6 times more species richness than puddles (Figures 1A,B; Supplementary Material S3).
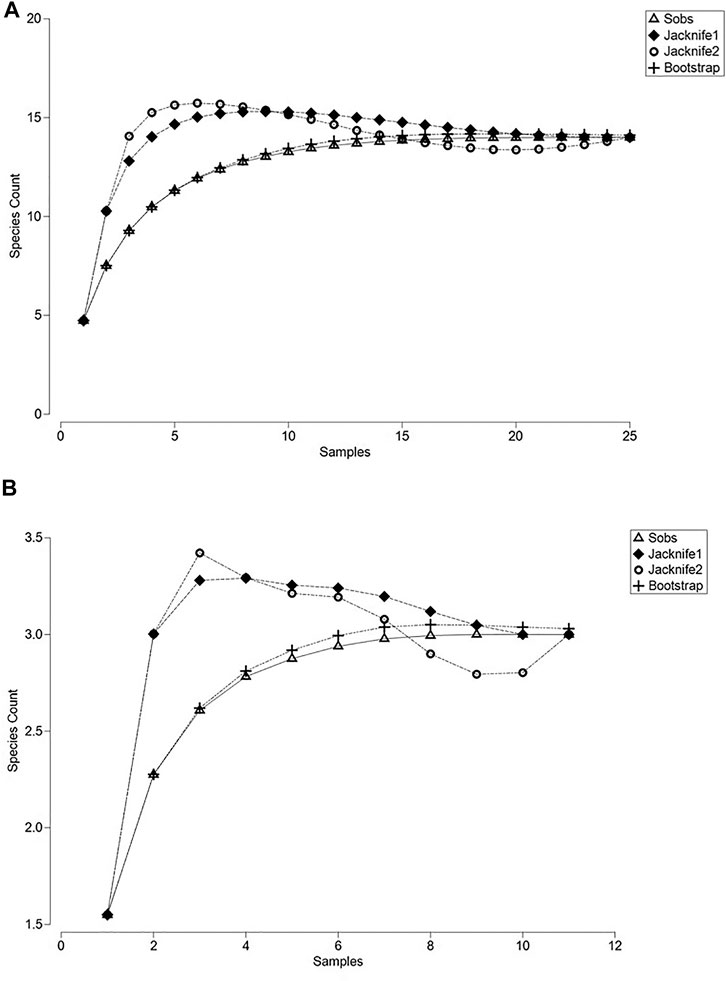
FIGURE 1. Species accumulation curves of anurans found in larval stage in water bodies in tropical flooded savanna of the Yopal Municipality (Casanare Department, Colombia). (A) Species accumulation curves of tadpoles in natural ponds. (B) Species accumulation curves of tadpoles in anthropogenic puddles.
The average similarity between the sampled water bodies was 0.6, with the lowest complementarity values were presented between ponds in ecotone vs in savanna (0.28) and in savanna vs in gallery forest edge (0.31). The highest complementarity values were found between puddles (in pasture and on-road) and ponds (values from 0.7 to 0.85; Table 3). There were only two species that were unique for a specific body of water inhabiting the natural pond in savanna (Leptodactylus insularum) and the pond in the ecotone between savanna and road (Pithecopus hypochondrialis), but their abundance changed during the periods of water accumulation (Table 2), defining their degree of association with a specific body of water (Supplementary Material S4).
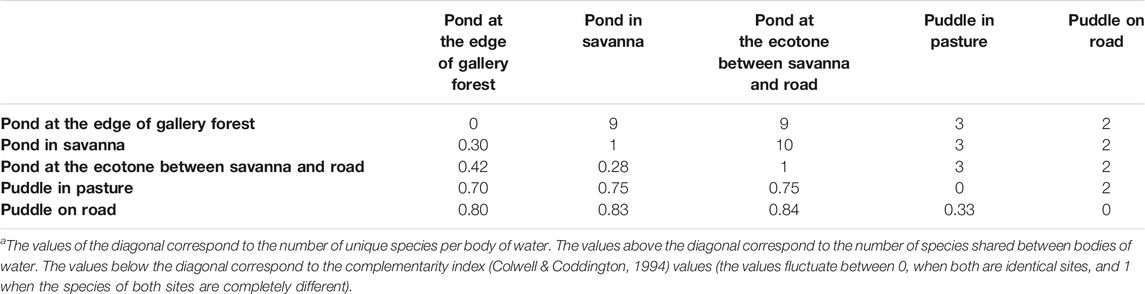
TABLE 3. Complementarity of anuran species in the larval developmental stage between five bodies of water in tropical flooded savanna of the Yopal Municipality (Casanare Department, Colombia) a.
The anuran assemblage structure was different between the three periods of water accumulation (Pseudo-F = 3.21, p (perm) = 0.029) and between the bodies of water nested in the original habitat (Pseudo-F = 3.12, p (perm) = 0.0001); this last factor had the highest estimated component of variation between all the evaluated factors (Table 4). In general, all the natural ponds were different from each other but there were no differences between the anthropogenic puddles in pastures and roads (t = 0.78; p (perm) = 0.61). The first two axes of the PCoA captured 50.7% of the variation in the anuran assemblage structure among the bodies of water (Figure 2; Supplementary Material S5). Along the first axis, the separation between anthropogenic puddles and natural ponds was evident. Natural ponds exhibited the greatest temporal variation along this axis and the species responsible for this differentiation were, in descendent order of association with the first coordinate the following: Dendropsophus mathiassoni, Scinax rostratus, Leptodactylus fuscus, Scinax ruber, and Pseudis paradoxa. The second coordinate represented the variability in assemblage structure in anthropogenic puddles (in road and pasture) and the species responsible for this variation were, in descendent order of association with the coordinate: Rhinella humboldti, Leptodactylus fuscus, and Leptodactylus fragilis (Figure 2).
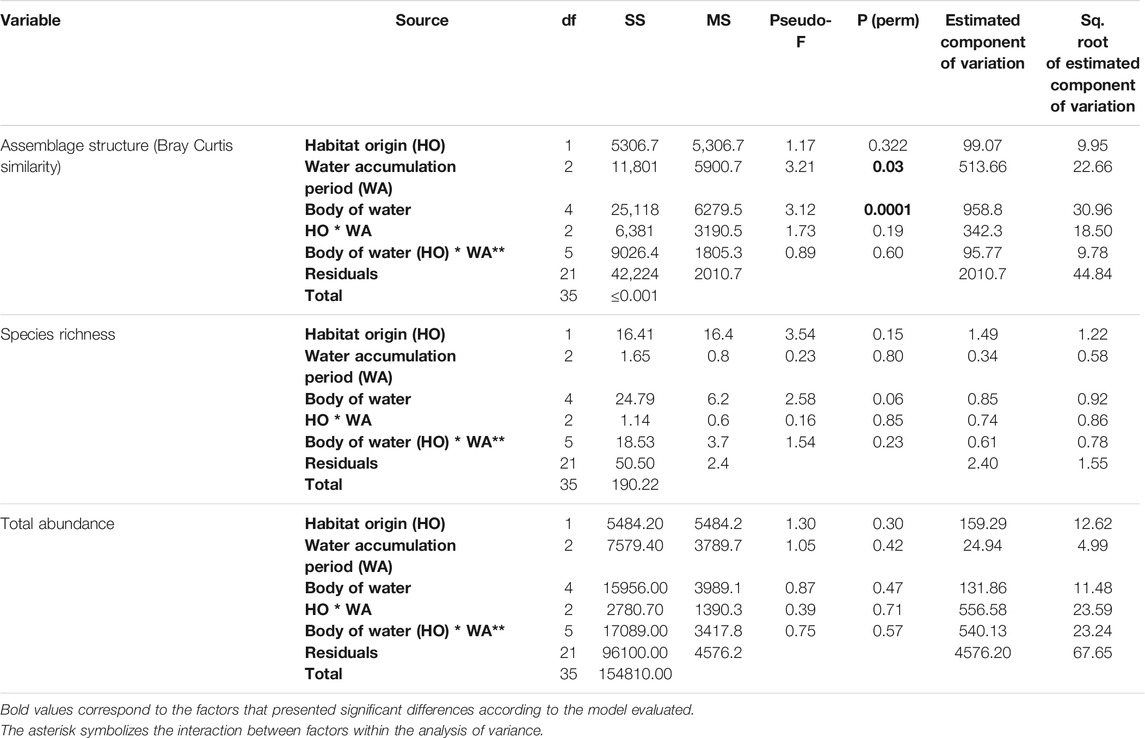
TABLE 4. Results from the three-way PERMANOVA analysis of the structure of anuran assemblages in larval stage inhabiting five bodies of water in tropical flooded savanna in the Yopal Municipality (Casanare Department, Colombia). The mixed model included habitat origin (HO), the body of water (HO), and the water accumulation (WA) period as factors.
