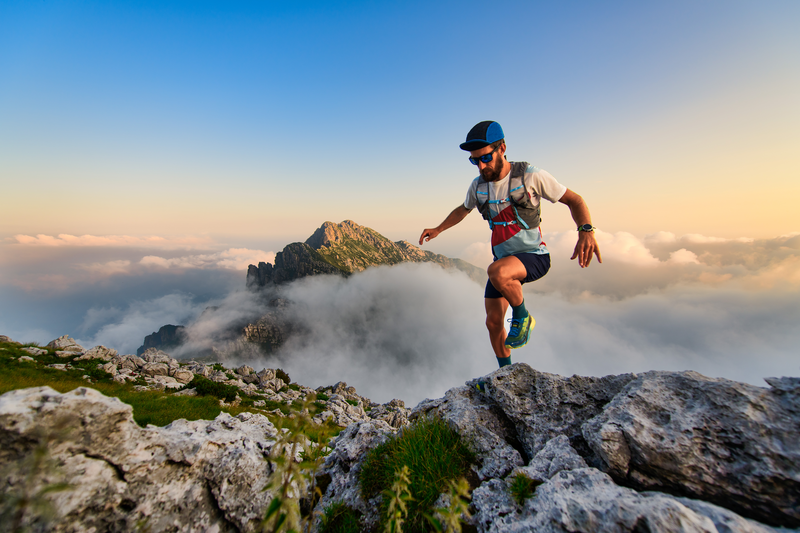
94% of researchers rate our articles as excellent or good
Learn more about the work of our research integrity team to safeguard the quality of each article we publish.
Find out more
MINI REVIEW article
Front. Environ. Sci. , 19 March 2021
Sec. Biogeochemical Dynamics
Volume 9 - 2021 | https://doi.org/10.3389/fenvs.2021.635114
This article is part of the Research Topic Nitrogen in the Environment View all 9 articles
Nitrogen (N) is the most critical element limiting agricultural production at a global scale. Despite many efforts, the N use efficiency (NUE) in agriculture remains in a range of less than 50%. Reaching targeted crop yields has resulted in N overuse, which is an economic and environmental concern worldwide. The continuous exploration of innovative solutions has led to the synthesis of novel nanomaterials, resulting in a powerful tool for the development of new technological products. Nanofertilizers are one of the most promising engineered materials that are being tested, either for soil or foliar applications. Encouraging results have been obtained using nanofertilizers in different plant species, however, limited information has been reported about its use in grasslands. Commonly, N is applied to grassland soils as granular fertilizers, which may result in significant losses via surface runoff or leaching, ammonia (NH3) volatilization and N oxides (N2O, NO, NOx) emissions. Nitrogen nanofertilizers are expected to increase NUE by improving the effectiveness of N delivery to plants and reducing N losses to the environment. Information on the efficiency of the use of N nanofertilizers in grasslands species is scarce and the application strategies that can be used to avoid N losses are poorly understood. New scenarios of increasing economic and environmental constraints may represent an opportunity for N nanofertilizers application in grasslands. This article reviews its potential use as an innovative approach to improve NUE and reduce N losses to the wider environment, analyzing potential shortcomings and future considerations for animal food chains.
The world population is estimated to exceed 9.7 billion by 2050 (FAO, 2018). Accordingly, it has been anticipated that current crop production needs to be increased by up to 70% to satisfy future food demands (Hunter et al., 2017). This great challenge will require combined efforts to preserve natural resources to support intensive agriculture while limiting detrimental impact on the environment (Lee et al., 2006; Hunter et al., 2017; Xie et al., 2019). The intensive use of mineral fertilizers and organic amendments has negatively affected soil and water quality worldwide (Bashir et al., 2020). In particular, the use of conventional N fertilizers has caused substantial N losses to the environment, triggering eutrophication of waters, soil acidification, and biodiversity loss (Banger et al., 2017).
Since the industrial revolution, the use of synthetic N fertilizers has led to the increase of atmospheric N2O, one of the most important anthropogenic greenhouse gases causing global warming (Davidson, 2009). Despite previous efforts, the Nitrogen Use Efficiency (NUE) in agricultural systems has remained low, meaning that on a global scale, more than 50% of the N applied to agricultural soils is potentially lost into the environment (Lassaletta et al., 2014). One of the major challenges of modern agriculture is to satisfy actual and future global food demands efficiently. The current NUE needs to be improved substantially by increasing the efficiency of agricultural systems, adopting environmentally sound agronomic practices, and exploring disrupting technologies.
Nanotechnology is the study, design, creation, synthesis, manipulation and application of nanometric scale materials, having one or more dimensions with sizes smaller than 100°nm (Lee and Moon, 2020). Nanomaterials differ from their original materials, and alterations in their physic-chemical properties are expected, acquiring exceptional properties, functionalities, and high reactivity given by its high surface area-volume ratio (Andrews et al., 2019). In the last few decades, nanotechnology has been considered a projecting technology with plentiful applications (Marchiol et al., 2020). A wide range of materials has been used to create nanoparticles like metal oxides, ceramics, magnetic materials, semiconductor, quantum dots, lipids, polymers (synthetic or natural), dendrimers and emulsions (Benelmekki, 2015; Kumar et al., 2018; Ruiz-Cañas et al., 2020). Accordingly, several nanotechnology applications have been developed and tested as potential agrochemicals such as bactericides, fungicides, growth regulators and fertilizers (Peters et al., 2014; Pestovsky and Martínez-Antonio, 2017). Nanofertilizers are defined as materials in the nanometer scale, usually in the form of nanoparticles, containing macro and micronutrients that are delivered to crops in a controlled mode (DeRosa et al., 2010; Adisa et al., 2019; Shang et al., 2019).
According to the type of formulation, nanofertilizers are classified into three categories: 1) nanoscale fertilizer, which corresponds to the conventional fertilizer reduced in size typically in the form of nanoparticles; 2) nanoscale additive fertilizer, is a traditional fertilizer containing a supplement nanomaterial; and 3) nanoscale coating fertilizer, refers to nutrients encapsulated by nanofilms or intercalated into nanoscale pores of a host material (Mastronardi et al., 2015). Encapsulated nutrients by films or held in nanopores within a carrier material such as clays have been used to form nanocomposite structures for controlling the nutrient release (Golbashy et al., 2016; Kottegoda et al., 2017; Borges et al., 2019; Tarafder et al., 2020).
