- 1Department of Hydromechanics and Hydrology, Federal University of Rio Grande do Sul, Porto Alegre, Brazil
- 2Department of Civil and Environmental Engineering, Federal University of Paraíba, João Pessoa, Brazil
Natural river flow provides the conditions required to sustain freshwater ecosystems, and the greater the departure from the natural regime, the greater the loss of those ecosystems. In South America, new hydropower dams are continuously being constructed and planned in regions within and around the Amazon basin and in the Upper Paraguay river basin, a region notable for the Pantanal, a huge wetland ecosystem that is largely dependent on the flow regime of the Paraguay river and its tributaries. In this context, it is meaningful to examine the hydrological changes caused by the major Manso dam, that is operating since 2001 at the headwaters of one of the major tributaries of the Paraguay river. This was done for the same case study by other authors in previous studies using only gauging stations data. However, those previous assessments were limited due to the confounding effects of climate variability and the necessity of relatively long term observed time series. Here, we applied a modeling approach to evaluate the changes in hydrological regime caused by Manso dam operation. Our modeling approach was based on the combination of the MGB large-scale hydrologic model with the SIRIPLAN large-scale wetland model. The models were applied, using river reaches from 2 to 10 km, in two scenarios during the period from 2003 to 2015. In the first scenario we used naturalized streamflow at the dam site as input to the hydrological model. In the second scenario we used observed reservoir outflow time series as input to the hydrological model. Our results show that Manso dam has a regulation effect that decreases high flows, increases low flows and reduces lateral connectivity. The decrease in high flows is more pronounced in the region upstream of the Pantanal floodplain, but not limited to, while increase in low flows extends into Pantanal. Timing of maximum and minimum flows is less affected, except for the river reach immediately downstream of the dam. Our results improve the assessment of spatial patterns of hydrologic alteration, giving more confidence in the assessment of magnitude and spatial extension of the effects of Manso dam in the Pantanal region.
Introduction
An underpinning assumption is that natural river flow provides the baseline for determining what is necessary for ecosystem maintenance, because ecosystems evolved under those conditions (Poff et al., 1997). In other words, a naturally variable regime of flow is required to sustain freshwater ecosystems, and the greater the departure from the natural regime, by water abstractions and flow regulation to maximize obtaining river goods, the greater the loss of those ecosystems (Poff et al., 2010).
River goods are defined as products that are of societal use when extracted or diverted from the river system (Brismar, 2002). Direct benefits or products include drinking, growing food, navigate, supporting industry and producing hydroelectric power. Besides, through river services, which are defined by Brismar (2002) as naturally generated and maintained processes by rivers that are of societal value, people can also collect indirect benefits, including recreation, soil wetting and fertilization of floodplains and deltas, cultural identity and ecosystem maintenance. Nevertheless, increases in water uses to maximize river goods normally result in decreased indirect benefits from river services. This occurs because river flow and water quality are major determinants of river ecosystem condition (Acreman, 2016).
Obtaining direct benefits from water by producing electric power in dams frequently leads to reduced indirect benefits (Ziv et al., 2012). Dams and reservoirs used for hydropower production impact ecosystems by river habitat fragmentation, habitat transformation from lotic to lentic, retention of sediments and nutrients and river flow alteration (FitzHugh and Vogel, 2011; Schmutz and Moog, 2018). Expanding hydropower have been considered one of the main emerging threats to freshwater biodiversity (Reid et al., 2019) and the alteration of river flow regimes for hydropower production is a critical factor responsible for decline in freshwater communities (Poff and Zimmerman, 2010). To put in a global perspective, according to Grill et al. (2019) only 37 per cent of rivers longer than 1,000 kilometers remain free-flowing over their entire length and 23 per cent flow uninterrupted to the ocean.
While in North America and Europe most dams have been constructed before the second half of the XX century, in countries with emerging economies the pace of dam construction is still high (Zarfl et al., 2015; Winemiller et al., 2016). In South America, for example, new hydropower dams are being constructed and planned in regions within and around the Amazon basin, leading to considerable concerns about the possible environmental consequences (Finer and Jenkins, 2012; Tundisi et al., 2014; Pavanato et al., 2016; Forsberg et al., 2017; Latrubesse et al., 2017; Timpe and Kaplan, 2017; Anderson et al., 2018; Fraser, 2018; Santos et al., 2018). Only in the Brazilian Amazon, over 200 new hydropower dams are predicted to be constructed in the next 30 years (Timpe and Kaplan, 2017). When considering small hydropower plants, Couto and Olden (2018) estimated that 82,891 small hydropower plants were operating or under construction in 150 countries and that another 181,976 new plants may be installed if all potential capacity were developed in the next decades, most of them in countries such as Russia, China, India, Brazil, and the United States. Despite being considered small, these dams also may have the capability to significantly alter the hydrological cycle, depending on its characteristic and the river they are located, especially considering hydrologic impact per megawatt (Timpe and Kaplan, 2017), to the point of affecting fauna and flora (Casas-Mulet et al., 2015; Bejarano et al., 2018; Mihalicz et al., 2019; Vehanen et al., 2020).
Regarding the expansion of the hydroelectric matrix in Brazil and its potential damages, besides the Amazon basin, another location where a large number of dams are planned is the Upper Paraguay river basin, a region that is notable for the Pantanal, a huge wetland ecosystem that is largely dependent on the flow regime of the Paraguay river and its tributaries. In this region, there is a debate about the potential impacts of new dam construction in the highlands (Planalto) on the ecosystems of the lower lying Pantanal (Bergier, 2013; Coelho da Silva et al., 2019; Medinas de Campos et al., 2020). In this context, it is worthwhile to carefully examine the hydrological changes that followed the construction of the major Manso dam, that started operation in 2000 at the headwaters of one of the major tributaries of the Paraguay river.
This task is not a completely original effort, since previous studies by Souza et al. (2009); Zeilhofer and de Moura (2009), and Timpe and Kaplan (2017) already analyzed hydrological changes downstream of the Manso dam. Zeilhofer and de Moura (2009) used streamflow data up to 2005, meaning that they analyzed only 4 years of data after the dam started to operate, while Souza et al. (2009) used slightly longer streamflow time series after the dam started to operate, by including data up to 2007. Both 4 and 6 years of data are normally considered to be unsatisfactory for the assessment of hydrological changes. Richter et al. (1997), for instance, propose a minimum of 20 years of pre and post-impact data. In the work by Souza et al. (2009), the authors tried to account for climatic variability by using benchmark gauging stations at rivers not influenced by Manso dam, but with limited success, since climatic variability plays an important role in the hydrological functioning of the region (Collischonn et al., 2001; Barros et al., 2004).
More recently, Timpe and Kaplan (2017) assessed hydrological regime changes due to the operation of several dams in Central and Northern Brazil, and showed that Manso dam has severe impacts on river regime, only second to the Balbina dam, on the Uatumã river, in the Amazon basin. However, their assessment was based on data from a single gauging station (Fazenda Raizama – ANA gauge 66231000) located just 15 km downstream of the dam. Therefore, the studies carried out on the impacts of the Manso dam have so far been restricted either in time, due to the low number of years used for the analysis of hydrological impacts, or in space, as they used few river gauges whose capacities to assess impacts are restricted to the locations where they are installed.
In order to overcome the limitations of previous studies, an alternative way to assess hydrologic alteration could be achieved by applying a distributed hydrological model with the ability of generating discharge time series at a multitude of points within the basin, and capable of representing scenarios with and without the dam operation in a realistic way. After proper parameter calibration, this model could then be applied using observed time series of rainfall, generating results of the two scenarios (with and without the dam) for the same period of time, thus taking complete control of the influence of climatic variability. A further advantage of this method is that it generates results not only at places where observation gauges exist, but at several ungauged sites too, allowing an assessment of spatial patterns of hydrologic alteration.