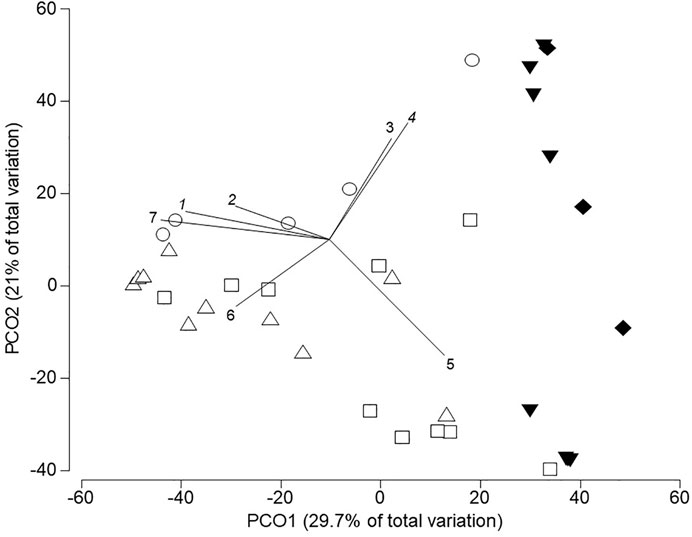
FIGURE 2. Ordination of anuran assemblages in larval developmental stage across five bodies of water in tropical flooded savanna of the Yopal Municipality (Casanare Department, Colombia). Vectors correspond to 7 out of 14 species that presented a high correlation (≥0.5) with the first two axes. Geometric figures represent each body of water surveyed in different times of the rainy season. White symbols correspond to natural ponds and black symbols to anthropogenic puddles as follows: ∆ pond in the edge of gallery forest; □ pond in savanna; ○ pond in the ecotone between savanna and road; ▼ Puddle in pasture; and ♦ Puddle on road. Species codes of anuran species are: 1. Scinax rostratus; 2. Scinax ruber; 3. Leptodactylus fragilis; 4. Rhinella humboldti; 5. Leptodactylus fuscus; 6. Pseudis paradoxa; 7. Dendropsophus mathiassoni.
Anuran assemblage’ Diversity and Environmental Correlates on Five Bodies of Water
Given the high degree of collinearity among the environmental variables, only 13 were considered as predictor variables in the models (Supplementary Material S2). The best fitted model included six predictor variables that explained the variation of anuran assemblage structure (AICc = 287.09; R2 = 0.476; RSS = 63.461; Table 5). The dbRDA subroutine allows us to identify the weight of each predictor variable in the model from the length of the vector in the ordination, which are, in descending order from the most to the least explanatory power: the height of plants, distance to the nearest native forest edge, distance to the natural lentic body of water, pH of the water, distance to the anthropic lentic body of water, and number of cattle (Figure 3). All of these variables were significant to the model and had a proportion of explained variance from 0.06 to 0.13 (Supplementary material S6).
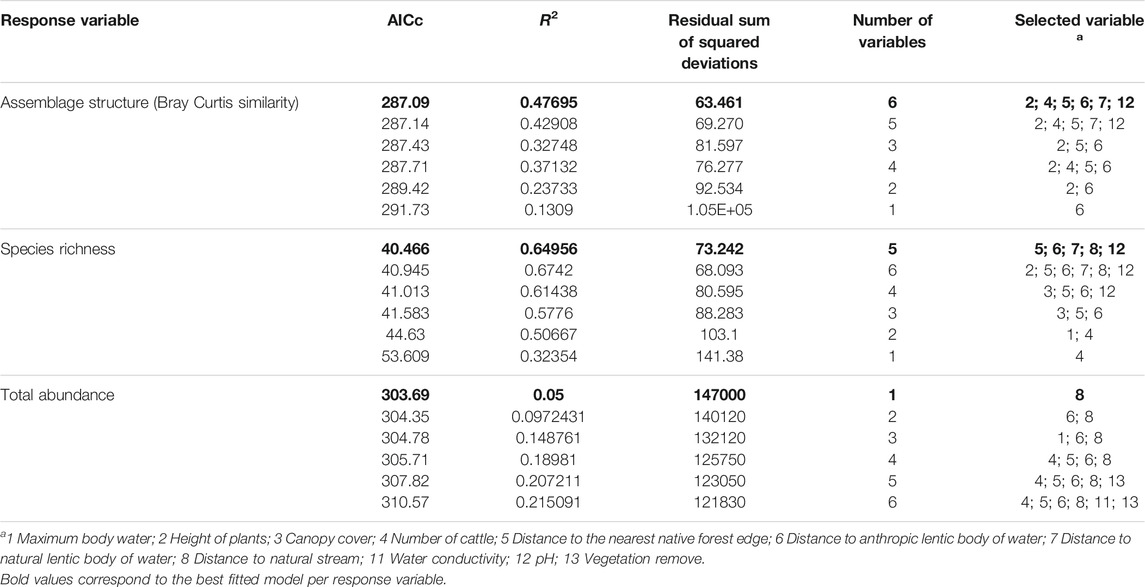
TABLE 5. Best fitted models per number of variables, ranked by the lowest to the highest AIC value, explaining anuran assemblage structure, species richness and total abundance in five bodies of water in tropical flooded savanna at the Yopal Municipality (Casanare Department, Colombia). The best-fitted model based on the value of AICc is shown in bold.
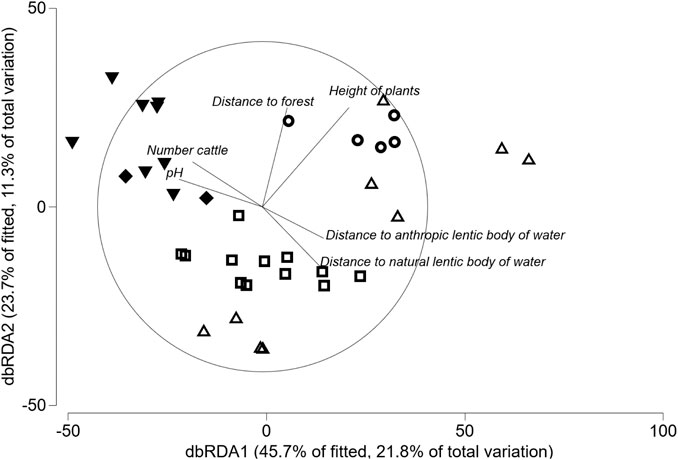
FIGURE 3. Distance-based redundancy analysis of changes in anuran assemblages based on changes in environmental variables in five bodies of water in tropical flooded savanna of the Yopal Municipality (Casanare Department, Colombia). Based on the best fitted model (DistLM), the first two dbRDA axes explained 32.6% of the variation in the anuran assemblage structure along bodies of water. Vectors correspond to the four most important environmental variables explaining changes in the anuran assemblage. The direction and the association strength between the environmental variables and the ordination axis are represented by arrow orientation and length. Geometric figures represent each body of water surveyed in different times of the rainy season, as follows: natural ponds (∆ Pond in the edge of gallery forest; □ Pond in savanna; ○ Pond in the ecotone between savanna and road); and anthropogenic puddles (▼ Puddle in pasture; and ♦ Puddle on road).
