- 1Department of Energy and Technology, Swedish University of Agricultural Sciences, Uppsala, Sweden
- 2Sanitation360 AB, Romakloster, Sweden
- 3Tampere University of Applied Sciences TAMK, Tampere, Finland
- 4Finnish Environment Institute SYKE, Helsinki, Finland
Alkaline dehydration can treat human urine to produce a dry and nutrient-rich fertilizer. To evaluate the technology at pilot-scale, we built a prototype with capacity to treat 30 L urine d–1 and field tested it for the first time at a military training camp in Finland. We operated the system for 3 months and monitored the recovery of nutrients, end-product composition, physicochemical properties and energy consumption. Results revealed that the system received less urine than anticipated, but achieved high dehydration rates (30–40 L d–1m–2), recovered 30 ± 6% N, and yielded end-products with 1.4% N, 0.9% P, and 8.3% K. However, we demonstrated that the system had potential to recover nearly 70% N and produce fertilizers containing 13.2% N, 2.3% P, and 6.0% K, if it was operated at the design capacity. The energy demand for dehydrating urine was not optimized, but we suggested several ways of reducing it. We also discussed concerns surrounding non-essential heavy metals, salts, and micropollutants, and how they can be overcome to safely recycle urine. Lastly, we pointed out the research gaps that need to be addressed before the technology can be implemented at larger scale.
Introduction
In the field of wastewater management, the idea of separately collecting and treating different fractions of wastewater at the point where it is produced is rather innovative (Larsen et al., 2013). A source-separating sanitation system treats various sub-streams of domestic wastewater according to their composition (Friedler et al., 2013). Such an approach allows resources present in wastewater to be recovered for reuse or recycling (e.g., plant-essential nutrients, water and heat). It can also prevent the dispersion of potentially harmful substances in the environment by removing them as close as possible to the source (e.g., micropollutants).
Although the idea of source separation has been around for a while, it has struggled to move from concept to practice (McConville et al., 2017). One of the hurdles to its implementation lies in developing technologies to recycle human urine, wastewater’s most nutrient-rich fraction (Winker et al., 2009). Several research groups across the world have been working on this niche topic and have investigated different options for on-site urine treatment, many of which are reviewed in Harder et al. (2019). At the Swedish University of Agricultural Sciences we have opted for an alternative approach, one where fresh urine is dehydrated to concentrate nutrients within an alkaline medium, resulting in the production of a dry fertilizer. This approach, which we call alkaline urine dehydration, has been studied extensively in the laboratory (Dutta and Vinneras, 2016; Senecal and Vinneras, 2017; Senecal et al., 2018; Simha et al., 2018, 2020a).
In early 2019, we built a pilot-scale dry sanitation system with capacity to treat 30 L urine d–1. The system was designed to add fresh human urine continuously to a mixture of wood ash and calcium hydroxide (to prevent enzymatic ureolysis) and to concentrate the urine by hot air convective dehydration. It was operated and monitored for a period of 3 months, the results of which are summarized in this paper. To assess whether alkaline dehydration is a suitable on-site urine treatment technology, we focused on the following aspects – (i) the recovery of nutrients, particularly nitrogen; (ii) the elemental composition of the end-product; (iii) the concentrations of micropollutants and heavy metals; and, (iv) the energy demand for dehydrating urine. We also highlighted aspects that need further research, before the technology can be practically implemented.
Piloting Context
The pilot-scale study was carried out at a Finnish Army training site (Camp Mauri) belonging to the Pori Brigade, which is garrisoned in Säkylä, Finland. The brigade trains more than 4000 conscripts every year and supports voluntary training exercises and courses for the Finnish Defence Forces’ reserves. A lot of the drills take place in forest terrain and specifically designed sites at the garrison area. Camp Mauri represents the latter and is used by people undergoing training and thus, it needs to provide adequate sanitation facilities. The camps normally have permanent toilets but may temporarily rent field toilets such as those used at events or use disposable box toilets if they are located in areas that are hard to access. However, due to the 2016 ban in Finland on the landfilling of biodegradable waste, sewage from the camps needs to be collected and transported to a nearby wastewater treatment plant (Ministry of the Environment, 2016). One way to reduce the need for sewage transportation from off-the-grid sites is to concentrate the urine at the point of collection (Simha et al., 2020b). The present study explored this possibility by implementing urine source-separation and on-site treatment with alkaline dehydration.
Materials and Methods
Alkaline Media
For preparing the dehydration media, we used a mixture of wood ash and calcium hydroxide. The ash was collected from a cattle farm burning wood chips for bioenergy production (Lövsta Kött AB, Sweden) and pre-treated in a furnace (LH30/12, Nabertherm GmbH, Germany) for 6 h at 550°C, as described in Simha et al. (2018). Calcium hydroxide (Ca(OH)2) was supplied by Nordkalk Corporation, Sweden and used without any pre-treatment. The media contained 18.75% Ca(OH)2 and 81.25% pre-treated wood ash by weight.
Pilot Design and Setup
An on-site urine collection and alkaline dehydration system was developed and installed at a training camp of the Pori Brigade, an Army Unit of the Finnish Defence Forces located in Säkylä, Finland. The pilot-scale setup (Figure 1) comprised of a modified portable dry sanitation system (Biomaja Nordic Oy, Finland), an alkaline urine dehydrator and an industrial dehumidifier (DSC-70ES, Woods AB, Sweden).
