- Department of Earth Sciences, Center for Earth System Research and Sustainability, Universität Hamburg, Hamburg, Germany
The digestion of biogenic organic matter is an essential step of sample preparation within microplastic analyses. Organic residues hamper the separation of polymer particles especially within density separation or polymer identification via spectroscopic and staining methods. Therefore, a concise literature survey has been undertaken to identify the most commonly applied digestion protocols with a special focus on water and sediments samples. The selected protocols comprise different solutions, concentrations, and reaction temperatures. Within this study we tested acids (nitric acid and hydrochloric acid), bases (sodium hydroxide and potassium hydroxide), and oxidizing agents [hydrogen peroxide, sodium hypochlorite and Fenton's reagent (hydrogen peroxide 30% in combination with iron(II)sulfate 0.27%)] at different concentrations, temperature levels, and reaction times on their efficiency of biogenic organic matter destruction and the resistance of different synthetic polymers against the applied digestion protocols. Tests were carried out in three parallels on organic material (soft tissue—leaves, hard tissue—branches, and calcareous material—shells) and six polymers (low-density polyethylene, high-density polyethylene, polypropylene, polyamide, polystyrene, and polyethylene terephthalate) in two size categories. Before and after the application of different digestion protocols, the material was weighed in order to determine the degree of digestion efficiency and polymer resistance, respectively. The efficiency of organic matter destruction is highly variable. Calcareous shells showed no to very low reaction to oxidizing agents and bases, but were efficiently dissolved with both tested acids at all concentrations and at all temperatures. Soft and hard tissue were most efficiently destroyed by sodium hypochlorite. However, the other reagents can also have good effects, especially by increasing the temperature to 40–50°C. The additional temperature increase to 60–70°C showed a further but less effective improvement, compared to the initial temperature increase. The resistance of tested polymer types can be rated as good except for polyamide and polyethylene terephthalate. Increasing the concentrations and temperatures, however, results in accelerated degradation of all polymers. This is most evident for polyamide and polyethylene terephthalate, which show losses in weight between 15 and 100% when the digestion temperature is increased. This effect is most pronounced for polyamide in the presence of acids and for polyethylene terephthalate digested with bases. As a concluding recommendation the selection of the appropriate digestion method should be specifically tested within initial pre-tests to account for the specific composition of the sample matrix and the project objectives.
Introduction
The investigation of environmental pollution by synthetic polymers and its effects is a rapidly developing research discipline. However, especially considering the detection of small particles, the separation of plastic particles from the sample matrix still poses a major challenge. The isolation of plastic particles requires the removal of the natural sample matrix consisting of mineral and biogenic organic substances. The optimal digestion method for the respective samples eliminates the biogenic organic matter as much as possible while preserving the target particles of synthetic polymers. For this purpose chemical digestion with acids, bases and oxidizing agents and enzymes are applied.
A number of studies address a comparison of different digestion methods to optimize the efficiency of biogenic organic matter destruction (Nuelle et al., 2014; Cole et al., 2015; Dehaut et al., 2016; Enders et al., 2017; Karami et al., 2017; Herrera et al., 2018; Hurley et al., 2018; Munno et al., 2018; Prata et al., 2019; Duan et al., 2020).
The efficiency of biogenic organic matter destruction is matrix-dependent. For example, applying strong acids, such as nitric acid (HNO3) led to very good digestion results regarding biota tissue, mainly consisting of proteins, carbohydrates and fats (Nuelle et al., 2014; Lusher et al., 2017; Naidoo et al., 2017). Karami et al. (2017) confirmed this for HNO3 and hydrochloric acid (HCl) digestion of fish tissue. Alkaline solutions, such as potassium hydroxide (KOH) also provide good results concerning the digestion of biotic tissue (Foekema et al., 2013; Nuelle et al., 2014; Collard et al., 2015; Dehaut et al., 2016; Karami et al., 2017; Prata et al., 2019). Enders et al. (2017) found highest efficiencies in applying KOH with NaClO (1:1) to fish stomach samples. These protocols are likely to be effective to destroy adhering biofilms that also predominately are composed of polysaccharides, proteins and lipids. Their elimination also is of great relevance since they may influence, e.g., physical behavior within density separation of sediment samples (Rummel et al., 2017; Harrison et al., 2018). However, KOH or sodium hydroxide (NaOH) are less successful when applied to water and sediment samples, as the biogenic organic material in these matrices often originates from plant material (including leafs, woody debris, and algae) and/or contains parts of carapace and shells (Duan et al., 2020). Therefore, in addition to calcareous substances, cellulose, hemicellulose, lignin, tannin, humic substances, and chitin, which are difficult to digest, must be eliminated (Herrera et al., 2018; Möller et al., 2020). For these sample matrices, oxidizing agents, such as hydrogen peroxide (H2O2) are increasingly implemented, also as Fenton's reagent and less frequently in combination with sodium hypochlorite (NaClO) (Enders et al., 2017; Karami et al., 2017; Hengstmann et al., 2018; Duan et al., 2020). Oxidizing agents are therefore considered to be promising candidates for a most efficient digestion of a wide range of sample matrices.
Besides efficiently destroying biogenic organic matter, applied protocols need to leave synthetic polymers unaffected concerning their weight, volume, shape, and if required, color. Furthermore, it is possible that the chemical structure or the surface structure and morphology of the polymers is affected and changed by the digestion methods (Enders et al., 2017). In order to detect these changes, further investigations by, e.g., scanning electron microscopy (SEM) or detailed spectroscopic analyses implementing μRaman-spectroscopy or FTIR-spectroscopy are necessary. It has been reported that under the influence of acids, in particular pH-sensitive synthetic polymers are altered. When exposed to HNO3, the degradation or complete destruction of polyamide (PA) (Dehaut et al., 2016; Roch and Brinker, 2017; Duan et al., 2020), and a critical melting of polystyrene (PS) (Claessens et al., 2013) occurred. Concentrated HNO3 and HCl may also dissolve nylon and partially degraded polyethylene (PE), polypropylene (PP), polystyrene (PS), polyethylene terephthalate (PET), and polyvinyl chloride (PVC) (Karami et al., 2017; Naidoo et al., 2017). Catarino et al. (2017) further reported that melding of PET and PE particles and complete dissolution of nylon fibers even occurs when using HNO3 in lower concentrations of 35%. Especially the application of HNO3 in higher concentrations at high temperatures significantly affects synthetic polymers with complete dissolution of PE and PS (Avio et al., 2015). Furthermore, HNO3 can cause yellowing of polymers, such as PE, PP, PA, and PS (Dehaut et al., 2016).
