- 1Department of Agricultural and Biosystems Engineering, Iowa State University, Ames, IA, United States
- 2Department of Agronomy, Iowa State University, Ames, IA, United States
- 3Department of Biological Sciences, Minnesota State University, Mankato, MN, United States
- 4Department of Horticulture, Iowa State University, Ames, IA, United States
Biochar application to the soil can improve soil quality and nutrient leaching loss from swine manure adapted soils. Our working hypothesis was that the biochar-incubated with manure could be a better soil amendment than conventional manure application. The manure-biochar application to the soil would decrease nutrient leaching from manure and increase plant-available nutrients. The study objectives were to 1) assess the physicochemical properties of the manure-biochar mixture after lab incubation and 2) evaluate the impact of biochar-treated swine manure on soil total C, N, and other major and minor nutrients in comparison to conventional manure application to soil. Three biochars 1) neutral pH red-oak (RO), 2) highly alkaline autothermal corn (Zea mays) stover (HAP), and 3) mild acidic Fe-treated autothermal corn stover (HAPE) were incubated with swine manure for a month. The biochar-manure mixture was applied in triplicate to soil columns with an application rate determined by the P2O5-P content in manure or manure-biochar mixtures after the incubation. The ammonium (NH4+), nitrate (NO3‒), and reactive P concentrations in soil column leachates were recorded for eight leaching events. Soil properties and plant-available nutrients were compared between treatments and control manure and soil. Manure-(HAP&HAPE) biochar treatments significantly increased soil organic matter (OM), and all biochar-manure mixture increased (numerically) soil total C, N, and improved soil bulk density. Concentrations of NH4+ and NO3‒ significantly increased in MHAPE column leachates during this 4-week study and the KCl-extractable NH4+ and NO3‒ in the soil at the end of the experiment. A significant reduction in soil Mehlich3 Cu was also observed for the manure-HAPE mixture compared with the manure control. The manure-red oak biochar significantly increased the soil Mn availability than other manure-biochar treatments or manure control. Overall, the manure-biochar incubation enabled biochar to stabilize the C and several nutrients from manure. The subsequent manure-biochar mixture application to soil improved soil quality and plant nutrient availability compared to conventional manure application. This proof-of-the-concept study suggests that biochars could be used to solve both environmental and agronomic challenges and further improve the sustainability of animal and crop production agriculture.
Introduction
Swine manure is a source of valuable nutrients (Chastain et al., 1999), but mismanagement or improper application to soils makes it a potential environmental threat. The application rate of animal manure is often exceeded beyond the plant requirement (Juergens-Gschwind, 1989), and this excessive manure application to the soil can result in an unintended N leaching loss to groundwater (Beckwith et al., 1998). High liquid manure application to soil could also contribute to heavy metals like copper and zinc accumulation to soil and plant systems that generate a risk of contaminating the animal food chain to a threat (Mantovi et al., 2003).
Corn (Zea mays) and soybeans (G. max) crop rotation is a common practice in Midwest United States, and the application of swine manure to fields has been a common practice to both dispose of the stored manure and to provide nutrients for crop growth. Soybean can positively respond to swine manure application as reported by previous studies (Killorn, 1998; Sawyer, 2001). Manure solids, undigested feed, and bedding material in the manure help build soil organic matter, improving soil structure and increasing soil water holding capacity and reducing nutrient leaching loss (Magdoff and Es, 2009). However, the use of liquid swine manure to soil may not increase soil C sequestration; instead, it can increase the native soil C decomposition (Angers et al., 2009), and as a result, leaching loss of macronutrients such as N and sedimental loss of P (Reid et al., 2018) can occur. Furthermore, C and N losses can also occur via greenhouse gas emissions from land-applied swine manure (Maurer et al., 2017a).
Biochar, a product of biomass pyrolysis, heating under low or no O2 conditions, has attracted much interest as a means of potentially solving soil problems (Laird, 2008). Biochar properties can be useful to address challenges in crop and livestock agriculture (Kalus et al., 2019). Freeze dry manure fertilization to the soil in the presence of biochar has shown a significant decrease in nutrient leaching loss, greater retention of plant nutrients, and improvement in soil C and N compared with control manure-treated soil (Laird et al., 2010a; Laird et al., 2010b). The same study also reported that biochar amendments followed by manure application increase the cation and anion exchange capacity of biochar, enable biochar to both adsorb and release nutrients to/from the soil, hence functioning as both a reservoir and slow-release source of plant nutrients. Biochar properties can be useful to address challenges in crop and livestock agriculture, as recently reviewed elsewhere (Kalus et al., 2019).
The application of alkaline biochar to soil can increase soil pH and reduce problems related to low soil pH, which typically occur after prolonged application of ammonium forms of N fertilizer. Studies have shown that biochar improves soil water holding capacity and is able to reduce soil bulk density (BD) (Rogovska et al., 2014). Unfortunately, most biochars have few positively charged surface sites and hence limited anion exchange capacity and ability to electrostatically adsorb nutrient anions such as PO43‒ and NO3‒ (Lawrinenko and Laird, 2015). There are consistent data in the literature supporting the electrostatic retention of positively charged ammonium (NH4+) but not of negatively charged PO43‒ and NO3‒ on biochar surfaces (Yao et al., 2012; Fidel et al., 2018). Iron (Fe) modification of biochar surfaces has been shown to be effective in enhancing PO43‒ adsorption (Wilfert et al., 2015).
In addition to biochar being proposed as a soil amendment, recent studies have shown that surficial application of biochar onto the swine manure can reduce emissions of odorous gases (Meiirkhanuly, 2019) and reduce volatilization loss of ammonia (Maurer et al., 2017b) or in other words biochar addition can improve the N content of manure in storage. Clearly, there is an opportunity to explore the tantalizing question if biochar can be used to address both environmental and crop production challenges in one system. We propose a novel concept of biochar utilization that can simultaneously improve the air quality of the animal production system and the sustainability of crop production agriculture. Biochar can be first used to mitigate gaseous emissions (Chen et al., 2020; Meiirkhanuly et al., 2020a) from stored manure and possibly retain more nutrients in the manure. When the swine manure pits are agitated and cleaned out, the mixture of swine manure and biochar will be pumped out and applied to soils. Environmental and agronomic benefits are expected due to decrease nutrient leaching from manure and increase plant-available nutrients. Biochar pH is important in this regard, and surficial treatment of an alkaline/neutral pH biochar to manure storage can change the manure pH near the manure-air interface within few days of application (Meiirkhanuly et al., 2020b). However, there is a gap in the literature on the use of the biochar-manure mixtures as a soil amendment to replace the conventional use of liquid swine manure. Also, the prospect of functionalizing biochar to improve the environmental sustainability of primary nutrients (e.g., P management with Fe-modified biochar surfaces) can be explored.