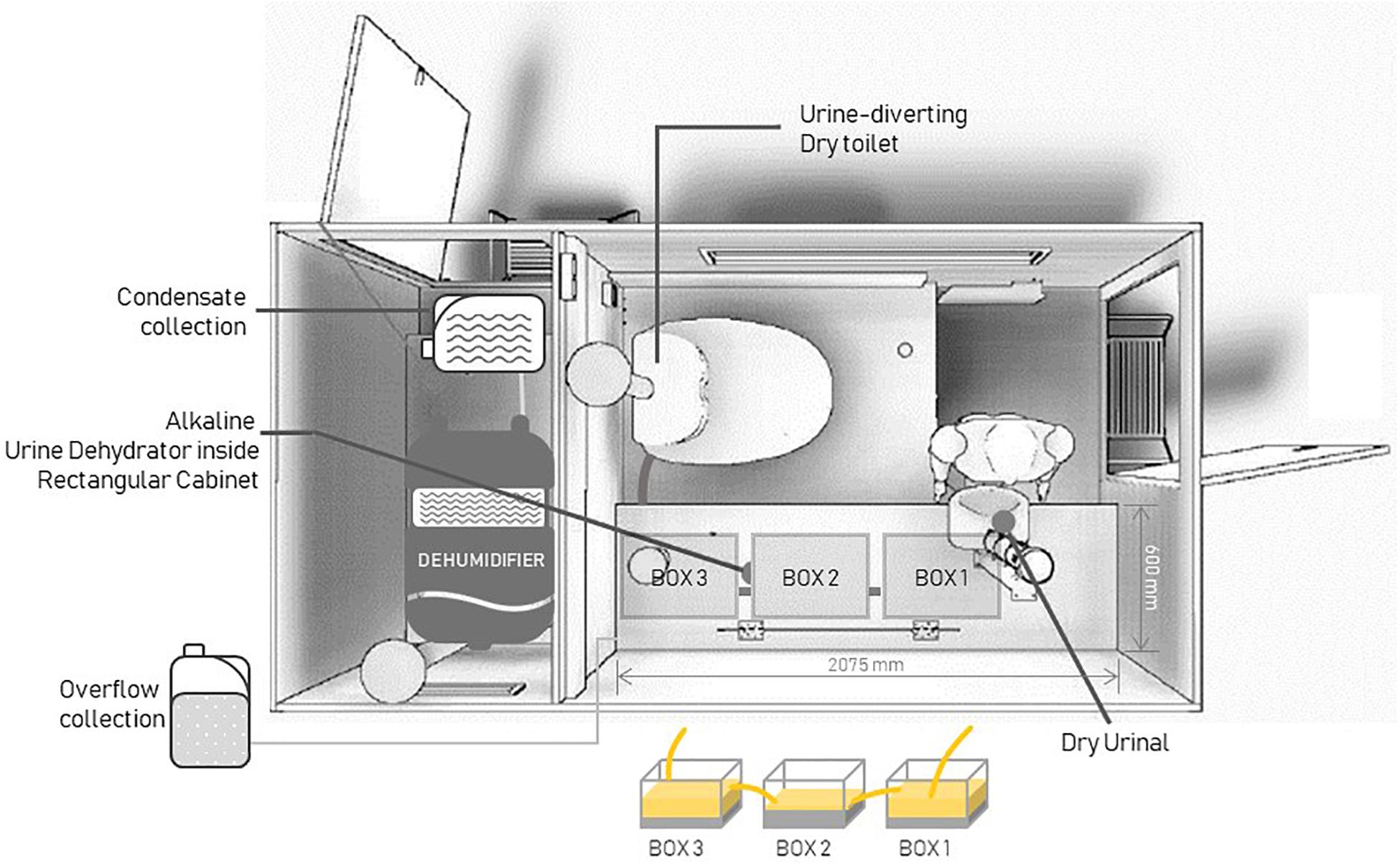
Figure 1. Pilot-scale setup for treating urine collected from the dry urinal and urine-diverting toilet by alkaline dehydration. Urine was treated in interconnected drying boxes (Box 1–Box 3) that were placed inside a rectangular cabinet and ventilated using a dehumidifier. Figure inset illustrates flow of urine between the boxes. Image modified with permission from Biomaja Nordic Oy, Finland.
The dry sanitation system comprised of two rooms. The larger room (2075 × 1600 × 2200 mm) had a dry urinal and a dry toilet (Biolan Oy, Finland) that was retrofitted using a Privy 501 kit (Separett AB, Sweden) to source-separate urine. The dehumidifier was placed in the smaller room (1000 × 1600 × 2200 mm) and provided airflow (450 m3 h–1 according to the manufacturer) throughout the system. The power rating of the dehumidifier was 630 W day–1 when operating at 20°C and 70% relative humidity.
A rectangular cabinet (2075 × 600 × 550 mm) located below the urinal that usually contains a urine collection tank was repurposed as an on-site urine dehydrator. In this cabinet, three polypropylene boxes (580 × 450 × 300 mm; Klämtare, IKEA of Sweden) were placed in a series configuration and interconnected with short (<200 mm) polypropylene pipes (Ø 50 mm). At the start of the treatment, all the boxes contained the drying media, a mixture of wood ash and Ca(OH)2. The boxes were covered with acrylic reinforced lids and each lid housed two heater fans (40–137, Biltema, Sweden) that provided air flow directly onto the surface of the drying media. The fans were fitted with a ceramic heating element and operated on two different settings that had a power rating of either 700 W or 1200 W.
A short pipe (<100 mm) connected Box 1 to the urinal while Box 3 was connected to the diverting toilet with a flexible hose (<500 mm). Urine entered Box 2 only when either or both the other boxes overflowed. It was important that the drying boxes were placed close to the point of urine collection since biofilms usually present in the piping network of larger collection systems can degrade urea into ammonia before the urine is alkalized to inhibit ureolysis. A 25 L jerry can containing 1 kg wood ash was placed outside the system and connected to the Box 3 to collect urine in case of a system overflow. For airflow, holes (Ø 80 mm) were drilled into the sides of the rectangular cabinet – the first served as the ambient air inlet to the dehydrator while the second interconnected the dry sanitation system’s two rooms and thus, the dehydrator with the dehumidifier. The dehumidifier condensate, if any, was pumped into a 25 L jerry can.
Pilot Operation
The pilot-scale system was designed to dry fresh urine collected from approximately 100 toilet users every day (or a maximum of 30 L urine day–1), for a period of 3 months between March and May 2019. The piloting was divided into three 1-month treatment periods. At the start of a treatment period, 6.5 kg wood ash and 1.5 kg Ca(OH)2 were added to each of the dehydrator’s three boxes, thoroughly mixed, and wetted with approximately 20 kg water box–1 (to prevent air suspension of the media).
The system was then switched on and allowed to dry out the water (around 1–2 days), after which the pilot operation was handed over to the Construction Establishment of Finnish Defence Administration. Over the month, the system was used by military personnel training at the camp site. At the end of the treatment, the system was switched off, the drying boxes were collected, emptied, and the end-product was sampled.
Monitoring and Analysis
Samples were taken for the drying media, the end-products, fresh urine as well as the dehumidifier condensate. Fresh urine could only be collected once during the pilot since it was not certain when the military personnel were at the site and using the sanitation system. The composition of the urine is shown in Table 1. The dehumidifier condensate was sampled if it was available during the site visits.
For sampling the end-product after every treatment period, the drying boxes were detached from the dehydrator and their contents were thoroughly mixed. For each box, composite samples were collected by blending grab samples taken from at least five random locations in the box. At least three composite samples were taken per box for every treatment period.
For all the collected samples, we analyzed for Total Solids (TS), Volatile Solids (VS), pH1:5 and EC1:5 as described in Simha et al. (2020a). We also analyzed for the concentrations of different elements and micropollutants – in total, 19 elements, 3 anions, 126 pharmaceuticals and 8 hormones were analyzed. The methods used for the various analyses are described in the Supplementary Information Tables S1, S2.
For monitoring air temperature and relative humidity in the system, data loggers (set to 5 min intervals) with inbuilt sensors (Tinytag Ultra 2 TGU-4500, Intab, United Kingdom) were placed at seven different positions in the system – ambient, dehydrator air inlet, dehumidifier inlet and outlet, and inside the three drying boxes (Supplementary Information, Supplementary Figure S1). The electricity consumption of the heater fans and the dehumidifier was monitored using plug-in meters (EMT707CTL, Clas Ohlson, United Kingdom).
Ethics Review
The ethics review of this study was carried out by the Ethics Committee of the Tampere Region, which handles the ethical reviews of proposed non-medical research. It operates under the guidelines issued by the Finnish National Board on Research Integrity (TENK). According to the review issued on 18 February 2019 (6/2019), the present study did not include ethical issues that would prevent its implementation as planned in the research proposal.