Strong bases can also have an influence on synthetic polymers. According to Dehaut et al. (2016) KOH digestion resulted in degradation of cellulose acetate (CA) and caused a significant weight increase for PS and weight decrease for polycarbonate (PC) (Hurley et al., 2018). When temperatures higher than 50°C were additionally applied, PVC also degraded and furthermore, a color alteration of nylon occurred (Karami et al., 2017). Dehaut et al. (2016) and Hurley et al. (2018) tested the application of sodium hydroxide (NaOH) resulting in degradation of CA, PC, and PET.
The use of oxidants, such as H2O2 at room temperature has only a minor effect on synthetic polymer weight and size but can lead to discoloration. However, an increase in temperature to 70–100°C is reported to cause a significant loss in weight and size of PA (Hurley et al., 2018; Duan et al., 2020).
The results of these studies are extremely valuable. However, an objective assessment of the actual efficiency of biogenic matter destruction or the resistance of plastics to the various digestion solutions is masked by the use of pre-specified protocols. Different concentrations, temperatures, and application times are used and compared in order to optimize the pre-selected protocols.
For an objective evaluation of chemical digestion methods with a focus on water and sediment samples, the present study examines acids, bases, and oxidizing agents in different concentration ranges at three identical temperature levels using identical methods. Within extensive laboratory tests, a total number of 40 protocols were evaluated with regard to their efficiency of biogenic organic matter destruction and their influence on synthetic polymers. The aim of our study is to provide a toolbox to assist the selection of digestion methods, to minimize the previously described laboratory analytical challenges and to represent a step toward the standardization of laboratory methods.
Materials and Methods
Digestion Solutions and Protocols Tested
Within this study, we tested acids (HNO3 20 and 65%, HCl 10 and 37%), bases (NaOH 2.5, 5, and 10 M or 4.7, 9.4, and 18.8%, respectively, KOH 1 and 4 M or 2.6 and 10.6%, respectively), and oxidizing agents [H2O2 30 and 50%, NaClO ~7.5 and 10%, Fenton's reagent (H2O2 30% and iron(II)sulfate 0.27%)]. The digestion protocols were carried out at three different temperature levels (20, 40–50, and 60–70°C) and reaction times (8–24 h or 7 days). For protocols with increased temperature (40–50 or 60–70°C), the samples were heated for 8 h of the overall exposure time of 24 h. The 7 d experiments were performed with H2O2 (30 and 50% at room temperature) and KOH (2.6 and 10.6% at room temperature). All experiments were conducted in three parallels. An overview of all tested protocols is provided in Table 1.
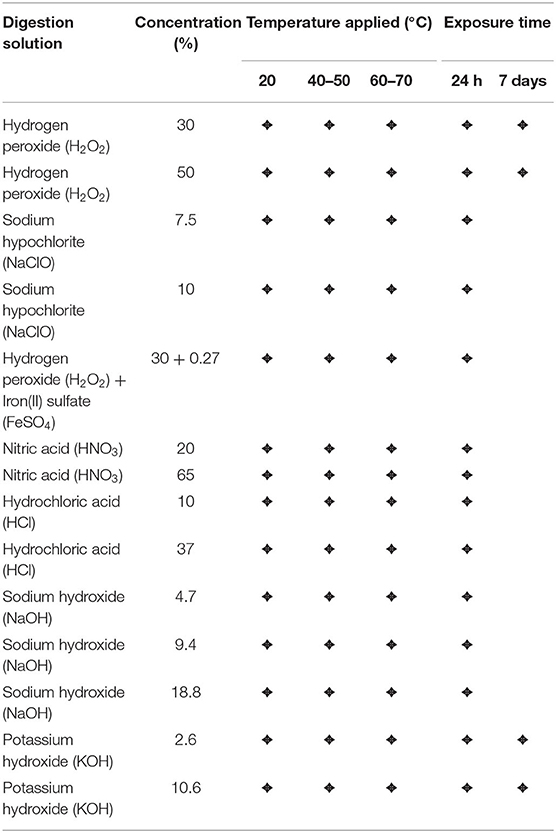
Table 1. List of the applied digestion solutions with corresponding concentrations, temperatures, and exposure times.
Origin and Processing of Synthetic Polymer Reference Particles
All protocols were tested for two size categories in three replicates, each. Virgin pellets of LDPE, HDPE, PP, PA, PS, and PET in nominal granule sizes of 1.0–5.0 mm were purchased from Goodfellow Cambridge Ltd.
