- 1Department of Oceanography and Coastal Sciences, Louisiana State University, Baton Rouge, LA, United States
- 2Department of Anthropology, University of North Carolina-Greensboro, Greensboro, NC, United States
- 3Department of Biology, University of Kentucky, Lexington, KY, United States
The world is urbanizing most rapidly in tropical to sub-temperate areas and in coastal zones. Climate change along with other global change forcings will diminish the opportunities for sustainability of cities, especially in coastal areas in low-income countries. Climate forcings include global temperature and heatwave increases that are expanding the equatorial tropical belt, sea-level rise, an increase in the frequency of the most intense tropical cyclones, both increases and decreases in freshwater inputs to coastal zones, and increasingly severe extreme precipitation events, droughts, freshwater shortages, heat waves, and wildfires. Current climate impacts are already strongly influencing natural and human systems. Because of proximity to several key warming variables such as sea-level rise and increasing frequency and intensity of heatwaves, coastal cities are a leading indicator of what may occur worldwide. Climate change alone will diminish the sustainability and resilience of coastal cities, especially in the tropical-subtropical belt, but combined with other global changes, this suite of forcings represents an existential threat, especially for coastal cities. Urbanization has coincided with orders of magnitude increases in per capita GDP, energy use and greenhouse gas emissions, which in turn has led to unprecedented demand for natural resources and degradation of natural systems and more expensive infrastructure to sustain the flows of these resources. Most resources to fuel cities are extracted from ex-urban areas far away from their point of final use. The urban transition over the last 200 years is a hallmark of the Anthropocene coinciding with large surges in use of energy, principally fossil fuels, population, consumption and economic growth, and environmental impacts such as natural system degradation and climate change. Fossil energy enabled and underwrote Anthropocene origins and fueled the dramatic expansion of modern urban systems. It will be difficult for renewable energy and other non-fossil energy sources to ramp up fast enough to fuel further urban growth and maintenance and reverse climate change all the while minimizing further environmental degradation. Given these trajectories, the future sustainability of cities and urbanization trends, especially in threatened areas like coastal zones in low-income countries in the tropical to sub-tropical belt, will likely diminish. Adaptation to climate change may be limited and challenging to implement, especially for low-income countries.
Introduction
For thousands of years, human societies have lived relatively sustainably in coastal areas. The beginnings of civilizations as characterized by monumental architecture, large public works projects, social stratification and writing was intimately tied to many of the first urban developments. Many of the first cities worldwide occurred in coastal areas and soil-rich lower river valleys where high natural resource energies subsidized the flowering of culture (Day et al., 2007; Day et al., 2012; Marquet et al., 2012; Gunn et al., 2019). Turning to the modern era, urbanization was a hallmark of the 20th century with a majority of the world’s population living in cities by 2010. The most urbanized countries have greater per capita GDP, energy use, and greenhouse gas emissions than predominantly rural countries (Burger et al., 2019). Coastal cities continue to be centers ever more so of economic activity, technological development, and culture. For example, Rappaport and Sachs (2002) reported that economic activities in the United States are overwhelmingly concentrated in coastal cities, which contribute very significantly to the nation’s productivity and quality of life.
However, the 20th century was also a time of dramatic change in the biogeosphere and society. There was dramatic exponential growth in many aspects of the coupled natural-human systems, currently being characterized as the Anthropocene, including population, carbon dioxide in the atmosphere, the size of the human economy, energy use, natural resource extraction and utilization, and information generation (Brown et al., 2014; Steffen et al., 2015; Burger et al., 2019). Environmental degradation and species extinctions also rose dramatically (Steffen et al., 2018; Ceballos et al., 2020). Population rose by more than a factor of seven from 1800 to 2010, 1 to 7.7 billion, and total energy consumption by much more (Schramski et al., 2015; Syvitski et al., 2020). During this same period, the utility of cities changed as the number of people living in cities of a million or more rose by a factor of about 500. Around 2010, for the first time in human history, more than half of the world’s population lived in cities—the urban transition. During the 19th and 20th centuries, the largest cities shifted from richer countries in higher latitudes (e.g., New York and London) to subtemperate and tropical areas and most urban growth is now taking place in developing countries and coastal areas. The combined impact of human activities has become so large that the earth moved into the new geological epoch, the Anthropocene (Steffen et al., 2018). Syvitski et al. (2020) report that human impacts had reached such high levels by the mid-20th century that the Earth System departed from its 10,000 years Holocene state to enter the Anthropocene.
Any consideration of the future of coastal cities must take into account the issues raised above. Coasts are at the forefront of global climate change impacts being led by sea-level rise, intense precipitation events, changes in freshwater input to the ocean, increased global warming and heat waves, poleward expansion of the tropical biological zone, increased intensity of tropical cyclones, and more extreme droughts and dramatic increases in wildfires. Each is discussed in more detail below. To these clearly global issues must be added local human impacts that are making coastal ecosystems and coastal cities less resilient and less sustainable. They include dams on rivers that reduce sediment input to coastal zones, massive water withdrawals from many rivers (e.g., the Tigris-Euphrates, Colorado, Nile and Indus), impoundment and reclamation of coastal areas, increased subsidence, separation of rivers from coastal systems by levees, and widespread contamination (Syvitski et al., 2009; Giosan et al., 2014; Tessler et al., 2015; Day et al., 2016; Day et al., 2019; Day et al., 2020).
In this article we consider the future of urban areas focusing on coastal cities, in the cumulative context of the impacts of the global change forcings outlined above. In doing so we address several fundamental 21st century questions. Urban growth over the last two centuries was sustained by abundant cheap energy, overwhelmingly fossil fuels (Burger et al., 2019). At the beginning of the industrial revolution, most large cities were in the temperate zone. However, large cities have increasingly developed in sub-temperate and tropical areas. The growth of cities led to a dramatic demand for goods and service provided by the biophysical base of the earth system (Brown et al., 2014; Steffen et al., 2015; Burger et al., 2019). This has brought about a dramatic degradation of natural systems on which humans depend. Along with dramatic increases in per capita energy use and GDP growth have come bourgeoning greenhouse gas emissions by two orders of magnitude (100-fold) or more as countries urbanized (Burger et al., 2019). A central question, then, is whether climate change in combination with other global trends are so profound that the sustainability of many cities, and especially coastal cities, is questionable. Will the trajectory of the urban transition continue through this century and into the next or will it stall or even reverse?
By addressing these questions, our review responds to the focus of this special issue Coastal Cities in a Changing Climate. We turn first to urbanization in the coastal zone, the growth of cities, and the urban transition. Because the focus of the special issue is on climate and coastal cities, we develop a detailed section on global climate change drivers including global warming, tropical cyclones, wildfires (and why this is important for some coastal cities), drought risk and water stress, and extreme precipitation events. We then integrate climate forcings with other megatrends in the context of the Great Acceleration, urbanization and ongoing Anthropocene evolution, including resource scarcity, challenges to the fossil energy-renewable energy transition, urban areas and disease, and food security.
This is not a detailed review of all available literature. We regard coastal cities as being leading indicators of the impacts of global change on urban areas. They are not only useful from the point of view of current trends in coastal cities evolution but also serve to show how long term-climate changes will impact all cities. As such, our selection criteria are focused on variables that most evidently impinge on coastal cities such as sea level and subsidence, increasingly heavy precipitation events resulting from tropical storms, freshwater stress and drought/wildfire dangers. On the social side, we pursue the thermodynamics of international commerce since coastal cities have been at the core of transportation and human population growth for 3,000 years, a tendency certainly not diminished by the current trend towards transportation on the seas to supply megacities. To obtain these measures, we select from key synthesis reviews such as the IPCC, UN population studies, the International Energy Agency and the United States NOAA that encompass key global and social change variable sets that could impact coastal city sustainability.
Urbanization in the Coastal Zone
We provide here more detailed information about growth in population and consumption, the urban transition, and the growth of coastal cities. In 1800, there were only two cities in the world with a million inhabitants. By 2018, there were about 550 cities of a million or more and by 2030 it is projected that there will be over 700 such cities. One in five people globally lives in cities with more than a million inhabitants. In 2010, there were 33 cities of 10 million inhabitants, the megacities, and by 2030 it is projected that that there will be 43. Only one of the top ten megacities in 2030 will be in a developed country (Tokyo).
Much of the growth of urban areas is happening in the coastal zone, especially between 30° north and south, which is home to more than 60% of the 33 largest megacities worldwide (see Figure 1, https://digitallibrary.un.org/record/3799524). Figure 1 shows coastal cities that will be increasingly impacted by sea level rise, especially after the mid 21st century. Oppenheimer et al. (2019) and IPCC (2019) reported projections of sea-level rise from 1986–2005 to 2081–2100 (values in parentheses are in meters, ranges are in brackets) (0.43 [0.29–0.59] for RPC2.6, 0.55 [0.39–0.72] for RPC4.5, and 0.84 [0.61–1.10] for RCP 8.5). Note that most of the cities in Figure 1 are in sub-temperate to tropical areas in less developed countries. In addition to sea-level rise, these cities will face the above variety of other climate forcings, which we will now review.
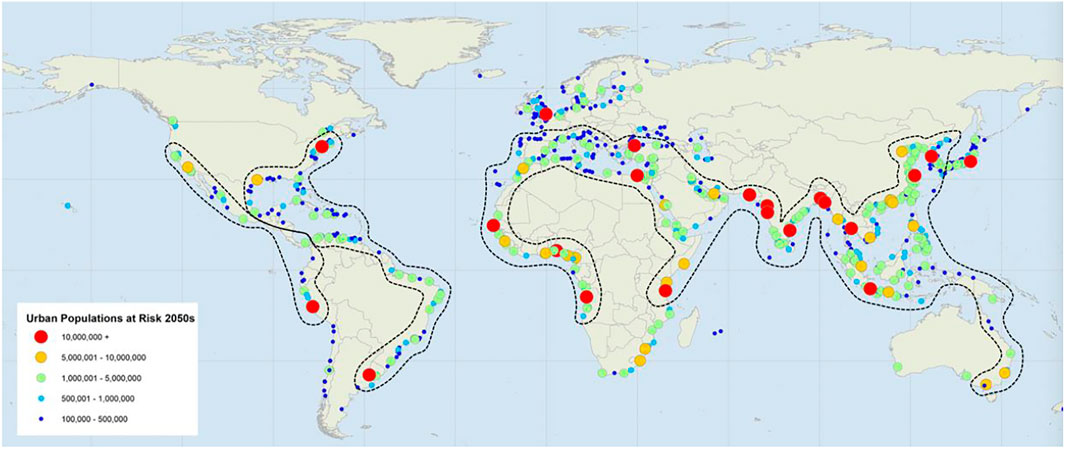
FIGURE 1. Colored dots show coastal cities that will be significantly impacted by sea-level rise. Note that many of the largest cities are on lower income tropical to sub-temperate coasts (C40.org, 2020). Coastal zones enclosed in the highlighted area will experience multiple climate stresses that will interact with other mega-trends that will lead to diminished resilience and sustainability (Modified from C40 2020. Reprinted by permission).
