- 1Department of Agricultural and Biosystems Engineering, Iowa State University, Ames, IA, United States
- 2Department of Applied Bioeconomy, Wrocław University of Environmental and Life Sciences, Wrocław, Poland
- 3Department of Animal Biosystems Sciences, Chungnam National University, Daejeon, South Korea
- 4Department of Civil, Construction and Environmental Engineering, Iowa State University, Ames, IA, United States
- 5Department of Mechanical Engineering, Bioeconomy Institute, Iowa State University, Ames, IA, United States
Ammonia (NH3) emissions from animal agriculture can cause eutrophication of water ecosystems and are precursors to secondary particulate matter (PM2.5). NH3 emissions from stored swine manure represent nutrient loss affecting the fertilizing value of manure. The short-term emission bursts occur when farmers agitate manure before emptying storage and fertilizing fields. There is no proven technology to mitigate gaseous emissions during agitation, while the hazards of acute releases (e.g., H2S) are well-known. Biochar mitigates NH3 emissions from manure over the long-term. The objective of this research was to evaluate the mitigation of acute NH3 emissions during/after agitation. Two biochars, highly alkaline and porous (HAP from corn stover) and red oak (RO), were tested. The 6 and 12 mm-thick layers of biochar powder were surficial applied followed by 3 min agitation. NH3 concentrations were measured before/during/after agitation. Mitigation was assessed by comparing: (i) the maximum (peak) flux, (ii) total emission (from agitation start till NH3 concentration returned to the before-agitation), and (iii) the total emissions during agitation. The 12 mm HAP significantly (p < 0.05) reduced (i–iii) by 63, 70, and 85%, respectively. The 6 mm HAP significantly reduced (i–iii) by 76, 75, and 78%, respectively. The 12 mm RO significantly reduced (i–iii) by 9, 53, and 57%, respectively. The 6 mm RO significantly reduced (i–iii) by 61, 86, and 63%, respectively. The NH3 emission kinetics model confirmed that a 6 mm dose was just as effective as the larger dose. More research is needed to optimize and scale-up mitigating emissions and retention of nutrients in manure with biochar.
Introduction
The Midwest United States has a significant presence of pork production. Many large swine barns use deep-pits to store manure under the slatted floor for up to 1 year. Ammonia (NH3) emissions from the livestock industry are a significant environmental concern. NH3 is an air pollutant, which can also cause eutrophication of water ecosystems (Hoff et al., 2006; Ni et al., 2009). NH3 also plays a significant part in forming secondary particulate matter (PM2.5) aerosols. Stored swine manure is a significant source of long-term NH3 emissions for most of the year. However, the short-term bursts of NH3 emissions can occur when swine farmers agitate manure prior to emptying storage pits before land application. Agitation releases hydrogen sulfide (H2S), with dangerously high concentrations resulting in human and animal fatalities (Chen et al., 2020c). While the issue of H2S emissions is relatively well-known, there is no data on the NH3 emissions during agitation. Workers are subjected to chronic exposure to NH3 and other gases released from manure year-round. The United States National Institute for Occupational Safety and Health (NIOSH) recommends the time-weighted average (TWA) 10-h concentration for NH3 at 25 ppm and a short-term exposure limit (ST) 15-min at 35 ppm (NIOSH, 1997).
Emissions of NH3 during agitation pose the loss of valuable nutrients from stored manure immediately prior to land-application. Agitating the manure breaks the entrapped gas bubbles, which causes a rapid increase in NH3 and H2S concentrations (Hoff et al., 2006; Ni et al., 2009). Besides, emissions of other gases, such as odorous volatile organic compounds (VOCs), pose a nuisance to the surrounding communities.
Proven technologies to mitigate these short-term releases of gases from manure during agitation are still needed. This is in addition to the perennial challenge to mitigate emissions year-round. Some swine farmers use commercial pit manure additives to control gaseous emissions during long-term storage. However, our recent evaluation of 12 commercial manure additives’ performance did not show overall statistically significant mitigation for gaseous emissions (Chen et al., 2020a,b). Still, science-based data is needed to evaluate additives’ effect on the mitigation of gases emitted from stored manure (Maurer et al., 2016) as the additives are user-friendly and do not require changes in existing barn structures. We have reported research on manure additives such as soybean peroxidase, zeolite, and biochar powder that shows the effectiveness of mitigating NH3, H2S, VOCs, and greenhouse gas (GHG) emissions from swine manure over extended periods (Cai et al., 2007; Parker et al., 2016; Kalus et al., 2017; Maurer et al., 2017a,b,c).
Our research with biochar shows that a 6∼12 mm thick layer can float on manure while mitigating gaseous emissions over the ∼30 days period. The mitigation effects on NH3 were typically the greatest on the first day of application and decreased over the trial duration (Meiirkhanuly et al., 2020b). The effect of biochar’s biweekly reapplication on gaseous emissions from swine manure was recently reported by Chen et al. (2021). The observation of decreased efficiency over time led us to explore the possibility of using surficial biochar treatment for short-term mitigation of NH3 emissions from swine manure. It was also apparent that biochar pH and other physicochemical properties can influence the spatial and temporal effects on pH near the liquid-gas interface owing to biochar addition to water (Meiirkhanuly et al., 2019) and manure surface (Meiirkhanuly et al., 2020a).
Maurer et al. (2017c) reported that biochar mitigated the NH3 emissions by 13–23% from the deep pit swine manure for the three 1-month long trials tested in that research. Dougherty et al. (2017) reported that low pH biochar (5 cm thick) covers significantly reduced the NH3 emissions of dairy manure from lagoons. Kalus et al. (2020a) used beechwood biochar as diet supplementation for broiler chickens, which significantly reduced NH3 emissions by up to 17%. However, Kalus et al. (2020b) reported the same kind of biochar that was fed to laying hens did not show an effect on NH3 emissions.
Biochar was proposed as a fertilizer, soil amendment, carbon source and adsorbent, and alternative fuel (Białowiec et al., 2018; Pulka et al., 2019; Stępień et al., 2019b). Biochar can be made via pyrolysis or torrefaction from biomass and waste (Kalus et al., 2019; Pulka et al., 2019; Stępień et al., 2019a,b; Syguła et al., 2019; Świechowski et al., 2019). Physicochemical properties of resulting biochars vary as a result of differences in feedstock and its pre-treatment, temperature, and time of the process (Kalus et al., 2019; Pulka et al., 2019; Stępień et al., 2019a,b; Syguła et al., 2019; Świechowski et al., 2019). More sustainable environmental goals could be achieved by optimizing biochar properties (e.g., pH, porosity, chemical moiety).
Most recently, Chen et al. (2020c) reported 80% short-term mitigation of H2S emissions during and post-agitation of swine manure using two biochars (pH of 7.5 and 9.2). In this study, we report on the short-term mitigation effect of the same two types of biochar on NH3 emissions during manure agitation.