For size category 1.0–5.0 mm, the virgin pellets were used as delivered by the manufacturer. Ten particles of each polymer per replicate were weighed to 0.01 mg accuracy (Cubis MSE324P, Sartorius) and transferred into glass test tubes. For size category 0.3–1.0 mm, the pellets were ground using a cutting mill (SM100, Retsch) and subsequent wet sieving in order to rinse off adhering smaller particles. About 150 mg of the respective polymers per replicate were weighed to 0.01 mg accuracy and transferred into glass test tubes. An aliquot of the respective digestion solution was slowly added in order to soak the complete sample material. The volumes applied depended on the incubation temperature (10 ml for room temperature and 20 ml for temperatures of 40–50 and 60–70°C). Test tubes dedicated to protocols with elevated temperatures were placed on sand baths (ST72, Harry Gestigkeit GmbH). For all temperatures, the exposure time was 24 h. Increased temperatures were applied for 8 h using a time-temperature controller. Subsequently, the samples of size category 1.0–5.0 mm were thoroughly rinsed through a stainless steel sieve with a mesh size of 1 mm (Retsch) with MilliQ water and were placed within covered glass petri dishes for subsequent drying at 40°C. Samples containing the smaller size fraction (0.3–1.0 mm) were filtered onto PC filters (Cyclopore, 5 μm, Whatman) by means of a stainless steel filtration device (3-fold Combisart, Sartorius) and were thoroughly rinsed with MilliQ water. Filter residues were immediately transferred into evaporating dishes with small amounts of MilliQ water that were placed into a drying oven at 40°C. After drying, all samples were evacuated within a desiccator until room temperature was achieved. Samples were then re-weighed to 0.01 mg accuracy. Based on the initial and final weight, the percentage change in weight of the respective synthetic polymer samples were calculated. For the comparability of results for large and small particles, the surface to volume ratio of plastic polymer particles were calculated based on mean dimensions and results were standardized to a surface to volume ratio of 2.
The polymers were photographed before and after the treatment to visually document possible changes in size, shape, or color (Supplementary Figures 1–5). Particles with visibly distinct changes in size, shape, or color were further investigated by μRaman spectroscopy (DXR2xi, ThermoFisher Scientific) to determine the potential effects of these visual alterations on the chemical structure of the polymer. For a spectroscopic analysis of possible changes in spectra by the respective application, single particles were placed on a microscope slide with tweezers for verification via μRaman-spectroscopy. Spectra were recorded using a 532 nm laser at 5–10 mW (25 μm confocal pinhole) and 100–10 Hz integrating 1,000 measurements. All spectra were smoothed (7 points) and the baseline was corrected automatically.
Material and Processing of Biogenic Organic Matter
Concerning the efficiency tests of digestion methods a focus was set on biogenic organic matter with high contents in cellulose and lignin typically present in sediment and water samples. Therefore, three different biogenic organic materials were tested: soft plant tissue (leaves, Fagus sylvatica), hard plant tissue (branches, Fagus sylvatica and Quercus robus) and calcareous shells (mussels, various marine species). Samples were shredded using a cutting mill (SM100, Retsch) for soft and hard tissue and a mortar for mussel shells. Subsequently, samples were sieved to gain pieces of 1.0–5.0 mm in longitudinal direction. Biogenic organic matter samples were dried at 40°C for 24 h and incubated in a desiccator until room temperature was achieved. Aliquots of about 150 mg were weighed to 0.01 mg accuracy (Sartorius, Cubis CPA 124S) and transferred to glass test tubes.
The further processing of biogenic samples was done in accordance to synthetic polymer tests described above. Chemical solutions were added and tubes were placed onto a sand bath when the protocol provides an increase in temperature. After the respective incubation time, samples were filtered and carefully rinsed into pre-weighed evaporating dishes with MilliQ water as it was done for the small plastic particles. After drying at 40°C, the loss of weight in biogenic matter was determined and calculated.
Statistical Analyses
Results were statistically analyzed using R statistics (R Core Team, 2017, Version 3.4.2) in an R Studio environment (RStudio Team, 2016, Inc., Version 1.1.383) and SPSS (IBM Version 26) concerning normality (Shapiro-Wilk), equality of variances (Levene's test), and analyses of variances (Mann-Whitney-U and Kruskall Wallis tests with subsequent Bonferroni correction and pairwise comparison, α = 0.05).
Results
Results on Resistance of Synthetic Polymer Particles
The results concerning the resistance of synthetic polymers against the applied reagents predominantly show good to very good results with weight changes < ±1% (see Figures 1A,B providing different y-scale dimensions). Figures 1A,B display mean weight differences with strong changes up to 100% standing for most destroying protocols. According to oxidizers, acids and bases the relevant protocols follow the order of different concentrations and then temperature levels.
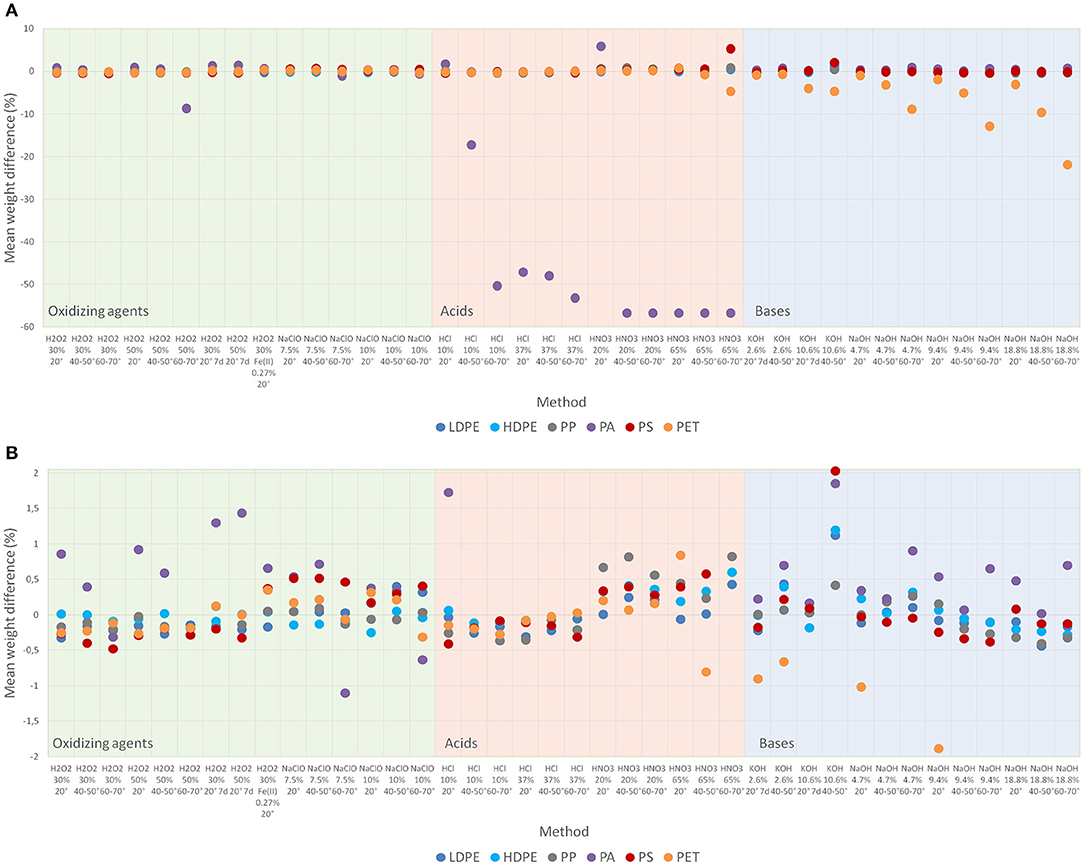
Figure 1. (A) Mean resistance of synthetic polymer particles against applied digestion protocols (expressed as mean difference in weight (%) of size standardized particles, full scale). (B) Mean resistance of synthetic polymer particles against applied digestion protocols (expressed as mean difference in weight (%) of size standardized particles, focused scale to values of ±2% difference in weight).