The study objectives were to 1) assess the physicochemical properties of the manure-biochar mixture after laboratory incubation and 2) evaluate the impact of biochar-treated swine manure on soil total C, N, and other major and minor nutrients in comparison to conventional manure application to soil. Our working hypothesis was that the biochar-treated manure application to the soil would decrease nutrient leaching from manure, increase plant-available nutrients, and improve soil, irrespective of the biochar feedstock type or the different biochar production process. We also hypothesized that differences among biochars influence soil nutrient availability and leaching. Three biochars 1) neutral pH red-oak (RO), 2) highly alkaline autothermal corn stover (HAP), and 3) mild acidic Fe-treated autothermal corn stover (HAPE) were incubated with swine manure for a month. This was followed by a controlled column leaching experiment for soils treated with biochar-manure mixtures followed. We characterized some physicochemical properties of the biochar-manure mixtures and investigated the impact of biochar-manure treatments on soil nutrient leachate, soil physicochemical properties (pH, bulk density, total C and N), and plant macronutrients N, P, and K availability. This research also addressed the impact of Fe-modified biochar application on manure to sorb nutrients (P) followed by soil application as an amendment.
Materials and Methods
Soil, Biochar, Manure, and Manure-Biochar Incubation
Hanlon (Coarse-loamy, mixed, superactive, mesic Cumulic Hapludolls) soil was collected from the iowa State University Applied Science/Moore research farm. Soybean and corn were mainly grown in rotation in these plots, and no swine manure application history in the last 20 years. Samples of soil surface (0–10 cm) were collected and stored in buckets with lids to keep the moisture at the field level. The buckets were stored at 4°C until analysis started two months after collection. The soil used in the study had a pH of 7.6, containing 2.84% of organic matter, 1.88% of total C and 0.17% of total N, and a TC/TN ratio of 11.1.
Fast pyrolysis (500°C) neutral pH (pH ∼7.5) red oak biochar (<2 cm) was obtained from a commercial producer (Avello Bioenergy, Iowa), and a fast pyrolysis high pH (pH∼ 9.2) corn stover biochar (HAP) and Fe-modified corn-stover (500°C) biochar (HAPE) with a moderately acidic pH were obtained by autothermal pyrolysis. A detail of the pyrolysis techniques of HAP and HAPE biochar production is given elsewhere (Polin et al., 2019; Rollag et al., 2020). The biochar properties, moisture, volatile matters, fixed C, ash content, total C, and total N were determined by following the method described by Rover et al. (2018).
The swine manure was collected from deep pit storage at an iowa Select Farms facility in the fall of 2019. The manure was stored in a bucket with a lid and stored (at 22–23°C) until incubated with biochar within one month of manure collection. About 250 g biochar (<1 mm) was surface applied on 1,000 g of manure and incubated (at 22–23°C) in an 8.5 L glass container (10 cm i. d. and 27 cm height) for one month at atmospheric condition. After the incubation period, the biochar and manure were mixed thoroughly to homogenize and stored in airtight glass at 4°C until analysis started one month later. A control manure sample was also incubated and mixed thoroughly under the same condition for comparison.
The mixture was analyzed for moisture, total C (TC), total N (TN), mineral content, organic matter (OM), nitrate-N, ammonium-N, P2O5-P, and K2O-K, and the data was provided on a % dry weight basis. Moisture and dry matter in the samples were measured by heating the samples for 16 h at 105–110°C. Organic matter was determined by heating the samples in a muffle furnace at 550°C for 2 h. The total C and N were analyzed by combustion using Elementar Vario Max CN Method 4.01 and 3.3, respectively. Sample nitrate and ammonium-N were measured by KCl extraction and determined on the FIA Lab flow injection autoanalyzer.
Soil Column Preparation and Leaching Experiment
The field moist soil was dried, mixed thoroughly to homogenize, sieved (<2 mm), and stored in a bucket with a lid for the column preparation. A total of 15 soil columns (25 cm height and 4.4 cm i. d.) were built from PVC tubes with a PVC male adapter sealed at the bottom using PVC cement. At the base of each column, 4.4 i. d. “air filter pad” was inserted, and then ∼2 g of 1 mm glass beads were added on top of that coarse sieve. Each column was filled with 250 g of dried (<2 mm) soil to a length of 15 cm, maintaining an approximate bulk density (BD) of 1.2 g/cm3. Water was then filled from the bottom of each column to the top of the soil to remove excess air trapped in the soil column and drained the water under gravity (Figure 1; Supplementary Figure S1) after 24 h. Pore volume was calculated from the weight difference of the before and after saturation of the columns. Based on the pore volume, 50 ml of DI water was added for each leaching event.
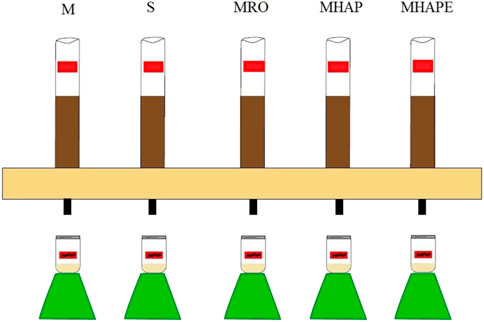
FIGURE 1. A schematic of the soil column leachate collection apparatus. Each column contains 250 g of soil, treated with one of five treatments. Two of these apparatuses were utilized, resulting in 15 columns; three trials for each of the five treatments. M = manure control; S = soil control; MRO = manure + red oak biochar; MHAP = manure + corn biomass feedstock autothermal alkaline porous biochar; MHAPE = manure + corn biomass feedstock autothermal porous Fe-engineered biochar. Supplementary Figure S1 for a photograph of the experimental setup.
De-ionized (DI) water (50 ml) was added from the top of each column, and the leachate was collected from the bottom to collect the baseline soil data two times during one week of column equilibration time. The columns were named depending on the treatments they received, M = manure control (manure added to the soil); S = soil control; MHAP = manure + corn biomass autothermal alkaline porous biochar; MHAPE = manure + corn feedstock autothermal porous Fe-engineered biochar. This study reported the data of a total of 15 columns with five treatments, three replicates for each treatment. The amount of biochar-manure mixture or manure addition was calculated based on the P2O5-P content of the mixture to make sure each column gets a recommended rate of P of 135 kg/ha (120 lb/acre) of soil for a corn-soybean rotational plot (Sawyer and Mallarino, 2016). Treatments were surface applied, and micro tillage was performed with a spatula to mix the treatments with to 3 cm of soil. The same tillage operation was also applied for the control of soil columns. After a week of column equilibration, 50 ml DI water from a beaker was added from the top of each column every three to four days (i.e., every leaching event) over three weeks period.
The leachate was collected overnight in labeled bottles, and the next morning the leachate was transferred to <0°C until analysis. Leachate was collected eight times (eight events) for the total experiment time. Leachates were filtered through a 0.45 µm syringe filter and analyzed for nitrate-N (vanadium III, sulfanilamide and N-(1-naphthyl)-ethylenediamine dihydrochloride), ammonium-N (salicylate and ammonia cyanurate method), and dissolved reactive phosphorus (DRP; malachite green method; D’Angelo et al., 2001) using a Synergy HTX Multi-Mode microplate reader (BioTek Instruments, Inc.) colorimetric method (Doane and Horwáth, 2003; De et al., 2019).