Global Climate Change Drivers
As mentioned above, climate change impacts are affecting the biogeosphere and society globally and coastal areas are at the forefront of the climate change experience of increasing temperature, sea-level rise, tropical cyclones, drought and water stress, wildfires, and extreme precipitation events (Goldenberg et al., 2001; Emanuel, 2005; Webster et al., 2005; Hoyos et al., 2006; FitzGerald et al., 2008; Pfeffer et al., 2008; Vermeer and Rahmstorf, 2009; Kaufmann et al., 2011; Min et al., 2011; Pall et al., 2011; IPCC, 2013; Horton et al., 2014; Mei et al., 2015; DeConto and Pollard, 2016; Koop et al. 2016). Added to global warming will be increasing energy costs leading to higher economic costs for energy-intensive activities to address climate threats (Tessler et al., 2015; Wiegman et al., 2017 as addressed below).
Temperature. Extreme heat is affecting increasingly larger areas of the globe. For example, Stott et al. (2004) reported that human activity was largely responsible for the European heat wave of 2003 (Figure 2). Xu et al. (2020) found that for the last six millennia of the Holocene, human populations occupied a relatively narrow part of the global climate envelope and production of crops and livestock was largely constrained to the same temperature range. The position of this climate envelope is projected to shift more in the next half century than it did in the past 6,000 years (Figure 3). Xu et al. conclude that a third of the human population will experience a mean annual temperature greater than 29°C by 2070, compared to 11–15°C for the past six millennia. The most dramatic reduction of temperature suitability is projected to occur in the tropics, subtropics and sub-temperate zone, especially along tropical coasts. Mora et al. (2017) also showed that projected climate change will lead to temperatures that exceed human thermoregulatory capacity and that 30% of the world’s population is already currently exposed to lethal temperature-humidity conditions (Figure 4). This is exacerbated by urban “heat-island” affects in humid coastal cities. By 2100, they projected that this percentage would increase from approximately 48–74% depending on future emissions. They further concluded that mortality from high heat is almost inevitable and will be much worse without reductions in greenhouse gas emissions. The use of non-metabolic energy to mitigate high temperatures through air-conditioning is less accessible in the lower-income tropics than in high-income countries (e.g., Hill et al., 2013).
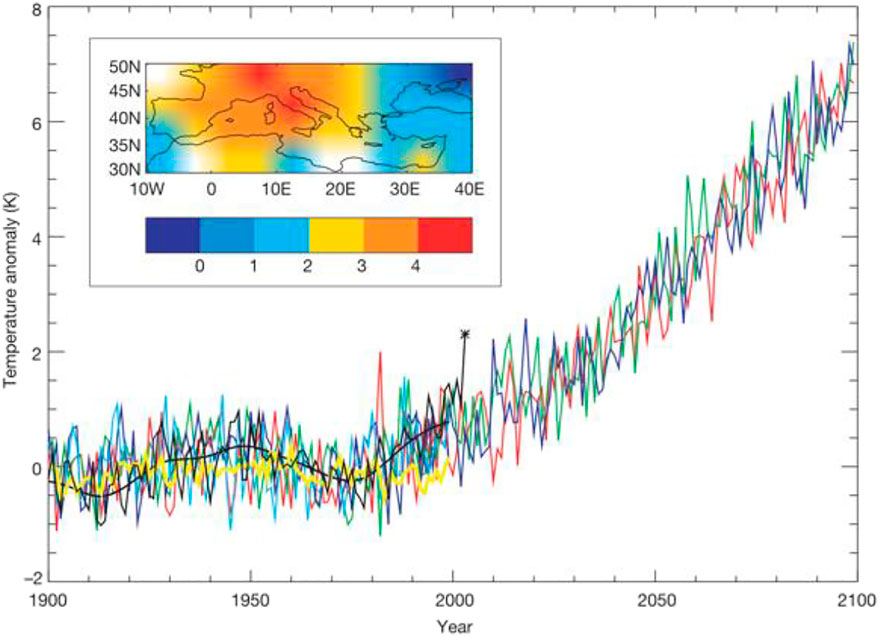
FIGURE 2. June–August temperature anomalies relative to the 1961–1900 mean for Europe (inset). Black line is observed temperatures and heavy black line is a low pass filtered temperature. Colors are future modeled temperatures; heavy yellow line is natural forcing. Observed temperature for 2003 is shown as a star (from Stott et al., 2004. Reprinted by permission).
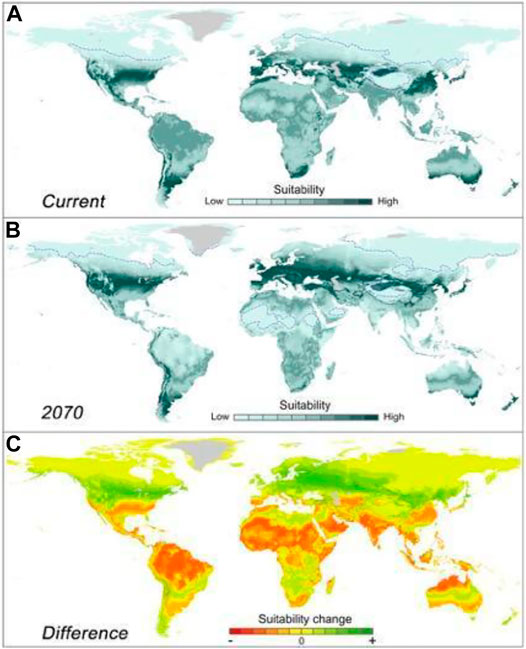
FIGURE 3. Projected geographical shift in the human temperature niche in terms of the suitability for human habitation. Large areas of the earth will have significantly lower suitability in terms of temperature, especially in the tropics and subtropics. Top panel. Current position of the human temperature niche. Mid panel. Change projected for 2070 with RCP8.5. Bottom panel. Bottom panel. Differences between the two maps. (Xu et al., 2020. Reprinted by permission).
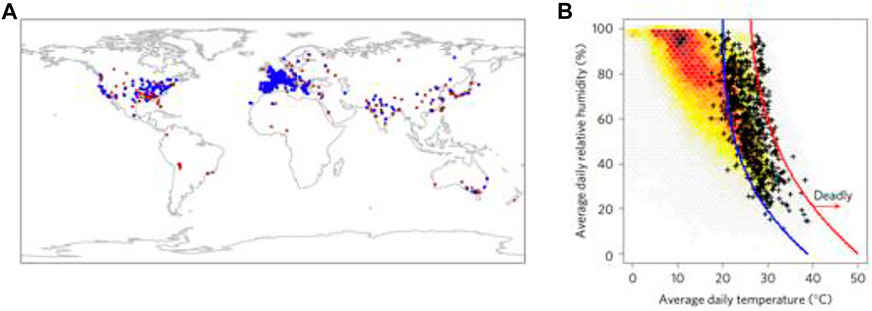
FIGURE 4. (A) Locations of recent recorded lethal heat events. (B) Mean daily surface air temperature and relative humidity during lethal heat events (black crosses) compared to non-lethal events (red to yellow). Blue line separates lethal and non-lethal events and red line is 95% probability threshold for lethal events (from Mora et al., 2017. Reprinted by permission).
Figure 5 shows that lethal heat waves are becoming more common globally. Based on tree ring reconstructions of heatwaves and soil moisture over the past 260 years, Zhang et al. (2020) documented an abrupt shift to a hotter and drier climate over inner East Asia that they described as crossing a tipping point. Over the past 2 decades, the East Asian climate system trend exceeds the natural variability range for this region that is potentially irreversible. If realized, it will impact the large populations in coastal areas of southeast Asia. Increasing heat waves will also pose challenges for food security, especially in low-income countries in the tropical belt (see below). Extreme heat will also impact wild and domestic food production and storage (Hammond et al., 2015), especially in coastal zones where livelihoods are often dependent on local and regional harvest and trade. This is discussed in more detail below.
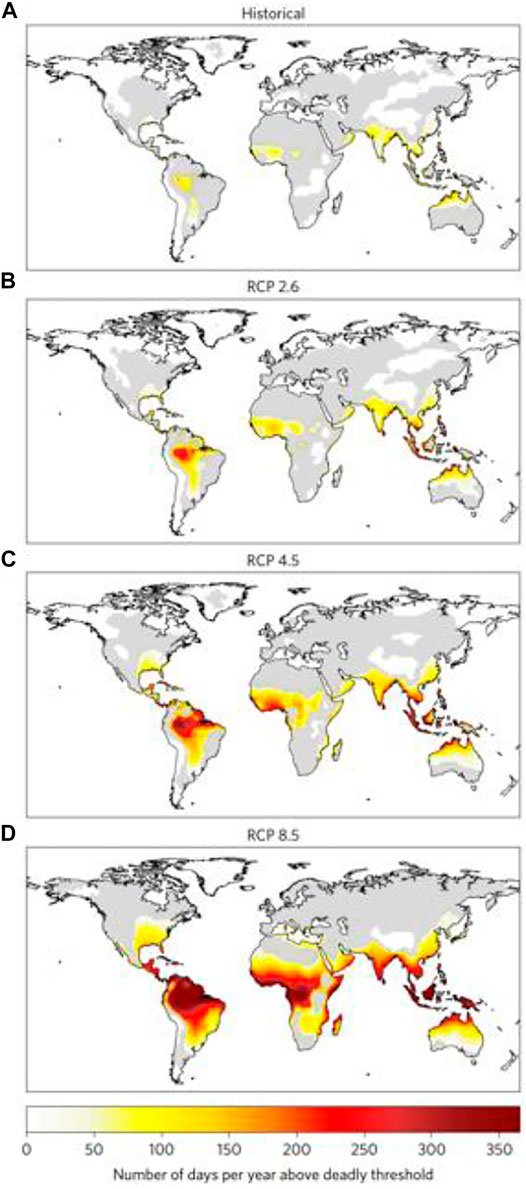
FIGURE 5. Global patters of the number of days per year above deadly threshold (to the left of the blue Line in Figure 4B) for 1995–2005 average and for different emission scenarios. From Mora et al. (2017). Reprinted by permission.