Nanotechnology applications in agriculture appear to be a promising approach, fostering the transformation of conventional production systems into upgraded agricultural practices with a clear emphasis on the development of more efficient and environmentally friendly methodologies (Duhan et al., 2017; Lowry et al., 2019). Nanofertilizers could be a crucial development in the protection of the environment because they can be applied in smaller quantities compared to traditional fertilizers (Adisa et al., 2019), hence reducing leaching, runoff, and gas emissions to the atmosphere (Manjunatha et al., 2016). At present, uncertainty exists about the production costs of nanofertilizers compared to conventional fertilizers, as well as the magnitude of the possible disruption in the existing conventional fertilizer industry (Dimkpa and Brindraban, 2018).
A comprehensive analysis of the potential benefits of using nitrogenous nanofertilizer in grasslands and its impact on N losses to the environment has to be addressed, considering, among others, its agronomic and physiological properties, growing rates, plant architecture and use, compared to annual and/or perennial crops, given that in perennial crops nutrient carryover is usually seen from one season to the next one due to retranslocation from the annual plant parts, which can naturally increase NUE (Weih et al., 2011) Additionally, perennial crops tend to have longer photosynthetic seasons resulting from earlier canopy development and longer green leaf duration, increasing seasonal light interception efficiencies and precipitation interception (Tilman et al., 2009), including retention of potential foliar applications. There are important factors to assess when using these new formulations applied to grass, to improve NUE use efficiency and losses, like N molecules type and size, temperature, specific surface area, and urease activity, among other considerations (Bowman and Paul, 1992; Ryle and Stanley, 1992; Henning et al., 2013a). This review focuses on the potential use of N nanofertilizers as a novel approach to improve NUE in grasslands and their role in reducing environmental impact, with a focus on the decrease of N losses into the environment.
Nitrogen use efficiency (NUE) is commonly used to estimate the conversion of N inputs into agricultural products and to indicate the risk of N losses to the broader environment (Oenema et al., 2014; Norton et al., 2015). The NUE is considered an easy-to-use indicator applicable to agriculture and food production-consumption systems (EU Nitrogen Expert Panel, 2015). It is expressed as a ratio of outputs over inputs and can be estimated using a range of metrics, such as plant growth per unit of N applied (e.g. fertilizer and manure); meat or milk production per unit of animal N intake; N exported from a farm per unit of N imported; or N consumed in food per unit of N used to produce the food (de Klein et al., 2017). The NUE values have to be interpreted in relation to productivity (N output) and N surplus (i.e., the difference between N input and harvested N output). The NUE may allow decision makers to examine differences in NUE between farms, between specific systems, between countries, and between years. It also allows identifying technical progress and the efficiency of policy measures. As such, NUE can serve as a valuable indicator for monitoring sustainable development in relation to food production and environmental challenges (EU Nitrogen Expert Panel, 2015). This parameter can be also used to express the amount of N applied as fertilizer, which is harvested in crop or pasture (de Klein et al., 2017), varying mostly around 30–35%. Nitrogen use efficiency increases significantly by synchronizing, as much as possible, N availability supply with N demand (e.g. Maddux and Barnes, 1985). Nanomaterials, given they may provide a slow, steady, and time-dependent release of essential nutrients, including N, represent an opportunity to improve NUE, also reducing N leaching or losses as NH3 volatilization, in agreement with Preetha and Balakrishnan (2017). Furthermore, an increase of up to threefold as well as an improvement in productivity by promoting seed germination, seedling growth, N metabolism, photosynthetic activity, protein synthesis, antioxidant defense among other benefits (Iqbal et al., 2019).
Nitrogen could be easily lost to the wider environment. The main pathways of N losses in grassland are gaseous emissions (NH3 and N2O) and nitrate leaching and runoff (NO3− and organic N) (Cameron et al., 2013). Agriculture is recognized as a major source of atmospheric NH3, which has been associated with soil acidification, acid particulate matter and rainfall, odors (Aneja et al., 2009), and it is also indirectly linked to N2O and global warming (IPCC, 2015). As an example, agriculture is a key contributor to NH3 volatilization, representing the main source of NH3 emissions in different countries (Pan et al., 2016), averaging 31% of the N applied to crops and grasslands (Silva et al., 2017). Another important pathway of N loss is denitrification as N2O, which is a potent greenhouse gas (GHG) contributing to the depletion of the ozone layer (Matheyarasu et al., 2016). Worldwide, 12% of N2O losses are attributed to synthetic fertilizers applied to agricultural soils (IPCC, 2015).
It has been estimated that agriculture due to leaching and surface runoff of NO3− represents between 37 and 82% of the N input into surface waters of Western Europe (Isermann, 1990), where the livestock sector in this continent contributes 73% of water pollution for both N and P (Leip et al., 2015). Traditionally, N has been applied to crops and pastures as granular formulation, and integrated into the soil nutrients cycle, where it can be absorbed by plants, fixed by the soil components, or lost from the soil-plant system through different pathways (Jadon et al., 2018). There are only a few studies evaluating N losses on grass species, focusing mainly on NH3 losses (Henning et al., 2013b; Schlossberg et al., 2018), and NH3 and N2O loss (Alfaro et al., 2018). Foliar N application, using traditional or enhanced fertilizers dissolved in water, and more recently, the use of nanoformulations, has been evaluated to increase NUE (Dimkpa et al., 2020).
The increasing demand for food globally will require more productive systems that use suitable and reliable technologies, ensuring low environmental impact in terms of soil and water pollution (Jyothi and Hebsur, 2017). Many agricultural management practices such as split N application, N localization, precision farming, use of liquid formulations, foliar sprays, and liming applications have been proposed to improve NUE (Sharma and Bali, 2018; Egan et al., 2019) in annual crops such as potato (Souza et al., 2020) and mix crop-livestock systems (Ershadia et al., 2020). In the case of grasslands, several management options have been studied including the traditional 4R approach for the use of fertilizer application (Right source, Right time, Right rate, Right place) (Snyder, 2017), the optimization of plant combinations according to the final purpose of the animal system including the more efficient cultivars and species (Pijlman et al., 2020), and the integration of the soil-plant-animal system, considering more efficient grazing regimes and N inputs on animal feed and manures as a source of organic fertilizer (Oenema et al., 2014; de Klein et al., 2017). Fertilizers and manures NUE may vary between 50 and 80% depending on agro-climatic conditions, soil parameters, types of pastures, and other factors (Powell et al., 2010). Authors agree that in pasture-based systems major progress in eco-efficiency will be achieved through the implementation of tailor-made aspects associated with operational management. Málinas et al. (2020), concluded that a combination of forage mixture with lower to medium N inputs could significantly contribute to increase NUE from c. 50–86%, and from 45 to 53%, respectively, while providing sustainable long-term grass yields across managed grasslands.
