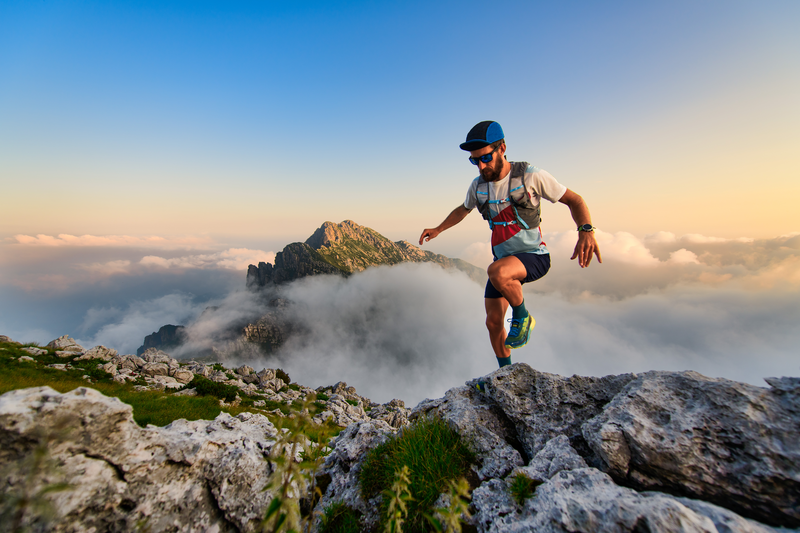
95% of researchers rate our articles as excellent or good
Learn more about the work of our research integrity team to safeguard the quality of each article we publish.
Find out more
ORIGINAL RESEARCH article
Front. Environ. Sci. , 02 August 2021
Sec. Conservation and Restoration Ecology
Volume 9 - 2021 | https://doi.org/10.3389/fenvs.2021.659521
Methods and devices specifically created for remote animal surveys and monitoring are becoming increasingly popular and effective. However, remote devices are also widely used in our societies for different, not scientific, goals. Ski resorts in the European Alps, for instance, use webcams to share panoramic views and promote themselves in the industry of winter recreational activities. We tested preinstalled webcam effectiveness as a remote tool for eco-ethological studies. Our target species was the Alpine Chough Pyrrhocorax graculus, a social and opportunistic corvid species of high mountain environments that attends ski resorts to feed on scraps discarded by high elevation bars and restaurants. We studied the effect of the winter presence of tourists and weather conditions on flocking behaviour at ski resorts. We used flock size and time spent at the ski resort as response variables, and assessed how strongly they were related to the number of tourists and weather conditions. We analysed about 13,500 pictures taken at 10 min intervals at three ski resorts sites in the European Alps in France, Italy and Switzerland. The number of birds was very different among the three study sites. Flock size and time spent were related to the same environmental drivers, but with different effect sizes in the three areas. The daily maximum flock size and the time spent at ski resorts increased with the number of tourists and decreased with temperature at two sites out of three. We also found that the presence of fresh snow caused a decrease in the maximum flock size in all ski resorts. In conclusion, Alpine Choughs modulated their presence at the ski resorts according to human presence and weather conditions, but these responses were context-dependent. Preinstalled webcams, despite a few caveats, can therefore be successfully employed in eco-ethological research. Webcams around the world are increasing in number and represent therefore a large potential resource. If webcam companies could be engaged to make some slight adjustments, without compromising their goals, then this could offer a new way to collect eco-ethological data.
Methods and devices for remote surveys and monitoring of animals are becoming increasingly popular and effective. Technological advances are opening a new world of studying movements, monitoring and conserving animal biodiversity. Scientists can now remotely observe relatively fine-scale movements or migratory patterns by using GPS wildlife tracking techniques. Animal-tracking devices have become smaller, lighter, cheaper, and more accurate, and they can store and transmit more data (Tibbetts 2017; Singh & Bais 2018). By combining animal-tracking and remote-sensing data, it is also possible to create models showing how individuals, animal groups, and species respond to seasonal or climatic changes (Tibbetts 2017). Remotely piloted aircraft (RPA; commonly known as “drones”) can count wildlife more accurately and precisely than researchers using ground-based methods (Hodgson et al., 2018). In the last years the use of camera traps (remote photography) increased the opportunity to collect remotely information about animal ecology in periods and in environments that are hard to be investigated, such as tropical forests or winters in alpine and polar environments (Kucera & Barrett 2011; Soininen et al., 2015; Pesaturo 2018; Niedballa et al., 2019). Webcams have also been used to study different aspects of bird ecology and behaviour (migration: Verstraeten et al., 2010; breeding: Schulwitz et al., 2018; bird interactions; Eichhorst 2018; Wood et al., 2020). All these techniques and devices have been specifically created or developed to study animals remotely. However, remote devices are now widely used in our societies for many different, not scientific, goals. Webcams, for instance, are used for remote control, remote education or e-learning and tourist promotion. Where and how webcams established for other purposes could be mined for data to provide important insights into behavior and ecology is a question which to our knowledge has not been addressed before. Ski resorts in the European Alps increasingly use high resolution webcams to share panoramic views and promote themselves in the industry of winter recreational activities. Cameras can record the presence of middle-size birds, and are equipped with remote access. Therefore, webcams may be a potential useful tool for eco-ethological studies without the physical presence of the observer in the field.