The research questions were:
(i) What should biochar dosage should be applied?
(ii) Will the NH3 emission rates be influenced by the agitation of manure with added (alkaline pH) biochar?
(iii) Will the mitigation effect be effective to meet the NIOSH recommendations for indoor air quality and occupational exposure, and
(iv) Are scale-up trials warranted?
The working hypothesis was that a larger dose of biochar would result in a higher NH3 mitigation effect. The dose was defined as the thickness of the surficial layer applied to manure. The experiments used a shorter agitation time and a smaller amount of manure for the proof-of-the-concept. Expected outcomes were to provide answers if the mitigation effect would be significant and practical enough to warrant further scale-up research.
Experiments
The experiments to evaluate the mitigation effect of two types of biochar powder on acute emissions of NH3 were conducted simultaneously with the mitigation of H2S. The comprehensive report on the mitigation of H2S emissions during manure agitation was reported recently by Chen et al. (2020c). In this manuscript, we focus solely on reporting the effect of biochars on NH3 emissions. Thus, all details of experimental design, experimental setup, data, and statistical analyses are presented elsewhere Chen et al. (2020c). Thus, this section is shortened to avoid redundancy. Briefly, the key facts are presented below.
The deep pit and agitation simulation was facilitated by 1.22 m × 0.38 m (height × diameter) manure storage. The manure’s working volume was 103.1 L, which is obtained from a local deep-pit swine barn with a pH of ∼7.5 (Meiirkhanuly et al., 2020b). A total of 9 manure storages were used for three scenarios with replication (n = 3). The headspace was closed and flushed with the controlled airflow. The headspace was flushed with 7.5 air exchanges per hour (ACH), representing the ventilation of deep-pit manure storage (MidWest Plan Service, 1983; Maurer et al., 2017b). A small transfer pump (0.1 hp, Little Giant, Fort Wayne, IN, United States) agitated the manure at 1.36 m3 h–h. Figure 1 illustrates the experimental setup and design.
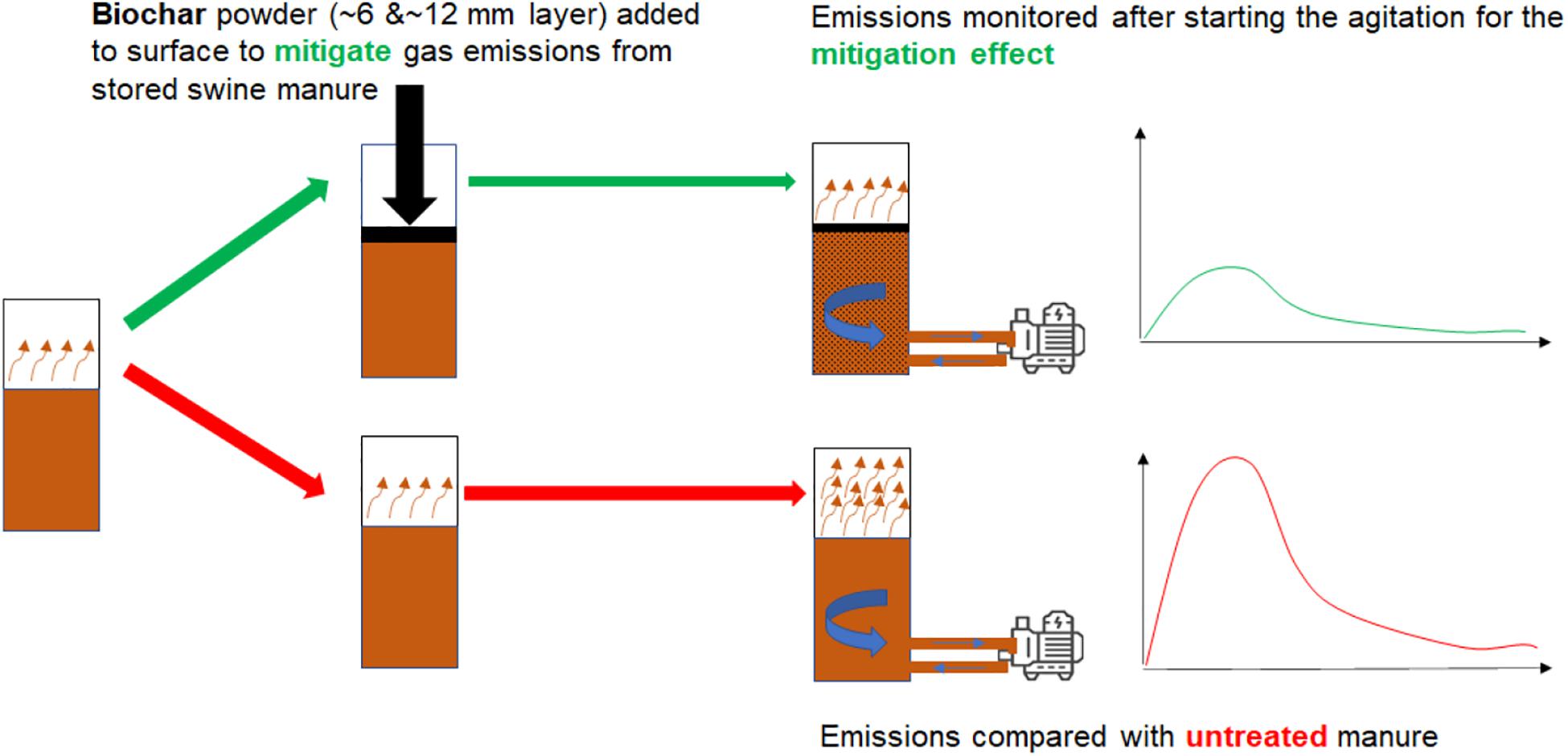
Figure 1. The proof-of-the-concept mitigation of acute NH3 emissions from swine manure with a surficial application of biochar powder immediately prior to agitation.
Biochar physicochemical properties were described elsewhere (Meiirkhanuly et al., 2019, 2020a,b). Briefly, some key properties are listed below. RO biochar was pyrolyzed at 500–550°C and a pH of 7.5. The highly alkaline (pH = 9.2) and porous (HAP) biochar was made from corn stover pyrolyzed at 500°C.
The headspace effluent was analyzed for NH3 concentrations. A real-time monitoring system equipped with electrochemical gas sensors (NH3/C-1000) (Smart Control & Sensing Inc., Daejeon, South Korea) was used to measure the real-time NH3 concentration (Wi et al., 2019; Lee et al., 2020). Standard NH3 gas was used to calibrate the analyzer before use (Wi et al., 2019; Lee et al., 2020).
The details of the experimental setup were published by Chen et al. (2020c). The agitation, lasting 3 min, was facilitated by pumping manure from the bottom to the middle of the storage. Three triplicated treatments for each biochar were set up:
(i) Control–manure not treated with biochar.
