- 1Zoology Department, University of British Columbia, Vancouver, BC, Canada
- 2Biodiversity Research Centre, University of British Columbia, Vancouver, BC, Canada
- 3Department of Conservation Ecology and Entomology, Stellenbosch University, Stellenbosch, South Africa
- 4Department of Evolutionary Biology and Environmental Studies, University of Zurich, Zurich, Switzerland
- 5Landscape Ecology and Environmental Systems Analysis, Institute of Geoecology, Technische Universität Braunschweig, Braunschweig, Germany
- 6Department of Water Resources and Ecosystems, IHE Delft Institute for Water Education, Delft, Netherlands
- 7Department of Soil Science, Stellenbosch University, Stellenbosch, South Africa
Freshwater habitats worldwide are experiencing many threats from environmental and anthropogenic sources, affecting biodiversity and ecosystem functioning. In Africa, particularly in Mediterranean climate zones, rapid human population growth is predicted to have great impact on natural habitats besides naturally occurring events such as unpredictable drought frequency and severity. Here, we analyze the potential correlation between odonate assemblage conservation priority (measured with the Dragonfly Biotic Index: DBI) and the magnitude of climate change and human perturbation in African regions with a dominant Mediterranean climate, namely Northern (NAR: Morocco, Algeria and Tunisia) and Southern African region (SAR: South Africa). Using a compilation of studies assessing odonate assemblages in lotic and lentic habitats of both regions (295 sites in NAR and 151 sites in SAR), we estimated DBI, temporal change in average annual temperature (T), annual precipitation (P), and human footprint index (HFI) in each site, then we tested whether sites with different levels of DBI were associated with different magnitudes of climatic and anthropogenic change. We estimated past (between 1980–1999 and 2000–2018) and future changes (between 1980–1999 and 2081–2100) in T and P based on three CMIP6 scenarios representing low (SSP126), moderate (SSP245), and high emission (SSP585), as well as the change in HFI from 1993 to 2009. We found that assemblages with higher DBI (i.e. higher conservation priority) encountered lower increase in T and slightly greater decrease in P than assemblages with lower DBI (i.e. lower conservation priority) in NAR during 1980–2018, but are projected to experience higher increase in T and lower decrease in P in future projections for 2081–2100. In SAR, the increase in T was mostly similar across assemblages but the decline in P was higher for assemblages with higher DBI during 1980–2018 and 2081–2100, suggesting that assemblages of higher conservation priority in SAR are threatened by drought. While HFI showed an overall increase in NAR but not in SAR, its temporal change showed only minor differences across assemblages with different DBI levels. We discuss the importance of management plans to mitigate the effects of climatic and anthropogenic threats, so improving conservation of odonate assemblages in these regions.
Introduction
Africa’s freshwater systems support highly diverse and endemic biota (Abell et al., 2008; Holland et al., 2012), and offer crucial ecological and socioeconomic services (Adekola et al., 2015; Wangai et al., 2016). African freshwater ecosystems have been, and continue to be, subject to many naturally- and anthropogenically-induced stress factors (Darwall et al., 2011). Firstly, climate change is expected to have a great impact on African natural ecosystems, due to increasing temperature and increased variability in the spatial distribution of rainfall (Faramarzi et al., 2013). Secondly, human population growth is the highest in the world, with an estimated growth from 1.3 billion today to 5.7 billion by 2100 (Gerland et al., 2014). Inevitably, this will put greater anthropogenic pressure on freshwater ecosystems from increased agricultural activity, industrial development, and rapidly emerging urbanization. All these pressures contribute to pollution, landscape fragmentation, habitat loss and modification (Hansen et al., 2013; Brandt et al., 2017).
Africa has a variety of climates spanning from extremely hot and dry deserts to tropical rainforest. The Mediterranean climate is restricted to parts of the Northern and Southern African regions, where summers are hot and dry, winters are wet and cool, and overall climatic conditions fluctuate regularly (Lionello et al., 2006). Mediterranean climate regions have witnessed one of the most severe warming events in the world, and increases in the severity and longevity of droughts (Molina et al., 2020), threatening their sensitive endemic fauna and flora (Myers et al., 2000). Even though Mediterranean species are adapted to climatic fluctuations, climate change scenarios projected for the next decades will pose serious challenges to local wildlife (Bucchignani et al., 2018), particularly to freshwater organisms (Bucak et al., 2017).
Effective conservation of freshwater biodiversity requires in-depth knowledge of the composition, distribution and abundance of communities as well as their sensitivity to various abiotic, biotic, and anthropogenic factors (Kietzka et al., 2019). Since the monitoring of entire ecosystems is challenging due to limitations in time, funds, expertise, and labor, it is strategic to use biological indicator species which reflect the overall health of the ecosystem (Stephenson et al., 2017; Stephenson et al., 2020). Odonates have been identified as valuable barometers of environmental changes in freshwater ecosystems (Córdoba-Aguilar, 2008; Hassall, 2015). Their ecological and biological ties to freshwater bodies is a key feature, as changes in the abiotic (temperature, salinity, nutrient levels, substrate composition) and biotic (vegetation structure and diversity, predation, competition, parasitism, cannibalism, and disease) parameters of the water are often reflected in their phenotype, behavior, distribution, and demography (Stoks and Córdoba-Aguilar, 2012). Male odonates are generally conspicuous morphologically, in terms of size and coloration, and behaviorally, through strong flight, and thus easy to detect by surveyors (Dijkstra and Clausnitzer, 2014). The fact that odonates are ectotherms means that their activity and vital biological processes are very sensitive to changes in temperature (Deutsch et al., 2008), which makes them reliable sentinels of climate change impacts on freshwater ecosystems (Hickling et al., 2005; Hassall et al., 2007).
Odonates of the Mediterranean climates in the Northern African region (Morocco, Algeria, and Tunisia) (NAR) and the Southern African region (South Africa) (SAR) have been well documented (Samraoui and Corbet, 2000; Riservato et al., 2009; Simaika and Samways, 2009a; Simaika and Samways, 2009b; Boudot and De Knijf, 2012; Hamzaoui et al., 2015; Korbaa et al., 2018; Khelifa, 2019). These two regions, at opposite ends of Africa, have different dragonfly faunas, and interestingly, host a wide range of freshwater habitats that experience different environmental and anthropogenic pressures (Darwall et al., 2009; García et al., 2010). Importantly however, both NAR and SAR are particularly prone to more frequent droughts in the future (Carrão et al., 2016) which will probably affect the distribution and abundance of freshwater biodiversity. In extreme cases, this could lead to local extinctions. Studies have already documented the impact of recent drought events on the distribution of threatened odonates in the two regions (Deacon et al., 2019; Samways et al., 2020; Khelifa et al., 2021a), sounding the alarm for urgent management plans.
Here, we analyze the potential correlation between the sensitivity of odonate assemblages to extinction, and the climatic and anthropogenic changes in NAR and SAR. The objectives were to: 1) comparatively assess the threats that species face in both regions; 2) evaluate the magnitude of change in temperature, precipitation, and drought between 1980 and 2018, as well as the projected change in the future (2081–2100) under three scenarios (Shared Socio-economic Pathways 126, 245, and 585) of CMIP6 climate model; 3) quantify the temporal change in human influence using human footprint index (HFI) of 1993 and 2009, and 4) assess how temporal variation in climate and HFI are correlated with assemblages of different levels of conservation priority. We finally suggest potential solutions to manage odonates and their habitats in NAR and SAR, with the ultimate aim to safeguard and manage freshwater ecosystems in general.
Materials and Methods
Study Area
The climate of the Mediterranean regions of NAR (Algeria, Tunisia, and Morocco) and SAR (South Africa) show similarities, although NAR is relatively hotter and drier than SAR (Figure 1). Both regions have in common hot, dry summers (Köppen-Geiger CSa) (Beck et al., 2018) and mild, wet winters. We followed the biome characterization of Thieme et al. (2005) to determine the Mediterranean region in NAR and SAR, and obtained the contours from ecoregions2017.appspot.com. The area of the Mediterranean region in NAR (0.67 M km2) is larger than that of SAR (0.11 M km2).
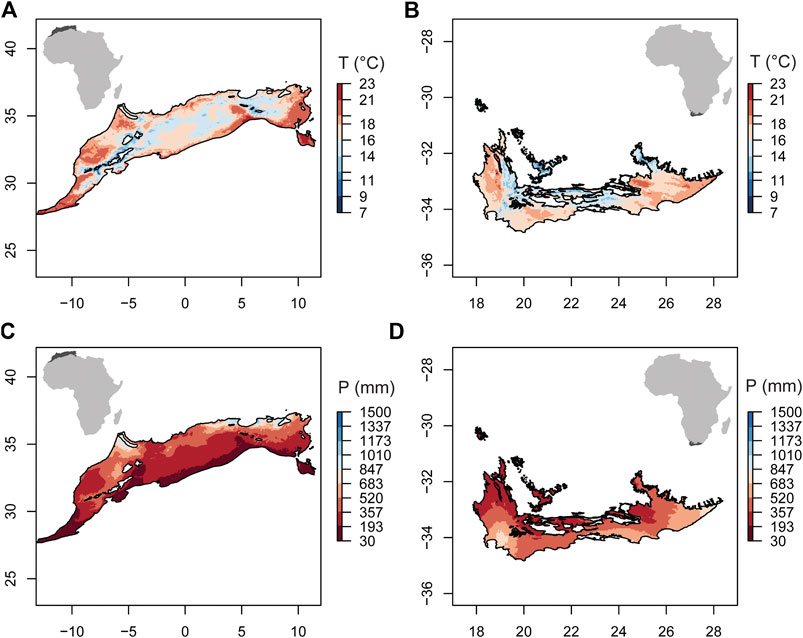
FIGURE 1. Annual average temperature (T) and annual precipitation (P) in the Mediterranean climate of the North African (NAR) and South African region (SAR). (A) T in NAR; (B) T in SAR. (C) P in NAR; and (D) P in SAR. Data represent an average across 39 years (1980–2018) and were obtained from WorldClim v2 (https://www.worldclim.org/data/worldclim21.html).
Environmental Characterization of the Study Area
To determine the regional climate conditions in NAR and SAR, we used the annual average temperature (T) and annual precipitation (P) averaged across 1980–2018 obtained from WorldClim v2 with 2.5-min spatial resolution (∼4.5 km at the equator) (Fick and Hijmans, 2017). To estimate recent climatic changes in NAR and SAR, we calculated the average annual temperature from the monthly temperature data for 1980–2018 (Harris et al., 2014). We used one-month values of the Standardized Precipitation Evapotranspiration Index (SPEI) to assess the temporal trend of drought in NAR and SAR during 1980–2018. These data were obtained from the global SPEI database (Vicente-Serrano and Staff, 2015). We specifically calculated the number of values lower than −1.5, which indicates severe drought, then analyzed the temporal trend of the frequency of these values to determine potential change in drought severity. To understand the temporal change in the human influence in NAR and SAR, we used the global human footprint index (HFI) for 1993 and 2009 (the only available years in the dataset) (Venter et al., 2016b; Venter et al., 2016a). This index has a spatial resolution of 1 km2 and was developed using eight types of human impacts, namely built environments, population density, electric infrastructure, croplands, pasture lands, roads, railways, and navigable waterways. HFI values range from 0 to 50 where higher values indicate higher human influence. It is important to note that here we treat climate change and human influence separately even though human activity influences climate. We refer to human influence as the direct impact of anthropogenic activity on the physical (non-climatic), chemical, and biological characteristics of the environment. To assess the change in HFI in aquatic systems, we calculated the average HFI using a 5 km-buffer around major lakes of NAR and SAR obtained from hydrosheds.org (Messager et al., 2016).
