- 1Department of Biosciences, Solomon Mahlangu College of Science and Education, Sokoine University of Agriculture, Morogoro, Tanzania
- 2Faculty of Life Sciences, Hamburg University of Applied Sciences, Hamburg, Germany
- 3Faculty of Sustainability Science, Institute of Ecology, Leuphana University Lüneburg, Lüneburg, Germany
This study was conducted in the Kilombero Valley Ramsar Site (KVRS), the largest low-altitude freshwater wetland in East Africa to: 1) determine pesticide contamination of soil, water, and sediment at different seasonal weather conditions 2) critically analyze the seasonal and spatial ecological impacts of pesticide contaminations under tropical weather conditions 3) estimate the potential environmental risks from pesticide exposure based on analytical data. Surface sediments, soil and water samples were collected from sugarcane, teak and rice plantations of the KVRS during the dry (January 2012) and the rainy (March–April 2013) seasons. Samples were screened for 97 different types of pesticide residues using and LC–MS and HPLC–MS. Physical-chemical properties of the sediment and soil samples and meteorological data were analyzed. 92% of soil and sediment samples had relatively low OM content (<5%) while 60% of samples had high clay content, indicating high cation exchange capacity that can bind pesticides to the soils. Field soil and air temperature ranging 28–35°C, total sunshine hours-208 h/month, photo radiation −764 MJ/m2, average precipitation 300 mm/month, wind speed >60 km/h, humidity >80% were recorded. Frequency of detection and concentration of pesticides residues depended on seasons. For the dry season sampling, glyphosate/AMPA (0.01–0.55 µg/g), and diuron (0.026–1.7 µg/g) were mostly detected in the sediment and soils. Monuron (1.28–3.9 µg/L) and propoxur (0.05–0.6 µg/L) were mostly detected in the rainy seasons (water and sediments). Glyphosate was above limit of detection in the dry season water samples indicating that its emission into the water system may have been directly through application. Maximum diuron concentrations in water were >30 times higher than the estimated predicted no-effect concentration (PNEC) value (0.054 µg/L) indicating a risk to aquatic community. Calculated pore water concentrations (0.992–0.081 µg/L), exceeded the estimated PNEC values during the dry season, indicating a risk to benthic organisms. Detection of pesticide is influenced by application, environmental factors and the timing of sampling. Further research is recommended to increase our knowledge on soil-water-sediment interactions in the tropics with regard to pesticides.
Introduction
Worldwide population growth and the impacts of climate change have increased global demands for natural resources and food, enhancing pressure on the environment due to the urgent need to cater for food insecurity (Godfray et al., 2010). Over the past 2–3 decades, agricultural practices in tropical areas have been intensified to fight food insecurity caused by changes in weather patterns (Lewis et al., 2016; Daam et al., 2019). Under the changing climate, farming activities in tropical areas are challenged largely by crop losses from pests and diseases (Poppy et al., 2014) and from soil nutritional deficiencies (Soares et al., 2019). Climate change will have an impact on weather conditions in tropical areas probably leading to agricultural shifts in types of crops grown, seasons and location of farming (Müller et al., 2011), magnitude and range of crop pests and the intensity of pesticides used to control the emerging pests (War et al., 2016). In areas of intensive pesticides use, seasonal changes of weather conditions will likely increase the risk of diffuse losses of pesticides from the field to other non-target areas (Koli et al., 2019). Besides, the ecological risks increase with pesticide applications, shifts in agricultural activities, and land-use changes due to climate change adaptation strategies (Wee and Aris, 2017). Volatilization and adsorption rates of pesticides from their target site are influenced by weather conditions (temperature, relative humidity, air/wind movement, solar intensity) and soil conditions (texture, moisture, organic matter content, and pH) (Bedos et al., 2002; Arias-Estévez et al., 2008).
Various studies have predicted that climate change will lead to increased pesticide use, thus intensifying negative toxicological impacts on the environment (Constantini, 2015; Daam et al., 2019; Koli et al., 2019). Fate and behaviour of toxic chemicals in the environment are affected differently by various aspects of climate change. Here, some ecosystems such as natural wetlands are expected to be more sensitive to those stressors than terrestrial ecosystems (Zhang et al., 2012).
This study was conducted in the Kilombero Valley Ramsar Site (KVRS) in Tanzania. This is a floodplain area, which was designated as a Ramsar site in 2002 (Ramsar, 2002). The floodplain is about 220 km long and 70 km wide in the Rufiji River catchment in southern Tanzania, with multiple river tributaries converging in the valley (Ramsar, 2002). The KVRS offers critical connection routes for wildlife populations moving between the Udzungwa range and the Selous Game Reserve. The valley also hosts a large number of the near-threatened puku antelope and various endemic bird species (Ramsar, 2002). Land-use changes due to rice growing, livestock grazing, human settlements, deforestation and development of infrastructure have drastically reduced the natural wetland habitat of the valley since the past 3 decades (Muro et al., 2017). The extent of pesticide use in Tanzania has rapidly increased over the last years. For instance, between 2007 and 2015, the number of registered pesticides tripled and reached a total of 1,390 in 2015 (Lahr et al., 2016). While the total national area that harvested 27 major crops increased between 2005 and 2013 by a factor of 1.5, pesticide imports quadrupled, without significant effect on crop yield (Lahr et al., 2016).
In many tropical countries, farming in wetland catchment areas is very common, and thus pesticides are detected in water, sediment and biota of agricultural areas and surrounding waterways (Wandiga, 2001; Osano et al., 2003). The Kilombero floodplains, is, one of the East Africa’s largest wetlands threatened by increased agro-pastoral activities and mechanized agriculture associated with intensive use of pesticides (Materu, 2015). Malpractices in pesticide application add to this burden, such as use of unregistered pesticides, inappropriate dosage (use of banned pesticides, lack of adherence to pre-harvest interval, use of a mixture of pesticides in a single spray, and inappropriate pesticide/crop combinations which are commonly reported (Lekei et al., 2014; Ngowi et al., 2016; Zikankuba et al., 2019). Pesticides which are improperly applied likely cause downstream river basin contamination and may destruct biodiversity hotspots and habitat for endemic species of flora and fauna. Published data and studies to elucidate the extent of pesticide pressure on the aquatic system of the Kilombero Ramsar site are limited. According to Lahr et al. (2016), no large-scale monitoring program for pesticide residues in soil and water is in place. This study aims to gain insight into the diversity and extent of pesticide distribution in the Kilombero valley and to assess whether there may exist or arise an environmental risk in the light of increased pesticide use under a changing climatic conditions.
Materials and Methods
Description of Study Area
This study was conducted in the Kilombero Valley Ramsar Site (KVRS), the largest low-altitude freshwater wetland in East Africa (MNRT, 2004). The Ramsar site (assigned since 2002) central coordinates points are 8°40′S and 36°10′E. It covers an area of 796,735 ha with a wetland catchment area of 40,000 km2 (Ramsar, 2002). The KVRS is situated in the conserved eastern arc mountains of Tanzania, between the Udzungwa catchment mountains reserve in the north-west and the Mahenge escarpment in the southern east (Ramsar, 2002). The Ramsar site has numerous temporary and permanent rivers (Figure 1), which serve as a source of freshwater and livelihood activities for surrounding communities. The KVRS is sub-humid with a mean annual temperature of 26°C and a mean annual total rainfall of 1,600 mm (Bakengesa et al., 2011). It has a bimodal rainfall pattern with short rains (light and sporadic) from December to February and long rains (heavy and regular downpour) from March to June (Bakengesa et al., 2011). Flooding peaks between March and April conveying important nutrients to the valley leading to highly inundated fertile and productive soils (SAGCOT, 2011). According to the Ramsar Advisory Mission Report of 2017, the KVRS is under intense pressure and subject to unsustainable levels of negative human-induced change which is compromising the ecological character of the site. There are massive land use changes especially for agriculture and livestock keeping, thus encroaching in all areas of wetlands and the native grass lands have been changed to arable lands (RAM, 2017). More than 60% of the area formerly covered by wetlands and grasslands in the Kilombero floodplain has been converted to arable land for monoculture cropping (rice, sugarcane or maize) (Muro et al., 2017). According to Tanzanian National Bureau of Statistics (NBS) report of 2016, the population in the three districts, where KVRS is located, is projected to grow by approximately 3% per annum over the next 20 years thus turning to about 1,548,215 people in 2036 (NBS, 2016). This population depends on these wetland catchment areas for their livelihoods activities mainly crop agriculture, livestock grazing and fishing.
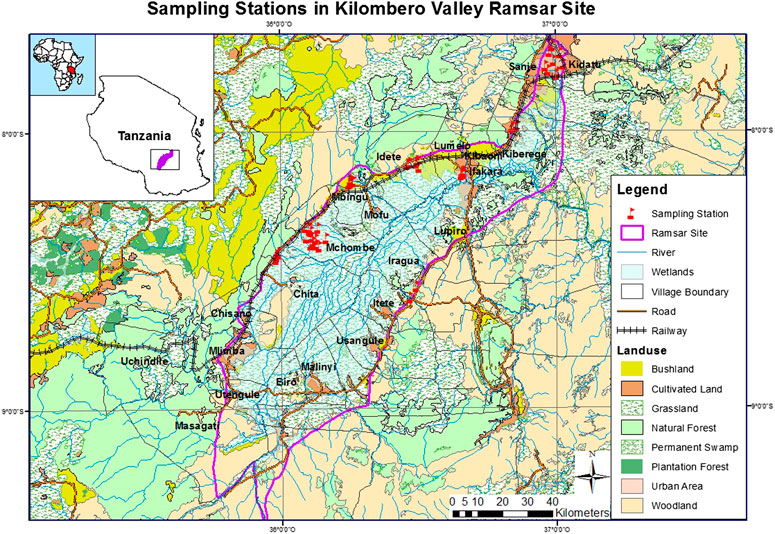
FIGURE 1. A map of Tanzania showing location of the Kilombero Ramsar Site and sampling stations (Materu and Heise, 2019) (adapted from Materu 2015).