The oceans are warming also. Oliver et al. (2021) reviewed prevalence of marine heatwaves that can be detected more accurately and easily by satellite observations. Global climate change has led to an increase in the frequency and duration of marine heatwaves (Figure 6). The impact of these heat waves on coastal systems is addressed later in the paper. The increasing frequency of marine heatwaves raises the question of ocean heat absorption capacity. Loeb et al. (2021) reported that the amount of heat stored by the earth system doubled from 2005 to 2021. While the exact global temperature at which the capacity of the oceans to absorb excess heat is not known, this great rate at which it is being used up suggests a rapidly approaching tipping point that will substantially and irreversibly change the locations of the human climate envelope.
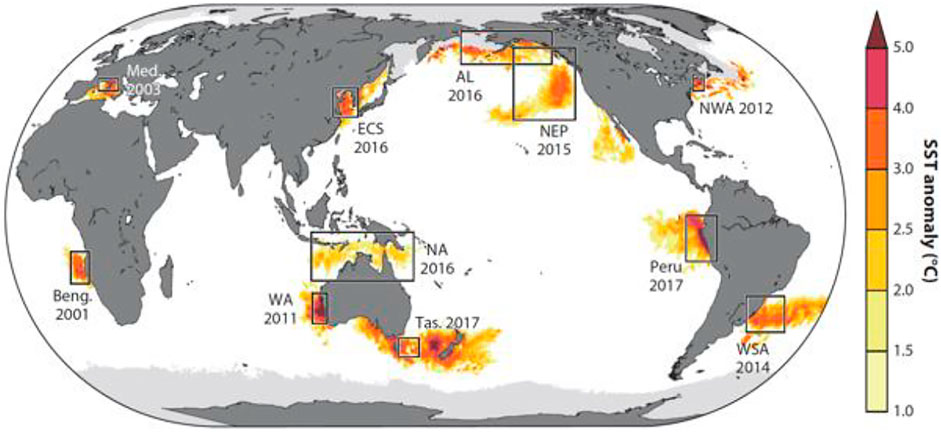
FIGURE 6. Example of Marine Heatwaves (MWH) since 2001 as indicated by sea surface temperature anomalies. These occur from high latitude to tropical waters. From Oliver et al. (2021). Reprinted by permission.
Sea-Level Rise. Eustatic sea-level rise is projected to increase by up to 1 m by 2100 and maybe more. It will increasingly impact coastal areas in this century. Especially vulnerable are coastal regions with large areas of near-sea level land (Figures 1, 7). Most important will be large deltas that are concentrated in the tropics and subtropics (Syvitski et al., 2009; Giosan et al., 2014; Day et al., 2016; Day et al., 2019). A number of megacities are located bordering on or in deltas (Yangon, Kolkata, Dhaka, Ho Chi Minh City, Shanghai, Cairo, Karachi, Lagos). Changes in the drainage basins of large deltas (freshwater withdrawal, sediment retention behind dams) and local changes (reclamation, hydrologic alteration) are decreasing the resilience of these areas (Giosan et al., 2014; Tessler et al., 2015; Day et al., 2016; Day et al., 2019). High subsidence rates are frequent due to geologic subsidence in deltas (due to compaction and consolidation of sediments) that lead to high rates of relative sea-level rise (subsidence plus eustatic sea-level rise). Subsidence is also caused by subsurface fluid withdrawal (Day et al., 2019). Sea-level rise can interact with ocean circulation to exacerbate coastal flooding and saltwater intrusion (Wahl et al., 2015; Lee and MingZhang. 2017; Pietrafesa et al., 2019).
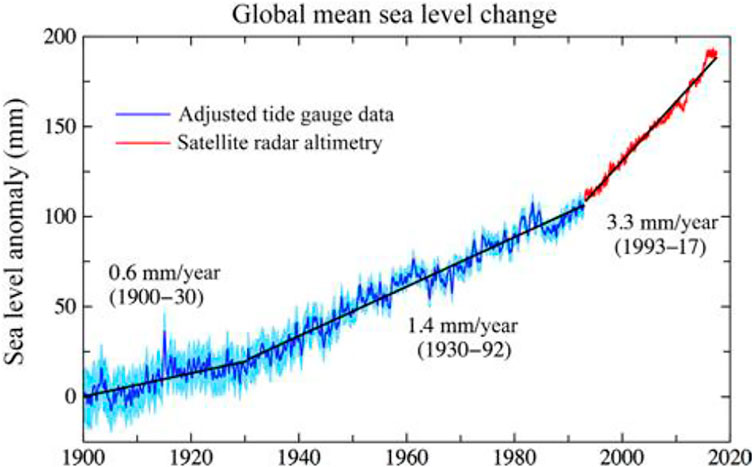
FIGURE 7. Rates of global sea-level rise from 1900 to 2015 (Hansen et al., 2015. Reprinted by permission).
Tropical Cyclones. Increasing warming of ocean surface waters has amplified the impacts of tropical cyclones. Emanuel (2005) reported that a 1°C increase in sea surface temperature in the tropics over the past half century generated approximately 80% increase in hurricane intensity or power. It has been reported that the frequency of the most intense tropical storms is increasing (Webster et al., 2005; Hoyos et al., 2006; Elsner et al., 2008; Bender et al., 2010). Mei et al. (2015) predicted climate change will increase already high average typhoon intensity in the Pacific area by 14% by 2100. Tropical cyclones are also intensifying more rapidly (Bhatia et al., 2019) as are large surge events (Grinsted et al., 2012). Li and Charkraborty (2020) analyzed the intensity of North Atlantic landfalling hurricanes over the last 50 years and found that the rate of decline in hurricane strength over land has decreased in inverse proportion to the rise in sea surface temperature causing greater flooding concerns in coastal and inland cities. Tropical cyclones impact the western basins of the north Atlantic and North Pacific, the northern part of the Indian Ocean, and parts of east Africa and Australia (Figure 8). Countries bordering the western Pacific and Indian Ocean are among the most populous and highest human population densities in the world (Burger et al., 2017; Burger and Fristoe 2018; Burger et al., 2019). This area contains many coastal megacities (Figure 1). In summary, it is projected that the frequency of the strongest tropical cyclones will increase, and these storms will be larger, intensify faster, have more precipitation, intensify faster, move slower, and decay more slowly over land.
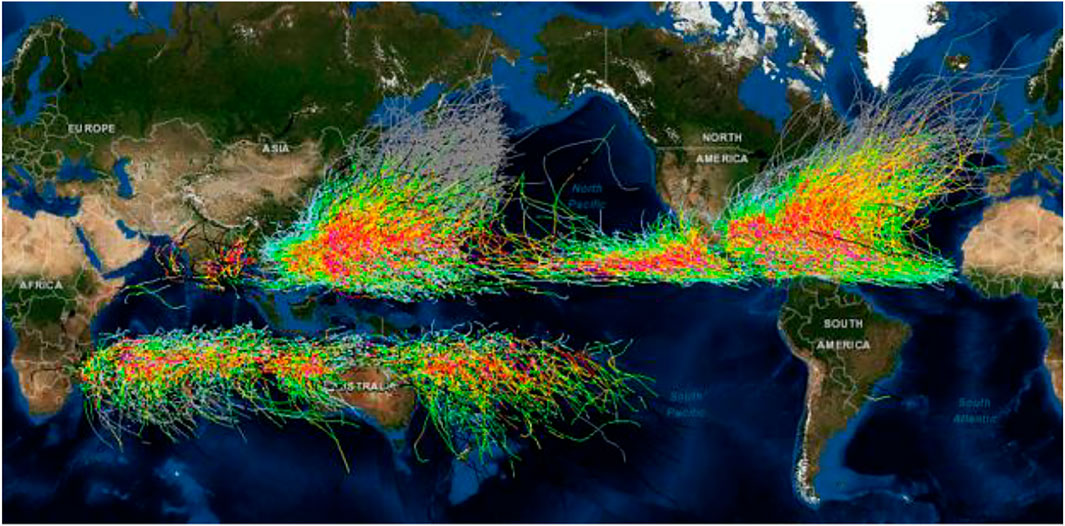
FIGURE 8. Global tropical storm tracks 1848–2013. Red and orange, Saffir-Simpson category 4 and 5, yellow and green category 1-3, blue tropical storms, and depressions. Source NOAA Digital GeoZone.
Wildfires. Wildfires are becoming more frequent, covering larger areas, and wildfire seasons are becoming longer. Robinne et al. (2020) analyzed global wildfire-water risks to human and natural systems using a global index to assess wildfire risk. Large, intense wildfires have occurred on the west coast and southwestern United States, Greece, Indonesia, Australia, central Chile, Amazona, and other areas in recent years. Wildfire risks can be particularly acute in water-insecure countries. Wildfire-induced risk is higher in low-income countries because of the lack of socio-economic capacity to respond to disasters (e.g., Dyer, 2009; Tessler et al., 2015). The wildfire-water index is high along the northern and southeastern coasts of Australia, the Philippines, the Indo-Pacific area, coastal northwestern Pacific, the Asian and east African coasts of the Indian Ocean, Mideast and Mediterranean coasts, western Europe from Spain to Scandinavia, tropical west Africa, the Atlantic, Gulf and Pacific coasts of North America, and Central America, and much of South America (Figure 9).
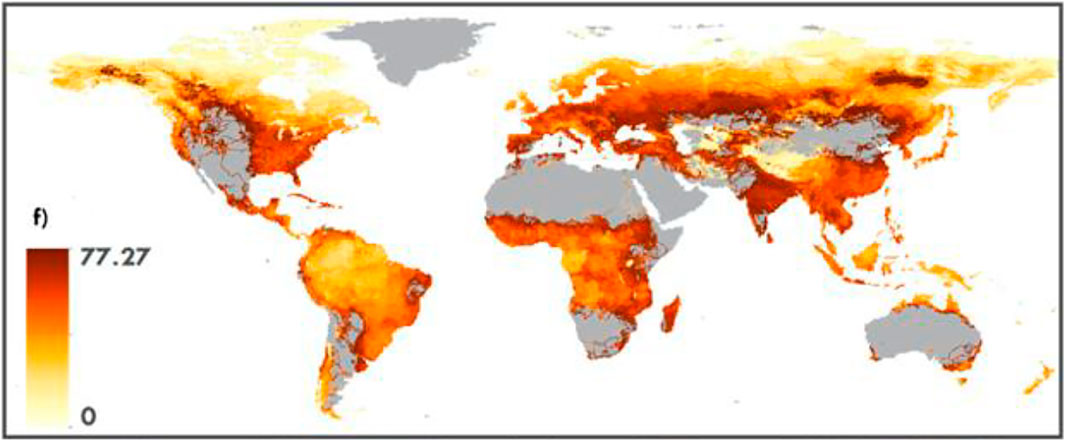
FIGURE 9. Wildlife-Water Risk index globally. Red areas have higher risks. Areas in gray were excluded from the analysis because of low precipitation and/or runoff–see Figure 10 for comparison. (Robinne et al., 2020, Reprinted by permission).