Oxidizing agents, in this case H2O2 and NaClO, marginally affected synthetic polymers except for PA. For PA, the application of H2O2 50% at temperatures between 60 and 70°C led to a mean decrease in weight of 8.7%. In contrast, other protocols using H2O2 led to an insignificant increase in weight of PA particles. This increase is weakened with rising temperatures but intensified for the 7 d period at room temperature. A slight decrease in weight for PA was further observed when applying NaClO (7.5%) at the temperature levels of 40–50 and 60–70°C (−1.1%, p = 0.020). PP might be slightly affected when higher concentrations of NaClO are provided at the highest temperature level of 60–70°C (p = 0.046, see Supplementary Table 1B).
Acidic digestion protocols showed the greatest influence again on PA. HCl was less aggressive than HNO3 at almost all concentrations and temperatures. Concerning HCl, the increase of concentration from 10 to 37% at 20°C led to degradation of PA. For low concentrations of HCl (10%), temperature is a major influencing factor. In this case, a temperature increase from 20 to 60–70°C leads to a reduction in weight for PA of up to 50.4% (p = 0.039).
The application of HNO3 at all concentrations and temperatures led to a severe destruction of PA except for concentrations of 20% at 20°C (mean + 5.9%). Even though PA was also dissolved in HNO3 at 20°C it re-precipitated when adding MilliQ-water and thus, could be reweighed (Supplementary Figure 2).
Furthermore, an insignificant increase in weight of PS was detected when applying HNO3 (65%) and raising the temperature level from 20 to 60–70°C (+0.4 vs. +5.3%). Visually, the generation of PS flakes (for particles of the size category 0.3–1.0 mm) was observed (Supplementary Figure 5). An opposite effect, with a significant loss in weight, is detected for PET when increasing the temperature level from 20 to 60–70°C (+0.8 vs. −4.7%, p = 0.002).
The protocols including the alkaline reagents NaOH and KOH resulted in good to very good resistances for all tested synthetic polymers except for PET. PET seems to be more affected by NaOH compared to KOH. Due to great variances, these differences are not significant, though. KOH shows an increasing loss in weight for PET with higher concentration (2.6 vs. 10.6%) at 40–50°C (−0.7 vs. −4.7%, p = 0.039). As for oxidizing agents, a slight increase in weight of PS particles is observed for alkaline protocols.
The spectroscopic examination of selected particles by μRaman-spectroscopy showed that for some of the particles distinct changes in the fingerprint region as well as in the C–H-stretch between the spectra before and after the treatment could be noticed. This was most notably recorded for PA but also PS and PET when HCl was applied at higher temperatures. Nevertheless, in all selected cases the polymer type was correctly identified (Supplementary Figure 6).
Results on Digestion Effectiveness of Biogenic Organic Matter
The effectiveness of digestion protocols for biogenic organic matter is highly variable and matrix dependent (Figure 2). Figure 2 displays mean weight differences with strong changes up to 100% standing for most effective protocols. According to oxidizers, acids and bases the relevant protocols follow the order of different concentrations and then temperature levels.
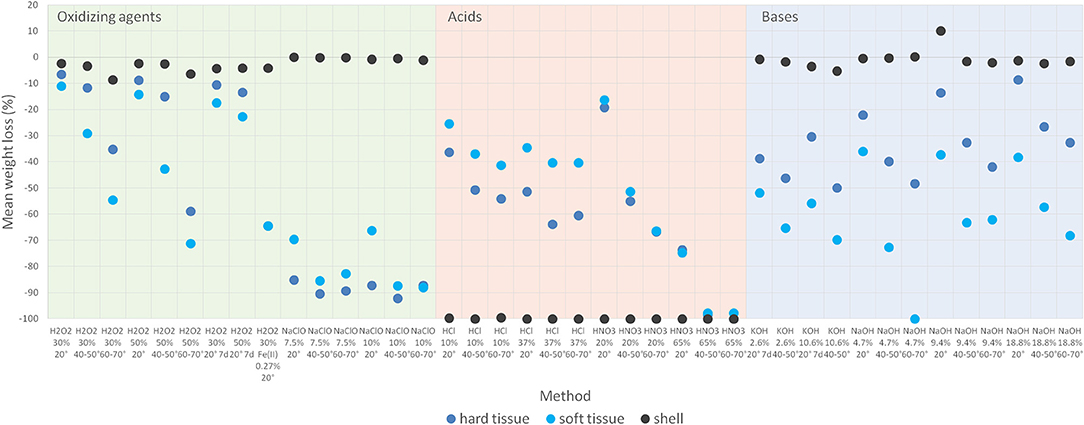
Figure 2. Efficiency of tested protocols concerning biogenic organic matter digestion given as mean loss in weight (%).