Species richness of anurans was explained by distance to the nearest native forest edge, distance to the anthropic lentic body of water, distance to the natural lentic body of water, distance to the natural stream, and pH (AICc = 40.466; R2 = 0.649; RSS = 73.242) (Table 5; Supplementary material S6). However, only the Distance to anthropogenic lentic water body (p = 0.02; Supplementary material S6) and pH (p = 0.04; Supplementary material S6) were significant to the model with a proportion of explained variance of 0.29 and 0.12, respectively. The total abundance of anurans was explained by distance to natural stream (AICc = 303.69; R2 = 0.05; RSS = 147000) (Table 5; Supplementary material S6), and it was significant to the model (p = 0.018) but with a low proportion of explained variance (0.05; Supplementary material S6). Finally, when evaluating the pattern of coherence and abundance for each of the species along with the environmental variables, differential patterns were found for the three predictor variables with the highest proportion of explained variance (Supplementary material S6). Along the gradient of pH values the species were grouped into six coherent clusters (Pi statistic = 3.63; p = 0.0001; Supplementary material S7A), along the height of plants above the water body they were grouped into four groups (Pi statistic = 4.74; p = 0.004; Supplementary material S7B) and along the gradient of distance to anthropic lentic body of water, the grouping of the species was not differentiated (Pi statistic = 5.56; p = 0.072; Supplementary material S7C).
Discussion
In this work, the sample size was small, having local results that do not represent the totality of the landscapes and bodies of water of the Orinoco region. Despite the limitation of the low sample size, this work is very relevant due to the detailed description of the temporal change, since the beginning of the rainy season, in the environmental variables and their relationship with the larval stage anuran assemblages. In this sense, the strength of the present study lies in the high degree of local detail in the description of a few water bodies but at no time should it be generalized to other natural ponds or anthropogenic puddles.
The richness estimators allowed a determination of inventory completeness of between 90 and 100% from the sampling techniques used, and from the sampling effort invested during the rainy season surveyed (Supplementary Material S3). The advantage of restricting the sampling to temporary lentic bodies of water in open areas is that the visual detection of tadpoles is high and the capture with a net is efficient. The sampling of tadpoles could complement the information on the abundance of the species in their adult form. As an example, in a study in the natural savannas of Venezuela, Tarano (2010) reports 26 adult individuals of Leptodactylus fuscus from a sampling effort of around 150 h/person. This study reported (with 120 h/person) 211 larvae of the same species. Such a trend is also seen in Leptodactylus fragilis (20 adults reported by Tarano 2010 vs 70 larvae reported in this study). This pattern of abundances also suggested that anurans have a higher rate of predation in the larval stage than in the adult, due to the high population sizes of macroinvertebrate predators of anuran larvae present in these natural ponds (Camacho- Reyes and Camacho- Rozo, 2010). The lentic bodies of water have a great variety of adult vertebrate animals, as well as aquatic insects and tadpoles of other species that exercise population control (Heyer et al., 1975; Azevedo-Ramos et al., 1999; Mcintyre et al., 2004; Teixeira-Borges and Duarte Rocha, 2013).
It is important to consider that the data of the present study are local and only represent the assemblages of anurans inhabiting our five temporary bodies of water during the rainy season of 2008, in which adults deposit their eggs in temporal lentic bodies of water, a place where their larvae also develop. Therefore, when comparing our results with previous studies in the same municipality of Yopal (Department of Casanare at the Orinoco region of Colombia; Acosta-Galvis, 2017; Pedroza-Banda et al., 2014), there still would be missing between three and five additional species in the inventory. However, it is worth noting the absence of species such as Rhinella marina and Engystomops pustulosus in our samplings as they are considered common in open natural savannas that also tolerate anthropogenic land-uses (Caceres-Andrade and Urbina-Cardona, 2009; Pedroza-Banda et al., 2014; Acosta-Galvis, 2017). The absence of these species may be due to changes in their reproductive times, to reduce interactions with other dominant species, such as Leptodactylus fuscus, in temporary lentic water bodies as previously reported by Downie et al. (2008) in an ex-situ experiment. However, to truly demonstrate in situ the temporal partitioning of the species within the assemblages, long-term monitoring of the larval stage anurans that inhabit the Orinoco region is essential.
Temporal Dynamics of Species of Anurans in a Larval Stage Between Bodies of Water
According to Angarita-Sierra (2014), the ecological dynamics of the amphibian assemblages in the flooded savannas of Colombia is mainly determined by three factors: 1) the marked seasonality between the dry and rainy seasons; 2) the flood pulse of the main streams and rivers, and 3) the degree of transformation of the native covers to give way to human activities. The occupation of anurans in those lentic water bodies, shown by the presence of larvae at the beginning of the rainy season, and their absence in these assemblages during the subsequent flood periods, show the high temporal dynamics in savanna ecosystems. Previous studies have indicated that these temporal dynamics could be mediated by biotic interactions (e.g., presence of competitors and predators in ponds; Camacho- Reyes and Camacho- Rozo, 2010) and environmental gradients (e.g., desiccation rate of the water body and dynamics in structural complexity; Ouchi-Melo et al., 2018; Pelinson et al., 2016).
The only three species that occupied the two surveyed anthropogenic puddles also occupied the three natural ponds throughout the flood period surveyed (Table 2; Supplementary Material S4). Studies in the savannas of Brazil demonstrate that puddles of anthropic origin show more fluctuating abiotic characteristics, evident in changes in the temperature and depth of the water body (Fletcher et al., 2018) that makes them inhospitable and unsuitable for many species of anurans, allowing the establishment of a limited group of species. In the present study, Leptodactylus fragilis, and Leptodactylus fuscus have been considered as anthropogenic habitat generalists, with low conservation concern (as has also been suggested by Heyer et al., 2010; Reynolds et al., 2004). The high abundance of Leptodactylus fragilis, Leptodactylus fuscus, and Rhinella humboldti in the puddle immersed in the pasture can be explained by traits allowing them to tolerate anthropogenic environments and adapt to rapid desiccation of water bodies, as well as the low supply of resources (McDiarmid & Altig, 1999; Wells, 2007; Lucas et al., 2008; Sanuy et al., 2008; Caceres-Andrade and Urbina-Cardona, 2009; Figueroa et al., 2009; Ouchi-Melo et al., 2018).
Relationship Between Anuran Traits and Response to Natural and Anthropogenic Habitats
The natural ponds had species of anurans that, given the similarity in their traits, are highly competitive and potentially predatory on the eggs of other anurans (sensuPetranka & Kennedy, 1999; Teixeira-Borges and Duarte Rocha, 2013). In turn, these bodies of water contained many aquatic insects (Camacho- Reyes and Camacho- Rozo, 2010) compared to pools of anthropic origin (unpublished data). Thus, the origin and size of the water body could affect the diversity of species and resources (Schriever & Williams, 2013; Teixeira-Borges and Duarte Rocha, 2013). Teixeira-Borges and Duarte Rocha, 2013 found that, in temporary water bodies, aquatic invertebrates are the main predators of anuran larvae (e.g., the eggs of Leptodactylus fuscus tend to be eaten by Diptera; Downie et al., 1995). Environmental gradients in ephemeral ponds change over time and show an irregular distribution of resources in space and time (Wells, 2007; Schalk et al., 2017). The ecological dynamics in these assemblages of tadpoles could not depend only on predation but on the trophic plasticity of the species to exploit a wide range of resources and reduce inter and intra-specific competition (McDiarmid & Altig, 1999; Wells, 2007; Eterovick et al., 2015; Schalk et al., 2017).
To get a more complete picture of the spatiotemporal dynamics of tadpole assemblages, it is necessary to study them from the perspective of trait-based ecology. The reproductive mode is a key functional trait of anurans as it condenses aspects ranging from life history to developmental biology, ecology, evolution, physiology, and behavior of species (Crump, 2015). Likewise, reproductive modes help to understand the use of habitat by species, although generalizations should not be made at the genus or family level (Haddad & Prado, 2005). Some studies have suggested that species that lay their eggs in lentic bodies of water and whose larvae develop there (without parental care) tend to be more tolerant of anthropogenic landscape transformation (Pineda & Halffter, 2004; Urbina-Cardona & Reynoso, 2009; Suazo-Ortuño et al., 2018). However, in the present study, we found divergence in habitat use by some species of the same genus. Leptodactylus fragilis, and Leptodactylus fuscus inhabited the anthropogenic puddles and showed a reproductive mode associated with oviposition within foam nests (Crump, 2015). Lynch (2006) previously suggested that the creation of foam nests can be a successful strategy to colonize highly dynamic and ephemeral anthropogenic bodies of water such as puddles. In contrast, Leptodactylus insularum was the most abundant (n = 442 individuals) lepdodactylid frog in this study, but it lived exclusively in the pond immersed in the natural savannah where abundant positions grouped tadpole schooling where the development stages were similar in the species of the same lot (unpublished data).