Although not a coast-specific climate forcing, wildfires can strongly impact coastal cities. Fires can directly threaten coastal cities such as Los Angeles and Sydney and they create extremely poor air quality. Wildfires are more common in arid areas and combine with drought to reduce the sustainability of coastal cities. An example is the coastal region of California. The Los Angeles-San Diego area is the second largest ex-urban area in the United States. This region obtains water from northern California, the Colorado River and Owens Valley, Nevada. Projections are for increasingly arid conditions in the Southwest, lower river discharge, and greater water demand (Ingram and Malamud-Roam, 2013; Milly and Dunne, 2020; Williams et al., 2020). Thus wildfires, drought, and water scarcity can combine with coastal climate forcings such as tropical cyclones and sea level rise to threaten the sustainability of coastal cities. The impact of these forcings may in part contribute to the current population decline in California.
Drought Risk and Water Stress. Climate change and human water demand is leading to more intense droughts (United Nations, Department of Economic and Social Affairs, Population Division., 2018). Global drought risk and water stress are related. These are especially intense and widespread around the Mediterranean, temperate forests, along the northern shore of the Indian Ocean, Middle East, and southeast Asia, and the American Southwest and much of southern Mexico and Mesoamerica, southeastern South America, and tropical west Africa (Figure 10).
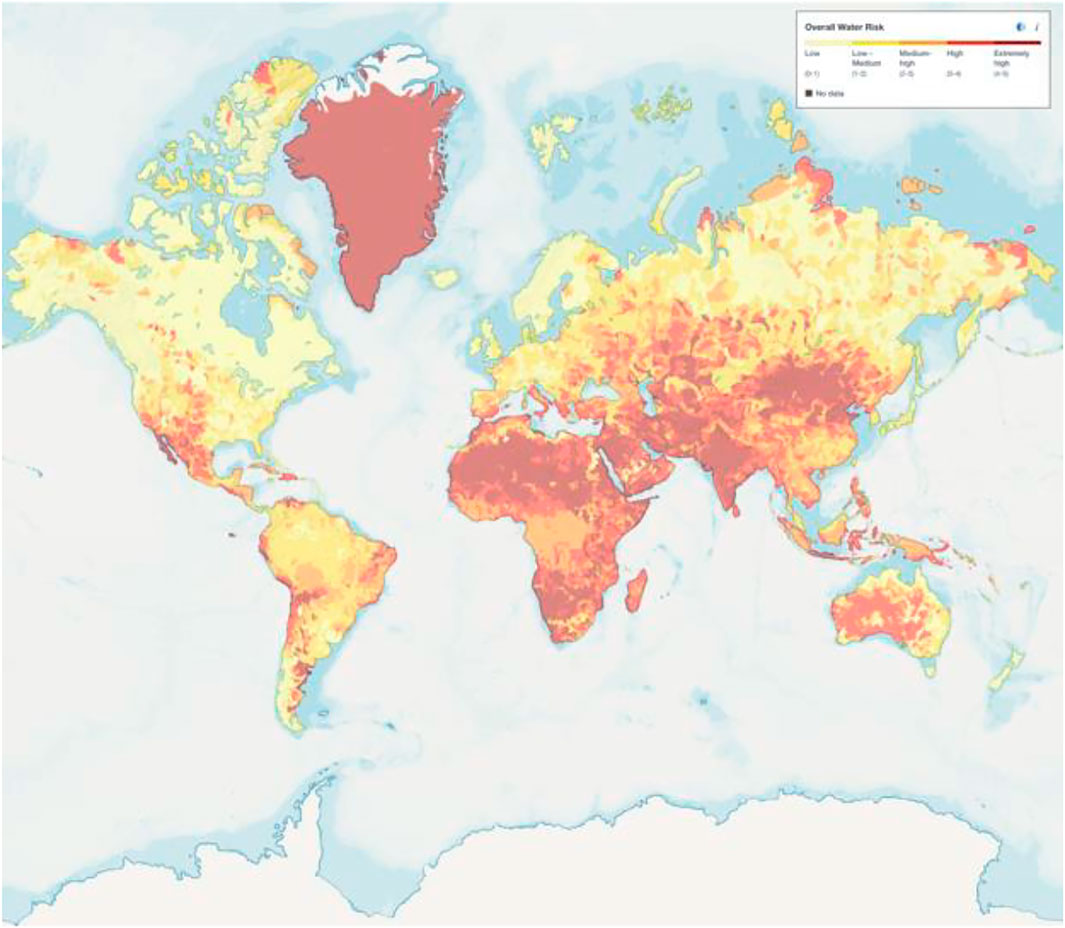
FIGURE 10. Global drought risk and water stress (https://wri.org/applications/aqueduct/water-risk-atlas/#/?advanced=false&basemap=hydro&indicator=w_awr_def_tot_cat&lat=-8.841651120809145&lng=35.06835937500001&mapMode=view&month=1&opacity=0.5&ponderation=DEF&predefined=false&projection=absolute&scenario=optimistic&scope=baseline&timeScale=annual&year=baseline&zoom=3). Used by permission, copywrite Beta Aqueduct Water Risk Atlas. Creative Commons (creativecommons.org/licenses/by/4.0/#). Use does not imply endorsement by Creative Commons or The Aqueduct.
Extreme Precipitation. Extreme precipitation events are becoming more common worldwide (Schiermeier, 2011). Using observations and modeling, Min et al. (2011) showed that human-induced increases in greenhouse gases have contributed to observed intensification of heavy precipitation events over a large part of northern hemisphere land areas (Figure 11). Pall et al. (2011) used modeling to evaluate the impacts of climate warming and concluded that in nine out of ten cases model results indicated that 20th century anthropogenic greenhouse gas emissions increased the risk of floods occurring in England and Wales in the autumn of 2000 by more than 20%. In two out of three cases by more than 90%. An example of an extreme, intense precipitation event is Hurricane Harvey, which in 2017 led to a total rainfall of about 1.5 m in three days when the hurricane became semi-stationary along the south Texas coast (Figure 12).
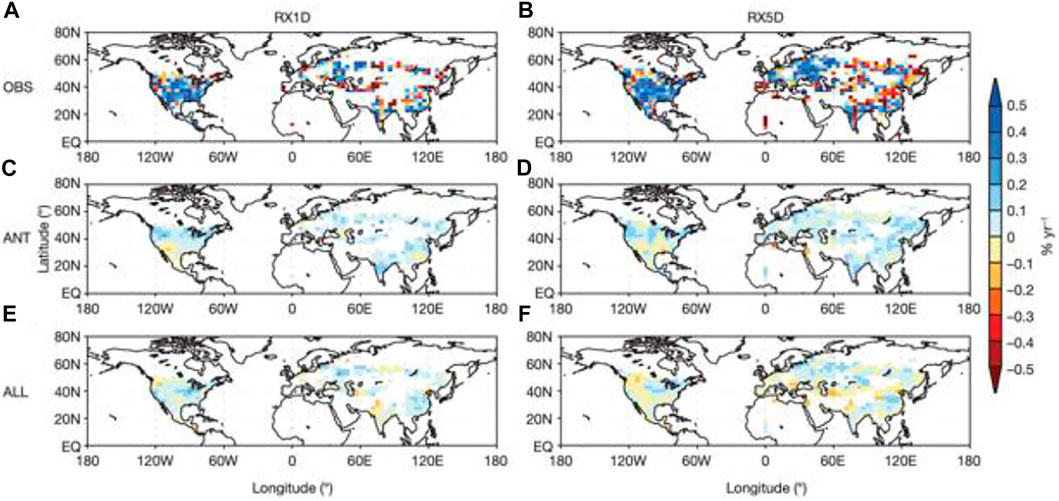
FIGURE 11. Comparison of geographical trends of extreme precipitation indices for 1951-1999 for annual maximum daily (RX1D) and five-day RX5D) precipitation amounts. (A, B) Observations, (C, D) model simulations, and (E, F) combined for observations (OBS), anthropogenic forcing (ANT), and anthropogenic plus natural forcings (ALL) (from Min et al., 2011, Reprinted by permission).
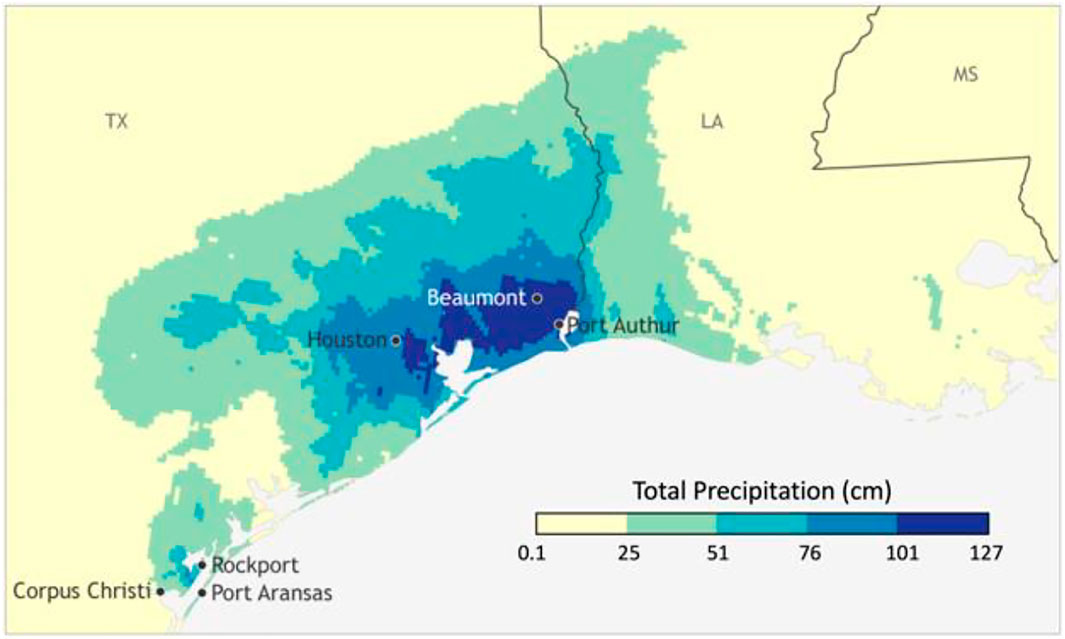
FIGURE 12. Rainfall during Hurricane Harvey in the Houston vicinity. Peak rainfall was more than 1.5m over three days. (modified from https://www.climate.gov/news-features/event-tracker/reviewing-hurricaneharveys-catastrophic-rain-and-flooding, accessed on 7 January 2021).