Characterization of the Threats and Conservation Priority of Odonate Assemblages
Odonates of NAR and SAR have been studied in some depth throughout the last few decades. To characterize the odonate assemblages that occupy the Mediterranean regions of NAR and SAR, we compiled a list of studies (from 2000 to present) that document the lotic and lentic odonates in NAR (Supplementary Table 1), and used the long-term monitoring data reported in Simaika and Samways (2009a) for SAR. Vagrants, such as Tramea basilaris in NAR, and Macrodiplax cora in SAR, were not included in our assessment.
To determine the major threats facing the odonate assemblages in the NAR and SAR, we used the nine threat categories of the IUCN Red List for local species: 1) residential and commercial development, 2) agriculture and aquaculture, 3) pollution, 4) natural system modifications, 5) climate change and severe weather, 6) human intrusions and disturbance, 7) energy production and mining, 8) biological resource use, and 9) invasive and other problematic species, genes and diseases (more details in Supplementary Table 2). Some widespread species did not have a particular response to environmental change, at least based on the currently available observations, and were categorized as “undetermined.” Based on expert knowledge alongside some previously-collected data (especially the impact of invasive alien trees in SAR), each species was assigned to one or multiple threat categories, depending on the observed sensitivities of wild populations. For example, Syncordulia legator in SAR is sensitive to invasive plants and natural system modifications, whereas Calopteryx exul in NAR is sensitive to agriculture and aquaculture, natural system modification, residential and commercial development, pollution, and climate change and severe weather. We then created a contingency table with all species and all threats for both NAR and SAR to 1) determine the most frequent threats for each region, and 2) test for potential differences between the two regions.
To determine the conservation priority of odonate assemblages, we used the Dragonfly Biotic index (DBI), originally developed for odonates found in South Africa (Samways and Simaika, 2016), and adapted to the odonate species of the NAR. This index is composed of three components (sub-indices) 1) species geographic distribution, 2) International Union for the Conservation of Nature/Species Survival Commission (IUCN/SSC) Red List conservation status, and 3) sensitivity to human disturbance, based on each species’ occupancy of fully natural vs. fully artificial habitats. At the species level, each of the three components of the index has a score ranging from 0 to 3. The DBI is the sum of the three components, and thus ranges from 0 to 9. For instance, a widespread, non-threatened, and highly-tolerant species to human disturbances scores 0 (0 + 0 + 0), whereas a localized endemic, highly threatened, and particularly sensitive species to anthropogenic pressure scores 9 (3 + 3 + 3). At the assemblage level, the sum of the DBI of all species at a given site (DBI site score) gives a useful metric of the ecological integrity and conservation priority. To compare sites (i.e. locality sampled in every study), the DBI is divided by the total number of species at the site (mean DBI), which corrects for differences in DBI scores arising from the difference in species richness (Simaika and Samways, 2009a). We used only mean DBI in our analysis of the conservation priority of assemblages in NAR and SAR.
Estimating Changes in Climatic and Anthropogenic Factors
To assess temporal patterns of climatic variables, we carried out two types of analyses. First, a linear regression of T and P across years (1980–2018) was fitted in all grid cells of the NAR and SAR. Second, to compare the past changes and future climate projections which are available on bidecadal periods in WorldClim v2, we calculate the average T and P for 1980–1999, 2000–2018, and 2081–2100, then used 1980–1999 as baseline period to measure the recent past change by subtracting T and P of 2000–2018 from T and P of 1980–1999 (past ΔT and ΔP), and the future change by subtracting T and P of 2081–2100 from T and P of 1980–1999 (future ΔT and ΔP). For future climate change, we used three scenarios corresponding to the shared socio-economic pathways (SSP) 126 (future change—scenario 1), 245 (future change - scenario 2), and 585 (future change—scenario 3) of the CMIP6 future climate projections (global climate models BCC-CSM2-MR) (Fick and Hijmans, 2017). These scenarios reflect Representative Concentration Pathways (RCPs) examining different possible future greenhouse gas emissions. RCP2.6 (low emission) scenario aims to limit the radiative forcing to 2.6 W m−2 at the end of the century. RCP4.5 (moderate emission) aims to stabilize radiative forcing at 4.5 W m−2 in the year 2100. In RCP8.5 (high emission), the greenhouse gas emissions and concentrations increase substantially over time, reaching a radiative forcing of 8.5 W m−2 at the end of the century.
To test whether odonate assemblages with different conservation priorities are associated with different magnitudes of changes in local climate, we tested the difference in T and P across two periods [past (1980–2018) and future (1980-2100) with three scenarios (SSP126, 254, and 585)] across three levels of mean DBI [low (0–1.5), intermediate (1.5–3.0), and high (>3.0)]. Similar analyses were carried out to determine whether odonate assemblages with different conservation priorities are associated with different extents of change in HFI between 1993 and 2009.
Statistical Analyses
All analyses were carried out using R 4.0.2 (R Development Core Team, 2020). The “raster” package (Hijmans et al., 2015) was used to read and analyze climate (WorldClim v2) and HFI data. We used a chi-squared test to determine whether there is a difference between regions in the frequency distribution of species across threats (species with undetermined threats were excluded). We used the calc function from the “raster” package to fit linear regressions of T and P across years (1980–2018) for each grid cell of the raster. Then we extracted the slope estimate and p-value. We assessed the temporal pattern of drought during 1980–2018 by calculating the number of grid cells with SPEI value <−1.5 every year for both NAR and SAR, and fitting a negative binomial regression of the number of grid cells against time (one-month interval for 39 years) using the “MASS” package (Venables and Ripley, 2002). We tested for potential difference between the mean DBI of NAR and SAR using a Wilcoxon test. To test differences in ΔT and ΔP across different levels of DBI (low, intermediate, and high) and period (past, future SSP126, 245, and 585), we used generalized least squares regression using the function gls with the package “nlme” (Pinheiro et al., 2020) which accounts for spatial autocorrelation. We selected the best spatial structure by comparing the AIC of models fitted with five different classes: spherical, exponential, Gaussian, linear, and rational quadratics, and choosing the one with the lowest AIC. Similarly, we tested for potential differences in the change in HFI among odonate assemblages with varying degrees of DBI by testing the effect of the interaction between DBI (low, medium and high) and period (1993 and 2009) on HFI using gls function and accounting for spatial autocorrelation. We evaluated model fit using R2 values calculated using the rsquared function from the piecewisesem package (Grueber et al., 2011).
Results
Threats to Odonate Assemblages
Our dataset included a total of 53 species (31 dragonflies and 22 damselflies) in NAR and 70 species in SAR (41 dragonflies and 29 damselflies). There was a significant difference in the type of threats that odonate assemblages in NAR and SAR encounter (χ2 = 34.0, df = 7, p < 0.0001) (Figure 2). Odonates in NAR are mostly threatened by pollution (56.6%), natural system modifications (37.7%), agriculture and aquaculture (37.7%), and climate change and/or severe weather (32.1%). Species in SAR are mostly threatened by agriculture and aquaculture (40.0%) and natural system modifications (34.3%), but also pollution (28.6%). Species with undetermined threats had a higher proportion in SAR (51.4%) than NAR (32.1%). While 14.3% of species were threatened by invasive species in SAR, only 3.8% of species in NAR showed sensitivity to this threat. In South Africa in particular, all species listed as Threatened or Near Threatened have the same primary threat: invasive alien trees shading out their natural habitat, which is the case in both rural and urban areas.
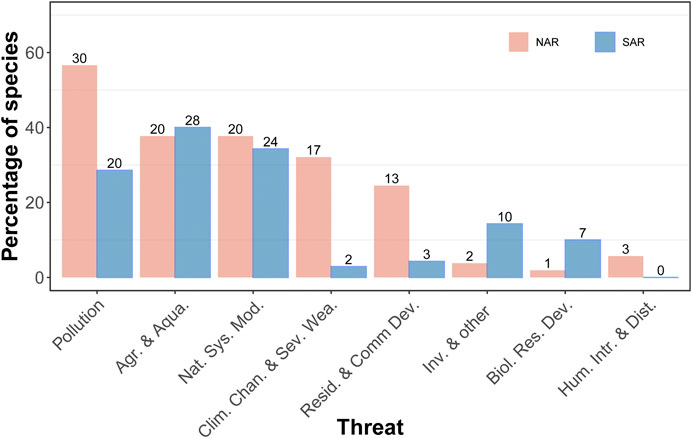
FIGURE 2. Percentage of odonate species threatened by nine different threats in the Mediterranean climate of Northern and Southern African region. The percentage was calculated with respect to the total number of species recorded for each region. Numbers on the bars are the number of species.
Human Influence
As the major threats were anthropogenic, we used Human Footprint Index (HFI: a metric indicating human influence) to determine the change in spatial distribution and magnitude of human influence between 1993 and 2009 (Figure 3). The overall HFI in NAR increased from 1993 to 2009 (gls: p < 0.0001), with the median increasing by 21.2% and the 90% percentile increasing by 25.5%. In SAR, there was no significant change in HFI between 1993 and 2009 (gls: p = 0.35), with the median remaining stable (0.26% decrease) and the 90% percentile decreasing by 4.7%. We found that the areas of low HFI (<20) decreased by 3.9% in NAR but increased by 0.13% in SAR, although this increase was not significant. The area of high HFI (>20) increased by 99% in NAR but declined by 2.6% in SAR. We further calculated the average HFI around 292 major lakes in NAR and 193 major lakes in SAR (using a 5 km-buffer) and found that the HFI increased in NAR (p < 0.0001) but did not change in SAR (p = 0.30) (Supplementary Figure 1).
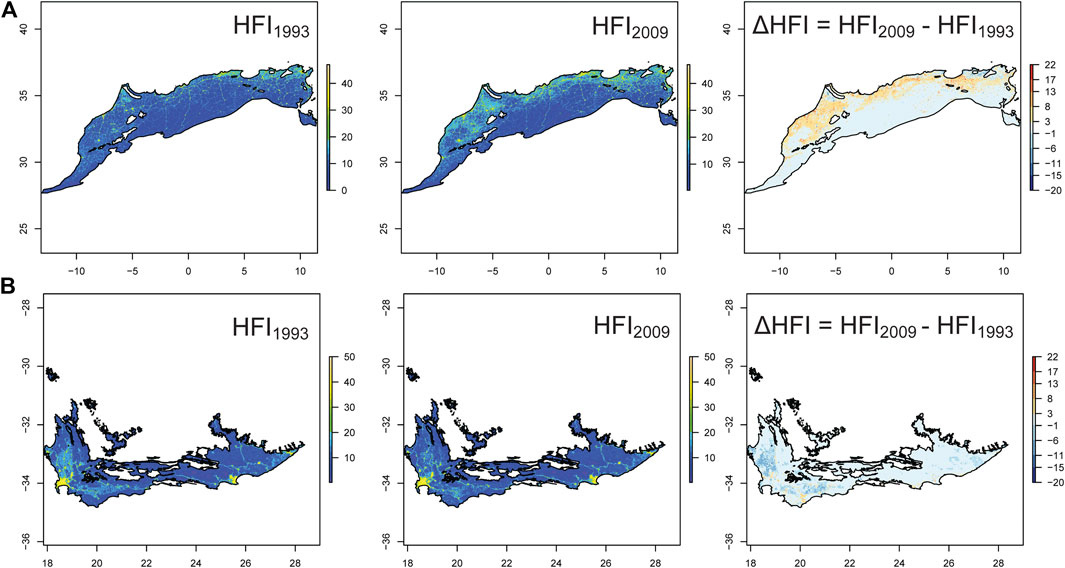
FIGURE 3. Change in Human Footprint Index (HFI) in the Mediterranean climate of North (NAR) (A) and South (SAR) (B) African regions between 1993 and 2009. The third panel shows the relative change in HFI by subtracting HFI of 2009 from HFI of 1993. HFI ranges from 0 (no human influence) to 50 (very high human influence).
