- 1Department of Agricultural and Biosystems Engineering, Iowa State University, Ames, IA, United States
- 2Department of Animal Biosystems Science, Chungnam National University, Daejon, Republic of South Korea
- 3Faculty of Life Sciences and Technology, Wroclaw University of Environmental and Life Sciences, Wroclaw, Poland
- 4U.S. Department of Agriculture, Agricultural Research Service, Bushland, TX, United States
Odorous gas emissions from swine production have been a concern for neighbors and communities near livestock farms. Manure storage is one of the main sources of gaseous emissions. Manure additive products are marketed as a simple solution to this environmental challenge. Manure additives are user-friendly for producers and can be applied (e.g., periodically poured into manure) without changing the current manure storage structure. Little scientific data exist on how these products perform in mitigating gaseous emissions from swine manure. The research objective was to evaluate the effectiveness of 12 marketed manure additives on mitigating odor, ammonia (NH3), hydrogen sulfide (H2S), greenhouse gases (GHG), and odorous volatile organic compounds (VOCs) from stored swine manure. A controlled pilot-scale setup was used to conduct 8-week long trials using manufacturer-prescribed dosages of additives into swine manures. Manure was outsourced from three swine farms to represent a variety of manure storage types and other factors affecting the properties. Measured gaseous emissions were compared between the treated and untreated manure. None of the tested products showed a significant reduction in gaseous emissions when all (n = 3) manures were treated as replicates. Selected products showed a wide range of statistically-significant reduction and generation of gaseous emissions when emissions were compared in pairs of manure types from one farm. The latter observation highlighted the lack of consistent mitigation of gaseous emissions by manure additives. The results of this study do not warrant full-scale trials with the tested products.
Introduction
The swine industry has a significant environmental and socio-economic challenge with the gaseous emissions that originate from the storage, handling, and land-application of swine manure. Emissions of ammonia (NH3), hydrogen sulfide (H2S), volatile organic compounds (VOCs) contribute to odor nuisance. A relatively small subset of VOCs (e.g., phenolics, fatty acids, sulfur-containing VOCs) has been consistently ranked and prioritized as significant contributors to the characteristic smell of swine odor downwind from farms (Koziel et al., 2006). Researchers have measured gaseous and odor emissions from animal buildings (Akdeniz et al., 2012a; Bereznicki et al., 2012; Cai et al., 2015). Efforts linking concentrations of gases and measured odor are challenging (Akdeniz et al., 2012b; Parker et al., 2012). Concerns about climate change are also relevant to greenhouse gas (carbon dioxide (CO2), nitrous oxide (N2O), and methane (CH4) emissions from stored manure and land-applied manure (Maurer et al., 2017a). Airborne particulate matter can also sorb VOCs and be a carrier of odor (Cai et al., 2006).
Progress is being made on developing and testing technologies for the mitigation of odor and gaseous emissions from swine farms (Maurer et al., 2016; Iowa State University Extension Outreach, 2020). However, the farm-scale adoption of a particular technology depends on a number of site-specific regulatory and socio-economic factors. The Air Management Practices Assessment Tool (Iowa State University Extension Outreach, 2020) listing 12 approaches to mitigate odor and gaseous emissions. These include manure additives, i.e., products for surficial application to stored manure. Researchers have reported developing and testing manure additives such as various types of biochars (Maurer et al., 2017b; Chen et al., 2020a; Meiirkhanuly et al., 2020a,b), zeolites (Cai et al., 2007), and peroxidase (Maurer et al., 2017c,d). The swine industry has access to a relatively wide range of commercial products marketed as manure additives.
Manure additives are user-friendly for farmers because they can be applied (e.g., periodically poured into manure) without changing the current manure storage structure. The active ingredients are often proprietary, but the majority of products claim to contain microbial flora aiming to minimize odor-causing populations. Unfortunately, little scientific data exist on how these marketed products perform in mitigating gaseous emissions from swine manure, and the impact on odor mitigation is relatively low (Iowa State University Extension Outreach, 2020).
The objective of this study was to test the effectiveness of 12 marketed manure additives on their mitigation of gaseous emissions of odor, ammonia (NH3), hydrogen sulfide (H2S), greenhouse gases (GHG), and odorous volatile organic compounds (VOCs) from stored swine manure. The products were selected based on their usage in commercial swine production in the U.S. The 12 products were Triune, Confine, Manure Master Plus (MMP), Sulfi-Doxx dry (Sulfi-Dox), Waste Away, Oxydol, Enviro Lagoon, Penergetic G, Manure Magic (MM), Sludge Away; LLMO-SST (LLMO), and More Than Manure (MTM).
Our working hypothesis was that the tested manure additives will effectively reduce gaseous emissions from different types of swine manure when tested on a pilot-scale using the manufacturer-prescribed dose. This research shows the side-by-side comparisons of commercial additives to help the pork industry understand their performance and potential impact on mitigating odor and gaseous emissions from manure. Additionally, A similar study on the evaluation of manure additives was carried out 20 years ago; however, many new products have been introduced since then (Heber et al., 2001). Farmers and the regulatory community need reliable scientific data on the performance of marketed manure additives that are popular with the U.S. Midwest pork industry.
Experiments
The “Experiments” section is largely reduced to the essentials to avoid redundant information that was described in detail in the “Methods” section of the recently published paper (Chen et al., 2020b). The Chen et al. (2020b) paper is focused on methods and raw data presentation in an organized fashion for transparency and reuse. This paper focuses on data analysis, results, discussion, and conclusions.
Experimental Design
Table 1 summarizes the additive products tested in the four, 8-week long Trials of the effectiveness for mitigation of gas emissions from stored swine manure.
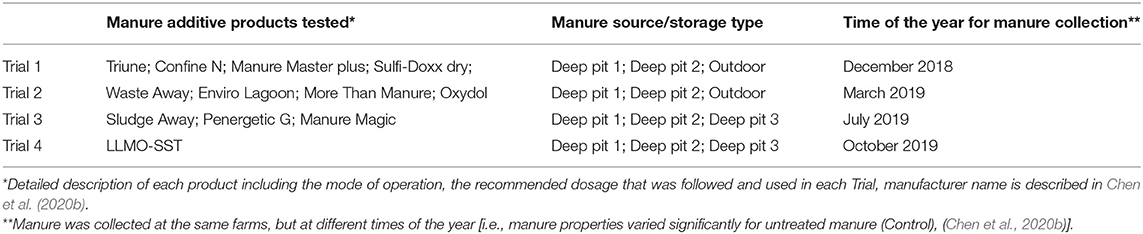
Table 1. Summary of four, 8-week long Trials to test the effectiveness of additive products for mitigation of gas emissions from stored swine manure.
A detailed description of the experimental design, key components of manure storage simulators, properties of manure, airflow control, gas (NH3, H2S, CO2, CH4, N2O), 11 odorous VOCs and odor concentration measurements are presented elsewhere (Chen et al., 2020b).
The experimental set up of this research was pilot-scale and aimed to simulate the deep pit swine manure storage structure. A total of 15 manure storage simulators are available, and each has a height of 1.22 meters (4 ft) and a diameter of 0.38 m (15 inches), as shown in Figure 1. Fresh manure was collected from three farms at different locations in Iowa. The detailed manure properties and manure collection are presented elsewhere (Chen et al., 2020b).