The Alpine Chough, Pyrrhocorax graculus, a middle-size Palearctic bird of high elevation ecosystems, is an excellent target species in this framework. It is a highly social, gregarious corvid, which may form groups of tens of individuals (Delestrade 1994, Rolando et al., 1997; Delestrade 1999). This species illustrates the complexity of the nature of interactions between humans and wild birds. An increasing number of alpine bird species are threatened directly or indirectly by human activities (Chamberlain et al., 2016; Lehikoinen et al., 2019). The construction of buildings and ski pistes or slopes for winter sports, for instance, causes severe habitat loss (Rolando et al., 2007; Rixen and Rolando 2013). Even the daily presence of people can potentially disturb wild birds, leading to modification in species physiology and behaviour (Ellenberg et al., 2007; Gill 2007; Arlettaz et al., 2015). The anthropogenic impact can be mitigated by positive effects, because human presence can be associated with increased food supply and nesting or roosting sites (Kurosawa et al., 2003; Oro et al., 2013; Jokimäki et al., 2017; West and Peery 2017). In opportunistic species, human derived food can improve individual fitness and reproductive outputs, especially when “natural” food is scarce (Acquarone et al., 2002; Oro et al., 2013; West and Peery 2017).
Vallino et al. (2019) demonstrated that visitor presence causes a reduction of feeding rates and stay times of Alpine Choughs foraging on alpine pastures. Interestingly, disturbance may be reduced by particular behavioural responses developed in the areas more frequently visited by tourists, such as reduced vigilance and flushing distance (Jiménez et al., 2013; Vallino et al., 2019). Despite the potential man-induced disturbance, Alpine Choughs frequent ski resorts where, especially in winter, they behave like scavengers by feeding on scraps discarded from high elevation bars and restaurants (Rolando and Patterson 1993; Delestrade 1995). Higher densities of birds occur around high elevation touristic areas where they move around restaurants when tourists are present (Storch and Leidenberger 2003). The potential foraging advantage of flocking, however, is unclear, because in larger flocks birds feed faster, but stay for a shorter time in patches (Delestrade, 1995; Rolando et al., 1997; Vallino et al., 2019). Recent long term research carried out in the French Alps showed that survival of females decreased with increased flock size, likely because their food provisioning is prevented by males, which are dominant (Chiffard et al., 2019).
Studies on Alpine Choughs are usually highly demanding in terms of fieldwork effort since they live in alpine environments. The use of webcams would be therefore very useful in this regard.The present pilot study aims to test the effectiveness of webcams as a remote tool for studying the effect of human presence and weather conditions on the flocking behaviour of Alpine Choughs at ski resorts in the European Alps. We focused on flock size and time spent at the site as response variables, and assessed if they were related to the number of tourists and weather conditions (daily minimum temperatures and snowfall). The results obtained are used as a proof of concept.
We remotely collected data on winter foraging flocks of Alpine Choughs at high altitude ski resorts by using Panomax webcams (Landesstraße, Henndorf, Austria, www.panomax.com). Panomax has 368 public webcams in 16 countries all around in the world, 70 of which are located in the European Alps. We focused on popular alpine ski resorts whose camera view included both restaurant terraces used by tourists and choughs to feed, and the roofs of buildings used by birds to rest. Among webcams meeting the above requirements, we chose three sites located in the French, Swiss and Italian Alps (Figure 1). Other webcams were excluded since one or more requirements were not met: several were located in the lowlands, while many of the high elevation webcams were placed in sites without restaurants and other buildings, or Alpine Choughs were not detected. At every site, cameras provided pictures of the area every 10 min, from 8 am to 5 pm. We focused on the winter period (800–1700 h) collecting a total of 13,608 pictures (i.e., 4,536 for each site, 84 days x 54 pictures/day), from December 14, 2018 until March 8, 2019. Three days were excluded on the Italian site because not all pictures on these days could be used, resulting in 13,446 pictures taken during 249 days. We used all pictures to count birds attending the ski resort at any time of the day; pictures taken between 12 am and 3 pm (when most of the skiers attended bars and restaurants, as indicated by the mean hourly number of people) were used to count the maximum number of tourists. People were counted in a priori defined areas including the restaurant terrace (Figure 2). For each picture, webcams also provided temperature at the webcam location from which we extracted daily minimum and maximum temperature. We considered snowfall events only when they were noticeable and pictures showed a visually detectable uniform layer of fresh snow, so we indicated the presence/absence of snow on a daily basis according to the presence/absence of fresh snow. Data from the pictures were obtained by the same observer (Cristina Vallino).
FIGURE 1. Location of the three study areas in the French, Swiss and Italian Alps. Grands Montets, Männlichen and Col Raiser are the French, Swiss and Italian site, respectively.
FIGURE 2. Webcam views of the three study sites, which include both the restaurant terraces used by tourists and birds to feed, and the roofs of buildings used by birds to rest. People were counted in a priori defined areas including the restaurant terrace. From top to bottom: the Italian site (Col Raiser, CR), the French site (Grands Montets, GM, i.e., Mont Blanc Natural Resort), and the Swiss site (Männlichen, M). Note that it was possible to zoom in to obtain a better detection of birds and/or tourists, when necessary.
The chosen sites were located on flat terrain delimited by cliffs. Surroundings were characterized by snow covered grasslands and ski pistes; the Swiss site showed no tree cover, while conifers were present in the French and in the Italian site.