Calculations
Treating urine by alkaline dehydration reduced its mass, the extent of which was calculated using Eq. 1.
where mass.cfWB is the mass concentration factor on wet basis and mmedia, murine, and mend–product are the weight of the drying media at the start of the treatment, the total urine added during the treatment, and the end-product, respectively.
To estimate the total urine inflow to the system (murine), we performed a mass balance for P since there is complete recovery of P from urine during alkaline dehydration (Simha et al., 2020a). We calculated urine’s contribution of P (mP, urine) to the total P recovered in the end-product (mP,end–product) using Eq. 2, where mP, media is the P content of the drying media at the start of the treatment.
The P content of the sampled urine (CP,urine) was measured as described in the earlier section. We also estimated the N content (Eq. 4) and P content (Eq. 5) of urine, as suggested by Jönsson and Vinnerås (2004). The data for total (mtotal protein) and vegetal protein (mvegetal protein) consumption of the system users was collected from the local canteen (See Supplementary Information, Supplementary Table S3) and the latest statistics for food supplied in Finland (FAO, 2017).
Following Simha et al. (2020a), we used Eq. 6 to determine the recovery of N in the form of urea:
where, i is the time period, urea.add and urea.rec are the amounts of urea added and recovered, respectively. The half-life (h1/2) of urea at different temperatures was estimated as: 1.352×106×e−(0.1257T). We assumed that, of all the N excreted in urine, only urea-N (85% of the total N) can be recovered by alkaline dehydration, while the remaining is lost.
Statistics
The data was tested for homogeneity of variances using the F-test and the Shapiro–Wilk test for normality. To identify significant differences between the drying boxes, either unpaired two-sample Student’s t-test (for homoscedastic data) or Welch’s t-test (for heteroscedastic data) was performed. The level of significance was set at p < 0.05. One-way analysis of variance was carried out to identify statistically significant differences in the urine treatment across the different treatment periods. When a significant difference was found, Tukey’s Honest Significant Difference (HSD) test at 95% confidence level was done.
Results
Mass Balance
The design capacity of the pilot was 900 L month–1 but the volume of urine it received was considerably less. Based on the mass balance of P (Eq. 2, 3) and the analysis of the sampled urine (CP,urine = 0.64 gPL−1), the incoming urine volume was estimated to be approximately 35, 62, and 90 L in March, April, and May, respectively (Supplementary Information, Supplementary Figure S2). However, if the composition of urine is estimated using the Jönsson and Vinnerås (2004) approach as 11.4 gNL−1 and 1.12 gPL−1, the urine volume treated by the pilot would have been 20, 25, and 51 L in March, April, and May, respectively. The three boxes of the alkaline dehydrator also received different amounts of urine – no urine entered Box 2 throughout the pilot testing, while Box 1 received 46 ± 22 L month–1 and Box 3 received 16 ± 7 L month–1.
The end-product from Box 1 weighed 13.3 ± 3.7 kg, while that from Box 3 weighed 10.5 ± 2 kg. The mass concentration factor revealed that the end-products weighed 3–5 times less than the added urine and drying substrate. The dehumidifier condensate was available only at the end of March and May, where the amounts collected were 2.4 and 22.2 kg, respectively.
Nitrogen Recovery
Based on the estimated urine volumes, the mass balance revealed that the recovery of N in the end-product was 29, 31 and 38% for March, April and May (Supplementary Information, Supplementary Figure S2). The recovery was lower if the N content of urine was estimated using the Jönsson and Vinnerås (2004) method. The expected recovery of N was 66%, while the expected urea-N recovery at this temperature is 78% (Eq. 6). This was calculated using the half-life of urea assuming a constant drying temperature of 60°C.
The two drying boxes received different amounts of urine and recovered different amounts of N (34 ± 5% in Box 1 and 27 ± 4% in Box 3), but the differences were not statistically significant (p > 0.05). For Box 1, the recovery of N was significantly different over the three treatment periods, yet this was not the case for Box 3.
The dehumidifier condensate also captured N – the concentration of NH4-N in the condensate was 194 ± 13 mgNL−1 and the total-N concentration was 511 ± 132 mgNL−1. However, in the calculations for overall N recovery of the pilot system, the N captured in the condensate was disregarded.
End-Product Composition
The N-P-K composition (as% of TS) of the end-products collected in March, April and May was 0.5–0.7–6.1, 0.8–0.8–6.8, and 1.4–0.8–7.4, respectively (Table 2). The maximum N content was 2.1%, for the end-product collected from Box 1 at the end of May. On average, the end-products contained 17% Ca, 2.8% Mg, 1% Mn and 0.4% S. The average Na + and Cl− concentrations were 0.9 and 1.3%, respectively. Heavy metals Cu and Zn were also detected – 1946 ± 320 and 3844 ± 523 , respectively. The average Cd content of the end-product was 529 ± 26 .
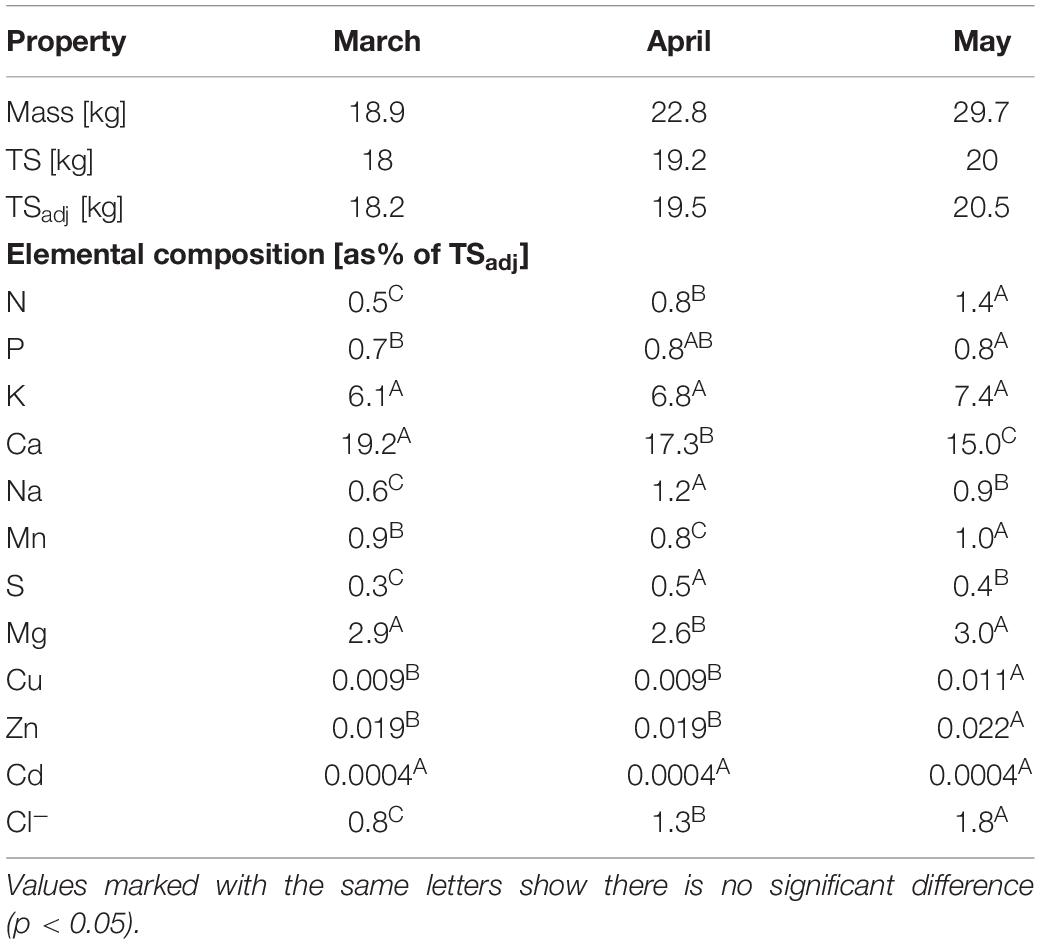
Table 2. The mass, total solids (TS), total solids adjusted for loss of urea (TSadj) and elemental composition of the end-products collected in the three treatment periods.