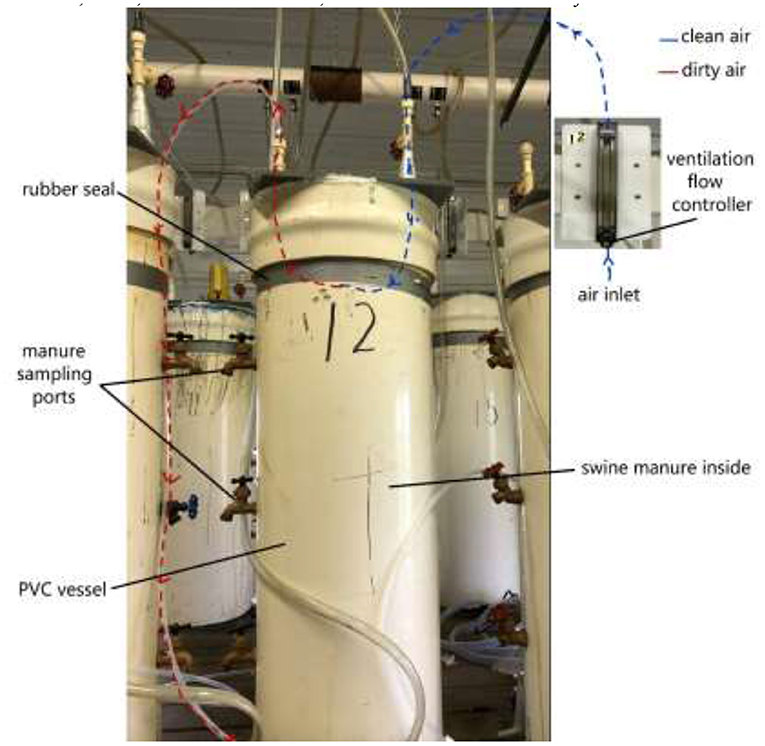
Figure 1. Pilot-scale setup of manure storage simulator to test the effectiveness of manure additives for mitigation of gas emissions. Airflow in the headspace is measured and controlled by a rotameter with a needle valve for each simulator. Gas samples are collected from the “dirty air” outlet. The mitigation effect was estimated by comparing the gaseous emissions between treated and untreated manure.
The manure storage simulators were initially filled with 74.6 L of swine manure. Every 2 weeks, 9.5 L of the manure from the same farm was added directly from the top of the simulators to simulate the manure addition in the real swine barn. The airflow rate was kept at 7.5 air exchange per hour (ACH) by FL-3839ST rotameters (Omega Engineering Inc, USA). Each Trial of the experiment lasted for 8 weeks. The baseline gas emissions from each simulator were measured for ~2 weeks before applying any treatment. The dosage of each product was followed by the recommended dosages on their product labels or websites. The gas concentration measurements for NH3 and H2S were done twice a week; GHG, odor concentrations, and VOCs were done weekly.
Ammonia and Hydrogen Sulfide
NH3 and H2S concentrations were measured with both Drager X-am 5600 portable gas analyzer and OMS-300, which can be used to measure real-time gas concentrations. OMS-300 is equipped with NH3/CR-1000 and H2S/C-50 electrochemical gas sensors (Wallisellen, Switzerland), and Drager X-am 5600 equipped with NH3 and H2S XS sensors (Luebeck, Germany) (Maurer et al., 2017b; Wi et al., 2019; Chen et al., 2020b).
Greenhouse Gases
Greenhouse gases were measured for CO2, N2O, and CH4. First GHG samples were collected with a syringe from the headspace of the manure simulators in 5.9 mL Extainer vials (Labco Limited, U.K.). All Extainer vials have been pre-cleaned with Helium gas (UHP 300) and vacuumed for over 7 cycles. Then the samples were analyzed with GHG-GC (SRI Instruments, Torrance, CA, USA) equipped with flame ionization detector (FID) and electron capture detector (ECD) (Maurer et al., 2017b; Chen et al., 2020b).
Volatile Organic Compounds
For VOC emissions, the manure storage air samples were collected in 1 L gas sampling glass bulbs (Supelco) by using portable sampling pumps. After bringing back to the lab, VOCs were absorbed with a 2 cm divinylbenzene/Carboxen/polydimethylsiloxane (DVB/Carboxen/PDMS) solid-phase microextraction (SPME) fiber (57384-U, Supelco, Bellefonte, PA, USA) for 50 min at lab temperature (23–24°C), then analyzed with a multidimensional GC-MS within 12 h of sample collection.
The SPME fiber loaded with VOCs was inserted into a 260°C G.C. (Microanalytics, Round Rock, TX, USA) inlet; VOCs were thermally desorbed for 2 min and analyzed by a mass spectrometer (Agilent, model 5973N, Santa Clara, CA, USA) (Chen et al., 2020b).
Odor
The odor samples were collected weekly by using Vac-U-Chamber (SKC Inc., Eighty-Four, PA, USA) and transfer back to the lab in 10 L Tedlar sample bags. Tedlar sample bags were flushed and vacuumed with air multiple times before using them. Within 12 h, all the odor samples were analyzed with AC'SCENT International Olfactometer (St. Croix Sensory Inc., Stillwater, MN, USA) using dynamic triangular forced-choice methods. There were four panelists, and each sample was evaluated twice by each panelist (Akdeniz et al., 2012a; Chen et al., 2020b).
Mitigation and Statistical Analyses
The experimental design was a completely randomized design. Gases such as NH3, H2S, CO2, N2O, and CH4 initially were all measured or analyzed in units of parts per million. Gas concentrations were first converted from the field condition to standard condition (1 atm, 25°C, and dry air). For odor, the unit was used Odor Units. For VOC, the peak area count was used.
The overall mean percent reduction to emissions was calculated with Equation 1:
Whereas, %R is the overall mean percent reduction, Econtrol is the average emission of the control, Etreatment is the average emission of the treatment.
The two-way ANOVA and Tukey-Kramer Method were used to determine the p-values of the reduction. All statistical analysis was done in JMP software (version Pro 15, SAS Institute, Inc., Cary, NC, USA). When a p-value is less than or equal to 0.05, the reduction is statistically significant.
All data were analyzed in two ways:
(a) Averaging results for treating all types of manure analyzed as replications (n = 3 replications, assuming manures from different farms are replicates).
(b) Treating all types of manure as being distinct (no replications, assuming manures from different farms are not replicates; comparing (n = 1) control vs. (n = 1) treatment using the same manure).
Results
In this research, a total of 12 manure additive products was evaluated (Table 1). Four products were evaluated in Trial 1, and another four products were evaluated in Trial 2; 3 products were evaluated in Trial 3; 1 product was evaluated in the last Trial (Trial 4). The percent reduction in gaseous emissions (%R) were all calculated by comparing emissions from the treated manure with the Control in the same Trial. The results are organized by Trials and presented in two types of tables for each Trial. The first table type summarizes results by (a) averaging results for treating all types of manure as replications (n = 3 replications, assuming manures from different sources are replicates). The second table type summarizes results by (b) treating all types of manure as being distinct (no replications, assuming manures from different sources are not replicates, comparing (n = 1) control vs. (n = 1) treatment using the same manure). Rows in each table are organized by targeted gases, starting with NH3 and followed by H2S, GHGs, odor, and odorous VOCs.
In addition, the Supplementary Material contains detailed comparisons of emissions for each targeted gas over 8 weeks of each Trial, illustrated with 130 figures (Supplementary Figures 1–130). Supplementary Tables 1–3 serve as a guide for finding results on a particular manure additive and targeted gas.
Trial 1 (Confine N, Triune, MMP, and Sulfi-Doxxdry)
The four products that were evaluated during Trial 1 of the experiment were Confine N, Triune, MMP, and Sulfi-doxxdry. The results of considering the three types of manure sources as triplicate were summarized in Table 2. The measurements over the 8-week of the experiment for all four productions are listed in Supplementary Material (Triune: Supplementary Figures 1–9; MMP: Supplementary Figures 10–18; Confine N: Supplementary Figures 19–27; Sulfi-dox: Supplementary Figures 28–36). For all targeted gases, there was not any statistically significant reduction found.