Column Soil Analysis
After the leachate collection was over, the columns were left for a week to drain the excess water from the clogged columns. Once the excess water drained out, the soil from each column was loosened using a long spatula, and soils were collected in Ziploc bags. The soil receiving treatments and control soil was then mixed thoroughly to homogenize, dried, sieved (<2 mm), and analyzed for pH (1:1; soil: water) following the method by McLean (1982) using the glass-electrode meter method. About 2 g soil was weighed, and soil OM was measured by loss on ignition at 360°C by (Schulte and Hopkins, 1996) method; and the total C and N were analyzed by combustion using Elementar Vario Max CN Method 4.01 and 3.3, respectively (Nelson and sommers, 1996). The extractant was prepared by weighing approximately 5 g soil in a 200 ml Nalgene bottle, shaken with KCl at 1:5 ratio for 30 min, and then filtered through Whatman grade 1 filter paper. This extractant was used to measure KCl-extractable soil nitrate-N (vanadium III, sulfanilamide, and N-(1-naphthyl)-ethylenediamine dihydrochloride) and ammonium-N (salicylate and ammonia cyanurate method) (Doane and Horwáth, 2003; De et al., 2019). The Mehlich3 extractable elements (P, K, Ca, Mg, Fe, Mn, Cu, and Zn) were extracted by a modified method of Mehlich (1984) and analyzed by ICP-OES.
Statistical Analysis
The statistical analysis was completed using R. The experiment has three biochar manure mixture, one manure control, and one soil control, with three replicates of each treatment a total of 15 columns. A mixed model was run to analyze the soil column leachate considering time as a factor, then Tukey’s pairwise comparison was used to compare treatment effect on total nitrate-N, ammonium-N, and DRP. To report the treatment effects on soil nitrate, ammonium, P, and all Mehlich3 extractable elements, a one-way ANOVA and Tukey’s pairwise comparison was performed. A p-value <0.05 was considered statistically significant.
Results
Biochar and Manure Properties Before Mixture Incubation
The swine manure used in this experiment had an alkaline pH of 9.2. Swine manure used in this study contained 37.4% TC and 18.1% of TN, and the TC/TN ratio was 2.1. As recorded, the pH of the autothermal corn stover biochar was mild acidic for HAPE (pH of 5.4) to highly alkaline (9.2) for HAP, and the pH of hardwood RO was 7.5, close to soil pH. The hardwood biochar had 78.5% TC and 0.6% TN by mass and contained 26.4% volatile matters, 15.8% ash, 54.8% fixed C by mass, as indicated by proximate analysis. Whereas the autothermal biochar HAP had 61.4% C and 1.2% TN by mass and contained 16.3% volatile matter, 46.8% ash, 35.0% fixed C by mass, as indicated by proximate analysis. The Fe-pretreated autothermal biochar HAPE had 36.4% TC and 1.2% TN by mass and contained 34.0% volatile matters, 40.0% ash, 24.0% fixed C by mass, as indicated by proximate analysis. The TC/TN ratio ranged between 30 and 130 among the biochars; hardwood RO had the highest carbon content (total and fixed), thus the highest TC/TN ratio. The ash content of RO biochar was the lowest among the three-biochar used in this experiment.
Incubation Effect on Biochar-Manure Physicochemical Parameters
The addition of biochar to the manure changes the physical appearance of manure (Supplementary Figure S2). After the one-month incubation, the control manure was liquid slurry with yellow patches on the surface, possibly representing a microbial colony developed during incubation and a persistent manure odor. On the contrary, no such color or odor was observed for any of the biochar samples. During incubation, biochar absorbed the manure moisture, and after mixing to homogenize, its texture resembled loose soil. The moisture content of biochar increased several folds by soaking the manure moisture (Table 1). As a result of incubation, the pH increased for all biochar-manure mixtures. An increase in total org-N for the biochar-manure mixture was observed for all biochar mixtures; however, the TC/TN ratio dropped for all biochar-manure mixtures compared with the biochar. The inorganic nutrients N, P, and K contents of all mixtures increased than biochar during incubation with manure (Table 1).
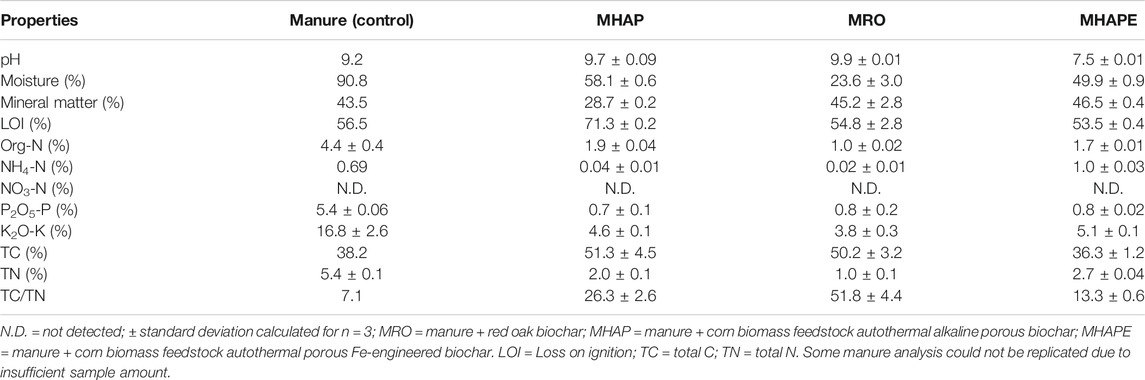
TABLE 1. Physicochemical properties of the different biochar-manure mixtures after incubation. Except for pH, all values were reported on a % dry weight basis.
The amount of treatment addition was based on the recommended rate of P (135 kg/ha or 120 lb/acre) to soil resulted in an addition of a different amount of all macro and micronutrients to each treatment (Table 2). Treatments end up with approximately 3.1 g of manure, 1.8 g of MRO, 4.9 of MHAP, and 3.25 g of MHAPE. The highest (by weight) amount of MHAP amendment added to each column resulted in significantly more N, K, Ca, and Mg addition than any other treatments. The biochar to manure ratio was the same for incubation; additionally, the total N of both HAP and HAPE was 1.2%, but the total N added by the MHAPE treatment was significantly lower than MHAP (Table 2). The control manure treatments added significantly more Cu (except for MHAPE) and Zn to soil than any manure-biochar treatment, and among all treatments, MRO treatment added the lowest amount of those two elements.

TABLE 2. Mass of elements added to the columns (mg) by the manure and manure-biochar treatment application. Different letters signify statistical differences between treatments at p < 0.05 (column-wise).