In this study, all eight plantations were surveyed prior to sampling campaign. Farming activities and pesticide spraying (in some farms) were observed. Interviews were conducted to 21 key informants (eight plantation managers, five agronomists and eight farm managers) on the extent and type of pesticide used in the area. Only registered pesticides are allowed to be used in Tanzania (TPRI, 2020). The registration is renewed every two years. Supplementary Table S1 provides an overview of registered pesticides to be used in rice and sugarcane farming in Tanzania.
According to interviews with sugar plantation agronomists during field surveys, the following pesticide formulations were mostly used in sugarcane farms;- herbicides both before/at planting of the cane and during the ratoon stage (pendimethaline, metribuzine, chlorimuron, paraquat, MSMA, MCPA, diuron, hexazinone, atrazine, ametryn, glyphosate, acetochlor, triclopyr, halosulfuronmethyl), fungicide (triadimefon, triadimenol, propiconazole). No any insecticides were mentioned.
The farm agronomists and plantation managers in rice plantations mentioned the following pesticide formulations as most frequently used in the rice plantations under various trade names; herbicides (2,4-D, glyphosate, oxadiazon, glufosinate-ammonium, halosulfuron-methyl, pyribenzoxin, propanil, triclopyr, fluazifop-P-butyl) insecticides (chloropyrifos and imidacloprid, paraquat (4,4′-bipyridine, esfenvalerate, fenitrothion, poly-1-p-menthene), pesticides (Thiamethoxam, chloranitraniliprole, diphacinone), fungicide (Thiophanate-methyl, tricyclazole).
For teak plantations herbicides (glyphosate, triclopyr), insecticide (sprayfilm 10- terpenic polymer) and fungicides (copper Oxychloride, and copper II sulphate) were listed as the most used chemicals.
Samples Collection, Preservation, Handling and Storage
Surface sediment, soil and water samples were collected during the rainy (March–April 2012) and dry seasons (January–February 2013) in eight sugarcane, rice and teak plantations of the KVRS (Table 1). A total number of 143 samples (58—fine surface sediment, 17—soil and 68—water) were collected from up/downstream rivers that drains through the agricultural farms, and from inlet and outlet canals that drain into or out of the eight surveyed sugarcane, rice and teak plantations.
Fine sediments samples were collected directly from either the surface banks or beneath a shallow sluggish aqueous layer using a stainless steel spoon. Water samples were collected by using previously acid washed plastic bottles either adjacent or at the same sites where the sediments were collected. During dry season, soil samples were randomly collected from newly ploughed or planted rice and sugarcane agricultural fields while avoiding areas of variability within the same field. Soil samples could not be collected during the rainy season because the sampling areas were completely flooded. All sampling bottles were pre-cleaned with tap water and laboratory detergent, soaked overnight in an acid bath (3M HCl), rinsed thoroughly 3 times with deionized water, air dried and packed in a big black polyethylene (PE) plastic to avoid contamination. GPS coordinates of each sample and all relevant sample information were recorded. Following collection, sediments and soil samples were transferred to the previously 95% ethanol washed dry polyethylene (PE) 50 ml size bottles with narrow neck. All sampling bottles were transferred immediately to the black PE bag to avoid contamination. All samples were preserved at the sampling site in an ice chest containing several cold packs before refrigerated at 4°C on the last day of sampling followed by one day holding time before transported to Germany by using ice chest boxes. At the laboratory, the water samples were filtered through a 0.45-mm nylon membrane (Millipore, MA, United States) to separate the water from the suspended particulate matter. Laboratory experiments were conducted in laboratory of Applied Aquatic Toxicology, Hamburg University of Applied Sciences and analytical laboratory of the Faculty of Sustainability, Leuphana University in Germany. Samples were deep frozen until analytical assessments were conducted.
Meteorological Information
Temperature, rainfall pattern, solar radiation and intensity, soil temperature, relative humidity and wind speed data were retrieved from daily meteorological records of two sampling plantations namely; KPL (Kilombero Rice plantations, Mngeta farms) and KSC (Kilombero sugarcane plantations, Kidatu K1-estate farms). The rest of the sampling plantations had no meteorological units. Nevertheless, KPL data (solar radiation, humidity, temperature and rainfall) could represent Chita Ns and Mbingu sisters’ farms, while KSC data (rainfall, humidity, temperature and wind speed) could represent KP (Kiberege agricultural prison farms). Meteorological data from these two plantations might not be a good representative of TAC, Teak Co, and IP, because they are located at different terrain and have different relief features from other stations. Therefore, no weather data were considered for TAC, Teak Co, and IP 3 stations.
Analysis of Physico-Chemical Properties of Soil and Sediment Samples
Dry matter content (dry weight/DW) was determined by differential weighing the aliquot of soil and sediment samples before and after drying at 105°C for 24 h. Organic Carbon (Corg) and nitrogen (Norg) for soil and sediment samples were determined using a CHN Perkin Elmer 2,400 Elemental Analyzer. All tools were cleaned with water and acetone after replica of each sample or blank or standard to avoid cross contamination. The standards were kept in airtight, dark bottles to minimize exposure to light and air. The machine was set to equilibrate for about 2.5 h to reach the combustion and reduction temperature of 980°C and 640°C respectively before calibrating with blanks and taking sample measurements. The total organic matter of the samples was calculated by multiplying the percentage of organic matter content (% Corg) with the empirical factor of 1.72, as carbon stoichiometrically contributes 50% of the organic matter (Schumacher (2002). pH values of soil and sediments were determined using 0.01M calcium chloride solutions according to standard operating procedures describe by Baxter (2018).
Electrical conductivity (EC) of unfiltered supernatant of an aliquot of the soil samples was measured using potassium chloride (KCL)—calibrated EC-meter into mill-Siemens per centimeter (mS/cm). The % salinity was calculated in reference to the EC of the KCL-calibration solution (0.528* EC [mS/cm] at 25°C) as described by FAO (1999). Grain size analysis was performed by GBA (Gesellschaft für Bioanalytik mbH) which employ the procedural and methods described by German National Standard (DIN 18123, 2011).
Pesticide Analysis
All water samples were filtered through a 0.45-mm nylon membrane (Millipore, MA, United States) to separate the water from the suspended particulate matter before pesticide analysis. Soil and sediment samples were dried at constant temperature in an oven at 30°C, and then were milled and sieved using a 2-mm mesh, for pesticide analysis. Between sediments and soil samples, the mills and sieves were cleaned to prevent any cross contamination between samples (Okada et al., 2018). Screening of pesticides from sediment, soil and water samples was conducted by the commercial laboratory GBA (Gesellschaft für Bioanalytic mbH)-Hamburg, which is accredited to be competent under the terms of DIN EN ISO/IEC 17025:2018 to carry out chemical analysis of water, sediment and soil following the respective DIN-ISO standards, and QS (quality and security) certified for pesticide analysis by the German agro-economy. Analysis of glyphosate and amino-methyl-phosphonic acid (AMPA) was carried out according to ISO 21458 (2008) by liquid chromatography mass spectrometry (LC-MS) following liquid-extraction.
All other pesticides were determined according to DIN 38407-35 (2010)-German standard methods for the examination of water, waste water and sludge-(jointly determinable substances group, F) using high performance liquid chromatography mass spectrometry (HPLC-MS). Two replicates of each sample eluates were used and isotopically labeled mineralized water (DIN-ISO 16308, 2017) was used as a blank control solution which was treated and analyzed in the same manner as the real samples. The blank values were 1/3 lower than the limits of quantification (LOQs). Pesticides were analyzed reaching different detection limits according to type of matrix and type of pesticide intended for detection as shown in the Supplementary Table S2. A total of 97 pesticides (67 herbicides, 5 herbicides degradates, 15-insecticide and 10 fungicides), which are listed in Table S2 Supplementary data, were analyzed. The concentration of each pesticide in the soils and sediments was expressed as µg/g whereas that in surface water was expressed as µg/L. In sediment and soil samples, the limit of detection (LoD) for each of the 97 analytes was 0.01 μg/g dry weights (dw). In water, LoDs for most pesticides were 0.05 µg/L. Chlorpyrifos had the second lowest LoD with 0.02 μg/L, the lowest being the one for glyphosate and AMPA with 0.01 µg/L. However, the use of a certain type of pesticides in the study plantations varied greatly, as was influenced by the type of weeds that have infested the field plots, inherent history of the effectiveness of the specific pesticide to be used at a time, and the season of the year (rainy or dry seasons). Therefore, for the purpose of this study, it was difficult to establish the amount of each type of pesticide used per year in a specific plantation because of the variability of use and targets being only on the areas pest infestations.
Statistical Analysis and Pesticides Environmental Risk Calculation
All statistical analysis and graphs were prepared with Graph Pad Prism5 statistical package. One-way analysis of variance (ANOVA) was used to compare grain size and nutrient ratio variation among the samples and seasonal variation of weather parameters among stations. With regard to the pesticides, the low number of detections in the matrices could not lead to any useful correlations or allow a sensible statistical analysis.
