- School of Earth and Environment, Anhui University of Science and Technology, Huainan, China
A total of 94 soil samples from different soil depths (0∼25 cm, 25∼50 cm) were collected of farmland soil around the plain of Beijing, and the concentrations of five heavy metal elements (Cd, Cr, Pb, As, and Hg) were measured using standard methods. The safety utilization evaluation method of heavy metals was based on three different evaluation methods. Then, the governance principles and specific management control strategy were determined in detail according to the core pollution source analysis of each safety grade zone. The results show that there are four different comprehensive safe utilization areas: safe, low-risk, medium-risk, and high-risk utilization. Among them, the study area was dominated by low-risk utilization areas, and the risk trend was gradually weakening from the center of the city to the periphery. Based on the characteristics of different security zones, this study puts on the governance principles of priority protection, long-term monitoring and moderate optimization, strengthens early warning, and cooperates with effective repair and priority governance. And then it puts forward practical control strategies according to the core pollution sources of each safety utilization zone. Our findings may provide a clear direction for rational utilization of land resources and renovation in the future.
Introduction
Soil is an organic part of the ecological environment, a natural environment on which mankind depends and also a natural resource for agricultural production. As an important line of defense for the protection of human health and environmental quality, soil quality is directly related to economic development, social progress, and people’s well-being (Ungureanu et al., 2017). With the rapid development of urbanization and industrialization in recent years, heavy metal contamination of soil has become more and more intense. Being ubiquitous, highly persistent, nonbiodegradable, and able to accumulate in soils at environmentally hazardous levels, heavy metal contamination directly causes serious harm to soil environment and indirectly damages human health through food intake and other related approaches (Mohammadi et al., 2018). According to a survey conducted by the Ministry of Agriculture of the People's Republic of China, 64.8% of the 1.4 million hm2 sewage-irrigated area in China suffers from heavy metal pollution. In view of that, scientific research on the status of heavy metal pollution in farmland soil is of great significance for agricultural production and soil safety (Zhang et al., 2014; Zhang et al., 2017).
At present, the related fields of soil heavy metals have led researchers to carry out several relevant studies. Most of the previous studies focused on the spatial distribution characteristics of soil heavy metals and the analysis of pollution sources (Manta et al., 2002; Da-wei and He-rong, 2017; Wang et al., 2019; Qu et al., 2020), the risk assessment of heavy metal pollution (Soå‚Ek-Podwika et al., 2016; Yan et al., 2016; Huang et al., 2018; Yang et al., 2018), and evaluation methods (Luo et al., 2017). However, there were few studies on the safety utilization of heavy metals from different soil depths.
Based on the monitored data, basic physicochemical properties of the soil, and related environmental factors, this essay took farmland soil around the plain of Beijing as the research object; analyzed the horizontal and vertical distribution characteristics of heavy metals (Cd, Cr, Pb, As, and Hg) in the soil of the study area; and used the geoaccumulation index, the improved geoaccumulation comprehensive index, and the potential ecological risk index and carried out the assessment of soil heavy metals. Then it puts forward a scheme for the regional division of the security of soil heavy metals. Furthermore, the core pollution sources of areas with different safety utilization grades were analyzed, and the governance principles and specific management control strategies of different grades of comprehensive utilization areas were determined. The research findings of this essay will provide scientific reference for the control of pollution risk of soil heavy metals and contribute to the efficient and safe utilization of soil.
Materials and Methods
Description of the Study Area
The study was conducted in an area of approximately 7779.12 km2 located in the southeast of Beijing. According to the digital elevation of Beijing, with the 100 m contour line as the boundary, the area with elevation ≤100 m was considered as the study area. The research area from north to south includes the water source protection zone (namely, Miyun, Pinggu, Huairou, and Changping), the agricultural security zone (namely, Shunyi, Tongzhou, Daxing, and Fangshan), and the urban living zone (Haidian, Shijingshan, Mentougou, Fengtai, and Chaoyang), a total of three functional areas and 13 districts and counties. The agricultural land planting patterns in the research area include many types, mainly grainfield, orchard, and vegetable land. Besides, fluvoaquic soil, cinnamon soil, and a small amount of paddy soil are the main soil types. Moreover, soil parent materials mainly include alluvial, proluvium, calcareous rock weathering, loess, and red-loess.
Sampling and Analysis
According to the distribution of agricultural land (grainfield, orchard, and vegetable plot) in the study area, a total of 94 samples of different planting patterns by setting different soil depths (0–25 cm, 25–50 cm) were collected, and the global positioning system (GPS) locator was applied to record its accurate coordinates and the attributes of the functional areas of the sampling points, planting patterns, soil types, and other basic attributes. A map indicating the field sampling points in the studied areas (sample locations) was given in Figure 1. In order to ensure the representativeness of the sample, soil samples were collected at the center point and two adjacent corners for each sample point, and the samples at the same depth were mixed to form the sample to be tested. The contact part of the sample with the metal sampling apparatus was removed in the field. After picking out visible impurities (such as the roots of the plants and debris), samples were naturally air-dried, crushed, and sieved for further analysis. Finally, elements including Pb, Cr, and Cd were detected by atomic absorption spectrometry, whereas As and Hg were detected by atomic fluorescence spectrometry.