Nutrients in Leached Water
Dissolved inorganic N and P leached out from all columns irrespective of treatments were low during this four-week study. However, the MHAPE treated columns released significantly high total NO3-N (p = 0.003) and NH4-N (p = 0.003) than manure control columns. An upward trend with time was observed for the cumulative NO3-N and NH4-N concentration in MHAPE treated column leachate during the last six leaching events (Figure 2, Supplementary Table S1), whereas control manure-treated columns NO3-N started to increase in leachate on 7th and 8th leaching events. At the beginning of the column experiment, the MHAPE had about 1% of ammonium (Table 1); higher concentrations in comparison to other treatments.
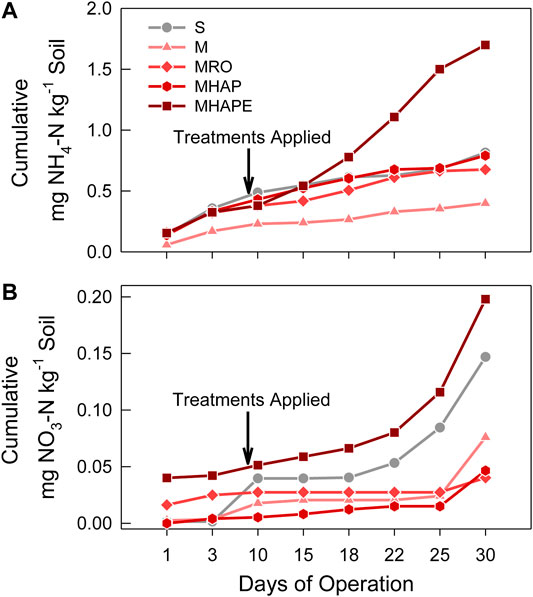
FIGURE 2. The effect of treatment on leachate cumulative concentrations of soil (A) ammonium and (B) nitrate. The mean was calculated for a sample of 3 replications. S = soil control; M = manure control; MRO = manure + red oak biochar; MHAP = manure + corn biomass feedstock autothermal alkaline porous biochar; MHAPE = manure + corn biomass feedstock autothermal porous Fe-engineered biochar.
An upward trend with time for the cumulative dissolved P in leachate for all treated columns was observed during the eight events of the leaching study; soil control columns released significantly (p < 0.05) higher amount of total DRP. However, no impact of manure or manure-biochar mixture applications to DRP in soil leachates were observed during the course of this study (Figure 3, Supplementary Table S1).
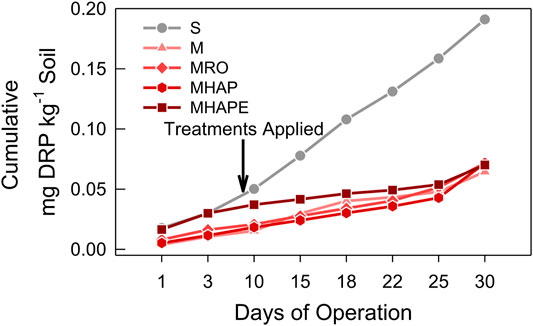
FIGURE 3. The effect of treatment on cumulative leachate concentrations of soil DRP. The mean was calculated for a sample of 3 replications. S = soil control; M = manure control; MRO = manure + red oak biochar; MHAP = manure + corn biomass feedstock autothermal alkaline porous biochar; MHAPE = manure + corn biomass feedstock autothermal porous Fe-engineered biochar.
Treatment Effect on Column Soil Properties
The columns were freely drained for the first four leachings, but the rate of water leaching for the last two events slowed; specifically, in the manure-treated columns. After eight events of leachate collection, the experiment was stopped due to longer leaching time and ponding on column surfaces. Columns with soil and biochar-manure treatments were relatively better drained in comparison to manure columns. Before the treatment application, the starting BD of each column was 1.2. After the treatment application and leaching experiment, the final soil BD ranged from 1.17 to 1.6 g/cm3, and for manure treated columns ended with higher BD in comparison to biochar-manure mixture receiving columns (Table 3). The application of manure and manure-biochar mixtures to the soil columns resulted in an increasing trend in the soil OM content, soil TC, and soil TN relative to control soil columns (Table 3).

TABLE 3. Column soil physicochemical properties after leaching events were completed, including the pH, bulk density, percentage of organic matter (OM), percentage of total C (TC), percentage of the total N (TN), and the total carbon to nitrogen (C/N) ratio. (Mean +/− standard deviation for n = 3 replicates; values in parentheses represent p-values; Bold signifies statistical significance for the difference from manure treatment (M) as control).
The TC/TN ratios were between 10.6 and 12.6. Manure-HAP and MHAPE biochar treated columns had significantly (p < 0.05) higher OM than manure or soil control columns. There was no significant change in TC and TN observed among manure or manure-biochar treatments. A slight change was observed in soil pH; manure-RO was significantly (p = 0.04) higher than manure and among all columns. The pH was mostly got buffered for the manure and manure-biochar treated columns and ranged between 7.3 and 7.6, i.e., close to soil pH. Before application to soil, the pH of all manure and manure-biochar mixtures, except HAPE, were highly alkaline (Table 1).
Before application to soil columns, manure had a %TN value of 5.4, the highest among any manure-biochar samples (Table 1). Manure-HAPE had 2.7% (1.7% Org-N +1% NH4-N) of TN before addition to soil columns; the highest %TN among the manure-biochar mixtures used in this study slightly increased the soil TN. In addition, MHAPE biochar treatment significantly increased soil NO3-N (p = 0.009) and NH4-N (p = 0.001) concentration than manure control after the leaching experiment (Figure 4). The soil columns not receiving any manure or biochar-manure mixture were not significantly different in NO3‒ and NH4+ than the control M, or MRO and MHAP treatments.
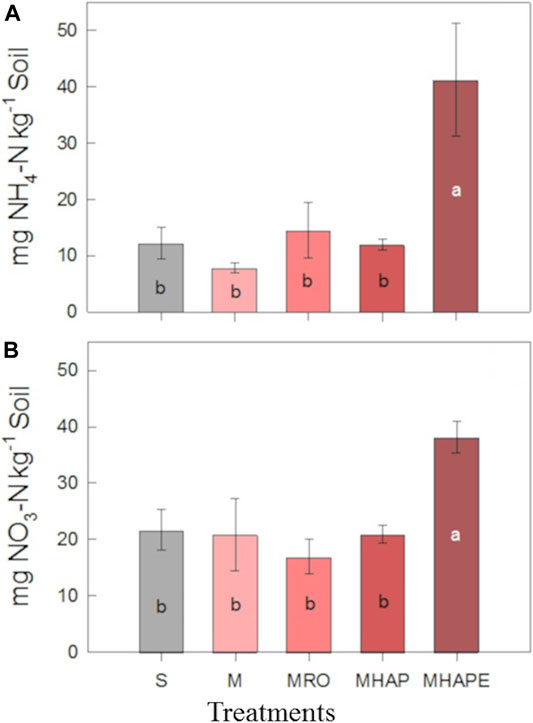
FIGURE 4. The effect of treatment on concentration of soil (A) ammonium and (B) nitrate. Soils from each column were homogenized and analyzed after the leaching experiment. Each bar represents an average of the concentrations of three replicates for that group. Error bars show the standard deviation (n = 3). S = soil control; M = manure control; MRO = manure + red oak biochar; MHAP = manure + corn biomass feedstock autothermal alkaline porous biochar; MHAPE = manure + corn biomass feedstock autothermal porous Fe-engineered biochar. Letters mark a significant difference between treatments at p < 0.05.