Habitat use by anurans and the oviposition period may vary among species with complex life cycles. While Leptodactylus fuscus, Leptodactylus fragilis, Elachistocleis ovalis, and Rhinella humbolti were present during the 3 months of sampling. We found eight species of Hylidae inhabiting the natural ponds but none in the anthropogenic puddles, although this Family presents the greatest diversity of reproductive modes worldwide (Haddad & Sawaya, 2000). The degree of specialization of some species of hylids can be seen in Pithecopus hypochondrialis, recorded in the only pond that had full canopy coverage over the body of water in natural savanna (Table 2; Supplementary Material S4). This species is seasonal and reproductively active in the rainy season, which is why it is associated with ephemeral bodies of water surrounded by shrub vegetation where adults live (Acosta-Galvis, 2017).
The plasticity of some species against the desiccation of ponds and the increase in environmental temperature has positive effects on the survival of populations and the colonization of transformed environments (Denver, 1997; Denver et al., 1998; Leips & Travis, 1994; Sanuy et al., 2008; Wells, 2007). Another important trait in amphibians is the thickness and permeability of the skin, as well as the strategies to reduce the loss of body water in dehydrating environments (Urbina-Cardona et al., 2014). In the present study, some of the species that occupied anthropogenic puddles (Rhinella humboldti, Leptodactylus fragilis, and Leptodactylus fuscus (Figure 2) have thick and less permeable skin in adulthood (Mendoza Roldán and Crawford, 2014), maintaining a constant loss of evaporative water between populations of different ecosystems (Cruz-Piedrahita et al., 2018). These traits allow them to move to and from aquatic breeding sites in highly transformed environments, reducing their risk of mortality due to dehydration (sensuNowakowski et al., 2017, 2018; Watling & Braga, 2015).
During the larval stage of the life cycle of anurans, the duration of metamorphosis and the size of the larva are two important traits for their fitness (Gascon, 1991; Leips & Travis, 1994; Hero et al., 1998; Wells, 2007; Sanuy et al., 2008; Figueroa et al., 2009; Eterovick et al., 2015). However, some species have differential strategies for colonizing ephemeral bodies of water and in general anthropized landscapes. Leptodactylus fuscus tadpoles can survive around 6 days out of the water, once the water body dries up, increasing body water retention mainly when individuals are all together (Downie & Smith, 2003; Venturelli & Klein, 2019). Rhinella humboldti tends to saturate bodies of water, ovipositing up to 5,000 eggs, increasing competition for resources with other species (Guayara-Barragán & Bernal, 2012). For its part, Leptodactylus fragilis has such a degree of physiological plasticity that it has even managed to invade island ecosystems, due to the strength of its vocalization in open areas (del Castillo Domínguez et al., 2021). The above strategies, based on functional traits, allow us to better understand the composition and diversity patterns of larval stage anurans found throughout the five water bodies studied.
Relationship Between Environmental Variables of the Habitat and the Diversity of Anurans in the Larval Stage
Another key aspect that defines habitat partition and use by tadpoles in temporary lentic bodies of water, depend on the dynamics in environmental variables (Caceres-Andrade and Urbina-Cardona, 2009; D’Amen et al., 2017; Ouchi-Melo et al., 2018; Wilbur, 1987). In the present study, it was shown how different facets of the taxonomic diversity of anurans (such as species richness and assemblage structure) are influenced by similar environmental variables such as height of plants, number of cattle, distance to the nearest native forest edge, distance to the anthropic lentic body of water, distance to the natural lentic body of water, and pH (Table 5; Figure 3). This suggests that, like the pattern found by Ouchi-Melo et al. (2018), the tadpoles that inhabit natural ponds, select similar environmental characteristics (Ouchi-Melo et al., 2018), since these types of water bodies are more heterogeneous than anthropogenic puddles (Supplementary Material S5), so natural pools have greater availability of food, space, and less probability of predation (Eterovick & Barata, 2006; Kopp et al., 2006).
We hypothesized that anuran diversity inhabiting natural ponds will be influenced by water body size and depth of bodies of the water and the distance from the bodies of water to different landscape elements. This study reinforces the importance of the distance to the edge of the nearest native forest and the distance to different type of bodies of water influencing the abundance and spatial distribution of tadpoles (Pelinson et al., 2016). These variables are important for the larval stage assemblage structure, as well as for species richness. Recent reviews of edge and matrix effects on amphibians indicate that the abundance of forest specialist species is affected up to 1900 m (mean depth = 408 m; median = 250 m) into the native forest, while matrix specialist species show changes in their abundance up to 770 m into the productive system (mean depth = 117; Pfeifer et al., 2017; Schneider-Maunoury et al., 2016).
The second hypothesis of the present study was that the vegetation cover would have a strong influence on the tadpoles inhabiting natural ponds because previous studies have suggested the survival of the larvae in temporary water bodies depends on the complexity in the surrounding plant structure allowing an adequate habitat for larval development (Pelinson et al., 2016; Ouchi-Melo et al., 2018). However, our results suggest that the height of plants, the distance to the nearest native forest edge and the distance to other lentic waterbodies are more important for the structure of the anuran assemblage as a refuge for the adults than the canopy cover over the pond. The selection of breeding microhabitats in anurans with reproductive modes associated with oviposition in lentic water bodies would not only be determined by the tolerance of the species to changes in environmental variables but also by their dispersal capacity to arrive from the remaining native forest (Pelinson et al., 2016). The connectivity between terrestrial habitats (e.g., forested areas) and aquatic reproductive sites is important for juvenile survival and dispersal (Souza et al., 2008; Costa et al., 2016). In contrast, Eterovick et al. (2015) found that developmental rates and fluctuating asymmetries in the larval stage of some anurans were not explained by landscape metrics. This inconsistency of our results pinpoints a major research challenge on the effects of landscape configuration and management practices for the assemblages of anurans in the larval stage inhabiting natural savannas (Iop et al., 2020). In the present study, we showed that some species of anurans oviposit on ponds that are highly affected by the constant presence of cattle (Table 2; Supplementary Material S1).
The presence and trampling of livestock can affect vegetation cover and alter water quality, reducing aquatic foraging and oviposition sites for anurans (Burton et al., 2009). However, we found that the number of cattle, which reached 89 for one of the puddles (Table 1), did not have a strong relationship with canopy cover, water pH, or conductivity (Supplementary Material S2). Some studies have even shown that the reproduction of L. fragilis may benefit from the trampling of cattle, because it generates small bodies of water without predators or competitors (Pedroza-Banda et al., 2014), like other species (Pseudopaludicola mystacalis; Pelinson et al., 2016), reinforced in the present study by the greater abundance of larvae of L. fragilis in puddles in pastures rather than in ponds in natural savanna or gallery forest (Table 2; Supplementary Material S4).
A third hypothesis was that anthropogenic puddles would have higher water temperature due to high solar exposure, determining low species richness and abundance of tadpoles. Scientific literature has suggested that abiotic factors such as environmental temperature, photoperiod, water level, and quality, directly influence tadpoles and the physiological mechanisms related to their growth and development (Denver, 1997; Figueroa et al., 2009; Sanuy et al., 2008). However, in the present study, the temperatures at the edge and center of ponds and puddles had similar values, of which the puddle on-road had slightly lower values due to the high canopy cover (Table 4). Due to the above, the environmental and water temperatures were not important for explaining changes in the assemblages of tadpoles. Thus, the pH of the water was the only environmental variable that allowed an explanation of the changes in the structure of the assemblages (Figure 3; Supplementary Material S7A). Many species require intermediate pH values in the water for their development, because, at low values, the ionic balance reduces the survival of organisms (Freda & Dunson, 1985; Thabah et al., 2018). The gradient in the pH values led to greater abundances of larvae between 5.2 and 6.5 for the natural ponds (1120 individuals; 61.8% of the larvae) and between 5.8 and 7 for the anthropogenic puddles. (415 individuals; 79.9% of the larvae). A similar pattern was found with the species richness of anurans in the three natural ponds, but not for the two anthropogenic puddles.