The application of H2O2 showed the highest efficiency of biogenic organic matter digestion in soft and hard tissue compared to shells. The digestion efficiency increased with rising concentrations from 30 to 50% and was enhanced even more with rising temperature from 20 to 60–70°C. Concerning soft tissue, this resulted in a maximum weight reduction of 71.1% (30%/20°C −11.0 vs. 50%/60–70°C −71.1%, p = 0.011). Similar effects were achieved for hard tissue with a mean weight decrease of 6.4% (30%/20°C) vs. 58.9% (50%/60–70°C, p = 0.036).
Adding Fenton's reagent also resulted in digestion of both soft and hard tissue of up to 64.4%. The elongation of the reaction time up to 7 d (20°C) resulted in only minor improvements of the digestion.
The application of NaClO provided even better results than H2O2 with loss in weight of up to 88.0% for soft tissue and 92.1% for hard tissue. The increase of the concentration from 7.5 to 10% did not result in a significant improvement. Increasing the temperature from 20 to 40–50°C led to a significantly better digestion result for soft tissue in NaClO (10%) (66.3 vs. 87.4%, p = 0.043). Further elevation of temperature did not cause any significant increase in the digestion of soft and hard tissue.
The calcareous material derived from shells was hardly digested by oxidizing agents. Only a slight increase of digestion efficiency with rising temperature can be observed for the H2O2 protocol.
The acid protocols with HCl and HNO3 were the only ones leading to satisfying results concerning digestion of calcareous material. Both reagents led to the dissolution with 99.5–100% loss in weight at all concentrations and applied temperatures. Concerning soft and hard tissue, the digestion efficiencies increase with rising concentrations and rising temperatures to maximum values of 74.7 and 73.5%, respectively. This effect is significant for soft tissue digested in HCl 10% when raising the temperature from 20 to 40–50°C (−25.3 vs. −41.2%, p = 0.043). Further increasing the temperature level does not lead to a significant enhancement in the digestion efficiency.
Only when applying HNO3 at 65% with elevated temperatures >40°C a nearly complete digestion of soft and hard tissue is achieved (97.8–100%).
Digestion protocols using alkaline solutions predominantly did not lead to sufficient results concerning biogenic organic matter destruction and were in a similar range as the protocols using acids except for calcareous material. The maximum values of weight reduction accounted for 49.8% (hard tissue, KOH 10.6%, 40–50°C) and 100% (soft tissue, NaOH, 4.7%, 60–70°C). In general, better results were achieved for both KOH and NaOH with higher temperature levels. Bases had no relevant effects on calcareous substances.
Discussion
Effects of Oxidizers on Synthetic Polymers and Biogenic Organic Matter
With regard to the resistance of polymers exposed to oxidizers, their influence on the tested synthetic polymers is low except for PA when exposed at higher reaction temperatures. The significant loss in weight for PA when exposed to H2O2 50% at 60–70°C is comparable to the findings of Hurley et al. (2018) applying a temperature of 70°C and describing a significant loss in weight (26.7%) and size (33.4%).
The application of H2O2 at all concentrations did not result in good digestion efficiencies of the tested biogenic organic material when applied at room temperature even with a prolonged reaction time of 7 days. The results could be distinctly improved by raising the temperature up to 70°C. This is in accordance with findings by Duan et al. (2020) who stated good digestion efficiencies of vegetal litter with stepwise addition of H2O2 and temperature increase to 100°C. Best digestion results on soft and hard tissue were obtained with NaClO with similar efficiencies for both tested concentrations. Again, an improvement of efficiencies was gained by increasing the temperature to 40–50% while no further progress was found for higher temperatures.
Effects of Acids on Synthetic Polymers and Biogenic Organic Matter
Based on synthetic polymer resistance, the digestion protocols applying acids were suitable at all concentrations and temperature levels except for PA. Digestion with HNO3 at different concentration levels (20 vs. 65%) even led to a significant degradation of PA (p = 0.037). As shown by Dehaut et al. (2016) and Duan et al. (2020) nitric acid may cause complete degradation, which is supported by our data, as well.
Furthermore, HCl only affected polymers when exposed to high concentrations and/or high temperatures. Acids in general have a larger influence on synthetic polymers compared to oxidizers being most pronounced for PA and to a lesser extent for PS and PET. This was also stated by other authors (Dehaut et al., 2016; Karami et al., 2017; Naidoo et al., 2017; Duan et al., 2020), which underlines these results. Other studies furthermore report the partial degradation of other synthetic polymers, such as PE, PP, and PVC by acids HCl and HNO3 (Karami et al., 2017; Naidoo et al., 2017). These findings are not confirmed within the present study due to differences in weight of < ±1% documented.
Effects of Bases on Synthetic Polymers and Biogenic Organic Matter
The application of alkaline protocols on the tested biogenic material had no or only very slight effects on calcareous material. The efficiencies on hard and soft tissues could be improved by applying elevated concentrations and temperatures. The protocols implementing bases did not achieve satisfying digestion efficiencies except for NaOH (4.7%) at the highest temperature level where an at least soft tissue was digested efficiently. Here, a maximum digestion efficiency of about 70% of soft tissue and about 50% of hard tissue could be obtained. This finding is underlined by the results of Duan et al. (2020) who digested vegetal litter with a mixture of NaOH 10 M and H2O2 at room temperature over 7 days.