Micropollutants
The concentrations of pharmaceuticals and hormones detected in fresh urine and the end-product collected from the second treatment period (April) is presented in Table 3. In the untreated urine, only 8 out of the 126 monitored pharmaceuticals and 4 out of the 8 hormones were detected. This included the following therapeutic groups: non-steroidal anti-inflammatory drugs (ibuprofen, naproxen), β-blockers (bisoprolol, metoprolol), analgesics (acetaminophen), CNS stimulants (caffeine), glucocorticosteroids (hydrocortisone), a decongestant (xylometazoline) and two antibiotics (tetracycline, doxycycline). After alkaline dehydration treatment, none of the hormones were detected in the solid end-product. However, the pharmaceuticals bisoprolol, metoprolol, ibuprofen, caffeine, xylometazoline and naproxen were detected. The pharmaceuticals partitioned primarily to the solid phase, as only caffeine (24 μgL−1) was detected in the dehumidifier condensate.
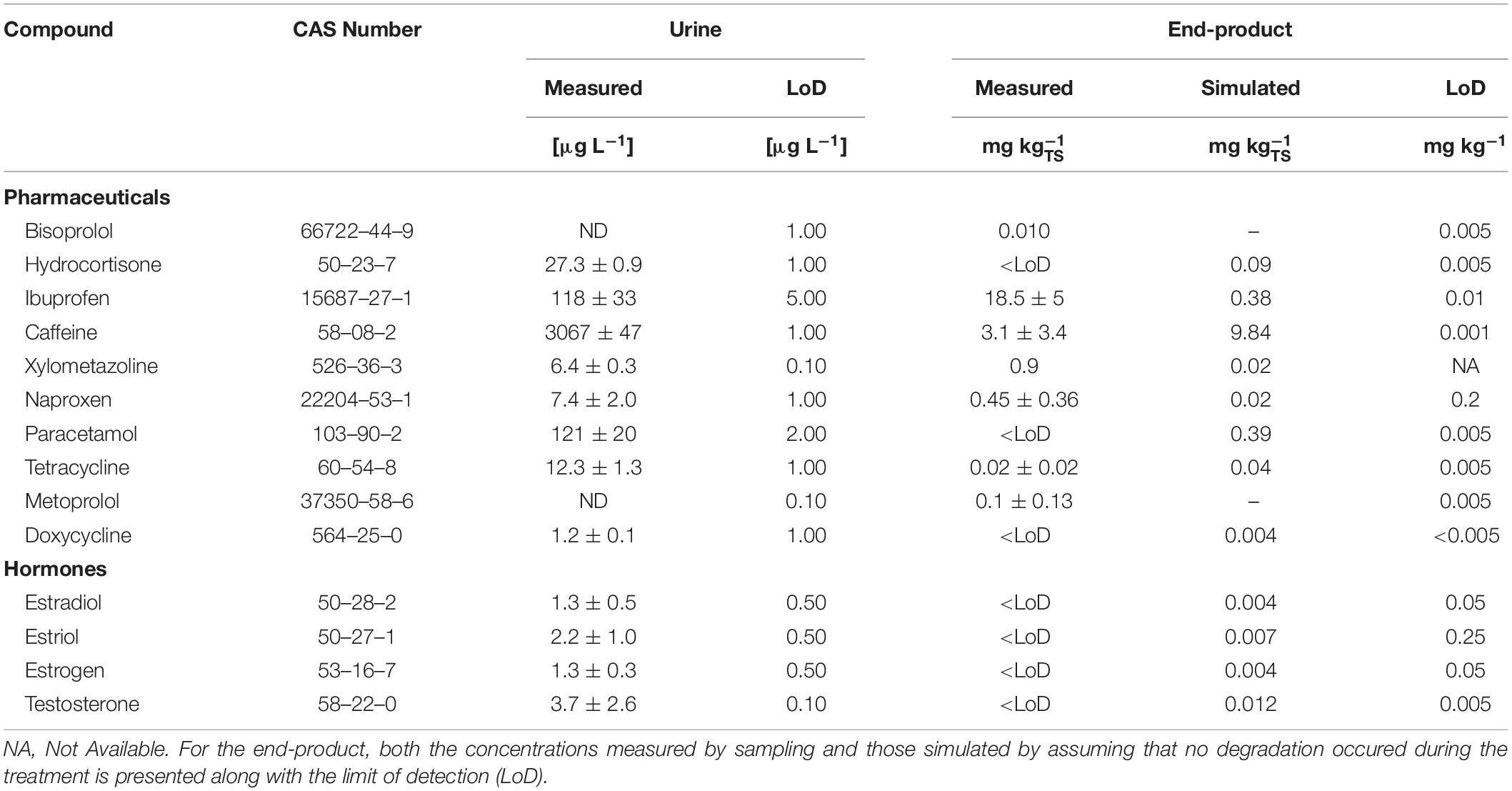
Table 3. Concentrations (mean ± standard deviation) of pharmaceuticals and hormone compounds detected in urine (n = 3) and in the end-product collected at the end of April (n = 2) on dry matter (TS) basis.
Physicochemical Properties
The drying substrate had an average pH1:5 of 13.3 ± 0.02 and EC1:5 of 49 ± 0.7 mS cm–1 at the start of the treatment. All through the pilot, the end-products had pH1:5 > 10, which is a pre-requisite to prevent enzymatic ureolysis (Geinzer, 2017). Box 3 had significantly higher alkalinity (p < 0.001) as well as electrical conductivity (p < 0.05) than Box 1 for all the treatment periods (Table 4). The dehumidifier condensate had a pH1:5 of 9.0 ± 0.1 and EC1:5 of 801 ± 5 μS cm–1. The average dry matter content of the end-products collected from Box 1 and Box 3 was 80 ± 16% and 88 ± 13%, respectively, while that of the condensate water was 99.9%. The average air temperature differed significantly between the two boxes (p < 0.001) but also between the different treatment periods (p < 0.05). For long periods, there was large temperature overshoot above the desired average drying temperature of 60°C, reaching temperatures up to 90°C.