For each detected compound, data on ecotoxicity and environmental properties were derived from the Pesticide Properties Database (PPDB). Following the procedure recommended in the Technical Guidance Document on environmental risk assessment, assessment factors (AF) were determined corresponding to the extent of eco-toxicological information. Predicted no effect concentrations were derived, dividing the lowest endpoint by the AF (European Chemicals Bureau, 2003).
Pore-water concentrations of non-ionic pesticides were calculated from total sediment concentrations on the basis of the equilibrium partition coefficient (DiToro et al., 1991), dividing total sediment concentrations by the product of mass fraction of organic carbon (foc) and the organic carbon/water partition coefficient (Koc). A foc of 2%, the median organic content of all sediment samples, was used for all calculations. Variation of the OM of sediment and soil samples collected from the same plantation ranged from 1.1 to 3.4, while the variation of OM among samples across plantations was as high as 14.31, indicating diverse range of physical chemical properties of the sampling stations.
Results
Physico-Chemical Analysis of Soil and Sediment Samples
The results of the physico-chemical characterization of the soils and sediments are shown in Table 2. The dry weight of the sediment samples ranges between 22.1 and 66.3%. Soil samples have dry weights between 53.5% and 81.4%, pointing to a generally higher water content of the sediment samples. Grain size of the sediment samples within a single plantation were in the same magnitude as soil samples. However, there were significant differences between the grain sizes amongst the samples (p < 0.05, 1-way ANOVA, post-hoc, Dunn’s Multiple comparison test) (Figure 2). The finest particle size fraction (<20 µm) was predominant in all samples. However, 61% and 69% of sediments (n = 58) and soil (n = 17) samples, respectively, had a silt-clay texture (<0.1 mm), while 39% and 31% of sediment and soil samples, respectively, were sandy-clay (0.10–2 mm). Of all samples (58-sediments and 17-soils) that were measured, only one sample, from Idete prison farms (IP), showed a neutral pH, while the rest of soil and sediment samples from all sampling stations were either slightly or acidic in nature (Table 2). The pH of the sediment samples varied between 5.1 (lowest; sample KSC3) and 7.1 (highest; sample IP1), whereas the pH of soil samples ranged from 4.8 (lowest; samples KP1, MBS8) to 5.8 (highest; samples KP2, MBS9), representing higher acidity of the top soil samples. The electrical conductivity (EC) of both the soil and the sediment samples was generally very low resulting in very low soluble salt contents of 0.02–0.03%.
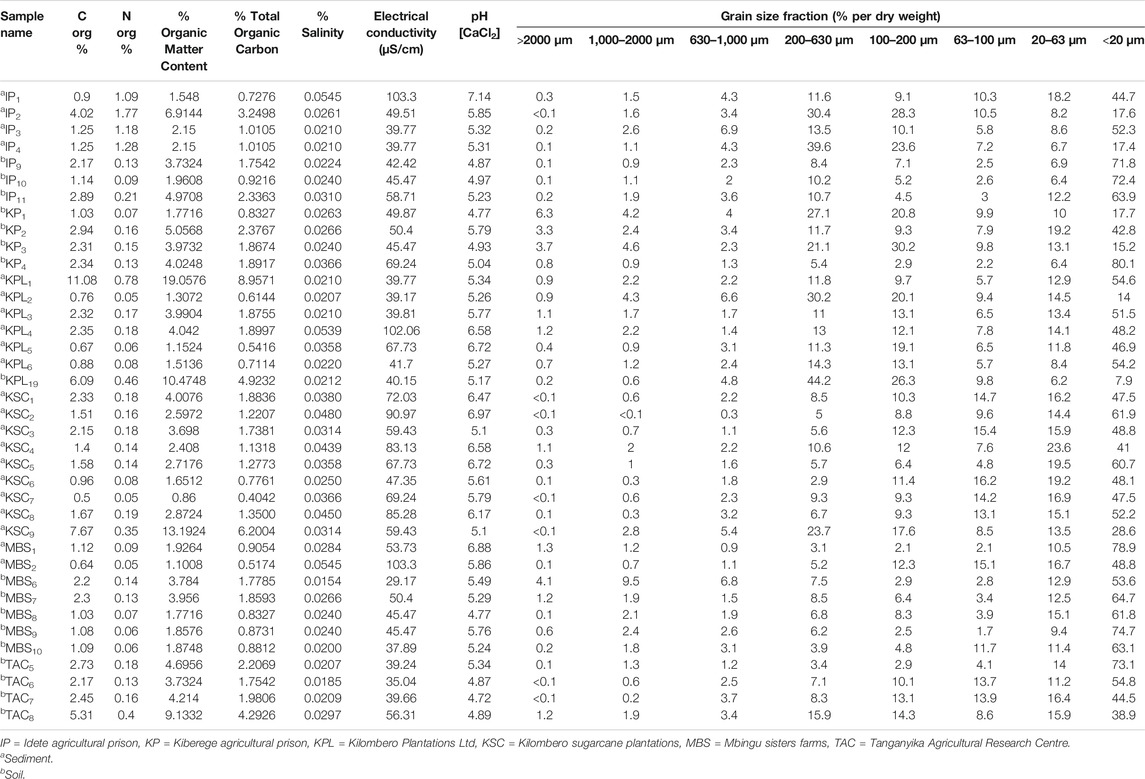
TABLE 2. A range of specific physical chemical parameters of sediment and soil samples from various sampling station of the study plantations.
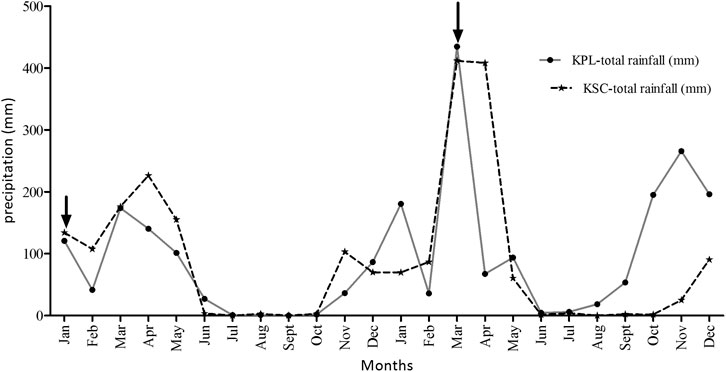
FIGURE 2. Monthly total rainfall pattern as recorded by KPL (Kilombero Rice plantations)-Mngeta and KSC-(Kilombero sugarcane plantation) (K1-estate) Kidatu weather stations for the period of 24 months from 2012 to 2013. Arrows indicate conditions during sampling seasons.
Sediment and soil total organic carbon (TOC) and organic matter (OM) of the samples collected from the same plantations were significantly correlated (r2 = > 0.9, p < 0.0001, Table 2). Soil samples had relatively lower total percentage of Corg and thus lower percentage of OM and TOC than sediment samples (Table 2). Only one sediment sample had a highest percentage OM and TOC of 19.07 and 8.96% respectively. The overall OM of the soil and sediment samples ranged from 1.78 to 10.48 and 0.86–19.07, respectively. Three sediment samples had >5% OM content (KPL1 = 19.0576, IP2 = 6.9144 and KSC9 = 13.1924), while for soils, three samples from different plantations had >5% OM (KP2 = 5.0568, TAC8 = 9.13 and KPL19 = 10.4748). The rest of the sediment and soil samples had a lower OM content (<5%). Table 3 shows the overview range values of each physical chemical parameter of soil and sediment samples measured in the field and laboratory.

TABLE 3. Summary of the range values of physical-chemical parameters measured in the field and in the laboratory for 58-sediment, 17-soil and 68-water samples.
Pesticide Screening Results
Frequency of Detection
From 97 pesticides that were screened for in the sediment, soil and water samples (Supplementary Table S2), and only 11 pesticides could be detected in any of the samples: chlorpyrifos, glyphosate, AMPA, atrazine, 2-hydroxyatrazin, hexazinone, metribuzin, diuron, desmethyl-diuron, monuron, and propoxur. For those pesticides above LoD, the frequency of detection (DF), calculated over all samples, was low and ranged from 1.4% (hexazinone) to 15.7% (glyphosate, diuron) (Table 4).
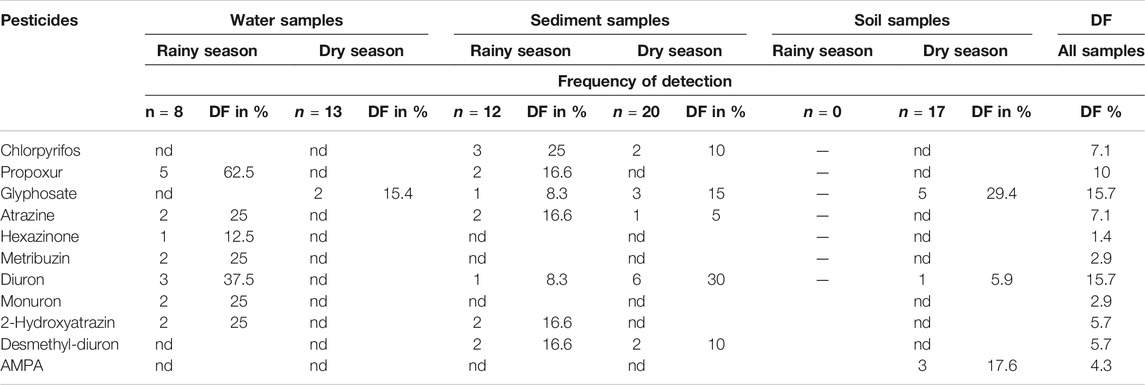
TABLE 4. Seasonal frequency of detection (DF) of pesticides in absolute numbers and in percent of samples per matrix and in all samples (nd: below detection limit). Shades: grey = insecticides, green = herbicides, yellow = metabolites.
