- 1Department of Applied Ecology, Biological Faculty, Saint Petersburg State University, Saint-Petersburg, Russia
- 2Forest Research Institute–Bulgarian Academy of Sciences, Sofia, Bulgaria
The investigation conducted was dialed to quantitative and qualitative evaluation of 15 priority polycyclic aromatic hydrocarbons (PAHs) and Potentially Toxic Elements (Cu, Pb, Zn, Cd, Ni, and Cr) is soils and cryoconites on “St. Kliment Ohridski” Antarctic station territory and its vicinities. Estimation of benzo(a)pyrene (BaP)–equivalents, PAHs and different PAHs isomer pair ratios were used for identification of general toxicity, nature and origin of individual PAHs and their groups. Total concentrations of PAHs in BaP–equivalents showed, that ∑15PAH of all selected points was higher than the threshold concentration (20 μg × kg−1–Russian environmental legislation) for benzo(a)pyrene. Different PAHs isomer ratios showed the natural (petrogenic) source of PAHs at all soils examples (except Cryosol Toxic Transportic). The maximum content among potentially toxic elements was recorded for Zn (75.7 mg × kg−1 at L26), the minimum for Cd (0.201 mg × kg−1 at L1A). Average concentrations of potentially toxic elements are generally lower compared to the results of previous studies. Application of Igeo index, characterizes the majority of the studied soils as unpolluted or practically unpolluted. Data obtained indicates that there is no current critical anthropogenic load on the environmental components of the landscapes investigated.
Introduction
Nowadays the Antarctica is one of the most interesting and informative environmental model for evaluation of possible contaminants accumulation in pristine ecosystems. This territory was not intensively affected by human and therefore it can be used as reference area for adaptation and harmonization of threshold concentrations and evaluation of current contamination rates (Tin et al., 2009).
The challenges of nature conservation in Antarctica indicated in the Protocol on Environmental Protection (Madrid Protocol, 1998), faced with the necessity of regulation and implementation of environmental management in vicinities of the Antarctic stations with aim to decrease of hydrocarbons combustion effects and further accumulation of polycyclic aromatic compounds in the components of cryogenic ecosystems of Antarctica. In order to understand the amount of combusted fuel at the stations, data of the expedition of the Bulgarian Antarctic Institute in 2012–2013 can be exampled and taken into account for further evaluation. With aim to support the expedition, 5000 L of diesel liquid fuel were brought to the «St. Kliment Ohridski » station (December 14, 2012–February 25, 2013) (BAI, 2013). Other authors noted that the stations of the year-round stay (station Bellingshausen) can spend up to 150 000 L of diesel fuel per year (Abakumov et al., 2015).
The use of fossil fuels with current techniques leads to regular spilling of oil products that contaminate the surface and soils of terrestrial environments, inland and oceanic waters (Kennicutt et al., 1991; Waterhouse, 2001; Aislabie et al., 2004; Frenot et al., 2005). Numerous logistic aspects in Antarctic region result in waste disposal management, which often leads to the sporadic transportation of the untreated sewage into the ocean and on the territory around the stations (Connor, 2008; Tin et al., 2009; Martins et al., 2010).
Data obtained in previous researches showed that the large fuel spills from transport and tourist vessels have been occurring since the 80s of the 20th century. For example, in 1987 there was a spilling effect of 600,000 L diesel fuel near United States Palmer Station, Antarctic Peninsula (Aronson et al., 2011). The spills of hydrocarbon compounds and exhaust gases are the cause of chemical contamination the terrestrial Antarctic ecosystems, which is the most characteristic environmental impact of human activities in Antartica Chen and Blume (1997), Aislabie et al. (2004), Bargagli (2006) Several chemical and biological studies conducted recently have shown the presence of atypical chemical contaminants such as Potentially Toxic Elements (PTEs), Polychlorinated Biphenyl (PCB) Polycyclic Aromatic Hydrocarbons (PAHs) and Polychlorinated Dibenzodioxins (PCDDs) in soils, coastal waters, micro and macroorganisms (Snape et al., 2001; Negri et al., 2006; Hale et al., 2008; Martins et al., 2010; Abakumov et al., 2014; Abakumov et al., 2015; Pourret and Hursthouse, 2019).
Human and animal activities played an important role in PAHs distribution in the soil (Na et al., 2020). Among chemical pollutants, polycyclic aromatic hydrocarbons (PAHs) and trace metals have a special place, as they can enter the Antarctic not only through local discharges and emissions, but also through transboundary transfer from tropical and temperate regions of the southern hemisphere (Aislabie et al., 1999; Bargagli, 2006; Bargagli, 2008; Abakumov et al., 2014). Polycyclic aromatic hydrocarbons represent organic compounds of benzene series differing in the number and position of benzene rings showed mutagenic and carcinogenic effects. The higher molecular weight PAHs (MW > 202) with 4–6 benzene rings are frequently related to burning processes and are highly toxic to organisms due to their carcinogenic and mutagenic potential (Yunker et al., 2002; Yang et al., 2008; Martins et al., 2010). PAHs are known as mobile compounds that tend to be dispersed in the biosphere. There are PAHs of natural and anthropogenic origin that are included in the permanent monitoring list by the EU and United States Environmental Protection Agency (Baek et al., 1991; Nisbet and Lagoy, 1992; Howsam and Jones, 1998). In the Polar Regions with low temperatures, PAHs are less subjected to microbial degradation; therefore, they are deposited and preserved in soils and cryoconite (Lodygin et al., 2008; Hodson, 2014; Abakumov et al., 2015; Cook et al., 2016).
The proportion (isomer pair ratios) between concentrations of natural and anthropogenic PAHs can serve as an index of the anthropogenic influences of soil and can used as tracers to identify possible sources of PAH (Pandey et al., 1999; Yunker et al., 2002; Li et al., 2017).
Potentially Toxic Elements can be transported over long distances through the atmospheric circulation, and eventually deposited through dry and wet deposition to the Antarctic regions (Bargagli, 2006, 2008; Trevizani et al., 2018; Liu et al., 2021). They were determined in fossil fuels contaminated soils in different regions of Antarctica (Padeiro et al., 2016; Smykla et al., 2018; Alekseev and Abakumov, 2020; Gran-Scheuch et al., 2020). Also these elements have carcinogenic and mutagenic effects and can damage cellular membranes, proteins, enzymes, and DNA (Beyersmann and Hartwig, 2008; Ali et al., 2013; Padeiro et al., 2016).
The main goal of this work is to estimate the level of pollution of soils and cryoconites with potentially toxic elements and polycyclic aromatic hydrocarbons on the territory of the Bulgarian Antarctic Station “St. Kliment Ohridski” and its vicinity (Livingstone Island, South Shetland Islands, Antarctic Peninsula). In abovementioned context this research was aimed: 1) to estimate the concentrations of 15 PAHs and 6 potentially toxic elements, 2) to assess the contamination of soils with PAHs, their benzapyrene equivalents were calculated, as well as to calculate various isomeric ratios of PAHs, in order to identify their possible source of origin and 3) to assess the nature of soil contamination by potentially toxic elements, we calculated a geoaccumulation index (Igeo).
Materials and Methods
The study was performed on 11 soil and cryoconite samples taken at the Bulgarian Antarctic Station “St. Kliment Ohridski” on Livingston Island (region of Antarctic Peninsula, Western Antarctica) during the Bulgarian Antarctic expedition realized form December 21, 2019 till January 6, 2020. The precise coordinates of the sampling points and geographic description of the area are presented in Table 1.