Nanofertilizers have been projected as a tool to meet sustainable intensification criteria in agricultural activities in the next 30 years due to the feasibility of synchronizing the release mechanism of nutrients (N and phosphorus, P) with an increment in crop yields and forage production while reducing the fertilization inputs (Kalia et al., 2019). Nanofertilizers can boost NUE by enabling a slow and constant release of nutrients thus assisting nutrient plant uptake (Jyothi and Hebsur, 2017; Kalia and Sharma, 2019). It has been reported that the use of nanofertilizers can improve crop production by up to 30% compared with traditional chemical fertilizers (Kah et al., 2018); however, there are also studies showing no advantage to using nanofertilizers over conventional fertilizers (Kopittke et al., 2019).
Nanofertilizers can be classified in nanoscale fertilizers, nanoscale additives, and nanoscale coatings (Mikkelsen, 2018). The release of nutrients that are immobilized or/and encapsulated into a particular nanocarrier (biologic, chemical and physical) is activated by three different factors. The biological factors are bacteria, fungi and other microorganisms that biodegrade the coating based on a biodegradable or synthetic polymeric material, thus allowing the release of nutrients and its fixation into the soil. The chemical-triggered mechanisms are moisture, solubilization, pH variation, soil type (Weeks and Hettiarachchi, 2019; Ramzan et al., 2020), and ion exchange reactions (Ribeiro and Carmo, 2019). The physical factors are ultrasound, magnetic field, heat and diffusion-controlled release (Mikkelsen, 2018; Ribeiro and Carmo, 2019).
Nanostructured 2D clays can be considered good candidates to carry nutrients because they are ionic systems characterized by the presence of anions and cations that compensate each other, leading to neutrality (Lazaratou et al., 2020). The ionic nature of the clays as well as their ability to host a wide range of organic and inorganic ions in combination with an elevated ion exchange capacity, may render them suitable for controlled or slow nutrients release and thus increasing NUE (Table 1). For example, Layered Double Hydroxide (LDH) (Ureña-Amate et al., 2011; Berber et al., 2013; Koilraj et al., 2013; Borges et al., 2019; Jiang et al., 2019), montmorillonite (MMT) (Golbashy et al., 2016), and Zeolite ((Manjaiah et al., 2019) are good examples of a 2D nanostructure clay with promising opportunities as nanofertilizers for grasslands. Another suitable nanomaterial, due to its intrinsic nutrient capacity is the Hydroxyapatite (HT), a nanoparticle that can supply P in a faster and more prolonged manner (biphasic pattern) (Montalvo et al., 2015; Kottegoda et al., 2017). The LDH has also been reported to have the ability to uptake P and to provide a beneficial effect in the soil due to buffer properties (Torres-Dorante et al., 2009; Silva et al., 2014; Benício et al., 2016).
TABLE 1. Potential advantages of nanofertilizers as an alternative to increasing nitrogen use efficiency.
Because grassland ecosystems present unique features compared to traditional annual or perennial crops, the approach for applying nanofertilizers should follow a particular strategy (Figure 1), considering grass harvest by cattle and the different grazing patterns during the year, with short (c. 10–14 days) or long periods (c. 40–60 days) between grazing cycles in managed systems in temperate regions (Whitehead, 2000). Commonly, pastures are composed of a mixture of plant species, each with a different nutrient demand, with annual, biannual or perennial species participating in the grassland ecosystem. Thus, the growth pattern varies among grassland types and so does their nutrients demand (Blair et al., 2014). The pasture type depends on animal system needs, such as grazing oriented, forage conservation or both. Nanofertilizers could be applied to the soil or directly over the plant as foliar sprays (Mahil and Kumar, 2019). Soil applied nanofertilizers enable the movement of nutrients in the soil, facilitating its release and penetration into the roots. Controlled-release and slow-release nanofertilizers are used to supply nutrients in suitable concentration to plants over a prolonged interval of time, avoiding the continuous fertilizer application, and reducing the environmental risks (Jiang et al., 2019; Jia et al., 2020; Yoon et al., 2020).
FIGURE 1. Determining factors for the development of new fertilizer application strategies using nanofertilizers in pastures.
Reduction of N losses has been reported using N charged porous nanomaterials, such as zeolites (Manikandan and Subramanian, 2017), clays (Sarkar et al., 2014), or biodegradable polymers such as chitosan (Sharif et al., 2018). These could be related to a gradual release rate of NH4+ and/or NO3− , that in turn limits soil available N, which could potentially be lost to the environment in agreement with Cardenas et al. (2013). Foliar application, on the other hand, has proven to be useful to supply small quantities of fertilizer to the crops and it is especially beneficial in the correction of micronutrient deficiency, while in the case of macronutrients it can supplement soil conventional fertilization, particularly when soils have limiting factors (Fageria et al., 2009). Within foliar fertilizers, urea dissolved in water has been commonly used as the main N source for foliar application in perennial and annual crops, and to a lesser extent, in grassland species (Bowman and Paul, 1992; Stiegler et al., 2011; Ramírez-Rodríguez et al., 2020), however, N volatilization losses could be high, which could limit its use. Because nutrients in foliar applications are delivered directly to the target in small amounts, foliar fertilization is potentially more beneficial to the environment compared to traditional root treatments. Nevertheless, even if foliar nutrient application presents several advantages and has increasing importance in agriculture. Many mechanisms controlling the penetration of nutrients to the plant are not fully understood and plant response to foliar applications varies widely (Lv et al., 2019).
Although encouraging NUE results have been found when using nanofertilizers, some limitations and adverse effects have also been reported (Iqbal et al., 2019). Most of the research has also only been carried out at a laboratory scale (Kah et al., 2018). Examples of shortcomings in the use of foliar fertilizers are that they require an available leaf area to be effective, and may cause scorching or burning if the concentration of the spray is too high (Achari and Kowshik, 2018). They also require perfect timing for the application, since climatic conditions affect effectiveness. The costs of multiple applications can be too high to be profitable, the standardization of the nanoformulations, and lack of size uniformity of the nanoparticles (Iqbal, 2019), and optimizing foliar applications of nanofertilizers are challenges that need to be addressed in future research.
Further research is required to better understand the role of different N forms in supplying these molecules (i.e. NO3−, NH4+) and their impact on NUE in pastures, including N transformation within the plant and their effect on reducing N losses to the environment. Additionally, the integration of this technology with the use of N cycling inhibitors may represent an opportunity by creating favoring synergetic effects (Figure 1). More information is required to understand whether nanofertilizers are fully transformed into ionic forms in the plant and later incorporated into proteins and different metabolites, or if some of them remain intact and reach consumers through the food chain (Iqbal, 2019).