Climate Change
Both average annual temperature (T) and annual precipitation (P) showed spatial variation in the temporal trends between 1980 and 2018 in both regions, but T exhibited clearer trends than P (Supplementary Figure 2). The average ± SD of the temporal slopes for T was 0.026 ± 0.005°C·yr−1 (range = 0.01─0.03°C·yr−1) in NAR, and 0.023 ± 0.002°C·yr−1 (0.02─0.03°C·yr−1) in SAR (Supplementary Figures 2A,B). In NAR, T increased from 16.9 ± 2.1°C (7.6─22.6°C) in 1980–1999 to 17.4 ± 2.13°C (8.2─23.1°C) in 2000–2018 (Figure 4A), and is projected to increase in 2081–2100 (Figure 4B) to 18.3 ± 2.0°C (9.1─24.1°C), 19.6 ± 2.0°C (10.5 ─25.4°C), 21.9 ± 1.9°C (13.0─27.8°C) for SSP126, SSP245, and SSP585, respectively. In SAR, T increased from 16.5 ± 1.7°C (10.5─19.6°C) in 1980–1999 to 17.0 ± 1.7°C (11.0─20.1°C) in 2000–2018 (Figure 4C), and is projected to increase in 2081–2100 (Figure 4D) to 17.6 ± 1.7°C (11.7─20.8°C), 18.4 ± 1.6°C (12.4─21.6°C), 19.8 ± 1.6°C (13.8─23.2°C) for SSP126, SSP245, and SSP585, respectively.
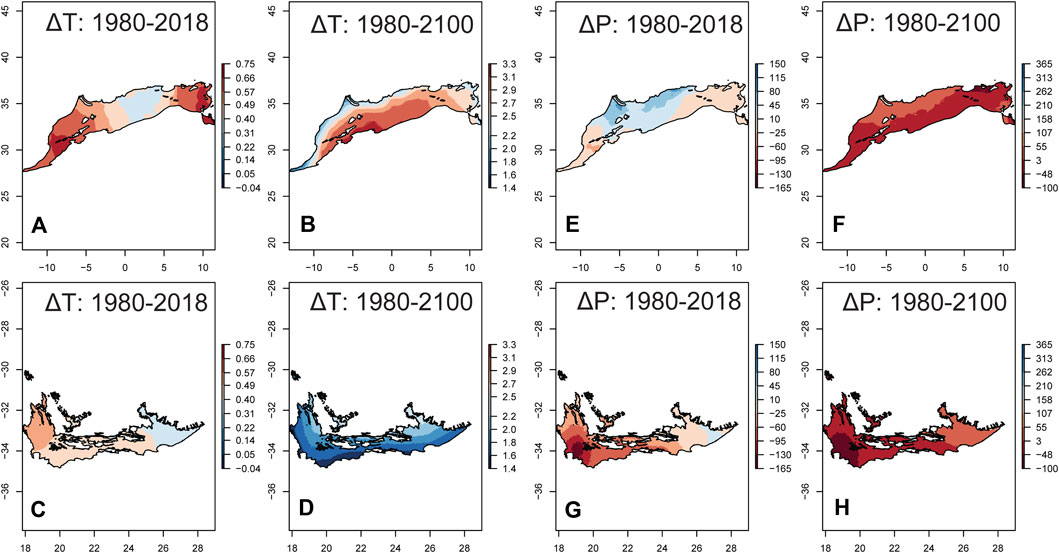
FIGURE 4. Temporal change in the average annual temperature (ΔT) and annual precipitation (ΔP) in the Mediterranean climate of North African (NAR) and South African regions (SAR). We used 1980–1999 as a reference period to calculate ΔT and ΔP. Temporal changes for 1980–2018 was calculated by subtracting the average for 2000–2018 from that of 1980–1999. ΔT and ΔP for 1980–2100 was calculated by subtracting the average for 2081–2100 (SSP 245) from that of 1980–2018. (A) ΔT 1980–2018 in NAR; (B) ΔT 1980–2100 in NAR; (C) ΔT 1980–2018 in SAR; (D) ΔT 1980–2100 in SAR; (E) ΔP 1980–2018 in NAR; (F) ΔP 1980–2100 in NAR; (G) ΔP 1980–2018 in SAR; (H) ΔP 1980–2100 in SAR.
The temporal variation for P showed larger percentage areas with significant trends in SAR (57.9%) than NAR (9.8%), and stronger magnitudes of decline in SAR compared to NAR (Supplementary Figures 2C,D). In NAR however, there were more positive (78.5%) than negative (21.5%) significant trends. Average ± SD of the temporal slopes of P across the entire region was 1.01 ± 1.20 mm yr−1 (−2.38─6.66) in NAR, and −2.46 ± 1.63 mm yr−1 (−7.33─−0.02) in SAR. In NAR, P slightly increased from 331.6 ± 170 mm (31–1480) in 1980–1999 to 348.5 ± 181.0 mm (33.7–1481) in 2000–2018 (Figure 4E) but is projected to decrease in 2081–2100 (Figure 4F) to 329 ± 167 mm (29-1422), 330 ± 171 mm (29–1388), 290 ± 144 mm (28–1239) for SPP126, SPP245, and SPP585 scenarios, respectively. In SAR, P decreased from 447.2 ± 141.8 mm (191–902) in 1980–2000 to 399 ± 136 mm (157–894) in 2001–2018 (Figure 4G), but is projected to increase in 2081–2100 (Figure 4H) to 437.1 ± 141 mm (182–938), 431 ± 143 mm (178–969), and 416.5 ± 143.2 mm (166–973) for SSP126, SSP245, and SSP585, respectively. To determine whether there was a trend in drought severity between 1980 and 2018, we analyzed the temporal trend of monthly SPEI values <−1.5 (indicating severe drought). We found that the frequency of these values (number of grid cells <−1.5) increased in NAR (GLM: z = 5.14, p = 0.003) and SAR (GLM: z = 1.00, p = 0.0003), but it was higher in NAR across the entire period (Supplementary Figure 3).
Relating Conservation Priority to Environmental Change
A total of 295 sites in NAR and 151 sites in SAR were used in the analysis of DBI values. The mean DBI values of NAR and SAR were significantly different (Wilcoxon: p < 0.0001), with SAR showing higher values (Figures 5A,B). In localities where odonate assemblages were surveyed, T showed an overall increase during 1980–2018, and is projected to increase further in 2081–2100 (Period, gls: p < 0.0001) under the three scenarios SSP126, SSP245 and SSP585 in both NAR and SAR (Table 1; Figure 5C). Interestingly, the change in T (ΔT) in NAR during 1980–2018 was lower in assemblages with higher DBI values (DBI, p < 0.0001), but in all three scenarios of future projection for 2081–2100, ΔT was higher in assemblages with higher DBI values (Period×DBI, p < 0.0001) (Table 1; Figure 5C). In SAR, ΔT increased during 1980–2018, and is projected to increased more in 2081–2100 under all scenarios (Period, gls: p < 0.0001). However, ΔT was similar across assemblages during 1980–2018 and 2081–2100 (DBI, p > 0.36; Period × DBI, p > 0.08), except in the intermediate priority assemblage in future scenario SSP585 where ΔT was higher than low and high priority assemblages (Period×DBI, p = 0.02) (Table 1; Figure 5D).
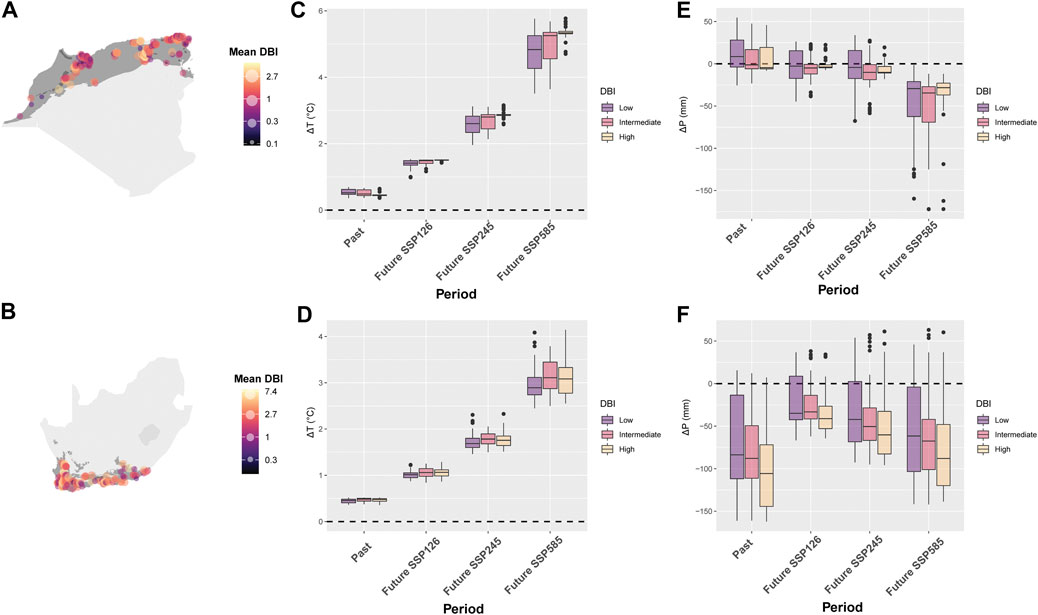
FIGURE 5. Change in average temperature (ΔT) and annual precipitation (ΔP) for odonate assemblages with low, intermediate, and high mean Dragonfly Biotic Index (DBI) in the Mediterranean climate of the North African Region (NAR) and South African Region (SAR). (A) mean DBI in all sites of NAR. (B) mean DBI in all sites of SAR. (C) ΔT in NAR. (D) ΔT in SAR. (E) ΔP in NAR. (F) ΔP in SAR. The period 1980–1999 is used as a reference period to calculate past (difference between 2000–2018 and 1980–1999) and future (difference between 2081–2100 and 1980–1999) change in climatic conditions. Three scenarios were used for the future projection of climate: SSP 126, 145, and 585.