Final Thoughts on the Conservation of Anurans in Transformed Savanna Landscapes
We found that, during a rainy season, the temporary lentic bodies of water, immersed in pastures and roads, were occupied by at least three species of anurans that may be using different strategies associated with their traits. The high plasticity of these species allows them to occupy and reproduce in these anthropogenic environments, which, due to the low quality of habitat for other species, it presents less pressure for competition and predation than in natural ponds. The livestock practices that include the passage of cattle over bodies of water and the transit of tractors through agricultural lands can generate a degradation in the quality of habitat for most species of anurans (Pelinson et al., 2016), coupled with the anthropogenic transformation of gallery forests and natural savannas for the expansion of pastures (Pedroza-Banda et al., 2014). To the extent that these anthropic activities affect emergent vegetation of water bodies (height of plants), water pH, and eliminate elements of native gallery forests, the survival of anurans that reproduce in lentic water bodies could be threatened, homogenizing assemblages of anurans with complex life cycles.
Based on the data obtained from the five temporary lentic water bodies in natural savannas, we suggest that future research should investigate the effect on larval stage anurans of the local management of different environmental variables. Preliminarily, our data suggest that it is important to keep the following variables within certain ranges of values: height of grasses between 3 and 65 cm, and pH values in water between 5.2 and 6.5. We suggest exploring management activities of the grasses and herbaceous plants in the natural ponds, maintaining a height greater than 13 cm could help reduce the dominance of the three generalist species that also occupied the anthropogenic puddles. However, future research needs to assess the degree of competition that Leptodactylus fragilis, Leptodactylus fuscus, and Rhinella humboldti generate with the other species of the anuran assemblage that inhabit temporary natural ponds during their larval stage.
The structure of the assemblages of tadpoles could be also influenced by variables of the landscape at a regional scale, such as the proximity of the ponds to the edge of the gallery forest and the other closer lentic waterbodies. This supports the importance of these landscape elements as critical in the connectivity of the local assemblages of larval stage anurans (Souza et al., 2008; Hernández-Ordóñez et al., 2015). However, it is essential to carry out studies of edge effects on the anuran assemblages of the ecosystems of the Orinoco basin that border different types of anthropic land use. This line of research is a high priority since the constant transformation of these ecosystems has conditioned the spatial distribution of adult anurans, due to their strong dependence on local environmental variables and the spatial distribution of bodies of water for oviposition (Pedroza-Banda et al., 2014; Ouchi-Melo et al., 2017, 2018). Spatio-temporal changes in the structure of the assemblages of anurans in the larval stage point to some species as possible indicators of the state of conservation of the ecosystem (Welsh & Ollivier, 1998). Given their functional traits, those species could be sensitive to local changes in environmental variables (Álvarez-Grzybowska et al., 2020) due to its low dispersion capacity and high permeability of the skin being affected by changes in the vegetation cover (McDiarmid & Altig, 1999; Wells, 2007).
Data Availability Statement
The original contributions presented in the study are included in the article/Supplementary Material, further inquiries can be directed to the corresponding author.
Ethics Statement
This work did not have an ethical evaluation because at the time of the undergraduate work in 2008 the institution (Universidad Pedagógica y Tecnológica de Colombia) did not have an ethics committee.
Author Contributions
CC-R led the writing of the original draft, with text contributions from NU‐C. CC‐R conducted the fieldwork and organized the data. NU‐C analyzed the data. CC‐R formatted the manuscript. All authors contributed to manuscript writing, review, editing, and approved the submitted version.
Conflict of Interest
The authors declare that the research was conducted in the absence of any commercial or financial relationships that could be construed as a potential conflict of interest.
Publisher’s Note
All claims expressed in this article are solely those of the authors and do not necessarily represent those of their affiliated organizations, or those of the publisher, the editors and the reviewers. Any product that may be evaluated in this article, or claim that may be made by its manufacturer, is not guaranteed or endorsed by the publisher.
Acknowledgments
David Sánchez and Jhon D. Lynch collaborated on the corroboration and taxonomic identification of specimens. Specimens were collected under the permit (Resolution 226) of the National Authority of Environmental Licenses (ANLA) and deposited in the Scientific Collection of the Universidad Nacional de Colombia at the Instituto de Ciencias Naturales. This manuscript is the third publication of the Semillero Javeriano en Ecología y Conservación de la Herpetofauna-SECAR.
Supplementary Material
The Supplementary Material for this article can be found online at: https://www.frontiersin.org/articles/10.3389/fenvs.2021.667448/full#supplementary-material
References
A. Angulo, J. V. Rueda-Almonacid, J. V. Rodriguez- Mahecha, and E. La Marca (2006). Técnicas de inventario y monitoreo para los anfibios de la región Tropical Andina (Conservaci).
Acosta-Galvis, A. R. (2017). Batracofauna de los bosques de niebla y estribaciones piemontanas en el municipio Yopal (Casanare: Colombia). Biota 18 (1), 281–314. doi:10.21068/c2017.v18n01a17
Álvarez-Grzybowska, E., Urbina-Cardona, N., Córdova-Tapia, F., and García, A. (2020). Amphibian Communities in Two Contrasting Ecosystems: Functional Diversity and Environmental Filters. Biodivers Conserv 29, 2457–2485. doi:10.1007/s10531-020-01984-w
Anderson, D. R. (2007). Model Based Inference in the Life Sciences: A Primer on Evidence. New York, NY: S. S. & B. Media (Ed.). Available at: https://books.google.es/books?hl=es&lr=&id=DlP_h4aMhiYC&oi=fnd&pg=PR3&dq=Model+based+inference+in+the+life+sciences:+a+primer+on+evidence&ots=9eREBRJzdS&sig=EyoDgP56KSPZwWsow64ztavwGwo#v=onepage&q=Model based inference in the life sciences%3A a primer on.
Anderson, M., and Braak, C. T. (2003). Permutation Tests for Multi-Factorial Analysis of Variance. J. Stat. Comput. Simulation 73 (2), 85–113. doi:10.1080/00949650215733
Anderson, M., Gorley, R. N., and Clarke, K. R. (2008). PERMANOVA+ for PRIMER. Guide to Software and Statistical Methods. Plymouth: PRIMER-E Ltd, 218.
Anderson, M. J., and Willis, T. J. (2003). Canonical Analysis of Principal Coordinates: a Useful Method of Constrained Ordination for Ecology. Ecology 84 (2), 511–525. doi:10.1890/0012-9658(2003)084[0511:caopca]2.0.co;2
Angarita-Sierra, T. (2014). Diagnosis del estado de conservación del ensamble de anfibios y reptiles presentes en los ecosistemas de sabanas inundables de la cuenca del río Pauto, Casanare, Colombia. Rev. Acad. Colomb. Cienc. Ex. Fis. Nat. 38 (146), 53–78. doi:10.18257/raccefyn.40
Angarita-Sierra, T. (2021). Biotic Assessment index Based on Anuran Species to Evaluate the Biotic Integrity of the Flooded Savannas Ecosystem from Pauto River basin (Casanare-Colombia). Revista Latinoamericana de Herpetologia 4 (1), 35–55.
Armenteras, D., Romero, M., and Galindo, G. (2005). Vegetation Fire in the Savannas of the. World Resource Rev. 17 (4), 531–543.