Pesticides were more often detected in water during the rainy season (7 pesticides, DF 12.5–62.5% of all samples) compared to the dry season (only glyphosate with a DF of 15.4%). In sediments, pesticides were present both in the rainy and in the dry season with the DF of seven substances ranging from 8.3 to 25%, and of five substances ranging between 5 and 30%, respectively. Soil samples had only been retrieved in the dry season and here, three substances were found in 5.9–29.4% of samples.
Of the detected pesticides, only chlorpyrifos and propoxur are applied as insecticides, while the others are herbicides and their derivatives. Substances with the highest incidence in water were propoxur (DF: 62.5%), followed by diuron (DF: 37.5%), both in the rainy season, while in the dry season, only glyphosate was found (15.4%). In sediment, chlorpyrifos was detected with highest frequency of 25% in the rainy season and diuron in the dry season in 30% of the tested samples. In soil glyphosate was present with a DF of 29.4%.
Environmental Concentrations
Table 5 indicates that maximal concentrations of the seven pesticides that were detected in sediment ranged from 0.064 (propoxur) to 1.83 µg/g (chlorpyrifos) in the rainy season and from 0.092 (chlorpyrifos) to 1.1 µg/g in the dry season (diuron). Maximum concentrations in soil were in the same order of magnitude as in sediment and only quantifiable for three substances. With 0.55 µg/g the glyphosate concentration was higher in soil than in sediment (0.19 µg/g). AMPA, its metabolite, was only detected in soil and reached similar concentrations (0.49 µg/g). Diuron was present but with 0.052 µg/g at a low level. In water samples, diuron and monuron were detected at relatively high concentrations with maximum values of 1.7 and 3.9 μg/L, respectively. The other pesticides reached concentrations between 0.083 µg/L (propoxur), and 0.52 µg/L (atrazine). The specific range and mean concentrations of detected pesticides in sample matrices collected during the dry and rainy seasons from various plantations are summarized in Supplementary Table S3, where it indicates that more variety of pesticides were detected in the Kilombero sugarcane farms than in any other plantations.
Table 5 also presents predicted no-effect concentrations (PNECs) that were derived from the shown ecotoxicological data. Dividing, the measured environmental concentrations (MEC) by the PNEC data results in a risk quotient which points to a potential risk when it is equal or above 1. Where sediments are concerned, pore water concentrations can be calculated for non-ionic substances to compare them with PNEC data. Based on pore water concentrations, the risk quotients were slightly above 1 for chlorpyrifos in the rainy season (RQ: 0.017/0.014 = 1.2) and for diuron in the dry season (RQ: 0.081/0.054 = 1.5). The diuron concentrations that had been measured in the water samples during the rainy season, however, exceed the calculated PNEC values by more than 30 times and therewith present a substantial risk (RQ: 1.7/0.054 = 31.5).

TABLE 5. Detected pesticides in the environmental sample matrices: Concentrations in water, soil and sediments were measured in the laboratory, pore water concentrations for non-ionic compounds are calculated (PWcalc).
Meteorological Information
There is no significant differences in both minimum and maximum average monthly temperatures as recorded by the two plantations (KPL and KSC) for two consecutive years (p > 0.05). However, Figure 3 indicates that there is extremely significant differences between the recorded daily or monthly average maximum and minimum temperatures (p < 0.001). Minimum and maximum temperatures of 12.1°C and 37.2°C were recorded in July (end of the rainy seasons) and December (dry seasons), respectively.
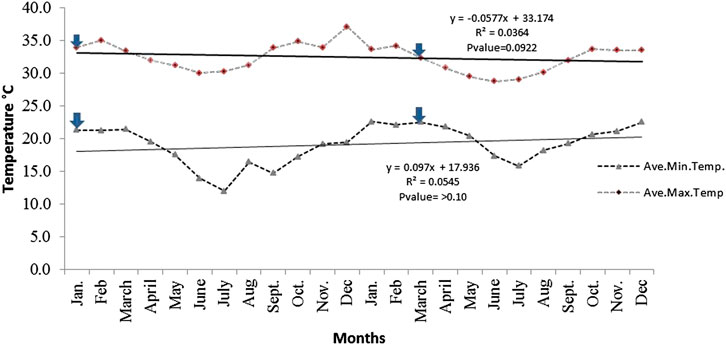
FIGURE 3. Average maximum and minimum monthly field temperatures recorded by KPL (Kilombero Rice plantations)-Mngeta and KSC-(Kilombero sugarcane plantation) (K1-estate) Kidatu weather stations for the period of 24 months in year 2012/2013. Arrows indicate conditions during sampling seasons.
Figure 2 shows that there is very significant differences in total monthly precipitation as recorded in the two plantations with different geographical features (p = 0.0024). Total rainfall fluctuates in different months, where some months such as September show no rainfall at all. Maximum rainfall of >70 mm/month and >400 mm/month were recorded in both plantations during March in 2012 and 2013, respectively (Figure 2).
Daily lowest and highest soil and air temperature (measured at a depth of 10 cm below the soil surface) in the field ranged from 12 to 37°C per day depending on the season. There is a significant differences in the mean soil temperatures per month (p = 0.01). However, mean monthly soil temperature was 27.7°C while minimum and maximum records were 25°C and 30°C (Figure 4). Recorded monthly average sunshine hours were 210.1 h/month while minimum and maximum total sunshine hour were 132.9 and 261.1 h/month, respectively (Figure 4). Annual mean atmospheric radiation was 628.6 MJ/m2 while the minimum and maximum monthly atmospheric radiation of 425.8 and 764 MJ/m2 were recorded in September 2013 and December 2012, respectively (Figure 4). Monthly mean wind speed for station A (KPL-Mngeta farms) varied from 9.25 km/hr in Nov 2013 to 39.0 km/hr in Feb 2012 while wind gust varied from 29.0 km/day in June 2012 to 66.0 km/day in Feb. 2012 (Figure 5A). Minimum and maximum monthly mean humidity was 40% and 95%, respectively (Figure 5A). Station B (KSC-Kidatu farms) recorded minimum monthly mean humidity of >40% and a monthly maximum mean humidity of >80%, mean wind speed of 2.98 km/hr and mean wind gust of 71.9 km/day (Figure 5B).
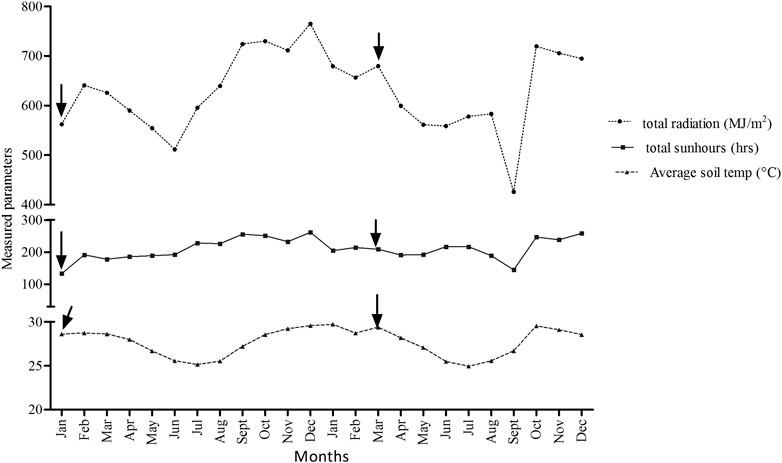
FIGURE 4. Average monthly record of photo radiation intensity, sun hours and soil temperature parameters as recorded by KSC (Kilombero sugarcane station) (K1-estate) Kidatu weather stations for the 24 months. Arrows indicate conditions during sampling seasons.
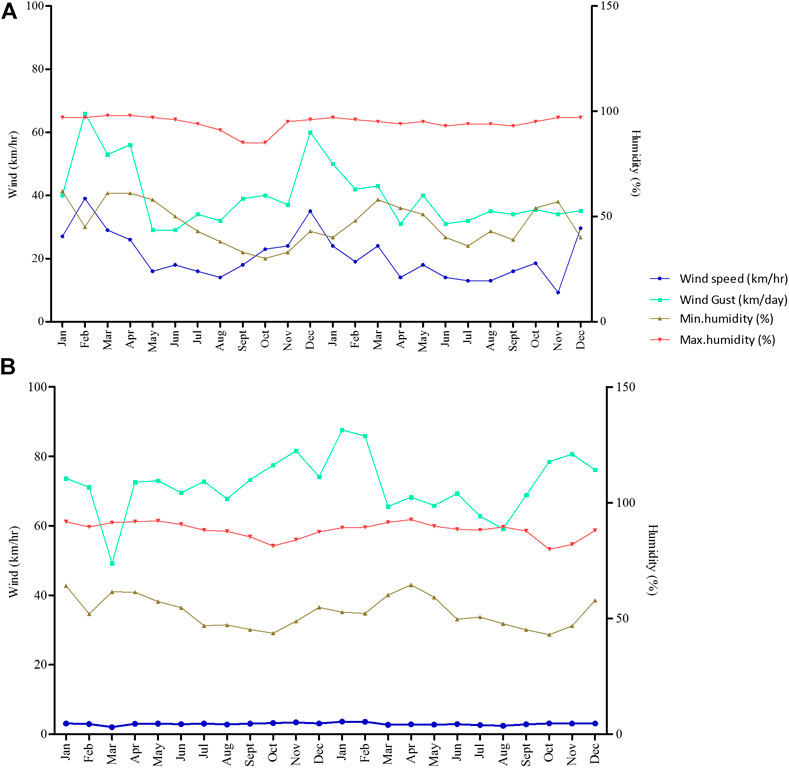
FIGURE 5. Wind and humidity parameters during the dry and rainy seasons for KPL (A) and KSC (B) plantations. Arrows indicate conditions during sampling seasons.