The French site was in the area surrounding the Lognan ski lift station named Grands Montets, below the Aiguille des Grands Montets in the Chamonix Mont-Blanc valley (45.9685°N, 6.943083°E, hereafter GM). GM was located at 1,972 m above sea level (m.a.s.l.) and consisted of a ski lift station and a restaurant with a terrace. Observations of Alpine Choughs with colour rings used for long term studies on this population revealed that a flock up to 100 individuals frequented GM around midday and in the afternoon, while during the morning they generally descended to the closest villages, Argentière and Le Tour (A. Delestrade personal observation).
The Italian site was at Col Raiser around Almhotel at Santa Cristina in Val Gardena, in the middle of the Dolomites, north eastern Italian Alps (46.584972°N, 11.745167°E, hereafter CR). CR was located at 2,106 m. a.s.l. and consisted of a ski lift station, a restaurant with a terrace and some chalets. Large flocks of up to 100 individuals were known to visit localities about 5 km from the site to feed on berries (such as Sorbus sp.) during winter. Irrespective of the season, birds might feed at high elevation around restaurants, at about 2,500 m.a.s.l., but they also had the possibility to descend to the valley bottom at about 1,000 m.a.s.l. (I. Prugger personal observation).
The Swiss site was in the resort of Männlichen (46.613139°N, 7.941083°E, hereafter M) and was located in Wengen, in the Swiss Bernese Pre-Alps. M is placed at 2,225 m.a.s.l. and consisted of a hostel, a restaurant with terrace and few cabins used as shops. During this study, we included data from both non holiday and holyday periods. In particular, we considered the interval of time between mid December and the beginning of March as representative of European holidays.
For the data analysis we used observations taken in a given day (every 10 minutes) to calculate two daily parameters of flocking behaviour. They were: 1) the maximum flock size and 2) the time spent at ski resort, measured as the number of pictures showing birds/day (daily presence). This resulted in 249 daily data based on the same number of pictures per day (54). We used a generalized linear model (GLM) to analyze maximum flock size and daily presence as both were counts, using a negative binomial distribution for the response variable to take into account over-dispersion when estimating parameter uncertainty (Gelman et al., 2020). We also considered a beta-binomial model for the daily presence (considering that we have a proportion of pictures with choughs out of 54 taken in a day; fitted using the betabin function in aod library; Lesnoff and Lancelot 2012), but results were similar to those obtained using a negative binomial distribution and we present only the latter in the main text. As predictor variables, we used day of year (with 1 = January 1), either as a linear effect or a second order polynomial, minimum temperature (Tmin,°C), maximum number of people, snow and holiday periods. We included day of year as a predictor to account for systematic seasonal changes that were not accounted for by the other covariates. Using maximum temperature (Tmax) as a predictor variable gave results similar to using Tmin, as Tmin and Tmax were highly correlated (CR: r = 0.88, GM: 0.88, M: 0.90), so we present results only for Tmin. To assess if holiday periods, snow, Tmin and maximum number of people had different effects at different sites, we included interactions between site and snow, holiday period, Tmin and maximum number of people. The relative fit of models was compared using the Akaike’s Information Criterion corrected for small sample size, AICc (Burnham and Anderson 2002; calculated using the dredge function in MuMIn with rank = “AICc”; Barton 2020). We used the simplest models among models with the lowest AICc values for inference (within a range of two for AICc; Burnham and Anderson 2002), but used the same model for the two response variables in order to get comparable estimates of effect sizes. Given that the best models included different interactions, we could not use model averaging. We calculated site-specific estimates and associated 95% confidence intervals as well as estimates and 95% CI for regression coefficient differences between sites, to quantify the evidence for differences between sites. Given that the scales of predictors (Maxpeople and Tmin) were quite different, we calculated standardized coefficients by using standardized predictors (with mean = 0 and st. dev. = 1) in the same models as above. We also calculated predicted effects for maximum number of people and Tmin by fixing all other predictors at either the mean of maximum number of people and Tmin (when predicting effect of Tmin and maximum number of people, respectively), and at days with no fresh snow and not holidays for snow and holidays. Predicted values and approximate 95% CI (±2 SE) were made on the link (=log) scale and back-transformed on the response scale by taking the exponential. Residual plots were used for the most complex models and the models used for inference to assess model fit. In particular, we assessed the autocorrelation of residuals, and as the first-order autocorrelation was <0.25 for all three sites, we used models with no autocorrelation.
We used R 4.0.5 (R Core Team 2021) for analyses and plots.
Out of 249 days, we observed fresh snowfall on 33 days (resp. CR: 9, GM: 14, M: 10), and we registered holidays on 114 days (CR: 14, GM: 44, M: 56). There were about the same maximum number of people at the three sites (CR: median = 30, GM: median = 45, M: median = 32.5; all ranges between 0 and 120). Maximum number of people was about the same on holidays than on other days at CR (holidays: mean = 28, other days: 30), but more people were counted on holidays at the two other sites (GM: Holidays: 53, other: 29; M: Holidays: 56, other: 13). Minimum and maximum temperature were also similar at the three sites (min T in°C: CR: mean = −7.7 [SD = 3.9], GM: −5.7 [4.3], M: −7.7 [3.6]; max T: CR: v1.7 [4.6], GM: 1.0 [5.2], M: −3.0 [4.3]).