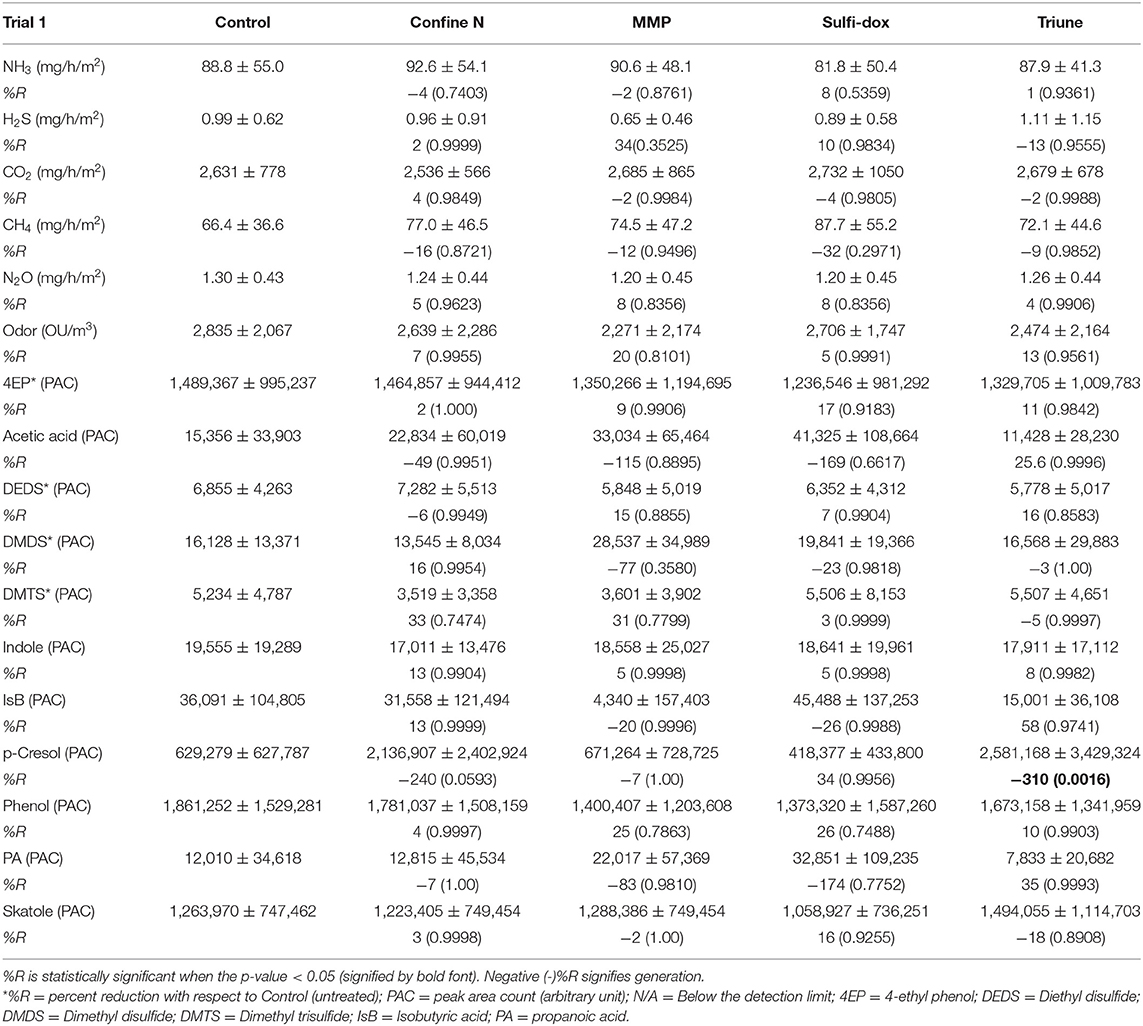
Table 2. Trial 1-comparison of averaged gaseous emissions (flux or arbitrary units for VOCs) and percent reductions (%R) from three types of manure sources (farms) with their standard deviations.
For NH3 emission, Sulfi-dox showed the highest percent reduction of 8% with a p-value of 0.5359. Confine N and MMP increased NH3 emissions. MMP and Sulfi-dox reduced the average H2S emissions by 34% with a p-value of 0.3525 and 10% with a p-value of 0.9834, respectively. Triune increased the H2S emissions by 13% with a p-value of 0.9555.
For GHG emissions, all four products did not have a significant impact on CO2 emissions. But all four products had increased the CH4 emissions production by 9~30%. MMP and Sulfi-dox reduced N2O emissions by 8%; Confine N and Triune reduced N2O emissions by 5 and 4%, respectively.
MMP had the highest percent reduction in Trial 1, 20%, and followed by Triune with a reduction of 13%. For VOC emissions, There were always mitigations in some targeted gases, and at the same time, generations in others. For example, MMP had mitigations in DEDS and DMTS by 15% and 32% but generated DMDS by 77%. Triune increased the p-cresol emission significantly by 310% with a p-value of 0.0016, and Confine N increased the p-cresol emission by 240% with a p-value of 0.0593.
The user's instructions for the manure additive products tested in this research did not specify “what kind of manure” they target to treat. These products are marketed for generic use in stored swine manure regardless of environmental conditions. Thus, we also analyzed the data by considering that the manure sources are “different” (no replication), which is summarized in Table 3 for Trial 1. Similar tables are also provided for Trials 2, 3, and 4.
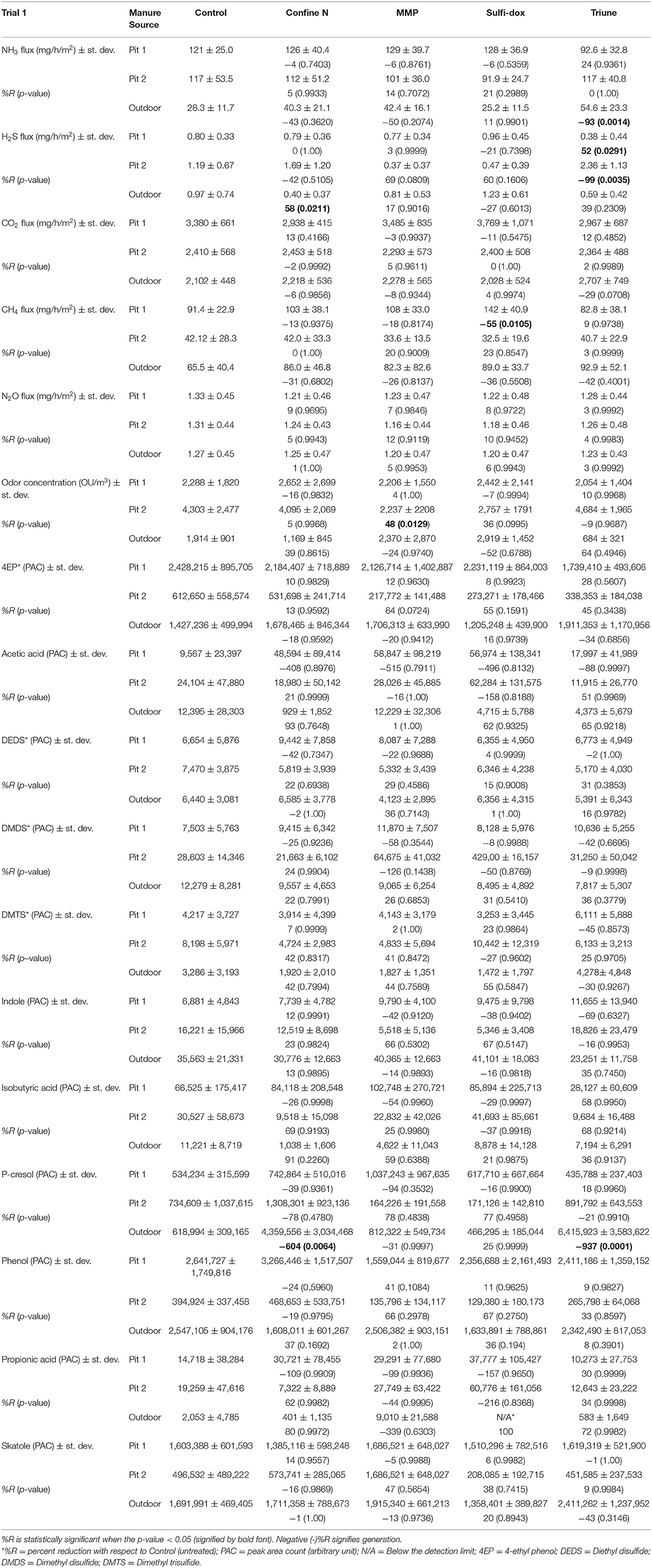
Table 3. Trial 1-comparison of averaged gaseous emissions (flux or arbitrary units for VOCs) and percent reductions (%R) analyzed separately for each type of manure sources (farms) with their standard deviations.