NaOH was reported to cause degradation on synthetic polymers. Similar to the study of Hurley et al. (2018) an increase in weight of PS was detectable when applying KOH 10.6% at 40–50°C (Hurley et al., 2018: +12.1 vs. 2.0% this study). The strongest degradation effect of all bases was observed for PET when treated with NaOH. The degradation of mass and size of PET through the application of 10 M NaOH has also been demonstrated within other studies (Dehaut et al., 2016; Hurley et al., 2018).
The alteration of synthetic polymers by digestion chemicals can result not only in a decrease but also in an increase in weight. This is especially given for certain protocols and the polymers PA and PS. Pretests with alkaline protocols revealed that it is of utmost importance to carefully rinse the samples since residues of the respective salts might still be present. This can lead to an overestimation in weight. The present experiments considered this fact, thus, the increase in weight of especially PA and PS is more indicative concerning an alteration due to an initial degradation at the surface and thus an increase in volume (as was also demonstrated by Dehaut et al., 2016 and Hurley et al., 2018) and finally structural changes within the synthetic polymer. Furthermore, the increase in weight also might be a result from the relaxation of the polymer structure and entrapping of water molecules (Lulu et al., 2019).
Effects of Rising Concentration and Temperature Levels
Some of the results are very convincingly demonstrating that both concentration of the agent and temperature level applied are of utmost importance for polymer resistance.
The influence of rising concentrations on the digestion of biogenic organic matter is partly masked by influences due to applied temperatures. Tests on significant differences by concentrations resulted in several tendencies (see Supplementary Table 1A). Most significant differences occurred concerning the HNO3 digestion of hard tissue at temperatures between 60 and 70°C when raising the concentration from 20 to 65% (p = 0.046). The digestion of soft tissue is significantly improved when the concentration of NaOH is increased from 4.7 to 9.4% (p = 0.037).
Except for PS and PA, effects are detected concerning the alteration of synthetic polymers through increasing the concentration of the digestion solutions (see Supplementary Table 1B).
In general, increasing the temperature leads to a significant improvement of the digestion results for soft and hard tissue concerning almost all tested reagents except for KOH (Supplementary Table 2A). Acid and alkaline treatments especially in lower concentrations showed a significant influence on hard tissue and soft tissue only when rising the temperature level from 20 to 60–70°C. Concerning oxidative treatment the results with H2O2 50% reveal the same temperature related tendency. With NaClO a significant improvement is already achieved when increasing temperatures from 20 to 40–50°C. A further elevation of the temperature to the level of 60–70°C only leads to a minor improvement of the digestion result with NaClO.
Similarly, the resistance of synthetic polymers especially of PA, PS, and PET is affected by increased temperatures at all concentrations applied (Supplementary Table 2B). Significant effects predominantly occur when the temperature level is raised from 20 to 60–70°C. Only PS is affected at 40–50°C when treated with H2O2 (30%). For example, HCl application on PA reveals that the destruction of the polymer is affected to a comparable extent by increasing the concentration from 10 to 37% as by raising the temperature from 20 to 60–70°C at the 10% HCl solution.
Though, when implementing H2O2 or Fenton's reagent for the digestion of organic-rich samples high temperatures might occur without externally supply due to exothermic oxidative reactions (Munno et al., 2018; Wiggin and Holland, 2019). Based on the results, it is recommended not to exceed temperatures of 40 or 50°C. This can either be achieved in reducing the concentrations and/or by placing the sample beakers into a cooling water bath. For better performance, also the application in multiple doses might be considered.
The results of the present study are based on weight changes. Therefore, a potential weight-neutral chemical or biological transformation of the parent polymers through the application of chemical digestion remains undetected. Hence, a possible under- or overestimation of the resistance of the polymers to the applied digestion methods cannot be excluded.
Conclusions
Taking into account both the resistance of synthetic polymers and the digestion efficiency of biogenic organic matter, our findings reveal that best results are achieved by applying oxidative treatment with NaClO. Both tested concentrations of 7.5 and 10% with further activation by a moderate increase in temperature to 40–50°C are considered to be highly effective for the digestion of soft and hard tissue. For the destruction of calcareous materials oxidizers are not suitable.
Acid digestion led to partial or complete degradation of some synthetic polymers, such as PA, PET, and PS. Furthermore, the application of acids on biogenic organic material did not lead to sufficient digestion results. Consequently, concerning biogenic organic material within sediments and water samples the application of acid digestion is not recommended except for sample matrixes with high contents of calcareous substances. In this case, the application of HCl at a maximum concentration of 10% at a reaction temperature of 20°C can be an essential part of the digestion protocol especially since it does not show any distinct effect on any of the synthetic polymers either.
Based on the results, digestion protocols implementing alkaline protocols are considered to be less suitable for their application on sediment and water samples, either. These samples are mainly characterized by higher proportions of cellulose, hemicellulose and lignin of plant residues that are only less affected by alkaline digestion. Furthermore, especially PET is affected by NaOH and to a lesser extent—KOH. PET constitutes the major part of fibers in environmental samples and should thus not be excluded by implementing protocols causing a relevant degradation.
Each sample matrix is different even amongst sediments from different origins or water samples from different marine or freshwater environments. Thus, pre-tests with different digestion protocols are highly recommend to achieve best digestion results with simultaneous consideration of the best possible protection of the targeted plastic particles. This often requires a multi-stage process in which several digestion solutions are applied sequentially. As a rule, intermediate rinsing must be carried out between the respective dosages, which in turn involves the risk of increased contamination.
Nevertheless, in larger-scale studies, e.g., examining an entire ecosystem and different matrices, care should be taken to ensure the greatest possible methodological homogeneity even within sample digestion, so that comparable data sets provide a representative overall picture.
We understand our results as a modular system, which makes it possible to develop a suitable sample-specific protocol (Figure 3). The first step in sample preparation should be an evaluation of the composition of the biogenic organic matter. On this basis, the suitable digestion solution(s) can then be selected and the efficiency of the method can be optimized, e.g., by combining several digestion stages and/or slightly increasing the reaction temperature.