Daily maximum flock size varied among sites with M, GM and CR showing the smallest (median = 2, min-max = 0–15) intermediate (median = 35, min-max = 0–109) and largest (median = 70, min-max = 0–150) maximum flock size, respectively (Supplementary Figure S1). Daily presence varied also among sites with CR and GM having the highest presence (Supplementary Figure S1; CR: median = 14, min-max = 0–37, GM: median = 14, min-max = 0–40) and M the lowest (median = 4, min-max = 0–23). Maximum flock size was related to the daily presence (i.e., the number of pictures with birds/day), but the relationship was site-dependent: the daily presence increased with the maximum flock size at M and GM, not at CR (Figure 3).
FIGURE 3. Variation of Number of presence (the number of pictures showing birds, i.e., daily presence) of Alpine Choughs according to maximum flock size and site. The daily presence increased with the maximum flock size at Männlichen and Grands Montets.
The model common to both response variables and with the best relative fit (Table 1 and Table 2) included an interaction between sites and Tmin, sites and maximum number of people as well as additive effects of snow and holidays. Daily maximum flock size was negatively affected by daily Tmin, with varying effect sizes, being weakest at CR and strongest at M (Figure 4, Table 3; difference in regression coefficient GM-CR: −0.059 [−0.12; −0.001], M-CR: −0.080 [−0.16; −0.001]). The effect of the maximum number of people present at restaurant terraces on daily maximum flock size, was positive and strong at M, weaker at GM and close to zero at CR (Figure 4, Table 1B; difference in regression coefficientGM-CR: 0.78 [−0.42; 1.97], M-CR: 2.15 [0.89; 3.41]). Standardized coefficients (Supplementary Table S1) showed that effect sizes of Tmin and maximum number of people were of comparable magnitude. Days with snow had smaller maximum flock size, and there was weak evidence for larger flock size on holidays (Table 3).
TABLE 1. A. Model selection for the generalized linear models analyzing variation of maximum flock size. Distribution was negative binomial (NB). Models were ranked according to AICc, and the best models within a Delta AICc (in AICc from the model with lowest AICc) of two are shown. The model used for inference is indicated in italic.
TABLE 2. A. Model selection for the generalized linear models analyzing Number of presence. Distribution was negative binomial (NB). Models were ranked according to AICc, and the best models within a Delta AICc of two are shown. The model used for inference is indicated in italic.
FIGURE 4. Maximum Number of People positively affects daily maximum flock size at the two sites (Grands Montets and Männlichen) with the smallest flocks of Alpine Choughs, whereas Minimum Temperature had a negative effect at the same two sites. Model fits (with 95% intervals) correspond to the model selected for inference (Table 1), and fixing other predictors at given values (see text).
TABLE 3. | Parameter estimates (with 95% CI in brackets) of the model analyzing variation of maximum flock size. Regression parameters for maxpeople and Tmin are given for each site, and coefficient differences between sites are given in the text. We used the same model as for analyzing Number of Presences to facilitate comparisons between response variables. For maximum number of people coefficients were multiplied by 100. For snow and Holiday, the coefficient is the difference on a log scale between days with snow (resp holiday) and no snow (resp no holiday). The theta parameter for the negative binomial was 2.28.
The daily presence was related to daily Tmin and the maximum number of people (Table 2), and also with variable effect sizes (Table 4). Minimum temperature led to a decrease of daily presence at M, but the effect was smaller at GM and negligible at CR (Figure 5, Table 4; difference in regression coefficients GM-CR: −0.025 [−0.083; 0.034], M-CR: −0.11 [−0.19; −0.03]). High tourist numbers led to an increase of daily presence at M, with a smaller effect at GM and no evidence for such an effect at CR (Figure 5, Table 4; difference in regression coefficient GM-CR: 1.29 [0.11; 2.47], M-CR: 3.22 [2.00; 4.43]). Standardized coefficients (Supplementary Table S2) showed that effect sizes of Tmin and maximum number of people were of comparable magnitude. Days with snow had lower daily presence whereas there was week evidence for a positive effect of holidays. Analysing the number of daily presence as a proportion (using a beta-binomial model to account for overdispersion) gave coefficients similar to those using a negative binomial for the number of presence (Supplementary Table S3).
TABLE 4. Parameter estimates (with 95% CI in brackets) of the model analyzing variation of Number of presence. Regression parameters for maxpeople and Tmin are given for each site, and coefficient differences between sites are given in the text. We used the same model as for analyzing Maximum flock size to facilitate comparisons between response variables. For maximum number of people coefficients were multiplied by 100. For snow and Holiday, the coefficient is the difference on a log scale between days with snow (resp holiday) and no snow (resp no holiday). The theta parameter for the negative binomial distribution was 2.40.
FIGURE 5. Maximum number of people positively affects number of presence (the number of pictures showing birds, i.e. daily presence) at two sites (Grands Montets and Männlichen), with the strongest effect at the site with small flock size (Männlichen). Model fits (with 95% intervals) correspond to the model selected for inference (Table 1), and fixing other predictors at given values (see text).
The results of the present study, obtained from the analyses of about 13,500 webcam pictures, identified some potential drivers of winter flocking behaviour of the Alpine Chough at ski resorts. We showed that Alpine Choughs feeding on scraps can modulate flock size and time spent around restaurants according to the number of tourists, cold intensity and snowfall. We utilized these results as a proof of concept, and concluded that webcams may be a useful remote tool for eco-ethological research.