Azevedo-Ramos, C., Magnusson, W. E., and Bayliss, P. (1999). Predation as the Key Factor Structuring Tadpole Assemblages in a Savanna Area in Central Amazonia. Copeia 1999, 22–33. doi:10.2307/1447381
Becker, C. G., Fonseca, C. R., Haddad, C. F. B., Batista, R. F., and Prado, P. I. (2007). Habitat Split and the Global Decline of Amphibians. Science 318 (5857), 1775–1777. doi:10.1126/science.1149374
Becker, C. G., Fonseca, C. R., Haddad, C. F. B., and Prado, P. I. (2010). Habitat Split as a Cause of Local Population Declines of Amphibians with Aquatic Larvae. Conservation Biol. 24 (1), 287–294. doi:10.1111/j.1523-1739.2009.01324.x
Blanco-Torres, A., Bastidas-Molina, B., and Parra-Torres, F. (2017). Variación espacial y temporal de la herpetofauna en ecosistemas de sabanas inundables de la Orinoquía-Colombia. Caldasia 39 (2), 354–369. doi:10.15446/caldasia.v39n2.63489
Burton, E. C., Gray, M. J., Schmutzer, A. C., and Miller, D. L. (2009). Differential Responses of Postmetamorphic Amphibians to Cattle Grazing in Wetlands. J. Wildl. Manag. 73 (2), 269–277. doi:10.2193/2007-562
Caceres-Andrade, S. P., and Urbina-Cardona, J. N. (2009). Anuran Ensembles Inhabiting Productive Systems and Forests at the Piedemonte Llanero, Meta Department, Colombia. Caldasia 31 (1), 175–194. doi:10.1007/s11482-014-9349-8
Camacho- Reyes, J., and Camacho- Rozo, C. (2010). Aspectos sobre la historia natural de macroinvertebrados en esteros semipermanentes de la altillanura en el departamento de Casanare. Orinoquía 14 (2), 71–82. doi:10.22579/20112629.92
Camacho-Rozo, C. P., and Camacho-Reyes, J. A. (2010). Listado preliminar de larvas de anuros presentes en lagunas tropicales semipermanente y charcos temporales de sabana en época de altas lluvias, Yopal- Casanare. Orinoquia 14 (2), 83–91. doi:10.22579/20112629.93
Clarke, K. R., and Gorley, R. N. (2015). “Getting Started with PRIMER V7. PRIMER-E,” in PRIMER-E: Plymounth (Zealand: Massey University Albany Campus), 2–20. Available at: http://updates.primer-e.com/primer7/manuals/Getting_started_with_PRIMER_7.pdf.
Clarke, K. R., Somerfield, P. J., and Gorley, R. N. (2008). Testing of Null Hypotheses in Exploratory Community Analyses: Similarity Profiles and Biota-Environment Linkage. J. Exp. Mar. Biol. Ecol. 366 (1–2), 56–69. doi:10.1016/j.jembe.2008.07.009
Colwell, R. K., and Coddington, J. A. (1994). Estimating Terrestrial Biodiversity through Extrapolation. Phil. Trans. R. Soc. Lond. B 345 (1311), 101–118. doi:10.1098/rstb.1994.0091
Cortés-Gómez, A. M., Castro-Herrera, F., and Urbina-Cardona, J. N. (2013). Small Changes in Vegetation Structure Create Great Changes in Amphibian Ensembles in the Colombian Pacific Rainforest. Trop. Conservation Sci. 6 (6), 749–769. doi:10.1177/194008291300600604
Costa, A., Posillico, M., Basile, M., and Romano, A. (2016). Conservation of Herpetofauna as a Function of Forestry. Ital. J. Agron. 11 (S1), 38–41.
Crump, M. L. (2015). Anuran Reproductive Modes: Evolving Perspectives. J. Herpetology 49 (1), 1–16. doi:10.1670/14-097
Cruz-Piedrahita, C., Navas, C. A., and Crawford, A. J. (2018). Life on the Edge: A Comparative Study of Ecophysiological Adaptations of Frogs to Tropical Semiarid Environments. Physiol. Biochem. Zool. 91 (1), 740–756. doi:10.1086/695705
Cubides, P. J. I., and Cardona, J. N. U. (2011). Anthropogenic Disturbance and Edge Effects on Anuran Assemblages Inhabiting Cloud forest Fragments in Colombia. NatCon 9 (1), 39–46. doi:10.4322/natcon.2011.004
D'Amen, M., Rahbek, C., Zimmermann, N. E., and Guisan, A. (2017). Spatial Predictions at the Community Level: from Current Approaches to Future Frameworks. Biol. Rev. 92 (1), 169–187. doi:10.1111/brv.12222
del Castillo Domínguez, S. L., González, C. A. M., Fernández, E. B., Pelea, L. P., Cézilly, F., and Bosch, R. A. (2021). Predicting the Invasion of the Acoustic Niche: Potential Distribution and Call Transmission Efficiency of a Newly Introduced Frog in Cuba. Perspect. Ecol. Conservation 19, 90–97. doi:10.1016/j.pecon.2020.12.002
Denver, R. J., Mirhadi, N., and Phillips, M. (1998). Adaptive Plasticity in Amphibian Metamorphosis: Response of Scaphiopus Hammondii Tadpoles to Habitat Desiccation. Ecology 79 (6), 1859–1872. doi:10.2307/176694
Denver, R. J. (1997). Proximate Mechanisms of Phenotypic Plasticity in Amphibian Metamorphosis. Am. Zool 37, 172–184. doi:10.1093/icb/37.2.172
Downie, J. R., Disney, R. H. L., Collins, L., and Hancock, E. G. (1995). A New Species ofMegaselia(Diptera, Phoridae) Whose Larvae Prey upon the Eggs ofLeptodactylus fuscus(Anura, Leptodactylidae). J. Nat. Hist. 29 (4), 993–1003. doi:10.1080/00222939500770371
Downie, J. R., and Smith, J. (2003). Survival of Larval Leptodactylus fuscus (Anura: Leptodactylidae) Out of Water: Developmental Differences and Interspecific Comparisons. J. Herpetology 37 (1), 107–115. doi:10.1670/0022-1511(2003)037[0107:SOLLFA]2.0.CO;2
Downie, J. R., Walsh, P. T., and Langhorne, C. (2008). Asymmetric Larval Competition Between Two Species of Neotropical Foam-Nesting Frogs: Leptodactylus Fuscus and Engystomops Pustulosus. J. Nat. Hist. 42 (31-32), 2151–2159. doi:10.1080/00222930802140178
Eterovick, P. C., Bar, L. F. F., Souza, J. B., Castro, J. F. M., Leite, F. S. F., and Alford, R. A. (2015). Testing the Relationship between Human Occupancy in the Landscape and Tadpole Developmental Stress. PLoS ONE 10 (3), e0120172. doi:10.1371/journal.pone.0120172
Eterovick, P. C., and Barata, I. M. (2006). Distribution of Tadpoles within and Among Brazilian Streams: The Influence of Predators, Habitat Size and Heterogeneity. Herpetologica 62, 365–377. doi:10.1655/0018-0831(2006)62[365:DOTWAA]2.0.CO;2
Etter, A., Mcalpine, C., and Possingham, H. (2008). Historical Patterns and Drivers of Landscape Change in Colombia since 1500: A Regionalized Spatial Approach. Ann. Assoc. Am. Geogr. 98 (1), 2–23. doi:10.1080/00045600701733911
Figueroa, L. R., Acosta, N. R., and Nuñez, A. (2009). Efectos de la desecación progresiva en el desarrollo larval de Pleurodema borellii. Métodos En Ecología y Sistemática 4 (2), 1–7.