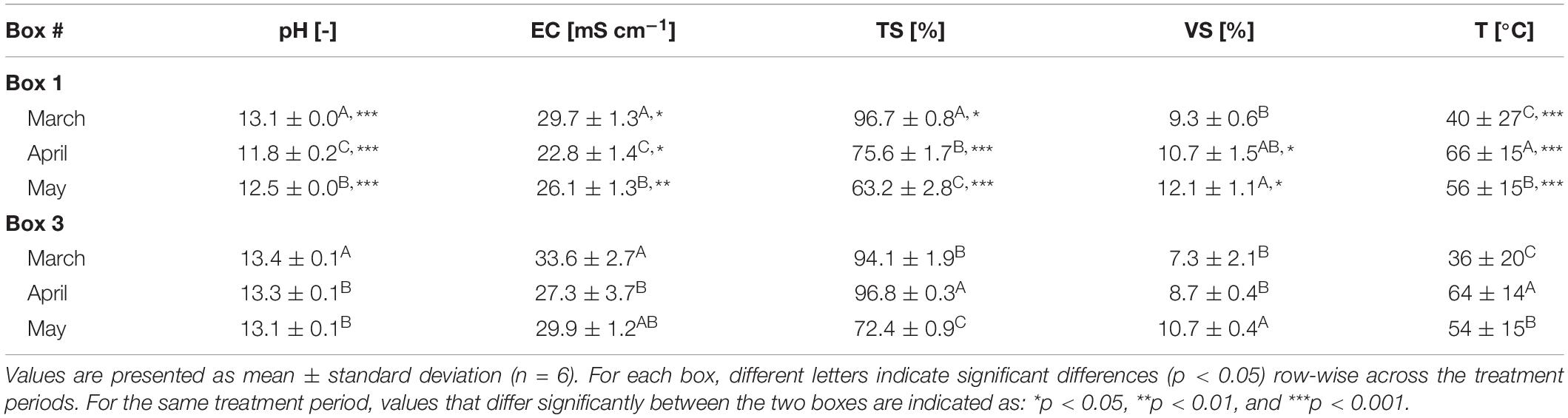
Table 4. The physicochemical properties of the end-products collected in the two boxes at the end of the three treatment periods.
Energy Demand
The pilot’s total electric energy consumption was 1913, 2315, and 1185 kWh during March, April, and May, respectively. The dehumidifier consumed a considerable amount of energy (521 ± 220 kWh month–1), but we disregarded this when relating the total energy demand with the amount of urine treated by the pilot. The average electric energy demand for alkaline dehydration of urine was 24.5 ± 13 kWh kg–1 urine. By using a conversion factor of 2.1 [for average European electricity mix; EC (2018)], the primary energy demand for the three treatment periods amounted to 76, 57, and 21 MJ kg–1 urine.
Discussion
Nutrients
The idea with most technologies treating source-separated urine including alkaline dehydration is to recover resources, primarily macronutrients. Our past studies (Senecal and Vinneras, 2017; Simha et al., 2018, 2020a) in the laboratory demonstrated the possibility of recovering almost all nutrients from urine, including N (>90%). This, however, was the not the case in our pilot-scale setup where the recovery of N was only 30 ± 6%, while we expected to recover 66% of the N at the design average treatment temperature of 60°C.
According to the thermodynamics of water evaporation, a large amount of heat (enthalpy of vaporization) is required for phase transition of liquid water to water vapor, approximately 2358 kJ kg–1 at 60°C. Water absorbs sensible heat from hot air to convert it into latent heat. This causes an evaporative cooling effect that reduces the air temperature. Our pilot system received <10 percent of the urine (<3 L d–1) than what was intended. As a result, the evaporative cooling effect reduced and the temperature in the drying boxes overshot, reaching up to 90°C. High temperature and high pH (>12) would have promoted chemical urea hydrolysis (Blakeley et al., 1982), which can explain the high N losses of the pilot system.
If the pilot system operated at the design capacity, both the N recovery as well as end-product composition would be very different. We estimate that drying 900 kg of urine with 24 kg of drying media (19.5 kg wood ash mixed with 4.5 kg Ca(OH)2) will result in approximately 54 kg of end-product (Figure 2). The N-P-K value (as% of TS) of the final product should be 9.9–1.5–6.0, and it should also contain 0.8% S, 10.2% Ca, 1.6% Mg, 4.6% Na and 6.9% Cl−. The end-product composition is similar to blended fertilizers (11–4.6–17.6) used in Finland and Sweden for vegetables, fruits and berries (Granngården, 2019; Yara, 2020). Blended mineral fertilizers can contain micronutrients such as Mg, S, B, Cu, Fe, Mn, Mo, and Zn, all of which are also present in urine and the end-product. Preliminary crop trials applying dried urine have been promising (Martin et al., 2017) and sociological studies have indicated positive consumer acceptance (Simha et al., 2017; Brun, 2018).
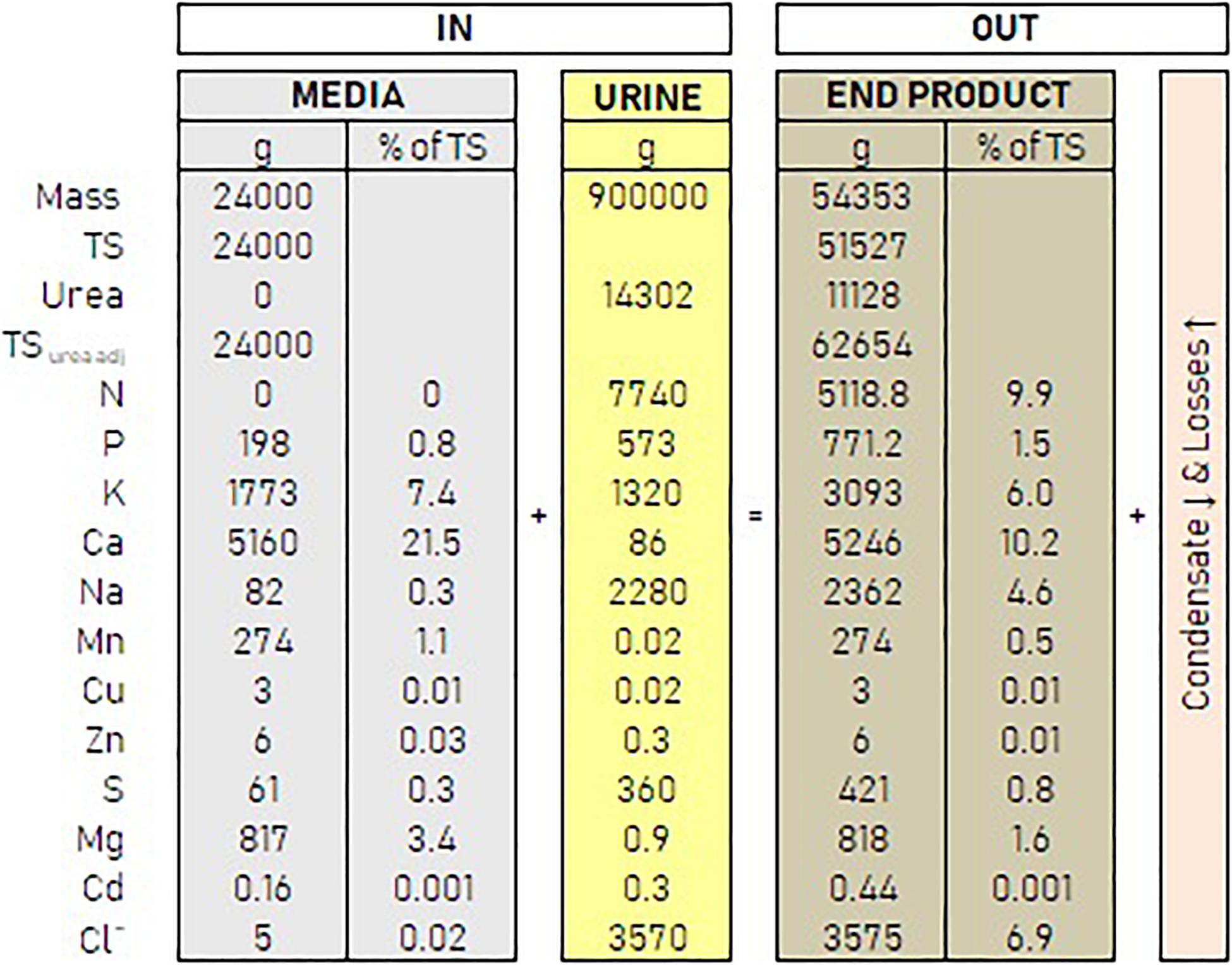
Figure 2. Simulated mass and nutrient balance (in g) for alkaline dehydration of 900 kg urine month–1 using the pilot-scale system. The end-product composition is shown as a% of TS that was adjusted for loss of urea. The measured composition of urine shown in Table 1 was used for the simulation and end-product mass was calculated using a mass.cfWB of 17 from Simha et al. (2020a).