The three study sites showed evident differences in the number of birds, with the smallest flocks at M and the largest at CR. The daily presence increased with the maximum flock size at M and GM, not at CR. Also, response variables were controlled by the same environmental drivers, but with different intensity in the three areas. The daily maximum flock size and the time spent at ski resorts (daily presence, measured as the number of pictures showing birds/day) increased with the number of tourists and decreased with temperature at two sites out of three. These relationships could represent strong effects, with for example the daily presence decreasing by a factor of 2–5 when the minimum temperature increased from −15 to 0 C (Figure 5).
Hence, Alpine Choughs modulated their presence around the restaurants according to human presence (which guarantees anthropogenic food availability) and weather conditions, but these responses were context-dependent.
We also found that the presence of fresh snow caused a decrease in the maximum flock size in all ski resorts. This may be explained by assuming snowfall has a significant effect on Alpine Chough movements, by inducing birds to move to lower areas for feeding, thus reducing flock size at higher ski resorts. Villages at lower elevations occur at all three sites. The small villages of the valley bottom around Chamonix (French site) were regularly visited by birds looking for food scraps (C. Vallino personal observation). The absence of evidence for an effect of holidays was likely due to the association with the number of people present, but was not consistent among sites.
A generic association between tourist presence and birds looking for food is well known in scavenger species. Storch and Leidenberger (2003) showed that in summer, during the post fledging period, Alpine Choughs moved around restaurants when tourists were present. Our results demonstrated that birds were also attracted by tourist activity in winter, and that in two sites out of three Alpine Choughs were not influenced by the presence of people per se, but rather by the number of people gathering at the site. These corvids have not only learned to associate the presence of people with the presence of food, but they also clearly know that more people discard more scraps.
In high elevation ecosystems, weather conditions may significantly affect several aspect of bird biology. Results of a 27 years demographic research project on marked Alpine Choughs at GM suggested that high winter and spring temperatures may affect spring survival, especially of females (Chiffard et al., 2019). The present study demonstrates that harsh weather conditions may also drive birds’ flocking behaviour. In two out of three sites, Alpine Choughs tended to concentrate and stay longer around restaurants of ski resorts (i.e., both the number of birds in flocks and the time spent at the site measured as daily presence increased) when cold intensity increased. Since human supplied leftovers represent predictable food (Bridge et al., 2009; Oro et al., 2013), we hypothesize that birds are prone to exploitation in case of increased need of energy intake, thus when the temperature drops and “natural” food sources are scarce.
The opportunistic use of food provided by man may be facilitated by the pronounced sociality of the Alpine Chough, that it is known to forage and move in flocks throughout the year (Cramp and Perrins 1994; Delestrade 1994; Rolando et al., 1997; Delestrade 1999). In the present study, the time spent at the ski-resorts (daily presence) increased with maximum flock size, but not all sites. Benefits derived from foraging in flocks include a reduced predation risk, possibly due either to the presence of more vigilant individuals, allowing a reduction of single birds’ investment in vigilance, or to a diminished risk of individual predation, when the predator can choose among many potential prey (Hamilton, 1971; Lima 1995; Roberts 1996; Rolando et al., 2001). Accordingly, vigilance is expected to decrease in larger groups since they potentially provide more safety against predators. Alpine Chough displays a decline in vigilance with group size (Vallino et al., 2019), and the same was shown in the Red-billed chough P. pyrrhocorax (Rolando et al., 2001). This relationship might help to understand the apparent paradox of birds exploiting human food scraps despite human disturbance. In large flocks, the disturbance due to the presence of people would be counterbalanced by benefits provided by the availability of anthropogenic food and, maybe, by a diminished vigilance activity.
In the Alpine Chough, the availability of anthropogenic food is also responsible for the disruption of winter movement patterns. In valleys with little ski development, flocks of Alpine Choughs showed circadian migration movements, flying towards snow free valley bottoms to look for food in the morning, and coming back to the roosts in high elevation cliffs in the afternoon. Vice versa, in ski developed resorts, birds stayed in town all day, roosting on buildings and opportunistically feeding on scraps (Rolando et al., 2003).
Some general and specific remarks are useful to underline the advantages derived from the use of webcams: 1) preinstalled webcams allow data collection for free, and can therefore be useful for projects with small budgets; 2) the use of this kind of technology allows a large amount of data to be collected in a shorter time frame than in the field (fieldwork is always time consuming); 3) working at high elevation can be prohibitive in cases of difficult weather conditions, whilst using webcams means observations can be collected in virtually all environmental conditions; 4) webcam monitoring of flock behavior may last all day long and over the winter season, which is not possible for most field observers.
This study also highlighted some caveats. First, we don’t know what a minimum species size would be to be sufficiently detectable by the webcams. As far as choughs are concerned, pictures captured only a part of the flock because not every bird was always located in front of the camera. Therefore, flock size is likely to be underestimated. Second, despite the use of high definition images, colour rings of marked individuals at GM could not be discerned. Therefore, the remote observer has no way of knowing whether the same individuals were attending the site over time, or if there was a high turnover of individuals. Third, we could not get information about the part of the environment that is not included in the webcam view. Parts of the above mentioned caveats can be mitigated by the installation of webcams specially set for research purposes, and the combination of remotely collected data with fieldwork or additional available data about study areas and populations (e.g., demographic data). Fieldwork can be useful to obtain more precise information about the effective flock size, the attendance of sites by marked birds, and therefore identify potential biases of webcam data and assess the overall importance of resorts for Choughs during the winter season. Moreover, visiting the study site is necessary to notice important features such as the presence of alternative food sources or other factors influencing bird movements within and between days. However, the information provided by webcams about factors influencing flock size and presence of birds, such as temperature and number of tourists, can be used to plan fieldwork with regards to these factors.