This model-based methodology was previously adopted by Ryo et al. (2015) who used a distributed hydrological model to quantify the spatial patterns of flow regime alterations along the Sagami River basin network under natural and altered flow conditions. The use of hydrological models was also suggested by Poff et al. (2010) as a part of the ELOHA framework for the definition of environmental flows and advocated by Richter et al. (1997) and Kennen et al. (2008). However, adopting the model-based assessment of hydrological change approach in the Upper Paraguay river basin is particularly challenging, due to the complexities of the physical system, with large floodplains and lakes, and very mild slopes, as discussed by Paz et al. (2010).
In the present paper, we took advantage of previously developed hydrological models in the Upper Paraguay river basin (Paz et al., 2011, 2014; Bravo et al., 2012), and in other environments with significant floodplains (Pontes et al., 2017; Fleischmann et al., 2018) and applied them to assess the magnitude and extension of fluvial regime changes imposed by the operation of Manso dam on rivers within the Pantanal, therefore supplementing previous assessments made by Souza et al. (2009) and Zeilhofer and de Moura (2009). Using computational modeling by combining the MGB large-scale hydrologic model with the SIRIPLAN large-scale wetland model, the effects of Manso dam on the hydrology of the Upper Paraguay River Basin could be identified continuously along the drainage network downstream. With this approach, we could assess hydrologic alteration in any segment of the river network, not only at gauges like on previous studies on the same dam. In addition, confounding climatic variability effects could be isolated since the only difference between the two simulated scenarios is the Manso dam operation. This differentiates the present work from previous studies about the influence of Manso dam operation on the hydrological regime of the Cuiabá river and also the fact that the present research is based on a larger discharge time series than the previous ones. Also, by representing the interaction between river and floodplain through modeling, we were able to better comprehend the impacts that a dam can have on the flow exchange between them and how far those impacts can go, which may be of interest for other locations upstream or in floodplains, not only in the Pantanal or Amazon region, where dams are operating or are intended to be installed.
Therefore, there are two main contributions of our paper. First, we define the magnitude, extent and spatial distribution of changes in the hydrological regime caused by a large dam in the Pantanal, which is a region of globally recognized ecological importance. This definition was made less influenced by other factors than previous studies, such as climate variability, and, therefore, makes it possible to analyze the influence of the dam’s operation more clearly. Second, we present an innovative way to analyze changes in hydrological regime by using hydrologic and hydrodynamic models of rivers with extensive floodplains, and with complex drainage patterns. Although the use of distributed hydrological models to assess and quantify the spatial patterns of flow regime alterations along the drainage network of a river basin have been presented before by Ryo et al. (2015), our study extends this approach to the wider and much more complex environment of the Pantanal wetland.
Materials and Methods
The South American Pantanal and the Upper Paraguay River basin
The Paraguay river is the major tributary of the Paraná river basin within the Paraná – La Plata river basin, draining an area of about 1.2 million km2 (Collischonn et al., 2016). The Upper Paraguay region is the 600,000 km2 basin upstream of the confluence of the Paraguay river with the Apa river, which marks the limits between Brazil and Paraguay (Figure 1). The region is within a seasonally dry tropical region, roughly defined by latitudes 14 and 33 South, and longitudes 53 and 60 West, and encompasses parts of three countries (Brazil, Bolivia, and Paraguay).
The landscape in the region is normally divided in two parts: the Planalto (Uplands) above nearly 200 m altitude; and the Pantanal (Wetland) below 200 m altitude. Rivers in the Planalto region are normally incised in bedrock, and flow into the Pantanal where they become alluvial (Assine et al., 2015). The Pantanal is an extensive low-lying region that was probably formed by subsidence followed by infilling with sediments from the Planalto, with 500 m of sediment deposits measured in some places (Assine et al., 2015). Deposition is still occurring, therefore, the Pantanal is a place of changing rivers with active and abandoned alluvial fans, which form a varied system (Assine et al., 2015). Due to this the Pantanal is a mosaic of variable flooded environments, including permanent lakes, water-filled depressions and small lakes that dry out seasonally, temporary flood drainages with sand or short grass and relatively permanent channels that connect flooded areas (Evans et al., 2010; Girard et al., 2010).
In terms of biodiversity, Pantanal is known for its numerous species and a rich diversity of aquatic and terrestrial ecosystems (Agência Nacional das Águas, 2004), being recognized by UNESCO as a World Biosphere Reserve and as a National Heritage by the National Constitution of Brazil. Pantanal presents a unique variety of fishes, although a considerable number have likely not been identified yet (Shimabukuro-Dias et al., 2017). Barletta et al. (2010) state that about 270 fish species have been reported in the Pantanal, while Petrere et al. (2002) cite estimates of 400 fish species. Both artisanal and recreational fisheries are mostly supported by large migratory species, and tourism fishing is gaining more importance in the last decades (Mateus et al., 2004; Barletta et al., 2010). Migratory movements comprise ascending displacements for spawning that can reach 400 km or even more for some species (Hahn et al., 2011; Barzotto and Mateus, 2017). These migrations are in phase with the rainy season, and the high fish productivity in the region is normally related to the seasonal inundation of floodplains (Barletta et al., 2010).
Over most of the region, rainfall in the six wettest months from October to March accounts for more than 80% of the annual total. Annual average precipitation varies from above 2000 mm in the Northeast of the basin to below 1000 mm in the West, along the border between Brazil and Bolivia (CPRM, 2011). Regularity of climate, with well-defined wet and dry seasons, together with the damping effect of floodplain inundation over the progress of the flood wave, results in a highly predicable flood pulse every year, with a single flood peak occurring in more or less the same time every year. This regular flooding and drying behavior, known as flood pulse, has strong influences on the regions ecological processes (Junk et al., 1989, 2006) and, also, strong consequences on human occupation of the Pantanal. This, together with access difficulties and isolation from major demographic centers hindered economic development and human occupation of the Pantanal. As a consequence, the region still has a very low demographic density and features unique natural landscapes, relatively untouched ecosystems, and traditional cultural practices and is known for its outstanding biological resources making it a priority for Brazilian and international conservation efforts (Junk and de Cunha, 2005; Schulz et al., 2019).
Flood waves formed in the Planalto rivers move relatively quickly to the Pantanal, where large portions of the water spread over the floodplain and deviate from the main channels through divergent drainage networks formed over alluvial fans (Paz et al., 2010; Assine et al., 2015). The overflow of the river channel and consequent floodplain inundation reduces peak discharges to more than one-half along the main rivers that flow into the Pantanal. These effects strongly modify the shape of hydrographs from upstream to downstream, as exemplified by Paz et al. (2010). Peak flows typically occur in February in the northeastern Planalto rivers, concomitantly with rainfall maximums. In the floodplains, on the other hand, flood peaks are delayed due to its slow movement, and typically occur in June, which corresponds to the dry season, in the middle of the Pantanal (Paz et al., 2010). The maximum area subject to inundation in the Pantanal, which include permanent open waters of river channels and lakes, is above 130,000 km2, while the long-term mean inundation area is around 35,000 km2, according to Hamilton et al. (2002).
Several studies relate the ecological functioning of the region to the fluvial regime, including floods, low water, timing of floods, and other abiotic variables related to hydrology. For instance, Bailly et al. (2008) stated that floods of the Cuiabá river, one of the most important tributaries of the Paraguay river, have an important role in the recruitment of species and influence spawning success as well as juvenile survival, and that floods are the principal trigger for the reproduction of many species of fishes. The overarching influence of the fluvial regime on the ecosystem was further highlighted in several other studies (Catella and Petrere, 1996; Petrere et al., 2002; Marchese et al., 2005; Costa and Mateus, 2009; Lourenço et al., 2012; Pinho and Marini, 2012; Ziober et al., 2012; Penha et al., 2015, 2017; Súarez and Scanferla, 2016; Wantzen et al., 2016; Barzotto and Mateus, 2017; Pereira and Súarez, 2018; Tondato et al., 2018; Santana et al., 2019).