Descriptive statistics analysis, coefficient of variation, and principal components analysis were carried out by using IBM SPSS software (version 21.0). The Empirical Bayesian Kriging (EBK) interpolation method in the ArcGIS 10.2 geostatistics module was applied to predict soil heavy metal samples data.
Evaluation Methods
Geoaccumulation Index
The geoaccumulation index method was proposed by Muller, a scientist at the University of Hamburg in Germany in 1969. It was used to quantitatively assess the degree of heavy metal pollution in sediments (Muller, 1969; Li et al., 2014). The calculation is
where
The background value of Beijing soil was used as the reference value (the background values of Cd, Cr, Pb, As, and Hg were 0.119, 29.8, 24.6, 7.09, and 0.08 mg·kg−1, respectively) (Jian, 1988; Chen et al., 2004). The classification standard of evaluation index was shown in Table 1.
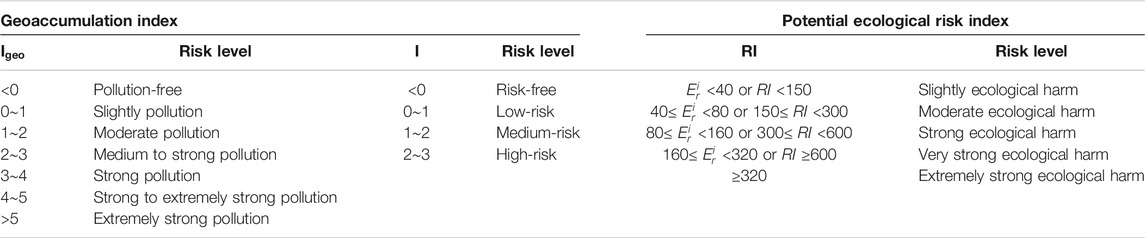
TABLE 1. The classification standard of evaluation index (Shi and Wang, 2013; Song et al., 2017).
In order to fully consider the different contribution ratios of each heavy metal element, to further compare the differences in the results of multifactor assessments, this study has made a certain degree of improvement based on the geoaccumulation index, has introduced the ecotoxicity of different heavy metals, and has calculated the weighted sum of the geoaccumulation index of different elements, so as to comprehensively analyze the environmental quality between elements or regions (Hakanson, 1980; Song et al., 2017). The calculation is
where
The classification standard of evaluation index was shown in Table 1.
Potential Ecological Risk Index
The potential ecological risk index method was proposed by the Swedish scientist Hakanson according to the characteristics and different effects of heavy metal elements on the environment. This method combined the ecological effects of heavy metals, environmental effects, and toxicology while considering the content of heavy metals in soil. It is an indicator that comprehensively reflects the potential of heavy metals on the ecological environment and is of great significance for the precontrol of soil environmental pollution (Liu W. et al., 2016; Janadeleh et al., 2018). The potential ecological risk index (RI) is calculated as follows:
where
The classification standard of evaluation index was shown in Table 1.
Results and Discussion
Analysis on the Characteristics of Heavy Metal Content in Soil
The basic descriptive statistics of the heavy metal concentrations analyzed in soil samples around the plain of Beijing were listed in Table 2 and Figure 2. In the soil depth of 0∼25 cm, the mean concentrations of Cd, Cr, Pb, As, and Hg were 0.124 ± 0.111 mg·kg−1, 34.793 ± 16.300 mg·kg−1, 8.776 ± 22.213 mg·kg−1, 7.936 ± 2.651 mg·kg−1, and 0.145 ± 0.165 mg·kg−1, respectively. Concentration levels of the measured elements in the soil samples, except Pb, were higher than the corresponding soil background values in Beijing, while the element of Hg was 1.8 times the background value. It can be seen that the economic development was accompanied by human activities which have caused the accumulation of heavy metals. The lower concentration of Pb may be due to the continuous increase of urban green coverage, which has a certain barrier and filter effect on heavy metals emitted by motor vehicles. In recent years, Beijing has adopted a large number of measures (such as new cylinder production technology and improved gasoline and diesel quality) to reduce the pollution of automobile exhaust gas which, to a certain extent, reduced the enrichment of Pb. The coefficient of variation (CV) of the heavy metals decreased in the order of Pb (2.531) >Hg (1.137) >Cd (0.895) >Cr (0.468) >As (0.334). CV for metals, except for Cr and As, was obtained as relatively high values. Besides, as can be seen from Figure 2 that the distribution of these three heavy metals was uneven and has great discreteness, the soil in this area was affected by exogenous Pb, Hg, and Cd, which was consistent with the research results of Chen Xiaolin et al. (Chen et al., 2012). In the soil depth of 25∼50 cm, the mean contents of Cd, Cr, Pb, As, and Hg were 0.087 ± 0.055 mg·kg−1, 34.806 ± 16.385 mg·kg−1, 8.012 ± 8.866 mg·kg−1, 7.894 ± 2.296 mg·kg−1, and 0.095 ± 0.094 mg·kg−1, respectively. Among them, Cr, As, and Hg were higher than the corresponding soil background values in Beijing; only the concentrations of Cr were higher than 0–25 cm, showing the double overlapping effect of man-made and natural geology. The CV of the heavy metals decreased in the order of Pb (1.107) >Hg (0.983) >Cd (0.633) >Cr (0.471) >As (0.291). The ranking of CV values was consistent with the soil depth of 0–25 cm.