Manure addition to soil columns had the highest soil Mehlich3 extractable P (Figure 5) and significantly higher (p < 0.001) in comparison to other treatments. Among the manure-biochar mixture treated soils, manure-RO (p < 0.05) and manure-HAP (p < 0.05) significantly increase Mehlich3 soil P and K than control soil. The manure-HAPE treatment did show a small numerical increase in Mehlich3 P (not significant; p > 0.05). However, Mehlich3 soil K concentration was highest and significantly higher (p < 0.001) than other manure biochar treatments (Figure 6) though the manure had a higher concentration of K2O-K compared with the manure-biochar mixtures.
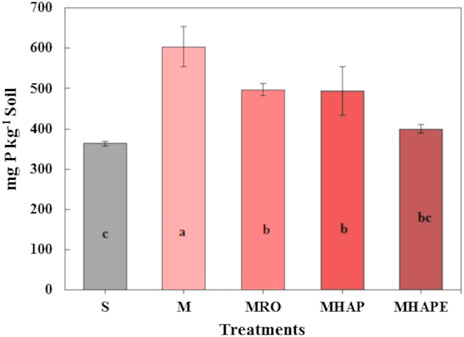
FIGURE 5. Comparison between treatments of the Mehlich3 extractable soil phosphorus. Soils from each column were homogenized and analyzed after the leaching experiment. Each bar represents an average of the concentrations of three replicates for that group. Error bars show the standard deviation (n = 3). S = soil control; M = manure control; MRO = manure + red oak biochar MHAP = manure + corn biomass feedstock autothermal alkaline porous biochar; MHAPE = manure + corn biomass feedstock autothermal porous Fe-engineered biochar. Letters mark a significant difference between treatments at p < 0.05.
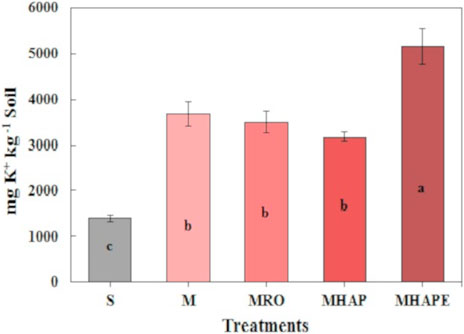
FIGURE 6. Comparison between treatments of the Mehlich3 extractable soil potassium. Soils from each column were homogenized and analyzed after the leaching experiment. Each bar represents an average of the concentrations of three replicates for that group. Error bars show the standard deviation (n = 3). S = soil control; M = manure control; MRO = manure and red oak biochar; MHAP = manure + corn biomass feedstock autothermal alkaline porous biochar; MHAPE = manure + corn biomass feedstock autothermal porous Fe-engineered biochar. Letters mark a significant difference between treatments at p < 0.05.
Biochar-manure mixture addition to soil columns did not impact the soil Mehlich3 extractable Ca and Fe concentrations (Table 4). However, MHAPE treatment had a significantly lower concentration of Mehlich3 extractable Mg (p = 0.03) and Cu (p = 0.005) than manure control. All manure-biochar treatments had a significantly (p < 0.05) low Mehlich3 extractable Zn compared to the control manure samples, and only MRO treatment had significantly high Mn (p = 0.02) compared to the control manure but not to the control soil column.

TABLE 4. Column soil physicochemical properties after leaching events were completed, including the elements, Ca, Mg, Fe, Cu, Mn, and Zn in mg/kg. (Mean ± standard deviation for n = 3 replicates; values in parentheses represent p-values; Bold signifies significantly different from manure treatment (M) as control).
Discussion
The high decomposition rate of soil OM can result in low C-sequestration and high loss of C to the atmosphere. Manure with a TC/TN ratio of ∼7 after incubation and even lower before incubation (∼2) (Table 1) supports the notion of N mineralization and volatilization loss to the atmosphere, more likely N loss as NH3 than C loss as CH4 or CO2. Substrate quality is an important deciding factor of its decomposition rate; a low TC/TN ratio means high quality and a readily available substrate to decomposing microbial group (Condron et al., 2010). Incubation of biochar (with a very high C/N ratio) with manure may have resulted in an N immobilization and improved the manure-biochar mixture total C/N ratio.
The treatment MRO had a C to N ratio of 51.8 after incubation, which ended up to 10.6 to the soil after the leaching experiment. Similarly, other manure-biochar mixtures resulted in a total C to N ratio near the soil total C to N ratio (∼12) upon application to the soil as an amendment. Also, an increase in OM, total C, and N speculates that the mixture has the capacity to improve C sequestration compared to manure only treatment. The addition of hardwood biochar showed a reduction in the mineralization loss of dairy manure C when biochar and manure were added to the field simultaneously, reported by a study by Lentz and Ippolito (2012). Manure-biochar mixture can alter soil physicochemical properties in a way that improves nutrient leaching loss from manure. This is because biochar application significantly increases the soil-specific surface area, is also capable of holding the plant available moisture considerably, and improved other plant nutrients availability effectively than soil (Laird et al. 2010b). Our data support the finding of Laird et al. 2010a resulting in a low BD and relatively high OM content in soil with biochar treatments to the manure-biochar soil system.
The soil used in this study was collected right after soybean harvest, and the crop impact may have reflected in soil NO3‒ and NH4+ concentrations; only MHAPE treated column had significantly high NO3‒and NH4+. Although the total N addition by manure-biochar mixture amendment was lower for MHAPE columns than manure treated column (Table 2), the MHAPE treatment resulted in an increase in soil KCl extractable inorganic-N (NO3‒ + NH4+) than control manure treatment. This observation suggests that MHAPE treatment may have increased soil microbial activity. The N-mineralization increase with biochar addition is consistent with previous studies that interpret biochar addition is linked with increases in soil microbial respiration (Laird et al., 2010a; Rogovska et al., 2011), which increases soil N mineralization. Application of Fe-modified manure-biochar (MHAPE) treatment to soil resulted in significantly higher NH4+ than any other manure-biochar mixture. This observation suggests that the biochar surface modification allows a relatively high H+ activity than other biochar treatments, capable of stabilizing the NH4+, and reducing N volatilization loss. Compared to other manure-biochar or control treatments, the MHAPE column also released significantly more nitrate and ammonium (p < 0.05) to the leachate. In plant-soil systems, these inorganic N forms are favorably taken up by plants directly (Tisdale et al., 1985). However, high mobility of nitrate, the N loss in this anion form from the soil in leachate, is well known (Syswerda et al., 2012) and ends up in groundwater contamination. The leaching experiment recorded a 0.4% nitrate-N of the total KCl-extractable soil nitrate that ended in the MHAPE water leachate. In comparison, about 3.5% of the KCl-extractable soil nitrate ended up in manure columns water leachate. This observation suggests that Fe modification to biochar not only increased the N-mineralization but simultaneously it positively impacted nitrate or ammonium sorption onto the biochar surface with less leaching loss than manure control.