Considering this strong dependency of the ecosystem on the natural hydrological regime in the Pantanal, it is expected that changes to the hydrological regime can severely impact ecosystem functioning, as reported in several other places in the world (Poff et al., 2010), including rivers in South America. River regime changes by dam operation have already impacted the ecosystem of the nearly located Paraná river basin, where flow regulation reduced the extent and duration of flood events, limiting or frustrating the reproductive processes of several fish species (Agostinho et al., 2004) and impacting fish populations (Agostinho et al., 2004, 2007, 2016).
According to the Brazilian Water Agency (ANA), there are currently 47 hydropower plants operating at the Upper Paraguay river basin, and more than 100 other hydroelectric facilities are currently in the proposed or construction stages (Medinas de Campos et al., 2020). Among the currently operating facilities, Manso dam is the one with the greatest potential of impacts on the hydrological regime of the Pantanal, due to its large reservoir, even though some regime changes have been reported for the relatively smaller Ponte de Pedra (Fantin-Cruz et al., 2015) dam.
The Manso Dam
Manso dam is a multipurpose dam that was conceived mainly for hydropower generation and flood control (Zeilhofer and de Moura, 2009; Paes and Brandão, 2013). The dam is located on the Manso River (Figure 1), one of the major rivers that form the Cuiabá River, at a point where drainage area is 9365 km2, and average discharge is estimated at 170 m3.s–1. The reservoir has a total volume of 7.3 × 109 m3 with an active storage capacity of 2.95 km3 in the elevation range between 278 m (minimum operational level) and 287 m (maximum normal operation level), and additional 0.45 km3 for flood control in the elevation range between 287 and 288.15 m (Paes and Brandão, 2013). The reservoir regulates the flow of the Manso river and of the Cuiabá river downstream. The dam is located almost 300 km upstream of the cities of Cuiabá and Várzea Grande, which are the main targets for flood control. Downstream from the city of Cuiabá, the Cuiabá river drains into the Pantanal, where it connects to lakes and the floodplain through side channels. The confluence of the Cuiabá and Paraguay river is located well within the Pantanal, at about 900 km downstream of the Manso dam.
The reservoir of Manso dam begun to be filled in November 1999, and the dam started operation in November 2000 (Furnas Centrais Elétricas, 2002). The reservoir was scheduled to be filled by the end of 2000 but due to lower than expected precipitation the complete filling only occurred in February 2002, when it reached the maximum level of 287.5 m (Shirashi, 2003).
Hydrological Modeling
We assessed hydrologic alteration caused by Manso dam operation in the Pantanal region using synthetic streamflow time series obtained by a combination of two hydrologic models and the Indicators of Hydrologic Alteration (Richter et al., 1996) metrics.
We divided the Upper Paraguay river basin in two regions, following a threshold altitude of 200 m (Assine et al., 2015). In regions above 200 m we applied the MGB large-scale hydrological model (Collischonn et al., 2007), further described in Section “Hydrologic Model of the Upper Basin (Planalto), Input Data, and Scenarios.” In regions below 200 m, where the drainage network is not dendritic, and where there are several interconnected lakes, temporary inundated areas and secondary channels, we applied the coupled 1D/2D SIRIPLAN model (Paz et al., 2011), further described in Section “Hydrologic Model of the Lower Basin (Pantanal).” The two modeling sub-regions of the basin are shown in Figure 2 along with the main river gauges used during the calibration of one model or another.
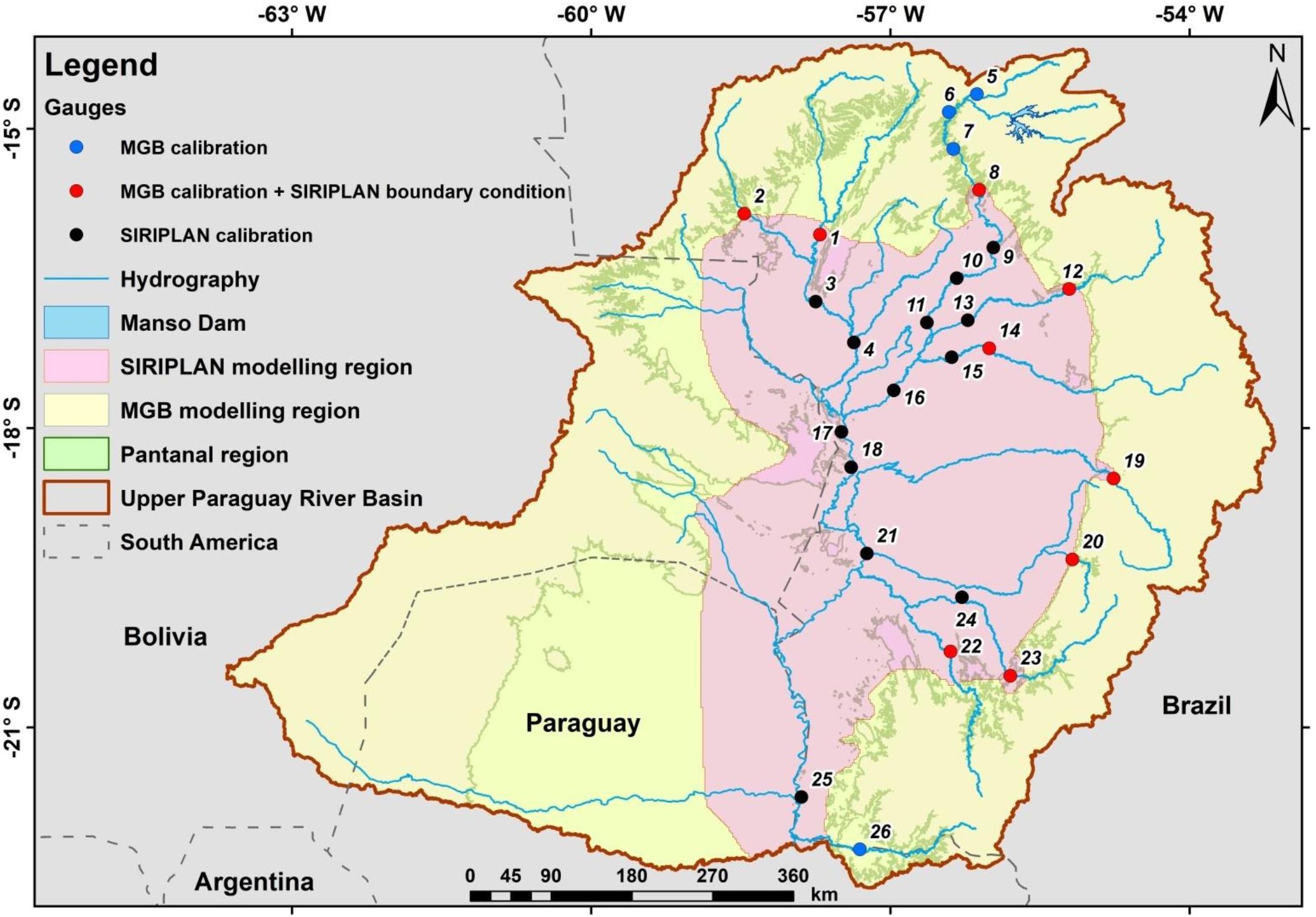
Figure 2. Modeling sub-regions of the Upper Paraguay basin (yellow = MGB model; pink = SIRIPLAN model) and gauges used for calibration (red points are boundary condition locations where streamflow time series calculated with MGB were transferred as input data to SIRIPLAN).