In general, the second approach to data analysis did not yield consistent results. For example, Triune showed generations of NH3 emission for outdoor storage manure by 93% with a p-value of 0.0014, but a 24% reduction on manure from deep pit 1 and no impact on NH3 emission from deep pit 2 manure. Triune also generated 937% more p-cresol with a p-value of 0.0001 in outdoor manure. A similar lack of trend also occurred for other products. Confine N showed a reduction of 58% with a p-value of 0.0211 for outdoor manure but generated 42% more H2S in manure from pit 2. Sulfi-dox generated 55% more on CH4 emission in deep pit 1 manure with a p-value of 0.0105. Confine N mitigated the odor concentration by 48% with a p-value of 0.0129 in manure from pit 2 and generated 604% more of p-cresol in outdoor manure with a p-value of 0.0064.
Trial 2 (WA, MTM, Enviro Lagoon, and Oxydol)
The four products tested in the second Trial were WA, MTM, Enviro Lagoon, and Oxydol. The analyzed results, which considering three types of manure sources were triplication, are summarized in Table 4. The measurements over the 8-week of the experiment for all four productions are listed in Supplementary Material (WA: Supplementary Figures 37–71; MTM: Supplementary Figures 72–80; Enviro Lagoon: Supplementary Figures 81–89; Oxydol: Supplementary Figures 90–98).
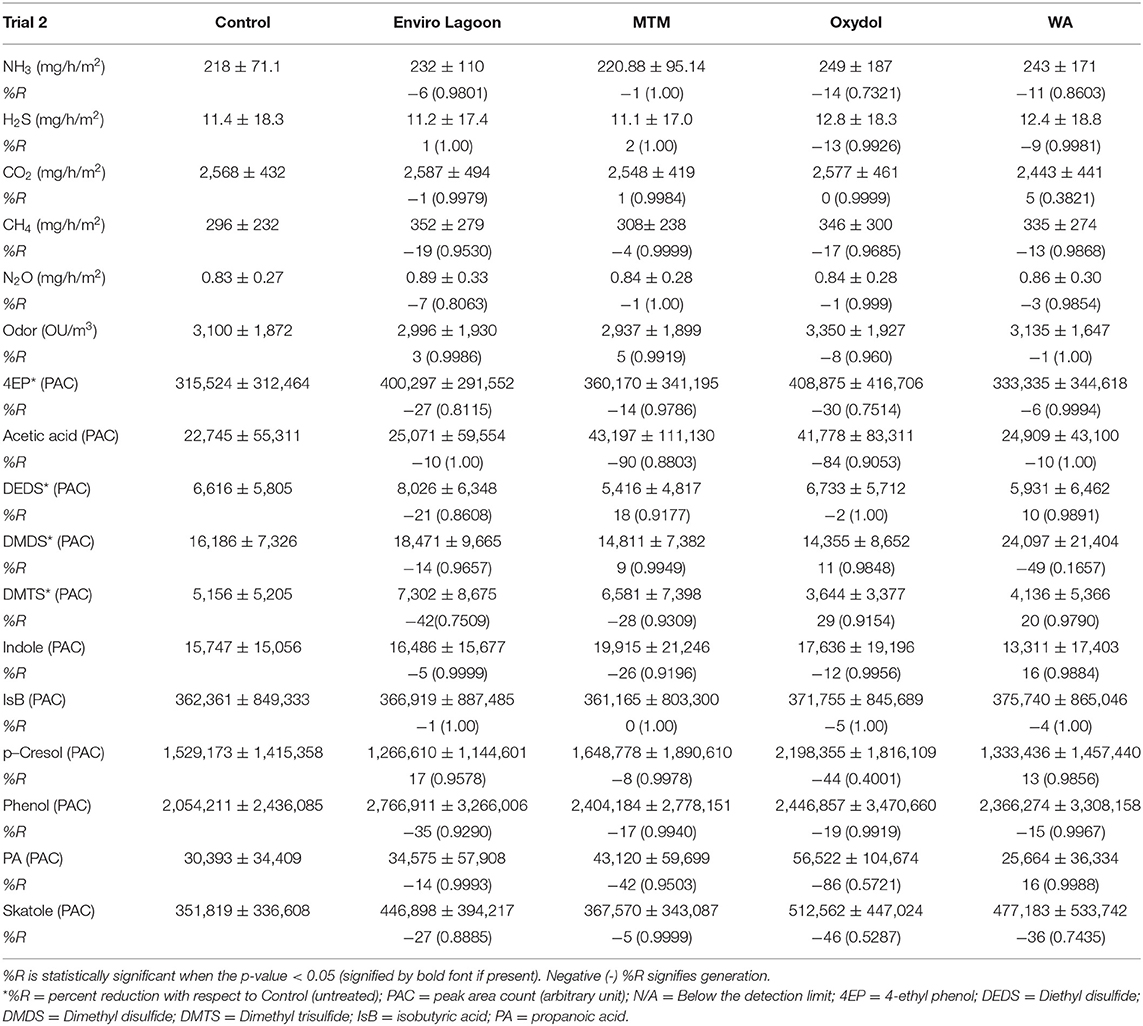
Table 4. Trial 2-comparison of averaged gaseous emissions from three manure sources (farms) with their standard deviations.
There was no significant reduction found for all of those four products. All four manure additives treatments increased NH3 emissions (p > 0.05). Oxydol and WA increased the H2S emissions by 9~13% (p > 0.05). There was no significant impact on CO2 and N2O emissions, and more CH4 emissions were generated for all four products. For odor concentrations, Enviro Lagoon and MTM treatments decreased by 3% with a p-value of 0.9986 and 5% with a p-value of 0.9919, whereas Oxydol and WA treatments increased by 8% with a p-value of 0.9600 and 1% with a p-value of 1.00, respectively.
A similar lack of trend was observed for VOCs as in Trial 1. There are random effects (p > 0.05) of mitigation and generations among the fatty acid, sulfide groups, and phenolic groups. But overall, no statistical significance was found in Trial 2 as we considering three manure sources as triplications.
In general, when we considered the manure sources are not replications, the second approach of data analysis did not yield consistent results to the %R in Trial 2 as shown in Table 5. One similar trend observed is that manure additives often have some degree of mitigation on one type of manure, but also generation in emissions in another manure source. Different targeted gases also might be the opposite results for the same kind of manure. Nevertheless, no matter which data analysis approach was used, the results were not statistically significant and lacked clear trends.