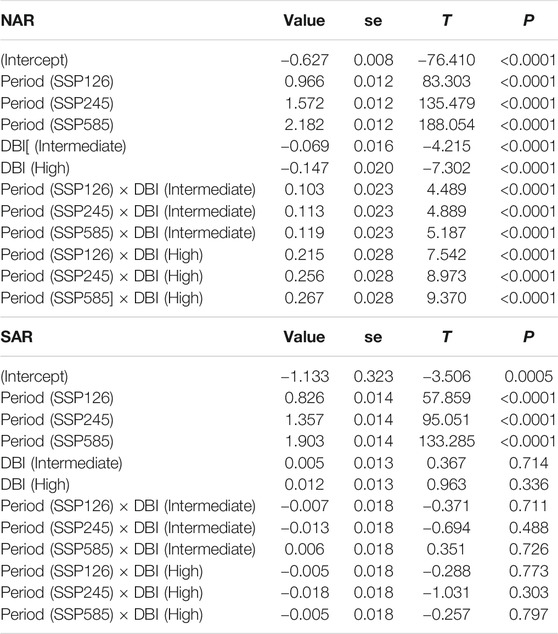
TABLE 1. Summary statistics of the generalized least squares model assessing the difference in the change in average annual temperature across past and future periods in the surveyed localities of odonate assemblages with varying DBI levels in North African and South African regions. Past period and low DBI are used as baseline levels. R-squared was 0.98 in NAR and 0.97 in SAR.
P showed no major pattern in surveyed localities in NAR during 1980–2018 [Intercept (ΔP 1980–2018), p = 0.27), but is projected to experience a severe decline in 2081–2100 in all future scenarios of climate change (Period, p < 0.0001) (Table 2; Figure 5E). Odonate assemblages with high DBI values were exposed to relatively drier conditions than low and intermediate levels of DBI [DBI (high), p = 0.02] during 1980–2018 in NAR (Table 2; Figure 5E). However, odonate assemblages with high DBI values are projected to experience slightly lower drought conditions than those with low and intermediate DBI values in the future, particularly for scenarios SSP126 [Period (SSP126) × DBI (high), p = 0.02] and SSP585 [Period (SSP585) × DBI (high), p = 0.0002]. In SAR, odonate assemblages with high DBI values experienced higher magnitude of ΔP in all periods [DBI (high), p = 0.003] (Table 2; Figure 5F) with stronger magnitudes in 1980–2018 than 2081–2100. Smallest ΔP was recorded in future scenario SSP126.
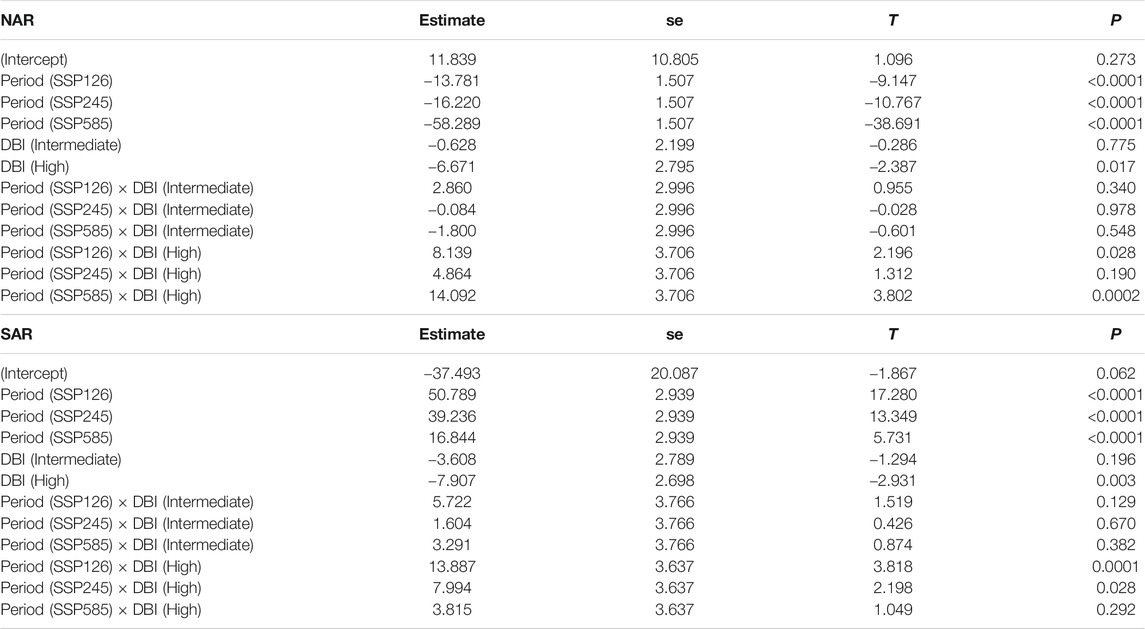
TABLE 2. Summary statistics of the generalized least squares model assessing the difference in the change in annual precipitation across past and future periods in the surveyed localities of odonate assemblages with varying DBI levels in North African and South African regions. Past period and low DBI are used as baseline levels. R-squared was 0.43 in NAR and 0.21 in SAR.
Our models testing for the temporal variation in HFI and its association with DBI had very low predictive power (Table 3), suggesting that most of the variation was not explained by DBI and year. In NAR, HFI slightly increased from 1993 to 2009 across assemblages with different values of DBI (gls: year, p < 0.0001), but low priority assemblages experienced slightly higher increase than intermediate [year × DBI (intermediate), p = 0.04] and high priority assemblages [gls: year × DBI (high), p = 0.03] (Table 3; Figure 6A). In SAR, there was no major difference in HFI across assemblages, although high priority assemblages showed slightly lower HFI [gls: DBI (high), p = 0.05]. We also found no significant change in HFI from 1993 to 2009, except in high priority assemblages where HFI slightly increased [gls: year × DBI (high), p = 0.03] (Table 3; Figure 6B).
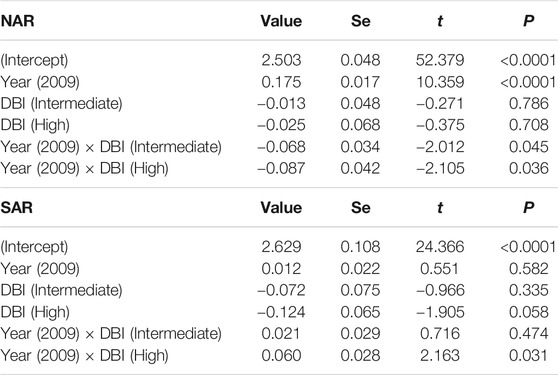
TABLE 3. Summary statistics of the generalized least squares model assessing the difference in the human footprint index (HFI) between 1993 and 2009 in the surveyed localities of odonate assemblages with varying DBI levels in North African and South African regions. Year 1993 and low DBI are used as baseline levels. R-squared was 0.02 in NAR and 0.01 in SAR. To meet the model assumptions, HFI was log transformed.
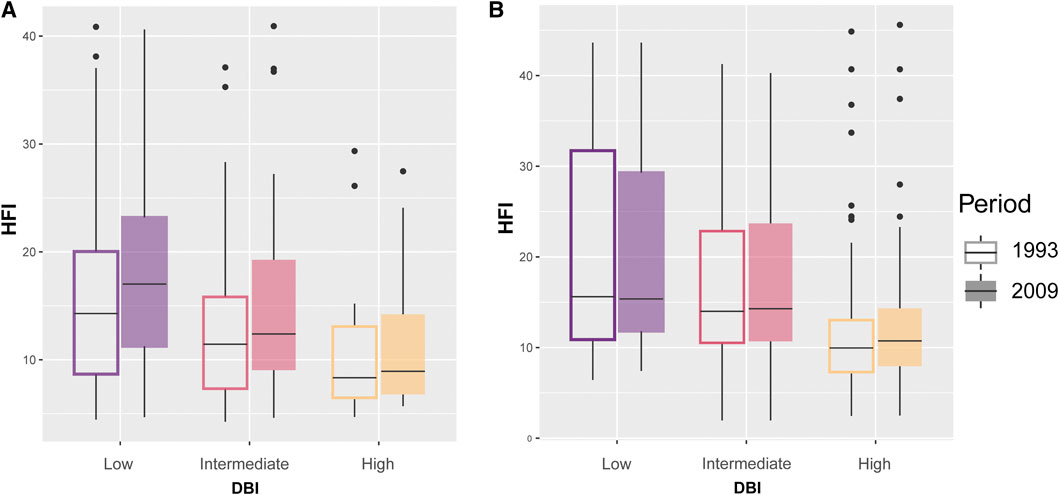
FIGURE 6. Change in human footprint index (HFI) for odonate assemblages with low, intermediate, and high mean Dragonfly Biotic Index (DBI) in the Mediterranean climate of (A) the North African region (NAR) and (B) South African region (SAR) between 1993 and 2009.
Discussion
We carried out an analysis of the environmental and anthropogenic threats to the odonates in the Mediterranean regions of northern (NAR) and southern Africa (SAR). We specifically looked at whether assemblages with different levels of conservation priority have encountered (years 1980–2018), and will experience (years 2081–2100), different magnitudes of climate change and increase in human influence. High DBI assemblages (i.e. odonates of high conservation priority) compared to low and intermediate DBI assemblages were less affected by increasing temperature in NAR but more affected by decreasing precipitation in SAR. Future climatic projections show that assemblages with high DBI values compared to other assemblages will be affected principally by increasing temperature in NAR, but affected more by decreasing precipitation in SAR. Odonate assemblages with lower DBI values experienced slightly greater increases in human influence than those with high DBI in NAR, but human influence did not change much in SAR across all assemblages.
Climate Change
Our results showed that NAR and SAR have experienced different magnitudes of climatic change with stronger warming in NAR, and more severe hydrological drought in SAR. However, future projections of climate revealed that temperature will continue to increase in NAR whereas precipitation will decrease in both NAR and SAR. These differences in climatic change suggest that odonate assemblages in NAR and SAR were and will be impacted differently by abiotic pressures. Although Mediterranean species are adapted to fluctuating climate, it is unclear how warming affects their thermal safety margin and extinction probability (Paaijmans et al., 2013). Increased temperatures might affect the life history of organisms by speeding up development rate and changing phenology and voltinism (Hassall et al., 2007; Braune et al., 2008; Richter et al., 2008), as well as their body size (Tseng et al., 2018). These changes typically apply to the entire freshwater community, and thus might impact the ecological interactions in the food web (Ledger et al., 2013; O’Gorman et al., 2019). Drought is also problematic, as it may lead to extinctions during times of prolonged water scarcity (Rocha-Ortega et al., 2020), such as the ones projected for SAR and parts of NAR.
Odonate assemblages with different conservation priorities showed different exposure to climate change in recent past decades. Assemblages with high conservation priorities experienced lower warming in NAR but higher warming in SAR than assemblages with low conservation priorities during 1980–2018. Precipitation in areas harboring high priority assemblages did not change much in NAR, but experienced stronger decline in precipitation than other assemblages in SAR. This could be detrimental for the most sensitive odonate assemblages (e.g. Chlorolestes apricans and Syncordulia venator) which typically have highly specific habitats of very limited extent. Some SAR species are well adapted to drought conditions (Jooste et al., 2020). Many odonates in SAR, even some Cape endemics, may also occupy artificial reservoirs as short-term habitats for adults to pull through periods of extended drought (Deacon et al., 2019). However, extensive and prolonged droughts might push these odonates beyond their adaptation limits. Other local sensitive species rely on perennial streams and rivers to reproduce, and thus are less ecologically flexible in case of changes in hydrological conditions. Even in NAR which is apparently less prone to changes in annual precipitation, recent drought has led to the extirpation of endemic lotic species which are dependent on flowing water to reproduce (Khelifa et al., 2021a).