(ii) Treatment 1–manure treated with a 6 mm thick layer of biochar powder.
(iii) Treatment 2–manure treated with a 12 mm thick layer of biochar powder.
The biochar dose was based on achieving either a 6 or 12 mm thick layer of biochar spread evenly over the manure surface. The headspace NH3 concentrations were continuously measured in the headspace exhaust as follows:
1. Stage 1 (No agitation), started after biochar application and before agitation for all three treatments (i–iii).
2. Stage 2 (Agitation). All three treatments (i–iii) during agitation.
3. Stage 3 (Post-agitation). All three treatments (i–iii) after agitation until the headspace NH3 concentration reached its initial state.
Data Analysis, the Kinetics of Emissions
The effect of biochar was determined by % reduction, defined as a relative difference between measured emissions from the Control (not treated) and Treatment (treated with biochar) manure. The % reduction was estimated as:
The emission data analysis consisted of the one-way analysis of variance (ANOVA) and Tukey–Kramer method using JMP (version Pro 14, SAS Institute, Inc., Cary, NC, United States). The statistical significance threshold of 0.05 was used for the total emissions for both overall and during 3 min agitation. The maximum NH3 concentrations were used for a pooled T-test to estimate the p-values. The post-agitation cumulative NH3 emission kinetics were fit into the Gompertz model (Hanusz et al., 2008):
Where E = NH3 emission flux, mg⋅m–e; E0 = NH3 maximum cumulative emission flux, mg⋅m–m; k = constant rate of the NH3 emission flux, s–e; t = time, s; and a1 = the inflection time of the cumulative NH3 emissions, s.
The cumulative emission kinetics were modeled with a non-linear regression (Statistica 13 software, TIBCO Software Inc., Palo Alto, CA, United States). The kinetic analysis was completed for each treatment and each repetition. The regression analysis results for each treatment (summarized in Appendix A) were used to estimate the mean E0, k, and a1 values in Eq. 2. The ANOVA was applied with post-hoc Tukey’s test (summarized in Appendix B) to indicate the average values’ statistical significance.
Results
Stage 1: Gaseous Emissions After Biochar Application and Before Agitation
Both treatments (6 and 12 mm) showed a significant reduction in emissions immediately after applying RO biochar. The 6 and 12 mm treatments reduced the NH3 emissions by 78.9 and 56.8%, respectively (Table 1). Similarly, the HAP biochar also effectively reduced NH3 emissions by 90.6% and ∼93% for the 6 and 12 mm dose immediately after application to the surface and prior to manure agitation (Table 1).

Table 1. NH3 emissions after (RO, red oak and highly alkaline and porous, HAP) biochar application (6 or 12 mm surficial dose) to manure surface and before agitation.
It is interesting to report the observation that the greater and thicker (12 mm) doses resulted in the wetter appearance of biochar that mixed more readily with manure in comparison to the 6 mm dose. The 12 mm dose had more of open (i.e., not covered with biochar) manure patches visible on the surface compared with the 6 mm dose. These observations were similar dose-dependent behavior with surficially applied soybean peroxidase treatment to swine manure, i.e., thicker and heavier dosages having a tendency to turn over and be less effectively covering the manure surface (Maurer et al., 2017b).
Stage 2: Gaseous Emissions During Agitation
Biochar (RO) (both the 6 and 12 mm) treatment significantly reduced the maximum NH3 peak emission of by 61.2% (p < 0.005) and 8.71% (p = 0.02137), respectively. In addition, a 62.7 and 56.8% (p < 0.0001) reduction in the total NH3 emission during the 3-min of agitation was estimated for the 6 and 12 mm treatments, respectively (Table 2 and Appendix Figure 1).

Table 2. Biochar (RO) treatment: the maximum peak flux and total NH3 emission during 3 min agitation (bold font signifies statistical significance).
Stage 3: Gaseous Emissions After Agitation
An immediate decrease in NH3 concentrations for both HAP and RO biochar treatments was measured when the agitation stopped (Appendix Figures 1, 2). The NH3 concentrations in the headspace were then tracked until they reached the pre-agitation levels. The cumulative NH3 emissions were reduced by 86.1 and 52.9% (p < 0.0001) with the RO treatment for 6 and 12 mm doses, respectively (Table 3).
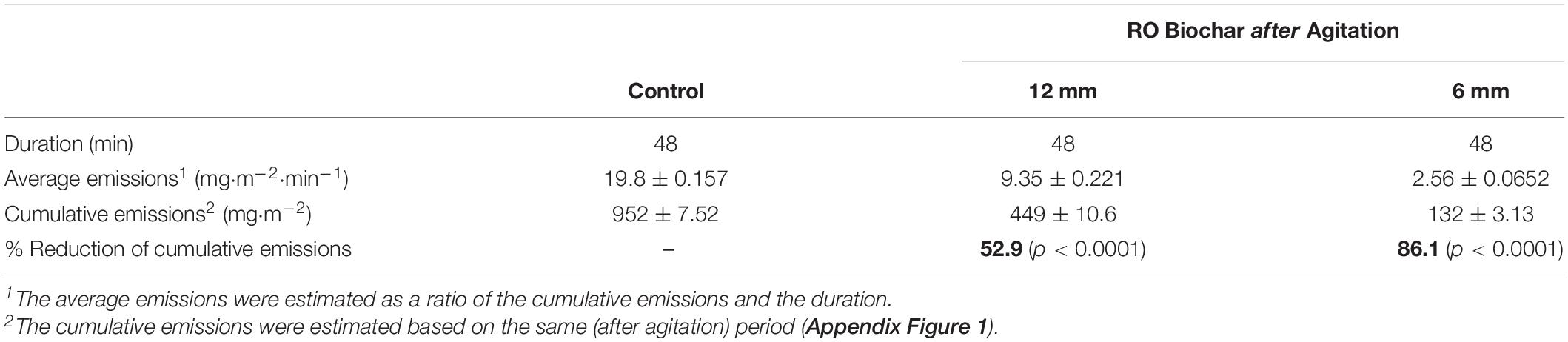
Table 3. Biochar (RO) treatment: the average flux and cumulative NH3 emission after agitation (bold font signifies statistical significance).
The HAP biochar reduced cumulative NH3 emissions by 74.5 and 70.0% (p < 0.0001) for 6 and 12 mm dose. respectively (Table 4).