Discussion
Pesticide Occurrences and Associated Drivers
Two sampling surveys were carried out over the course of one year—in the rainy and dry seasons. No regular monitoring was applied. Accordingly, this study enables a glimpse of the pesticide contamination in the Kilombero valley and will need to be followed by more regular and thorough studies. But this glimpse allowed the authors to come up with a number of observations:
Of Ninety Seven analyzed pesticides, only eleven were above the limit of detection in some samples, and also these had a low detection frequency over all samples of less than 20%. Most of these pesticides were herbicides, and only two insecticides (chlorpyrifos and propoxur). Considering the kind of pesticides that were imported between July 2013 and June 2014 to Tanzania, fungicides made up 57% of the total in metric tons, while herbicides and insecticides imports were much smaller (21 and 12%, respectively) (Lahr et al., 2016), however no fungicides were detected in this study. This implies that environmental concentrations will, however, first of all be related to type and volumes of pesticides applied. As Lahr et al. (2016) pointed out there are no published data on applied volumes with regard to region or crop type in Tanzania or for individual regions or plantations. However, they cite data from the Kilombero District Agricultural Team that show the amount of pesticide quantities distributed by retailers in Kilombero district. They also pointed out that that amongst many registered pesticides in Tanzania, only a few dominate the market such as the insecticides pirimiphos-methyl and endosulfan, and the herbicides 2, 4-D, glyphosate and paraquat. This means for future studies, a limited spectrum of pesticides screening should be expected. On the other side, attention must be paid, that relevant substances are not overlooked. Neither paraquat nor the mentioned insecticides were among the pesticides that were searched for in our survey. Further studies will thus have to modify the range of analytes.
Activities and processes that are related to tropical seasons comprise among others:
1 ‐ Pesticide application before the sampling survey: whereby for rain fed—lowland farming, majority of pesticides are used during the dry seasons when the farms are prepared for the onset of first rainfalls, and during weeding seasons after the crops have emerged after onset of rainfalls or following harvesting seasons. Therefore, it is obvious that different types of pesticide residues will be detected depending on the seasons (Daam et al., 2019).
2 ‐ Increased precipitation and surface-run off: whereby during the rainy season (March/April) may not only lead to increased concentrations in the receiving waters, but may also cause enhanced degradation of pesticides in the flood plain sediments due to the high water content (Vryzas, 2018).
3 ‐ Higher temperatures: whereby during the dry seasons may increase the pesticide degradation in tropical soils (Daam et al., 2019).
Glyphosate was the only detectable pesticide in water that was sampled during the dry season but not in the rainy season, however, it was detected in sediments in both sampling surveys. Glyphosate is registered in Tanzania for control of annual grasses and broad leaved weeds (non-selective) on various crops including rice and sugarcane. It is applied for killing broad weeds prior to tilling the land for the succeeding rice growing season in the lowland rain-fed rice farms (Mbapila and Kibanda, 2006; Rodenburg et al., 2019). In sugarcane farms, it is also used for non-selective weed control under minimum tillage to supplement hand weeding (TPRI, 2011). This suggests more extensive use of glyphosate during the dry season before the onset of rainfalls. Therefore, emission into the water system may have been directly through application, as opposed to atrazine, metribuzin, and 2-hydroxyatrazine (O’Brien et al., 2016). These are pre- and post-emergence herbicides which were more frequently detected in the rainy season water than in sediments samples collected along sugar cane plantations areas. Surface run-off may have led to increased emissions to the aquatic system.
Out of 58-sediments and 17-soil samples that were measured, only three soil and three sediment samples had a higher OM content (<5%) and in four of such samples glyphosate was detected. This indicates high mobility of glyphosate and other organic contaminants in the areas where such samples were collected. Degradation and mobility of pesticides from the point of application depends on environmental conditions and the extent of adsorption of the pesticide under any particular soil type, while soil solution conditions depend on the nature and properties of the soil and the physico-chemical properties of the pesticide (Eklo, et al., 2019). For instance, pesticides with relatively low Koc (less than 1,900 L/kg) such as diuron and propoxur, long hydrolysis and photolysis half-lives (Supplementary Table S4) are prone to off-site movement in surface runoff and for permeable soils they can easily leach to ground water (Wauchope et al., 2002).
92% of the Kilombero soil and sediment samples had relatively low OM content (Table 2). and high content of clay, indicating high cation exchange capacity that can bind pesticides and thus limit the mobility of the pesticide within the area. The physical chemical properties of soils and sediment samples varied from one station to another, indicating variation in soil texture and SOM contents. The Harmonized World Soil Database (HWSD) indicates that the soil types of the KVRS wetland catchment vary spatially as fluvisols, acrisols, nitisols, lixisols, and cambisols (Dewitte et al., 2013). In this study, soil and sediment samples were taken in the valley where fluvisols are pre-dominant (Dewitte et al., 2013), whereby silty clay content were also high. Soils and sediments from fluvisols are less permeable compared to sandy-loam soils, as characterized by grain size analysis (Table 2), thus may allow water and other soluble contaminants to percolate through easily (FAO, 2006). Therefore, the findings of this study can just be an indication of how variable the SOM content within the same plantations fields can be, and thus for a better understanding further detailed studies on the link between soil types, SOM and soil texture in the KVRS catchment will add an understanding on the sorption and adsorption of a variety of pesticides in the area.
In sediment samples, chlorpyrifos, glyphosate, atrazine, diuron and its metabolite were detected both during the rainy and dry seasons. With the exception of diuron, concentrations were similar or lower during the dry season. Degradation half-lives of most pesticides are considerably increased if sediments turn anoxic during the rainy season (Viti et al., 2020), which may obscure the seasonal influence and explain the detection frequency. Low concentrations during the dry season might be due to increased microbial degradation rates in sediments (which still have water content) because of the availability of oxygen, but may also be a result of acidity (pH values ranged from 5.1 to 7.1) and photo-degradation by the increased solar radiation (Muskus, et al., 2020).
Diuron, however, was present in higher concentrations in sediments in the dry season than in the rainy season, even though it could not be detected in the water phase, excluding the option of recent application prior to the sampling. With a half-life in aerobic soil of 372 days compared to 995 days under anaerobic conditions (Kollman and Segawa, 1995), it may persist in anoxic sediments for a long time. With a log Kow of 2.87, it will partition to sediment surfaces to some extent, which explains detection in sediments but not in the overlying waters in the dry season.
Environmental Risks are Associated with Only Few of the Detected Substances
Of the eight pesticides and three metabolites detected, diuron and chlorpyrifos were measured in concentrations, which were close to the respective Predicted No Effect (PNEC) level (chlorpyrifos) or exceeded it substantially (diuron): Maximum diuron concentrations in water were >30 times higher than the estimated PNEC value of 0.054 μg L−1 rendering it likely that the aquatic community would be affected. Diuron is especially toxic to algae, inhibiting the photosystem II and may thus especially harm primary producers (Thomas et al., 2020).
Diuron has been detected in monitoring programs across tropical areas such as Australia, Brazil, Chile and Hawai (Davis et al., 2012; Lewis et al., 2016). As a pre-emergence herbicide that is applied to soils, it will be especially prone to surface run-off. This is reflected by the highest concentration detected in the water samples from the rainy season, overriding any dilution effects from increased water discharges. With concentrations bet n 0.053–1.7 μg L−1, even though detected only in three out of eight water samples, these are in the lower range of concentrations measured by O’Brien et al. (2016) in the downstream of a heavily irrigated cropping area in the dry-tropics (0.01–12.8 μg L−1). Occurrence of diuron in water samples in their study was highly variable but was highest following application and decreasing with the onset of rainfalls. This is in line with the detection of diuron in sediment and water samples collected in rice plantations during the mid of the rainy seasons in the first weeding season. Studies by Davis et al. (2013) showed that while majority of pesticides are transported in the dissolved form, diuron is transported in a particulate matter bound form. This indicates the importance of timing sampling surveys to rainfall events.
Chlorpyrifos, a pesticide potentially causing adverse effects in the aquatic system, is not classified as persistent in soils, sediments and water under the Stockholm Convention or EC regulations (Giesy et al., 2014). Its degradation half-life in soils is within 17–95 days much lower under anaerobic conditions than that of diuron (Kollman and Segawa, 1995) which explains the detection of lower concentrations during dry conditions with low surface run-off than the rainy seasons. Chlorpyrifos is an organophosphate insecticide which shows highest toxicity to crustaceans, followed by insects, but less sensitive to fish (Giddings et al., 2014). With a logKow of 4.7 and a bio-concentration factor of 1,374 (PPDB), its uptake into organisms is elevated, but information on biomagnification is scarce. While Giddings et al. (2014) assume a low environmental risk from chlorpyrifos in the United States, the concentrations detected in our study are higher than either of the concentrations cited by Mackay et al. (2014) (45–120 ng g−1, compared to eight cited studies reporting sediment concentrations of 0.01–10 ng g−1 in Mackay et al., 2014). Due to the fast degradation of chlorpyrifos within a few days after application, these concentrations indicate frequent emissions, reducing the time for recovery of organisms due to multiple exposures. Therewith, a risk to sensitive organism is probable. The more so, as Daam and van den Brink (2007) determined a NOEC for the most sensitive organisms to chlorpyrifos in a microcosms experiment to be 0.005 μg L−1 which is lower than the PNEC of 0.017 μg L−1 considered in this study and would point to a much higher risk from chlorpyrifos than assessed here.