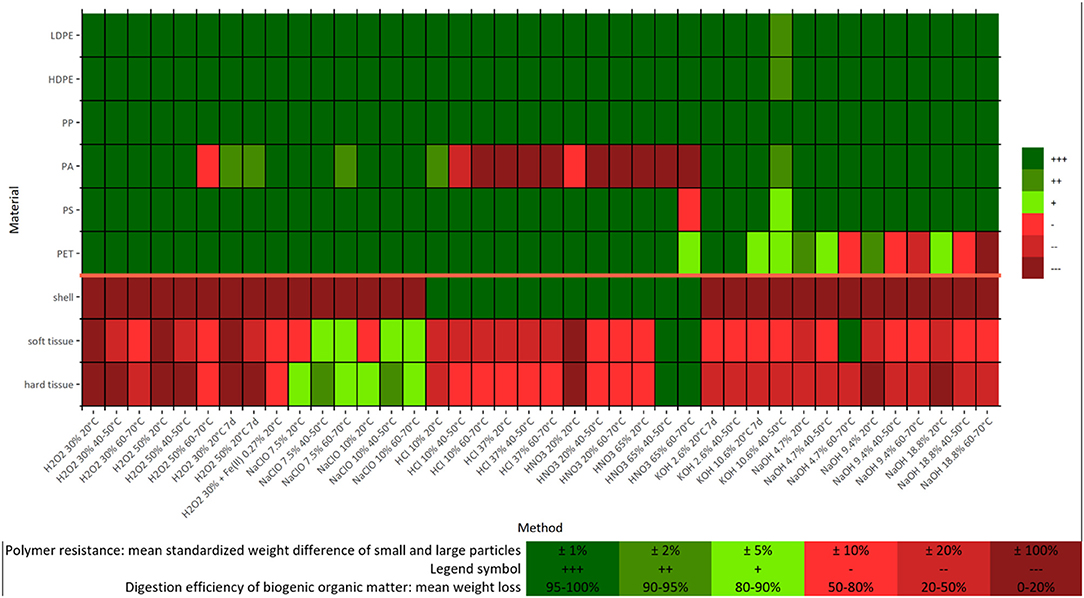
Figure 3. Evaluation of tested digestion protocols on synthetic polymer resistance (mean standardized efficiency of small and large particles) and digestion efficiency of biogenic organic matter.
Data Availability Statement
The original contributions presented in the study are included in the article/Supplementary Material, further inquiries can be directed to the corresponding author/s.
Author Contributions
FP and EF contributed equally to the investigation, conception of the experimental setup, conducting laboratory experiments, and equal contribution to the manuscript. FP calculated the results, standardized values, and developed the included figures. All authors contributed to the article and approved the submitted version.
Conflict of Interest
The authors declare that the research was conducted in the absence of any commercial or financial relationships that could be construed as a potential conflict of interest.
Acknowledgments
The authors would like to thank Philip John Mordecai for help on extensive lab experiments on biogenic organic matter samples and Elena Hengstmann and Matthias Tamminga for their useful comments on an earlier version of the manuscript.
Supplementary Material
The Supplementary Material for this article can be found online at: https://www.frontiersin.org/articles/10.3389/fenvs.2020.572424/full#supplementary-material
References
Avio, C. G., Gorbi, S., and Regoli, F. (2015). Experimental development of a new protocol for extraction and characterization of microplastics in fish tissues: first observations in commercial species from Adriatic Sea. Mar. Environ. Res. 111, 18–26. doi: 10.1016/j.marenvres.2015.06.014
Catarino, A. I., Thompson, R., Sanderson, W., and Henry, T. B. (2017). Development and optimization of a standard method for extraction of microplastics in mussels by enzyme digestion of soft tissues: standard method for microplastic extraction from mussels. Environ. Toxicol. Chem. 36, 947–951. doi: 10.1002/etc.3608
Claessens, M., Van Cauwenberghe, L., Vandegehuchte, M. B., and Janssen, C. R. (2013). New techniques for the detection of microplastics in sediments and field collected organisms. Mar. Pollut. Bull. 70, 227–233. doi: 10.1016/j.marpolbul.2013.03.009
Cole, M., Webb, H., Lindeque, P. K., Fileman, E. S., Halsband, C., and Galloway, T. S. (2015). Isolation of microplastics in biota-rich seawater samples and marine organisms. Sci. Rep. 4:4528. doi: 10.1038/srep04528
Collard, F., Gilbert, B., Eppe, G., Parmentier, E., and Das, K. (2015). Detection of anthropogenic particles in fish stomachs: an isolation method adapted to identification by Raman spectroscopy. Archiv. Environ. Contamin. Toxicol. 69, 331–339. doi: 10.1007/s00244-015-0221-0
Dehaut, A., Cassone, A.-L., Frère, L., Hermabessiere, L., Himber, C., Rinnert, E., et al. (2016). Microplastics in seafood: benchmark protocol for their extraction and characterization. Environ. Pollut. 215, 223–233. doi: 10.1016/j.envpol.2016.05.018
Duan, J., Han, J., Zhou, H., Lau, Y. L., An, W., Wei, P., et al. (2020). Development of a digestion method for determining microplastic pollution in vegetal-rich clayey mangrove sediments. Sci. Total Environ. 707:136030. doi: 10.1016/j.scitotenv.2019.136030
Enders, K., Lenz, R., Beer, S., and Stedmon, C. A. (2017). Extraction of microplastic from biota: recommended acidic digestion destroys common plastic polymers. ICES J. Mar. Sci. 74, 326–331. doi: 10.1093/icesjms/fsw173
Foekema, E. M., Gruijter, C. D., Mergia, M. T., van Franeker, J. A., Murk, A. T. J., and Koelmans, A. A. (2013). Plastic in North Sea Fish. Environ. Sci. Technol. 47, 8818–8824. doi: 10.1021/es400931b
Harrison, J. P., Hoellein, T. J., Sapp, M., Tagg, A. S., Ju-Nam, Y., and Ojeda, J. J. (2018). “Microplastic-associated biofilms: a comparison of freshwater and marine environments,” in Freshwater Microplastics, eds M. Wagner and S. Lambert (Cham: Springer), 181–201.