All the manure and manure-biochar treated columns received 135 kg/ha (120 lb/acre) P2O5-P at the beginning, and only the soil-treated column released significantly high DRP in leachate during the leaching experiment. Sorption of phosphate-P on biochar varies with biochar feedstock and process temperature (Yao et al., 2012), in pure systems and in the absence of competitive ions. The adsorbate's sorption performance depends on the clean adsorbent’s surface contact time, and in addition to that, Phosphate-P sorption diminishes with an increase in competitive ions and humic acids in the solution (Chen et al., 2002). Swine manure is a source of competitive ions and humic acids; thus, the phosphate from swine manure sorption on biochar surface is a complex phenomenon. The dissolved reactive P (DRP) concentration was low (<0.05 mg/kg) for manure and manure-biochar treated column, and biochar-manure columns were not significantly different from manure column. This observation contrasts with the study by Laird et al., 2010a showed a significantly more P (mg/column) in manure-treated soil column leachate than biochar + manure treated soil columns with manure addition. Besides, Laird et al., 2010a added 102 mg total P per kg of soil to the swine manure treated columns, which differs from our application rate of 60 mg/kg of soil. At the end of the leaching experiment, the manure treated columns had a significantly (p < 0.05) higher amount of Mehlich3-P than manure-biochar treated columns. This observation is different from findings reported by Lentz and Ippolito 2012; this two-year field study reported no difference in soil available P between plots receiving treatments of dairy manure and manure and biochar added simultaneously. In the current study, the difference between manure Mehlich-P and manure-biochar suggests that the applied P in manure-biochar could be associated with the mineral phases of biochar or associated with biochar-OM, not evaluated or reported in this study. The lowest P content of Mehlich3-P in Fe-modified biochar among all manure-biochar treatments suggests a P sorption on oxy-hydroxide phases of Fe-biochar (Bakshi et al., 2019, Bakshi et al., 2021) was not extracted with Mehlich3.
In addition to other major and minor plant nutrients, swine manure is a good source of K (Chastain et al., 1999). A significantly high K (p < 0.05) concentration was found in our manure and manure-biochar treated columns compared with control soil columns. All manure-biochar treated columns received significantly more K than manure control through the treatments (Table 1). However, soil K in manure-biochar columns, MHAP and MRO, did not statistically differ from the manure-treated soil column. Treatment MHAPE mixture had a significantly (p < 0.05) high amount of soil K in comparison to all treatments. This result suggests that Fe-modified biochar was capable of stabilizing K better than other biochars used in this study.
The addition of MRO to soil increased the soil Mn availability significantly than manure or other biochar manure treatments. This effect may or may not be related to the significant increase in pH of this manure-wood biochar mixture to soil than other treatments. Lentz and Ippolito, 2012 also reported an increase in the soil Mn availability by adding wood biochar and manure to the soil.
Biochar applications in crop agriculture are often limited by the heavy metal content associated with some types of feedstock and sources (Pulka et al., 2020). Biochar can also be sorbing heavy metals and making them less available to soil exchange sites for the plant to uptake (Zhang et al., 2013). In addition to other nutrients, manure contains several heavy metals. The higher application of manure application to support the recommended macro plant nutrients could also deliver a significantly high concentration of the Cu and Zn like heavy metals, as observed in the study. Copper or Zn toxicity is not an issue in the Midwest United States, but a high concentration of Cu or Zn could be detrimental for soil microbial communities and plants. Bakshi et al. (2014) reported that biochar could immobilize soil Cu and make it less available for the plants to uptake. The MHAPE treatment had a significantly low soil Mehlich3 Cu compared to manure treated column soil, and simultaneously it provided the recommended amount of plant nutrients.
This relatively short control leaching experiment may not be representative of the manure-biochar impact on the soil at the field scale. A more extended soil experiment is essential to carry out to verify the long-term impact of the liquid swine manure-biochar mixture on soil nutrient availability to plants. Also, the slow leachate flow rate of the manure treated control columns made the comparisons challenging for this leaching experiment. Properties of the manure-biochar mixture can vary depending on the manure type, biochar feedstock, biochar production technique, and manure-biochar incubation time, which were not evaluated in this experiment. Microbial biomass was not determined in the study could be one of the limitations of this work; moreover, swine manure from only one representative source and only one soil type was used.
We recommend undertaking socioeconomic analyses for the farm-scale use of biochar treatment to improve the sustainability of the animal and crop production systems. The cost analysis deserves a much broader scope and effort involving analyses for the whole animal and crop production system with consideration of local/regional scales regarding the legal, regulatory, policy, market, labor, soil-water-air quality issues. The cost of biochar treatment will likely decrease if the swine industry adopts biochar as a means to mitigate gaseous emissions from stored manure (e.g., Chen et al., 2020).
Conclusion
The results of this short soil leaching experiment suggest that biochar-manure mixture application to agricultural soils significantly improved soil OM compared to the conventional liquid swine manure treatments to the soil, possibly biochar C have stabilized the manure C. In addition, biochar-manure mixture application improved (numerically, without statistical significance) TC, TN, and BD compared to the conventional liquid swine manure treatments to the soil. Biochar-manure mixtures impacted the availability of macro and micronutrients in soil (depending on the biochar type) differently than conventional manure application, as observed by this column leaching experiment. Additionally, the MHAPE treatment to soil significantly increased Mehlich3 K, KCl-extractable NO3-N, and soil OM content compared with the control soil or manure treated soil. Although the total NO3-N and NH4-N concentrations in leachates of MHAPE columns were significantly higher among all treatments, these values were significantly lower (2 vs. 80 mg/kg) than soil NO3-N and NH4-N concentrations found at the end of the experiment. A long-term field experiment is warranted to examine the biochar-manure mixture's long-term environmental implication on plant-soil biota.
Data Availability Statement
The original contributions presented in the study are included in the article/Supplementary Material, further inquiries can be directed to the corresponding author/s.
Author Contributions
Conceptualization, CB, JK; methodology, CB, MD, ML, and AS; software, CB, DB; validation, CB, JK; formal analysis, CB, DB, and MD; investigation, CB and DB; resources, CB, JK, MD, and BC; data curation, CB and JK; writing—original draft preparation, CB and DB; writing—review and editing, CB, DB, JK, AS, and ML; visualization, CB, DB and MD; supervision, CB and JK; project administration, CB and JK; funding acquisition, JK, CB, AS, and ML All authors have read and agreed to the published version of the manuscript.
Funding
The authors are thankful to the Leopold Center for Sustainable Agriculture for ‘Improving sustainability of iowa agriculture: synergy between improved nutrient recycling, solving livestock odor problems, and crop production’ grant #LCSA-AES-Koziel-2020–2. Partial funding came from iowa State University’s Freshmen Honors Program (Darcy Bonds) and the iowa Agriculture and Home Economics Experiment Station: project number IOW05556 (Future Challenges in Animal Production Systems: Seeking Solutions through Focused Facilitation, sponsored by Hatch Act and State of iowa funds; JK).