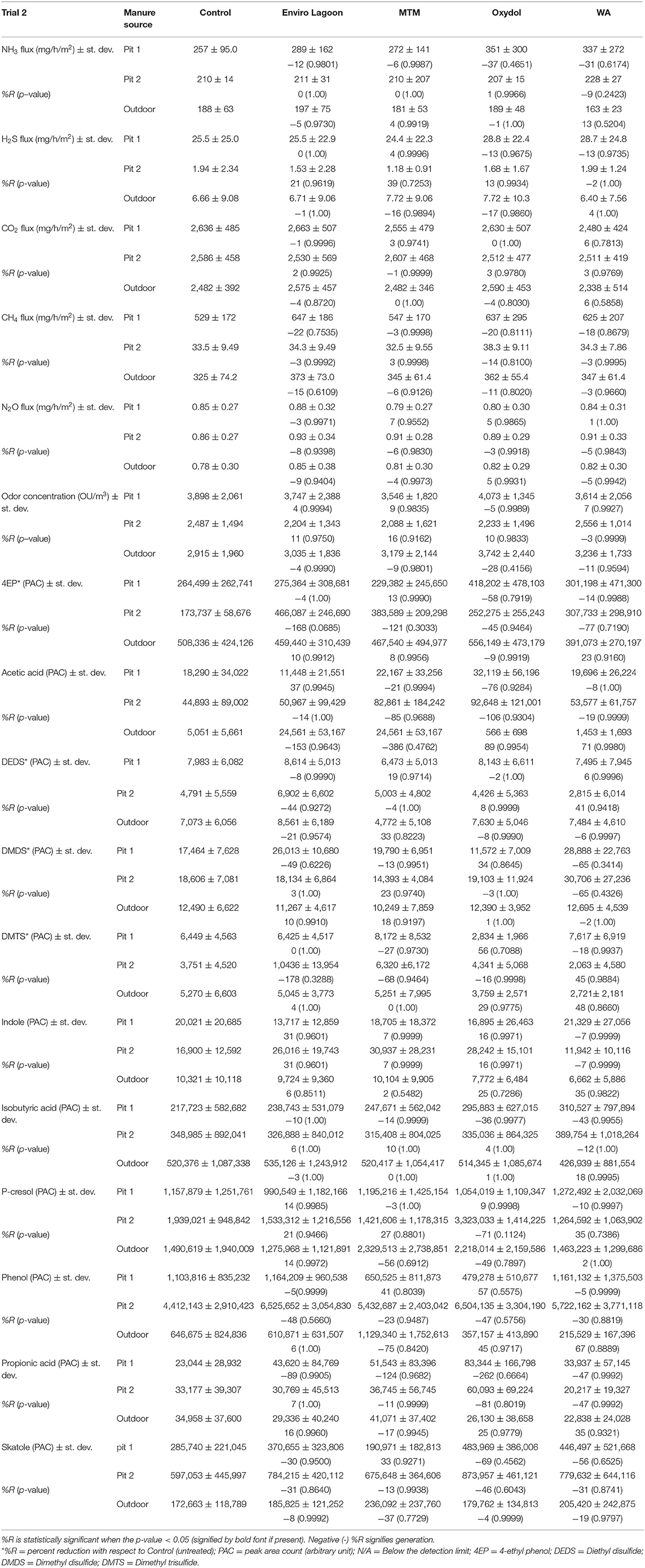
Table 5. Trial 2-comparison of gaseous emissions from each of the three manure sources (farms) with their standard deviations.
Trial 3 (MM, Penergetic G, Sludge Away)
The three products included in the third Trial were MM, Penergetic G, and Sludge Away. The measurements over the 8-week of the experiment for the three productions are listed in Supplementary Material (Sludge Away: Supplementary Figures 99–107; Penergetic G: Supplementary Figures 108–116; MM: Supplementary Figures 117–125). When we consider the manure to be replicated, there was no overall statistical significance to %R in any of the products tested in Trial 3, as shown in Table 6. For NH3 emissions, MM showed a 5% reduction, whereas Penergetic G and Sludge Away showed 4 and 5% generations, respectively. For H2S emission, MM and Penergetic G showed 18 and 25% reductions, Sludge Away still had 6% generation. For GHG emissions, MM showed a 4% generation in CO2 emission, a 31% generation in CH4 emission, and a 3% reduction in N2O; Penergetic G had a 4% generation in CO2 emission, a 37% generation in CH4 emission, and a 9% reduction in N2O emission. For odor, there was no statistical effect associated with all three products (≤3%R). For VOC emissions, no statistically significant %R was found, similar to the lack of trends observed in the first two Trials.
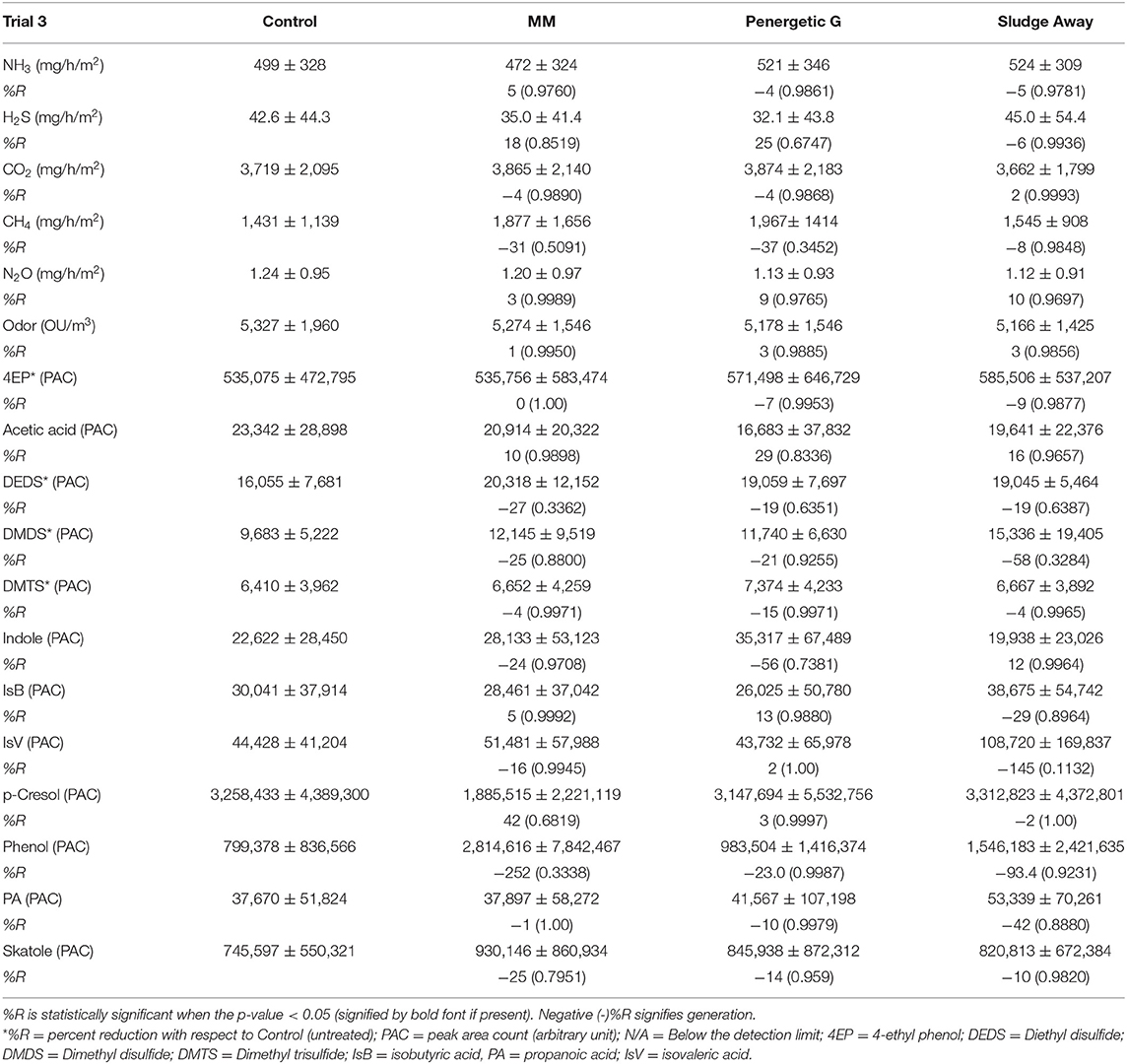
Table 6. Trial 3-Comparison of averaged gaseous emissions from three manure sources (farms) with their standard deviations.