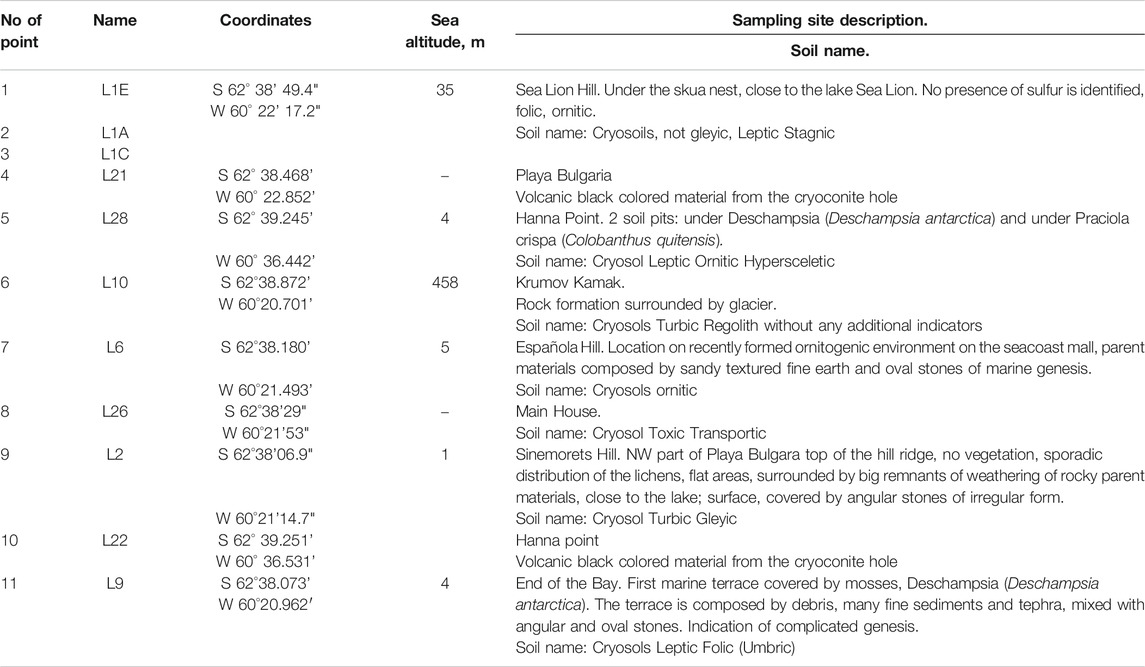
TABLE 1. Sampling points coordinates, geographic description and soil type of the study area (WRB, 2015).
The soils were collected from the 0–10 cm depth and cryconites from cryoconite holes, all samples were saved in polyethylene bags, then was transported to the station laboratory air dried, after what the artificial remnants and roots have been removed, soils have been sieved through 2 mm and transported to Saint-Petersburg State University laboratories in polyethylene bags.
The Livingston Island is locating at 62°38′29″S and 60°21′53″W (Figure 1). The “St. Kliment Ohridski” station situated 88 km southwest of station Bellingshausen (King George Island), 796 km southeast of Diego Ramirez Islands (the southernmost land of South America), 2,96 km of Spanish summer station “Juan Carlos I”, 28, 55 km from Chilean–United States station “Shirreff Base” (Ivanov, 2015).
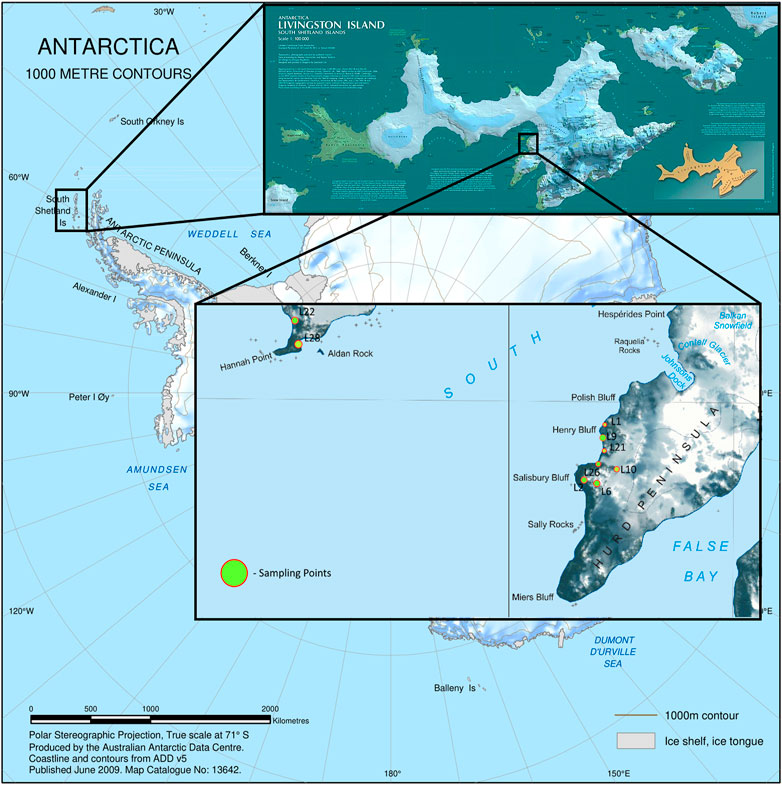
FIGURE 1. Location map of St. Kliment Ohridski station (AAD, 2009; Ivanov, 2015).
The first permanent (no wintering) station on Livingston Island was the Spanish one “Juan Carlos I” (62°39'46"S, 60°23'20"W), built from 7 to January 11, 1988 (Ivanov, 2015). Another scientific base on Livingston Island is the Chilean–United States facility Shirreff Base (62°28'12"S, 60°46'17"W), with its two sections named Guillermo Mann Base and Shirreff Field Station, and opened in 1990/91 and 1996/97, respectively, (CEP, 2011). The Bulgarian station appeared a few years later than the Spanish one. The “St. Kliment Ohridski” base was opened during the 1993/94-summer season on the Livingston Island. Infrastructure of station includes kitchen, messroom, living rooms, food storage, toilets and bathrooms.
All three bases are permanent settlements, although inhabited only during the summer season, with accommodation capacity for a total of 54 persons. In particular, most of the solid waste is shipped for dumping outside Antarctica, with incineration gradually phased out. As elsewhere in Antarctica, the island’s bases use electricity produced mostly by diesel generators (Ivanov, 2015).
The Geoaccumulation Index (Igeo) allows us to classify seven levels (Table 2) of soil contamination, from Practically unpolluted (Igeo ≤ 0) to Extremely polluted (Igeo> 5) (Muller, 1979; Jiang et al., 2019). The generic calculation formula is as follows:
where Cn–the measured concentration of the element in soil, Bn–the geochemical background value.
The content of Potentially Toxic Elements was determined following the standard ISO 11047–1998 “Soil Quality-Determination of Cadmium (Cd), Cobalt (Co), Copper (Cu), Lead (Pb), Manganese (Mg), Nickel (Ni), and Zinc (Zn) in Aqua Regia Extracts of Soil - Flame and Electrothermal Atomic Absorption Spectrometric” method at Atomic absorption spectrophotometer Kvant 2M (Moscow, Russia) (ISO, 1998).
The concentrations of 15 PAHs–naphthalene (NAP), acenaphthene (ANA), fluorene (FLU), phenanthrene (PHE), anthracene (ANT), fluoranthene (FLT), pyrene (PYR), benzo(a)anthracene (BaA), chrysene (CHR), benzo(b)fluoranthene (BbF), benzo(k)fluoranthene (BkF), 3,4-benzo(a)pyrene (BaP), dibenzo (a,h)anthracene (DBA), dibenzo (g,h,i)perylene (BPE), and indeno (1,2,3-c,d) pyrene (IPY) in soils and cryoconites on the territory of Bulgarian Antarctic base and its vicinities were determined on the basis of State Standard (GOST R 8.563–96), which is based on method Flyuorat-02-Panorama, Russia (GOST, 1996).
Extraction of PAHs was carried out at room temperature with a mixture of hexane-acetone (1:1) with ultrasonic treatment of the extraction system in an ultrasonic bath Branson 5510 (United States) (EPA, 2007). PAHs fraction was cleaned by using column chromatography on silica gel (SW, EPA, 1996). Qualitative and quantitative determination of PAHs in soils was carried out by the method of reversed-phase high-efficiency liquid chromatography in the gradient mode and spectrofluorometric detection on the chromatograph “Lumahrom” (“Lumex”, Russia). Chromatography was performed at a temperature of 30°C on a column of Supelco Supelcosil ™ LC-PAH 5 µm (25 cm × 2.1 mm). The acetonitrile–water gradient was used as a mobile phase. Sample volume of 10 µl was introduced with the help of a dosing crane. Individual PAHs were identified with chromatography mass spectrometry (spectrograph model: Shimadzu QP 5050A, Japan) (Gilichinsky et al., 2010). The detection limits of the studied PAHs are: NAP–16 μg × kg−1; ANA–1.5 μg × kg−1; FLU–1.4 μg × kg−1; PHE–2.0 μg × kg−1; ANT–0.3 μg × kg−1; FLT–6.7 μg × kg−1; PYR–6.7 μg × kg−1; BaA–1.5 μg × kg−1; CHR–0.8 μg × kg−1; BbF–1.4 μg × kg−1; BkF–0.3 μg × kg−1; BaP–0.3 μg × kg−1; DBA–1.8 μg × kg−1; BPE–1.6 μg × kg−1; IPY–6.7 μg × kg−1. Identification of PAHs was carried out by retention times and comparison of fluorescence spectra of components released the column with standard PAHs spectra. The quantitative analysis of PAHs was carried out using the external standard method. To assess the accuracy of the method, the above-described analytical procedure was subjected to the standard of bottom sediments containing surfactants (Standard Reference Material® 1944 New York/New Jersey Waterway Sediment -NIST, United States) (Agency, 1986; PND, 2003; Gabov et al., 2007; Gabov et al., 2008).