Future Risks of Pesticides Exposure in the KVRS under the Projected Scenarios of Climate Change
Based on our demonstration in this paper the study is very important for East African region as it has such a high ecological relevance to the region and to the country in general. Since the KVRS catchment is intensively used for agricultural activities with high rate of application of pesticides and fertilizers, the quality of the soil for farming might be decreasing drastically and the ecological relevance of the Ramsar might be lost. It is predicted that, as climate changes, global pesticide use will increase in order to combat emergence of new pests and diseases (Boxall et al., 2009; Delcour et al., 2015), further affecting non-target organisms. Besides, with intensification of agricultural activities in the Kilombero wetlands catchment areas, the concentration of pesticides and other toxic chemicals in the environments will also continue to increase due to increased use for yield and profits maximization. Therefore, climate change likely can lead to deleterious ecological impacts in the KVRS such as physiological stress, species decline in both terrestrial and aquatic ecosystems due to altered habitats resulting from changes in rainfall patterns, salinity, pH, thermal regimes, and stratification of aquatic systems (Buisson et al., 2013).
Climate patterns model projections until 2060 by Näschen et al. (2019), for future climate scenarios for the Kilombero valley catchment indicates increasing trend in temperatures especially in the dry seasons (June–October), thus an increase in the drought risk in the area. The model has predicted an increase in annual precipitation pattern, which is however, unaffected by the dry seasons. The highest increase of precipitation is predicted to occur between February and March (+157 mm) while decreasing in April by −47 mm. In this study, Figure 2 indicates that KVRS receives a monthly total precipitation ranging from zero during the dry seasons to 400 mm in the rainy seasons, while total annual precipitation ranges from 700 to 1,500 mm. This implies that heavy precipitations may play a major role in washing off the pesticides from the treatment sites, accelerating surface run off, which has a potential of posing a toxicological risk to aquatic ecosystems. Surface particle runoff during heavy rains in the KVRS may result into increased turbidity of shallow waters and hence decrease photolysis and disappearance rates of certain pesticides (Lahr et al., 2000).
The model by Näschen et al. (2019) also predicts an increase in the rate of evapotranspiration and the water yield which is closely linked to the precipitation trends and surface run off. In this study, recorded total monthly sunshine hours for KVRS varied from 132.9 to 261.1 h while monthly atmospheric radiation ranged from 425.8 to 764 MJ/m2 (Figure 4). This implies that there might be high evaporation rate of pesticides from application sites thus causing ambient air pollution. A study by Magallona (1989) showed that high sunlight intensity in the tropics causes direct photolytic effects and hence enhances photodecomposition of toxicants. Moreover, monthly mean wind speed for weather station A (KPL-Mngeta farms) varied from 9.25 to 39.0 km/hr (Figure 5A) while weather station B (KSC-Kidatu farms) recorded a mean wind speed of 2.98 km/h (Figure 5B). This implies that pesticides can easily be dispersed from one point to another through wind dispersion, thus a wider river basin might potentially be affected by diffuse source of pesticides. The trend line graphs in Figure 3 shows that for the KVRS the average maximum temperatures (33°C) during the dry seasons fluctuate slightly with an average increase of 0.098°C/month, while average minimum temperatures (17°C) decreases at an average of 0.25°C per month. Since, volatilization of pesticides and fertilizers from soil and vegetation increases with temperatures (Yeo et al., 2003); there will be increasing risks of atmospheric diffuse contamination as temperature rises. Figure 5 indicates that the monthly humidity of the area ranges from 40 to 95% while daily lowest and highest soil and air temperature in the field ranged from 12 to 37°C per day depending on the season, indicating a potential influence to soil microorganism activities. Climate change associated stressors such as elevated ambient temperatures have a potential to affect the toxicokinetics and toxicodynamics of pesticides, thus increasing the toxicity of certain pesticides by altering their mechanistic toxicity (Jacobson et al., 2008; DeLorenzo et al., 2009). A study by Mattei et al. (2019), shows that pesticide degradation is enhanced by humidity, temperature, and pH, thus their degradation might lead to high levels of structural modifications which might produce degradates with an even higher impact on both human health and environment than the parent compound. Studies by Bouseba et al. (2009) indicates that degradation of pesticides such as 2, 4-D is reduced (with increase in persistence) in areas characterized by elevated soil temperatures and limited rainfall. A study by Benoit et al. (2007) indicate that a slight decrease of soil temperature may modify herbicide degradation, increased leaching and can drastically increase the bioavailability of some pesticides such as metribuzin. Viswanathan and Krishnamurti (1989) showed that solubility of organochlorine insecticides in water increased with ambient temperature, thus leading to greater uptake and toxicity to aquatic biota.
Conclusion
Concentrations of pesticide residues in the environment are a consequence of application, precipitation, temperature, soil organic carbon content and the chemical properties such as persistence, solubility and volatility. Projections of climatic changes indicate, that some of these processes will be directly affected (increased precipitation, and temperature), and likely followed by indirect consequences (reduction of soil organic carbon content, increase of pesticide application). The extent and direction to which a particular system will develop is currently, on the basis of present data, unpredictable. Whether pesticides’ degradation will dominate over increased surface run-off or whether especially pesticides that are persistent under anoxic conditions will gain importance due to accumulation in anaerobic sediments will depend on the degree, to which environmental factors change and will depend on the type of pesticide applied. In order to not shift a toxic burden from soil to the aquatic systems, it seems of uttermost importance to increase our knowledge on soil-water-sediment interactions in the tropics with regard to pesticides and largely improve our system understanding. Further research is recommend to model the potential of aquatic risks of pesticides in tropical agronomic areas as influenced by application rates, chemical properties of individual pesticide soil organic carbon content and tropical meteorological parameters.
Data Availability Statement
The original contributions presented in the study are included in the article/Supplementary Material, further inquiries can be directed to the corresponding author.
Author Contributions
The authors SM, SH, and BU contributed directly to the concepts, analyses, writing of the paper and development of conclusions. SM and SH collected field samples, BU provided technical assistance on the assessment of the soil and sediment physical parameters. SH calculated the risks from pesticides exposure and SM drafted, formatted and prepared the paper for submission. All authors read and approved the final manuscript.
Funding
This work was supported by the Schlumberger Foundation-Faculty for the Future fellowship for SM (2011-2014) and German Academic Exchange Service (DAAD) grants fellowships for SM (2011-2015).
Conflict of Interest
The authors declare that the research was conducted in the absence of any commercial or financial relationships that could be construed as a potential conflict of interest.
Acknowledgments
The content of this manuscript has been published [IN PART] as part of the PhD Thesis of SILVIA MATERU [Materu, S. F. (2015) Applicability of a Biotest Battery Developed for Temperate Regions to Tropical Environments: Implications for Sustainable Wetland Management –A Case Study of Kilombero Ramsar Site”. PhD Thesis, Leuphana University of Lüneburg, Germany]. We sincerely acknowledge the support of the managers of the study plantations/farms, for providing necessary meteorological data and allowing us to collect samples. We are very grateful to the agronomists/farm managers of the study plantations for their assistance during sampling campaigns. We acknowledge the critical comments of the three anonymous reviewers that have helped to improve this article.
Supplementary Material
The Supplementary Material for this article can be found online at: https://www.frontiersin.org/articles/10.3389/fenvs.2021.599814/full#supplementary-material.
References
Arias-Estévez, M., López-Periago, E., Martínez-Carballo, E., Simal-Gándara, J., Mejuto, J.-C., and García-Río, L. (2008). The mobility and degradation of pesticides in soils and the pollution of groundwater resources. Agric. Ecosyst. Environ. 123 (4), 247–260. doi:10.1016/j.agee.2007.07.011
Bakengesa, S., Munishi, P., and Navrud, S. (2011). “Potential climate change impacts on direct economic values from wildlife in the Kilombero Ramsar site, Tanzania,” in Experiences of climate change adaptation in Africa. Climate change management. Editor W. Leal Filho (Berlin: Springer), 33–53. doi:10.1007/978-3-642-22315-0_3
Baxter, T. E. (2018). Laboratory determination of soil pH by extraction with a calcium chloride ionic strength-stabilizing solution Standard operating procedures SOP AMBL-205-C. Appl. Microbiol. Biotechnol. Lab. 1–5., Available at: www.cefns.nau.edu>sop>SOP_AMBL_205C_pH_Soil.