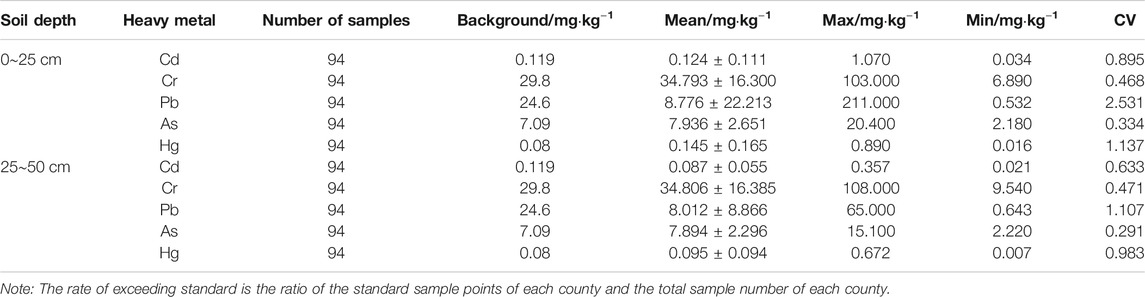
TABLE 2. Statistical descriptive of the heavy metal concentrations analyzed in soil samples in the study area.
Pearson correlation analysis was conducted to evaluate the relationships between the five heavy metals from different soil depths (Figure 3). The red lines in the upper triangle were fitted lines of the locally weighted scatterplot smoothing, the histograms in the diagonal indicated the data value distribution of the corresponding variables, and the correlation coefficients between different paired variables were shown in the lower triangles. The correlation coefficients among the five heavy metals (Cd, Cr, Pb, As, and Hg) from different soil depths (0∼25 cm, 25∼50 cm) were 0.674, 0.579, 0.290, 0.859, and 0.743, respectively, which showed that there were positive correlations among the five heavy metals from different soil depths. There had been a significantly (p < 0.01) positive correlation with Cr (0∼25 cm)-Hg (0∼25 cm, 25∼50 cm), Hg (0∼25 cm)-Pb(25∼50 cm), and Cd (25∼50 cm)-As (25∼50 cm) and a significant (p < 0.05) correlation with Cr (0∼25 cm, 25∼50 cm)-Pb(25∼50 cm), Pb(0∼25 cm)- Hg (25∼50 cm). These groups of heavy metals exhibited significant positive correlation, indicating that they generally had some homology or compound pollution, while negative correlation indicates that they may not had homology.
Classification of the Safety Utilization Zones of Heavy Metals in Soil
Based on the three evaluation methods of the geoaccumulation index, the improved geoaccumulation comprehensive index, and the potential ecological risk index, we put forward a scheme for the regional division of the security of soil heavy metals. The division scheme and its expression were shown in Table 3. The land was divided into four grades: Grade I (safe utilization zone), where the heavy metal concentrations and other pollutants in the soil were low, and the environmental quality keeps a clean state. In Grade Ⅱ (low-risk zone), there was a small amount of accumulation of heavy metals and other pollutants in the soil. The environmental quality was light pollution, and the soil and crops began to be contaminated. In Grade Ⅲ (medium-risk zone), the heavy metals and other pollutants in the region have been enriched significantly, the surrounding environment has suffered moderate pollution, and the soil and crops have been threatened to a certain degree. In Grade Ⅳ (high-risk zone), the heavy metal concentrations and other pollutants in this area were high, which was much higher than the relevant environmental quality standards, and will cause serious damage to soil, crops, and even human life and health.