In general, when we considered the manure sources are not replications, we found the statistical significance of %R for one product and one manure, and one VOC, as shown in Table 7. MM showed an 83% reduction with a p-value of 0.02 in acetic acid emitted from the manure of pit 2. However, a statistically significant generation was also observed. Sludge Away showed 307% generation with a p-value of 0.0067 and 149% generation with a p-value of 0.0044 in p-cresol and phenol, respectively, emitted from the manure of deep pit 1.
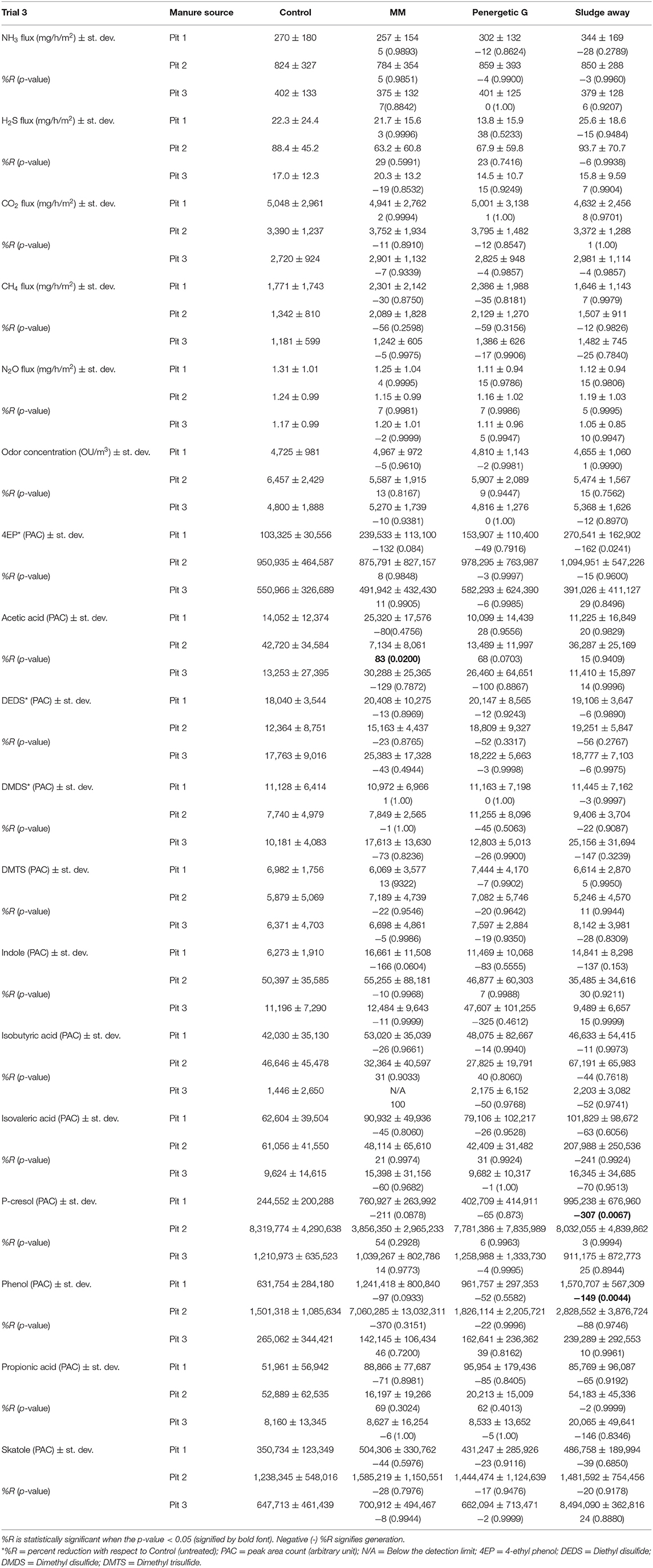
Table 7. Trial 3-comparison of gaseous emissions from each of the 3 manure sources (farms) with their standard deviations.
In general, the lack of statistical significance and trends was observed for the remainder of targeted gases. For NH3 emissions, MM showed 5% reductions for manure from pit 1 and 2, a 7% reduction for manure in pit 3; Penergetic G showed 12% and 4% generations in manure from pit 1 and 2, respectively, and no impact on emission for pit 3; Sludge Away showed 28 and 3% generations in manure from pit 1 and 2, a 6% reduction for manure from pit 3. For H2S emissions, MM showed 3% and 29% reductions in manure from pit 1 and 2, a 19% generation in manure from pit 3; Penergetic G showed 38, 23, and 15% reductions in manure from pit 1, 2, and 3, respectively; Sludge Away showed 15 and 6% generations in manure from pit 1 and 2, a 7% reduction in manure from pit 3. For GHG emissions, MM, Penergetic G, and Sludge Away showed 2, 1, and 8% reduction in CO2 emissions, respectively, in manure from pit 1; MM and Penergetic had 11 and 12% generations in CO2 emissions emitted from pit 2, and Sludge Away showed 1% reduction; MM, Penergetic G, and Sludge Away showed 7, 4, and 4% generations in CO2 emissions from pit 3. All three products showed generations in CH4 emissions, except manure from pit 1 treated with Sludge Away with a 7% reduction. All three products showed reductions in all manure sources, excepted a 2% generation in manure from pit 3 treated with MM. Odor and VOCs shared a similar lack of trends like the first two Trials in which emissions were reduced in one or two types of manure but also generated in other types of manure sources.
Trial 4 (LLMO-SST)
One product, LLMO-SST, was tested in the last Trial. H2S emissions and selected VOCs were not detected in this Trial (Supplementary Figures 126–130). When we consider the manure to be replicated, there was no statistically significant %R found, as shown in Table 8. LLMO showed a 5% reduction in NH3 emission, a 3% reduction in CO2 emission, a 13% generation in CH4 emission, no impact on N2O emission, and a 1% reduction in odor concentration. For VOCs, we still observed the random pattern in which some gases were reduced, and other gases were generated.
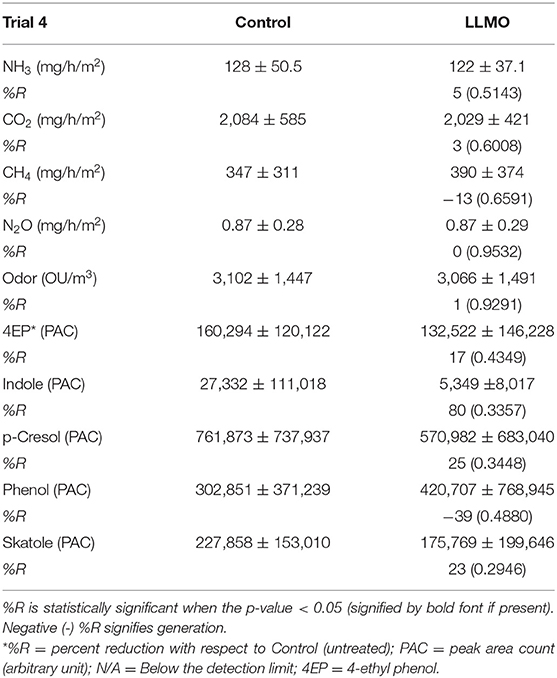
Table 8. Trial 4-comparison of averaged gaseous emissions from three manure sources (farms) with their standard deviations.
Table 9 below summarized the results analyzed by each manure source for Trial 4. For NH3 emissions, LLMO showed a 24% generation with a p-value of 0.0272 in manure from pit 1, but a 20% reduction with a p-value of 0.0101 in manure from pit 2. The rest of the detected gases still did not show any statistical significance, except for a 57% reduction with a p-value of 0.0381 for skatole emission from pit 3.