Fletcher, R. J., Didham, R. K., Banks-Leite, C., Barlow, J., Ewers, R. M., Rosindell, J., et al. (2018). Is Habitat Fragmentation Good for Biodiversity?. Biol. Conservation 226, 9–15. doi:10.1016/j.biocon.2018.07.022
Freda, J., and Dunson, W. A. (1985). Field and Laboratory Studies of Ion Balance and Growth Rates of Ranid Tadpoles Chronically Exposed to Low pH. Copeia 1985, 415–423. doi:10.2307/1444853
Gascon, C. (1991). Population- and Community-Level Analyses of Species Occurrences of Central Amazonian Rainforest Tadpoles. Ecology 72 (5), 1731–1746. doi:10.2307/1940972
Goodenough, A. E., Hart, A. G., and Stafford, R. (2012). Regression with Empirical Variable Selection: Description of a New Method and Application to Ecological Datasets. PLoS ONE 7 (3), e34338. doi:10.1371/journal.pone.0034338
Guayara-Barragán, M. G., and Bernal, M. H. (2012). Fecundidad y fertilidad en once especies de anuros Colombianos con diferentes modos reproductivos. Caldasia 34 (2), 483–496. doi:10.1670/14-097
Haddad, C. F. B., and Prado, C. P. A. (2005). Reproductive Modes in Frogs and Their Unexpected Diversity in the Atlantic Forest of Brazil. BioScience 55 (3), 207. doi:10.1641/0006-3568(2005)055[0207:rmifat]2.0.co;2
Haddad, C. F. B., and Sawaya, R. J. (2000). Reproductive Modes of Atlantic Forest Hylid Frogs: A General Overview and the Description of a New Mode. Biotropica 32, 862–871. doi:10.1646/0006-3606(2000)032[0862:RMOAFH]2.0.CO;2
Hernández-Ordóñez, O., Urbina-Cardona, N., and Martínez-Ramos, M. (2015). Recovery of Amphibian and Reptile Assemblages during Old-Field Succession of Tropical Rain Forests. Biotropica 47 (3), 377–388. doi:10.1111/btp.12207
Hero, J.-M., Gascon, C., and Magnusson, W. E. (1998). Direct and Indirect Effects of Predation on Tadpole Community Structure in the Amazon Rainforest. Austral Ecol. 23 (5), 474–482. doi:10.1111/j.1442-9993.1998.tb00755.x
Heyer, R., Acosta-Galvis, A., Mijares, A., Solís, F., Ibáñez, R., Hammerson, G., et al. (2010). Leptodactylus Fragilis. The IUCN Red List of Threatened Species. doi:10.2305/IUCN.UK.2004.RLTS.T57129A11588348.en
Heyer, W. R., Donnelly, M. A., Mcdiarmid, R. W., Hayek, L.-A., and Foster, M. (1994). Measuring and Monitoring Biological Diversity. Standard Methods for Amphibians. 1st ed. Smithsonian Institution Press.
Heyer, W. R., McDiarmid, R. W., and Weigmann, D. L. (1975). Tadpoles, Predation and Pond Habitats in the Tropics. Biotropica 7 (2), 100. doi:10.2307/2989753
Iop, S., Gomes dos Santos, T., Zanini Cechin, S., Velez-Martin, E. D., Pillar, V., and Inacio Prado, P. (2020). The Interplay Between Local and Landscape Scales on the Density of Pond-Dwelling Anurans in Subtropical Grasslands. Biotropica 52 (5), 913–926. doi:10.1111/btp.12794
Johnson, J. B., and Omland, K. S. (2004). Model Selection in Ecology and Evolution. Trends Ecol. Evol. 19 (2), 101–108. doi:10.1016/j.tree.2003.10.013
Kopp, K., Wachlevski, M., and Eterovick, P. C. (2006). Environmental Complexity Reduces Tadpole Predation by Water Bugs. Can. J. Zool. 84 (1), 136–140. doi:10.1139/z05-186
Lasso, C., Rial, A., Giuseppe, C., Machado-Allison, A., and Trujillo, F. (2014). XI Humedales de la Orinoquia (Colombia-Venezuela). Recursos Hidrobiológicos y Pesqueros Continentales de Colombia. Bogotá: Instituto de Investigación de Recursos Biológicos Alexander von Humboldt (IAvH).
Lasso, C. A., Usma, J. S., Trujillo, F., and Rial, A. (2010). Biodiversidad de la cuenca del Orinoco: bases científicas para la identificación de áreas prioritarias para la conservación y uso sostenible de la biodiversidad (Bogota: Instituto de Investigación de Recursos Biológicos Alexander von Humboldt, WWF Colombia, Fundación Omacha, Fundación La Salle e Instituto de Estudios de la Orinoquia (Universidad Nacional de Colombia)).
Legendre, P., and Andersson, M. J. (1999). Distance-based Redundancy Analysis: Testing Multispecies Responses in Multifactorial Ecological Experiments. Ecol. Monogr. 69, 1–24. doi:10.1890/0012-9615(1999)069[0001:DBRATM]2.0.CO;2
Leips, J., and Travis, J. (1994). Metamorphic Responses to Changing Food Levels in Two Species of Hylid Frogs. Ecology 75 (5), 1345–1356. doi:10.2307/1937459
Lucas, E. M., Brasileiro, C. A., Oyamaguchi, H. M., and Martins, M. (2008). The Reproductive Ecology ofLeptodactylus fuscus(Anura, Leptodactylidae): New Data from Natural Temporary Ponds in the Brazilian Cerrado and a Review throughout its Distribution. J. Nat. Hist. 42 (35–36), 2305–2320. doi:10.1080/00222930802254698
Lynch, J. D., and Suárez Mayorga, Á. M. (2011). Clave ilustrada de los renacuajos en las tierras bajas al oriente de los Andes, con énfasis en hylidae. Caldasia 33 (1), 235–270.
Lynch, J. D. (2006). The Tadpoles of Frogs and Toads Found in the Lowlands of Northern Colombia. Revista de La Academia Colombiana de Ciencias Exactas, Físicas y Naturales 30 (116), 443–457.
Magurran, A. E. (2004). Measuring Biological Diversity. 2nd ed. Oxford: U. K. Blackwell Science Ltd. Available at: http://www.wiley.com/WileyCDA/WileyTitle/productCd-0632056339.html.
Mcardle, B. H., and Anderson, M. J. (2001). Fitting Multivariate Models to Community Data: a Comment on Distance-Based Redundancy Analysis. Ecology 82 (1), 290–297. doi:10.1890/0012-9658(2001)082[0290:fmmtcd]2.0.co;2
McDiarmid, R. W., and Altig, R. (1999). Tadpoles. The Biology of Anuran Larvae (The Univer). Chicago.
Mcintyre, P. B., Baldwin, S., and Flecker, A. S. (2004). Effects of Behavioral and Morphological Plasticity on Risk of Predation in a Neotropical Tadpole. Oecologia 141, 130–138. doi:10.1007/s00442-004-1652-x
Mendoza Roldán, J. S., and Crawford, A. J. (2014). Amphibian Epithelial and Morphological Adaptations to Dry Habitats: A Preliminary Survey of Adaptive Trait Variation Among Colombian Dry forest Anurans. Universidad de lo Andes.
Nowakowski, A. J., Watling, J. I., Thompson, M. E., Brusch, G. A., Catenazzi, A., Whitfield, S. M., et al. (2018). Thermal Biology Mediates Responses of Amphibians and Reptiles to Habitat Modification. Ecol. Lett. 21 (3), 345–355. doi:10.1111/ele.12901
Nowakowski, A. J., Watling, J. I., Whitfield, S. M., Todd, B. D., Kurz, D. J., and Donnelly, M. A. (2017). Tropical Amphibians in Shifting thermal Landscapes under Land-Use and Climate Change. Conservation Biol. 31 (1), 96–105. doi:10.1111/cobi.12769
Ouchi-Melo, L. S., Gonçalves-Souza, T., Garey, M. V., and de Cerqueira, D. (2017). Tadpole Species Richness within Lentic and Lotic Microhabitats: An Interactive Influence of Environmental and Spatial Factors. Herpetological J. 27 (4), 339–345.