Statistical data processing and visualization was performed using Statsoft Statistica 12, GraphPad Prizm 9.0.0 and QGIS 3.16.
Results and Discussion
Concentration and Origin of PAHs
Some data on detected PAHs concentrations in soils of Livingstone Island (Bulgarian Antarctic Station “St. Kliment Ohridski” and its vicinity) are given in the Figure 2. It should be noted that at all sampling points (except L26) the differences in concentrations are minor. For example, naphthalene content in non-toxic soils types varies from 48 to 70 μg × kg−1, phenanthrene (PHE) from 20 to 28 μg × kg−1 (Figure 2). Concentrations of other PAHs in all natural soils are almost the same. Calculated index ∑15PAHs for all selected samples of natural soil ranged from 170 to 200 μg × kg−1. ∑7carcinogenicPAHs (CHR, BbF, BkF, BaP, DBA, BPE, IPY) is 37 μg × kg−1.
The following concentrations of PAHs in soils which are not subject to anthropogenic influence were noted in the earlier research in different regions of Antarctica. McMurdo Dry Valley (control site): NAP <30 μg × kg−1, ANA <30 μg × kg−1, PHE <30 μg × kg−1, FLU <30 μg × kg−1, ANT <30 μg × kg−1, FLT <30 μg × kg−1, PYR <30 μg × kg−1, BaA <30 μg × kg−1 (Aislabie et al., 1999). Admiralty Bay, King George Island: Ulmann Point ∑PAHs <10 μg × kg−1, Botany Point ∑PAHs <30 μg × kg−1, Monsimet Cove ∑PAHs <15 μg × kg−1 for some higher molecular PAHs (FLU, PYR, BaA, CHR, BkF, BbF, BaP, IPY, DBA, BPE) (Martins et al., 2010).
The content of PAHs in the vicinity of Russian Antarctic stations was studied by Abakumov et al. (2015); in these works, the list of identified PAHs is similar to our work. Haswell Archipelago: ∑15PAHs = 22.8 μg × kg−1. Hudson Mountains: ∑15PAHs = 33.9 μg × kg−1. Vicinity of Leningradskaya station ∑15PAHs = 21.7 μg × kg−1. The vicinities of Mirny station ∑15PAHs = 90.9 μg × kg−1 (Abakumov et al., 2015).
PAHs concentrations in Antarctica are often from soils and sediments located in highly contaminated areas where accidental spills have occurred, or close to research stations (Cabrerizo et al., 2012). On the Admiralty Bay, King George Island, Antarctica the maximum PAHs (high molecular PAHs (FLU, PYR, BaA, CHR, BkF, BbF, BaP, IPY, DBA, BPE)) concentration at Ferraz station occurred at 3.5 cm soil layer (454.9 μg × kg−1). The PAHs isomer pair ratio analysis showed that the major sources of PAHs are fossil fuels/petroleum (gasoline and diesel). The authors suggested that this may be related to activities at British station "G" in 1960s (established at the location of the Ferraz station). In areas of Admiralty Bay with less anthropogenic impact PAHs content is significantly lower (from 11.8 to 270.5 μg × kg−1) (Martins et al., 2004; Martins et al., 2010).
In the McMurdo Dry Valley region around Scott Base, Marble Point and the Wright Valley near Lake Vanda, ∑15PAHs (same with our PAHs list) content of fuel oil-contaminated soils ranged from 41 to 8105 μg × kg−1 of dried soil. At the Scott Base PAHs levels of 362 μg × kg−1 in drum storage site were detected in the 0–2 cm surface soil layer compared with 8105 μg×kg−1 in the 2–10 cm depth and 2543 μg × kg−1 in the deeper 20–30 cm layer. At McMurdo Dry Valley reference points PAHs concentrations ranged from 15 to 45 μg × kg−1 dry weight (Aislabie et al., 2004).
Previous investigations in anthropogenic loaded areas in Antarctica have reported PAHs levels in soils in the vicinity of stations Bellingshausen and Henryk Artstowski (King George Island, South Shetland Islands) and station Academician Vernadsky (Galindez Island, Argentinian Islands). At some points around the station Academician Vernadsky, the content of ∑15PAHs (same with our PAHs list) reaches 94771.2 μg × kg−1. The maximum level of contamination is observed near the aerology building and diesel station, the contribution of HMW PAHs exceeds 20%. At Bellingshausen station the maximum content of ∑15PAHs in soil at the diesel station is up to 911 μg × kg−1, with a higher contribution of HMW PAHs. In the vicinity of the Henryk Artstowski station ∑15PAHs content ranges from 114 to 188.3 μg × kg−1 (Abakumov et al., 2014; Abakumov et al., 2015).
Previously, investigations of PAHs content in different regions and climatic conditions have already been conducted. The values of ∑15PAHs (same with our list) ranged from 36.9 to 323 μg × kg−1 (dry weight) in soil (HMW PAHs prevailed), from 154 to 231 μg × kg−1 in moss, and from 48 to 333 μg × kg−1in reindeer dung at Ny-Ålesund, Svalbard in the Arctic (Wang et al., 2009).
For a comparative assessment, it is important to cite the results of some studies of PAH content in soils of anthropogenic-loaded urbanized areas. Investigations of ∑15PAHs (same with our PAHs list) content in soils of urbanized areas show the following results: Ljubljana 218–4,490 μg × kg−1, Torino 148–3,410 μg × kg−1, while soil samples from Glasgow show very high values 1490–5,1800 μg × kg−1. Analyzed PAHs ratios indicated pyrogenic origin of chemicals as result of anthropogenic contribution (Morillo et al., 2007). The range concentrations of Σ16PAHs [16 USEPA priority PAHs (identical to our list, but also includes Acenaphtylene)] in urban soils in Clay county, Ocala, Pensacola, and West Palm Beach were, respectively, 797–7909, 950–1,1451, 922–1,7698, and 1,133–3,0691 μg×kg−1. PAHs sources in urban soils of Pensacola were dominated by vehicle emissions, those in Ocala and West Palm Beach were dominated by biomass combustion, and those in Clay county were dominated by petrogenic sources (Gao et al., 2019). In soils of Vasilievsky Island in St. Petersburg were detected extremely high concentrations of PAHs. The concentration of Σ15PAHs (same with our PAHs list) is getting to the level 8,200 μg × kg−1. The maximum PAH concentrations were detected in the soil along the highway with heavy traffic and considerable emission of combustion gases (Lodygin et al., 2008). It is well seen that the PAH content in soils subjected to permanent anthropogenic load is significantly higher compared to almost all the results obtained for the Antarctic territories.
In our investigation at the point with the highest anthropogenic load (L26, main house) ∑15PAHs equals 445,1 μg × kg−1 (highest contribution: NAP 170 μg × kg−1, PYR 60 μg × kg−1, ANA 41 μg × kg−1). At the other points ∑15PAHs from 170 to 200 μg × kg−1 (Highest contribution: NAP 48–70 μg × kg−1, PHE 20–28 μg × kg−1). Compared with the studies mentioned above, it should be recognized that the anthropogenic load on the vicinities of Bulgarian Antarctic Station is not critical. It should be noted that station St. Kliment Ohridski is inhabited only in summer, unlike the other Antarctic stations (station Bellingshausen, station Academician Vernadsky, station Henryk Artstowski, station Scott Base, station Ferraz). This certainly has a positive effect on the anthropogenic impact on the vicinity of the station.
Based on the obtained data, it could be concluded that baseline sources for PAHs in Cryosols of Livingstone Island are found.