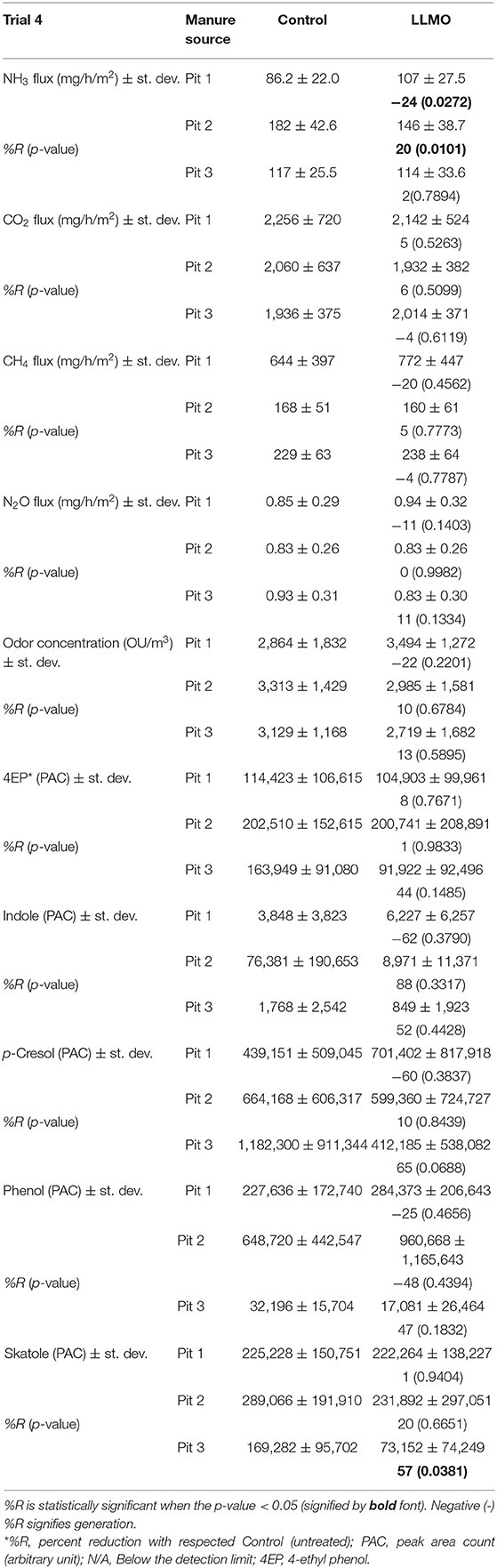
Table 9. Trial 4-comparison of gaseous emissions from each of the three manure sources (farms) with their standard deviations.
Discussion
The results of this pilot-scale study aimed to be fair in the presentation of results pertaining to each of the commercial products that were tested in a particular set of conditions. The presented results could help the farmers and the swine industry to find the detailed performance data of all the targeted gases for the particular tested product. The results also provided the important metric of the popular technology used by some U.S. farmers to mitigate gaseous emission for the environmental regulatory agencies and researchers.
The side-by-side comparison of the 12 manure additives tested in this study did not show that treatment with any product resulted in statistically significant and comprehensive reductions to emissions of targeted gases such as NH3, H2S, GHGs, VOCs, and odor. Very similar findings were reported on the pilot-scale study of manure additives conducted almost 20 years ago (Heber et al., 2001). Maurer et al. (2016) summarized the performance data for technologies to control gaseous emissions in which manure additives have shown inconsistent performance, and little or no data was available for testing on farm/field scales. The lack of consistent, significant, and comprehensive performance in mitigating gaseous emissions at lab-scale is often precluding the continuation of testing and scaling to the pilot- and farm-trials. This is also the major recommendation from this research, i.e., the results for the 12 manure additives tested in this study do not warrant scaling up tests and studies.
However, the experimental manure additives evaluated in recent years have shown the potential to mitigate the emissions from manure. Maurer et al. (2017b) showed up to 68% reduction of NH3 and 80–90% reduction on the various types of VOCs by using soybean peroxidase with calcium peroxide in a pilot-scale study. The same additive (soybean peroxidase + calcium peroxide) was found to be effectively mitigating NH3, H2S, and some targeted VOCs on the farm-scale study (Maurer et al., 2017d). In recent years of studies of using the various types of biochar as manure additives, there are many significant reductions of targeted gases reported. Biochar made from pine could mitigate NH3 but the generation of CH4 (Maurer et al., 2017c). Biochar made from corn stover and red oak could mitigate NH3 and some targeted VOCs (Meiirkhanuly et al., 2020b). Chen et al. (2020a) showed a significant reduction of H2S emissions during the manure agitation.
Manure additives, especially the ones with physical and chemical modes of treatment, still have the potential to comprehensively mitigate gaseous emissions from swine manure. However, the impact of these manure additives such as (biochar, soybean peroxide) still needed further research on larger scales and farms with different manure management systems. Most recently, research has shown that biochar-treated manure can have beneficial agronomic effects, as reported by Banik et al. (2020). There is an early indication that biochar-manure mixture has the potential to further improve the nitrogen and carbon cycling and sustainability of animal and crop production.
Conclusions
The following conclusions can be made based on the results of this research:
1. This pilot-scale study of 12 marketed manure additive products did not show a consistent, comprehensive, and overall statistically significant reduction for mitigation of gaseous emissions from swine manure for targeted gases including NH3, H2S, GHGs, VOCs, and odor.
2. The more detailed analysis based on separating each treated manure indicates large variability in performance ranging from mitigation to generation of targeted gaseous emissions.
3. Manure additives tested based on microbial mode action did not show consistent effects in mitigating emissions.
4. The manufacturer-prescribed dosages of products were not effective in pilot-scale testing that used manure from different swine farms and storage systems.
5. Based on the lack of mitigating effect of gaseous emissions in this pilot-scale study, testing of these 12 manure additives products on farms is not recommended.
Data Availability Statement
The original contributions presented in the study are included in the article/Supplementary Materials, further inquiries can be directed to the corresponding author.
Author Contributions
JK and DA: conceptualization, validation, and resources. BC, JK, and DA: methodology. BC: formal analysis and wrote—original draft preparation. BC, HM, ML, ZM, PL, JW, and CB: investigation. BC and JK: data curation. BC, JK, SO'B, and AB: wrote—review and editing. BC and ML: visualization. JK: supervision. JK, DP, and DA: project administration and funding acquisition. All authors contributed to the article and approved the submitted version.
Funding
This research was partially funded by the National Pork Board and the Indiana Pork Producers Association, grant NBP-17-158: Evaluation of current products for use in deep pit swine manure storage structures for mitigation of odors and reduction of NH3, H2S, and VOC emissions from stored swine manure (2018–2020, PI JK). In addition, this research was partially supported by the Iowa Agriculture and Home Economics Experiment Station, Ames, Iowa. Project no. IOW05556 (Future Challenges in Animal Production Systems: Seeking Solutions through Focused Facilitation) sponsored by Hatch Act and State of Iowa funds. The authors would like to thank the Ministry of Education and Science of the Republic of Kazakhstan for supporting Zhanibek Meiirkhanuly with an M.S. study scholarship via the Bolashak Program. The authors would also like to thank the Fulbright Foundation for funding the project titled Research on pollutants emission from Carbonized Refuse Derived Fuel into the environment, completed by AB at the Iowa State.
Conflict of Interest
The authors declare that the research was conducted in the absence of any commercial or financial relationships that could be construed as a potential conflict of interest.
Supplementary Material
The Supplementary Material for this article can be found online at: https://www.frontiersin.org/articles/10.3389/fenvs.2020.613646/full#supplementary-material
Detailed graphical comparisons of emissions for each targeted gas over 8 weeks of each Trial, illustrated with 130 figures (Supplementary Figures 1–130). Supplementary Tables 1–3 serve as a guide for finding results on a particular manure additive and targeted gas.