The information presented here shows that current and future climate forcings will affect almost all of the earth’s coasts. A broad zone that includes tropical to sub-temperate regions around the globe will be affected by most of the forcings discussed above (Figure 1). It includes a large proportion of the world’s population, almost all of the world’s major deltas, and most coastal megacities. It should be noted that climate change forcings that are not normally considered “coastal” forcings can impact coastal cities. For example, wildfires are becoming more intense and the length of the wildfire season in many areas is increasing. Coastal cities in some areas affected by wildfires that are normally not impacted by coastal climate forcings such as sea level rise or tropical cyclones can become less resilient and sustainable because of global change-fueled forcings such as intensification of wildfires and drought, especially when combined with other global change drivers. Some examples include southern California and northwestern Mexico, the Mediterranean and Middle East, central Chile, and the southeastern coast of Australia.
To directly address the concerns of the special issue on Coastal Cities in a Changing Climate, we suggest that climate change alone will threaten the sustainability and resilience of many coastal cities, especially in lower-income countries in the tropical to sub-temperate zone. Indeed, current climate impacts are already dramatically impacting coastal cities in advance of more inland cities. However, the nature of cities themselves in combination with other global change forcings suggest that the trend towards further urbanization may become infeasible as this century progresses. Taken together the global change forcings have contributed to a new state of the earth/societal system that has been termed the Anthropocene. We address these issues in following sections.
The Great Acceleration, Urbanization, and Resource Scarcity
Acceleration. Twentieth century science has led to increasing recognition of the impact of human activities on the biogeosphere (Steffen et al., 2011; Steffen et al., 2015). Because of this, there is a growing consensus that earth has entered a new geological epoch called the Anthropocene (Crutzen, 2002; Syvitski et al., 2020). This is especially apparent in the second half of the 20th century, the so-called exponential century or Great Acceleration (Steffen et al., 2018). Climate change and related mega-trends such as energy scarcity, population growth, massive resource consumption, and environmental degradation will impact natural and social systems globally, but the impact is being felt earlier and stronger in many coastal systems, especially in lower latitudes (e.g., Poff et al., 2002; Tessler et al., 2015; Day et al., 2018; Day and Rybczyk, 2019). Growing environmental impacts and decreasing energy availability will likely combine to limit options for restoration and management of coastal zones and complicate human response to mitigate climate change affects in coastal cities (Day et al., 2007; Tessler et al., 2015; Day et al., 2016; Wiegman et al., 2017).
Various points of view have developed over the future of the human population. In 2021, global population reached 7.7 billion. The United Nations, Department of Economic and Social Affairs, Population Division, (2019) projects that global population will continue to grow but at a slowing rate through the 21st century and that total population will reach 8.5 billion in 2030, 9.7 billion in 2050, and 10.9 billion by 2100. However, the demographic transition comes at the cost of increasing per capita consumption (Moses and Brown, 2003; DeLong et al., 2010) and may be limited by available resources.
Alternatively, Vollset et al. (2020) modeled future population on purely demographic drivers, fertility, migration, and mortality. He projects that global population will peak at 9.73 billion in 2064 and then a decline to 8.79 billion by 2100. To this can be added other important factors affecting population decline, female educational attainment, access to contraception, and increased per capita energy use that coincides with increased economic development (Moses and Brown, 2003). Thus, such scenarios of populations peaking and declining can be questioned given currently available energy reserves (DeLong et al., 2010; Burger, 2019). In the meantime, the high-income world continues to be the major consumers of fossil fuels, renewable biocapacity and contributor of greenhouse gases, all of which could attenuate the advance toward population peaking.
Urbanization. As noted above, an important defining characteristic of urbanization is the global urban transition. This occurred around 2010 when the proportion of the world’s population living in urban areas exceeded 50% and coincided with dramatic growth of megacities. This is fundamentally different from the way that our species lived for almost the entire period it has existed (about 300,000 years; Burger et al., 2017; Burger and Fristoe, 2018). As noted above, since 1800 the global population has grown by about 7.5 times while the growth of cities of more than a million people has grown by a factor of more than 500. In the mid-1800’s England was the first country to go through the urban transition. The urban transition has been interpreted as an encouraging sign that the human population and economy are on a path toward greater global sustainability according to some authors (e.g., Jenks and Burgess, 2000; Newman, 2006; Glaeser, 2011; Rose, 2016) who have suggested that concentrating the Earth’s growing population and industrial-technological-information economy in urban centers will reduce human impacts on the environment by packing more people in efficient cites.
However, this is likely not the case because modern megacities are dependent on energy and material flows from ex-urban environments globally (Rees and Wackernagel, 1996; Poumanyvong and Kaneko, 2010; Burger et al., 2012; Rees, 2012; Burger et al., 2017; Burger et al., 2019; Schramski et al., 2015; Baabou et al., 2017; Isman et al., 2018). It has been shown that per capita GDP is related to per capita energy use (Odum, 1971; Brown et al., 2011; Brown et al., 2014; Hall and Klitgaard, 2017). Brown et al. (2011), Brown et al. (2014), and Schramski et al. (2015) and others have concluded that the current economic paradigm based on increasing human population, economic development, and standard of living is no longer compatible with the biophysical limits of a finite Earth. The continuing global economic failure to maintain past growth rates is due to scarcity of critical resources including oil, gas, water, arable land (especially soil carbon and other depleting nutrients), metals, rare earths, fertilizers, fisheries, and wood (Burger et al., 2012). As the modern industrial-technological-informational-economy expanded and urbanization increased over the 20th century, it grew by consuming the Earth’s natural resources at unsustainable rates. Correlations between per capita GDP and per capita consumption of energy and other resources across nations and over time demonstrate how economic growth and development depend on the capital of natural resources or ecosystem services as well as cheap energy. There has been a decades-long trend of decreasing per capita consumption of multiple important commodities that indicate that this overexploitation has created an unsustainable bubble of population and economy (Burger et al., 2012). Portland Oregon is illustrative of the problem of the “green” city. Burger et al. (2012) reported that Portland, in spite of its reputation, used vast quantities of gasoline, natural gas, electricity, water, food, and other resources and released large amounts of liquid sewage, CO2, and solid waste. Domestic and international trade involved millions of tons annually. With respect to these flows, they concluded green Portland was about average for a United States city of comparable size with respect to the flows mentioned.
Energy. Per capita energy use and per capita greenhouse gas emissions in different countries varies by over two orders of magnitude and is related to the degree of urbanization (Burger et al., 2019). Energy use per capita is about 500 W in the poorest countries, barely more than the 100 W of human biological metabolism. In the richest countries, it is more than 10,000 W because human metabolism has been supplemented more than 100-fold from exogenous, ex-urban energy sources, mostly fossil fuels as well as enormous resources extracted from the biogeosphere. Data on a variety of variables that reflect standard of living and quality of life (Brown et al., 2014), including indices such as the human development index, wealth and poverty, infant mortality, food consumption, ownership patterns, education, and research spending, are strongly related to per capita GDP, which is strongly related to per capita energy consumption.
Two central questions relative to this dilemma is whether renewable energy can be ramped up fast enough to replace fossil fuel decline (due either to depletion of economically viable fossil fuel reserves or to conscious decisions to reduce fossil fuel use to avoid climate impacts) and the degree that renewables can replace all important uses of fossil fuels such as heavy industry, transportation, and food production (Smil, 2017; Day et al., 2018; EIA(Energy Information Agency), 2019; IEA(International Energy Agency) 2020; Smil, 2021).
To avoid temperature increases greater than 2 C, fossil fuels must be largely phased out by 2050 (IPCC, 2019; Oppenheimer et al., 2019). The IEA(International Energy Agency). (2020) reported that global reliance on fossil fuels decreased from 95% of total primary energy in 1975 to about 85% in 2020. They project that 70–75% of global primary energy consumption may be met via fossil fuels in 2040. If new renewables are to replace fossil fuels by mid-century, they must increase by more than 10-fold in 3 decades. It took total fossil fuel growth 77 years to increase by a factor of 10 to reach 2010 levels (Maggio and Caddiola, 2012). Thus, to meet fossil fuel phase out by 2050, new renewables growth must be much faster than fossil fuel growth in the 20th century.
As noted above, per capita GDP, per capita energy use and per capita greenhouse gas emissions for most countries of the world increased as the level of urbanization increased (Burger et al., 2019). In all high-income countries, the proportion of the population living in urban areas is greater than 80%. As the proportion of the urban population increases in countries as they go through the urban transition, so does GDP (Burger et al., 2019). The increase is dramatic with per capita GDP, energy consumption and greenhouse gas emissions increasing by 100 to 1,000 fold (2–3 orders of magnitude) from the poorest and least urbanized nations to the richest and most urbanized nations.
It has been argued that the shift to a service economy results in lower per capita energy consumption because by shifting from producing goods to providing services, a society can reduce consumption and result in lower per capita energy use (Hawken et al., 2000; –See Heiskanen and Jalas, 2000; Heiskanen et al., 2001; Romm, 2002; Victor, 2010 for reviews). Direct energy intensity of value added and direct energy use per worker are significantly lower for services compared to industry for the United States. However, Fix (2019) showed that fossil fuel use per capita and CO2 emissions per capita were significantly and positively related to the percent of the population in the service sector. This is consistent with the findings of Burger et al. (2019) showing increasing per capita energy use and greenhouse gas emissions and that the service sector accounted for a greater proportion of the economy with increasing urbanization.
Urban Areas and Disease. Urbanization complicates global health and facilitates epidemics of infectious diseases (Neiderud, 2015). With the rapid urbanization that has taken place over the past century, rural areas can become incubators for epidemics that can spread more rapidly to cities via global transportation, becoming worldwide threats. The current COVID-19 pandemic is an illustrative example. Among different cities and within individual cities, there can be very different levels of social security and access to modern health care, as for example the differences between slums and wealthy neighborhoods. The former are often very crowded and lack access to freshwater and sanitation facilities. Thus, the hyper-densities of modern cities (Burger et al., 2017) can be catalysts for rapid spread of infectious diseases due to such factors as tourism and international trade.