According to the qualitative division scheme in Table 3, the comprehensive zoning of farmland soil safety and utilization in Beijing plain area was shown in Figure 4. It divided the 0∼25 cm farmland soil of the study area into three grades: low-risk zone (Grade Ⅱ), medium-risk zone (Grade Ⅲ), and high-risk zone (Grade Ⅳ). The low-risk area was about 4819.325 km2, accounting for 61.952% of the total area of the study area. It is mainly distributed in most areas outside the city center perimeter. The medium-risk area was approximately 2583.294 km2, accounting for 33.208% of the total area of the study area. It was mainly distributed around the urban area, the northern part of Miyun, the middle of Pinggu, and a small area in the southeast of Tongzhou. The high-risk area was about 376.500 km2, accounting for 4.840% of the total area of the study area. It was concentrated in Mentougou, Shijingshan, and parts of their borders with Haidian and Fengtai. Meanwhile, the study area in the soil depth of 25∼50 cm also can be divided into three grades: safe utilization area (Grade I), low-risk area (Grade II), and middle-risk area (Grade III). Among them, the safe utilization zone was about 6.563 km2, accounting for only 0.084% of the total area of the study area, located in the northern part of Huairou. The low-risk area was about 6518.052 km2, accounting for 83.789% of the total area of the study area. It was mainly distributed in most areas outside the city center perimeter. The medium-risk area was about 1255.399 km2, accounting for 16.138% of the total area of the study area. It was distributed in the areas surrounding the city center perimeter such as Haidian, Fengtai, Chaoyang, Mentougou, Shijingshan, and the middle part of Pinggu.
On the whole, the safety classification of heavy metals in most parts of the study area was low-risk. Since plastic films in agricultural land in Beijing was very common, there will be large amounts of plastic residues in the soil. In plastics, heavy metals were often used as stabilizers (Zheng et al., 2005a). Moreover, agricultural activities such as pesticides and chemical fertilizers increased heavy metals in the soil; for example, livestock breeders will put additives containing heavy metals in their feedstuffs, after which dung of livestock and poultry will be widely used in agricultural lands (Chen et al., 2005). As a result, heavy metals in the area will create a wide range of low-risk areas.
Analysis of Core Pollution Sources in Different Security Utilization Zones
Feature extraction was performed according to principal component analysis to further analyze the source of heavy metals in the study area. The load of principal component factors was obtained as shown in Table 4. Furthermore, since the heavy metal concentrations of each soil sample only represented the soil environment of the sample itself (Liu P. et al., 2016), therefore it was necessary to interpolate the information of samples to obtain surface information and then evaluate the spatial distribution of the five heavy metals. At the same time, combined with the results of the principal component analysis for the purpose of determining the source that caused heavy metal enrichment in the end, normal quantile plot of geoaccumulation index of different heavy metals and after Gaussian transformation were shown in Figure 5. The numerical distribution was approximately normal. Therefore, the normal distribution index interpolation analysis can be performed without the need of normal distribution transformation. The results were shown in Figure 6.
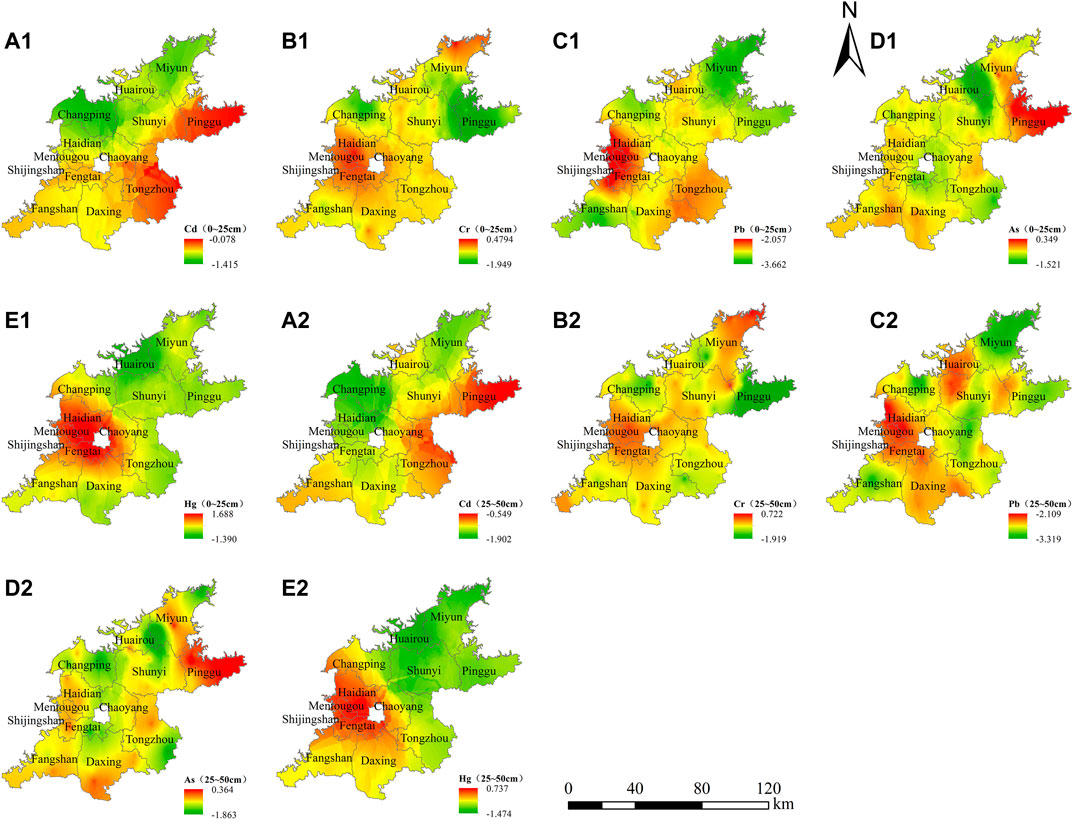
FIGURE 6. Spatial distribution of heavy metal evaluation results in different soil depths. (A1–E1) correspond to the spatial distribution of heavy metal (Cd, Cr, Pb, As, and Hg) evaluation results in 0–25 cm soil depths, while (A2–E2) corresponding to the spatial distribution of heavy metal (Cd, Cr, Pb, As, and Hg) evaluation results in 25–50 cm soil depths.