References
Akdeniz, N., Jacobson, L. D., Hetchler, B. P., Bereznicki, S. D., Heber, A. J., Koziel, J. A., et al. (2012a). Odor and odorous chemical emissions from animal buildings: part 2. Odor emissions. Trans. ASABE 55, 2335–2345. doi: 10.13031/2013.42495
Akdeniz, N., Jacobson, L. D., Hetchler, B. P., Bereznicki, S. D., Heber, A. J., Koziel, J. A., et al. (2012b). Odor and odorous chemical emissions from animal buildings: part 4–correlations between sensory and chemical concentrations. Trans. ASABE 55, 2347–2356. doi: 10.13031/2013.42496
Banik, C., Koziel, J., De, M., Bonds, D., Chen, B., Singh, A., et al. (2020). Soil nutrients and carbon dynamics in the presence of biochar-swine manure mixture under controlled leaching experiment using a midwestern USA soil. [Preprint] 2020090551. doi: 10.20944/preprints202009.0551.v2
Bereznicki, S. D., Heber, A. J., Akdeniz, N., Jacobson, L. D., Hetchler, B. P., Heathcote, K. Y., et al. (2012). Odor and odorous chemical emissions from animal buildings: Part 1. Project overview, collection methods, and quality control. Trans. ASABE 55, 2325–2334. doi: 10.13031/2013.42497
Cai, L., Koziel, J. A., Lo, Y., and Hoff, S. J. (2006). Characterization of volatile organic compounds and odorants associated with swine barn particulate matter using solid-phase microextraction and gas chromatography–mass spectrometry–olfactometry. J. Chromatogr. A 1102, 60–72, doi: 10.1016/j.chroma.2005.10.040
Cai, L., Koziel, J. A., Nguyen, A. T., Liang, Y., and Xin, H. (2007). Evaluation of zeolite for control of odorants emissions from simulated poultry manure storage. J. Environ. Quality 36, 184–193. doi: 10.2134/jeq2006.0052
Cai, L., Koziel, J. A., Zhang, S., Heber, A. J., Cortus, E. L., Parker, D. B., et al. (2015). Odor and odorous chemical emissions from animal buildings: part 3. Chemical emissions. Trans. ASABE 58, 1333–1347. doi: 10.13031/trans.58.11199
Chen, B., Koziel, J. A., Banik, C., Ma, H., Lee, M., Wi, J., et al. (2020b). Emissions from swine manure treated with current products for mitigation of odors and reduction of NH3, H2S, VOC, and GHG emissions. Data 5:54. doi: 10.3390/data5020054
Chen, B., Koziel, J. A., Bialowiec, A., Lee, M., Ma, H., Meiirkhanuly, Z., et al. (2020a). The impact of surficial biochar treatment on acute H2S emissions during swine manure agitation before pump-out: proof-of-the-concept. Catalysts 10:940. doi: 10.3390/catal10080940
Heber, A. J., Ni, J., Sutton, A. L., Patterson, J. A., Fakhoury, K., Kelly, D., et al. (2001). Laboratory testing of commercial manure additives for swine odor control (Final Report). Ames, IA: USDA-ARS-National Swine Research and Information Center.
Iowa State University Extension and Outreach (2020). Air Management Practices Assessment Tool Website. Available online at: https://www.extension.iastate.edu/ampat/manure-storage-and-handling (accessed on 28 August 2020).
Koziel, J. A., Cai, L., Wright, D., and Hoff, S. (2006). Solid phase microextraction as a novel air sampling technology for improved, GC-Olfactometry-based, assessment of livestock odors. J. Chromatogr. Sci. 44, 451–457. doi: 10.1093/chromsci/44.7.451
Maurer, D., Koziel, J. A., Harmon, J. D., Hoff, S. J., Rieck-Hinz, A. M., and Andersen, D. S. (2016). Summary of performance data for technologies to control gaseous, odor, and particulate emissions from livestock operations: air management practices assessment tool (AMPAT). Data Brief 7, 1413–1429. doi: 10.1016/j.dib.2016.03.070
Maurer, D. L., Koziel, J. A., and Bruning, K. (2017a). Field scale measurement of greenhouse gas emissions from land applied swine manure. Front. Environ. Sci. Eng. 11:1. doi: 10.1007/s11783-017-0915-9
Maurer, D. L., Koziel, J. A., Bruning, K., and Parker, D. B. (2017c). Pilot-scale testing of renewable biocatalyst for swine manure treatment and mitigation of odorous VOCs, ammonia and hydrogen sulfide emissions. Atmos. Environ. 150, 313–321. doi: 10.1016/j.atmosenv.2016.11.021
Maurer, D. L., Koziel, J. A., Bruning, K., and Parker, D. B. (2017d). Farm-scale testing of soybean peroxidase and calcium peroxide for surficial swine manure treatment and mitigation of odorous VOCs, ammonia and hydrogen sulfide emissions. Atmos. Environ. 166, 467–478. doi: 10.1016/j.atmosenv.2017.07.048
Maurer, D. L., Koziel, J. A., Kalus, K., Andersen, D. S., and Opalinski, S. (2017b). Pilot-scale testing of non-activated biochar for swine manure treatment and mitigation of ammonia, hydrogen sulfide, odorous volatile organic compounds (VOCs), and greenhouse gas emissions. Sustainability 9, 929. doi: 10.3390/su9060929
Meiirkhanuly, Z., Koziel, J. A., Bialowiec, A., Banik, C., and Brown, R. C. (2020a). The proof-of-the concept of biochar floating cover influence on swine manure pH: implications for mitigation of gaseous emissions from area sources. Front. Chem. 8:656. doi: 10.3389/fchem.2020.00656
Meiirkhanuly, Z., Koziel, J. A., Chen, B., Białowiec, A., Lee, M., Wi, J., et al. (2020b). Mitigation of gaseous emissions from swine manure with the surficial application of biochars. Atmosphere 11:1179. doi: 10.3390/atmos11111179
Parker, D. B., Koziel, J. A., Cai, L., Jacobson, L., Akdeniz, N., Bereznicki, S., et al. (2012). Odor and odorous chemical emissions from animal buildings: part 6 – odor activity value. Trans. ASABE 55, 2357–2368. doi: 10.13031/2013.42498
Keywords: odor mitigation, sustainable agriculture, air quality, gaseous emissions, environmental technologies, animal production systems, swine manure, waste management
Citation: Chen B, Koziel JA, Banik C, Ma H, Lee M, Wi J, Meiirkhanuly Z, O'Brien SC, Li P, Andersen DS, Białowiec A and Parker DB (2020) Mitigation of Odor, NH3, H2S, GHG, and VOC Emissions With Current Products for Use in Deep-Pit Swine Manure Storage Structures. Front. Environ. Sci. 8:613646. doi: 10.3389/fenvs.2020.613646
Received: 05 October 2020; Accepted: 02 December 2020;
Published: 23 December 2020.
Edited by:
Andrew Hursthouse, University of the West of Scotland, United KingdomReviewed by:
Michael Edward Deary, Northumbria University, United KingdomMukesh Kumar Awasthi, Northwest A&F University, China
Copyright © 2020 Chen, Koziel, Banik, Ma, Lee, Wi, Meiirkhanuly, O'Brien, Li, Andersen, Białowiec and Parker. This is an open-access article distributed under the terms of the Creative Commons Attribution License (CC BY). The use, distribution or reproduction in other forums is permitted, provided the original author(s) and the copyright owner(s) are credited and that the original publication in this journal is cited, in accordance with accepted academic practice. No use, distribution or reproduction is permitted which does not comply with these terms.
*Correspondence: Jacek A. Koziel, a296aWVsJiN4MDAwNDA7aWFzdGF0ZS5lZHU=