In this pilot study, we tested the effectiveness of preinstalled webcams as a remote tool for eco-ethological research. We studied the effect of the presence of winter tourists and weather conditions on the flocking behaviour of a middle size corvid, the Alpine Chough (Pyrrhocorax graculus) and used the results as a proof of concept.
We focused on flock size and time spent at the site as dependent variables, and tested if they were correlated with the number of tourists and/or weather conditions (minimum temperatures and snowfall events). Results suggested that Alpine Choughs tended to concentrate opportunistically around restaurants when the number of tourists or the intensity of cold increased. Therefore, birds could modulate their presence at the feeding sites according to environmental variation that can be directly quantified from the same pictures. This tuning capability may suggest that the Alpine Choughs frequenting high elevation resorts in winter have developed addiction to anthropogenic food, raising concerns about the long term effects on adaptation processes and on preservation of populations. To better understand the effect of this kind of food, future research should compare reproductive outputs (offspring weight and survival, for instance) of supplemented and not supplemented populations. Moreover, given that the effect of anthropogenic food may depend on the type of food provisioned (Plummer at al. 2018), specific analyses of nutritional profiles should also be planned. The results obtained suggest that preinstalled webcams have a few caveats, but they can be successfully employed in eco-ethological research, on condition that the use of remotely collected data is combined with prior and simultaneous fieldwork, thus allowing the evaluation of data in the light of more precise and contextual information recorded in the field. Using machine learning algorithms can also make counting of birds and people more efficient (e.g., Miele et al., 2021), given that person identification is not possible so that privacy issues are not a concern.
As pointed out before, Panomax at the time of the data collection for the present study had about 360 webcams around the world: whether other companies operate similar systems they represent a huge potential resource. If these companies could be engaged to make some slight adjustments, without compromising their goals, such as installing additional webcams or directing the existing ones towards roosting sites, then this could offer a new branch of research.
The raw data supporting the conclusions of this article will be made available by the authors, without undue reservation.
Ethical review and approval was not required for the animal study because the study is based on pictures taken by already installed webcams and did not rely on any direct manipulations of the animals.
CV and AD conceived the ideas and designed the methodology. CV collected the data and NGY analysed the data. CV, NGY, AR and AD led the writing of the manuscript, contributed critically to the drafts and all authors gave final approval for publication.
The authors declare that the research was conducted in the absence of any commercial or financial relationships that could be construed as a potential conflict of interest.
All claims expressed in this article are solely those of the authors and do not necessarily represent those of their affiliated organizations, or those of the publisher, the editors and the reviewers. Any product that may be evaluated in this article, or claim that may be made by its manufacturer, is not guaranteed or endorsed by the publisher.
We thank Panomax for having allowed us to use webcam data to realize the study and Iacun Prugger who provided useful information about Alpine Chough movements in the Italian site. Dan Chamberlain checked the English and provided useful comments and suggestions on a first draft of the paper.
The Supplementary Material for this article can be found online at: https://www.frontiersin.org/articles/10.3389/fenvs.2021.659521/full#supplementary-material.
Acquarone, C., Cucco, M., Cauli, S. L., and Malacarne, G. (2002). Effects of Food Abundance and Predictability on Body Condition and Health Parameters: Experimental Tests with the Hooded Crow. Ibis 144, E155–E163. doi:10.1046/j.1474-919X.2002.t01-2-00094_1.x
Arlettaz, R., Nusslé, S., Baltic, M., Vogel, P., Palme, R., Jenni-Eiermann, S., et al. (2015). Disturbance of Wildlife by Outdoor winter Recreation: Allostatic Stress Response and Altered Activity-Energy Budgets. Ecol. Appl. 25, 1197–1212. doi:10.1890/14-1141.1
Barton, K. (2020). MuMIn: Multi-Model Inference. R package version 1.43.17. available at: https://CRAN.R-project.org/package=MuMIn.
Bridge, E. S., Schoech, S. J., Bowman, R., and Wingfield, J. C. (2009). Temporal Predictability in Food Availability: Effects upon the Reproductive axis in Scrub-Jays. J. Exp. Zool. 311A (1), 35–44. doi:10.1002/jez.493
Burnham, K. P., and Anderson, D. R. (2002). Model Selection and Multimodel Inference. A Practical Information-Theoretic Approach. Second Edition. New York: Springer-Verlag.
Chamberlain, D. E., Pedrini, P., Brambilla, M., Rolando, A., and Girardello, M. (2016). Identifying Key Conservation Threats to Alpine Birds through Expert Knowledge. PeerJ 4, e1723. doi:10.7717/peerj.1723
Chiffard, J., Delestrade, A., Yoccoz, N. G., Loison, A., and Besnard, A. (2019). Warm Temperatures during Cold Season Can Negatively Affect Adult Survival in an alpine Bird. Ecol. Evol. 9, 12531–12543. doi:10.1002/ece3.5715
Cramp, S., and Perrins, C. M. (1994). The Birds of the Western Palearctic, Vol. VIII. Crows to Finches. In Handbook of the Birds of Europe, the Middle East, and North Africa: The Birds of the Western Palearctic. Oxford University Press.