Bedos, C., Cellier, P., Calvet, R., Barriuso, E., and Gabrielle, B. T. (2002). Mass transfer of pesticides into the atmosphere by volatilization from soils and plants: overview. Agronomie 22 (1), 21–33. doi:10.1051/agro:2001003
Benoit, P., Perceval, J., Stenrød, M., Moni, C., Eklo, O. M., Barriuso, E., et al. (2007). Availability and biodegradation of metribuzin in alluvial soils as affected by temperature and soil properties. Weed Res. 47 (6), 517–526. doi:10.1111/j.1365-3180.2007.00589.x
Bouseba, B., Zertal, A., Beguet, J., Rouard, N., Devers, M., Martin, C., et al. (2009). Evidence for 2,4-D mineralisation in Mediterranean soils: impact of moisture content and temperature. Pest Manag. Sci. 65 (9), 1021–1029. doi:10.1002/ps.1789
Boxall, A. B., Hardy, A., Beulke, S., Boucard, T., Burgin, L., Falloon, P. D., et al. (2009). Impacts of climate change on indirect human exposure to pathogens and chemicals from agriculture. Environ. Health Perspect. 117 (4), 508. doi:10.1289/ehp.0800084
Buisson, L., Grenouillet, G., Villéger, S., Canal, J., and Laffaille, P. (2013). Toward a loss of functional diversity in stream fish assemblages under climate change. Glob. Change Biol. 19, 387–400. doi:10.1111/gcb.12056
Constantini, D. (2015). Land-use changes and agriculture in the tropics: pesticides as an overlooked threat to wildlife. Biodivers. Conserv. 24, 1837–1839. doi:10.1007/s10531-015-0878-8
Daam, M. A., Chelinho, S., Niemeyer, J. C., Owojori, O. J., De Silva, P. M. C. S., Sousa, J. P., et al. 2019). Environmental risk assessment of pesticides in tropical terrestrial ecosystems: test procedures, current status and future perspectives. Ecotoxicol. Environ. Saf. 181, 534–547. doi:10.1016/j.ecoenv.2019.06.038
Daam, M. A., and Van den Brink, P. J. (2007). Effects of chlorpyrifos, carbendazim, and linuron on the ecology of a small indoor aquatic microcosm. Arch. Environ. Contam. Toxicol. 53, 22–35. doi:10.1007/s00244-006-0001-y
Davis, A. M., Lewis, S. E., Bainbridge, Z. T., Glendenning, L., Turner, R. D., and Brodie, J. E. (2012). Dynamics of herbicide transport and partitioning under event flow conditions in the lower Burdekin region, Australia. Mar. Pollut. Bull. 65 (4-9), 182–193. doi:10.1016/j.marpolbul.2011.08.025
Davis, A. M., Thorburn, P. J., Lewis, S. E., Bainbridge, Z. T., Attard, S. J., Milla, R., et al. (2013). Environmental impacts of irrigated sugarcane production: herbicide run-off dynamics from farms and associated drainage systems. Agric. Ecosyst. Environ. 180, 123–135. doi:10.1016/j.agee.2011.06.019
Delcour, I., Spanoghe, P., and Uyttendaele, M. (2015). Literature Review: impact of climate change on pesticide use. Food Res. Int. 68, 7–15. doi:10.1016/j.foodres.2014.09.030
DeLorenzo, M. E., Wallace, S. C., Danese, L. E., and Baird, T. D. (2009). Temperature and salinity effects on the toxicity of common pesticides to the grass shrimp, Palaemonetes pugio. J. Environ. Sci. Health B 44, 455–460. doi:10.1080/03601230902935121
Dewitte, O., Jones, A., Spaargaren, O., Breuning-Madsen, H., Brossard, M., Dampha, A., et al. (2013). Harmonisation of the soil map of Africa at the continental scale. Geoderma 211–212, 138–153. doi:10.1016/j.geoderma.2013.07.007
Di Toro, D. M., Zarba, C. S., Hansen, D. J., Berry, W. J., Swartz, R. C., Cowan, C. E., et al. (1991). Technical basis for establishing sediment quality criteria for nonionic organic chemicals using equilibrium partitioning. Environ. Toxicol. Chem. 10, 1541–1583. doi:10.1002/etc.5620101203
DIN 18123 (2011). Soil, investigation and testing - determination of grain-size distribution. Berlin, Germany.
DIN 38407-35 (2010). German standard methods for the examination of water, waste water and sludge- jointly determinable substances (group F)– part-35: determination of selected phenoxyalkyl carbonic acids and further acid plant treatment agents- method using high performance liquid chromatography and mass spectrometric detection (HPLC-MS/MS) (F-35). Berlin, Germany.
DIN-ISO 16308 (2017). Determination of glyphosate and AMPA -High performance liquid chromatography (HPLC) with tandem mass spectrometric detection. Berlin, Germany: DIN Deutsches Institut für Normung e. V.
Eklo, O. M., Almvik, M., Hole, H., Nyborg, Å. A., Stenrød, M., Borgå, K., et al. (2019). Degradation and mobility of pesticides in Norwegian soils. Ejnfs, 303–305. doi:10.9734/ejnfs/2019/v9i330071
European Chemicals Bureau (2003). Technical guidance document on risk assessment. Part II. Chapter 3. Environmental risk assessment. European Commission. Joint research Centre, ISPRA. Office for Official Publications of the European Communities, Luxembourg. Available at: http://echa.europa.eu/documents/10162/16960216/tgdpart2_2ed_en. pdf.
FAO (2006). Guidelines for soil description. 4th Edition. Rome, Italy: Food and Agriculture Organization of the United Nations.
FAO (1999). Soil salinity assessment: methods and interpretation of electrical conductivity measurement Chapter 2. in FAO irrigation and drainage paper No 57. Rome, Italy.
Giddings, J. M., Williams, W. M., Solomon, K. R., and Giesy, J. P. (2014). “Risks to aquatic organisms from use of chlorpyrifos in the United States,” in Ecological risk assessment for chlorpyrifos in terrestrial and aquatic systems in the United States. Editors J. P. Giesy, and K. R. Solomon (Cham: Springer), 119–162. doi:10.1007/978-3-319-03865-0_5
Giesy, J. P., Solomon, K. R., Mackay, D., and Anderson, J. (2014). Evaluation of evidence that the organophosphorus insecticide chlorpyrifos is a potential persistent organic pollutant (POP) or persistent, bioaccumulative, and toxic (PBT). Environ. Sci. Eur. 26, 29. doi:10.1186/s12302-014-0029-y
Godfray, H. C., Beddington, J. R., Crute, I. R., Haddad, L., Lawrence, D., Muir, J. F., et al. (2010). Food security: the challenge of feeding 9 billion people. Science 327, 812–818. doi:10.1126/science.1185383
ISO 21458 (2008). Water quality: determination of glyphosate and AMPA-Method using high performance liquid chromatography (HPLC) and fluorometric detection. Berlin, Germany.
Jacobson, T., Prevodnik, A., and Sundelin, B. (2008). Combined effects of temperature and a pesticide on the Baltic amphipod Monoporeia affinis. Aquat. Biol. 1, 269–276. doi:10.3354/ab00028
Koli, P., Bhardwaj, N. R., and Mahawer, S. K. (2019). “Agrochemicals: harmful and beneficial effects of climate changing scenarios,” in Climate change and agricultural ecosystems. Editors K. K. Choudhary, A. Kuar, and K. A. Singh (Sawton, Cambridge: Woodhead Publishing), 65–94. doi:10.1016/B978-0-12-816483-9.00004-9
Kollman, W. S., and Segawa, R. (1995). Interim report of the pesticide chemistry database. Environmental Hazards Assessment Program, State of California, Environmental Protection Agency, Department of Pesticide Regulation, Environmental Monitoring and Pest Management Branch.
Lahr, J., Buij, R., Katagira, F., and Van Der Valk, H. (2016). Pesticides in the Southern Agricultural Growth Corridor of Tanzania (SAGCOT): a scoping study of current and future use, associated risks and identification of actions for risk mitigation. Wageningen Environmental Research. https://www.wur.nl/nl. Accessed 28 November 2020.
Lahr, J., Diallo, A. O., Gadji, B., Diouf, P. S., Bedaux, J. J. M., Badji, A., et al. (2000). Ecological effects of experimental insecticide applications on invertebrates in Sahelian temporary ponds. Environ. Toxicol. Chem. 19 (5), 1278–1289. doi:10.1002/etc.5620190509
Lekei, E. E., Ngowi, A. V., and London, L. (2014). Farmers' knowledge, practices and injuries associated with pesticide exposure in rural farming villages in Tanzania. BMC Public Health 14 (1), 389. doi:10.1186/1471-2458-14-389
Lewis, S. E., Silburn, D. M., Kookana, R. S., and Shaw, M. (2016). Pesticide behavior, fate, and effects in the tropics: an overview of the current state of knowledge. J. Agric. Food Chem. 64, 3917–3924. doi:10.1021/acs.jafc.6b01320
Mackay, D., Giesy, J. P., and Solomon, K. R. (2014). “Fate in the environment and long-range atmospheric transport of the organophosphorus insecticide, chlorpyrifos and its oxon,” in Ecological risk assessment for Chlorpyrifos in terrestrial and aquatic systems in the United States. Cham: Springer, 35–76. doi:10.1007/978-3-319-03865-0_3
Magallona, E. (1989). “Effects of insecticides in rice ecosystems in Southeast Asia,” in Ecotoxicology and climate: with special reference to hot and cold climates. Editors P. Bourdeau, J. A. Haines, W. Klein, and C. R. Krishna Murti (New York: John Wiley & Sons Ltd), 265–297.
Materu, S. F. (2015). Applicability of a Biotest Battery developed for temperate regions to tropical environments: Implications for sustainable wetland management –A Case study of Kilombero Ramsar SitePhD Thesis. Germany: Leuphana University of Lüneburg.