Particular attention should be paid to PAHs concentrations at the sampling point L26 (Hanna Point, Main House.) This point is located directly at the station “St. Kliment Ohridski”. The soil type here is referred to Cryosol Toxic Transportic (WRB, 2015). ∑15PAHs at this point is 455.1 μg × kg−1 (the main contribution is formed by: NAP 170 μg × kg−1, PYR 60 μg × kg−1, ANA 41 μg × kg−1, FLT 38 μg × kg−1, IPY 25.8 μg × kg−1, BaP 19 μg × kg−1 (Figure 2). ∑7carcinogenicPAHs is 92.8 μg × kg−1. Values of PAHs concentrations in the Toxic soil are much higher in comparison with the other studied natural soils.
Normally, PAHs can associate not only combustion processes but they are also found in sewage effluents, small oil spillages and natural sources including petroleum seeps (Martins et al., 2010). In order to identify the source of PAHs origin, different PAHs isomer pair ratios were calculated. This method of identification shows itself as a reliable and widely used in studies to identify the nature of the origin of PAHs (Yunker et al., 2002). Used PAH isomer pair ratios are shown in the Table 3.
Analyzing the results of PAHs isomer pair ratios calculations (Table 4) conclusions about the nature of PAHs origin in soils and cryoconites in the Bulgarian Antarctic Station “St. Kliment Ohridski” and its vicinity could be outlined.
The value of the ratio ∑PyrPAHs/∑15PAHs showed that all of the PAHs studied are results of petroleum spills, combustion processes or products of a baseline source. However, this indicator is too general and in order to better understand the processes of PAHs origin, it should rely on the results of previous ratio calculations. Also the ratio ∑ LMW PAHs/∑ HMW PAHs has shown that PAHs at all sampling points (except L21) have petrogenic origin.
The ANT/(ANT + PHE) value shows that all points except L26 have a natural origin (i.e. the Baseline source). At point L26 these PAHs are the result of the combustion processes. FLU/(FLU + PYR) ratio value characterize how as baseline source of this PAHs.
The results obtained by calculating the ratio BaA/(BaA + CHR) and IPY/(IPY + BPE) are of interest. Most often, the authors refer to PAHs as combustion products with results similar to ours (Yunker et al., 2002; Martins et al., 2010). However, Khaustov and Redina (2016) noted that the value of these ratios from 0,19 to 1,0 is typical for the background concentration of PAHs data in soils of northern and middle taiga (Khaustov and Redina, 2016). Since the climatic conditions of Livingstone Island are similar to those of the northern taiga, it should be recognized that the occurrence of these PAHs is linked more to natural than anthropogenic sources. Most sources use these two ratios to identify PAHs in locations with high anthropogenic load (Yan et al., 2006; Jiang et al., 2019; Gardes et al., 2020). Since there is no practice of burning wood fuel, coal and grass under Antarctic conditions, these two ratios have shown controversial results. Also, some researchers notes PAHs concentration in the particle phase was influenced significantly by temperature in the west Antarctic atmosphere (Na et al., 2020). The values of all other obtained isomeric ratios are well matched with the conditions of anthropogenic load and the possible influence of external factors on the nature of the origin of PAHs. The BaP/BPE value that all points except L26 have non-traffic source origin. From the PHE/ANT and FLU/PYR ratio value it can be seen that these PAHs at point L26 are formed as a result of pyrogenic processes, from other samples the values are characteristic for petrogenic processes of PAHs formation.
For cross-checking the nature of the origin of PAHs, bi-plots were constructed between some values of the obtained relations (Figure 3).
As can be seen in the figure above, the correlation values show high statistical correlation between each other (r2 > 0.66). In all the plotted figures, the soil sample L26, which was sampled directly in the territory of the Antarctic station, stands apart. All PAHs for this soil, which are shown on the visualized ratios, are of pyrogenic (combustion) origin. The existence of PAHs of pyrogenic origin is caused by the incinerator using the diesel fuel used to eliminate waste, as well as by the diesel electric generator that supplies the station with electric power.
BaP-Equivalence of PAHs Concentrations
Taking into account that not all chemical contaminants have maximum permissible concentration (MPC) in soils, especially regional MPCs for Antarctic continent, BaP-equivalence of PAHs concentrations were calculated. Since in the EU and the United States PAHs in various environments are controlled based on the calculation of human health risks, it was decided to use the standards adopted in Russian environmental legislation for convenience.
In Russia, the control of concentrations of various PAHs in the environment is based on monitoring 3-4 benzo(a)pyrene. According to the environmental protection laws, its maximum permissible concentration (MPC) in soil is 20 μg×kg−1 (Hygienic Norms, 2006). The methods of calculation of BaP-equivalents are well studied and have long been applied in studies of anthropogenic impact on natural territories (USEPA, 1993; Verbruggen et al., 2001; Jung et al., 2010). The method makes it possible to evaluate the effect of both the PAHs complex and each of them individually (Bari et al., 2010; Jennings, 2012).
BaP-equivalents values were calculated by simply multiplication of PAHs concentrations by Toxic Equivalent Factor (TEF) values (Nisbet and Lagoy, 1992). Benzo(a)pyrene was among the first chemical carcinogens identified more than 70°years ago. Many jurisdiction use TEFs applied to their BaP-equivalents to regulate other PAH (Loeb and Harris, 2008; Jennings, 2012; Shamilishvili et al., 2016).
The values of toxicity of investigated PAHs by 3-4 benzo(a) pyrene are presented on the Figure 4. As can be seen from the results obtained, for toxic soil (point L26) BaP-equivalents ∑15PAHs is 56.2 μg × kg−1, which is more than 2 times higher than the standards of Russian environmental legislation. The main contribution to BaP-equivalents ∑15PAHs is formed by: DBA 30.0 μg × kg−1, BaP 19.0 μg × kg−1, IPY 2.58 μg × kg−1. ∑7carcinogenicPAHs is 54.6 μg × kg−1, which is also more than 2 times higher than the Russian standard. It should be noted that the share of carcinogenic PAHs in BaP-equivalents is more than 90 percent.
For natural Antarctic soils the proportion of different PAHs in BaP – equivalents are significantly different from toxic soil sample. For almost all samples of natural soil ∑15PAHs in BaP-equivalents is close to 34 μg × kg−1. The main contribution in all cases is DBA 30 μg × kg−1, IPY 2 μg × kg−1, the remaining PAHs are significantly smaller. ∑7carcinogenicPAHs in BaP-equivalents for all natural soils is close to 33 μg × kg−1 with a DBA share of over 90%.
Taking into account that DBA concentrations in toxic and natural soils is a same (6 μg × kg−1), one might say that baseline source of DBA in BaP-equivalents in soils and cryoconites more than Russian MPC of 3-4 benzo(a)pyrene.
Content of Potentially Toxic Elements
Figure 5 shows the content of Potentially Toxic Elements in soils and cryoconites. Zn has the highest content of all metals. In the soil sample from the anthropogenic-loaded area (L26) the Zn concentration is 75.7 mg × kg−1. The lowest concentration was detected in sample L1A–16.5 mg × kg−1. High concentrations of Zn were recorded at L6 and L10, although soils in these areas are not subjected to anthropogenic load. As can be seen in the diagram presented in Figure 4, the variability of concentrations of other metals is significantly lower compared to zinc. The content does not change significantly. The minimum content among all Potentially Toxic Elements is observed for Cd, the maximum concentration is fixed in point L10–0.509 mg × kg−1.
Potential Toxic Elements in soils with anthropogenic load (Cryosol Toxic Transportic) following relation: Zn > Cu > Pb > Ni > Cr > Cd. Cryosol Ornitic: Zn > Ni > Cu > Pb > Cr > Cd. Typihical Cryosols and Cryoconites in most cases: Zn > Cu > Ni > Pb > Cr > Cd.