The MGB model of the upper basin was applied first, and output of this model was introduced as boundary conditions for the SIRIPLAN model, which was applied in sequence, at the nine most important inflow points to the Pantanal, as shown by the gauges in red in Figure 2. The blue gauges inside the SIRIPLAN modeling region were used for calibration of this model.
MGB was applied in two scenarios: with and without Manso dam operation, applying methods described in Section “Hydrologic Model of the Upper Basin (Planalto), Input Data, and Scenarios.” Results of the MGB model in the two scenarios were then used as boundary conditions (input variables) to the SIRIPLAN model. Table 1 presents the characteristics of the main river gauges used for calibration and the performance measures Nash-Sutcliffe, used for calibration of maximum flows, Nash-Sutcliffe of the logarithm of the flows, used for calibration of minimum flows, and the volumetric error (BIAS). Further details on the calibration can be found in the work by Paz et al. (2011).
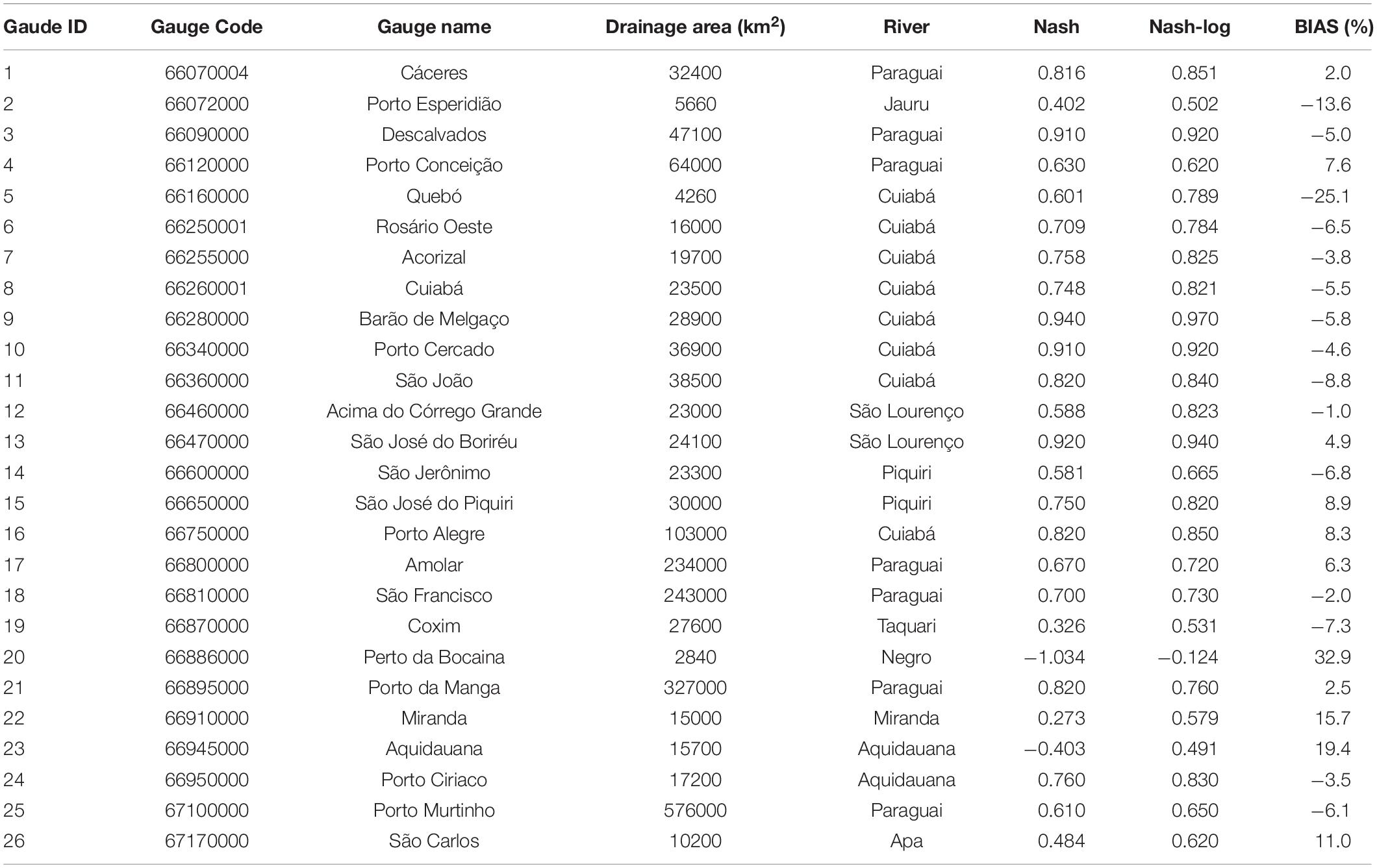
Table 1. Characteristics of the main river gauges presented in Figure 2, used during calibration of the MGB and SIRIPLAN models, and the performance measures obtained.
Both MGB and SIRIPLAN are distributed models and provide results of daily streamflow and water stage at river segments of 2 to 10 km covering the whole drainage network of the Upper Paraguay river basin, at least for rivers with drainage area above 20 km2. Time series covering the period from 01/01/2003 to 31/12/2015, considering the scenarios with and without Manso dam, were then analyzed using the Indicators of Hydrologic Alteration (Richter et al., 1996). These metrics include mean monthly flows, minimum and maximum river discharge values for 1, 3, 7, 30 and 90 days, Julian day of the maximum and minimum flows, as further described in Section “Assessment of Hydrological Change.”
Therefore, for each river segment, two different IHA metric values were obtained, one for each scenario. These two values were subsequently compared and the magnitude of change was estimated as a relative departure of the original value for the majority of the metrics, or as an absolute deviation in the case of the Julian day of minimum and maximum flows. This methodology allowed us to obtain results of the spatial variability of dam impact.
Hydrologic Model of the Upper Basin (Planalto), Input Data, and Scenarios
MGB is a semi-distributed, process-based model developed for large-scale to continental regions. It was first presented by Collischonn et al. (2007), and subsequently improved by Paiva et al. (2011); Pontes et al. (2017), and Fleischmann et al. (2018). The model was used before in several large basins of South America, including assessments of climate change impacts in the Amazon (Sorribas et al., 2016); potential impacts of dams on fluvial ecosystems (Forsberg et al., 2017); hydrological reanalysis (Wongchuig et al., 2019); water management scenarios in transboundary river basins (Gorgoglione et al., 2019); streamflow forecasting (Fan et al., 2015); and continental hydrological modeling for South America (Siqueira et al., 2018).
In its most recent version, MGB divides the river basin in relatively small unit-catchments. This unit-catchments are defined based on the contribution areas that drains to river segments, which are divided with constant length (Siqueira et al., 2016). Within each unit-catchment, Hydrological Response Units (HRU’s) are defined based on soil type and land use, and for each one the water and energy budget is computed through the soil-vegetation system, as described by Collischonn et al. (2007). Surface, subsurface and groundwater outflows from water balance are routed to the main river of the unit catchment using linear reservoirs, while flow propagation through drainage networks is computed using either the Muskingum-Cunge method or 1D hydrodynamic equations (Pontes et al., 2017). To access the influence of dams and reservoir operation within MGB it can be done by introducing internal boundary conditions at the dam location, and forcing the model with observed reservoir outflow data, or by specifying reservoir operation rules (Fleischmann et al., 2019).