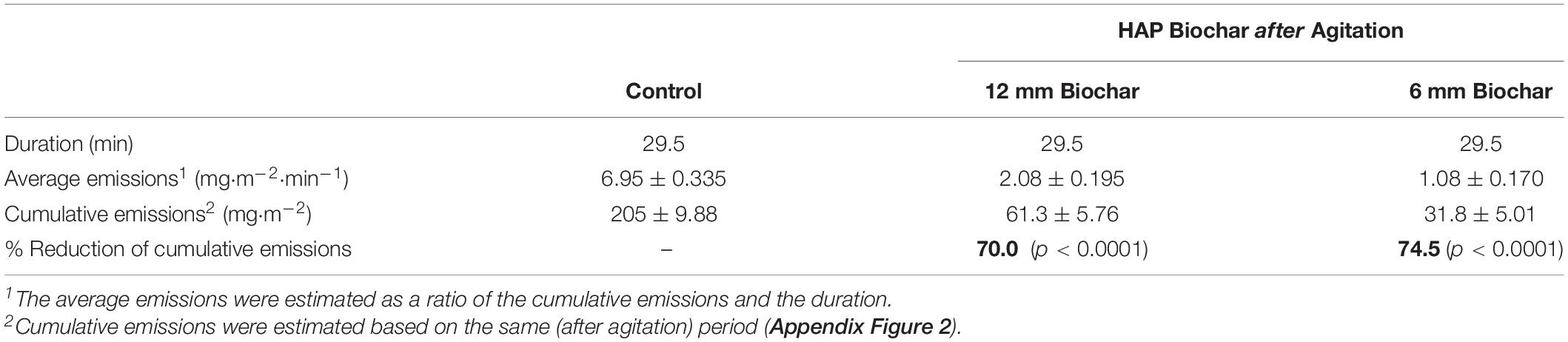
Table 4. Biochar (HAP) treatment: the average flux and cumulative NH3 emission after agitation (bold font signifies statistical significance).
The NH3 in the headspace of RO-treated manure needed a longer time to return to the initial state compared with the HAP treatment (Appendix Figures 1, 2). The acute emissions of NH3 were higher in the experiments testing the RO biochar (Appendix Figure 1) compared with the HAP biochar (Appendix Figure 2). The control concentrations exceeded the range of the NH3 sensor. While this is not an optimal result from the standpoint of accurate measurement, it highlights manure’s potential to generate significant acute releases of NH3. Also, the % reductions affected by the sensor were underestimated. It is also important to highlight that the differences in the control concentrations for the RO and HAP experiments result from the differences in manure used in RO and HAP experiments, which was collected at the same farm, yet at two different times for the RO and HAP trials. This difference did not affect the paired treatment vs. control comparison of results for each biochar tested.
Kinetics of the Post-agitation NH3 Emissions
The kinetics modeling was completed to evaluate the biochar type and dose effect. The E0 parameter in Eq. 2 describes the potential of NH3 emission during an “infinite” time. The cumulative emission during the post-agitation showed a significant (p < 0.05) effect of the HAP treatment on the maximum cumulative flux (Figure 2), yet no differences between the biochar doses (Figure 2 and Appendix Table 1). In contrast, the 12 mm treatment of RO biochar did not significantly influence the potential maximum cumulative flux (Figure 2 and Appendix Table 1). The practical implication is that the lowest dose (6 mm) showed the statistical significance of E0, suggesting that a low biochar dose could be just as effective as the 12 mm for both the RO and HAP biochar.
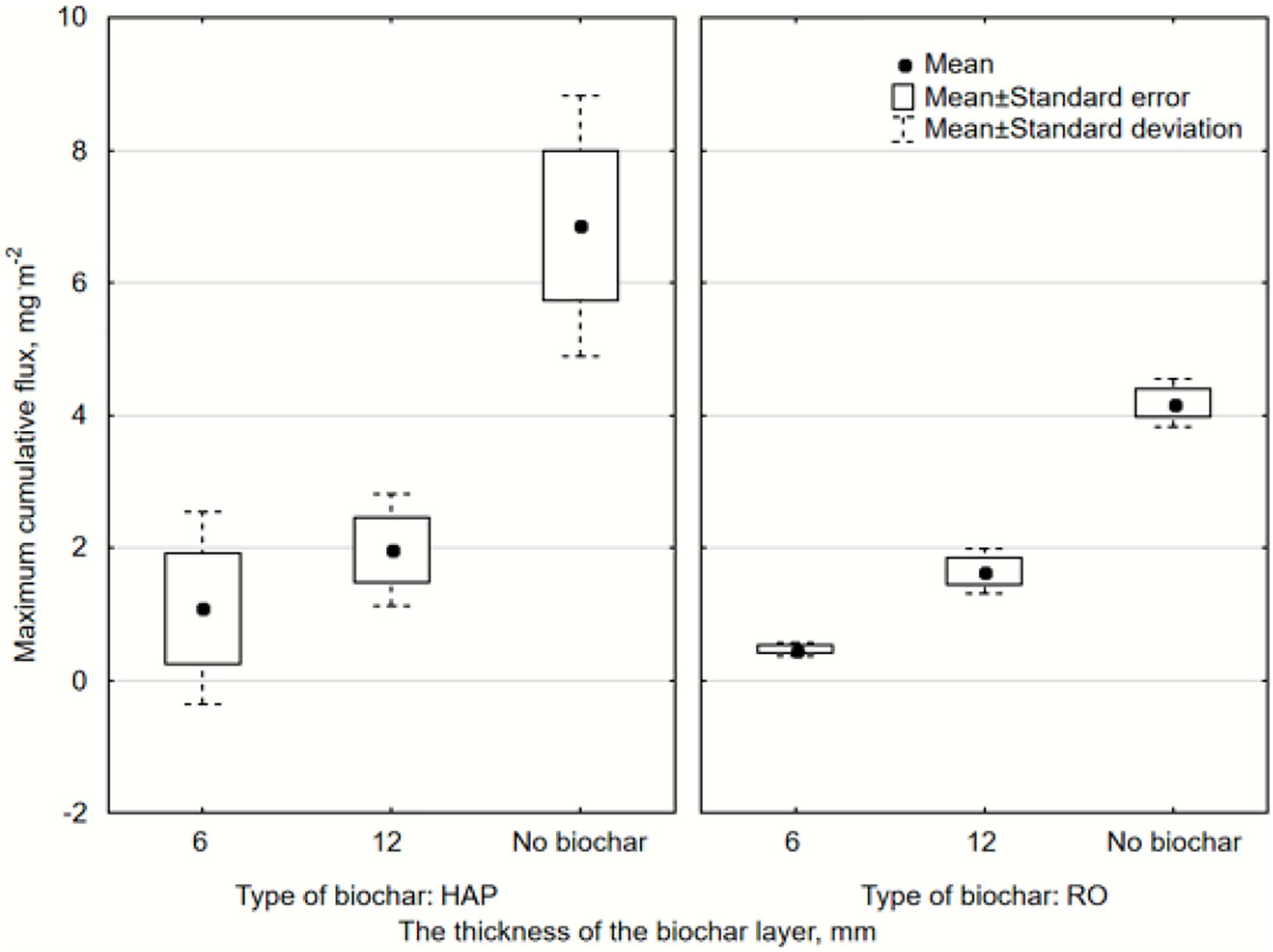
Figure 2. The differences between the maximum cumulative NH3 flux per biochar type and dose (thickness of the biochar layer). Both HAP treatments and 6 mm RO treatment were significant (p < 0.05), 12 mm treatment was insignificant (p = 0.107289); No significant difference between the dosages (Appendix Table 1).