Conflict of Interest
The authors declare that the research was conducted in the absence of any commercial or financial relationships that could be construed as a potential conflict of interest.
Acknowledgments
We are thankful to David Laird (Department of Agronomy, ISU) for initial consultations about the experiment and Qinglong Tian (Department of Statistics, ISU) for his help with the statistical model. The authors also thank Wyatt Murphy from the Department of Agricultural and Biosystems Engineering, iowa State University (ABE, ISU) for building the column leachate setup; Peiyang Li and Samuel C. O’Brien (ABE, ISU) for their help with soil sampling, and Zhanibek Meiirkhanuly (ABE, ISU) for the outsourcing of biochar.
Supplementary Material
The Supplementary Material for this article can be found online at: https://www.frontiersin.org/articles/10.3389/fenvs.2021.609621/full#supplementary-material.
References
Angers, D. A., Chantigny, M. H., MacDonald, J. D., Rochette, P., and Côté, D. (2009). Differential retention of carbon, nitrogen and phosphorus in grassland soil profiles with long-term manure application. Nutr. Cycl. Agroecosys. 86, 225–229. doi:10.1007/s10705-009-9286-3
Bakshi, S., He, Z. L., and Harris, W. G. (2014). Biochar amendment affects leaching potential of copper and nutrient release behavior in contaminated sandy soils. J. Environ. Qual. 43, 1894–1902. doi:10.2134/jeq2014.05.0213
Bakshi, S., Laird, D. A., Smith, R. G., and Brown, R. C. (2021). Capture and release of orthophosphate by Fe-modified biochars: mechanisms and environmental applications. ACS Sustain. Chem. Eng. 9 (2), 658. doi:10.1021/acssuschemeng.0c06108
Bakshi, S., Laird, D. A., Smith, R. G., and Brown, R. C. (2019). Phosphate sorption onto modified biochar surface,” in The Proceedings of the Biochar and Bioenergy 2019 Conference, Fort Collins, CO, June 30–July 3, 2019.
Beckwith, C. P., Cooper, J., Smith, K. A., and Shepherd, M. A. (1998). Nitrate leaching loss following application of organic manures to sandy soils in arable cropping. i. effects of application time, manure type, overwinter crop cover and nitrification inhibition. Soil Use Manag. 14, 123–130. doi:10.1111/j.1475-2743.1998.tb00135.x
Chastain, J. P., Camberato, J. J., Albrecht, J. E., and Adam, J. (1999). Swine manure production and nutrient content South Carolina confined animal manure managers certification Program. Clemson, SC: Clemson University, 1–17.
Chen, B., Koziel, J. A., Białowiec, A., Lee, M., Ma, H., Li, P., et al. (2020). The impact of surficial biochar treatment on acute H2S emissions during swine manure agitation before pump-out: proof-of-the-concept. Catalysts 10, 940. doi:10.3390/catal10080940
Chen, J. P., Chua, M. L., and Zhang, B. (2002). Effects of competitive ions, humic acid, and pH on removal of ammonium and phosphorous from the synthetic industrial effluent by ion exchange resins. Waste Manag. 22 (7), 711–719. doi:10.1016/S0956-053X(02)00051-X
Condron, L., Stark, C., O’Callaghan, M., Clinton, P., and Huang, Z. (2010). “The role of microbial communities in the formation and decomposition of soil organic matter,” in Soil microbiology and sustainable crop production. Editors G. Dixon, and E. Tilston (Dordrecht, Netherlands: Springer).
De, M., Riopel, J. A., Cihacek, L. J., Lawrinenko, M., Baldwin-Kordick, R., Hall, S. J., et al. (2019). Soil health recovery after grassland reestablishment on cropland: the effects of time and topographic position. Soil Sci. Soc. Am. J. 84 (2), 568–586. doi:10.1002/saj2.20007
Doane, T. A., and Horwáth, W. R. (2003). Spectrophotometric determination of nitrate with a single reagent. Anal. Lett. 36, 2713–2722. doi:10.1081/al-120024647
D’Angelo, E., Crutchfield, J., and Vandiviere, M. (2001). Rapid, sensitive, microscale determination of phosphate in water and soil. J. Environ. Qual. 30, 2206–2209. doi:10.2134/jeq2001.2206
Fidel, R. B., Laird, D. A., and Spokas, K. A. (2018). Sorption of ammonium and nitrate to biochars is electrostatic and pH-dependent. Sci. Rep. 8, 17627. doi:10.1038/s41598-018-35534-w
Juergens-Gschwind, S. (1989). “Ground water nitrates in other developed countries (Europe)- relationships to land use patterns,” in Nitrogen management and ground water protection. Editor R. F. Follett (Amsterdam, Netherlands: Elsevier), 75–138.
Kalus, K., Koziel, J., and Opaliński, S. (2019). A review of biochar properties and their utilization in crop agriculture and livestock production. Appl. Sci. 9, 3494. doi:10.3390/app9173494
Killorn, R. (1998). Effect of Frequency of swine manure application on the yield of corn and soybean. Swine Research Report 1998 37. Available at: https://www.extension.iastate.edu/Pages/ansci/swinereports/asl-1593.pdfhttps://www.extension.iastate.edu/Pages/ansci/swinereports/asl-1593.pdf (Accessed September 1, 2020).
Laird, D. A, Fleming, P., Wang, B., Horton, R., and Karlen, D. (2010a). Biochar impact on nutrient leaching from a midwestern agricultural soil. Geoderma 158, 436–442. doi:10.1016/j.geoderma.2010.05.012
Laird, D. A., Fleming, P., Davis, D. D., Horton, R., Wang, B., and Karlen, D. L. (2010b). Impact of biochar amendments on the quality of a typical Midwestern agricultural soil. Geoderma 158, 443–449. doi:10.1016/j.geoderma.2010.05.013
Laird, D. A. (2008). The charcoal vision: a win-win-win scenario for simultaneously producing Bioenergy, permanently sequestering carbon, while improving soil and water quality. Agron. J. 100, 178–181. doi:10.2134/agronj2007.0161
Lawrinenko, M., and Laird, D. A. (2015). Anion exchange capacity of biochar. Green Chem. 17, 4628–4636. doi:10.1039/c5gc00828j
Lentz, R. D., and Ippolito, J. A. (2012). Biochar and manure affect calcareous soil and corn silage nutrient concentrations and uptake. J. Environ. Qual. 41, 1033–1043. doi:10.2134/jeq2011.0126
Magdoff, F., and Es, H. V. (2009). “Animal manures for increasing organic matter and supplying nutrients,” in Building soils for better crops sustainable soil management. 3rd Edn (Brentwood, MD: USDA SARE), 129–140.