In order to do so for the Manso Dam case the model was calibrated using precipitation and river discharge data obtained mainly from the National Water Agency (ANA) in Brazil. We used data from 153 precipitation gauges and a total of 42 streamflow gauges distributed over the whole Upper Paraguay river basin to calibrate the model, focusing on the Planalto region. The main gauges are presented in Figure 2. Rainfall information outside Brazil was obtained from the Multi-Source Weighted-Ensemble Precipitation (MSWEP) product, that is based mainly on satellite precipitation estimates.
Operational data of the Manso dam were also obtained from ANA, including observed outflow time series and naturalized time series from 01/01/2001 to 31/12/2015. Naturalized streamflow is an information routinely estimated for the main hydropower reservoirs in Brazil by ANA and ONS (Operador Nacional do Sistema) (Agência Nacional da Água, 2011) and corresponds to streamflow that would be observed at the dam site if there was no flow regulation by reservoirs and no water abstractions upstream.
After model calibration and verification MGB was applied in two scenarios during the period from 01/01/1985 to 31/12/2015. In the first scenario, intended to represent the natural condition, we used naturalized streamflow at the dam site as input to the hydrological model. In the second scenario, intended to represent the impact condition, we used observed reservoir outflow time series as input to the hydrological model. In those two scenarios the rainfall-runoff processes upstream of the Manso dam have been turned off, since the dam was represented as a boundary condition. However, in the remaining area of the basin, the rainfall-runoff and flood routing processes have been ordinarily represented by the model.
Hydrologic Model of the Lower Basin (Pantanal)
Outputs from the hydrological model described in Section “Hydrologic Model of the Upper Basin (Planalto), Input Data, and Scenarios” were introduced as inputs for the hydrologic hydrodynamic model of the Pantanal wetland, called SIRIPLAN (Paz et al., 2011, 2014). SIRIPLAN is composed by a 1D hydrodynamic model based on a solution of the full Saint Venant equations for river networks (Tucci, 1978) coupled to a 2D raster-based inundation model, similar to the LISFLOOD-FP model (Bates et al., 2010).
The 1D model simulates the flow routing along the river drainage system, considering cross sections restricted to the main channels. The raster-based model simulates the water accumulation and the 2D propagation of inundation over the floodplains. A water exchange scheme is used to simulate channel outflows to the floodplain and from the floodplain back into the channel (Paz et al., 2011). Additionally, the vertical processes of precipitation, evapotranspiration and infiltration in the floodplain are simulated in the 2D part of SIRIPLAN, following the methods described by Paz et al. (2014).
SIRIPLAN was previously applied in the same region by Paz et al. (2011, 2014). A total of 3965 km of main river channels and 219,514 km2 of floodplains were represented by the model. Rivers were discretized in 2 km long computational reaches, and floodplains were divided in 46,741 square-grid elements of 0.02 × 0.02 degrees (approximately 2 × 2 km). The model was calibrated by comparing discharge hydrographs and water level time series at several gauging stations on the rivers Paraguay, Cuiabá, and other tributaries, and satisfactorily reproduced the hydrological regime in most of the basin. SIRIPLAN is arguably the most detailed and accurate hydrologic-hydrodynamic model that has been applied to the whole Pantanal.
In addition to daily river discharge and water level time series along the main rivers and over the floodplain, SIRIPLAN’s outputs include the amount of water that is delivered from the rivers to the floodplains, and backward. This feature is particularly interesting in the present context of evaluating the hydrological changes due to the operation of an upstream located dam, since hydrological changes may be not limited to river discharges, but possibly include river-floodplain interaction.
Assessment of Hydrological Change
For the assessment of hydrological change, we examined results from the natural and altered hydrological scenarios using the 28 out of the 32 descriptors of river regime proposed by Richter et al. (1996) in their Indicators of Hydrologic Alteration (IHA) methodology. The IHA descriptors are biologically relevant hydrological indices that cover flow magnitude, frequency, duration, timing, and rate of change believed to be essential to ecosystem health (Poff et al., 1997), and are given in Table 2. Among the 32 IHA descriptors, we observed that the four metrics related to high and low flow pulses were hypersensitive to small changes in the hydrographs due to the way the low flow and high flow thresholds are defined, so we skipped all those four descriptors of group 4 (Table 2).
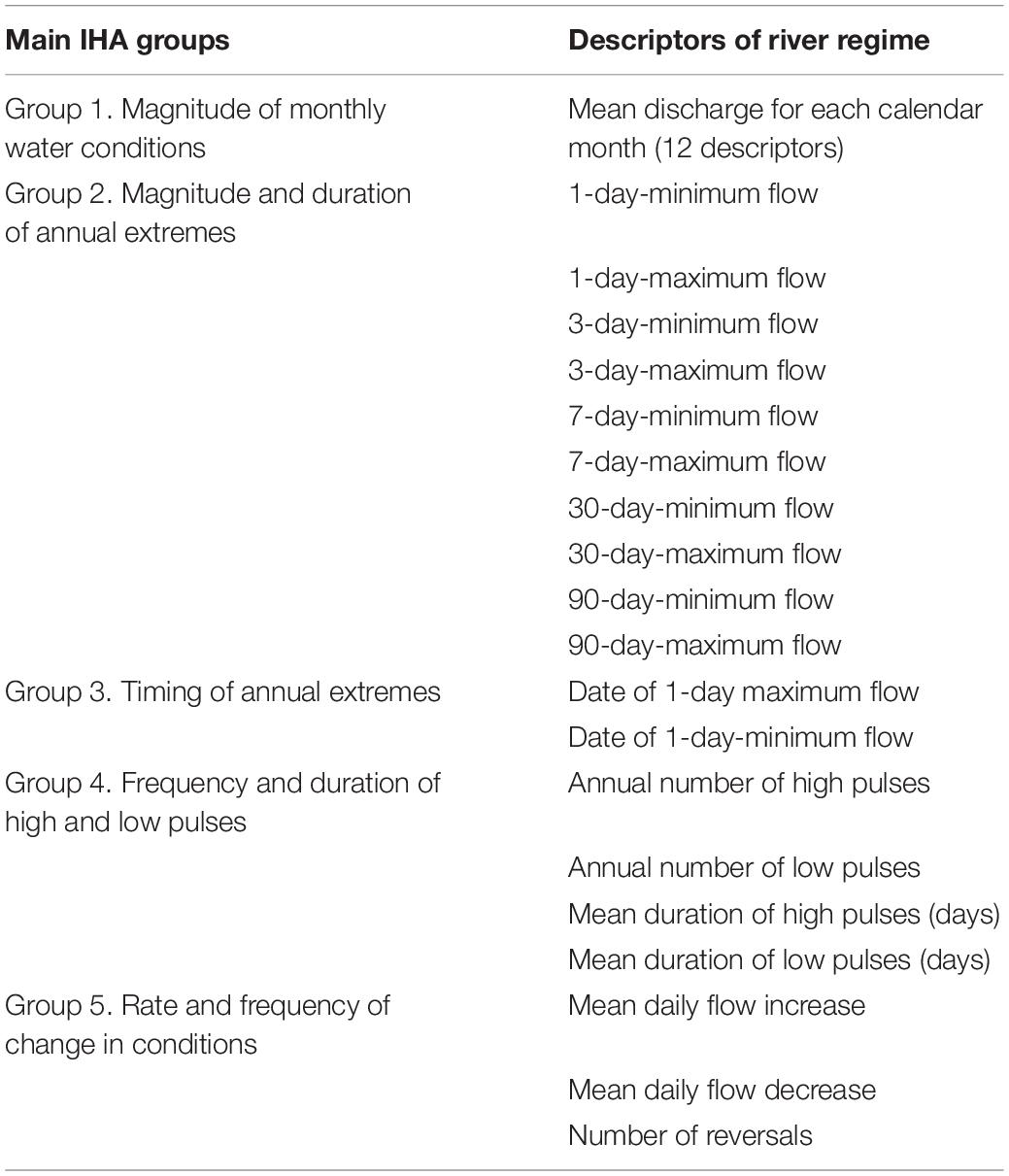
Table 2. Indicators of Hydrologic Alteration (IHA) flow regime descriptors (from Richter et al., 1996).