As noted, the conditions leading to the spread of infectious, as well as lifestyle diseases, are common in cities in poorer countries. This is exacerbated in cities in coastal areas in tropical and subtropical areas where much urban growth is taking place. An interesting case study is the ancient civilization of the Maya culture that progressed its urbanization from villages in southern lowland swamps 3,000 years ago, to magnificent templed cities on promontories by 1,500 years ago. This was followed by the famous Maya collapse, but the collapse was actually only of the interior cities. Coastal cities then moved to the fore of commerce by capitalizing on the efficiency of maritime transportation. The lesson to be learned was/is that large, dense urban populations cannot sustain themselves in challenging tropical environments where they are highly susceptible to pandemics. Rather they had to be installed on high terrain, in porous substrates to avoid water contamination and enable annual flushing of the cities by rains. This however unexpectedly exposed them to extended droughts in the AD 800’s that diminished their sustaining clean water resource, equivalent to our singular reliance on fossil energy of today’s society (Scarborough et al., 2012; Gunn et al., 2019a; Gunn et al., 2019b).
Food security. Both climate change and resource scarcity will have consequences for food security. We are already seeing the effects of extreme heat waves on food production. Schramski et al. (2019) discussed future trends affecting the sustainability of food self-sufficiency. The ability to feed the world’s growing population over the past century and a half depended on decreasing food loses, increasing food yields, and cheap fossil fuels and transportation networks to distribute food globally (Hammond et al., 2015). Food self-sufficiency at the country level has been declining over the last 4 decades as the number of countries able to provide sufficient local food declines. Food imports have been able to offset most of the country declines. This is based on cheap fossil fuels and technological advances in a mechanized food production system with a high level of food preservation. Schramski et al. (2019) argue that global changes in climate, energy availability and other factors will lead to challenges in providing sufficient food for a growing urban population. As discussed earlier, Xu et al. (2020) reported that the narrowing range of the global climate envelope that supports life will impact production of crops and livestock as well as human health. Australia for example, is already experiencing mass die-offs in marine and terrestrial environments due to increasing heatwaves (Ruthrof et al., 2018).
Marine heatwaves also pose a threat to marine fisheries and coastal economic livelihoods. An example is the impact of the 2013–2016 marine heatwave in the northeast Pacific. Cheung and Frolicher (2020) reported that modeling studies indicate that the heatwave caused biomass decreases and shifts in biogeography of northeast Pacific fish stocks. The heatwave has increased the likelihood of harmful algal blooms, shifted distributions of marine life, and changed food webs. Barbeaux et al. (2020) reported that the heatwave led to shifts in species distribution and reduced productivity of Pacific cod in the Gulf of Alaska. The 2013–2015 heat wave increased algal toxins in shellfish and led to the closure of the Dungeness crab fishery.
The global change impacts discussed above have disproportionate impacts on low-income countries. For example, Tessler et al. (2015) reported that combined changes in land use, regional water management, subsidence, climate change, and increasing energy costs will increase risks significantly resulting in non-sustainable outcomes for deltaic coasts. Although both developed and developing countries are exposed to risks, high-income countries presently limit their risk by expensive and energy-intensive infrastructure and coastal defenses. In a world characterized by increasingly scarce and expensive energy, maintaining and repairing such infrastructure will likely become unsustainable. More generally, the impacts of climate change in combination with other global change forcings will fall disproportionately on lesser developed countries, especially large cities in the coastal areas, which are more at risk from climate change. Local and regional food insecurity may additionally be exacerbated by more frequent and longer heat-waves that can impact fisheries and marine resource populations.
Summary and Conclusions
Urbanization by the human species started 5,000 years ago worldwide and in the 21st century is rapidly expanding at an unprecedented pace surpassing 50% around 2010. Urbanization is occurring most rapidly in the tropical and sub-tropical zones and in coastal areas, so they represent a potential view of the future of urbanization as a whole. Climate change in conjunction with other global change impacts will likely diminish the sustainability of cities, especially in coastal areas of low-income countries. Climate change impacts include sea-level rise, temperature increase, increasing frequency of the most intense tropical cyclones, changes in freshwater input to coastal zones, and increasingly severe extreme precipitation events, droughts, freshwater shortages, heat waves, and wildfires. Climate change alone will diminish the sustainability and resilience of coastal cities, but combined with other global changes, this suite of forcings represent an existential threat to coastal cities. Urbanization has brought about two orders-of-magnitude (100-fold) increases in per capita GDP, energy use and greenhouse gas emissions, which in turn has led to unprecedented demand for natural resources and degradation of natural systems that provide these resources (Burger et al., 2019). The urban transition coincides with the Great Acceleration of the Anthropocene, both of which reflect the vastly increased use of energy, principally fossil fuels. These energy sources enabled and underwrote the dramatic expansion of the urban age and fueled the Great Acceleration. It is questionable whether renewable energy can ramp up fast enough to fuel further urban growth, reverse climate change, and minimize further environmental degradation. Continuing the trend toward urbanization is questionable given these trajectories, especially in vulnerable low-income cities in coastal zones in the tropical belt.
Data Availability Statement
The original contributions presented in the study are included in the article/supplementary material, further inquiries can be directed to the corresponding author.
Author Contributions
All authors listed have made a substantial, direct, and intellectual contribution to the work and approved it for publication.
Funding
Partial funding of this research was supported by aggregate funding from University of North Carolina-Greensboro Office of Research and Engagement and the UNCG University Libraries.
Conflict of Interest
The authors declare that the research was conducted in the absence of any commercial or financial relationships that could be construed as a potential conflict of interest.
The handling editor declared past collaboration with one of the co-authors, JD.
Publisher’s Note
All claims expressed in this article are solely those of the authors and do not necessarily represent those of their affiliated organizations, or those of the publisher, the editors and the reviewers. Any product that may be evaluated in this article, or claim that may be made by its manufacturer, is not guaranteed or endorsed by the publisher.
Acknowledgments
We thank Rachael Hunter and Robert Lane for help with the manuscript. JB was supported by the Bridging Biodiversity and Conservation Science Program in the Arizona Institutes of Resilience at the University of Arizona.
References
Baabou, W., Grunewald, N., Ouellet-Plamondon, C., Gressot, M., and Galli, A. (2017). The Ecological Footprint of Mediterranean Cities: Awareness Creation and Policy Implications. Environ. Sci. Pol. 69, 94–104. doi:10.1016/j.envsci.2016.12.013
Barbeaux, S. J., Holsman, K., and Zador, S. (2020). Marine Heatwave Stress Test of Ecosystem-Based Fisheries Management in the Gulf of Alaska Pacific Cod Fishery. Front. Mar. Sci. 7, 1–21. doi:10.3389/fmars.2020.00703
Bender, M. A., Knutson, T. R., Tuleya, R. E., Sirutis, J. J., Vecchi, G. A., Garner, S. T., et al. (2010). Modeled Impact of Anthropogenic Warming on the Frequency of Intense Atlantic Hurricanes. Science 327, 454–458. doi:10.1126/science.1180568
Bhatia, K. T., Vecchi, G. A., Knutson, T. R., Murakami, H., Kossin, J., Dixon, K. W., et al. (2019). Recent Increases in Tropical Cyclone Intensification Rates. Nat. Commun. 10, 1–9. doi:10.1038/s41467-019-08471-z
Brown, J. H., Burger, J. R., Burnside, W. R., Chang, M., Davidson, A. D., Fristoe, T. S., et al. (2014). Macroecology Meets Macroeconomics: Resource Scarcity and Global Sustainability. Ecol. Eng. 65, 24–32. doi:10.1016/j.ecoleng.2013.07.071
Brown, J. H., Burnside, W. R., Davidson, A. D., DeLong, J. P., Dunn, W. C., Hamilton, M. J., et al. (2011). Energetic Limits to Economic Growth. BioScience 61, 19–26. doi:10.1525/bio.2011.61.1.7
Burger, J. R., Allen, C. D., Brown, J. H., Burnside, W. R., Davidson, A. D., Fristoe, T. S., et al. (2012). The Macroecology of Sustainability. PLOS Biol. 10, e1001345. doi:10.1371/journal.pbio.1001345
Burger, J. R., Brown, J. H., Day, J. W., Flanagan, T. P., and Roy, E. D. (2019). The central Role of Energy in the Urban Transition: Global Challenges for Sustainability. Biophys. Econ. Resour. Qual., 1–13. doi:10.1007/s41247-019-0053-z
Burger, J. R., and Fristoe, T. S. (2018). Hunter-gatherer Populations Inform Modern Ecology. Proc. Natl. Acad. Sci. USA. 115, 1137–. doi:10.1073/pnas.1721726115
Burger, J. R. (2019). Fueling Fertility Is Emptying the Planet's Resources. BioScience 69, 757–758. doi:10.1093/biosci/biz084
Burger, J. R., Weinberger, V. P., and Marquet, P. A. (2017). Extra-metabolic Energy Use and the Rise in Human Hyper-Density. Sci. Rep. 7, 1–5. doi:10.1038/srep43869
C40.org (2020). Staying Afloat: The Urban Response to Sea Level Rise. Available at: https://www.C40.org/other/the-future-we-don-t-want-staying-afloat-the-urban-response-to-sea-level-rise. (Accessed May 23, 2021).
Ceballos, G., Ehrlich, P. R., and Raven, P. H. (2020). Vertebrates on the Brink as Indicators of Biological Annihilation and the Sixth Mass Extinction. Proc. Natl. Acad. Sci. USA. 117, 13596–13602. doi:10.1073/pnas.1922686117
Cheung, W. W. L., and Frölicher, T. L. (2020). Marine Heatwaves Exacerbate Climate Change Impacts for Fisheries in the Northeast Pacific. Sci. Rep. 10, 6678. doi:10.1038/s41598-020-63650-z
Day, J., D’Elia, C., Wiegman, A., Rutherford, J., Hall, C., Lane, R., et al. (2018). The Energy Pillars of Society: Perverse Interactions of Human Resource Use, the Economy, and Environmental Degradation. Biophysical Econ. Resource Qual. 3, 2. doi:10.1007/s41247-018-0035-65
Day, J., Gunn, J., Folan, W., Yáñez-Arancibia, A., and Horton, B. (2007). Emergence of Complex Societies after Sea Level Stabilized. EOS 88, 170–171. doi:10.1029/2007eo150001
Day, J., Ramachandran, R., Giosan, L., Syvitski, J., Kemp, P., Wolanski, E., et al. (2019). “Delta Winners and Losers in the Anthropcene,” in Coasts and Estuaries - the Future (Amsterdam: Elsevier), 153–172.