Delestrade, A. (1994). Factors Affecting Flock Size in the Alpine Chough Pyrrhocorax Graculus. Ibis 136, 91–96. doi:10.1111/j.1474-919X.1994.tb08135.x
Delestrade, A. (1995). Impact of Human Activity on Foraging Flocks and Populations of the Alpine Chough Pyrrhocorax graculus. Avocetta 19, 189–193.
Delestrade, A. (1999). Foraging Strategy in a Social Bird, the Alpine Chough: Effect of Variation in Quantity and Distribution of Food. Anim. Behav. 57, 299–305. doi:10.1006/anbe.1998.0951
Eichhorst, B. (2018). Internet Webcams Provide Opportunities for College Student Research on Animal Behavior and Ecology: An Example with Birds. Am. Biol. Teach. 80, 680–685. doi:10.1525/abt.2018.80.9.680
Ellenberg, U., Setiawan, A. N., Cree, A., Houston, D. M., and Seddon, P. J. (2007). Elevated Hormonal Stress Response and Reduced Reproductive Output in Yellow-Eyed Penguins Exposed to Unregulated Tourism. Gen. Comp. Endocrinol. 152, 54–63. doi:10.1016/j.ygcen.2007.02.022
Gelman, A., Hill, J., and Vehtari, A. (2020). Regression and Other Stories. Cambridge: Cambridge University Press. doi:10.1017/9781139161879
Gill, J. A. (2007). Approaches to Measuring the Effects of Human Disturbance on Birds. Ibis 149, 9–14. doi:10.1111/j.1474-919X.2007.00642.x
Hamilton, W. D. (1971). Geometry for the Selfish Herd. J. Theor. Biol. 31, 295–311. doi:10.1016/0022-5193(71)90189-5
Hodgson, J. C., Mott, R., Baylis, S. M., Pham, T. T., Wotherspoon, S., Kilpatrick, A. D., et al. (2018). Drones Count Wildlife More Accurately and Precisely Than Humans. Methods Ecol. Evol. 9, 1160–1167. doi:10.1111/2041-210X.12974
Jiménez, G., Meléndez, L., Blanco, G., and Laiolo, P. (2013). Dampened Behavioral Responses Mediate Birds' Association with Humans. Biol. Conservation 159, 477–483. doi:10.1016/j.biocon.2012.10.030
Jokimäki, J., Suhonen, J., Vuorisalo, T., Kövér, L., and Kaisanlahti-Jokimäki, M.-L. (2017). Urbanization and Nest-Site Selection of the Black-Billed Magpie ( Pica pica ) Populations in Two Finnish Cities: From a Persecuted Species to an Urban Exploiter. Landscape Urban Plann. 157, 577–585. doi:10.1016/j.landurbplan.2016.0800110.1016/j.landurbplan.2016.08.001
Kucera, T. E., and Barrett, R. H. (2011). “A History of Camera Trapping,” in Camera Traps in Animal Ecology. Editors A. F. O’Connell, J. D. Nichols, and K. U. Karanth (Tokyo: Springer).
Kurosawa, R., Kono, R., Kondo, T., and Kanai, Y. (2003). Diet of Jungle Crows in an Urban Landscape. Glob. Environ. Res. 7, 193–198. doi:10.1007/s10071-011-0439-9
Lehikoinen, A., Brotons, L., Calladine, J., Campedelli, T., Escandell, V., Flousek, J., et al. (2019). Declining Population Trends of European Mountain Birds. Glob. Change Biol. 25, 577–588. doi:10.1111/gcb.145210.1111/gcb.14522
Lesnoff, M., and Lancelot, R. (2012). Aod: Analysis of Overdispersed Data. R package version 1.3.1, URL:available at: http://cran.r-project.org/package=aod.
Lima, S. L. (1995). Back to the Basics of Anti-predatory Vigilance: the Group-Size Effect. Anim. Behav. 49, 11–20. doi:10.1016/0003-3472(95)80149-9
Miele, V., Dussert, G., Spataro, B., Chamaillé‐Jammes, S., Allainé, D., and Bonenfant, C. (2021). Revisiting Animal Photo‐identification Using Deep Metric Learning and Network Analysis. Methods Ecol. Evol. 12, 863–873. doi:10.1111/2041-210X.13577
Niedballa, J., Wilting, A., Sollmann, R., Hofer, H., and Courtiol, A. (2019). Assessing Analytical Methods for Detecting Spatiotemporal Interactions between Species from Camera Trapping Data. Remote Sens Ecol. Conserv. 5, 272–285. doi:10.1002/rse2.107
Oro, D., Genovart, M., Tavecchia, G., Fowler, M. S., and Martínez-Abraín, A. (2013). Ecological and Evolutionary Implications of Food Subsidies from Humans. Ecol. Lett. 16, 1501–1514. doi:10.1111/ele.12187
Pesaturo, J. (2018). Camera Trapping Guide: Tracks, Sign, and Behavior of Eastern Wildlife. Guilford, CT: Stackpole Books, 1–264.
Plummer, K. E., Bearhop, S., Leech, D. I., Chamberlain, D. E., and Blount, J. D. (2018). Effects of winter Food Provisioning on the Phenotypes of Breeding Blue Tits. Ecol. Evol. 8, 5059–5068. doi:10.1002/ece3.4048
R Core Team (2021). R: A Language and Environment for Statistical Computing. Vienna, Austria: R Foundation for Statistical Computing.