The k constant in Eq. 2 presents the rate of NH3 emissions. Neither of the biochar types significantly influenced (p > 0.05) the k constant (Figure 3 and Appendix Table 2). One possible explanation could be due to high standard deviations (especially for the HAP).
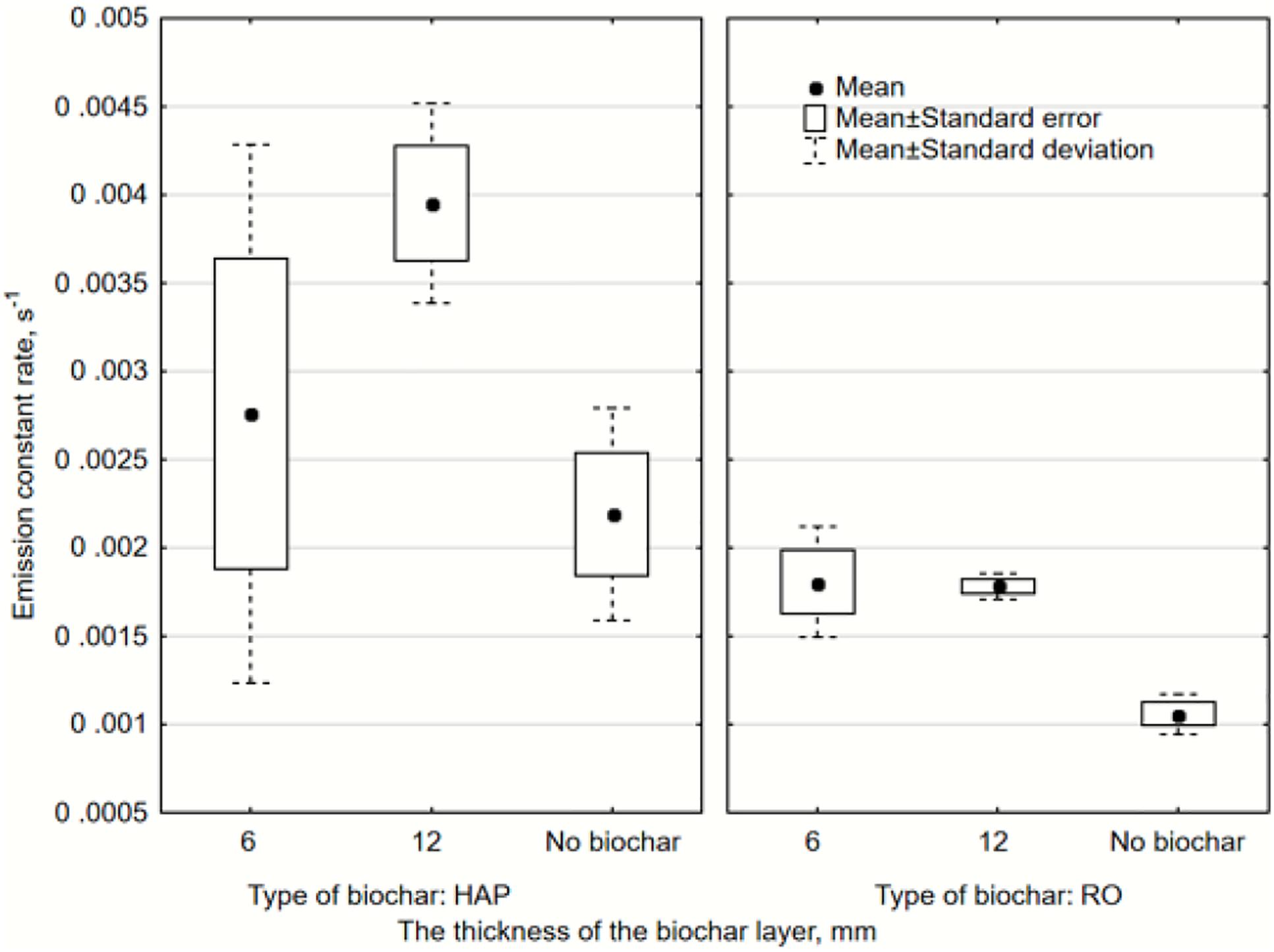
Figure 3. The differences between NH3 emission constant rates k per the type and dose (thickness of the layer) of biochar. The biochar treatments showed no significant differences (p > 0.05) from the Control; no significant difference between dosages for both types of biochar (p > 0.05, Appendix Table 2).
The HAP biochar treatment reduced the total NH3 emission of by 77.8 and 85.2% (p < 0.0001) for the 6 and 12 mm dose, respectively. In addition, a 75.7% (p = 0.02154) and 63.3% (p = 0.04642) reduction in the maximum peak flux of NH3 was observed for the 6 and 12 mm treatment, respectively (Table 5 and Appendix Figure 2).

Table 5. Biochar (HAP) treatment: the maximum peak flux and total NH3 emission during 3 min agitation (bold font signifies statistical significance).
The a1 parameter in Eq. 2 represents the time when the emission rate starts to “slow” down, illustrated by the inflection of the cumulative NH3 emission curve. The HAP biochar did not significantly influence (p > 0.05) the inflection time of the NH3 emission, whereas both dosages of RO biochar showed a significant effect (Figure 4 and Appendix Table 3). The lowest a1 were observed for 6 mm RO biochar, further highlighting the lower dose’s effectiveness.