A better understanding of the advantages of nanomaterials is needed (Table 1), more and higher quality data is required on materials characterization, comprehensive comparisons with non-nano formulations, and field studies (Kah et al., 2018). Additionally, adapted nutrient fertilizer application strategies will need to be adjusted to take advantage of the benefits provided, to consider the inclusion of precision agriculture, such as drones fitted with cameras to gather multispectral images detecting N concentration in the grass within the paddock, avoiding over-application (e.g. urine patches), and repeated applications at low rates, with a potential increase in costs. All these factors will need to be addressed in an integrated manner (Figure 1) to account for potential downfalls and take full advantage of the opportunities and synergies the use of nanofertilizers may provide for the sustainable future of grassland production. The future development and adoption of these molecules as an answer for increasing food production with higher nutrient efficiency will need to balance economic and environmental costs of production with the potential reduction of environmental impact and yield increases. For this, a Life Cycle Assessment (LCA) may represent an opportunity for an integrated analysis of its use, considering yield productivity, environmental implications, and impact on food chains. The need to validate the pros and cons of nanofertilizers under representative field grasslands conditions to address the questions arising from stakeholders also remains as a pending task before the widespread adoption of this technology.
MA was the research leader for the FONDECYT Grant 1180775, and contributed to the manuscript, including NUE in grasslands concepts section of the manuscript, and contributed to the discussion, as well as the general editing of the manuscript. JM was a researcher on the nano fertilizer project and coordinated and contributed to manuscript conceptualization; writing-original draft preparation; writing-review and editing. FS was a researcher on the nano fertilizer project and was responsible for the N losses section of the manuscript and contributed to the discussion and editing of the manuscript. LPA led the manuscript sections on nanofertilizer types and the potential nanostructures used in pastures. SH participated in the manuscript sections on nanofertilizer types and the potential nanostructures that could be used in pastures. MR contributed to the general discussion and provided a practical overview of nanoformulations and their use in grasslands.
We would like to acknowledge Fondo Nacional de Desarrollo Científico y Tecnológico de Chile (FONDECYT), grant 1180775.
The authors declare that the research was conducted in the absence of any commercial or financial relationships that could be construed as a potential conflict of interest.
The authors would like to thank the Instituto de Investigaciones Agropecuarias-Chile.
Abd El-Azeim, M. M., Sherif, M. A., Hussien, M. S., Tantawy, I. A. A., and Bashandy, S. O. (2020). Impacts of nano- and non-nanofertilizers on potato quality and productivity. Acta Ecol. Sin. 40, 388. doi:10.1016/j.chnaes.2019.12.007
Abdel-Aziz, H. M. M., Hasaneen, M. N. A., and Omer, A. M. (2016). Nano chitosan-NPK fertilizer enhances the growth and productivity of wheat plants grown in sandy soil. Span J. Agric. Res. 14, e0902. doi:10.5424/sjar/2016141-8205
Achari, G. A., and Kowshik, M. (2018). Recent developments on nanotechnology in agriculture: plant mineral nutrition, health, and interactions with soil microflora. J. Agric. Food Chem. 66, 8647–8661. doi:10.1021/acs.jafc.8b00691
Adisa, I. O., Pullagurala, V. L. R., Peralta-Videa, J. R., Dimkpa, C. O., Elmer, W. H., Gardea-Torresdey, J. L., et al. (2019). Recent advances in nano-enabled fertilizers and pesticides: a critical review of mechanisms of action. Environ. Sci. Nano 6, 2002–2030. doi:10.1039/C9EN00265K
Alfaro, M., Salazar, F., Hube, S., Ramírez, L., and Mora, M. S. (2018). Ammonia and nitrous oxide emissions as affected by nitrification and urease inhibitors. J. Soil Sci. Plant Nutr. 18, 479–486. doi:10.4067/S0718-95162018005001501
Andrews, D., Nann, T., and Lipson, R. H. (2019). Comprehensive nanoscience and nanotechnology. Cambridge, MA: Academic Press.
Aneja, V. P., Schlesinger, W. H., and Erisman, J. W. (2009). Effects of agriculture upon the air quality and climate: research, policy, and regulations. Environ. Sci. Technol. 43, 4234–4240. doi:10.1021/es8024403
Banger, K., Yuan, M., Wang, J., Nafziger, E. D., and Pittelkow, C. M. (2017). A vision for incorporating environmental effects into nitrogen management decision support tools for U.S. Maize production. Front. Plant Sci. 8, 1270. doi:10.3389/fpls.2017.01270
Bashir, I., Lone, F. A., Bhat, R. A., Mir, S. A., Dar, Z. A., and Dar, S. A. (2020). Concerns and threats of contamination on aquatic ecosystems. in Bioremediation and Biotechnology, Sustainable Approaches to Pollution Degradation, Berlin, Germany: Springer. 1–26. doi:10.1007/978-3-030-35691-0_1
Benelmekki, M. (2015). “An introduction to nanoparticles and nanotechnology,” in Designing hybrid nanoparticles (San Rafael, CA: Morgan & Claypool Publishers), 1. doi:10.1088/978-1-6270-5469-0ch1
Benício, L. P. F., Constantino, V. R. L., Pinto, F. G., Vergütz, L., Tronto, J., and da Costa, L. M. (2016). Layered double hydroxides: new technology in phosphate fertilizers based on nanostructured materials. ACS Sustain. Chem. Eng. 5, 399. doi:10.1021/acssuschemeng.6b01784
Berber, M. R., Hafez, I. H., Minagawa, K., and Mori, T. (2013). A sustained controlled release formulation of soil nitrogen based on nitrate-layered double hydroxide nanoparticle material. J. Soils Sediments 14, 60–66. doi:10.1007/s11368-013-0766-3