Rixen, C., and Rolando, A. (2013). The Impacts of Skiing and Related Winter Recreational Activities on Mountain Environments. Bussum, Netherlands: Bentham Books. doi:10.2174/97816080548861130101
Roberts, G. (1996). Why Individual Vigilance Declines as Group Size Increases. Anim. Behav. 51, 1077–1086. doi:10.1006/anbe10.1006/anbe.1996.0109
Rolando, A., Laiolo, P., and Carisio, L. (2003). Urbanization and the Flexibility of the Foraging Ecology of the Alpine Chough Pyrrhocorax Graculus in winter. Revue d'Ecologie (Terre Vie) 58, 337–352.
Rolando, A., Caldoni, R., De Sanctis, A., and Laiolo, P. (2001). Vigilance and Neighbour Distance in Foraging Flocks of Red-Billed Choughs, Pyrrhocorax Pyrrhocorax. J. Zoolog. 253, 225–232. doi:10.1017/S095283690100019X
Rolando, A., Caprio, E., Rinaldi, E., and Ellena, I. (2007). The Impact of High-Altitude Ski-Runs on Alpine Grassland Bird Communities. J. Appl. Ecol. 44, 210–219. doi:10.1111/j.1365-2664.2006.01253.x
Rolando, A., Laiolo, P., and Formica, M. (1997). A Comparative Analysis of the Foraging Behaviour of the Chough Pyrrhocorax Pyrrhocorax and the Alpine Chough Pyrrhocorax Graculus Coexisting in the Alps. Ibis 139, 461–467. doi:10.1111/j-1474-919X.1997.tb04659.x10.1111/j.1474-919x.1997.tb04659.x
Rolando, A., and Patterson, I. J. (1993). Foraging Behaviour and Diet of the Alpine ChoughPyrrhocorax Graculus in the Italian Alps in Summer. J. Ornithol. 134, 181–187. doi:10.1007/BF01640087
Schulwitz, S. E., Spurling, D. P., Davis, T. S., and McClure, C. J. W. (2018). Webcams as an Untapped Opportunity to Conduct Citizen Science: Six Years of the American Kestrel Partnership's KestrelCam. Glob. Ecol. Conservation 15, e00434. doi:10.1016/j.gecco.2018.e00434
Singh, J., and Bais, B. (2018). Wildlife Tracking with Latest Electronic Technology. Int. J. Avian Wildl. Biol. 3, 238–240. doi:10.15406/ijawb.2018.03.00093
Soininen, E. M., Jensvoll, I., Killengreen, S. T., and Ims, R. A. (2015). Under the Snow: a New Camera Trap Opens the white Box of Subnivean Ecology. Remote Sens Ecol. Conserv 1, 29–38. doi:10.1002/rse2.2
Storch, I., and Leidenberger, C. (2003). Tourism, Mountain Huts and Distribution of Corvids in the Bavarian Alps, Germany. Wildl. Biol. 9, 301–308. doi:10.2981/wlb.2003.018
Tibbetts, J. H. (2017). Remote Sensors Bring Wildlife Tracking to New Level. Biosciences 67, 411–417. doi:10.1093/biosci/bix033
Vallino, C., Caprio, E., Genco, F., Chamberlain, D., Palestrini, C., Roggero, A., et al. (2019). Behavioural Responses to Human Disturbance in an alpine Bird. J. Ornithol 160, 763–772. doi:10.1007/s10336-019-01660-z
Verstraeten, W. W., Vermeulen, B., Stuckens, J., Lhermitte, S., Van der Zande, D., Van Ranst, M., et al. (2010). Webcams for Bird Detection and Monitoring: a Demonstration Study. Sensors 10, 3480–3503. doi:10.3390/s100403480
West, E. H., and Peery, M. Z. (2017). Behavioral Mechanisms Leading to Improved Fitness in a Subsidized Predator. Oecologia 184, 787–798. doi:10.1007/s00442-017-3898-0
Keywords: European Alps, corvids, ecology, Pyrrhocorax graculus, wildlife remote detection, ski resort, tourist, webcams
Citation: Vallino C, Yoccoz NG, Rolando A and Delestrade A (2021) Webcams as a Remote Tool for Eco-ethological Research: A Study on the Alpine Chough. Front. Environ. Sci. 9:659521. doi: 10.3389/fenvs.2021.659521
Received: 27 January 2021; Accepted: 20 July 2021;
Published: 02 August 2021.
Edited by:
Mário Gabriel Santiago Santos, University of Trás-os-Montes and Alto Douro, PortugalReviewed by:
Martin Thomas Falk, University of South-Eastern Norway, NorwayCopyright © 2021 Vallino, Yoccoz, Rolando and Delestrade. This is an open-access article distributed under the terms of the Creative Commons Attribution License (CC BY). The use, distribution or reproduction in other forums is permitted, provided the original author(s) and the copyright owner(s) are credited and that the original publication in this journal is cited, in accordance with accepted academic practice. No use, distribution or reproduction is permitted which does not comply with these terms.
*Correspondence: Nigel Gilles Yoccoz, bmlnZWwueW9jY296QHVpdC5ubw==
Disclaimer: All claims expressed in this article are solely those of the authors and do not necessarily represent those of their affiliated organizations, or those of the publisher, the editors and the reviewers. Any product that may be evaluated in this article or claim that may be made by its manufacturer is not guaranteed or endorsed by the publisher.
Research integrity at Frontiers
Learn more about the work of our research integrity team to safeguard the quality of each article we publish.