Future projections of climate change predict that areas where high-conservation priority odonate assemblages live will encounter greater warming in both NAR and SAR, but more so in NAR compared to SAR. The higher increase in temperature for the most sensitive assemblages, particularly in SSP585, will push the currently extreme thermal conditions to the highest levels of recent history which might exceed the current critical tolerable maximum of some species (Deutsch et al., 2008).
Future projections of precipitation predict that all assemblages will encounter relatively similar drought conditions, but the magnitudes will be much greater than those recorded in the last few decades in NAR compared to SAR. This suggests that the environmental conditions which are currently posing challenges to the most sensitive species in both NAR and SAR will become even more pronounced in the future, especially those already threatened. These expected climatic changes might cause major hydrological perturbations that increase the likelihood that perennial habitats become temporary habitats or even vanish completely. Hence, there is a high risk of extinction for the most threatened and endemic species in NAR and SAR as they strictly inhabit perennial lotic habitats.
There are two major challenges related to these projected thermal and hydrological changes. First, a large fraction of the Mediterranean climate in Africa is expected to shift to more desert-like climate by the end of the century (Beck et al., 2018) which potentially changes the landscape and various ecological processes. Second, wetlands in African Mediterranean-type climate are not only relatively rare but they are projected to encounter a significant habitat loss in the next decades (Xi et al., 2021). Together with the natural ecological barriers (e.g. deserts, ocean and mountains), these changes will reduce opportunities for species to find potential refuges to escape adverse climatic stressors, particularly for species with a specialized ecological niche or limited dispersal ability. For example, the distribution of North African odonates is restricted by the Mediterranean Sea in the north, and the vast Sahara desert in the south. Therefore, unlike the typical dispersal opportunity that populations at leading or trailing edges have to move northwards (northern hemisphere) or southwards (southern hemisphere) to escape climate warming (Hickling et al., 2005; Angert et al., 2011; Wiens, 2016; Gilbert et al., 2020), many species, particularly those of high-conservation priority, will have to adapt to the novel yet severe environmental conditions. Thus, much of the local Mediterranean odonate diversity is at great risk of extinction should appropriate management measures not be taken.
For the NAR, because elevation range shifts are expected to happen as a response to climate warming, it is crucial to assess the future habitat suitability at high elevation sites, especially for endemic species such as C. exul, G. lucasii, and Onychgomphus boudoti (Khelifa, 2013; Ferreira et al., 2014; Zebsa et al., 2015), which have restricted distributions and specific habitat requirements (Figures 7A–C). It is likely that the currently colder high elevation areas, even after warming, will not provide the optimal conditions for species with specialized niches and habitats (e.g. terrestrial habitat characteristics, physiochemical parameters of the water, benthic habitat structure, predator and prey communities). In such cases, it is strategic to anticipate potential habitat improvements should the species’ ecological niche is no longer be available, or in worst cases, undertake translocation of some of the remaining individuals to other suitable watersheds.
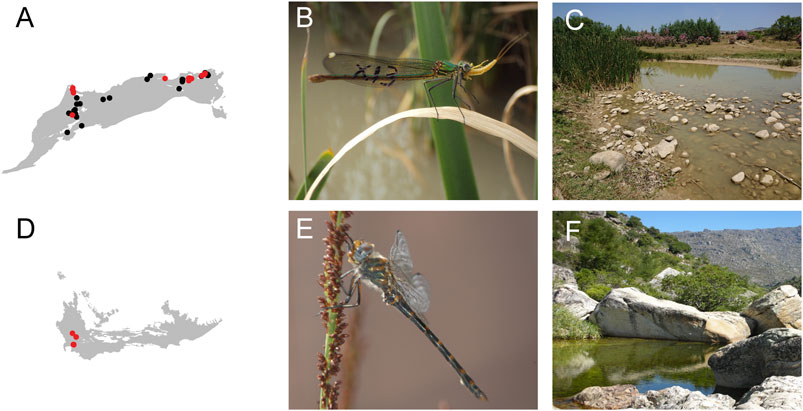
FIGURE 7. Distribution and habitat characteristics of a North and a South African endemic odonate. (A) Geographic distribution of Calopteryx exul in North Africa. (B) Female adult C. exul eating a mayfly. (C) Typical habitat of C. exul. The picture indicates the habitat of the species in a dry year (2018) where the floating leaves for oviposition become rare due to drought. (D) Geographic distribution of one of the world’s rarest dragonflies, Syncordulia serendipator. (E)S. serendipator male. (F) Typical habitat of S. serendipator. The species lives in a few open deposition pools high in the Cape Fold mountains, and highly susceptible to further climate change as it has nowhere else to go. Red and black points indicate extant and uncertain occurrence, respectively.
For the SAR, among these measures are maintenance of higher elevation streams and standing water bodies, so that functional connectivity remains, possibly enabling species to shift in elevation, a response they appear to have sown in the past (Samways and Deacon, 2021). However, two very rare and localized species, Syncordulia serendipator (Figures 7D–F) and Orthetrum rubens, which are extreme habitat specialists (the first in unusual rocky streams with open deposition pools, the second in depression pools) currently living at the highest elevations possible in terms of presence of their habitat, are at great risk of extinction.
Anthropogenic Pressure
Based on HFI, there has been increasing pressure from human activities on local freshwater ecosystems over the last few decades in NAR but not in SAR. Interestingly, high conservation priority assemblages were recorded in areas with lower degrees of human influence compared to low and intermediate priority assemblages. This suggests that the distribution of sensitive odonate species is limited to areas with low human disturbance, which have declined severely in NAR. However, it is unclear whether the low DBI, reflecting the absence of threatened species, might be due to the historical absence of threatened species or non-detection of local extinction as a consequence of high human influence. In SAR, the slow growth of human influence could be due to the higher initial level of HFI compared to NAR, which slows the expansion of land use (e.g. urbanization, agriculture...) and makes the change in human impacts more difficult to detect.
Most freshwater habitats are currently affected by adverse impacts including damming of lotic habitats, industrial pollution, increasing domestic wastewater, and excessive irrigation demands (Droogers et al., 2012; Khelifa et al., 2021a). Given that the human influence will continue in the future due to human population growth, strategic plans for future freshwater habitat conservation are essential. In SAR, in particular, impacts such as damming, pollution, domestic wastewater discharge and irrigation demands have had less effects on some odonate assemblages of SAR, as most Cape endemics occur on high mountain slopes with less urban development. Furthermore, other threats of urbanization have been offset by considerable artificial pond building and management (Samways et al., 2020), and through the removal of the key threat of invasive alien trees (see below).
Recommendations for Conservation and Management
Given the multiple naturally- and anthropogenically-induced threats that odonate assemblages face in NAR and SAR, it is crucial to develop strong recommendations and management plans to maintain and improve the conservation status of local biodiversity. Here we discuss eight critical points to improve current conservation plans and guide policy-makers.
1) Climate and anthropogenic datasets. The recent increase in the availability of global high-resolution datasets of climate and human perturbation opens more opportunities to deepen our understanding of biodiversity response to various environmental stressors and facilitate the task of management and conservation. For example, climate forecast should be used to anticipate future effects of abiotic conditions on habitats and species, preferably with information on the expected response of assemblages to temperature and drought derived from historical data. This will help identify regions that need more conservation attention and immediate actions such as those hosting endemic fauna and experiencing rapid climate change. Furthermore, we still lack understanding of the direct and indirect effects of anthropogenic pressure on the freshwater biota. Composite indices like human footprint index are useful indicators of the overall anthropogenic pressure, but we still need to compare their impact on biodiversity with individual stressors to test their predictive power. More data are needed to understand the potential interaction between anthropogenic pressure and climate change and set strategic conservation measures that involve offsetting human influence in areas where the magnitude of climate change is projected to be strong.
2) Climate change and drought. Although the focal species here are adapted to occasional dry conditions, anthropogenic impact might exacerbate the effect of drought on odonate assemblages. There also needs to be some recognition that not all biological assemblages are affected by climate change in a similar way. Nevertheless, enforcing laws that limit water pumping of lotic habitats, which is widespread in NAR, is essential, particularly during dry years. Establishing a better management plan for dam water by increasing the frequency of major dam discharges during extremely dry periods is critical for maintaining the integrity of watercourses, so ensuring the availability of reproductive habitats, and reducing extinction rates (Khelifa et al., 2021a). Providing artificial waterbodies that resemble natural habitats (e.g. vegetation cover) may also play a crucial role as refuges for threatened species during dry years or as hosts to establish new populations, so increasing odonate resilience to climate change (Suhling et al., 2017; Deacon et al., 2019).
3) Pollution. Odonates are often exposed to wide array of pollutants in their natural habitats, but the understanding of their impacts on odonates in NAR and SAR needs more attention. NAR rivers have been exposed to different heavy metals, pesticides, and other organic compounds (Guettaf et al., 2017), even at sites where endemic and threatened odonates occur (e.g. Calopteryx exul) (Khelifa et al., 2016b). In SAR, there is still limited evidence to date of a sustained effect of pollution (Das et al., 2008), but indirect effects through reduced quality and sometimes quantity of food is highly likely (Agboola et al., 2019). The purification of domestic and industrial waste water before reaching the rivers and other waterbodies could improve the water quality and ecosystem functioning substantially.
4) Farming and forestry. Most wetlands and rivers are bordered by highly transformed agricultural land with intensified farming systems that rely heavily on pesticides and fertilizers (Khelifa et al., 2021b), affecting the diversity and composition of odonate assemblages (Kietzka et al., 2018), particularly specialists. Shifting to organic farming will reduce harmful toxic run-off and fertilizer-induced eutrophication, which is a major problem in NAR. As farms are often used by odonates as foraging sites, including some threatened endemics (Zebsa et al., 2015), reducing pesticide use will greatly reduce non-target mortality and increase food availability. Increasing habitat heterogeneity on farms by leaving more native terrestrial habitats could also be a highly effective strategy for ecologically-sensitive odonates (Briggs et al., 2019; Garibaldi et al., 2020).
In many parts of north-eastern region of South Africa, implementation of large-scale networks of conservation corridors of indigenous landscapes among plantation forestry blocks is a huge and successful initiative conserving biodiversity. Odonate assemblages have benefitted greatly, as these corridors improve functional connectivity among river and pond habitats, so improving ecological resilience of dragonflies in transformed landscapes (Deacon et al., 2018; Briggs et al., 2019; Kietzka et al., 2021).
5) Increasing sampling effort. While the number of surveys has increased during the last few decades, many areas (particularly in NAR), and which potentially host new populations and species, are still unexplored. In both regions, recent explorations have led to the discovery of new species (Dijkstra et al., 2007; Ferreira et al., 2014), new populations of threatened endemics (Chelli et al., 2019) and other regionally rare species (e.g. Kipping et al., 2012; Deacon and Samways, 2017; Khelifa and Zebsa, 2018). These important findings suggest that there is great merit in additional exploration to find new odonate species, even in areas with comparatively high sampling effort (Deacon et al., 2020). The continuation of such efforts will improve the reliability of species distribution estimates and the conservation plans of threatened species in particular.