It can be seen from Table 4 that in 0∼25 cm soil depth the contribution rate of principal component 1 (PC1) was 27.935%, and the positive load of Cr and Hg was greater. Therefore, PC1 mainly reflected the enrichment information of Cr and Hg. With reference to Figure 6, we can see that the two heavy metals were concentrated on Mentougou, Shijingshan, and parts of their borders with Haidian and Fengtai (Figure 6b1, Figure 6b2). Mining was one of the most polluting sources of heavy metals in the soil of Mentougou now, and the massive accumulation of Hg was closely related to mining and transportation processes (Xing et al., 2016). In addition, Hg was often regarded as a sign element of burning coal and garbage burning. Shougang Group was located in Shijingshan district; the production process was accompanied by a large amount of oil, burning of coal and other fossil fuels, and the consumption of transportation vehicles, which will cause soil pollution in the area and even affect the surrounding Haidian and Fengtai (Zheng, et al., 2005a). There was a landfill site in Nankou Town in the western part of Changping where a large number of heavy metals (mainly Hg) will enter the atmosphere and migrate in the surrounding area for a long distance around it (Zheng et al., 2005b). In addition, the large amount of dust generated by mining will affect the environment as a result of the wind power. Long-term leaching of large amounts of coal gangue and tailings slag caused heavy metal elements (such as Hg and Cr) in the ore body which can be moved to the surrounding soil environment (Xing et al., 2016). For the soil heavy metal Cr, Xiaoni Huo proposed that the enrichment of Cr mainly comes from mining and sewage irrigation in the study of soil heavy metals in Beijing (Huo, et al., 2009). Therefore, PC1 represented industrial sources such as mining and coal combustion. The contribution of principal component 2 (PC2) was 20.718% and its load at the Cd content was higher, and Pb and Hg were negative load. The Cd element filled the Pinggu and Tongzhou regions (Figure 6a1), but it did not pollute the study area. Considering that all the samples in this study came from farmland soil, to some extent, unreasonable application of pesticides and chemical fertilizers in the actual agricultural production process will lead to the accumulation of Cd in the soil, and the heavy metal Cd may be derived from the above agricultural production activities. Therefore, PC2 can be expressed as the pollution of agricultural production activities such as pesticides and chemical fertilizers. The contribution rate of principal component 3 (PC3) was 20.238%, in which As had a high positive load and was mainly concentrated in the Pinggu area. It is generally known that As was usually regarded as the accompanying element of Au. Since the Wanzhuang gold ore was located in Pinggu, mining of the gold ore will activate the originally stable As in the area and then cause damage to the soil through the river system (Hu et al., 2013), which will cause the enrichment of As element for a long time. So, PC3 can be interpreted as the mining of gold mines.
At 25∼50 cm soil depth, the contribution rate of principal component 1 (PC1) accounted for 32.279% of the total variable, and heavy metals Hg and Pb had larger positive loads. Because they had a high coefficient of variation, and there were a few samples with high content and large degree of dispersion, it can be considered that it was mainly controlled by human factors. As can be seen from Figure 6, both Hg and Pb were concentrated around the urban area (Figure 6e2, Figure 6c2), in which heavy metal Pb did not cause pollution in the study area, while Hg elements caused a slight pollution risk in some areas near the urban area. As smelting of steel was the main industry in Shijingshan area, there was a large amount of vehicle transportation and fossil fuel combustion. In addition, the urban population was large and the density of motor vehicles was high; the emission of vehicle exhaust leads to the increase of Pb in the atmosphere and then atmospheric deposition causes its concentration to increase. Zheng Yuanming proposed that human activities such as atmospheric deposition, landfill, and pesticide application were the main factors leading to the accumulation of heavy metal Pb in Beijing (Zheng, et al., 2005b). Combined with the analysis of the pollution source of Hg element in 0∼25 cm soil, PC1 can be interpreted as pollution from coal burning, combustion of refuse, and vehicle emission from mining transportation. The contribution rate of the main component 2 (PC2) was 25.489%. Among them, Cd and As were loaded heavily. In addition to the accumulation of Cd in soil by using nitrogen, phosphorus, and potassium fertilizers, the wastewater, tailings, and residues produced in activities such as mining, mineral processing, and smelting would aggravate this element pollution. The main accumulation areas of the two heavy metals were mainly concentrated in Pinggu mining area (Figure 6a2, Figure 6d2), in which As produces mild pollution in this area. Meanwhile, combined with the analysis of the pollution source of As in 0∼25 cm soil, PC2 represented industrial activities such as mining. The contribution rate of the main component 3 (PC3) was 20.478%, and the load of only the heavy metal Cr was the highest. Studies have shown that it was less controlled by human activities, mainly affected by weathering and erosion of rocks (Zheng, et al., 2005c). The variation of Cr in 25∼50 cm soil was relatively small, and the heavy metals of Pb, Hg, and Cd, which were mainly affected by external sources, had lower loading in PC3. In summary, PC3 can be regarded as a natural source of soil parent material and weathering products.