Blair, J., Nippert, J., and Briggs, J. (2014). Grassland ecology. Ecol. Environ. 389–423. doi:10.1007/978-1-4614-7501-9_14
Borges, R., Wypych, F., Petit, E., Forano, C., and Prevot, V. (2019). Potential sustainable slow-release fertilizers obtained by mechanochemical activation of MgAl and MgFe layered double hydroxides and K2HPO4. Nanomaterials 9, 183. doi:10.3390/nano9020183
Bowman, D. C., and Paul, J. L. (1992). Foliar absorption of urea, ammonium, and nitrate by perennial ryegrass tryegrass turf. jashs 117, 75–79. doi:10.21273/JASHS.117.1.75
Cameron, K. C., Di, H. J., and Moir, J. L. (2013). Nitrogen losses from the soil/plant system: a review. Ann. Appl. Biol. 162, 145–173. doi:10.1111/aab.12014
Cardenas, L. M., Hatch, D. J., Scholefield, D., Jhurreea, D., Clark, I. M., Hirsch, P. R., et al. (2013). Potential mineralization and nitrification in volcanic grassland soils in Chile. Soil Sci. Plant Nutr. 59, 380–391. doi:10.1080/00380768.2013.789395
Chhowalla, M. (2017). Slow release nanofertilizers for bumper crops. ACS Cent. Sci. 3, 156−157. doi:10.1021/acscentsci.7b00091
Davidson, E. A. (2009). The contribution of manure and fertilizer nitrogen to atmospheric nitrous oxide since 1860. Nat. Geosci. 2, 156–157. doi:10.1038/ngeo608
de Klein, C. A. M., Monaghan, R. M., Alfaro, M., Gourley, C. J. P., Oenema, O., and Powell, J. M. (2017). Nitrogen performance indicators for dairy production systems. Soil Res. 55, 479. doi:10.1071/SR16349
DeRosa, M. C., Monreal, C., Schnitzer, M., Walsh, R., and Sultan, Y. (2010). Nanotechnology in fertilizers. Nat. Nanotechnol. 5, 91. doi:10.1038/nnano.2010.2
Dimkpa, C. O., and Bindraban, P. S. (2018). Nanofertilizers: new products for the industry? J. Agric. Food Chem. 66, 6462–6473. doi:10.1021/acs.jafc.7b02150
Dimkpa, C. O., Fugice, J., Singh, U., and Lewis, T. D. (2020). Development of fertilizers for enhanced nitrogen use efficiency - trends and perspectives. Sci. Total Environ. 731, 139113. doi:10.1016/j.scitotenv.2020.139113
Duhan, J. S., Kumar, R., Kumar, N., Kaur, P., Nehra, K., and Duhan, S. (2017). Nanotechnology: the new perspective in precision agriculture. Biotechnol. Rep. 15, 11–23. doi:10.1016/j.btre.2017.03.002
Egan, G., Mckenzie, P., Crawley, M., and Fornara, D. A. (2019). Effects of grassland management on plant nitrogen use efficiency (NUE): evidence from a long-term experiment. Basic Appl. Ecol. 41, 33. doi:10.1016/j.baae.2019.10.001
Eichert, T., Kurtz, A., Steiner, U., and Goldbach, H. E. (2008). Size exclusion limits and lateral heterogeneity of the stomatal foliar uptake pathway for aqueous solutes and water-suspended nanoparticles. Physiol. Plant 134, 151–160. doi:10.1111/j.1399-3054.2008.01135.x
Ershadi, S. Z., Dias, G., Heidari, M. D., and Pelletier, N. (2020). Improving nitrogen use efficiency in crop-livestock systems: a review of mitigation technologies and management strategies, and their potential applicability for egg supply chains. J. Clean. Prod. 265, 121671. doi:10.1016/j.jclepro.2020.121671
EU Nitrogen Expert Panel (2015). Nitrogen Use Efficiency (NUE) - an indicator for the utilization of nitrogen in agriculture and food systems. Wageningen, Netherlands: Wageningen University.
Fageria, N. K., Filho, M. P. B., Moreira, A., and Guimarães, C. M. (2009). Foliar fertilization of crop plants. J. Plant Nutr. 32, 1044–1064. doi:10.1080/01904160902872826
FAO (2018). The future of food and agriculture – Alternative pathways to 2050. Summary version. Rome, Italy, 60 Licence: CC BY-NC-SA 3.0 IGO. Available at: http://www.fao.org/3/CA1553EN/ca1553en.pdf.
Golbashy, M., Sabahi, H., Allahdadi, I., Nazokdast, H., and Hosseini, M. (2016). Synthesis of highly intercalated urea-clay nanocomposite via domestic montmorillonite as eco-friendly slow-release fertilizer. Arch. Agron. Soil Sci. 63, 84. doi:10.1080/03650340.2016.1177175
Henning, S. W., Branham, B. E., and Mulvaney, R. L. (2013b). Response of turfgrass to urea-based fertilizers formulated to reduce ammonia volatilization and nitrate conversion. Biol. Fertil. Soils 49, 51–60. doi:10.1007/s00374-012-0696-z
Henning, S. W., Mulvaney, R. L., and Branham, B. E. (2013a). Factors affecting foliar nitrogen uptake by creeping bentgrass. Crop Sci. 53, 1778. doi:10.2135/cropsci2012.11.0650
Hunter, M. C., Smith, R. G., Schipanski, M. E., Atwood, L. W., and Mortensen, D. A. (2017). Agriculture in 2050: recalibrating targets for sustainable intensification. BioScience 67, 386–391. doi:10.1093/biosci/bix010
Iqbal, M. A. (2019). Nano-fertilizers for sustainable crop production under changing climate: a global perspective. London, United Kingdom: IntechOpen, doi:10.5772/intechopen.89089
Iqbal, M., Umar, S., and Mahmooduzzafar, (2019). Nano-fertilization to enhance nutrient use efficiency and productivity of crop plants. in Nanomater. Plant Potential, 473–505. doi:10.1007/978-3-030-05569-1_19
Isermann, K. (1990). Share of agriculture in nitrogen and phosphorus emissions into the surface waters of Western Europe against the background of their eutrophication. Fertilizer Res. 26, 253–269. doi:10.1007/BF01048764
Jadon, P., Selladurai, R., Yadav, S. S., Coumar, M. V., Dotaniya, M. L., Singh, A. K., et al. (2018). Volatilization and leaching losses of nitrogen from different coated urea fertilizers. J. Soil Sci. Plant Nutr. 18, 1036–1047. doi:10.4067/S0718-95162018005002903
Jia, C., Lu, P., and Zhang, M. (2020). Preparation and characterization of environmentally friendly controlled release fertilizers coated by leftovers-based polymer. Processes 8, 417. doi:10.3390/pr8040417