6) Invasive trees and fish. The introduction of alien organisms is a major problem that needs close attention from conservation authorities and prompt management plans (Samways, 2008; Modiba et al., 2017). In SAR, rare and endemic odonates are threatened overwhelmingly by invasive alien trees (mostly Acacia spp.) along running water courses in particular. However, the large-scale national Working for Water Program (Van Wilgen et al., 1998) has removed alien trees from along many of these water courses, resulting in many species, including several endemics and threatened species, recovering remarkably well (Samways et al., 2005; Samways and Sharratt, 2010). This highly active and positive conservation activity has even resulted in downlisting of some species on the Red List (e.g. Syncordulia spp.). In NAR, a direct impact of invasive trees on odonates has not been documented yet, but its occurrence is also likely given the presence of invasive plants in both lentic and lotic habitats (Chekchaki et al., 2020). However, the introduction of alien fish (e.g. common carp and mosquitofish) is a known major threat to NAR wetlands (Kara, 2012), with potential major direct and indirect effects on different ecological processes in the food web (Vilizzi et al., 2015). Some promising practices involving using rotenone to kill invasive fish while allowing for the recovery of macroinvertebrate communities have been applied by nature conservation authorities (e.g. CapeNature and SANParks in South Africa) (Modiba et al., 2017).
7) Increasing the number of protected areas. Legislation for freshwater protected areas is mainly focused on large vertebrates (mammals in SAR, and both mammals and birds in NAR) and plants, which to some extent are umbrella taxa for other organisms, including macroinvertebrates. In NAR, most protected wetlands are large lentic waterbodies that host many water birds. Even natural reserves where relict endangered populations occur are still affected by human pressure, particularly livestock grazing and water pumping (Khelifa et al., 2016a). Unfortunately, local North African lotic habitats that do not harbor a large number of water birds often host most endemic threatened species of odonates. The fact that populations of threatened lotic endemic species in the European Mediterranean [e.g. the Endangered Coenagrion intermedium and Boyeria cretensis in Crete (Müller, 2008; Boudot and Kalkman, 2015)] are also vanishing due to anthropogenic activities highlights the increased human influence on lotic Mediterranean species. Thus, the integration of the key lotic habitats into the list of protected areas is fundamental for the conservation of odonates, and it should involve the maintenance of the integrity of the riparian zones and the implementation of large-scale networks of conservation corridors (Briggs et al., 2019). In SAR, most sites of particular conservation concern in terms of odonate endemism fall within formally protected areas, although some areas with exceptionally high species richness are outside of formal reserves (Deacon et al., 2020). Small private nature reserves also have high value for conserving relict populations of endemic odonates.
8) Recognition of odonates as bioindicators. Macroinvertebrates in general are interesting bioindicators, providing reliable information on environmental perturbation faster than other taxa because of their shorter life cycles. Integrating macroinvertebrates into biodiversity monitoring schemes could generate new streams of information that complement data on other taxa, to compile useful long-term high-resolution multi-taxon databases. In NAR, macroinvertebrates are not used in the biodiversity monitoring of either lentic or lotic habitats. In fact, most data on macroinvertebrates are collected by students and researchers. Given the lack of funding and interest in using macroinvertebrates as bioindicators of aquatic habitat quality, it is critical to establish a strong link between researchers and a network of community scientists to monitor macroinvertebrates using modern technology (e.g. mobile applications and open databases) to collect, share, manage, and analyze data (Stephenson et al., 2017). In SAR, odonates form integral parts of the South African Scoring System (SASS), a biomonitoring tool for establishing ecological integrity of lotic habitats, which is not yet regularly applied in neighboring countries. The DBI is a highly practical and effective alternative to SASS, and has also been highly effective for prioritizing both lentic and lotic sites for conservation (Samways and Simaika, 2016), with some scope for expanding to neighboring African countries (Vorster et al., 2020).
Conclusion
The odonate assemblages in both NAR and SAR regions are exposed to multiple naturally- and anthropogenically-induced threats, which may be exacerbated by future climate change and human population growth. NAR wetlands appear to be threatened by both climatic and anthropogenic threats, whereas SAR wetlands seem to be threatened mainly by climatic factors, especially drought. Mitigation of the threats could be achieved if effective action plans are implemented, especially using DBI methodology. However, while the DBI is valuable for assessing changes in assemblage structure (through presence vs. absence of species at any one site), there is still a lack of standardized population monitoring schemes which focus on changes in population dynamics. Luckily, the well-advanced knowledge and experience in applied conservation in SAR is a goldmine for researchers and policymakers in NAR (and elsewhere) for facing common threats and to improve the conservation status of threatened species. Future collaboration between researchers of the two regions is thus advisable. However, introducing insect conservation as one of the main criteria in establishing protected areas and conservancies still remains a challenging task that requires education, awareness-raising, and public engagement and advocacy.
Data Availability Statement
The original contributions presented in the study are included in the article/Supplementary Material, further inquiries can be directed to the corresponding author.
Author Contributions
Conceptualization: RK, CD, MS, and FS; Methodology: RK, MS, FS, and JS; Formal analysis and investigation: RK and HM; Writing—original draft preparation: RK; Writing—review and editing: RK, HM, CD, JS, MS, and FS. Funding acquisition: RK, MS, and FS; Supervision: MS and FS.
Funding
The project was funded by Swiss National Science Foundation (P2ZHP2_175028). CD and MS are funded by Mondi Group.
Conflict of Interest
The authors declare that the research was conducted in the absence of any commercial or financial relationships that could be construed as a potential conflict of interest.
Publisher’s Note
All claims expressed in this article are solely those of the authors and do not necessarily represent those of their affiliated organizations, or those of the publisher, the editors and the reviewers. Any product that may be evaluated in this article, or claim that may be made by its manufacturer, is not guaranteed or endorsed by the publisher.
Acknowledgments
We thank the two reviewers for their insightful comments and suggestions which greatly improved our former version of the manuscript. We are grateful to Mohamed Khalil Mellal for data collection.
Supplementary Material
The Supplementary Material for this article can be found online at: https://www.frontiersin.org/articles/10.3389/fenvs.2021.660163/full#supplementary-material
References
Abell, R., Thieme, M. L., Revenga, C., Bryer, M., Kottelat, M., Bogutskaya, N., et al. (2008). Freshwater Ecoregions of the World: a New Map of Biogeographic Units for Freshwater Biodiversity Conservation. BioScience 58, 403–414. doi:10.1641/b580507
Adekola, O., Mitchell, G., and Grainger, A. (2015). Inequality and Ecosystem Services: the Value and Social Distribution of Niger Delta Wetland Services. Ecosystem Serv. 12, 42–54. doi:10.1016/j.ecoser.2015.01.005
Agboola, O. A., Downs, C. T., and O'brien, G. (2019). Macroinvertebrates as Indicators of Ecological Conditions in the Rivers of KwaZulu-Natal, South Africa. Ecol. indicators 106, 105465. doi:10.1016/j.ecolind.2019.105465
Angert, A. L., Crozier, L. G., Rissler, L. J., Gilman, S. E., Tewksbury, J. J., and Chunco, A. J. (2011). Do species' Traits Predict Recent Shifts at Expanding Range Edges? Ecol. Lett. 14, 677–689. doi:10.1111/j.1461-0248.2011.01620.x
Beck, H. E., Zimmermann, N. E., Mcvicar, T. R., Vergopolan, N., Berg, A., and Wood, E. F. (2018). Present and Future Köppen-Geiger Climate Classification Maps at 1-km Resolution. Scientific data 5, 1–12. doi:10.1038/sdata.2018.214
Boudot, J.-P., and De Knijf, G. (2012). Nouvelles données sur les Odonates du Maroc oriental et méridional (Odonata). Martinia 28, 1–28.
Boudot, J., and Kalkman, V. (2015). Atlas of the Dragonflies and Damselflies of Europe. Netherlands: KNN Vuitgeverij.
Brandt, M., Rasmussen, K., Peñuelas, J., Tian, F., Schurgers, G., Verger, A., et al. (2017). Human Population Growth Offsets Climate-Driven Increase in Woody Vegetation in Sub-saharan Africa. Nat. Ecol. Evol. 1, 1–6. doi:10.1038/s41559-017-0081
Braune, E., Richter, O., Söndgerath, D., and Suhling, F. (2008). Voltinism Flexibility of a Riverine Dragonfly along thermal Gradients. Glob. Change Biol. 14, 470–482. doi:10.1111/j.1365-2486.2007.01525.x
Briggs, A. J., Pryke, J. S., Samways, M. J., and Conlong, D. E. (2019). Complementarity Among Dragonflies across a Pondscape in a Rural Landscape Mosaic. Insect Conserv Divers. 12, 241–250. doi:10.1111/icad.12339
Bucak, T., Trolle, D., Andersen, H. E., Thodsen, H., Erdoğan, Ş., Levi, E. E., et al. (2017). Future Water Availability in the Largest Freshwater Mediterranean lake Is at Great Risk as Evidenced from Simulations with the SWAT Model. Sci. Total Environ. 581-582, 413–425. doi:10.1016/j.scitotenv.2016.12.149
Bucchignani, E., Mercogliano, P., Panitz, H.-J., and Montesarchio, M. (2018). Climate Change Projections for the Middle East-North Africa Domain with COSMO-CLM at Different Spatial Resolutions. Adv. Clim. Change Res. 9, 66–80. doi:10.1016/j.accre.2018.01.004
Carrão, H., Naumann, G., and Barbosa, P. (2016). Mapping Global Patterns of Drought Risk: An Empirical Framework Based on Sub-national Estimates of hazard, Exposure and Vulnerability. Glob. Environ. Change 39, 108–124. doi:10.1016/j.gloenvcha.2016.04.012
Chekchaki, S., Zaafour, M.-D., and Beddiar, A. (2020). Cartographie diachronique de la dynamique forestière et évolution de l'invasion des subéraies et des eucalyptaies par l'Acacia noir (Acacia mearnsii De Wild) dans le Parc National d'El Kala (Nord-Est algérien). physio-geo 15, 233–257. doi:10.4000/physio-geo.11877
Chelli, A., Zebsa, R., and Khelifa, R. (2019). Discovery of a New Population of the Endangered Calopteryx Exul in central North Algeria (Odonata: Calopterygidae). Notulae Odonatologicae 9, 150–154. doi:10.5281/zenodo.3539748
Córdoba-Aguilar, A. (2008). Dragonflies and Damselflies: Model Organisms for Ecological and Evolutionary Research. Oxford: OUP. doi:10.1093/acprof:oso/9780199230693.001.0001
Darwall, W., Tweddle, D., Smith, K., and Skelton, P. (2009). The Status and Distribution of Freshwater Biodiversity in Southern Africa. Switzerland: IUCN and Grahamstown.