The total contributions of the three principal components from different soil depths were 68.502% (0–25 cm) and 78.246% (25–50 cm), respectively. In general, the main sources of heavy metals in soils can be divided into natural sources (parent materials) and human activities. Among them, the source of human activities was the main reason for the accumulation of heavy metals in the area, such as mining, vehicle transportation, coal burning, garbage burning, and pesticide and fertilizer application.
Management and Control Strategy of Land Safety Utilization
According to the characteristics of different security zones, combined with the source apportionment results of soil heavy metal pollution in the study area, we proposed the rationalization proposals for the land of zoning utilization and management based on the principles of safety utilization, targeted governance and adjust measures to local conditions. The specific content was depicted in Table 5.
It can be seen from Table 5 that the safe utilization zone should be based on the principle of priority protection and strictly control the emergence of new sources of pollution in the surrounding areas so as to maintain the state of security in the region. Low-risk zone should be based on long-term monitoring and moderate optimization as the purpose of governance, reduce the intervention of known sources of pollution, and propose corresponding economically feasible measures. Ultimately, it makes the state of land in the region slowly transition to a safe state. Medium-risk zone should comply with the principle of strengthening early warning and cooperating with effective repair, focusing on the state of land in real time and strengthening early warning measures. At the same time, methods such as chemical leaching, phytoremediation, and biodegradation can be used to reduce the effectiveness of heavy metals in soil and crops. High-risk zone should take priority governance as the purpose of governance. At the same time, actively adopting restoration measures to protect human health from harm is needed. Ultimately, it was planned to increase the status of the land around the region by one grade after several repair cycles.
Conclusion
The three evaluation results of the geoaccumulation index, the improved geoaccumulation index, and the potential ecological risk index showed that most of the study areas were within the slight risk; the city center perimeter was the main enrichment zone for heavy metals, and its distribution showed a trend of weakening from the center of the city to the periphery. Meanwhile, as the urban living zones of Haidian, Shijingshan, Mentougou, Fengtai, and Chaoyang districts, whether the ecological risks brought by the heavy metal enrichment will cause harm to the health of the surrounding environment and the residents in the area should be worthy of attention. The main sources of heavy metals in the soil can be divided into natural sources and human activities, among which the anthropogenic source was the main reason for the accumulation of heavy metals. According to the scheme for the regional division of the security of soil heavy metals which we put, the land was divided into four types of zones: safe, low-risk, medium-risk, high-risk utilization. The results showed that the low-risk states dominated in the study area. Furthermore, in the light of various grades of core pollution sources, we took priority protection, long-term monitoring and moderate optimization, strengthened early warning and cooperated with effective repair, priority governance as a governance purpose to put forward specific management and control strategies.
This study took farmland soil around the plain of Beijing as the research object, putting forward a scheme for the regional division of the security of soil heavy metals based on the different evaluation methods. Future studies will incorporate more indicators to propose a more comprehensive and systematic land regionalization scheme and aim at the pollution characteristics of each area to provide more accurate, economical, and feasible remediation measures, making the research of more practical significance.
Data Availability Statement
The raw data supporting the conclusions of this article will be made available by the authors, without undue reservation.
Author Contributions
CK focused on the implementation of the main work, and SZ focused on the supplement and improvement after the completion of this research.
Funding
This work was supported by the National Key Research and Development Program of China (2016YFD0300801) and the National Natural Science Foundation of China (41471186).
Conflict of Interest
The authors declare that the research was conducted in the absence of any commercial or financial relationships that could be construed as a potential conflict of interest.
Acknowledgments
The authors would like to thank the local people for their cooperation and all those who helped us with this research.
References
Chen, T. B., Zheng, Y. M., Chen, H., Wu, H. T., Zhou, J. L., Luo, J. F., et al. (2005). Arsenic accumulation in soils for different land use types in Beijing. Geog. Res. 24 (2), 229–(235.) [in Chinese, with English summary]. doi:10.11821/yj2005020009
Chen, T. B., Zheng, Y. M., Chen, H., and Zheng, G. D. (2004). Background concentrations of soil heavy metals in Beijing. Huan Jing Ke Xue 25 (1), 117–(122.) [in Chinese, with English summary]. doi:10.13227/j.hjkx.2004.01.026
Chen, X. L., Yang, D., Hu, D. Q., Lian, D. Q., and Wang, X. D. (2012). Beijing city soil heavy metal distribution and evaluation: take inner 5th ring road area for an example. Environ. Sci. Technol. 35 (s2), 78–(81.) [in Chinese, with English summary].