All the 28 metrics of groups 1, 2, 3, and 5 were obtained for every year along the simulation period (from 2003 to 2015) for the two scenarios (with and without dam operation), and its average value (mean of the 12 simulation years) in the two scenarios were compared to assess the magnitude of change, following equations 1 (for groups 1, 2, and 5) or Eq. 2 (for group 3).
were X0 is the value of the descriptor in the reference scenario (no dam operation); XD is the value of the descriptor in the altered scenario (with dam operation); J0 is the Julian day of the maximum or minimum in the reference scenario; JD is the Julian day of the maximum or minimum in the altered scenario.
We understand that applying Eq. 1 to changes in timing would lead to the wrong conclusion that a 20 days change in events typically occurring earlier during the hydrological year (lower Julian day values) represents a larger relative change than a 20 days change (delay or anticipation) occurring later in the hydrological year. Therefore, we used Eq. 2 to calculate relative change in minimum and maximum dates, as a substitute of Eq. 1, because we understand that a 20 day change should represent the same relative change, independently of the date in the reference scenario (J0), and that the worst change in timing would be a delay or anticipation of half year (or 365/2 = 182.5 days), and this should be equivalent to 100% change.
Besides, to calculate average Julian dates we used circular statistics. Circular statistics is a subfield of statistics, devoted to the development of statistical techniques for data on an angular scale, for which there is no designated zero, and the designation of high and low values is arbitrary, such as the direction of wind, the time of the day, or the day of the year, as in statistics of group 3 (Berens, 2009).
Finally, we compared results of the 28 descriptors at all river computational elements of the model downstream of the dam, resulting in 857 points where the IHA descriptors were compared. In order to present the results in a synthesized way and also in the form of maps, the averages of the alteration of all the descriptors within the same group were performed for each simulated model element.
Results
River discharge time series were calculated at river reaches 2 to 10 km long over the whole Upper Paraguay river basin during the period from 2003 to 2015, considering two scenarios: with and without dam operation. In the first scenario, intended to represent the natural or reference condition, we used naturalized streamflow at the dam site as input to the hydrological model. In the second scenario, intended to represent the impact condition, we used observed reservoir outflow time series as input to the hydrological model.
Hydrologic Alteration Along the Main River
In order to evaluate the changes between the two scenarios, hydrographs where plotted at six locations downstream of the dam (Figure 3). The results show that just downstream of the dam, the regulation effect of Manso dam operation clearly reduced high flows and increased low flows (Figure 3B). Low flows are increased from about 60 m3.s–1 to circa 130 m3.s–1, while maximum flows are reduced from up to 2,100 m3.s–1 to below 900 m3.s–1 at all times. The timing of maximum flows was also altered, with maximum flows occurring 3 to 17 days later in the scenario with dam operation.
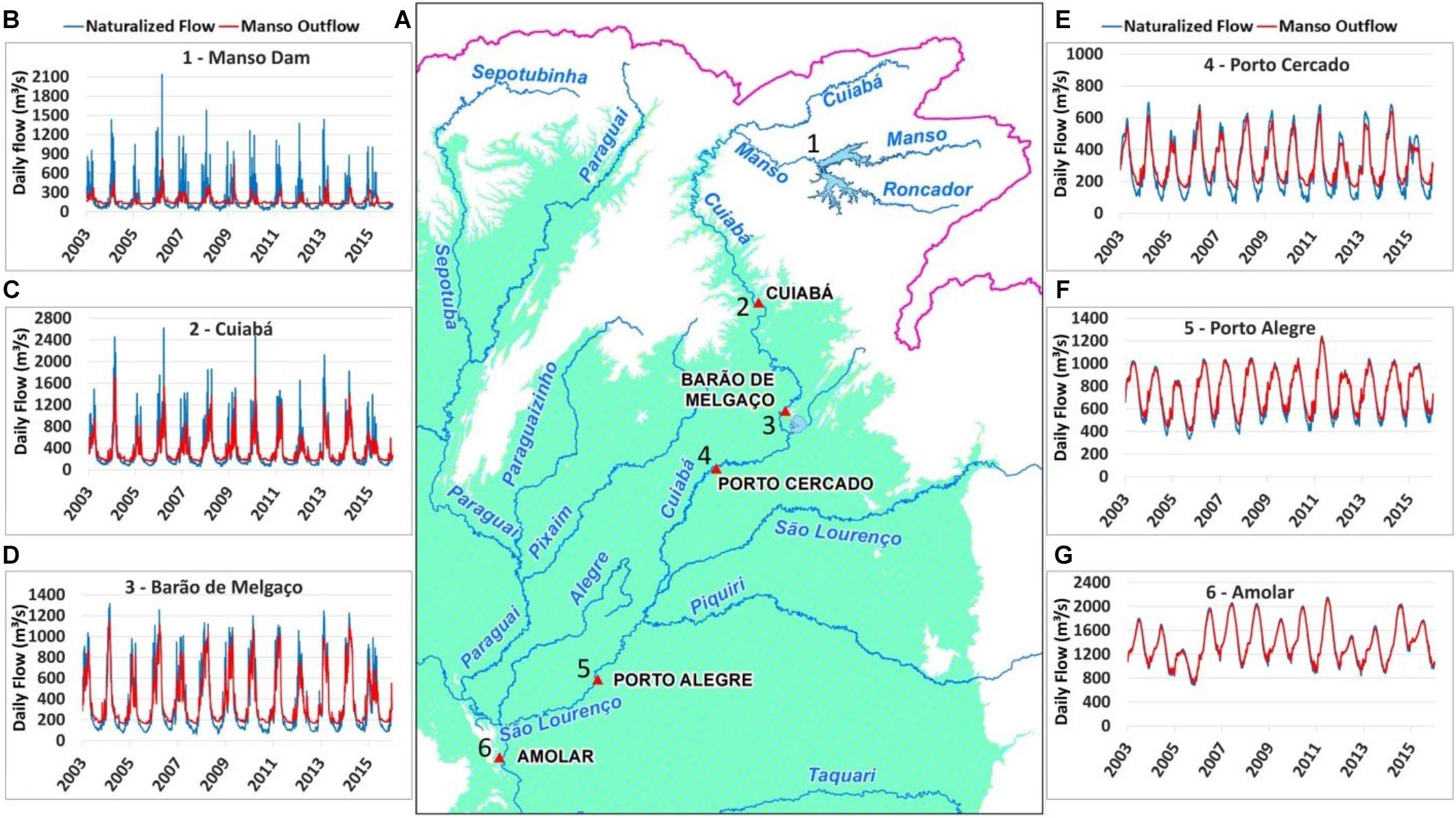
Figure 3. Location (A) of the hydrographs produced after simulation with naturalized flow (blue) and Manso outflow (red) as input condition at: Manso dam (B), Cuiabá gauge (C), Barão de Melgaço gauge (D), Porto Cercado gauge (E), Porto Alegre gauge (F), and Amolar gauge (G).
By Cuiabá city, nearly 300 km downstream of the dam, and also the point where the Cuiabá river leaves the upper region and flows into the Pantanal, the same pattern of alteration is still clearly visible (Figure 3C). Low flows are increased from about 93 to 170 m3.s–1, while maximum flows are reduced from the range 1,390–2,617 m3.s–1 to below 1,723 m3.s–1 at all times and can be as low as 650 m3.s–1. The timing of maximum flows was also altered, with maximum flows occurring 2 to 5 days later in the scenario with dam operation.