Day, J., and Rybczyk, J. (2019). “Global Change Impacts on the Future of Coastal Systems: Perverse Interactions Among Climate Change, Ecosystem Degradation, Energy Scarcity and Population,” in Coasts and Estuaries – the Future. Editors E. Wolanski, J. Day, M. Elliott, and R. Ramachandran (Amsterdam: Elsevier), 635–654. doi:10.1016/b978-0-12-814003-1.00036-8
Day, J. W., Agboola, J., Chen, Z., D’Elia, C., Forbes, D. L., Giosan, L., et al. (2016). Approaches to Defining Deltaic Sustainability in the 21st century. Estuarine, Coastal Shelf Sci. 183, 275–291. doi:10.1016/j.ecss.2016.06.018
Day, J. W., Gunn, J. D., Folan, W. J., Yáñez-Arancibia, A., and Horton, B. P. (2012). The Influence of Enhanced post-glacial Coastal Margin Productivity on the Emergence of Complex Societies. The J. Isl. Coastal Archaeology. 7, 23–52. doi:10.1080/15564894.2011.650346
DeConto, R. M., and Pollard, D. (2016). Contribution of Antarctica to Past and Future Sea-Level Rise. Nature 531, 591–597. doi:10.1038/nature17145
DeLong, J., Burger, O., and Hamilton, M. (2010). Current Demographics Suggest Future Energy Supplies Will Be Inadequate to Slow Human Population Growth. Plos One. 5 (10), e13206. doi:10.1371/journal.pone.0013206
Dyer, C. L. (2009). “From the Phoenix Effect to Punctuated Entropy: The Culture of Response as a Unifying Paradigm of Disaster Mitigation and Recovery,” in The Political Economy of Hazards and Disasters. Editors E. Jones, and A. Murphy (Lanham, MD: AltaMira Press), 343–356.
EIA(Energy Information Agency) (2019). International Energy Outlook. Washington DC: EIAAvailable at: https://www.eia.gov/160.
Elsner, J. B., Kossin, J. P., and Jagger, T. H. (2008). The Increasing Intensity of the Strongest Tropical Cyclones. Nature 455, 92–95. doi:10.1038/nature07234
Emanuel, K. (2005). Increasing Destructiveness of Tropical Cyclones over the Past 30 Years. Nature 436, 686–688. doi:10.1038/nature03906
FitzGerald, D. M., Fenster, M. S., Argow, B. A., and Buynevich, I. V. (2008). Coastal Impacts Due to Sea-Level Rise. Annu. Rev. Earth Planet. Sci. 36, 601–647. doi:10.1146/annurev.earth.35.031306.140139
Fix, B. (2019). Dematerialization through Services: Evaluating the Evidence. Biophys. Econ. Resour. Qual. 4, 1–17. doi:10.1007/s41247-019-0054-y
Giosan, L., Syvitski, J., Constantinescu, S., and Day, J. (2014). Climate Change: Protect the World's Deltas. Nature 516, 31–33. doi:10.1038/516031a
Goldenberg, S. B., Landsea, C., Mestas-Nunez, A., and Gray, W. (2001). The Recent Increase in Atlantic hurricane Activity: Causes and Implications. Science 293, 474–479. doi:10.1126/science.1060040
Grinsted, A., Moore, J., and Jevrejeva, S. (2012). Homogeneous Record of Atlantic hurricane Surge Threat since 1923. PNAS 109, 48. doi:10.1073/pnas.1209542109/-/DCSupplemental
Gunn, J. D., Day, J. W., Folan, W. J., and Moerschbaecher, M. (2019a). Geo-cultural Time: Advancing Human Societal Complexity within Worldwide Constraint Bottlenecks-A Chronological/Helical Approach to Understanding Human-Planetary Interactions. Biophys. Econ. Resour. Qual. 4, 10. doi:10.1007/s41247-019-0058-7
Gunn, J. D., Folan, W. J., Torrescano-Valle, N., Faust, B. B., Geovannini-Acuña, H. Z., and Siemens, A. H. (2019b). “From Calakmul to the Sea: The Historical Ecology of a Classic Maya City that Controlled the Candelaria/Champoton Watersheds,” in The Holocene and Anthropocene Environmental History of Mexico: A Paleoecological Approach on Mesoamerica. Editors N. Torrescano Valle, G.A. Islebe, and P.D. Roy (New York: Springer Nature)), 209–248. doi:10.1007/978-3-030-31719-5_11
Hammond, S. T., Brown, J. H., Burger, J. R., Flanagan, T. P., Fristoe, T. S., Mercado-Silva, N., et al. (2015). Food Spoilage, Storage, and Transport: Implications for a Sustainable Future. BioSci 65, 758–768. doi:10.1093/biosci/biv081
Hansen, J., Sato, M., Hearty, P., Ruedy, R., Kelley, M., Masson-Delmotte, V., et al. (2015). Ice Melt, Sea Level Rise and Superstorms: Evidence from Paleoclimate Data, Climate Modeling, and Modern Observations that 2 C Global Warming Is Highly Dangerous. Atmos. Chem. Phys. 16, 3761–3812.
Hawken, P., Lovins, A., and Lovins, P. L. (2000). Natural Capitalism: Creating the Next Industrial Revolution. Boston: US Green Building Council.
Heiskanen, E., Halme, M., Jalas, M., Kärnä, A., and Lovio, R. (2001). Dematerialization: The Potential of ICT and Services. Tech. rep. Helsinki: Finnish Ministry of the Environment.
Heiskanen, E., and Jalas, M. (2000). Dematerialization through Services—A Review and Evaluation of the Debate. Tech. rep. Helsinki: Finnish Ministry of the Environment.
Hill, R. W., Muhich, T. E., and Humphries, M. M. (2013). City-Scale Expansion of Human Thermoregulatory Costs. PLOS ONE. 8, e76238. doi:10.1371/journal.pone.0076238
Horton, B. P., Rahmstorf, S., Engelhart, S. E., and Kemp, A. C. (2014). Expert Assessment of Sea-Level Rise by AD 2100 and AD 2300. Quat. Sci. Rev. 84, 1–6. doi:10.1016/j.quascirev.2013.11.002
Hoyos, C. D., Agudelo, P., Webster, P., and Curry, J. (2006). Deconvolution of the Factors Contributing to the Increase in Global hurricane Intensity. Science 312, 94–97. doi:10.1126/science.1123560
IEA(International Energy Agency) (2020). World Energy Outlook. Paris, France: IEA ParisAvailable at: https://www.iea.org/reports/world-energy-outlook-2021.
Ingram, L., and Malamud-Roam, F. (2013). The West without Water. Berkeley: University of California Press, 256.
IPCC (2013). “Climate Change 2013: The Physical Science Basis,” in Contribution of Working Group 1 to the Fifth Assessment Report of the Intergovernmental Panel on Climate Change Cambridge. Geneva, Switzerland: IPCC, 1535.
IPCC (2019). “Summary for Policymakers,” in IPCC Special Report on the Ocean and Cryosphere in a Changing Climate. Editors H.-O. Pörtner, D. C. Roberts, V. Masson-Delmotte, P. Zhai, M. Tignor, E. Poloczanskaet al. Geneva, Switzerland: IPCC.
Isman, M., Archambault, M., Racette, P., Konga, C. N., Llaque, R. M., Lin, D., et al. (2018). Ecological Footprint Assessment for Targeting Climate Change Mitigation in Cities: a Case Study of 15 Canadian Cities According to Census Metropolitan Areas. J. Clean. Prod. 174, 1032–1043. doi:10.1016/j.jclepro.2017.10.189
Jenks, M., and Burgess, R. (2000). Compact Cities: Sustainable Urban Forms for Developing Countries. Abingdon, UK: Taylor & Francis.
Kaufmann, R. K., Kauppi, H., Mann, M. L., and Stock, J. H. (2011). Reconciling Anthropogenic Climate Change with Observed Temperature 1998-2008. Proc. Natl. Acad. Sci. 108, –. doi:10.1073/pnas.1102467108
Kopp, R. E., Kemp, A. C., Bittermann, K., Horton, B. P., Donnelly, J. P., Gehrels, W. R., et al. (2016). Temperature-driven Global Sea-Level Variability in the Common Era. Proc. Natl. Acad. Sci. USA. 113, E1434–E1441. doi:10.1073/pnas.1517056113
Lee, S. B., LiZhang, M. F., and Zhang, F. (2017). Impact of Sea Level Rise on Tidal Range in Chesapeake and Delaware Bays. J. Geophys. Res. Oceans. 122 (5), 3917–3938. doi:10.1002/2016JC012597
Li, L., and Chakraborty, P. (2020). Slower Decay of Landfalling Hurricanes in a Warming World. Nature 587, 230–. doi:10.1038/s41586-020-2867-7
Loeb, N. G., Johnson, G. C., Thorsen, T. J., Lyman, J. M., Rose, F. G., and Kato, S. (2021). Satellite and Ocean Data Reveal Marked Increase in Earth's Heating Rate. Geophys. Res. Lett, 1–8. doi:10.1029/2021GL093047
Maggio, G., and Cacciola, G. (2012). When Will Oil, Natural Gas, and Coal Peak?. Fuel 98, 111–123. doi:10.1016/j.fuel.2012.03.021
Marquet, P. A., Santoro, C. M., Latorre, C., Standen, V. G., Abades, S. R., Rivadeneira, M. M., et al. (2012). Emergence of Social Complexity Among Coastal hunter-gatherers in the Atacama Desert of Northern Chile. Proc. Natl. Acad. Sci. 109, 14754–14760. doi:10.1073/pnas.1116724109
Mei, W., Xie, S.-P., Primeau, F., McWilliams, J. C., and Pasquero, C. (2015). Northwestern Pacific Typhoon Intensity Controlled by Changes in Ocean Temperatures. Sci. Adv. 1, e1500014. doi:10.1126/sciadv.1500014
Milly, P. C. D., and Dunne, K. A. (2020). Colorado River Flow Dwindles as Warming-Driven Loss of Reflective Snow Energizes Evaporation. Science 367, 1252–1255. doi:10.1126/science.aay9187
Min, S.-K., Zhang, X., Zwiers, F. W., and Hegerl, G. C. (2011). Human Contribution to More-Intense Precipitation Extremes. Nature 470, 378–381. doi:10.1038/nature09763
Mora, C., Dousset, B., Caldwell, I. R., Powell, F. E., Geronimo, R. C., Bielecki, C. R., et al. (2017). Global Risk of Deadly Heat. Nat. Clim Change. 7, 501–506. doi:10.1038/nclimate3322
Moses, M. E., and Brown, J. H. (2003). Allometry of Human Fertility and Energy Use. Ecol. Lett. 6, 295–300. doi:10.1046/j.1461-0248.2003.00446.x
Neiderud, C.-J. (2015). How Urbanization Affects the Epidemiology of Emerging Infectious Diseases. Infect. Ecol. Epidemiol. 5, 27060. doi:10.3402/iee.v5.27060
Newman, P. (2006). The Environmental Impact of Cities. Environ. Urbanization. 18, 275–295. doi:10.1177/0956247806069599
Oliver, E., Benthuysen, J., Darmaraki, S., Donat, M., Hobday, A., Holbrock, N., et al. (2021). Marine Heatwaves. Annu. Rev. Mar. Sci. 13, 312–342. doi:10.1146/annurev-marine-032720-095144
Oppenheimer, M., Glavovic, B. C., Hinkel, J., van de Wal, R., Magnan, A. K., Abd-Elgawad, A., et al. (2019). “Sea Level Rise and Implications for Low-Lying Islands, Coasts and Communities,” in IPCC Special Report on the Ocean and Cryosphere in a Changing Climate. Editors H. O. Pörtner, D. C. Roberts, V. Masson-Delmotte, P. Zhai, M. Tignor, E. Poloczanskaet al. Geneva, Switzerland: IPCC.