Jiang, X., Yan, B., Chen, J., Li, W., Hu, J., and Guan, Y. (2019). Transport and retention of phosphorus in soil with addition of Mg-Al layered double hydroxides: effects of material dosage, flow velocity and pH. Chem. Eng. J. 378, 122154. doi:10.1016/j.cej.2019.122154
Jyothi, T. V., and Hebsur, N. S. (2017). Effect of nanofertilizers on growth and yield of selected cereals - a review. Ag 38. doi:10.18805/ag.v38i02.7942
Kah, M., Kookana, R. S., Gogos, A., and Bucheli, T. D. (2018). A critical evaluation of nanopesticides and nanofertilizers against their conventional analogues. Nat. Nanotechnol. 13, 677–684. doi:10.1038/s41565-018-0131-1
Kalia, A., Sharma, S. P., and Kaur, H. (2019). “Nanoscale fertilizers: harnessing boons for enhanced nutrient use efficiency and crop productivity,” in Nanobiotechnology applications in plant protection: volume 2 nanotechnology in the Life sciences. Editors K. A. Abd-Elsalam, and R. Prasad (Cham, Switzerland: Springer International Publishing)), 191–208. doi:10.1007/978-3-030-13296-5_10
Kalia, A., and Sharma, S. P. (2019). “Nanomaterials and vegetable crops: realizing the concept of sustainable production,” in Nanoscience for sustainable agriculture. Editors R. N. Pudake, N. Chauhan, and C. Kole (Cham, Switzerland: Springer International Publishing), 323–353. doi:10.1007/978-3-319-97852-9_15
Koilraj, P., Antonyraj, C. A., Gupta, V., Reddy, C. R. K., and Kannan, S. (2013). Novel approach for selective phosphate removal using colloidal layered double hydroxide nanosheets and use of residue as fertilizer. Appl. Clay Sci. 86, 111–118. doi:10.1016/j.clay.2013.07.004
Kopittke, P. M., Lombi, E., Wang, P., Schjoerring, J. K., and Husted, S. (2019). Nanomaterials as fertilizers for improving plant mineral nutrition and environmental outcomes. Environ. Sci. Nano 6, 3513–3524. doi:10.1039/C9EN00971J
Kottegoda, N., Sandaruwan, C., Priyadarshana, G., Siriwardhana, A., Rathnayake, U. A., Berugoda Arachchige, D. M., et al. (2017). Urea-Hydroxyapatite nanohybrids for slow release of nitrogen. ACS Nano 11, 1214–1221. doi:10.1021/acsnano.6b07781
Kottegoda, N., Munaweera, I., Madusanka, N., and Karunaratne, V. (2011). A green slow-release fertilizer composition based on urea-modified hydroxyapatite nanoparticles encapsulated wood. Curr. Sci. 101, 73–78.
Kumar, R., Ashfaq, M., and Verma, N. (2018). Synthesis of novel PVA-starch formulation-supported Cu-Zn nanoparticle carrying carbon nanofibers as a nanofertilizer: controlled release of micronutrients. J. Mater. Sci. 53, 7150–7164. doi:10.1007/s10853-018-2107-9
Kusumastuti, Y., Istiani, A., Rochmadi, , and Purnomo, C. W. (2019). Chitosan-based polyion multilayer coating on NPK fertilizer as controlled released fertilizer. Adv. Mater. Sci. Engineering 2019, 1. doi:10.1155/2019/2958021
Lazaratou, C. V., Vayenas, D. V., and Papoulis, D. (2020). The role of clays, clay minerals and clay-based materials for nitrate removal from water systems: a review. Appl. Clay Sci. 185, 105377. doi:10.1016/j.clay.2019.105377
Lee, D. R., Barrett, C. B., and McPeak, J. G. (2006). Policy, technology, and management strategies for achieving sustainable agricultural intensification. Agric. Econ. 34, 123–127. doi:10.1111/j.1574-0864.2006.00112.x
Lee, Y.-C., and Moon, J.-Y. (2020). Introduction to nanotechnology and bionanotechnology,” in Introduction to bionanotechnology. Editors Y.-C. Lee, and J.-Y. Moon (Singapore: Springer), 1–14. doi:10.1007/978-981-15-1293-3_1
Leip, A., Billen, G., Garnier, J., Grizzetti, B., Lassaletta, L., Reis, S., et al. (2015). Impacts of European livestock production: nitrogen, sulphur, phosphorus and greenhouse gas emissions, land-use, water eutrophication and biodiversity. Environ. Res. Lett. 10, 115004. doi:10.1088/1748-9326/10/11/115004
Liu, R., and Lal, R. (2015). Potentials of engineered nanoparticles as fertilizers for increasing agronomic productions. Sci. Total Environ. 514, 131–139. doi:10.1016/j.scitotenv.2015.01.104
Lowry, G. V., Avellan, A., and Gilbertson, L. M. (2019). Opportunities and challenges for nanotechnology in the agri-tech revolution. Nat. Nanotechnol. 14, 517–522. doi:10.1038/s41565-019-0461-7
Lv, J., Christie, P., and Zhang, S. (2019). Uptake, translocation, and transformation of metal-based nanoparticles in plants: recent advances and methodological challenges. Environ. Sci. Nano 6, 41–59. doi:10.1039/C8EN00645H
Maddux, L., and Barnes, P. (1985). Effects of time and rate of applied nitrogen and nitrapyrin on irrigated corn. J. Fertil. issues 2, 124–129.
Mahil, E. I. T., and Kumar, B. N. A. (2019). Foliar application of nanofertilizers in agricultural crops – a review. 11.
Malekian, R., Abedi-Koupai, J., and Eslamian, S. S. (2011). Influences of clinoptilolite and surfactant-modified clinoptilolite zeolite on nitrate leaching and plant growth. J. Hazard. Mater. 185, 970–976. doi:10.1016/j.jhazmat.2010.09.114
Malinas, A., Rotar, I., Vidican, R., Iuga, V., Pacurar, F., Malinas, C., et al. (2020). Designing a sustainable temporary grassland system by monitoring nitrogen use efficiency. Agronomy 10 (1), 149. doi:10.3390/agronomy10010149
Manikandan, A., and Subramanian, K. S. (2017). Study on mitigation of ammonia volatilization loss in urea through adsorbents. Jans 9, 688–692. doi:10.31018/jans.v9i2.1258
Manjaiah, K., Mukhopadhyay, R., Paul, R., Datta, S., Periyamuthu, K., and Sarkar, B. (2019). Clay minerals and zeolites for environmentally sustainable agriculture. in Modified clay and zeolite nanocomposite materials. Amsterdam, Netherlands: Elsevier. 309–329. doi:10.1016/B978-0-12-814617-0.00008-6
Manjunatha, S. B., Biradar, D., and Aladakatti, Y. (2016). Nanotechnology and its applications in agriculture: a review. J. Farm Sci. 29, 1–13.