At Barão de Melgaço, approximately 420 km downstream of the dam, the hydrograph shows that changes in low flows are still clearly visible with an increase from about 90 m3.s–1 in the scenario without dam operation to about 165 m3.s–1 in the scenario with dam operation (Figure 3D). Changes in maximum flows, on the other hand, are less marked. This occurs because maximum flows are naturally decreased while the flood wave moves from Cuiabá to Barão de Melgaço, due to river outflowing to the floodplains, mainly through distributary channels. Maximum river discharges at Barão de Melgaço are in the range of 980 to 1,315 m3.s–1 in the scenario without dam operation and drop to about 750 and 1,140 m3.s–1 at the same events in the scenario with dam operation. Changes in the timing of maximum flows at Barão de Melgaço due to dam operation are lower than 5 days.
Circa 550 km downstream of the dam, at Porto Cercado, and already well within the Pantanal, changes in low flows are still clearly visible, while changes in maximum flows are slightly perceived. At this location, changes in timing of maximum flows are practically absent (Figure 3E). The same behavior is observed at Porto Alegre (Figure 3F), located 825 km downstream of the dam. At this point, dissemblance between the hydrographs are only clearly perceptible at low flows but, even so, with small differences. Finally, at Amolar, after the confluence with the Paraguay river, there is practically no difference between the hydrographs for both scenarios (Figure 3G).
It is clear in all IHA groups that changes are higher just downstream of the dam, and progressively decrease downstream, as the Cuiabá river flows into the Pantanal (Figure 4). This is due probably to both the entry of large tributaries into the impacted network, as is the case of the São Lourenço river already within the Pantanal, and due to river-floodplain interaction. It is noticeable at Table 3, which summarizes the results found regarding IHA statistics for the locations previously analyzed (Figure 3), that in January (wet season) average flows decrease more than 44% just downstream of the dam (point 1), more than 22% at Cuiabá (2) and more than 13% at Barão do Melgaço (3). August/September (dry season) average flows increase more than 140% at the dam (1), and more than 70% as far as Porto Cercado (point 4), located more than 500 km downstream of the dam.
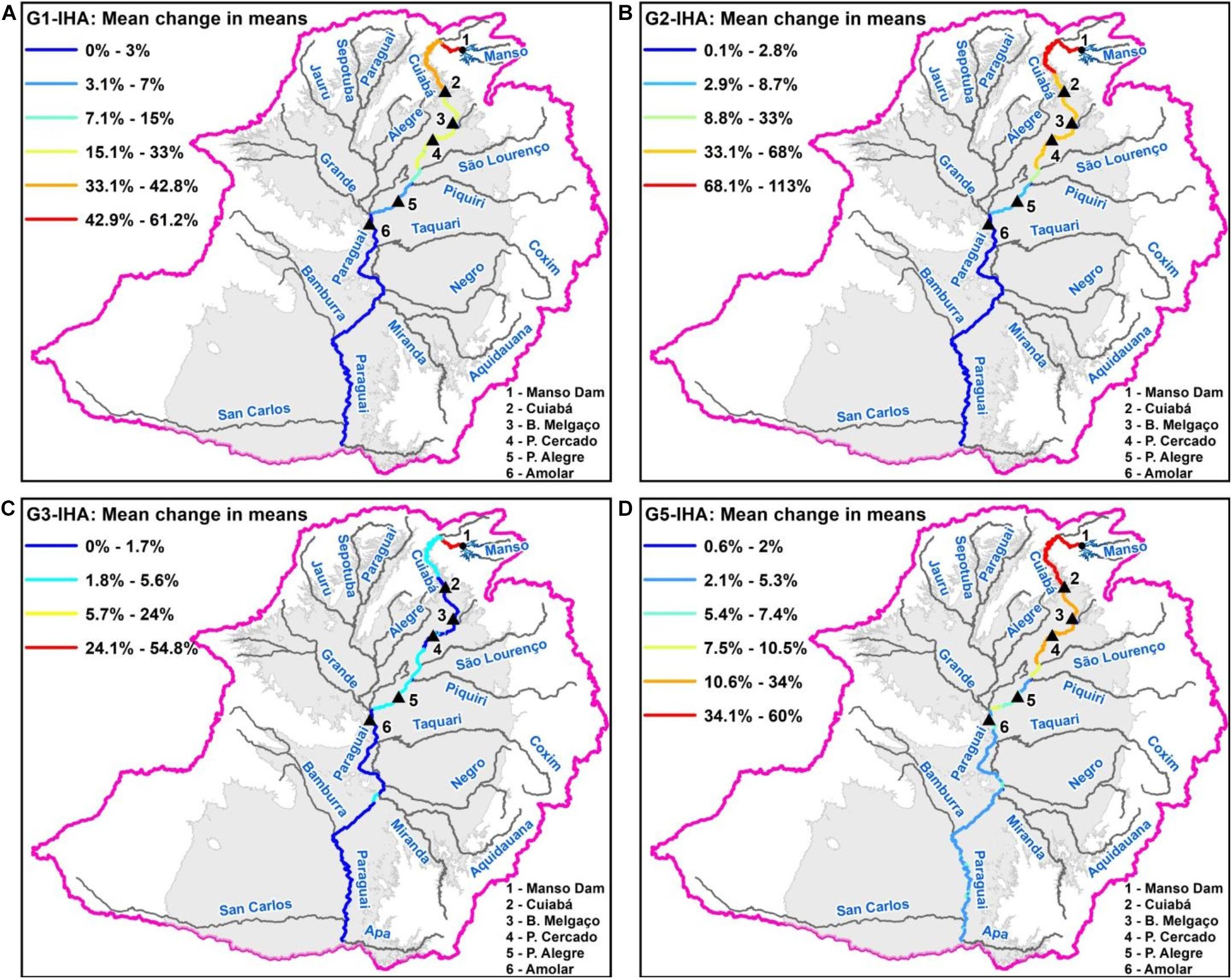
Figure 4. Mean changes in means from Groups 1 (A), 2 (B), 3 (C), and 5 (D) of IHA statistics due to Manso operation.
Minimum flows increase substantially due to dam operation as far as Porto Cercado (point 4), and are still present as far as Amolar (point 6), on the Paraguay river. Changes in timing of both minimum and maximum flows are large just downstream of the dam (point 1), but decrease rapidly with distance downstream. Rates of change decrease at all six points downstream of the dam, with greater values close to the dam, and lower values as the Cuiabá river enters the Pantanal. Nevertheless, decreases of the rates of change larger than 5% are still present at Amolar (point 6), which is on the river Paraguay, and is nearly 900 km downstream of the dam. Finally, the number of flow reversal increases as far as Porto Cercado (point 4).
Figure 4 shows how Manso dam operation changes the average descriptors from IHA along the rivers of the Upper Paraguay basin. These maps suggest that the effects of Manso dam operation are practically dissipated downstream of the points where the Cuiabá river meets the São Lourenço and Piquiri rivers.
Changes in River-Floodplain Interaction
To investigate how Manso dam operation affects the river-floodplain interaction we investigated hydrographs of the outflows from the river to the floodplain. The river-floodplain exchange flow rates simulated in both scenarios were obtained from SIRIPLAN model, with and without the operation of the dam. We selected the approximately 140 km reach between Cuiabá (point 2) and Barão do Melgaço (point 3), which was described by Paz et al. (2014) as a reach where the river mainly loses water to the floodplain, and summed all outflows from the river to the floodplain, resulting in hydrographs of river-floodplain interaction over the entire 140 km reach. River-floodplain interaction is highest during the wet season, and to illustrate this interaction we selected two outflow events that occurred in January/February: one for a relatively wet year (2010) and one for a relatively dry year (2012).