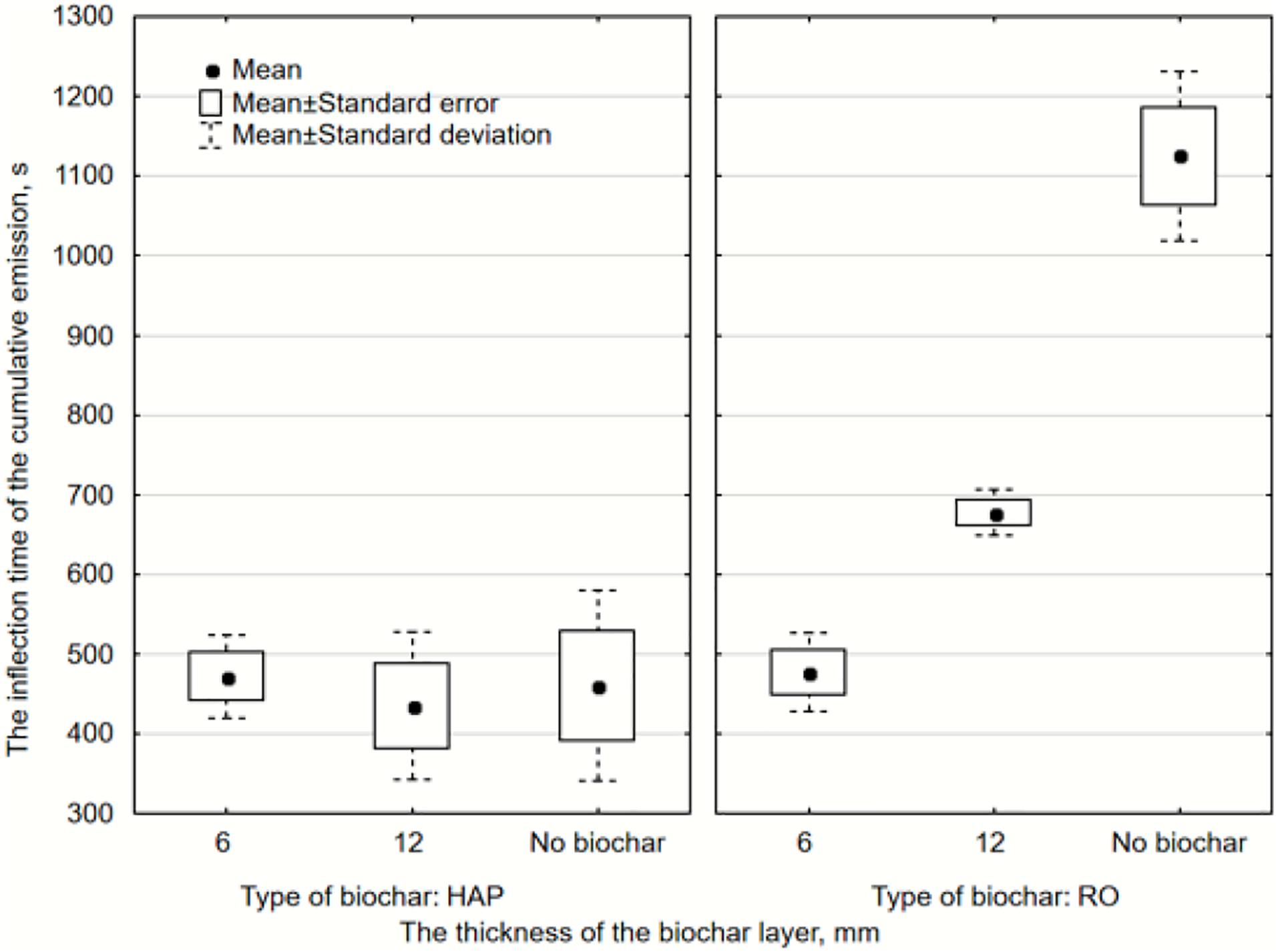
Figure 4. Comparison of the inflection time a1 of the cumulative NH3 emission per biochar type and the dose (thickness of the layer). The biochar treatments showed significant differences (p < 0.05) from the control; insignificant differences (p > 0.05) between the dosages (Appendix Table 3).
Discussion
Both types and dosages of biochar treatments showed effectiveness in mitigating the NH3 emissions during the manure agitation. The resulting NH3 concentrations were still not below the concentrations of NIOSH PELs. However, both biochars have the potential to reduce NH3 emissions.
These proof-of-the-concept experiments showed the short-term effect of mitigating acute NH3 emissions from swine manure agitation using biochar powder. The emissions were greatly reduced right after biochar powder was evenly applied to the manure surface. Both RO and HAP biochar showed significant (p < 0.05) reductions of NH3 emissions during the 3-mins of agitation and the maximum peak flux. Up to ∼80% reductions were observed for both dosages of HAP treatments, but no statistical differences between the treatments. During the post-agitation stages, the 6 mm dose of both types of biochar showed slightly higher % reductions than a 12 mm dose and no statistical differences between the dosages. Chen et al. (2020c) observed that most of the lower dosage of biochar powder was still floating on the manure surface. On the other hand, the 12 mm biochar dose was visibly wetter, was mixed more readily with manure, and open patches of not-covered manure were observed. Maurer et al. (2017a) observed similar (less effective) results with larger doses of surficially applied soybean peroxidase treatment to manure. The pH of RO biochar was 7.5, whereas HAP biochar was 9.2, which RO biochar should have theoretically better NH3 emission reductions as the pka of ammonia (NH3) to ammonium (NH4+) is 9.26. The effects of biochar pH could be explored further by applying custom blends of biochars to mitigate emissions from manure for a range of target gaseous pollutants and their pka’s.
Both dosages of HAP biochar showed significant (p < 0.05) impacts on the maximum cumulative NH3 flux, whereas the RO biochar only had 6 mm dosages showed a significant (p < 0.05) impact. The 12 mm dosages of RO biochar did not show a significant impact; one possible reason might be due to the relatively high standard deviations.
There was no significant impact of both types of biochar on the NH3 emission constant rate (k). For inflection time (a1) of the cumulative NH3 emissions, only (both) RO biochar treatments’ dosages showed significant impacts. The HAP biochar’s pH might be the reason that caused the no impact on the inflection time as a pka for NH3, and NH4+ is 9.26, and the HAP biochar was 9.2.
This study focused on the proof-of-the-concept for acute NH3 emissions mitigation and did not aim to compare the two types of biochar. The high variations among the replications could be caused by high heterogenicity of the stored manure properties (i.e., stratified, biologically active, not a well-mixed solution, with local solids aggregates, and zones with different physicochemical and biological properties). Therefore, one possible solution is to work with artificial surrogate manure (if a particular mechanism behind the mitigation needs to be isolated).
This study showed the potential uses of biochar to reduce the acute NH3 emissions from agitated stored manure. The next logical step we took was to explore the novel application of biochar and swine manure mixture to reduce nutrient leaching from soil and increase plant-available nutrients (Banik et al., 2021a,b). The study reported that using biochar and manure mixture significantly increased the organic matter, total carbon (C), and total N of the soil.
Thus, we propose using biochar to clean air, improve the safety of manure agitation, valorizing biochar-treated swine manure as a fertilizer, and improving nitrogen and carbon cycling in the animal-crop agriculture systems. Biochar can be first used to mitigate odorous gaseous emissions during long-term storage (Meiirkhanuly et al., 2020b), followed by the additional biochar addition immediately before the manure agitation (as shown in this study), largely lowering the occupational inhalation exposure risks for both NH3 and H2S (Chen et al., 2020c). After the swine manure and biochar mixture is pumped out and applied to soils, the mixture provides more nutrients and potentially lowers leaching risks for the crop field (Banik et al., 2021a,b).