Previously, some results of studies of the content of potentially toxic elements in soils, cryoconites and ornithogenic sediments in the Antarctic Continent have already been published. In the cryoconites King George Island recorded the content of potentially toxic elements in the following order: Zn > Cu > Ni > Cr > Pb > Cd (Polyakov et al., 2020). In the anthropogenically loaded soils of Robert Island near The Chilean refuge Luis Risopatrón, the concentrations were in the following order Cr > Cu > Zn > Ni > Pb > Cd (Neto et al., 2017). Technosols from Hope Bay, Esperanza Station showed relation Pb > Zn > Cu > Ni > Cr > Cd. With an extremely high Pb content of 19381 mg × kg−1 and Cd content of 44 mg × kg−1 (Bueno Guerra et al., 2011). King George Island, Stranger Point, ornitoghenic soils near Gentoo penguin colonies, showed potentially toxic elements ratios: Cu > Zn > Pb > Cr > Ni > Cd, at a Cu concentration of 389.98 mg × kg−1 and a Cd concentration of 3.93 mg × kg−1 (Celis et al., 2015). Unfortunately, the soils of Livingston Island are poorly investigated in terms of heavy metals concentration, so below is a comparison with the results of few studies of soils of Livingston Island and King George Island, as it is similar in climate conditions and is a short distance to Livingston Island. Vlček et al. (2017) present data for pristine soils on Livingston Island (Hannah point area), which are similar to our results for undisturbed soils. The authors note the highest Zn concentration (55 mg × kg−1) in undisturbed soils, which is identical to our data (Vlček et al., 2017). The results for the other heavy metals are also similar. The investigations Santos et al. (2005); Vlček et al. (2017); Bueno et al. (2018) provide data on the content of trace metals both in pristine soils of King George Island and for anthropogenically loaded areas near the Antarctic stations. The data given by these researchers for pristine soils are generally similar to ours. For anthropogenically loaded territories, there are similarities in the content of Cu, Zn, and Pb. The average Zn 63.1, Cu 52.2, and Pb 6.3 mg × kg−1 were found in the soils of Artigas Antarctic Scientific Base (Bueno et al., 2018). For soils of Brazilian Antarctic Station Comandante Ferraz (Ferraz), data on average Zn concentrations of 52 mg/kg are given (Santos et al., 2005). We also recorded the maximum concentrations of Zn, Cu and Pb among all the studied metals in a soil sample taken on the territory of the station “St. Kliment Ohridski” (point L26).
Statistical analysis of the obtained data matrix of trace elements concentrations showed that most elements are in close correlation relationship (Table 5).
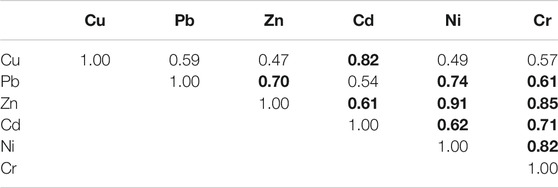
TABLE 5. Spearman Rank Order Correlations (r) between concentrations of potentially toxic elements (Bolded correlations are significant at p < 0.05).
Significant Spearman correlation coefficients (r > 0.61) were obtained practically for the elements. High correlation values between Ni and Zn (r − 0.91), Cr and Zn (r − 0.85), and between Cd and Cu (r − 0.82) are especially significant.
Classification of Soil Contaminations
Livingston Island is not well surveyed in terms of background concentrations of trace metals in the soil, so we used concentrations of trace metals in King George Island soils to calculate the geoaccumulation index (Igeo). King George Island is the closest large island to Livingston Island, they are very similar in climatic conditions and are part of the South Shetland Islands. There are many publications on heavy metal content in the pristine soils of King George Island (Amaro et al., 2015; Dalfior et al., 2016; Alekseev and Abakumov, 2020; Polyakov et al., 2020). Based on these studies, we calculated average values of heavy metal concentrations in pristine soils of the South Shetland Islands. The following values of the geochemical background value (Bn) were obtained: Cu–63, Pb–5.65, Zn–42.6, Cd–0.25, Ni–11.83, and Cr–22.95 mg × kg−1.
In most of the studied soils, the values of the Igeo for all the studied potentially toxic elements are less than zero (Igeo ≤ 0), which allows us to classify them as Practically unpolluted (Figure 6).
Values above zero are recorded at L28, L10, and L26 sampling points. At L28 point, the Igeo value for Cd is 3.46. This soil is characterized as Highly polluted (3 < Igeo ≤ 4). Also a high level of Cd content compared with background values was recorded at point L10, the values of Igeo index equal to 2.26. Cd contamination is characterized as Moderately to highly polluted (2 < Igeo ≤ 3). The highest values of the Igeo index are found at L26 point. Here Pb and Zn contamination is characterized as Highly polluted (3 < Igeo ≤ 4). Igeo values for Pb and Zn at point L26 are 3.85 and 3.82, respectively. It should be noted that the study area near point L26, is anthropogenically loaded, soil samples were taken directly in the territory of the Bulgarian Antarctic station. Bueno et al. (2018) reports data on soil contamination of Ferraz station (King George Island) based on Igeo index calculations. The level of contamination of Zn (noderatlely to highly polluted) and Cr (Moderately polluted) is identified (Bueno et al., 2018). It can be noted a similarity with the character of soil contamination on the territory of the station “St. Kliment Ohridski”.
Averaging the Igeo values for all points, the ratio of the pollution level is as follows Cr > Cu > Pb > Cd > Ni > Zn. With values for Cr and Zn equal to −0.32 and −1.94, respectively. The average values for all potentially toxic elements are characterized as Practically unpolluted (Igeo ≤ 0).
Data Availability Statement
The raw data supporting the conclusions of this article will be made available by the authors, without undue reservation.
Author Contributions
EA–field work, conceptualization, TN–laboratory analyzes, data processing, MG–writing manuscript, RY–field work, data processing. All authors have read and agreed to the published version of the manuscript.
Funding
This work was supported by Russian Foundation for Basic Research, Projects No 18–04–00900, 19–54–18003 and 19–05–50107.
Conflict of Interest
The authors declare that the research was conducted in the absence of any commercial or financial relationships that could be construed as a potential conflict of interest.
Acknowledgments
The authors are very grateful to the participants and organizers of the Russian and Bulgarian Antarctic National Expeditions. And Research park of Saint-Petersburg State University, Chemical Analysis and Materials Research Centre for assistance with routine soil analyses.
References
AAD (2009). Antarctica: 1000 Metre Contours. 1: 45 000 000. Australia: Australian Antarctic Division.
Abakumov, E., Lodygin, E., Gabov, D., and Krylenkov, V. (2014). [Polycyclic Aromatic Hydrocarbons Content in Antarctica Soils as Exemplified by the Russian Polar Stations]. Gig Sanit 1, 31–35.
Abakumov, E. V., Parnikoza, I. Y., Lupachev, A. V., Lodygin, E. D., Gabov, D. N., and Kunakh, V. A. (2015). [CONTENT OF POLYCYCLIC AROMATIC HYDROCARBONS IN SOILS OF ANTARCTIC STATIONS REGIONS]. Gig Sanit 94, 20–25.
Agency, U. S. E. P. (1986). SW-846 Test Method 8310: Polynuclear Aromatic Hydrocarbons. Washington, DC: US EPA.
Aislabie, J., Balks, M., Astori, N., Stevenson, G., and Symons, R. (1999). Polycyclic Aromatic Hydrocarbons in Fuel-Oil Contaminated Soils, Antarctica. Chemosphere 39 (13), 2201–2207. doi:10.1016/s0045-6535(99)00144-7
Aislabie, J. M., Balks, M. R., Foght, J. M., and Waterhouse, E. J. (2004). Hydrocarbon Spills on Antarctic Soils: Effects and Management. Environ. Sci. Technol. 38 (5), 1265–1274. doi:10.1021/es0305149
Alekseev, I., and Abakumov, E. (2020). The Content and Distribution of Trace Elements and Polycyclic Aromatic Hydrocarbons in Soils of Maritime Antarctica. Environ. Monit. Assess. 192 (11), 1–22. doi:10.1007/s10661-020-08618-2
Ali, H., Khan, E., and Sajad, M. A. (2013). Phytoremediation of Heavy Metals-Concepts and Applications. Chemosphere 91 (7), 869–881. doi:10.1016/j.chemosphere.2013.01.075
Amaro, E., Padeiro, A., de Ferro, A. M., Mota, A. M., Leppe, M., Verkulich, S., et al. (2015). Assessing Trace Element Contamination in Fildes Peninsula (King George Island) and Ardley Island, Antarctic. Mar. Pollut. Bull. 97 (1-2), 523–527. doi:10.1016/j.marpolbul.2015.05.018
Aronson, R. B., Thatje, S., McClintock, J. B., and Hughes, K. A. (2011). Anthropogenic Impacts on Marine Ecosystems in Antarctica. Ann. New York Acad. Sci. 1223 (1), 82–107. doi:10.1111/j.1749-6632.2010.05926.x
Baek, S., Field, R., Goldstone, M., Kirk, P., Lester, J., and Perry, R. (1991). A Review of Atmospheric Polycyclic Aromatic Hydrocarbons: Sources, Fate and Behavior. Water Air Soil Pollut. 60 (3-4), 279–300. doi:10.1007/bf00282628
BAI (2013). Exchange of Information in Accordance with Article VII (5) Bulgarian Antarctic Activities 2012/2013 [Online]. Sofia, Republica Bulgaria: Bulgarian Antarctic Institute Available: http://www.ats.aq/devAS/..%5Cdocuments%5Cie%5Cbgann13e.doc [Accessed].