Ouchi-Melo, L. S., Meynard, C. N., Gonçalves-Souza, T., and de Cerqueira Rossa-Feres, D. (2018). Integrating Phylogenetic and Functional Biodiversity Facets to Guide Conservation: a Case Study Using Anurans in a Global Biodiversity Hotspot. Biodivers Conserv 27, 3247–3266. doi:10.1007/s10531-018-1600-4
Pedroza-Banda, R., Ospina-Sarria, J. J., Angarita-Sierra, T., Anganoy-Criollo, M., and Lynch, J. D. (2014). Estado del conocimiento de la fauna de anfibios y reptiles del departamento de Casanare, Colombia. Rev. Acad. Colomb. Cienc. Ex. Fis. Nat. 38 (146), 17. doi:10.18257/raccefyn.37
Pelinson, R. M., Garey, M. V., and Rossa-Feres, D. C. (2016). Effects of Grazing Management and Cattle on Aquatic Habitat Use by the Anuran pseudopaludicola Mystacalis in Agro-savannah Landscapes. PLoS ONE 11 (9), e0163094–14. doi:10.1371/journal.pone.0163094
Petranka, J. W., and Kennedy, C. A. (1999). Pond Tadpoles with Generalized Morphology: Is it Time to Reconsider Their Functional Roles in Aquatic Communities?. Oecologia 120 (4), 621–631. doi:10.1007/s004420050898
Pfeifer, M., Lefebvre, V., Peres, C. A., Banks-Leite, C., Wearn, O. R., Marsh, C. J., et al. (2017). Creation of forest Edges Has a Global Impact on forest Vertebrates. Nature 551 (7679), 187–191. doi:10.1038/nature24457.Creation
Pineda, E., and Halffter, G. (2004). Species Diversity and Habitat Fragmentation: Frogs in a Tropical Montane Landscape in Mexico. Biol. Conservation 117 (5), 499–508. doi:10.1016/j.biocon.2003.08.009
Rangel - Ch., J. O. (2015). La biodiversidad de Colombia: significado y distribución regional. Rev. Acad. Colomb. Cienc. Ex. Fis. Nat. 39 (51), 176. doi:10.18257/raccefyn.136
Reynolds, R., Caramaschi, U., Mijares, A., Acosta-Galvis, A., Heyer, R., Lavilla, E., et al. (2004). Leptodactylus fuscus. The IUCN Red List of Threatened Species 2004: e.T57129A11588348. doi:10.2305/IUCN.UK.2004.RLTS.T57129A11588348.en
Rodriguéz, M., Rudas, G., Andrade, G., Uribe, E., Cartro, L., Durán, A., et al. (2011). La mejor orinoquia que podemos construir. elementos para la sostenibilidad ambiental del desarrollo, 144.
Sanuy, D., Oromí, N., and Galofré, A. (2008). Effects of Temperature on Embryonic and Larval Development and Growth in the Natterjack Toad (Bufo calamita) in a Semi-arid Zone. Anim. Biodiversity Conservation 31 (1), 41–46. Available at: http://hdl.handle.net/10459.1/63141.
Schalk, C. M., Montaña, C. G., Winemiller, K. O., and Fitzgerald, L. A. (2017). Trophic Plasticity, Environmental Gradients and Food‐web Structure of Tropical Pond Communities. Freshw. Biol. 62 (3), 519–529. doi:10.1111/fwb.12882
Schneider-Maunoury, L., Lefebvre, V., Ewers, R. M., Medina-Rangel, G. F., Peres, C. A., Somarriba, E., et al. (2016). Abundance Signals of Amphibians and Reptiles Indicate strong Edge Effects in Neotropical Fragmented forest Landscapes. Biol. Conservation 200, 207–215. doi:10.1016/j.biocon.2016.06.011
Schriever, T. A., and Williams, D. D. (2013). Influence of Pond Hydroperiod, Size, and Community Richness on Food-Chain Length. Freshw. Sci. 32 (3), 964–975. doi:10.1899/13-008.1
Somerfield, P. J., and Clarke, K. R. (2013). Inverse Analysis in Non-parametric Multivariate Analyses: Distinguishing Groups of Associated Species Which Covary Coherently across Samples. J. Exp. Mar. Biol. Ecol. 449, 261–273. doi:10.1016/j.jembe.2013.10.002
Souza, V. M. d., Souza, M. B. d., and Morato, E. F. (2008). Efeitos da sucessão florestal sobre a anurofauna (Amphibia: Anura) da Reserva Catuaba e seu entorno, Acre, Amazônia sul-ocidental. Rev. Bras. Zool. 25 (1), 49–57. doi:10.1590/S0101-81752008000100008
Suazo Oruño, I. (2009). Efectos de la conversión del bosque tropical caducifolio a mosaicos agrícolas sobre ensamblajes herpetofaunisticos. Mexico City: Universidad Nacional Autónoma de México.
Suazo-Ortuño, I., Benítez-Malvido, J., Marroquín-Páramo, J., Soto, Y., Siliceo, H., and Alvarado-Díaz, J. (2018). Resilience and Vulnerability of Herpetofaunal Functional Groups to Natural and Human Disturbances in a Tropical Dry forest. For. Ecol. Manag. 426, 145–157. doi:10.1016/j.foreco.2017.09.041
Tárano, Z. (2010). Advertisement Calls and Calling Habits of Frogs from a Flooded Savanna of Venezuela. South Am. J. Herpetology 5 (3), 221–240. doi:10.2994/057.005.0308
Teixeira-Borges, V. N., and Duarte Rocha, C. F. (2013). Effects of Behavioral and Morphological Plasticity on Risk of Predation in a Neotropical Tadpole. Oecologia Australis 17 (2), 217–228. doi:10.4257/oeco.2013.1702.04TROP
Thabah, C., Devi, L., Hooroo, R., and Dey, S. (2018). Morphological Alterations in the External Gills of Some Tadpoles in Response to pH. J. Morphol. Sci. 35 (2), 142–152. doi:10.1055/s-0038-1669476
Ugland, K. I., Gray, J. S., and Ellingsen, K. E. (2003). The Species-Accumulation Curve and Estimation of Species Richness. J. Anim. Ecol. 72 (5), 888–897. doi:10.1046/j.1365-2656.2003.00748.x
Urbina-Cardona, J. N., Navas, C. A., González, I., Gómez-Martínez, M. J., Llano-Mejía, J., Medina-Rangel, G. F., et al. (2014). “Determinantes de la distribución de los anfibios en el Bosque Seco Tropical de Colombia: herramientas para su conservación,” in El bosque seco tropical en Colombia. Editors C. Pizano, and H. García (Bogotá: Instituto), 163–189.
Urbina-Cardona, J. N., and Reynoso, V. H. (2009). Uso del microhábitat por hembras grávidas de la rana de hojarasca Craugastor loki (shannon y werler, 1955) en la Selva Alta Perennifolia de Los Tuxtlas, Veracruz - México. Revista Mexicana de Biodiversidad 80 (2), 571–573. doi:10.22201/ib.20078706e.2009.002.630
Venturelli, D. P., and Klein, W. (2019). Effect of Hydric Stress on Locomotion and Morphology of Tadpoles from Temporary Ponds. J. Exp. Zool. 331 (3), 175–184. doi:10.1002/jez.2251
Watling, J. I., and Braga, L. (2015). Desiccation Resistance Explains Amphibian Distributions in a Fragmented Tropical forest Landscape. Landscape Ecol. 30 (8), 1449–1459. doi:10.1007/s10980-015-0198-0
Wells, K. D. (2007). The Ecology and Behavior of Amphibians. Chicago: The University of Chicago Press. doi:10.7208/chicago/9780226893334.001.0001
Welsh, H. H., and Ollivier, L. M. (1998). Stream Amphibians as Indicators of Ecosystem Stress: A Case Study from California’s Redwoods. Ecol. Appl. 8, 1118–1132. doi:10.1890/1051-0761(1998)008[1118:SAAIOE]2.0.CO;2
Whittaker, R. H. (1952). A Study of Summer Foliage Insect Communities in the Great Smoky Mountains. Ecol. Monogr. 22 (1), 1–44. doi:10.2307/1948527
Keywords: community ecology, habitat gradients, beta diversity (β), anura amphibian, habitat split
Citation: Camacho-Rozo CP and Urbina-Cardona N (2021) Tadpoles Inhabiting Natural and Anthropogenic Temporary Water Bodies: Which Are the Environmental Factors that Affect the Diversity of the Assemblages?. Front. Environ. Sci. 9:667448. doi: 10.3389/fenvs.2021.667448
Received: 13 February 2021; Accepted: 18 August 2021;
Published: 10 September 2021.
Edited by:
Orsolya Valkó, Hungarian Academy of Science, HungaryReviewed by:
Andres Garcia, National Autonomous University of Mexico, MexicoLu Zhang, Sun Yat-Sen University, China
Copyright © 2021 Camacho-Rozo and Urbina-Cardona. This is an open-access article distributed under the terms of the Creative Commons Attribution License (CC BY). The use, distribution or reproduction in other forums is permitted, provided the original author(s) and the copyright owner(s) are credited and that the original publication in this journal is cited, in accordance with accepted academic practice. No use, distribution or reproduction is permitted which does not comply with these terms.
*Correspondence: Claudia Patricia Camacho-Rozo, Y2xhdWRpYXAuY2FtYWNob3JAamF2ZXJpYW5hLmVkdS5jbw==