Darwall, W., Smith, K., Allen, D., Holland, R., Harrison, I., and Brooks, E. (2011). The Diversity of Life in African Freshwaters: Underwater, under Threat: An Analysis of the Status and Distribution of Freshwater Species throughout mainland Africa. Cambridge, United Kingdom and Gland, Switzerland: IUCN. xiii+347pp+4pp cover. doi:10.1093/oxfordhb/9780199556137.003.0019
Das, S. K., Routh, J., Roychoudhury, A. N., and Val Klump, J. (2008). Major and Trace Element Geochemistry in Zeekoevlei, South Africa: a Lacustrine Record of Present and Past Processes. Appl. Geochem. 23, 2496–2511. doi:10.1016/j.apgeochem.2008.02.011
Deacon, C., and Samways, M. J. (2017). Conservation Planning for the Extraordinary and Endangered Spesbona Damselfly. J. Insect Conserv 21, 121–128. doi:10.1007/s10841-017-9960-8
Deacon, C., Samways, M. J., and Pryke, J. S. (2018). Artificial Reservoirs Complement Natural Ponds to Improve Pondscape Resilience in Conservation Corridors in a Biodiversity Hotspot. PloS one 13, e0204148. doi:10.1371/journal.pone.0204148
Deacon, C., Samways, M. J., and Pryke, J. S. (2019). Aquatic Insects Decline in Abundance and Occupy Low‐quality Artificial Habitats to Survive Hydrological Droughts. Freshw. Biol. 64, 1643–1654. doi:10.1111/fwb.13360
Deacon, C., Samways, M. J., and Pryke, J. S. (2020). Determining Drivers of Dragonfly Diversity Patterns and the Implications for Conservation in South Africa. Biol. Conservation 245, 108548. doi:10.1016/j.biocon.2020.108548
Deutsch, C. A., Tewksbury, J. J., Huey, R. B., Sheldon, K. S., Ghalambor, C. K., Haak, D. C., et al. (2008). Impacts of Climate Warming on Terrestrial Ectotherms across Latitude. Proc. Natl. Acad. Sci. 105, 6668–6672. doi:10.1073/pnas.0709472105
Dijkstra, K.-D. B., and Clausnitzer, V. (2014). The Dragonflies and Damselflies of Eastern Africa: Handbook for All Odonata from Sudan to Zimbabwe. Tervuren, Belgium: Royal Museum for Central Africa / Musée Royal de l'Afrique Centrale.
Dijkstra, K.-D. B., Samways, M. J., and Simaika, J. P. (2007). Two New Relict Syncordulia Species Found during Museum and Field Studies of Threatened Dragonflies in the Cape Floristic Region (Odonata: Corduliidae). Zootaxa 1467, 19–34. doi:10.11646/zootaxa.1467.1.2
Droogers, P., Immerzeel, W. W., Terink, W., Hoogeveen, J., Bierkens, M. F. P., Van Beek, L. P. H., et al. (2012). Water Resources Trends in Middle East and North Africa towards 2050. Hydrol. Earth Syst. Sci. 16, 3101–3114. doi:10.5194/hess-16-3101-2012
Faramarzi, M., Abbaspour, K. C., Ashraf Vaghefi, S., Farzaneh, M. R., Zehnder, A. J. B., Srinivasan, R., et al. (2013). Modeling Impacts of Climate Change on Freshwater Availability in Africa. J. Hydrol. 480, 85–101. doi:10.1016/j.jhydrol.2012.12.016
Ferreira, S., Velo-Antón, G., Brochard, C., Vieira, C., Alves, P. C., Thompson, D. J., et al. (2014). A Critically Endangered New Dragonfly Species from Morocco: Onychogomphus Boudoti Sp. Nov. (Odonata: Gomphidae). Zootaxa 3856, 349–365. doi:10.11646/zootaxa.3856.3.3
Fick, S. E., and Hijmans, R. J. (2017). WorldClim 2: New 1‐km Spatial Resolution Climate Surfaces for Global Land Areas. Int. J. Climatol 37, 4302–4315. doi:10.1002/joc.5086
García, N., Cuttelod, A., and Malak, D. A. (2010). The Status and Distribution of Freshwater Biodiversity in Northern Africa. Gland, Switzerland: IUCN.
Garibaldi, L. A., Oddi, F. J., Miguez, F. E., Bartomeus, I., Orr, M. C., Jobbágy, E. G., et al. (2020). Working Landscapes Need at Least 20% Native Habitat. Conservation Lett. 14, e12773. doi:10.1111/conl.12773
Gerland, P., Raftery, A. E., ev ikova, H., Li, N., Gu, D., Spoorenberg, T., et al. (2014). World Population Stabilization Unlikely This century. Science 346, 234–237. doi:10.1126/science.1257469
Gilbert, S. L., Broadley, K., Doran‐Myers, D., Droghini, A., Haines, J. A., Hämäläinen, A., et al. (2020). Conservation Triage at the Trailing Edge of Climate Envelopes. Conservation Biol. 34, 289–292. doi:10.1111/cobi.13401
Grueber, C. E., Nakagawa, S., Laws, R. J., and Jamieson, I. G. (2011). Multimodel Inference in Ecology and Evolution: Challenges and Solutions. J. Evol. Biol. 24, 699–711. doi:10.1111/j.1420-9101.2010.02210.x
Guettaf, M., Maoui, A., and Ihdene, Z. (2017). Assessment of Water Quality: a Case Study of the Seybouse River (North East of Algeria). Appl. Water Sci. 7, 295–307. doi:10.1007/s13201-014-0245-z
Hamzaoui, D., Hafiane, M., Mebarki, M., Arab, A., Alfarhan, A. H., and Samraoui, B. (2015). The Gomphidae of Algeria and the Maghreb: Status, Ecology and Conservation (Insecta: Odonata). Int. J. Odonatology 18, 175–191. doi:10.1080/13887890.2015.1043655
Hansen, M. C., Potapov, P. V., Moore, R., Hancher, M., Turubanova, S. A., Tyukavina, A., et al. (2013). High-resolution Global Maps of 21st-century forest Cover Change. Science 342, 850–853. doi:10.1126/science.1244693
Harris, I., Jones, P. D., Osborn, T. J., and Lister, D. H. (2014). Updated High-Resolution Grids of Monthly Climatic Observations - the CRU TS3.10 Dataset. Int. J. Climatol. 34, 623–642. doi:10.1002/joc.3711
Hassall, C., Thompson, D. J., French, G. C., and Harvey, I. F. (2007). Historical Changes in the Phenology of British Odonata Are Related to Climate. Glob. Change Biol 13, 933–941. doi:10.1111/j.1365-2486.2007.01318.x
Hassall, C. (2015). Odonata as Candidate Macroecological Barometers for Global Climate Change. Freshw. Sci. 34, 1040–1049. doi:10.1086/682210
Hickling, R., Roy, D. B., Hill, J. K., and Thomas, C. D. (2005). A Northward Shift of Range Margins in British Odonata. Glob. Change Biol 11, 502–506. doi:10.1111/j.1365-2486.2005.00904.x
Hijmans, R. J., Van Etten, J., Cheng, J., Mattiuzzi, M., Sumner, M., Greenberg, J. A., et al. (2015). Package ‘raster’. R Package 734.
Holland, R. A., Darwall, W. R. T., and Smith, K. G. (2012). Conservation Priorities for Freshwater Biodiversity: the Key Biodiversity Area Approach Refined and Tested for continental Africa. Biol. Conservation 148, 167–179. doi:10.1016/j.biocon.2012.01.016
Jooste, M. L., Samways, M. J., and Deacon, C. (2020). Fluctuating Pond Water Levels and Aquatic Insect Persistence in a Drought-Prone Mediterranean-type Climate. Hydrobiologia 847, 1315–1326. doi:10.1007/s10750-020-04186-1
Kara, H. M. (2012). Freshwater Fish Diversity in Algeria with Emphasis on Alien Species. Eur. J. Wildl. Res. 58, 243–253. doi:10.1007/s10344-011-0570-6
Khelifa, R., and Zebsa, R. (2018). Rediscovery of the Regionally Critically Endangered Dragonfly Lindenia Tetraphylla in Northeast Algeria after 170 Years of Apparent Absence (Odonata: Gomphidae). Notulae Odonatologicae 9, 50–54. doi:10.5281/zenodo.1481065
Khelifa, R., Mellal, M. K., Zouaimia, A., Amari, H., Zebsa, R., Bensouilah, S., et al. (2016a). On the Restoration of the Last Relict Population of a Dragonfly Urothemis Edwardsii Selys (Libellulidae: Odonata) in the Mediterranean. J. Insect Conserv 20, 797–805. doi:10.1007/s10841-016-9911-9
Khelifa, R., Zebsa, R., Amari, H., Mellal, M. K., Mahdjoub, H., and Kahalerras, A. (2016b). A Hotspot for Threatened Mediterranean Odonates in the Seybouse River (Northeast Algeria): Are IUCN Population Sizes Drastically Underestimated? Int. J. Odonatology 19, 1–11. doi:10.1080/13887890.2015.1133331
Khelifa, R., Mahdjoub, H., Baaloudj, A., Cannings, R. A., and Samways, M. J. (2021a). Effects of Both Climate Change and Human Water Demand on a Highly Threatened Damselfly. Scientific Rep. 11, 7725. doi:10.1038/s41598-021-86383-z
Khelifa, R., Mahdjoub, H., Baaloudj, A., Cannings, R. A., and Samways, M. J. (2021b). Remarkable Population Resilience in a North African Endemic Damselfly in the Face of Rapid Agricultural Transformation. Insects 12, 353. doi:10.3390/insects12040353
Khelifa, R. (2013). Flight Period, Apparent Sex Ratio and Habitat Preferences of the Maghribian Endemic Calopteryx Exul Selys, 1853 (Odonata: Zygoptera). Revue d’Ecologie (La Terre et La Vie) 68, 37–45.