Balmer, J. E., Hung, H., Yu, Y., Letcher, R. J., and Muir, D. C. G. (2019). Sources and Environmental Fate of Pyrogenic Polycyclic Aromatic Hydrocarbons (PAHs) in the Arctic. Emerging Contaminants 5, 128–142. doi:10.1016/j.emcon.2019.04.002
Bargagli, R. (2006). Antarctic Ecosystems: Environmental Contamination, Climate Change, and Human Impact. Berlin, Germany: Springer Science & Business Media.
Bargagli, R. (2008). Environmental Contamination in Antarctic Ecosystems. Sci. total Environ. 400 (1-3), 212–226. doi:10.1016/j.scitotenv.2008.06.062
Bari, M. A., Baumbach, G., Kuch, B., and Scheffknecht, G. (2010). Particle-phase Concentrations of Polycyclic Aromatic Hydrocarbons in Ambient Air of Rural Residential Areas in Southern Germany. Air Qual. Atmos. Health 3 (2), 103–116. doi:10.1007/s11869-009-0057-8
Beyersmann, D., and Hartwig, A. (2008). Carcinogenic Metal Compounds: Recent Insight into Molecular and Cellular Mechanisms. Arch. Toxicol. 82 (8), 493–512. doi:10.1007/s00204-008-0313-y
Budzinski, H., Jones, I., Bellocq, J., Pierard, C., and Garrigues, P. (1997). Evaluation of Sediment Contamination by Polycyclic Aromatic Hydrocarbons in the Gironde Estuary. Mar. Chem. 58 (1-2), 85–97. doi:10.1016/s0304-4203(97)00028-5
Bueno, C., Kandratavicius, N., Venturini, N., Figueira, R., Pérez, L., Iglesias, K., et al. (2018). An Evaluation of Trace Metal Concentration in Terrestrial and Aquatic Environments Near Artigas Antarctic Scientific Base (King George Island, Maritime Antarctica). Water Air Soil Pollut. 229 (12), 1–11. doi:10.1007/s11270-018-4045-1
Bueno Guerra, M. B., Schaefer, C. E. G. R., Rosa, P. d. F., Simas, F. N. B., Pereira, T. T. C., and Pereira-Filho, E. R. (2011). Heavy Metals Contamination in Century-Old Manmade Technosols of Hope Bay, Antarctic Peninsula. Water Air Soil Pollut. 222 (1-4), 91–102. doi:10.1007/s11270-011-0811-z
Cabrerizo, A., Dachs, J., Barceló, D., and Jones, K. C. (2012). Influence of Organic Matter Content and Human Activities on the Occurrence of Organic Pollutants in Antarctic Soils, Lichens, Grass, and Mosses. Environ. Sci. Technol. 46 (3), 1396–1405. doi:10.1021/es203425b
Celis, J. E., Barra, R., Espejo, W., Gonzalez-Acuna, D., and Jara, S. (2015). Trace Element Concentrations in Biotic Matrices of Gentoo Penguins (Pygoscelis Papua) and Coastal Soils from Different Locations of the Antarctic Peninsula. Water Air and Soil Pollution 226(1). doi:10.1007/s11270-014-2266-5
CEP (2011). Management Plan for Antarctic Specially Protected Area (ASPA) No. 149 CAPE SHIRREFF AND SAN TELMO ISLAND. LIVINGSTON ISLAND, SOUTH SHETLAND ISLANDS. Buenos Aires, Argentina.
Chen, J., and Blume, H. (1997). Impact of Human Activities on the Terrestrial Ecosystem of Antarctica: a Review. Polarforschung 65 (2), 83–92.
Chunhui, W., Shaohua, W., Shenglu, Z., Yaxing, S., and Jing, S. (2017). Characteristics and Source Identification of Polycyclic Aromatic Hydrocarbons (PAHs) in Urban Soils: a Review. Pedosphere 27 (1), 17–26. doi:10.1016/S1002-0160(17)60293-5
Connor, M. A. (2008). Wastewater Treatment in Antarctica. Polar Rec. 44 (2), 165–171. doi:10.1017/s003224740700719x
Cook, J., Edwards, A., Takeuchi, N., and Irvine-Fynn, T. (2016). Cryoconite. Prog. Phys. Geogr. Earth Environ. 40 (1), 66–111. doi:10.1177/0309133315616574
Dalfior, B. M., Roriz, L. D., Junior, R. F., de Freitas, A. C., da Silva, H. E., Carneiro, M. T. W., et al. (2016). Evaluation of Pb, Cd, Sn, Co, Hg, Mo and as in Soil from Fildes Peninsula-Antarctica. Quimica Nova 39 (8), 893–900. doi:10.21577/0100-4042.20160134
EPA, U. (2007). Method 3550C–Ultrasonic Extraction. Test. Methods Evaluating Solid Waste, Physical/chemical Methods 3, 1–17.
Fang, G., Wu, Y.-S., Chen, M.-H., Ho, T.-T., Huang, S.-H., and Rau, J.-Y. (2004). Polycyclic Aromatic Hydrocarbons Study in Taichung, Taiwan, during 2002?2003. Atmos. Environ. 38 (21), 3385–3391. doi:10.1016/j.atmosenv.2004.03.036
Frenot, Y., Chown, S. L., Whinam, J., Selkirk, P. M., Convey, P., Skotnicki, M., et al. (2005). Biological Invasions in the Antarctic: Extent, Impacts and Implications. Biol. Rev. 80 (1), 45–72. doi:10.1017/s1464793104006542
Gabov, D. N., Beznosikov, V. A., and Kondratenok, B. M. (2007). Polycyclic Aromatic Hydrocarbons in Background Podzolic and Gleyic Peat-Podzolic Soils. Eurasian Soil Sc. 40 (3), 256–264. doi:10.1134/s1064229307030039
Gabov, D. N., Beznosikov, V. A., Kondratenok, B. M., and Yakovleva, E. V. (2008). Formation of Polycyclic Aromatic Hydrocarbons in Northern and Middle Taiga Soils. Eurasian Soil Sc. 41, 1180–1188. doi:10.1134/s1064229308110069
Gao, P., Xu, M., Liu, Y., da Silva, E. B., Xiang, P., and Ma, L. Q. (2019). Emerging and Legacy PAHs in Urban Soils of Four Small Cities: Concentrations, Distribution, and Sources. Sci. Total Environ. 685, 463–470. doi:10.1016/j.scitotenv.2019.05.403
Gardes, T., Portet-Koltalo, F., Debret, M., Humbert, K., Levaillant, R., Simon, M., et al. (2020). Temporal Trends, Sources, and Relationships between Sediment Characteristics and Polycyclic Aromatic Hydrocarbons (PAHs) and Polychlorinated Biphenyls (PCBs) in Sediment Cores from the Major Seine Estuary Tributary, France. Appl. Geochem. 122, 104749. doi:10.1016/j.apgeochem.2020.104749
Gilichinsky, D., Abakumov, E., Abramov, A., Fyodorov-Davydov, D., Goryachkin, S., Lupachev, A., et al. (2010). Soils of Mid and Low Antarctic: Diversity, Geography, Temperature Regime. World Congress of Soil Science, Soil Solutions Q20 for a Changing World. Brisbane, Australia, 1–6.
GOST, R. (1996). R 8.563–96. The State System of Measurements: Methods of Performing Measurements (in Russian).