Pall, P., Aina, T., Stone, D. A., Stott, P. A., Nozawa, T., Hilberts, A. G. J., et al. (2011). Anthropogenic Greenhouse Gas Contribution to Flood Risk in England and Wales in Autumn 2000. Nature 470, 382–385. doi:10.1038/nature09762
Pfeffer, W. T., Harper, J. T., and O'Neel, S. (2008). Kinematic Constraints on Glacier Contributions to 21st-century Sea-Level Rise. Science 321, 1340–1343. doi:10.1126/science.1159099
Pietrafesa, L., Zhang, H., Bao, S., Gayes, P., and Hallstrom, J. (2019). Coastal Flooding and Inundation and Inland Flooding Due to Downstream Blocking. Jmse 7 (10), 336. doi:10.3390/jmse7100336
Poff, L., Brinson, M., and Day, J. (2002). Aquatic Ecosystems & Global Climate Change: Potential Impacts on Inland Freshwater and Coastal Wetlands Ecosystems in the United States. Arlington, VA: Pew Center on Global Climate Change.
Poumanyvong, P., and Kaneko, S. (2010). Does Urbanization lead to Less Energy Use and Lower CO2 Emissions? A Cross-Country Analysis. Ecol. Econ. 70, 434–444. doi:10.1016/j.ecolecon.2010.09.029
Rappaport, J., and Sachs, J. (2002). The U.S. As a Coastal Nation. Federal Reserve Bank of Kansas City paper RWP 01-11. 38 p with appendices.
Rees, W. E. (2012). Cities as Dissipative Structures: Global Change and the Vulnerability of Urban Civilization,” in Sustainability Science. Editors W. Weinstein, and R. Turner, New York, NY: Springer, 243–274.
Rees, W., and Wackernagel, M. (1996). Urban Ecological Footprints: Why Cities Cannot Be Sustainable-And Why They Are a Key to Sustainability. Environ. Impact Assess. Rev. 16, 223–248. doi:10.1016/s0195-9255(96)00022-4
Robinne, F.-N., Bladon, K. D., Miller, C., Parisien, M.-A., Mathieu, J., and Flannigan, M. D. (2020). A Spatial Evaluation of Global Wildfire-Water Risks to Human and Natural Systems. Sci. Total Environ., 610-611, 1193–1206. doi:10.1016/j.scitotenv.2017.08.112
Romm, J. (2002). The Internet and the New Energy Economy. Resour. Conservation Recycling. 36, 197–210. doi:10.1016/s0921-3449(02)00084-8
Ruthrof, K. X., Breshears, D. D., Fontaine, J. B., Froend, R. H., Matusick, G., Kala, J., et al. (2018). Subcontinental Heat Wave Triggers Terrestrial and marine, Multi-Taxa Responses. Sci. Rep. 8, 13094. doi:10.1038/s41598-018-31236-5
Scarborough, V. L., Dunning, N. P., Tankersley, K. B., Carr, C., Weaver, E., Grazioso, L., et al. (2012). Water and Sustainable Land Use at the Ancient Tropical City of Tikal, Guatemala. Proc. Natl. Acad. Sci. 109, 12408–12413. doi:10.1073/pnas.1202881109
Schiermeier, Q. (2011). Increased Flood Risk Linked to Global Warming. Nature 470, 316. doi:10.1038/470316a
Schramski, J. R., Gattie, D. K., and Brown, J. H. (2015). Human Domination of the Biosphere: Rapid Discharge of the Earth-Space Battery Foretells the Future of Humankind. Proc. Natl. Acad. Sci. USA. 112, 9511–9517. doi:10.1073/pnas.1508353112
Schramski, J. R., Woodson, C. B., Steck, G., Munn, D., and Brown, J. H. (2019). Declining Country-Level Food Self-Sufficiency Suggests Future Food Insecurities. Biophys. Econ. Resour. Qual. 4. doi:10.1007/s41247-019-0060-0
Steffen, W., Broadgate, W., Deutsch, L., Gaffney, O., and Ludwig, C. (2015). The Trajectory of the Anthropocene: the Great Acceleration. Anthropocene Rev. 2, 81–98. doi:10.1177/2053019614564785
Steffen, W., Grinevald, J., Crutzen, P., and McNeill, J. (2011). The Anthropocene: Conceptual and Historical Perspectives. Phil. Trans. R. Soc. A. 369, 842–867. doi:10.1098/rsta.2010.0327
Steffen, W., Rockström, J., Richardson, K., Lenton, T. M., Folke, C., Liverman, D., et al. (2018). Trajectories of the Earth System in the Anthropocene. Proc. Natl. Acad. Sci. USA. 115, 8252–8259. doi:10.1073/pnas.1810141115
Stott, P. A., Stone, D. A., and Allen, M. R. (2004). Human Contribution to the European Heatwave of 2003. Nature 432, 610–614. doi:10.1038/nature03089
Syvitski, J., Walters, C., Day, J., Milliman, J., Summerhayes, C., Steffen, W., et al. (2020). An Extraordinary Outburst of Human Energy Consumption and Resultant Geological Impacts Beginning Around 1950 CE Initiated the Proposed Anthropocene Epoch. Commun. Earth Environ. 1, 1–13. doi:10.1038/s43247-020-00029 doi:10.1038/s43247-020-00049-8
Syvitski, J. P. M., Kettner, A. J., Overeem, I., Hutton, E. W. H., Hannon, M. T., Brakenridge, G. R., et al. (2009). Sinking Deltas Due to Human Activities. Nat. Geosci. 2, 681–686. doi:10.1038/ngeo629
Tessler, Z. D., Vörösmarty, C. J., Grossberg, M., Gladkova, I., Aizenman, H., Syvitski, J. P. M., et al. (2015). Profiling Risk and Sustainability in Coastal Deltas of the World. Science 349, 638–643. doi:10.1126/science.aab3574
United Nations, Department of Economic and Social Affairs, Population Division (2019). World Population Prospects 2019: Highlights (ST/ESA/SER.A/423). (Accessed 8 May, 2021). https://population.un.org/wpp/
United Nations, Department of Economic and Social Affairs, Population Division. (2018). The World’s Cities in 2018—Data Booklet (ST/ESA/ SER.A/417). Available at: https://www.flickr.com/photos/thisisinbalitimur/28066940649.
Vermeer, M., and Rahmstorf, S. (2009). Global Sea Level Linked to Global Temperature. Proc. Natl. Acad. Sci. 106, 21527–21532. doi:10.1073/pnas.0907765106
Vollset, S. E., Goren, E., Yuan, C.-W., Cao, J., Smith, A. E., Hsiao, T., et al. (2020). Fertility, Mortality, Migration, and Population Scenarios for 195 Countries and Territories from 2017 to 2100: a Forecasting Analysis for the Global Burden of Disease Study. The Lancet. 396, 1285–1306. doi:10.1016/s0140-6736(20)30677-2
Wahl, T., Jain, S., Bender, J., Meyers, S. D., and Luther, M. E. (2015). Increasing Risk of Compound Flooding from Storm Surge and Rainfall for Major US Cities. Nat. Clim Change. 5, 1093–1097. doi:10.1038/nclimate2736
Webster, P. J., Holland, G., Curry, J., and Chang, H. (2005). Changes in Tropical Cyclone Number, Duration, and Intensity in a Warming Environment. Science 309, 1844–1846. doi:10.1126/science.1116448
Wiegman, A. R. H., Day, J. W., D'Elia, C. F., Rutherford, J. S., Morris, J. T., Roy, E. D., et al. (2017). Modeling Impacts of Sea-Level Rise, Oil price, and Management Strategy on the Costs of Sustaining Mississippi delta Marshes with Hydraulic Dredging. Sci. Total Environ. 618, 1547–1559. doi:10.1016/j.scitotenv.2017.09.314
Williams, A. P., Cook, E. R., Smerdon, J. E., Cook, B. I., Abatzoglou, J. T., Bolles, K., et al. (2020). Large Contribution from Anthropogenic Warming to an Emerging North American Megadrought. Science 368, 314–318. doi:10.1126/science.aaz9600
Xu, C., Kohler, T. A., Lenton, T. M., Svenning, J.-C., and Scheffer, M. (2020). Future of the Human Climate Niche. Proc. Natl. Acad. Sci. USA. 117:11350, 11355. doi/doi:10.1073/pnas.1910114117
Keywords: climate change, Anthropocene, deltas, sustainability, tropical coasts, megacities
Citation: Day JW, Gunn JD and Burger JR (2021) Diminishing Opportunities for Sustainability of Coastal Cities in the Anthropocene: A Review. Front. Environ. Sci. 9:663275. doi: 10.3389/fenvs.2021.663275
Received: 02 February 2021; Accepted: 15 July 2021;
Published: 02 August 2021.
Edited by:
Bruce Christopher Glavovic, Massey University, New ZealandReviewed by:
Robert James Nicholls, University of East Anglia, United KingdomLynn Donelson Wright, Southeastern Universities Research Association, United States
Charles Reid Nichols, Marine Information Resources Corporation (MIRC), United States
Copyright © 2021 Day, Gunn and Burger. This is an open-access article distributed under the terms of the Creative Commons Attribution License (CC BY). The use, distribution or reproduction in other forums is permitted, provided the original author(s) and the copyright owner(s) are credited and that the original publication in this journal is cited, in accordance with accepted academic practice. No use, distribution or reproduction is permitted which does not comply with these terms.
*Correspondence: John W. Day, johnday@lsu.edu