Using a dehumidifier for venting out the exhaust air produced condensate water containing nearly 0.5 gN L–1, because the N loss from the drying boxes was high. Even if N losses can be limited, we can expect the condensate water to contain some N. One way to manage this water would be to soil infiltrate it, especially since it does not contain P (not detected, detection limit 0.01 mg L–1). This discharge would be within the Finnish regulations concerning the environmental loading of treated wastewater, even in groundwater sensitive areas (Ministry of the Environment, 2011).
Energy
The energy demand of the pilot (21–76 MJ kg–1 urine) was far more than what we expected, as operating it at the design capacity would consume 10–12 MJ kg–1 urine; the calculation assumes that 30 L urine d–1 is treated for 30 days with six fans having a power rating of 1200 W operating continuously. The energy needed for treating urine at a wastewater treatment plant is 0.9 MJ kg–1, and that for producing mineral NPK fertilizer equivalent to what is excreted in urine is 0.4 MJ kg–1 (See Supplementary Information, Supplementary Table S4). Therefore, even at its design capacity, the pilot would have consumed ten times more energy than the conventional scenario. The energy demand is, however, comparable to what incineration toilets typically consume, 6–15 MJ kg–1 urine, as they require 0.4–2 kWh flush–1.
Optimizing the energy consumption was not an objective of this study, especially since this was the first field test. The minimum primary energy demand for evaporating water under the pilot conditions is approximately 5.3 MJ kg–1 (170 kJ kg–1 to increase temperature from 20 to 60°C and 2360 kJ kg–1 for phase transition, and primary energy factor of 2.1). If 85% of this energy can be recovered, e.g., with a heat pump, the energy demand for drying urine can potentially be reduced to 0.8 MJ kg–1.
There are many ways to significantly reduce the energy demand of the system. The heater fans used in the pilot setup had a power rating of 700/1200 W, but could be used intermittently or substituted with ones having lower rating. The exhaust air from the system had a temperature of 35 ± 14°C but was simply vented out. Heat from the exhaust air could be recovered (around 60–95%) using air-to-air heat exchangers or heat pumps.
Substances of Concern
One concern against the use of urine as fertilizer is its high salt concentration, since its repeated application may cause soil salinization (Mnkeni et al., 2008). The most abundant salt excreted in urine is NaCl (Putnam, 1971). This study estimated that <5% Na+ and around 7% Cl– is present in the end-product obtained from alkaline urine dehydration (Figure 2). The Cl– concentration is similar to that in blended mineral fertilisers used in Finland and Sweden [e.g., Yara Mila 21–4–7; Yara (2020)]. The Na+ concentration is high but may not be an issue as salts can normally be flushed out of soil during irrigation, rainfall events or leached by drainage (Guizani et al., 2016). However, if dried urine fertilizer is to be applied in arid regions, the Na+ concentration needs to be considered.
The concentration of heavy metals excreted in urine is low and compares favorably with mineral fertilizers (Jönsson et al., 1997). Yet, the contribution of non-essential heavy metals by the drying media can be large (Simha et al., 2020a). Of particular concern is Cd present in wood ash, as it can accumulate in food chains and risk public health (Roberts, 2014). The end-products obtained in this pilot study had an average Cd concentration of 529 ± 76 , which can be reduced to <370 by operating the system at design capacity. Yet, the Cd content is much higher than what is normally found in farmyard manure on organic cattle farms in Sweden (Jönsson et al., 2004). It is, however, less than the upcoming European Cd limits (EU, 2019) for solid organic fertilizers (1.5 mg) and organo-mineral fertilizers with <5% P2O5 (3 mg). Given these considerations, we recommend applying urine dried in wood ash only in forestry, where Cd is not a major concern (Pitman, 2006) and where the limit values are not exceeded. For agricultural applications, wood-based ash should be avoided as drying media, as several other equally effective substrates that are essentially Cd-free can be used instead (Simha et al., 2020a).
Residues of pharmaceuticals, their metabolites and transformation products are excreted via urine (Lienert et al., 2007), and there is concern against applying urine or urine-based fertilizers in agriculture (Winker, 2009). Yet, wastewater treatment plants only partially eliminate these compounds, as evidenced by their detection in urban drinking water reservoirs (Levén et al., 2016). The current best practice is to apply pharmaceutical residues in soil where there is higher likelihood for their degradation (Viskari et al., 2018; Simha et al., 2020b). In the current study, we detected very few (8 out of 126) pharmaceutical compounds in the urine and their concentrations after urine dehydration were low. This is to be expected since the users of our pilot-scale system were mainly healthy young adults undergoing military training. However, the urine was sampled only once during the study which is why some compounds (e.g., bisoprolol) were measured in the end-product but were not detected in the urine. Also, the concentration of some compounds (ibuprofen and xylometazoline) was higher than their simulated concentration, which was calculated by assuming that no degradation occurred during dehydration. The rest of the pharmaceuticals were either below the LoD or below their simulated concentration. This indicated that they were either difficult to extract from the alkaline substrate or that they were degraded during treatment. The fate of pharmaceuticals following alkaline urine dehydration is not well known and is based on previous studies on biodegradability of pharmaceuticals in water and soil, e.g., Barra Caracciolo et al. (2015). Further research is needed to understand the fate and degradation of pharmaceuticals in different matrixes in the environment.