Gran-Scheuch, A., Ramos-Zuñiga, J., Fuentes, E., Bravo, D., and Pérez-Donoso, J. M. (2020). Effect of Co-contamination by PAHs and Heavy Metals on Bacterial Communities of Diesel Contaminated Soils of South Shetland Islands, Antarctica. Microorganisms 8 (11), 1749. doi:10.3390/microorganisms8111749
Hale, R. C., Kim, S. L., Harvey, E., La Guardia, M. J., Mainor, T. M., Bush, E. O., et al. (2008). Antarctic Research Bases: Local Sources of Polybrominated Diphenyl Ether (PBDE) Flame Retardants. Environ. Sci. Technol. 42 (5), 1452–1457. doi:10.1021/es702547a
Hodson, A. J. (2014). Understanding the Dynamics of Black Carbon and Associated Contaminants in Glacial Systems. WIREs Water 1 (2), 141–149. doi:10.1002/wat2.1016
Howsam, M., and Jones, K. C. (1998). Sources of PAHs in the Environment. in PAHs and Related Compounds (Berlin, Heidelberg: Springer), 137–174. doi:10.1007/978-3-540-49697-7_4
Hwang, H.-M., Wade, T. L., and Sericano, J. L. (2004). Destabilized Lysosomes and Elimination of Polycyclic Aromatic Hydrocarbons and Polychlorinated Biphenyls in Eastern Oysters (Crassostrea virginica). Environ. Toxicol. Chem. 23 (8), 1991–1995. doi:10.1897/03-467
Hygienic Norms, G. (2006). 2.1. 7.2041 06 “Maximum Permissi Ble Concentrations (MPC) of Chemical Substances in Soil. Approved by the Chief Sanitary Inspector of the Russian Federation on January 23.
ISO, S. Q. (1998). Determination of Cadmium, Chromium, Cobalt, Copper, Lead, Manganese, Nickel and Zinc in Aqua Regia Extracts of Soil. Flame and Electrothermal Atomic Absorption Spectrometric Methods. International Organization of Standardization. Geneva.
Ivanov, L. (2015). General Geography and History of Livingston Island. Sofia: Bulgarian Antarctic Research: a Synthesis. St. Kliment Ohridski University Press, 17–28.
Jennings, A. A. (2012). Worldwide Regulatory Guidance Values for Surface Soil Exposure to Carcinogenic or Mutagenic Polycyclic Aromatic Hydrocarbons. J. Environ. Manag. 110, 82–102. doi:10.1016/j.jenvman.2012.05.015
Jiang, F., Ren, B., Hursthouse, A., Deng, R., and Wang, Z. (2019). Distribution, Source Identification, and Ecological-Health Risks of Potentially Toxic Elements (PTEs) in Soil of Thallium Mine Area (Southwestern Guizhou, China). Environ. Sci. Pollut. Res. 26 (16), 16556–16567. doi:10.1007/s11356-019-04997-3
Jung, K. H., Yan, B., Chillrud, S. N., Perera, F. P., Whyatt, R., Camann, D., et al. (2010). Assessment of Benzo(a)pyrene-Equivalent Carcinogenicity and Mutagenicity of Residential Indoor versus Outdoor Polycyclic Aromatic Hydrocarbons Exposing Young Children in New York City. Ijerph 7 (5), 1889–1900. doi:10.3390/ijerph7051889
Kennicutt, M. C., Sweet, S. T., Fraser, W. R., Stockton, W. L., and Culver, M. (1991). Grounding of the Bahia Paraiso at Arthur Harbor, Antarctica. 1. Distribution and Fate of Oil Spill Related Hydrocarbons. Environ. Sci. Technol. 25 (3), 509–518. doi:10.1021/es00015a020
Khairy, M. A., Kolb, M., Mostafa, A. R., EL-Fiky, A., and Bahadir, M. (2009). Risk Assessment of Polycyclic Aromatic Hydrocarbons in a Mediterranean Semi-enclosed Basin Affected by Human Activities (Abu Qir Bay, Egypt). J. Hazard. Mater. 170 (1), 389–397. doi:10.1016/j.jhazmat.2009.04.084
Khaustov, A., and Redina, M. (2016). Indicator Ratio of Concentrations of Polycyclic Aromatic Hydrocarbons for Geoecological Studies of Environmental and Technical Objects. Geoekologiya Inzhenernaya Geol. Gidrogeol. Geokriol. 3, 220–233.
Li, Q., Kang, S., Wang, N., Li, Y., Li, X., Dong, Z., et al. (2017). Composition and Sources of Polycyclic Aromatic Hydrocarbons in Cryoconites of the Tibetan Plateau Glaciers. Sci. Total Environ. 574, 991–999. doi:10.1016/j.scitotenv.2016.09.159
Liu, K., Hou, S., Wu, S., Zhang, W., Zou, X., Yu, J., et al. (2021). Assessment of Heavy Metal Contamination in the Atmospheric Deposition during 1950-2016 A.D. From a Snow Pit at Dome A, East Antarctica. Environ. Pollut. 268 (Pt B), 115848. doi:10.1016/j.envpol.2020.115848
Lodygin, E. D., Chukov, S. N., Beznosikov, V. A., and Gabov, D. N. (2008). Polycyclic Aromatic Hydrocarbons in Soils of Vasilievsky Island (St. Petersburg). Eurasian Soil Sc. 41 (12), 1321–1326. doi:10.1134/s1064229308120107
Loeb, L. A., and Harris, C. C. (2008). Advances in Chemical Carcinogenesis: a Historical Review and Prospective. Cancer Res. 68 (17), 6863–6872. doi:10.1158/0008-5472.can-08-2852
Mandalakis, M., Tsapakis, M., Tsoga, A., and Stephanou, E. G. (2002). Gas-particle Concentrations and Distribution of Aliphatic Hydrocarbons, PAHs, PCBs and PCDD/Fs in the Atmosphere of Athens (Greece). Atmos. Environ. 36 (25), 4023–4035. doi:10.1016/s1352-2310(02)00362-x
Madrid Protocol. (1998). Protocol on Environmental Protection to the Antarctic Treaty, Madrid, 4 October 1991, 30 ILM 1461 (Accessed January 14, 1998).
Martins, C. C., Bícego, M. C., Rose, N. L., Taniguchi, S., Lourenço, R. A., Figueira, R. C. L., et al. (2010). Historical Record of Polycyclic Aromatic Hydrocarbons (PAHs) and Spheroidal Carbonaceous Particles (SCPs) in Marine Sediment Cores from Admiralty Bay, King George Island, Antarctica. Environ. Pollut. 158 (1), 192–200. doi:10.1016/j.envpol.2009.07.025
Martins, C. C., Bícego, M. C., Taniguchi, S., and Montone, R. C. (2004). Aliphatic and Polycyclic Aromatic Hydrocarbons in Surface Sediments in Admiralty Bay, King George Island, Antarctica. Antartic Sci. 16 (2), 117–122. doi:10.1017/s0954102004001932
Morillo, E., Romero, A. S., Maqueda, C., Madrid, L., Ajmone-Marsan, F., Grcman, H., et al. (2007). Soil Pollution by PAHs in Urban Soils: a Comparison of Three European Cities. J. Environ. Monit. 9 (9), 1001–1008. doi:10.1039/b705955h
Muller, G. (1979). Schwermetalle in den Sedimenten des Rheins: Veranderungen seit 1971 Umschau, 79, 778–783. (in German).
Na, G., Gao, Y., Li, R., Gao, H., Hou, C., Ye, J., et al. (2020). Occurrence and Sources of Polycyclic Aromatic Hydrocarbons in Atmosphere and Soil from 2013 to 2019 in the Fildes Peninsula, Antarctica. Mar. Pollut. Bull. 156, 111173. doi:10.1016/j.marpolbul.2020.111173
Negri, A., Burns, K., Boyle, S., Brinkman, D., and Webster, N. (2006). Contamination in Sediments, Bivalves and Sponges of McMurdo Sound, Antarctica. Environ. Pollut. 143 (3), 456–467. doi:10.1016/j.envpol.2005.12.005
Neto, E. D., Guerra, M. B. B., Thomazini, A., Daher, M., de Andrade, A. M., and Schaefer, C. (2017). Soil Contamination by Toxic Metals Near an Antarctic Refuge in Robert Island, Maritime Antarctica: A Monitoring Strategy. Water Air Soil Pollut. 228 (2). doi:10.1007/s11270-017-3245-4
Nisbet, I. C. T., and Lagoy, P. K. (1992). Toxic Equivalency Factors (TEFs) for Polycyclic Aromatic Hydrocarbons (PAHs). Regul. Toxicol. Pharmacol. 16 (3), 290–300. doi:10.1016/0273-2300(92)90009-x
Padeiro, A., Amaro, E., dos Santos, M. M. C., Araújo, M. F., Gomes, S. S., Leppe, M., et al. (2016). Trace Element Contamination and Availability in the Fildes Peninsula, King George Island, Antarctica. Environ. Sci. Process. Impacts 18 (6), 648–657. doi:10.1039/c6em00052e
Pandey, P. K., Patel, K. S., and Lenicek, J. (1999). Polycyclic Aromatic Hydrocarbons: Need for Assessment of Health Risks in India? Study of an Urban-Industrial Location in India. Environ. Monit. Assess. 59 (3), 287–319. doi:10.1023/a:1006169605672
PND, F. (2003). 16.1: 2: 2.2: 3.39-03. High Efficient Liquid Chro Matography Method to Determine the Mass Fraction of Benzo (A) Pyrene in Soils, Bottom Sediments, and Solid Wastes with a Lyumakhrom Liquid Chromatograph (in Russian).