Marchiol, L., Iafisco, M., Fellet, G., and Adamiano, A. (2020). “Chapter Two - nanotechnology support the next agricultural revolution: perspectives to enhancement of nutrient use efficiency,” in Advances in agronomy. Editor D. L. Sparks (Cambridge, MA: Academic Press), 27–116. doi:10.1016/bs.agron.2019.12.001
Mastronardi, E., Tsae, P., Zhang, X., Monreal, C., and Derosa, M. (2015). Strategic role of nanotechnology in fertilizers: potential and limitations. Berlin, Germany: Springer. 25–67. doi:10.1007/978-3-319-14024-7_2
Matheyarasu, R., Seshadri, B., Bolan, N. S., and Naidu, R. (2016). Assessment of nitrogen losses through nitrous oxide from abattoir wastewater-irrigated soils. Environ. Sci. Pollut. Res. Int. 23, 22633–22646. doi:10.1007/s11356-016-7438-y
Mikkelsen, R. (2018). Nanofertilizer and Nanotechnology: a quick look. Better Crops 102, 18–19. doi:10.24047/BC102318
Montalvo, D., McLaughlin, M. J., and Degryse, F. (2015). Efficacy of hydroxyapatite nanoparticles as phosphorus fertilizer in andisols and oxisols. Soil Sci. Soc. America J. 79, 551–558. doi:10.2136/sssaj2014.09.0373
Norton, R., Davidson, E., and Roberts, T. (2015). “Nitrogen use efficiency and nutrient performance indicators,” in Glob. Partnersh. Nutr. Manag. (Washington, DC: Task Team Workshop Wash. DC), 1–14.
IPCC (2015). Climate change 2014: mitigation of climate change ; summary for policymakers technical Summary ; part of the working group III contribution to the fifth asessment rreport of the intergovernmental panel on climate change. O. Edenhofer, R. Pichs-Madruga, Y. Sokona, J. C. Minx, E. Farahani, S. Kadneret al. (Geneva, Switzerland: Intergovernmental Panel on Climate Change).
Oenema, O., de Klein, C., and Alfaro, M. (2014). Intensification of grassland and forage use: driving forces and constraints. Crop Pasture Sci. 65, 524–537. doi:10.1071/cp14001
Pan, B., Lam, S. K., Mosier, A., Luo, Y., and Chen, D. (2016). Ammonia volatilization from synthetic fertilizers and its mitigation strategies: a global synthesis. Agric. Ecosyst. Environ. 232, 283–289. doi:10.1016/j.agee.2016.08.019
Pereira, E. I., da Cruz, C. C. T., Solomon, A., Le, A., Cavigelli, M. A., and Ribeiro, C. (2015). Novel slow-release nanocomposite nitrogen fertilizers: the impact of polymers on nanocomposite properties and function. Ind. Eng. Chem. Res. 54, 3717–3725. doi:10.1021/acs.iecr.5b00176
Pérez-de-Luque, A. (2017). Interaction of nanomaterials with plants: what do we need for real applications in agriculture? Front. Environ. Sci. 5, 12. doi:10.3389/fenvs.2017.00012
Perrin, T. S., Drost, D. T., Boettinger, J. L., and Norton, J. M. (1998). Ammonium‐loaded clinoptilolite: a slow‐release nitrogen fertilizer for sweet corn. J. Plant Nutr. 21, 515–530. doi:10.1080/01904169809365421
Pestovsky, Y. S., and Martínez-Antonio, A. (2017). The use of nanoparticles and nanoformulations in agriculture. J. Nanosci. Nanotechnol. 17, 8699–8730. doi:10.1166/jnn.2017.15041
Peters, R., Brandhoff, P., Weigel, S., Marvin, H., Bouwmeester, H., Aschberger, K., et al. (2014). Inventory of Nanotechnology applications in the agricultural, feed and food sector. Parma, Italy: EFSA Supporting Publications. 11. doi:10.2903/sp.efsa.2014.EN-621
Pijlman, J., Berger, S. J., Lexmond, F., Bloem, J., van Groenigen, J. W., Visser, E. J. W., et al. (2020). Can the presence of plantain (Plantago lanceolataL.) improve nitrogen cycling of dairy grassland systems on peat soils? New Zealand J. Agric. Res. 63, 106–122. doi:10.1080/00288233.2019.1698620
Powell, J. M., Gourley, C. J. P., Rotz, C. A., and Weaver, D. M. (2010). Nitrogen use efficiency: a potential performance indicator and policy tool for dairy farms. Environ. Sci. Pol. 13, 217–228. doi:10.1016/j.envsci.2010.03.007
Preetha, P. S., and Balakrishnan, N. (2017). A review of nano fertilizers and their use and functions in soil. Int. J. Curr. Microbiol. App. Sci 6, 3117–3133. doi:10.20546/ijcmas.2017.612.364
Purnomo, L., Billen, G., Grizzetti, B., Anglade, J., and Garnier, J. (2014). 50 year trends in nitrogen use efficiency of world cropping systems: the relationship between yield and nitrogen input to cropland. Environ. Res. Lett. 9, 105011. doi:10.1088/1748-9326/9/10/105011
Raguraj, S., Wijayathunga, W. M. S., Gunaratne, G. P., Amali, R. K. A., Priyadarshana, G., Sandaruwan, C., et al. (2020). Urea-hydroxyapatite nanohybrid as an efficient nutrient source in Camellia sinensis (L.) Kuntze (tea). J. Plant Nutr. 43 (15), 2383–2394. doi:10.1080/01904167.2020.1771576
Ramírez-Rodríguez, G. B., Miguel-Rojas, C., Montanha, G. S., Carmona, F. J., Dal Sasso, G., Sillero, J. C., et al. (2020). Reducing nitrogen dosage in Triticum durum plants with urea-doped nanofertilizers. Nanomaterials 10, 1043. doi:10.3390/nano10061043
Ramzan, S., Rasool, T., Bhat, R. A., Ahmad, P., Ashraf, I., Rashid, N., et al. (2020). Agricultural soils a trigger to nitrous oxide: a persuasive greenhouse gas and its management. Environ. Monit. Assess. 192, 436. doi:10.1007/s10661-020-08410-2
Ribeiro, C., and Carmo, M. (2019). Why nonconventional materials are answers for sustainable agriculture. MRS Energy Sustain. 6 (1), E9. doi:10.1557/mre.2019.7
Ruiz-Cañas, M., Quintero, H., Corredor, L., Manrique, E., and Romero Bohórquez, A. (2020). New nanohybrid based on hhydrolyzed polyacrylamide and silica nanoparticles: morphological, structural and thermal properties. Polymers 12 (5), 1152–1166. doi:10.3390/polym12051152
Ryle, G. J. A., and Stanley, J. (1992). Effect of elevated CO2 on stomatal size and distribution in perennial ryegrass. Ann. Bot. 69, 563–565. doi:10.1093/oxfordjournals.aob.a088387