Conclusion and Implementation Outlook
There were several positives from this pilot-scale study. To begin with, we managed to design and engineer an on-site urine treatment system with capacity to dry 30–40 L urine d–1 m–2, replicating results from earlier laboratory-scale experiments (Simha et al., 2020a). While the recovery of N was less than what we expected, our modeling showed that the technology has potential to recover the majority of urine’s nutrients and yield end-products with high fertilizer value (13.2–2.3–6.0, N-P-K as% of TS), if it is operated at full capacity. The pilot’s energy demand was clearly higher than the conventional scenario, but by recovering energy (e.g., with a heat pump) it may be possible for the treatment to be energetically more favorable. This field study demonstrated that alkaline dehydration of urine is scalable and that with further research and improvements, it can be implemented in practice. An advantage of alkaline dehydration is that it is modular. Our study built three drying modules, each with dimensions of 580 × 450 × 300 mm and capacity to treat 10 L urine d–1. Simply by interlinking the modules and duplicating them, larger volumes of urine can be treated and the technology can be scaled up.
Results of our case study in Finland have a much broader context and may find use in other settings. This could be other military training sites; for instance, at the thirteen UN peacekeeping camps across the globe, where more than 100,000 UN peacekeepers (troops, police, and civilian personnel) have been deployed (United Nations Peacekeeping, 2020). Here, there is an annual potential of collecting 400 t N and 18 t P as dry concentrated urine-based fertilizer. Shelters that are managed by the International Federation of Red Cross and Red Crescent Societies following natural disasters, or shelter clusters coordinated by the UN Refugee Agency may also be appropriate settings for implementing on-site urine dehydration treatment. In 2018 alone, more than 70 million people (of which there were nearly 26 million refugees) were forcibly displaced (UNHCR, 2019) and needed shelter or various forms of shelter assistance. The need for developing better approaches to manage and recycle toilet wastes in such settings and circumstances is therefore large and urgent.
Data Availability Statement
The raw data supporting the conclusions of this article will be made available by the authors, without undue reservation.
Author Contributions
All authors contributed to the study conceptualization and design, manuscript revision, read and approved the submitted version. PS and CK constructed and installed the pilot-scale system. PS, CK, and E-LV performed monitoring, data collection, and formal analysis with inputs from BV and RM. BV and E-LV supervised the study, provided resources and acquired funding. PS wrote the first draft of the manuscript.
Funding
This work was supported by grants from the Swedish Research Council, “UDT 2.0 – Urine Dehydration Technology for Sanitation 2.0” (Grant Number 2018-05023) and the Finnish Innovation Fund SITRA, “Mobile nutrient recovery under field conditions (MORTTI).”
Conflict of Interest
BV and PS are owners of Sanitation360 AB but receive no salary.
The remaining authors declare that the research was conducted in the absence of any commercial or financial relationships that could be construed as a potential conflict of interest.
Acknowledgments
In addition to the authors, the active working group of the MORTTI project included the following people who are gratefully acknowledged – Johanna Kallio from the Finnish Environment Institute, Inka Honkala from the Tampere University of Applied Sciences (TAMK), Sari Huuhtanen and Susanna Pakula from the Global Dry Toilet Association of Finland (Huussi Ry), Eliisa Pärttö and Nina Kokko from the Construction Establishment of the Finnish Defence Administration (PHRAKL), as well as Briitta Vilkki from the 2nd Logistics Regiment of the Finnish Defence Forces (2LOGR). Giulio Zorzetto, Evgheni Ermolaev, Sven Smårs, Göran Gullberg, and Chinmoy Deb helped with the construction of the pilot-scale system. Staff at the TAMK Construction and Environmental Engineering Lab provided support during the system integration. Thanks also goes to Terhi Helkala, Environmental Specialist with the Pori Brigade as well as Seija Haapamäki and Jani Haikonen from TAMK. Volunteers from the Finnish Defence Forces used our system in the field and their donations helped us perform valuable research. Nordkalk supported the project by supplying Ca(OH)2.
Supplementary Material
The Supplementary Material for this article can be found online at: https://www.frontiersin.org/articles/10.3389/fenvs.2020.570637/full#supplementary-material
References
Barra Caracciolo, A., Topp, E., and Grenni, P. (2015). Pharmaceuticals in the environment: biodegradation and effects on natural microbial communities. A review. J. Pharm. Biomed. Anal. 106, 25–36. doi: 10.1016/j.jpba.2014.11.040
Blakeley, R. L., Treston, A., Andrews, R. K., and Zerner, B. (1982). Nickel(II)-promoted ethanolysis and Hydrolysis of N-(2-Pyridylmethyl)urea. A model for urease. J. Am. Chem. Soc. 104, 612–614. doi: 10.1021/ja00366a040
Brun, F. (2018). Freins et leviers à l’emploi de Fertilisants à Base d’urine Humaine en Agriculture en Île-de-France. Paris: Laboratoire Eau Environnement et Systèmes Urbains.
Dutta, S., and Vinneras, B. (2016). Fertilizer from dried human urine added to ash and lime – a potential product from eco-sanitation system. Water Sci. Technol. 74, 1436–1445. doi: 10.2166/wst.2016.324
EC (2018). Directive (EU) 2018/2002 of the European Parliament and of the Council of 11 December 2018 Amending Directive 2012/27/EU on Energy Efficiency (Text with EEA Relevance) in PE/54/2018/REV/1, European Parliament & Council of the European Union. Brussels: Publications office of the European Union.
EU (2019). Regulation (EU) 2019/1009 of the European Parliament and of the Council. Brussels: The European Parliament.
FAO (2017). “New food balances,” in Protein Supply Quantity, (Rome: Food and Agriculture Organization of the United Nations). Available online at: http://www.fao.org/faostat/en/#data/FBS
Friedler, E., Butler, D., and Alfiya, Y. (2013). “Wastewater composition,” in Source Separation and Decentralization for Wastewater Management, eds T. A. Larsen, K. M. Udert, and J. Lienert (London: IWA Publishing), 241–257.
Geinzer, M. (2017). Inactivation of the Urease Enzyme by Heat and Alkaline pH Treatment. Master’s thesis, Swedish University of Agricultural Sciences, Uppsala.
Granngården (2019). Gödsel. Available online at: https://www.granngarden.se/tradgard/godsel/c/tradgardsgodsel (accessed November 6, 2019).
Guizani, M., Fujii, T., Hijikata, N., and Funamizu, N. (2016). Salt removal from soil during rainy season of semi-arid climate following an assumed salt accumulation from previous cultivations fertilized with urine. Euro Mediterr. J. Environ. Integr. 1:10. doi: 10.1007/s41207-016-0010-9
Harder, R., Wielemaker, R., Larsen, T. A., Zeeman, G., and Öberg, G. (2019). Recycling nutrients contained in human excreta to agriculture: pathways, processes, and products. Crit. Rev. Environ. Sci. Technol. 49, 695–743. doi: 10.1080/10643389.2018.1558889
Jönsson, H., Stenström, T. A., Svensson, J., and Sundin, A. (1997). Source separated urine-nutrient and heavy metal content, water saving and faecal contamination. Water Sci. Technol. 35, 145–152. doi: 10.2166/wst.1997.0338
Jönsson, H., Stintzing, A. R., Vinnerås, B., and Salomon, E. (2004). Guidelines on the Use of Urine and Faeces in Crop Production. Stockholm: Stockholm Environment Institute.