Different types of biochar can be evaluated in the future because biochar can be made from many sources of abundant and low-value biomass and organic waste, and their properties can be modified, blended to achieve particular end-goals. Since this study is only a proof-of-the-concept, we also need to evaluate biochar’s performance in larger-scales (farm-scale) and using longer (e.g., 1∼3 h) agitation time. Also, considering the practical challenge of applying light powder biochar to a large manure surface, we proposed using pelletized biochar as more user-friendly and safer.
Conclusion
The highly alkaline and porous (HAP) and red oak (RO) biochar powder treatments have the potential to reduce the short-term NH3 emission during manure agitation. Both the 6 mm and 12 mm RO biochar treatment significantly (p < 0.0001) reduced the total emission of NH3 by 86.1 and 52.9%, respectively. The 6 mm and 12 mm RO biochar treatment resulted in a 61.2% (p = 0.0002) and 8.71% (p = 0.0214) reduction in the maximum peak flux of NH3, respectively. Both RO treatments significantly reduced the emissions during the 3-min agitation by 62.7% (6 mm) and 56.8% (12 mm). Both the 6 mm and 12 mm HAP biochar treatment significantly (p < 0.0001) reduced the total emission of NH3 by 74.5 and 70.4%, respectively. The 6 mm and 12 mm HAP biochar treatment resulted in 75.7% (p = 0.0215) and 63.3% (p = 0.0464) reduction in the maximum peak flux of NH3, respectively. Also, both 6 mm and 12 mm HAP treatments significantly (p < 0.0001) reduced the emissions during the 3-min agitation by 77.8 and 85.2%, respectively. A lower biochar dose (6 mm) appears to be as effective as the larger dose (12 mm). More research is needed to optimize and scale-up biochar’s effectiveness in mitigating NH3 emissions and retaining nutrients in manure.
Data Availability Statement
The original contributions presented in the study are included in the article/Supplementary Material, further inquiries can be directed to the corresponding author/s.
Author Contributions
JK and BC: conceptualization and methodology. BC and AB: software. JK and AB: validation and supervision. BC: formal analysis and writing, original draft preparation. BC, ML, HM, PL, and ZM: investigation. JK and RB: resources, project administration, and funding acquisition. BC, JK, and AB: data curation. BC, SO’B, JK, and AB: writing, review and editing. BC, HM, and AB: visualization. All authors have read and agreed to the published version of the manuscript.
Funding
This research was partially funded by the United States Department of Energy–National Institute for Food and Agriculture, grant # 2018-10008-28616: “Valorization of biochar: Applications in anaerobic digestion and livestock odor control” (2018–2020, PI RB). Partial support came from (1) the Iowa Agriculture and Home Economics Experiment Station, Ames, Iowa. Project no. IOW05556 (Future Challenges in Animal Production Systems: Seeking Solutions through Focused Facilitation) sponsored by Hatch Act and State of Iowa funds, JK; (2) the Ministry of Education and Science of the Republic of Kazakhstan for supporting ZM with an M.S. study scholarship via the Bolashak Program; and (3) the Fulbright Poland Foundation for funding the project titled “Research on pollutants emission from Carbonized Refuse Derived Fuel into the environment,” completed by AB at the Iowa State University.
Conflict of Interest
The authors declare that the research was conducted in the absence of any commercial or financial relationships that could be construed as a potential conflict of interest.
Supplementary Material
The Supplementary Material for this article can be found online at: https://www.frontiersin.org/articles/10.3389/fenvs.2021.613614/full#supplementary-material
References
Banik, C., Koziel, J. A., Bonds, D., Singh, A., and Licht, M. (2021a). Comparing biochar-swine manure mixture to conventional manure impact on soil nutrient availability and plant uptake – a greenhouse study. Preprints doi: 10.20944/preprints202102.0417.v2
Banik, C., Koziel, J. A., De, M., Bonds, D., Chen, B., Singh, A., et al. (2021b). Soil nutrients and carbon dynamics in the presence of biochar-swine manure mixture under controlled leaching experiment using a Midwestern Mollisols. Front. Environ. Sci. doi: 10.3389/fenvs.2021.609621 [Epub ahead of print].
Białowiec, A., Micuda, M., and Koziel, J. A. (2018). Waste to carbon: densification of torrefied refuse-derived fuel. Energies 11:3233. doi: 10.3390/en11113233
Cai, L., Koziel, J. A., Liang, Y., Nguyen, A. T., and Xin, H. (2007). Evaluation of zeolite for Control of odorants emissions from simulated poultry manure storage. J. Environ. Qual. 36, 184–193. doi: 10.2134/jeq2006.0052
Chen, B., Koziel, J. A., Banik, C., Ma, H., Lee, M., O’Brien, S. C., et al. (2021). Mitigation of gaseous emissions from stored swine manure with biochar: effect of dose and reapplication on a pilot-scale. Atmosphere 12:96. doi: 10.3390/atmos12010096
Chen, B., Koziel, J. A., Banik, C., Ma, H., Lee, M., Wi, J., et al. (2020a). Emissions from swine manure treated with current products for mitigation of odors and reduction of NH3, H2S, VOC, and GHG emissions. Data 5:54. doi: 10.3390/data5020054
Chen, B., Koziel, J. A., Banik, C., Ma, H., Lee, M., Wi, J., et al. (2020b). Mitigation of odor, NH3, H2S, GHG, and VOC emissions with current products for use in deep-pit swine manure storage structures. Front. Environ. Sci. 8:613646. doi: 10.3389/fenvs.2020.613646
Chen, B., Koziel, J. A., Białowiec, A., Lee, M., Ma, H., Li, P., et al. (2020c). The impact of surficial biochar treatment on acute H2S emissions during swine manure agitation before pump-out: proof-of-the-concept. Catalysts 10:940. doi: 10.3390/catal10080940
Dougherty, B., Gray, M., Johnson, M. G., and Kleber, M. (2017). Can Biochar covers reduce emissions from manure lagoons while capturing nutrients? J. Environ. Qual. 46, 659–666. doi: 10.2134/jeq2016.12.0478
Hanusz, Z., Siarkowski, Z., and Ostrowski, K. (2008). Application of the Gompertz model in agricultural engineering. Inz. Rol. 7, 71–77.