Mantovi, P., Bonazzi, G., Maestri, E., and Marmiroli, N. (2003). Accumulation of copper and zinc from liquid manure in agricultural soils and crop plants. Plant Soil 250, 249–257. doi:10.1023/a:1022848131043
Maurer, D., Koziel, J. A., and Bruning, K. (2017a). Field scale measurement of greenhouse gas emissions from land applied swine manure. Front. Environ. Sci. Eng. 11 (3), 1. doi:10.1007/s11783-017-0915-9
Maurer, D. L., Koziel, J. A., Kalus, K., Andersen, D., and Opalinski, S. (2017b). Pilot-scale testing of non-activated biochar for swine manure treatment and mitigation of ammonia, hydrogen sulfide, odorous volatile organic compounds (VOCs), and greenhouse gas emissions. Sustainability 9 (6), 929. doi:10.3390/su9060929
McLean, E. O. (1982). “Soil pH and lime requirement,” in Methods of soil analysis, part 2. 2nd Edn. Editor A. L. Page (Madison, WI: Agronomy Monographs), 9, 199–223.
Mehlich, A. (1984). Mehlich 3 soil test extractant: a modification of Mehlich 2 extractant. Commun. Soil Sci. Plant Anal. 15, 14091416. doi:10.1080/00103628409367568
Meiirkhanuly, Z.et al. (2019). Evaluation of biochar for mitigation of ammonia, hydrogen sulfide, odorous volatile organic compounds, and greenhouse gases emissions from swine manure. Publisher ProQuest Dissertation publishing. ISBN: 9781088368053.
Meiirkhanuly, Z., Koziel, J. A., Chen, B., Białowiec, A., Lee, M., and Wi, J.et al. (2020a). Mitigation of gaseous emissions from swine manure with the surficial application of biochars. Atmosphere 11, 1179. doi:10.3390/atmos11111179
Meiirkhanuly, Z., Koziel, J. A., Bialowiec, A., Banik, C., and Brown, R. C. (2020b). The proof of concept of biochar floating cover influence on swine manure pH, Implications of gaseous emission from area sources. Front. Chem. 8, 656. doi:10.3389/fchem.2020.00656
Nelson, D. W., and Sommers, L. E. (1996). “Total carbon, organic carbon, and organic matter,” in Methods of soil analysis: Part 3 Chemical methods. 3rd Edn, Editors D. L. Sparks, A. L. Page, P. A. Helmke, R. H. Loeppert, P. N. Soltanpour, M. A. Tabatabaiet al. (Madison, WI: SSSA Special Publications), 961–1010.
Polin, J. P., Carr, H. D., Whitmer, L. E., Smith, R. G., and Brown, R. C. (2019). Conventional and autothermal pyrolysis of corn stover: overcoming the processing challenges of high-ash agricultural residues. J. Anal. Appl. Pyroly. 143, 104679. doi:10.1016/j.jaap.2019.104679
Pulka, J., Manczarski, P., Stępień, P., Styczyńska, M., Koziel, J. A., and Białowiec, A. (2020). Waste-to-carbon: is the torrefied sewage sludge with high ash content a better fuel or fertilizer? Materials 13 (4), 954. doi:10.3390/ma13040954
Reid, K., Schneider, K., and McConkey, B. (2018). Components of phosphorous loss from agricultural landscapes, and how to incorporate them into risk assessment tools. Front. Earth Sci. 6, 135. doi:10.3389/feart.2018.00135
Rogovska, N., Laird, D., Cruse, R., Fleming, P., Parkin, T., and Meek, D. (2011). Impact of biochar on manure carbon stabilization and greenhouse gas emissions. Soil Sci. Soc. Am. J. 75, 871–879. doi:10.2136/sssaj2010.0270
Rogovska, N., Laird, D. A., Rathke, S. J., and Karlen, D. L. (2014). Biochar impact on midwestern mollisols and maize nutrient availability. Geoderma 230-231, 340–347. doi:10.1016/j.geoderma.2014.04.009
Rollag, S. A., Lindstrom, J. K., and Brown, R. C. (2020). Pretreatments for the continuous production of pyrolytic sugar from lignocellulosic biomass. Chem. Eng. J. 385, 123889. doi:10.1016/j.cej.2019.123889
Rover, M., Smith, R., and Brown, R. C. (2018). Enabling biomass combustion and co-firing through the use of lignocol. Fuel 211, 312–317. doi:10.1016/j.fuel.2017.09.076
Sawyer, J. E., and Mallarino, A. (2016). Using manure nutrients for crop production. Extension and Outreach. Ames, IA: Iowa State University.
Sawyer, J. E. (2001). “Nitrogen fertilizer and swine manure application to soybean,” in Proceedings of the 13th Annual Integrated Crop Management Conference, Ames, IA, December 6, 2001.
Schulte, E. E., and Hopkins, B. G. (1996). “Estimation of soil organic matter by weight loss-on ignition,” in Soil organic matter: analysis and interpretation. Editors F. R. Magdoff, M. A. Tabatabai, and E. A. Hanlon (Madison, WI: SSSA Special Publications), 21–32.
Tisdale, S. L., Nelson, W. L., and Beaton, J. D. (1985). Soil fertility and fertilizers. 4th Edn. New York, NY: Macmillan.
Wilfert, P., Kumar, P. S., Korving, L., Witkamp, G.-J., and van Loosdrecht, M. C. M. (2015). The relevance of phosphorus and iron chemistry to the recovery of phosphorus from wastewater: a review. Environ. Sci. Technol. 49, 9400–9414. doi:10.1021/acs.est.5b00150
Yao, Y., Gao, B., Zhang, M., Inyang, M., and Zimmerman, A. R. (2012). Effect of biochar amendment on sorption and leaching of nitrate, ammonium, and phosphate in a sandy soil. Chemosphere 89, 1467–1471. doi:10.1016/j.chemosphere.2012.06.002
Keywords: C-mineralization, carbon sequestration, nutrient cycling, sustainability, waste management, animal agriculture, N-immobilization, N-mineralization
Citation: Banik C, Koziel JA, De M, Bonds D, Chen B, Singh A and Licht MA (2021) Biochar-Swine Manure Impact on Soil Nutrients and Carbon Under Controlled Leaching Experiment Using a Midwestern Mollisols. Front. Environ. Sci. 9:609621. doi: 10.3389/fenvs.2021.609621
Received: 23 September 2020; Accepted: 18 February 2021;
Published: 15 April 2021.
Edited by:
Muhammad Shaaban, Bahauddin Zakariya University, PakistanReviewed by:
Ronghua Li, South China Agricultural University, ChinaEduardo Guimarães Couto, Federal University of Mato Grosso, Brazil
Copyright © 2021 Banik, Koziel, De, Bonds, Chen, Singh and Licht. This is an open-access article distributed under the terms of the Creative Commons Attribution License (CC BY). The use, distribution or reproduction in other forums is permitted, provided the original author(s) and the copyright owner(s) are credited and that the original publication in this journal is cited, in accordance with accepted academic practice. No use, distribution or reproduction is permitted which does not comply with these terms.
*Correspondence: Jacek A. Koziel, koziel@iastate.edu