Da-wei, H., and He-rong, G. (2017). Sources analysis and content characteristics of soil heavy metal in Sunan mining area, China. Earth Environ. 45 (5), 546–554. doi:10.14050/j.cnki.1672-9250.2017.05.008
Hakanson, L. (1980). An ecological risk index for aquatic pollution control. a sedimentological approach. Water Res. 14 (8), 975–1001. doi:10.1016/0043-1354(80)90143-8
Hu, Y., Zhou, L., and Wei, C. (2013). Study on spatial variability of soil heavy metals environments and its pollution characteristics in Beijing water protective area. Chin. J. Soil Sci. 44 (6), 1483–(1490.) [in Chinese, with English summary]. doi:10.1016/0043-1354(80)90143-8
Huang, D., Gui, H., Lin, M., and Peng, W. (2018). Chemical speciation distribution characteristics and ecological risk assessment of heavy metals in soil from Sunan mining area, Anhui province, China. Hum. Ecol. Risk Assess. 24 (6), 1694–1709. doi:10.1080/10807039.2017.1422973
Huo, X., Li, H., Zhang, W., Sun, D., Zhou, L., and Li, H. (2009). Multi-scale spatial structure of heavy metals in Beijing cultivated soils. Trans. Chin. Soc. Agric. Eng. 25 (3), 000223–(000229.) [in Chinese, with English summary].
Janadeleh, H., Jahangiri, S., and Kameli, M. A. (2018). Assessment of heavy metal pollution and ecological risk in marine sediments (a case study: Persian Gulf). Hum. Ecol. Risk Assess. 24 (8), 2265–2274. doi:10.1080/10807039.2018.1443792
Jian, L. (1988). The handbook of the environmental background value[M]: Beijing: China Environmental Science Press [in Chinese, with English summary].
Li, Z., Ma, Z., van der Kuijp, T. J., Yuan, Z., and Huang, L. (2014). A review of soil heavy metal pollution from mines in China: pollution and health risk assessment. Sci. Total Environ. 468–469, 843–853. doi:10.1016/j.scitotenv.2013.08.090
Liu, P., Wu, K., Luo, M., Li, C., Zhu, P., Zhang, Q., et al. (2016). Evaluation of agricultural land soil heavy metal elements exceed standards and safe utilization zones. Trans. Chin. Soc. Agric. Eng. 32 (23), 254–262. [in Chinese, with English summary]. doi:10.11975/j.issn.1002-6819.2016.23.035
Liu, W., Yang, J. J., Wang, J., Wang, G., and Cao, Y. E. (2016). Contamination assessment and sources analysis of soil heavy metals in opencast mine of East Junggar basin in Xinjiang. Huan Jing Ke Xue 37 (5), 1938–1945. [in Chinese, with English summary]. doi:10.13227/j.hjkx.2016.05.043
Luo, C., Bi, J., Xiao, G., and Zhang, F. (2017). Pollution characteristics and assessment of heavy metals in soil of different industry zones of Ningdong base in Ningxia, China. Ecol. Environ. Sci. 26 (7), 1221–1227. [in Chinese, with English summary]. doi:10.16258/j.cnki.1674-5906.2017.07.019
Manta, D. S., Angelone, M., Bellanca, A., Neri, R., and Sprovieri, M. (2002). Heavy metals in urban soils: a case study from the city of Palermo (Sicily), Italy. Sci. Total Environ. 300 (1), 229–243. doi:10.1016/s0048-9697(02)00273-5
Mohammadi, A., Hajizadeh, Y., Taghipour, H., Mosleh Arani, A., Mokhtari, M., and Fallahzadeh, H. (2018). Assessment of metals in agricultural soil of surrounding areas of Urmia Lake, Northwest Iran: a preliminary ecological risk assessment and source identification. Hum. Ecol. Risk Assess. 24 (8), 2070–2087. doi:10.1080/10807039.2018.1438173
Muller, G. (1969). Index of geoaccumulation in sediments of the Rhine river. Geojournal 2 (108), 108–118.