Hoff, S. J., Bundy, D. S., Nelson, M. A., Zelle, B. C., Jacobson, L. D., Heber, A. J., et al. (2006). Emissions of ammonia, hydrogen sulfide, and odor before, during and after slurry removal from a deep-pit swine finisher. J. Air Waste Manag. Assoc. 56, 581–590. doi: 10.1080/10473289.2006.10464472
Kalus, K., Konkol, D., Korczyński, M., Koziel, J. A., and Opaliński, S. (2020a). Effect of biochar diet supplementation on chicken broiler performance, NH3 and odor emissions, and meat consumer acceptance. Animals 10:1539. doi: 10.3390/ani10091539
Kalus, K., Konkol, D., Korczyński, M., Koziel, J. A., and Opaliński, S. (2020b). Laying hens biochar diet supplementation—effect on performance, excreta N content, NH3 and VOCs emissions, egg traits and egg consumers acceptance. Agriculture 10:237. doi: 10.3390/agriculture10060237
Kalus, K., Koziel, J. A., and Opaliński, S. (2019). A review of biochar properties and their utilization in crop agriculture and livestock production. Appl. Sci. 9:3494. doi: 10.3390/app9173494
Kalus, K., Opaliński, S., Maurer, D., Rice, S., Koziel, J. A., Korczyński, M., et al. (2017). Odour reducing microbial-mineral additive for poultry manure treatment. Front. Environ. Sci. Eng. 11:7. doi: 10.1007/s11783-017-0928-4
Lee, M., Wi, J., Koziel, J. A., Ahn, H., Li, P., Chen, B., et al. (2020). Effects of UV-A light treatment on ammonia, hydrogen sulfide, greenhouse gases, and ozone in simulated poultry barn conditions. Atmosphere 11:283. doi: 10.3390/atmos11030283
Maurer, D. L., Koziel, J. A., Bruning, K., and Parker, D. B. (2017a). Farm-scale testing of soybean peroxidase and calcium peroxide for surficial swine manure treatment and mitigation of odorous VOCs, ammonia and hydrogen sulfide emissions. Atmos. Environ. 166, 467–478. doi: 10.1016/j.atmosenv.2017.07.048
Maurer, D. L., Koziel, J. A., Bruning, K., and Parker, D. B. (2017b). Pilot-scale testing of renewable biocatalyst for swine manure treatment and mitigation of odorous VOCs, ammonia and hydrogen sulfide emissions. Atmos. Environ. 150, 313–321. doi: 10.1016/j.atmosenv.2016.11.021
Maurer, D. L., Koziel, J. A., Harmon, J. D., Hoff, S. J., Rieck-Hinz, A. M., and Andersen, D. S. (2016). Summary of performance data for technologies to control gaseous, odor, and particulate emissions from livestock operations: air management practices assessment tool (AMPAT). Data Brief. 7, 1413–1429. doi: 10.1016/j.dib.2016.03.070
Maurer, D. L., Koziel, J. A., Kalus, K., Anderson, D. S., and Opalinski, S. (2017c). Pilot-scale testing of non-activated biochar for swine manure treatment and mitigation of ammonia, hydrogen sulfide, odorous volatile organic compounds (VOCs) and greenhouse gas emissions. Sustainability 9:929. doi: 10.3390/su9060929
Meiirkhanuly, Z., Koziel, J. A., Białowiec, A., Banik, C., and Brown, R. C. (2019). The-proof-of-concept of biochar floating cover influence on water pH. Water 11:1802. doi: 10.3390/w11091802
Meiirkhanuly, Z., Koziel, J. A., Białowiec, A., Banik, C., and Brown, R. C. (2020a). The proof-of-the concept of biochar floating cover influence on swine manure pH: implications for mitigation of gaseous emissions from area sources. Front. Chem. 8:656. doi: 10.3389/fchem.2020.00656
Meiirkhanuly, Z., Koziel, J. A., Chen, B., Białowiec, A., Lee, M., Wi, J., et al. (2020b). Mitigation of gaseous emissions from swine manure with the surficial application of biochars. Atmosphere 11:1179. doi: 10.3390/atmos11111179
MidWest Plan Service (1983). Structures and Environment Handbook, 11 Edn. Ames, IA: Midwest Plan Service.
Ni, J. Q., Heber, A. J., Sutton, A. L., and Kelly, D. T. (2009). Mechanisms of gas releases from swine wastes. Trans. ASABE 52, 2013–2025. doi: 10.13031/2013.29203
NIOSH (1997). NIOSH Pocket Guide to Chemical Hazards. Cincinnati, OH: National Institute for Occupational Safety and Health.
Parker, D. B., Hayes, M., Brown-Brandl, T., Woodbury, B. L., Spiehs, M. J., and Koziel, J. A. (2016). Surface application of soybean peroxidase and calcium peroxide for reducing odorous VOC emissions from swine manure slurry. Appl. Eng. Agric. 32, 389–398. doi: 10.13031/aea.32.11672
Pulka, J., Manczarski, P., Koziel, J. A., and Białowiec, A. (2019). Torrefaction of sewage sludge: kinetics and fuel properties of biochars. Energies 12:565. doi: 10.3390/en12030565
Stępień, P., Serowik, M., Koziel, J. A., and Białowiec, A. (2019a). Waste to carbon: estimating the demand for production of carbonized refuse-derived fuel. Sustainability 11:5685. doi: 10.3390/su11205685
Stępień, P., Świechowski, K., Hnat, M., Kugler, S., Stegenta-DŁbrowska, S., Koziel, J. A., et al. (2019b). Waste to carbon: biocoal from elephant dung as new cooking fuel. Energies 12:4344. doi: 10.3390/en12224344
Świechowski, K., Stegenta-DŁbrowska, S., Liszewski, M., BŁbelewski, P., Koziel, J. A., and Białowiec, A. (2019). Oxytree pruned biomass torrefaction: process kinetics. Materials 12:3334. doi: 10.3390/ma12203334
Syguła, E., Koziel, J. A., and Białowiec, A. (2019). Proof-of-concept of spent mushrooms compost torrefaction–studying the process kinetics and the influence of temperature and duration on the calorific value of the produced biocoal. Energies 12:3060. doi: 10.3390/en12163060
Keywords: waste management, nitrogen, air pollution, agricultural safety, fertilizer, biocoal, odor, Gompertz model
Citation: Chen B, Koziel JA, Białowiec A, Lee M, Ma H, O’Brien S, Li P, Meiirkhanuly Z and Brown RC (2021) Mitigation of Acute Ammonia Emissions With Biochar During Swine Manure Agitation Before Pump-Out: Proof-of-the-Concept. Front. Environ. Sci. 9:613614. doi: 10.3389/fenvs.2021.613614
Received: 05 October 2020; Accepted: 17 March 2021;
Published: 09 April 2021.
Edited by:
Andrew Hursthouse, University of the West of Scotland, United KingdomReviewed by:
Zhengqin Xiong, Nanjing Agricultural University, ChinaSven Gjedde Sommer, Aarhus University, Denmark
Michael Edward Deary, Northumbria University, United Kingdom
Copyright © 2021 Chen, Koziel, Białowiec, Lee, Ma, O’Brien, Li, Meiirkhanuly and Brown. This is an open-access article distributed under the terms of the Creative Commons Attribution License (CC BY). The use, distribution or reproduction in other forums is permitted, provided the original author(s) and the copyright owner(s) are credited and that the original publication in this journal is cited, in accordance with accepted academic practice. No use, distribution or reproduction is permitted which does not comply with these terms.
*Correspondence: Jacek A. Koziel, koziel@iastate.edu