Hengstmann, E., Tamminga, M., vom Bruch, C., and Fischer, E. K. (2018). Microplastic in beach sediments of the Isle of Rügen (Baltic Sea)–implementing a novel glass elutriation column. Mar. Pollut. Bull. 126, 263–274. doi: 10.1016/j.marpolbul.2017.11.010
Herrera, A., Garrido-Amador, P., Martínez, I., Samper, M. D., López-Martínez, J., Gómez, M., et al. (2018). Novel methodology to isolate microplastics from vegetal-rich samples. Mar. Pollut. Bull. 129, 61–69. doi: 10.1016/j.marpolbul.2018.02.015
Hurley, R. R., Lusher, A. L., Olsen, M., and Nizzetto, L. (2018). Validation of a method for extracting microplastics from complex, organic-rich, environmental matrices. Environ. Sci. Technol. 52, 7409–7417. doi: 10.1021/acs.est.8b01517
Karami, A., Golieskardi, A., Choo, C. K., Romano, N., Ho, Y. B., and Salamatinia, B. (2017). A high-performance protocol for extraction of microplastics in fish. Sci. Total Environ. 578, 485–494. doi: 10.1016/j.scitotenv.2016.10.213
Lulu, L., Junhao, Q., Zihua, Y., Daihuan, C., Chunxia, Z., Pengzhi, H., et al. (2019). A simple method for detecting and quantifying microplastics utilizing fluorescent dyes–Safranine T, fluorescein isophosphate, Nile red based on thermal expansion and contraction property. Environ. Pollut. 255:113283, doi: 10.1016/j.envpol.2019.113283
Lusher, A. L., Welden, N. A., Sobral, P., and Cole, M. (2017). Sampling, isolating and identifying microplastics ingested by fish and invertebrates. Anal. Methods 9, 1346–1360. doi: 10.1039/C6AY02415G
Möller, J. N., Löder, G. J., and Laforsch, C. (2020). Finding microplastics in soils: a review of analytical methods. Environ. Sci. Technol. 54, 2078–2090. doi: 10.1021/acs.est.9b04618
Munno, K., Helm, P. A., Jackson, D. A., Rochman, C., and Sims, A. (2018). Impacts of temperature and selected chemical digestion methods on microplastic particles. Environ. Toxicol. Chem. 37, 91–98. doi: 10.1002/etc.3935
Naidoo, T., Goordiyal, K., and Glassom, D. (2017). Are nitric acid (HNO3) digestions efficient in isolating microplastics from juvenile fish? Water Air Soil Pollut. 228:470. doi: 10.1007/s11270-017-3654-4
Nuelle, M. T., Dekiff, J. H., Remy, D., and Fries, E. (2014). A new analytical approach for monitoring microplastics in marine sediments. Environ. Pollut. 184, 161–169. doi: 10.1016/j.envpol.2013.07.027
Prata, J. C., da Costa, J. P., Duarte, A. C., and Rocha-Santos, T. (2019). Methods for sampling and detection of microplastics in water and sediment: a critical review. Trends Anal. Chem. 110, 150–159. doi: 10.1016/j.trac.2018.10.029
R Core Team (2017). R: A Language and Environment for Statistical Computing. Available online at: https://www.R-project.org/
Roch, S., and Brinker, A. (2017). Rapid and efficient method for the detection of microplastic in the gastrointestinal tract of fishes. Environ. Sci. Technol. 51, 4522–4530. doi: 10.1021/acs.est.7b00364
RStudio Team (2016). RStudio: Integrated Development for R. Boston, MA: RStudio, PBC. Available online at: http://www.rstudio.com/
Rummel, C. D., Jahnke, A., Gorokhova, E., Kühnel, D., and Schmitt-Jansen, M. (2017). Impacts of biofilm formation on the fate and potential effects of microplastic in the aquatic environment. Environ. Sci. Technol. Lett. 4, 258–267. doi: 10.1021/acs.estlett.7b00164
Wiggin, K. J., and Holland, E. B. (2019). Validation and application of cost and time effective methods for the detection of 3-500 μm sized microplastics in the urban marine and estuarine environments surrounding Long Beach, California. Mar. Pollut. Bullet. 143, 152–162. doi: 10.1016/j.marpolbul.2019.03.060
Keywords: microplastics, sample purification, synthetic polymer resistance, digestion efficiency, biogenic organic matter, protocol evaluation
Citation: Pfeiffer F and Fischer EK (2020) Various Digestion Protocols Within Microplastic Sample Processing—Evaluating the Resistance of Different Synthetic Polymers and the Efficiency of Biogenic Organic Matter Destruction. Front. Environ. Sci. 8:572424. doi: 10.3389/fenvs.2020.572424
Received: 14 June 2020; Accepted: 30 November 2020;
Published: 23 December 2020.
Edited by:
Andrew Turner, University of Plymouth, United KingdomReviewed by:
Dietmar Schlosser, Helmholtz Centre for Environmental Research (UFZ), GermanyJoana Correia Prata, University of Aveiro, Portugal
Copyright © 2020 Pfeiffer and Fischer. This is an open-access article distributed under the terms of the Creative Commons Attribution License (CC BY). The use, distribution or reproduction in other forums is permitted, provided the original author(s) and the copyright owner(s) are credited and that the original publication in this journal is cited, in accordance with accepted academic practice. No use, distribution or reproduction is permitted which does not comply with these terms.
*Correspondence: Elke Kerstin Fischer, ZWxrZS5maXNjaGVyQHVuaS1oYW1idXJnLmRl