Materu, S. F., and Heise, S. (2019). Eco-toxicity of water, soil, and sediment from agricultural areas of Kilombero Valley Ramsar wetlands, Tanzania. Ecosyst. Health Sustainability. 5, 256–269. doi:10.1080/20964129.2019.1695545
Mattei, C., Wortham, H., and Quivet, E. (2019). Heterogeneous degradation of pesticides by OH radicals in the atmosphere: influence of humidity and particle type on the kinetics. Sci. Total Environ. 664, 1084–1094. doi:10.1016/j.scitotenv.2019.02.038
Mbapila, J., and Kibanda, N. (2006). Development and promotion of wild rice management strategies for the lowlands of southern Tanzania. Final Technical Report, Project R8477. https://agris.fao.org/agris-search/search.do?recordID=GB2012104197. (Accessed November 19, 2020).
MNRT (2004). Ministry of natural resources and Tourism: the development and Implementation of an Integrated management plan of Kilombero valley flood plain- Ramsar site identification report. Tanzania: Dar es Salaam, 160.
Müller, C., Cramer, W., Hare, W. L., and Lotze-Campen, H. (2011). Climate change risks for African agriculture. Proc. Natl. Acad. Sci. USA 108 (11), 4313–4315. doi:10.1073/pnas.1015078108
Muro, J., Lopez, A., Strauch, A., Steinbach, S., Truckenbrodt, J., and Thonfeld, F. (2017). “25 years of landscape changes in the Kilombero floodplain, Tanzania,” in Worldcover 2017 Conference, 14–16 March 2017. Frascati (Rome), Italy: European Space Agency-ESRIN.
Muskus, A. M., Krauss, M., Miltner, A., Hamer, U., and Nowak, K. M. (2020). Degradation of glyphosate in a Colombian soil is influenced by temperature, total organic carbon content and pH. Environ. Pollut. 259, 113767. doi:10.1016/j.envpol.2019.113767
Näschen, K., Diekkrüger, B., Leemhuis, C., Seregina, L., and van der Linden, R. (2019). Impact of climate change on water resources in the Kilombero Catchment in Tanzania. Water 11 (4), 859. doi:10.3390/w11040859
NBS (2016). The National Bureau of Statistics, Tanzania in Figures, United Republic of Tanzania, Dares salaam, 17–23. http://www.nbs.go.tz/index.php/en/tanzania-in-figures/277-tanzania-in-figures-2016.
Ngowi, A., Mrema, E., and Kishinhi, S. (2016). Pesticide health and safety challenges facing informal sector workers. New Solut. 26 (2), 220–240. doi:10.1177/1048291116650262
Okada, E., Pérez, D., De Gerónimo, E., Aparicio, V., Massone, H., and Costa, J. L. (2018). Non-point source pollution of glyphosate and AMPA in a rural basin from the southeast Pampas, Argentina. Environ. Sci. Pollut. Res. Int. 25 (15), 15120–15132. doi:10.1007/s11356-018-1734-7
Osano, O., Nzyuko, D., Tole, M., and Admiraal, W. (2003). The fate of chloroacetanilide herbicides and their degradation products in the Nzoia Basin, Kenya. Ambio 32 (6), 424–427. doi:10.1579/0044-7447.2003032[0424:TFOCHA]2.0.CO;2
O’Brien, D., Lewis, S., Davis, A., Gallen, C., Smith, R., Turner, R., et al. (2016). Spatial and temporal variability in pesticide exposure downstream of a heavily irrigated cropping area: application of different monitoring techniques. J. Agric. Food Chem. 64, 3975–3989. doi:10.1021/acs.jafc.5b04710
Poppy, G. M., Jepson, P. C., Pickett, J. A., and Birkett, M. A. (2014). Achieving food and environmental security: new approaches to close the gap. Philos. Trans. R. Soc. Lond. B, Biol. Sci. 369, 20120272. doi:10.1098/rstb.2012.0272
RAM (Ramsar Advisory Mission) Report (2017). Kilombero valley, United Republic of Tanzania, Ramsar site No. 1173.
Ramsar (2002). Information sheet on Ramsar wetlands, the Kilombero valley flood plain, United Republic of Tanzania.
Rodenburg, J., Johnson, J.-M., Dieng, I., Senthilkumar, K., Vandamme, E., Akakpo, C., et al. (2019). Status quo of chemical weed control in rice in sub-Saharan Africa. Food Sec. 11 (1), 69–92. doi:10.1007/s12571-018-0878-0
SAGCOT (2011). Southern agricultural growth Corridor of Tanzania: Investment Blueprint, dar es salaam, Tanzania.
Schumacher, B. A. (2002). Methods for the determination of total organic carbon (TOC) in soils and sediments, NCEA-C- 1282 ERASC. USA: Las Vegas.
Soares, J. C., Santos, C. S., Carvalho, S. M. P., Pintado, M. M., and Vasconcelos, M. W. (2019). Preserving the nutritional quality of crop plants under a changing climate: importance and strategies. Plant Soil 443 (1–2), 1–26. doi:10.1007/s11104-019-04229-0
Thomas, M. C., Flores, F., Kaserzon, S., Reeks, T. A., and Negri, A. P. (2020). Toxicity of the herbicides diuron, propazine, tebuthiuron, and haloxyfop to the diatom Chaetoceros muelleri. Sci. Rep. 10 (1), 19592–19614. doi:10.1038/s41598-020-76363-0
TPRI (2011). Tanzania Pesticide Research Institute: List of Registered Pesticides in Tanzania. (Made under section 18 of the Plant Protection Act, 1997 and Plant Protection Regulations GN 401 of 1999).
TPRI (2020). Tanzania Pesticide Research Institute: Provisional list of registered pesticides in Tanzania, June 2020. (Made under section 18 of the Plant Protection Act, 1997 and Plant Protection Regulations GN 401 of 1999), Arusha.
Viswanathan, P., and Krishnamurti, C. (1989). “Effects of temperature and humidity on ecotoxicology of chemicals,” in Ecotoxicology and climate: with special references to hot and cold climates. Editors P. Bourdeau, J. A. Haines, W. Klein, and C. R. Krishna Murti (New York: John Wiley & Sons Ltd), 139–154.
Viti, M. L., Mendes, K. F., dos Reis, F. C., Guimarães, A. C. D., Soria, M. T. M., and Tornisielo, V. L. (2020). Characterization and metabolism of bound residues of three herbicides in soils amended with sugarcane waste. Sugar Technol. 23, 23–37. doi:10.1007/s12355-020-00884-1
Vryzas, Z. (2018). Pesticide fate in soil-sediment-water environment in relation to contamination preventing actions. Curr. Opin. Environ. Sci. Health 4, 5–9. doi:10.1016/j.coesh.2018.03.001
Wandiga, S. O. (2001). Use and distribution of organochlorine pesticides. The future in Africa. Pure Appl. Chem. 73 (7), 1147–1155. doi:10.1351/pac200173071147
War, A. R., Taggar, G. K., War, M. Y., and Hussain, B. (2016). Impact of climate change on insect pests, plant chemical ecology, tritrophic interactions and food production. Int. J. Clin. Biol. Sci. 1 (02), 16–29. http://oar.icrisat.org/id/eprint/9735.
Wauchope, R. D., Yeh, S., Linders, J. B., Kloskowski, R., Tanaka, K., Rubin, B., et al. (2002). Pesticide soil sorption parameters: theory, measurement, uses, limitations and reliability. Pest Manag. Sci. 58 (5), 419–445. doi:10.1002/ps.489
Wee, S. Y., and Aris, A. Z. (2017). Ecological risk estimation of organophosphorus pesticides in riverine ecosystems. Chemosphere 188, 575–581. doi:10.1016/j.chemosphere.2017.09.035
Yeo, H., Choi, M., Chun, M. Y., and Sunwoo, Y. (2003). Concentration distribution of polychlorinated biphenyls and organochlorine pesticides and their relationship with temperature in rural air of Korea. Atmos. Environ. 37 (27), 3831–3839. doi:10.1016/S1352-2310(03)00456-4
Zhang, X., Shen, Y., Yu, X. Y., and Liu, X. J. (2012). Dissipation of chlorpyrifos and residue analysis in rice, soil and water under paddy field conditions. Ecotoxicol. Environ. Saf. 78 (0), 276–280. doi:10.1016/j.ecoenv.2011.11.036
Keywords: aquatic life, Ramsar wetlands, pesticides, weather conditions, tropics
Citation: Materu SF, Heise S and Urban B (2021) Seasonal and Spatial Detection of Pesticide Residues Under Various Weather Conditions of Agricultural Areas of the Kilombero Valley Ramsar Site, Tanzania. Front. Environ. Sci. 9:599814. doi: 10.3389/fenvs.2021.599814
Received: 28 August 2020; Accepted: 25 January 2021;
Published: 09 March 2021.
Edited by:
C. Marjorie Aelion, University of Massachusetts Amherst, United StatesReviewed by:
Kelly L Smalling, United States Geological Survey (USGS), United StatesElena Martínez Carballo, University of Vigo, Spain
Copyright © 2021 Materu, Heise and Urban. This is an open-access article distributed under the terms of the Creative Commons Attribution License (CC BY). The use, distribution or reproduction in other forums is permitted, provided the original author(s) and the copyright owner(s) are credited and that the original publication in this journal is cited, in accordance with accepted academic practice. No use, distribution or reproduction is permitted which does not comply with these terms.
*Correspondence: Silvia F. Materu, c21hdGVydUBzdWEuYWMudHo=