Jönsson, H., and Vinnerås, B. (2004). “Adapting the nutrient content of urine and faeces in different countries using FAO and Swedish data,” in Proceedings of the Ecosan–Closing the Loop. The 2nd International Symposium on Ecological Sanitation, Incorporating the 1st IWA Specialist Group Conference on Sustainable Sanitation, Bonn.
Larsen, T. A., Udert, K. M., and Lienert, J. (2013). Source Separation and Decentralization for Wastewater Management. London: IWA Publishing.
Levén, L., Gros Calvo, M., Dalahmeh, S., Ljung, E., Lundin, G., Ahrens, L., et al. (2016). Läkemedel I källsorterat Klosettvatten och latrin – Behandling och Risker (In Swedish), JTI-Rapport 2016, Kretslopp & Avfall nr 54, JTI, Uppsala, Sweden, 2016. Uppsala: JTI – Institutet för jordbruks- och miljö teknik.
Lienert, J., Burki, T., and Escher, B. I. (2007). Reducing micropollutants with source control: substance flow analysis of 212 pharmaceuticals in faeces and urine. Water Sci. Technol. 56, 87–96. doi: 10.2166/wst.2007.560
Martin, T., Houot, S., Levavasseur, F., and Esculier, F. (2017). “Valorisation des urines comme source d’azotepour les plantes: une expérimentation en serre,” in Assemblée Générale du SOERE PRO: INRA, (Paris: French National Research Institute for Agriculture).
McConville, J. R., Kvarnström, E., Jönsson, H., Kärrman, E., and Johansson, M. (2017). Source separation: challenges & opportunities for transition in the swedish wastewater sector. Resour. Conserv. Recyc. 120, 144–156. doi: 10.1016/j.resconrec.2016.12.004
Ministry of the Environment (2011). Government Decree on Treating Domestic Wastewater in Areas Outside Sewer Networks (209/2011) Forest Ministry of the Environment. Helsinki: Ministry of the Environment.
Ministry of the Environment (2016). Government Decree on Landfills 331/2013. New Delhi: Forest Ministry of the Environment.
Mnkeni, P. N., Kutu, F. R., Muchaonyerwa, P., and Austin, L. M. (2008). Evaluation of human urine as a source of nutrients for selected vegetables and maize under tunnel house conditions in the Eastern Cape, South Africa. Waste Manag. Res. 26, 132–139. doi: 10.1177/0734242X07079179
Pitman, R. M. (2006). Wood ash use in forestry – a review of the environmental impacts. Forestry 79, 563–588. doi: 10.1093/forestry/cpl041
Putnam, D. F. (1971). Composition and Concentrative Properties of Human Urine. Washington, DC: National Aeronautics and Space Administration.
Roberts, T. L. (2014). Cadmium and phosphorous fertilizers: the issues and the science. Proc. Eng. 83, 52–59. doi: 10.1016/j.proeng.2014.09.012
Senecal, J., Nordin, A., Simha, P., and Vinneras, B. (2018). Hygiene aspect of treating human urine by alkaline dehydration. Water Res. 144, 474–481. doi: 10.1016/j.watres.2018.07.030
Senecal, J., and Vinneras, B. (2017). Urea stabilisation and concentration for urine-diverting dry toilets: Urine dehydration in ash. Sci. Total Environ. 586, 650–657. doi: 10.1016/j.scitotenv.2017.02.038
Simha, P., Lalander, C., Nordin, A., and Vinnerås, B. (2020a). Alkaline dehydration of source-separated fresh human urine: preliminary insights into using different dehydration temperature and media. Sci. Total Environ. 733:313. doi: 10.1016/j.scitotenv.2020.139313
Simha, P., Senecal, J., Gustavsson, D. J. I., and Vinnerås, B. (2020b). “Resource recovery from wastewater: a new approach with alkaline dehydration of urine at source,” in Current Developments in Biotechnology and Bioengineering: Sustainable Bioresources for Emerging Bioeconomy, eds A. Pandey, R. Kataki, D. Pant, and S. Khanal (Amsterdam: Elsevier Publications), 205–221.
Simha, P., Lalander, C., Vinneras, B., and Ganesapillai, M. (2017). Farmer attitudes and perceptions to the re-use of fertiliser products from resource-oriented sanitation systems – The case of Vellore, South India. Sci. Total Environ. 581–582, 885–896. doi: 10.1016/j.scitotenv.2017.01.044
Simha, P., Senecal, J., Nordin, A., Lalander, C., and Vinnerås, B. (2018). Alkaline dehydration of anion–exchanged human urine: volume reduction, nutrient recovery and process optimisation. Water Res. 142, 325–336. doi: 10.1016/j.watres.2018.06.001
UNHCR (2019). Global Trends Forced Displacement in 2018. Geneva: United Nations High Commissioner for Refugees.
United Nations Peacekeeping (2020). Peacekeeping Operations Fact Sheet. New York, NY: United Nations Peacekeeping.
Viskari, E.-L., Grobler, G., Karimäki, K., Gorbatova, A., Vilpas, R., and Lehtoranta, S. (2018). Nitrogen recovery with source separation of human urine—preliminary results of its fertiliser potential and use in agriculture. Front. Sustain. Food Syst. 2:32. doi: 10.3389/fsufs.2018.00032
Winker, M. (2009). Pharmaceutical Residues in Urine and Potential Risks Related to Usage as Fertiliser in Agriculture, Ph. D thesis, Technische Universität Hamburg, Hamburg.
Winker, M., Vinneras, B., Muskolus, A., Arnold, U., and Clemens, J. (2009). Fertiliser products from new sanitation systems: their potential values and risks. Bioresour. Technol. 100, 4090–4096. doi: 10.1016/j.biortech.2009.03.024
Yara (2020). YaraMila Lannoitteet. Available online at: https://www.yara.fi/lannoitus/lannoitteet/yaramila/ (accessed June 4, 2020).
Keywords: circular bioeconomy, decentralized systems, nutrient recycling, resource recovery, sanitation, source-separation, urine drying, wastewater treatment
Citation: Simha P, Karlsson C, Viskari E-L, Malila R and Vinnerås B (2020) Field Testing a Pilot-Scale System for Alkaline Dehydration of Source-Separated Human Urine: A Case Study in Finland. Front. Environ. Sci. 8:570637. doi: 10.3389/fenvs.2020.570637
Received: 08 June 2020; Accepted: 24 August 2020;
Published: 11 September 2020.
Edited by:
Efthalia Chatzisymeon, The University of Edinburgh, United KingdomReviewed by:
Yung-Tse Hung, Cleveland State University, United StatesPaula Loureiro Paulo, Federal University of Mato Grosso do Sul, Brazil
Copyright © 2020 Simha, Karlsson, Viskari, Malila and Vinnerås. This is an open-access article distributed under the terms of the Creative Commons Attribution License (CC BY). The use, distribution or reproduction in other forums is permitted, provided the original author(s) and the copyright owner(s) are credited and that the original publication in this journal is cited, in accordance with accepted academic practice. No use, distribution or reproduction is permitted which does not comply with these terms.
*Correspondence: Prithvi Simha, Prithvi.Simha@slu.se; prithvi.simha@mespom.eu; prithvisimha092@gmail.com