Sarkar, S., Datta, S. C., and Biswas, D. R. (2014). Effect of fertilizer loaded nanoclay/superabsorbent polymer composites on nitrogen and phosphorus release in soil. Proc. Natl. Acad. Sci. India, Sect. B Biol. Sci. 85, 415. doi:10.1007/s40011-014-0371-2
Schlossberg, M. J., McGraw, B. A., and Sebring, R. L. (2018). Ammonia volatilization from putting greens foliarly fertilized by conventional or stabilized urea. Agric. Environ. Lett. 3, 180019. doi:10.2134/ael2018.04.0019
Shang, Y., Hasan, M. K., Ahammed, G. J., Li, M., Yin, H., and Zhou, J. (2019). Applications of nanotechnology in plant growth and crop protection: a review. Molecules 24, 2558–2580. doi:10.3390/molecules24142558
Sharif, R., Mujtaba, M., Ur Rahman, M., Shalmani, A., Ahmad, H., Anwar, T., et al. (2018). The multifunctional role of chitosan in horticultural crops; a review. Molecules 23, 872. doi:10.3390/molecules23040872
Sharma, L., and Bali, S. (2018). A review of methods to improve nitrogen use efficiency in agriculture. Sustainability 10, 51. doi:10.3390/su10010051
Silva, A. G. B., Sequeira, C. H., Sermarini, R. A., and Otto, R. (2017). Urease inhibitor NBPT on ammonia volatilization and crop productivity: a meta-analysis. Agron. J. 109, 1–13. doi:10.2134/agronj2016.04.0200
Silva, V. d., Mangrich, A. S., and Wypych, F. (2014). Liberação de nitrato de hidróxidos duplos lamelares como potenciais fertilizantes de liberação lenta. Rev. Bras. Ciênc. Solo 38, 821–830. doi:10.1590/S0100-06832014000300013
Snyder, C. S. (2017). Enhanced nitrogen fertiliser technologies support the “4R” concept to optimise crop production and minimise environmental losses. Soil Res. 55, 463–472. doi:10.1071/sr16335
Souza, E. F. C., Soratto, R. P., Sandaña, P., Venterea, R. T., and Rosen, C. J. (2020). Split application of stabilized ammonium nitrate improved potato yield and nitrogen-use efficiency with reduced application rate in tropical sandy soils. Field Crops Res. 254, 107847. doi:10.1016/10.1016/j.fcr.2020.107847
Stiegler, J. C., Richardson, M. D., and Karcher, D. E. (2011). Foliar nitrogen uptake following urea application to putting green turfgrass species. Crop Sci. 51, 1253. doi:10.2135/cropsci2010.06.0377
Tarafder, C., Daizy, M., Alam, M. M., Ali, M. R., Islam, M. J., Islam, R., et al. (2020). Formulation of a hybrid nanofertilizer for slow and sustainable release of micronutrients. Acs Omega 5, 23960–23966. doi:10.1021/acsomega.0c03233
Tilman, D., Socolow, R., Foley, J. A., Hill, J., Larson, E., Lynd, L., et al. (2009). Energy. Beneficial biofuels--the food, energy, and environment trilemma. Science 325 (5938), 270–271. doi:10.1126/science.1177970
Torres-Dorante, L. O., Lammel, J., and Kuhlmann, H. (2009). Use of a layered double hydroxide (LDH) to buffer nitrate in soil: long-term nitrate exchange properties under cropping and fallow conditions. Plant Soil 315, 257–272. doi:10.1007/s11104-008-9748-4
Ureña-Amate, M. D., Boutarbouch, N. D., Socias-Vicianadel, M. d. M. M., and González-Pradas, E. (2011). Controlled release of nitrate from hydrotalcite modified formulations. Appl. Clay Sci. 52, 368–373. doi:10.1016/j.clay.2011.03.018
Weeks, J. J., and Hettiarachchi, G. M. (2019). A review of the latest in phosphorus fertilizer technology: possibilities and pragmatism. J. Environ. Qual. 48, 1300–1313. doi:10.2134/jeq2019.02.0067
Weih, M., Asplund, L., and Bergkvist, G. (2011). Assessment of nutrient use in annual and perennial crops: a functional concept for analyzing nitrogen use efficiency. Plant Soil 339, 513–520. doi:10.1007/s11104-010-0599-4
Whitehead, D. C. (2000). Nutrient elements in grassland: soil-plant-animal relationships. Wallingford, United Kingdom: CABI Publishing.
Xie, H., Huang, Y., Chen, Q., Zhang, Y., and Wu, Q. (2019). Prospects for agricultural sustainable intensification: a review of research. Land 8, 157. doi:10.3390/land8110157
Yoon, H. Y., Lee, J. G., Esposti, L. D., Iafisco, M., Kim, P. J., Shin, S. G., et al. (2020). Synergistic release of crop nutrients and stimulants from hydroxyapatite nanoparticles functionalized with humic substances: toward a multifunctional nanofertilizer. ACS Omega 5, 6598–6610. doi:10.1021/acsomega.9b04354
Keywords: nitrogen nanofertilizers, nitrogen losses, nitrogen use efficiency, nanofertilizers in grasslands, nitrogen based nanomaterials
Citation: Mejias JH, Salazar F, Pérez Amaro L, Hube S, Rodriguez M and Alfaro M (2021) Nanofertilizers: A Cutting-Edge Approach to Increase Nitrogen Use Efficiency in Grasslands. Front. Environ. Sci. 9:635114. doi: 10.3389/fenvs.2021.635114
Received: 29 November 2020; Accepted: 08 February 2021;
Published: 19 March 2021.
Edited by:
Yupeng Wu, Huazhong Agricultural University, ChinaReviewed by:
Mahmud Hossain, Bangladesh Agricultural University, BangladeshCopyright © 2021 Mejias, Salazar, Pérez Amaro, Hube, Rodriguez and Alfaro. This is an open-access article distributed under the terms of the Creative Commons Attribution License (CC BY). The use, distribution or reproduction in other forums is permitted, provided the original author(s) and the copyright owner(s) are credited and that the original publication in this journal is cited, in accordance with accepted academic practice. No use, distribution or reproduction is permitted which does not comply with these terms.
*Correspondence: M. Alfaro, bWFsZmFyb0BpbmlhLmNs
Disclaimer: All claims expressed in this article are solely those of the authors and do not necessarily represent those of their affiliated organizations, or those of the publisher, the editors and the reviewers. Any product that may be evaluated in this article or claim that may be made by its manufacturer is not guaranteed or endorsed by the publisher.
Research integrity at Frontiers
Learn more about the work of our research integrity team to safeguard the quality of each article we publish.