Qu, M., Chen, J., Huang, B., and Zhao, Y. (2020). Source apportionment of soil heavy metals using robust spatial receptor model with categorical land-use types and RGWR-corrected in-situ FPXRF data. Environ. Pollut. 270, 116220. doi:10.1016/j.envpol.2020.116220
Shi, Z. F., and Wang, L. (2013). Contents of soil heavy metals and evaluation on the potential pollution risk in Shenmu mining area. J. Agro-Environ. Sci. 32 (6), 1150–1158. [in Chinese, with English summary]. doi:10.11654/jaes.2013.06.010
Soå‚Ek-Podwika, K., Ciarkowska, K., and Kaleta, D. (2016). Assessment of the risk of pollution by sulfur compounds and heavy metals in soils located in the proximity of a disused for 20 years sulfur mine (SE Poland). J. Environ. Manage. 180, 450–458. doi:10.1016/j.jenvman.2016.05.074
Song, J. X., Zhu, Q., Jiang, X. S., Zhao, H. Y., Liang, Y. H., Luo, Y. X., et al. (2017). GIS-based heavy metals risk assessment of agricultural soils-a case study of Baguazhou, Nanjing. Acta Pedol. Sin. 54 (1), 81–91. [in Chinese, with English summary]. doi:10.11766/trxb201603100033
Ungureanu, T., Iancu, G. O., Pintilei, M., and Chicoș, M. M. (2017). Spatial distribution and geochemistry of heavy metals in soils: a case study from the NE area of Vaslui county, Romania. J. Geochem. Explor. 176, 20–32. doi:10.1016/j.gexplo.2016.08.012
Wang, S., Cai, L.-M., Wen, H.-H., Luo, J., Wang, Q.-S., and Liu, X. (2019). Spatial distribution and source apportionment of heavy metals in soil from a typical county-level city of Guangdong province, China. Sci. Total Environ. 655 (MAR.10), 92–101. doi:10.1016/j.scitotenv.2018.11.244
Xing, Y., Yan, G., Hou, Q., Sun, N., and Pan, X. (2016). Spatial distribution and pollution characteristics of heavy metals in soil of Mentougou mining area of Beijing city, China. J. Agric. Resour. Environ. 33 (6), 499–507. [in Chinese, with English summary]. doi:10.13254/j.jare.2016.0088
Xu, Z. Q., Ni, S., Tuo, X. G., and Zhang, C. J. (2008). Calculation of heavy metals’ toxicity coefficient in the evaluation of potential ecological risk index. Environ. Sci. Technol. 31 (2), 112–115. [in Chinese, with English summary]. doi:10.3969/j.issn.1003-6504.2008.02.030
Yan, W., Liu, D., Peng, D., Mahmood, Q., Chen, T., Wang, Y., et al. (2016). Spatial distribution and risk assessment of heavy metals in the farmland along mineral product transportation routes in Zhejiang, China. Soil Use Manage. 32 (3), 338–349. doi:10.1111/sum.12268
Yang, Q., Li, Z., Lu, X., Duan, Q., Huang, L., and Bi, J. (2018). A review of soil heavy metal pollution from industrial and agricultural regions in China: pollution and risk assessment. Sci. Total Environ. 642 (nov.15), 690–700. doi:10.1016/j.scitotenv.2018.06.068
Zhang, J., Ma, C., Kuang, H., and Zhou, A. (2017). Assessment of heavy metals pollution in soil of Qingdao based on matter-element extension model. China Environ. Sci. 37 (2), 661–668. [in Chinese, with English summary]. doi:10.3969/j.issn.1000-6923.2017.02.037
Zhang, X. M., Zhang, X. Y., Zhong, T. Y., and Jiang, H. (2014). Spatial distribution and accumulation of heavy metal in arable land soil of China. Huan Jing Ke Xue 35 (2), 692–703. [in Chinese, with English summary]. doi:10.13227/j.hjkx.2014.02.047
Zheng, Y., Chen, T., Chen, H., Zheng, G., and Luo, J. (2005b). Lead accumulation in soils under different land use types in Beijing city. Acta Geog. Sin. 60 (5), 791. doi:10.3321/j.issn:0375-5444.2005.05.010
Zheng, Y. M., Chen, T., Zheng, G. D., Huange, Z., and Lou, J. (2005c). Chromium and nickel accumulations in soils under different land uses in Beijing municipality. Resour. Sci. 27 (6), 162–166. [in Chinese, with English summary]. doi:10.3321/j.issn:1007-7588.2005.06.026
Keywords: farmland soil, heavy metals, core pollution sources, safety utilization, zoning, control strategy
Citation: Kong C and Zhang S (2021) Security Regional Division of Farmland Soil Heavy Metal Elements in North of the North China Plain. Front. Environ. Sci. 9:639460. doi: 10.3389/fenvs.2021.639460
Received: 09 December 2020; Accepted: 25 January 2021;
Published: 24 March 2021.
Edited by:
Chunhao Gu, University of Delaware, United StatesReviewed by:
Mingkai Qu, Institute of Soil Science (CAS), ChinaYingui Cao, China University of Geosciences, China
Wenji Zhao, Capital Normal University, China
Copyright © 2021 Kong and Zhang. This is an open-access article distributed under the terms of the Creative Commons Attribution License (CC BY). The use, distribution or reproduction in other forums is permitted, provided the original author(s) and the copyright owner(s) are credited and that the original publication in this journal is cited, in accordance with accepted academic practice. No use, distribution or reproduction is permitted which does not comply with these terms.
*Correspondence: Shiwen Zhang, bWFtaW4xMTkwQDEyNi5jb20=