Polyakov, V., Abakumov, E., and Mavlyudov, B. (2020). Black Carbon as a Source of Trace Elements and Nutrients in Ice Sheet of King George Island, Antarctica. Geosciences 10 (11). doi:10.3390/geosciences10110465
Pourret, O., and Hursthouse, A. (2019). It's Time to Replace the Term "Heavy Metals" with "Potentially Toxic Elements" when Reporting Environmental Research. Ijerph 16 (22), 4446. doi:10.3390/ijerph16224446
Ravindra, K., Wauters, E., and Van Grieken, R. (2008). Variation in Particulate PAHs Levels and Their Relation with the Transboundary Movement of the Air Masses. Sci. Total Environ. 396 (2-3), 100–110. doi:10.1016/j.scitotenv.2008.02.018
Santos, I. R., Silva-Filho, E. V., Schaefer, C. E. G. R., Albuquerque-Filho, M. R., and Campos, L. S. (2005). Heavy Metal Contamination in Coastal Sediments and Soils Near the Brazilian Antarctic Station, King George Island. Mar. Pollut. Bull. 50 (2), 185–194. doi:10.1016/j.marpolbul.2004.10.009
Shamilishvili, G., Abakumov, E., Gabov, D., and Alekseev, I. (2016). [Features of Fractional Composition of Polycyclic Aromatic Hydrocarbons and Multielement Contamination of Soils of Urban Territories and Their Hygienic Characteristics (On the Example of Soils of Functional Zones of Saint-Petersburg)]. Gig Sanit 95 (9), 827–837.
Smykla, J., Szarek-Gwiazda, E., Drewnik, M., Knap, W., and Emslie, S. D. (2018). Natural Variability of Major and Trace Elements in Non-ornithogenic Gelisoils at Edmonson Point, Northern Victoria Land, Antarctica. Polish Polar Res. 39 (1), 19–50. doi:10.24425/118737
Snape, I., Riddle, M. J., Stark, J. S., Cole, C. M., King, C. K., Duquesne, S., et al. (2001). Management and Remediation of Contaminated Sites at Casey Station, Antarctica. Polar Rec. 37 (202), 199–214. doi:10.1017/s0032247400027236
Soclo, H. H., Garrigues, P., and Ewald, M. (2000). Origin of Polycyclic Aromatic Hydrocarbons (PAHs) in Coastal Marine Sediments: Case Studies in Cotonou (Benin) and Aquitaine (France) Areas. Mar. Pollut. Bull. 40 (5), 387–396. doi:10.1016/s0025-326x(99)00200-3
Tin, T., Fleming, Z. L., Hughes, K. A., Ainley, D. G., Convey, P., Moreno, C. A., et al. (2009). Impacts of Local Human Activities on the Antarctic Environment. Antartic Sci. 21 (1), 3–33. doi:10.1017/s0954102009001722
Tobiszewski, M., and Namieśnik, J. (2012). PAH Diagnostic Ratios for the Identification of Pollution Emission Sources. Environ. Pollut. 162, 110–119. doi:10.1016/j.envpol.2011.10.025
Trevizani, T. H., Petti, M. A. V., Ribeiro, A. P., Corbisier, T. N., and Figueira, R. C. L. (2018). Heavy Metal Concentrations in the Benthic Trophic Web of Martel Inlet, Admiralty Bay (King George Island, Antarctica). Mar. Pollut. Bull. 130, 198–205. doi:10.1016/j.marpolbul.2018.03.031
U.S. EPA. Provisional Guidance for Quantitative Risk Assessment of Polycyclic Aromatic Hydrocarbons (PAH). Washington, DC: U.S. Environmental Protection Agency, Office of Research and Development, Office of Health and Environmental Assessment.
Verbruggen, E. M. J., Posthumus, R., and Van Wezel, A. (2001). Ecotoxicological Serious Risk Concentrations for Soil, Sediment and (Ground) Water: Updated Proposals for First Series of Compounds. doi:10.2118/68741-ms
Vlček, V., Juřička, D., and Míková, J. (2017). Heavy Metal Concentration in Selected Soils and Sediments of Livingston Island, Deception Island, King George Island, James Ross Island (Antarctica). Czech Polar Rep. 7 (1), 18–33.
Wang, Z., Ma, X., Na, G., Lin, Z., Ding, Q., and Yao, Z. (2009). Correlations between Physicochemical Properties of PAHs and Their Distribution in Soil, Moss and Reindeer Dung at Ny-Ålesund of the Arctic. Environ. Pollut. 157 (11), 3132–3136. doi:10.1016/j.envpol.2009.05.014
Waterhouse, E. J. (2001). Ross Sea region 2001: a state of the environment report for the Ross Sea region of Antarctica. Editor E. J. Waterhouse (Christchurch, NZ: New Zealand Antarctic Institute (Antarctica New Zealand)).
IUSS Working Group WRB (2015). World Reference Base for Soil Resources 2014, update 2015. International soil classification system for naming soils and creating legends for soil maps. World Soil Resources Reports No. 106. Rome: FAO.
Yan, B., Abrajano, T. A., Bopp, R. F., Benedict, L. A., Chaky, D. A., Perry, E., et al. (2006). Combined Application of δ13C and Molecular Ratios in Sediment Cores for PAH Source Apportionment in the New York/New Jersey Harbor Complex. Org. Geochem. 37 (6), 674–687. doi:10.1016/j.orggeochem.2006.01.013
Yang, Z., Feng, J., Niu, J., and Shen, Z. (2008). Release of Polycyclic Aromatic Hydrocarbons from Yangtze River Sediment Cores during Periods of Simulated Resuspension. Environ. Pollut. 155 (2), 366–374. doi:10.1016/j.envpol.2007.11.007
Yunker, M. B., Macdonald, R. W., Vingarzan, R., Mitchell, R. H., Goyette, D., and Sylvestre, S. (2002). PAHs in the Fraser River Basin: a Critical Appraisal of PAH Ratios as Indicators of PAH Source and Composition. Org. Geochem. 33 (4), 489–515. doi:10.1016/s0146-6380(02)00002-5
Keywords: livingston island, isomer ratios, trace metals, pollution assessment, geoaccumulation index, sources
Citation: Abakumov E, Nizamutdinov T, Yaneva R and Zhiyanski M (2021) Polycyclic Aromatic Hydrocarbons and Potentially Toxic Elements in Soils of the Vicinity of the Bulgarian Antarctic Station “St. Kliment Ohridski” (Antarctic Peninsula). Front. Environ. Sci. 9:656271. doi: 10.3389/fenvs.2021.656271
Received: 20 January 2021; Accepted: 12 April 2021;
Published: 29 April 2021.
Edited by:
Zhu Li, Institute of Soil Science (CAS), ChinaReviewed by:
Omar Richard Harvey, Texas Christian University, United StatesTatiana Minkina, Southern Federal University, Russia
Copyright © 2021 Abakumov, Nizamutdinov, Yaneva and Zhiyanski. This is an open-access article distributed under the terms of the Creative Commons Attribution License (CC BY). The use, distribution or reproduction in other forums is permitted, provided the original author(s) and the copyright owner(s) are credited and that the original publication in this journal is cited, in accordance with accepted academic practice. No use, distribution or reproduction is permitted which does not comply with these terms.
*Correspondence: Evgeny Abakumov, e_abakumov@mail.ru