Khelifa, R. (2019). Sensitivity of Biodiversity Indices to Life History Stage, Habitat Type and Landscape in Odonata Community. Biol. Conserv. 237, 63–69. doi:10.1016/j.biocon.2019.06.010
Kietzka, G. J., Pryke, J. S., and Samways, M. J. (2018). Comparative Effects of Urban and Agricultural Land Transformation on Odonata Assemblages in a Biodiversity Hotspot. Basic Appl. Ecol. 33, 89–98. doi:10.1016/j.baae.2018.08.008
Kietzka, G. J., Pryke, J. S., Gaigher, R., and Samways, M. J. (2021). Webs of Well-Designed Conservation Corridors Maintain River Ecosystem Integrity and Biodiversity in Plantation Mosaics. Biol. Conservation 254, 108965. doi:10.1016/j.biocon.2021.108965
Kipping, J., Martens, A., and Suhling, F. (2012). Africa's Smallest Damselfly-A New Agriocnemis from Namibia (Odonata: Coenagrionidae). Org. Divers. Evol. 12, 301–306. doi:10.1007/s13127-012-0084-4
Korbaa, M., Ferreras-Romero, M., Ruiz-García, A., and Boumaiza, M. (2018). TSOI− a New index Based on Odonata Populations to Assess the Conservation Relevance of Watercourses in Tunisia. Odonatologica 47, 43–72. doi:10.5281/zenodo.1239945
Ledger, M. E., Brown, L. E., Edwards, F. K., Milner, A. M., and Woodward, G. (2013). Drought Alters the Structure and Functioning of Complex Food Webs. Nat. Clim Change 3, 223–227. doi:10.1038/nclimate1684
Lionello, P., Malanotte-Rizzoli, P., Boscolo, R., Alpert, P., Artale, V., Li, L., et al. (2006). “The Mediterranean Climate: an Overview of the Main Characteristics and Issues,” in Mediterranean Climate Variability. Editors P. Lionello, P. Malanotte-Rizzoli, and R. Boscolo (Amsterdam: Elsevier), 1–26. doi:10.1016/s1571-9197(06)80003-0
Messager, M. L., Lehner, B., Grill, G., Nedeva, I., and Schmitt, O. (2016). Estimating the Volume and Age of Water Stored in Global Lakes Using a Geo-Statistical Approach. Nat. Commun. 7, 1–11. doi:10.1038/ncomms13603
Modiba, R. V., Joseph, G. S., Seymour, C. L., Fouché, P., and Foord, S. H. (2017). Restoration of Riparian Systems through Clearing of Invasive Plant Species Improves Functional Diversity of Odonate Assemblages. Biol. Conserv. 214, 46–54. doi:10.1016/j.biocon.2017.07.031
Molina, M., Sánchez, E., and Gutiérrez, C. (2020). Future Heat Waves over the Mediterranean from an Euro-coRDeX Regional Climate Model Ensemble. Scientific Rep. 10, 1–10. doi:10.1038/s41598-020-65663-0
Müller, O. (2008). Larval Habitats and Life History of the Crete Island endemicBoyeria cretensis(Odonata: Aeshnidae). Int. J. Odonatology 11, 195–207. doi:10.1080/13887890.2008.9748323
Myers, N., Mittermeier, R. A., Mittermeier, C. G., Da Fonseca, G. A. B., and Kent, J. (2000). Biodiversity Hotspots for Conservation Priorities. Nature 403, 853–858. doi:10.1038/35002501
O’Gorman, E. J., Petchey, O. L., Faulkner, K. J., Gallo, B., Gordon, T. A., Neto-Cerejeira, J., et al. (2019). A Simple Model Predicts How Warming Simplifies Wild Food Webs. Nat. Clim. Change 9, 611–616. doi:10.1038/s41558-019-0513-x
Paaijmans, K. P., Heinig, R. L., Seliga, R. A., Blanford, J. I., Blanford, S., Murdock, C. C., et al. (2013). Temperature Variation Makes Ectotherms More Sensitive to Climate Change. Glob. Change Biol. 19, 2373–2380. doi:10.1111/gcb.12240
Pinheiro, J., Bates, D., Debroy, S., and Sarkar, D.R Core Team (2020). Nlme: Linear and Nonlinear Mixed Effects Models. R Package Version 3.1-151. URL: https://CRAN.R-project.org/package=nlme>.
R Development Core Team, (2020). R: A Language and Environment for Statistical Computing. Vienna, Austria: R Foundation for Statistical Computing.
Richter, O., Suhling, F., Müller, O., and Kern, D. (2008). A Model for Predicting the Emergence of Dragonflies in a Changing Climate. Freshw. Biol. 53, 1868–1880. doi:10.1111/j.1365-2427.2008.02012.x
Riservato, E., Boudot, J.-P., Ferreira, S., Jovíc, M., Kalkman, V. J., Schneider, W., et al. (2009). The Status and Distribution of Dragonflies of the Mediterranean BasinGland, Switzerland and Malaga. IUCN (International Union for Conservation of Nature): Spain.
Rocha-Ortega, M., Rodríguez, P., Bried, J., Abbott, J., and Córdoba-Aguilar, A. (2020). Why Do Bugs Perish? Range Size and Local Vulnerability Traits as Surrogates of Odonata Extinction Risk. Proc. R. Soc. B. 287, 20192645. doi:10.1098/rspb.2019.2645
Samraoui, B., and Corbet, P. S. (2000). The Odonata of Numidia, Northeastern Algeria Part I Status and Distribution. Int. J. Odonatology 3, 11–25. doi:10.1080/13887890.2000.9748133
Samways, M. J., and Deacon, C. (2021). “Extinction Reprieve for the Ancient and Imperiled Dragonflies at the Southern Tip of Africa,” in Imperiled: Encyclopedia of Conservation. Editor S. Elias (Paris Elsevier) in press. doi:10.1016/b978-0-12-821139-7.00047-7
Samways, M. J., and Sharratt, N. J. (2010). Recovery of Endemic Dragonflies after Removal of Invasive Alien Trees. Conserv. Biol. 24, 267–277. doi:10.1111/j.1523-1739.2009.01427.x
Samways, M. J., and Simaika, J. P. (2016). Manual of Freshwater Assessment for South Africa: Dragonfly Biotic index. in Suricata 2. Pretoria, South Africa: South African National Biodiversity Institute (SANBI).
Samways, M. J., Taylor, S., and Tarboton, W. (2005). Extinction Reprieve Following Alien Removal. Conserv. Biol. 19, 1329–1330. doi:10.1111/j.1523-1739.2005.00176.x
Samways, M. J., Deacon, C., Kietzka, G. J., Pryke, J. S., Vorster, C., and Simaika, J. P. (2020). Value of Artificial Ponds for Aquatic Insects in Drought-Prone Southern Africa: a Review. Biodivers Conserv 29, 3131–3150. doi:10.1007/s10531-020-02020-7
Samways, M. J. (2008). “Dragonflies as Focal Organisms in Contemporary Conservation Biology,” in Dragonflies & Damselflies: Model Organisms for Ecological and Evolutionary Research. Oxford, UK: Oxford University Press, 97–108. doi:10.1093/acprof:oso/9780199230693.003.0008
Simaika, J. P., and Samways, M. J. (2009a). An Easy-To-Use index of Ecological Integrity for Prioritizing Freshwater Sites and for Assessing Habitat Quality. Biodivers Conserv. 18, 1171–1185. doi:10.1007/s10531-008-9484-3
Simaika, J. P., and Samways, M. J. (2009b). Reserve Selection Using Red Listed Taxa in Three Global Biodiversity Hotspots: Dragonflies in South Africa. Biol. Conserv. 142, 638–651. doi:10.1016/j.biocon.2008.11.012
Stephenson, P. J., Bowles-Newark, N., Regan, E., Stanwell-Smith, D., Diagana, M., Höft, R., et al. (2017). Unblocking the Flow of Biodiversity Data for Decision-Making in Africa. Biol. Conservation 213, 335–340. doi:10.1016/j.biocon.2016.09.003
Stephenson, P. J., Ntiamoa-Baidu, Y., and Simaika, J. P. (2020). The Use of Traditional and Modern Tools for Monitoring Wetlands Biodiversity in Africa: Challenges and Opportunities. Front. Environ. Sci. 8, 61. doi:10.3389/fenvs.2020.00061
Stoks, R., and Córdoba-Aguilar, A. (2012). Evolutionary Ecology of Odonata: a Complex Life Cycle Perspective. Annu. Rev. Entomol. 57, 249–265. doi:10.1146/annurev-ento-120710-100557
Suhling, F., Martens, A., and Suhling, I. (2017). Long-distance Dispersal in Odonata: Examples from Arid Namibia. Austral Ecol. 42, 544–552. doi:10.1111/aec.12472
Thieme, M. L., Abell, R., Burgess, N., Lehner, B., Dinerstein, E., and Olson, D. (2005). Freshwater Ecoregions of Africa and Madagascar: A Conservation Assessment. Washington, DC: Island Press.
Tseng, M., Kaur, K. M., Soleimani Pari, S., Sarai, K., Chan, D., Yao, C. H., et al. (2018). Decreases in Beetle Body Size Linked to Climate Change and Warming Temperatures. J. Anim. Ecol. 87, 647–659. doi:10.1111/1365-2656.12789
Van Wilgen, B. W., Le Maitre, D. C., and Cowling, R. M. (1998). Ecosystem Services, Efficiency, Sustainability and Equity: South Africa's Working for Water Programme. Trends Ecol. Evol. 13, 378. doi:10.1016/s0169-5347(98)01434-7
Venables, W. N., and Ripley, B. D. (2002). Modern Applied Statistics with S New York: Springer-Verlag.
Venter, O., Sanderson, E. W., Magrach, A., Allan, J. R., Beher, J., Jones, K. R., et al. (2016a). Global Terrestrial Human Footprint Maps for 1993 and 2009. Sci. Data 3, 160067. doi:10.1038/sdata.2016.67
Venter, O., Sanderson, E. W., Magrach, A., Allan, J. R., Beher, J., Jones, K. R., et al. (2016b). Sixteen Years of Change in the Global Terrestrial Human Footprint and Implications for Biodiversity Conservation. Nat. Commun. 7, 12558. doi:10.1038/ncomms12558
Vicente-Serrano, S. M. (2015). The Climate Data Guide: Standardized Precipitation Evapotranspiration Index (SPEI).National Center for Atmospheric Research StaffRetreived from: https://climatedataguide.ucar.edu/climate-data/standardized-precipitation-evapotranspiration-index-speihttps://climatedataguide.ucar.edu/climate-data/standardized-precipitation-evapotranspiration-index-spei (Accessed January 15, 2021).
Vilizzi, L., Tarkan, A. S., and Copp, G. H. (2015). Experimental Evidence from Causal Criteria Analysis for the Effects of Common CarpCyprinus Carpioon Freshwater Ecosystems: A Global Perspective. Rev. Fish. Sci. Aquacult. 23, 253–290. doi:10.1080/23308249.2015.1051214
Vorster, C., Samways, M. J., Simaika, J. P., Kipping, J., Clausnitzer, V., Suhling, F., et al. (2020). Development of a New Continental-Scale Index for Freshwater Assessment Based on Dragonfly Assemblages. Ecol. Indicators 109, 105819. doi:10.1016/j.ecolind.2019.105819
Wangai, P. W., Burkhard, B., and Müller, F. (2016). A Review of Studies on Ecosystem Services in Africa. Int. J. Sustainable Built Environ. 5, 225–245. doi:10.1016/j.ijsbe.2016.08.005
Wiens, J. J. (2016). Climate-related Local Extinctions Are Already Widespread Among Plant and Animal Species. Plos Biol. 14, e2001104. doi:10.1371/journal.pbio.2001104
Xi, Y., Peng, S., Ciais, P., and Chen, Y. (2021). Future Impacts of Climate Change on Inland Ramsar Wetlands. Nat. Clim. Chang. 11, 45–51. doi:10.1038/s41558-020-00942-2
Keywords: odonates, freshwater, Africa, drought, warming, human footprint index, management
Citation: Khelifa R, Deacon C, Mahdjoub H, Suhling F, Simaika JP and Samways MJ (2021) Dragonfly Conservation in the Increasingly Stressed African Mediterranean-Type Ecosystems. Front. Environ. Sci. 9:660163. doi: 10.3389/fenvs.2021.660163
Received: 28 January 2021; Accepted: 15 July 2021;
Published: 05 August 2021.
Edited by:
Eugenia López-López, Instituto Politécnico Nacional de México (IPN), MexicoReviewed by:
Aliny P. F. Pires, Rio de Janeiro State University, BrazilAlex Bush, Lancaster University, United Kingdom
Copyright © 2021 Khelifa, Deacon, Mahdjoub, Suhling, Simaika and Samways. This is an open-access article distributed under the terms of the Creative Commons Attribution License (CC BY). The use, distribution or reproduction in other forums is permitted, provided the original author(s) and the copyright owner(s) are credited and that the original publication in this journal is cited, in accordance with accepted academic practice. No use, distribution or reproduction is permitted which does not comply with these terms.
*Correspondence: Rassim Khelifa, cmFzc2lta2hlbGlmYUBnbWFpbC5jb20=