Along the reach between Cuiabá and Barão do Melgaço, from 22 February to 07 March 2010 in both scenarios (with and without Manso dam operation) the peak outflow from the river to the floodplain is above 1200 m3.s–1 in the reference scenario (no dam operation) while it is lower than 700 m3.s–1 in the altered scenario (Figure 5A). Hydrograph volume is also lower in the altered scenario by nearly 40%, meaning that river-floodplain interaction is weaker. Meanwhile, from 25 January to February 1st 2012 the outflow peak in the scenario without Manso is close to 600 m3.s–1, while in the altered scenario (with Manso dam operation) there is no outflow from river to floodplain at all (Figure 5B).
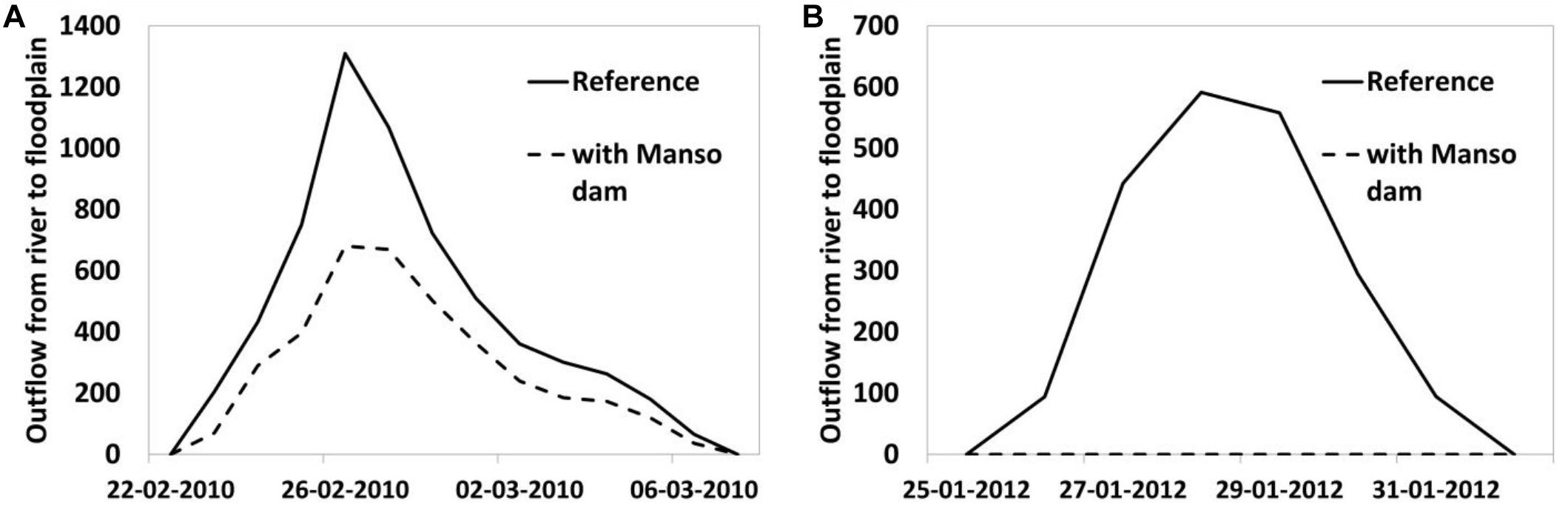
Figure 5. Outflow hydrographs from river to floodplain along the reach of the Cuiabá river between Cuiabá (point 2) and Barão de Melgaço (point 3) in the reference (no dam) and altered (with dam) scenarios: (A) wet year (2010); (B) dry year (2012).
The same pattern shown in the two described events occur every year, with outflow hydrographs being reduced by the dam operation. Average peak outflows during the years 2003 to 2015 are 64% lower in the altered scenario than in the reference scenario. This result suggests that despite high flows along the main river within the Pantanal (downstream of Cuiabá) are relatively less altered by Manso dam operation, the river-floodplain interaction seems to be severely impacted, at least along the reach between Cuiabá and Barão de Melgaço. The intensity of river-floodplain interaction in the form of outflows from the river to the floodplain seems to be lessened along an important 140 km reach, and, therefore, river-floodplain connectivity, including the flux of sediments, nutrients, fish eggs and larvae may be weakened.
Discussion
Our results show that, as expected, Manso dam has a regulation effect and decreases high flows and increases low flows. The decrease in high flows is somewhat limited to the region upstream of the Pantanal floodplain, while the increase in low flows extends well into the Pantanal. Despite the attenuation of the effects from Manso dam operation while discharge dislocates from the dam toward the Pantanal, noticeable changes can extend more than 500 km downstream of the dam. The Cuiabá river discharge within the Pantanal is more impacted by dam operation during low flows than during high flows, suggesting that the outflow from the Cuiabá to the Pantanal during floods may also be strongly affected by dam operation.
Timing of maximum and minimum flows is less affected by dam operation, except for the river reach immediately downstream of the dam. In comparison to the work by Souza et al. (2009) the present work indicates that changes in 1-day-minimum flow and 1-day-maximum flow go further than those authors indicated. Also, differently from Zeilhofer and de Moura (2009), who noticed an average flow decreases at Cuiabá gauge on the months of November (10%) and December (30%) in the period from 2002 to 2005 due to Manso operation, our findings, which englobes a different and wider period, indicate at the same location an increase of 9.7% of average flow in November and a decrease of 13.8% in December. Overall, our results are in accordance with the analysis provided by Timpe and Kaplan (2017), who analyzed the impact of Manso dam on the Manso river itself, at the Fazenda Raizama gauge. However, we found a much higher impact of Manso dam operation on minimum and maximum flows (112% in average) that those authors (40%), which may be related to the methodology and the time period used.
Regarding river-floodplain interaction in the region where the Cuiabá river flows into the Pantanal, it was strongly affected by Manso dam operation. By lowering maximum flows of the Cuiabá river, dam operation decreases the magnitude of overflows from the river to the floodplain, reducing the lateral connectivity of water. Similar observations were pointed out by Graf (2006) in the Marias River (United States) where floodplain areas were deactivated due to the operation of the Tiber Dam. To avoid this type of impact, an operating rule could be studied for the Manso dam that would mutually optimize urban flood reduction, power generation and maintaining connectivity between the river and the floodplain, especially in times where these connections are most important for fauna. At the same time, other measures to contain floods could be evaluated in the urban centers most affected by floods in the Cuiabá River in order to allow the powerplant to release more flow. These kinds of approaches have already start to be done in locations such the São Francisco river, in Brazil, where changes in river regime caused by flow regulation by dams since the 70s resulted in impacts on fishes (Pompeu and Godinho, 2006; Santos et al., 2012). Current initiatives are trying to minimize these impacts by changing operation rules of the main reservoir to give way to supplementary water releases during downstream floods (Godinho et al., 2007).
Our findings improve the assessment of spatial patterns of hydrologic alteration, giving more confidence in the assessment of magnitude and spatial extension of the effects of Manso dam in the Pantanal region. By using a model-based approach we avoided the confounding effects of climatic variability, extended the period of analysis, and improved the analysis of the spatial variability of hydrologic alteration over the river network, because the assessment of results was not restricted to river gauges. This approach and the models used could improve the assessment of hydrological changes due to dams operation in other locations, specially complex river systems with wetlands where there are planned dams or where impacts have already been noticed such as the ones caused by the Ponte de Pedra Dam (Fantin-Cruz et al., 2015), also located in the Pantanal, or the Balbina Dam (Fearnside, 1989; da Rocha et al., 2019), located in the Amazon Basin.
Data Availability Statement
Publicly available datasets were analyzed in this study. This data can be found here: https://www.ana.gov.br/sar0/MedicaoSin, http://www.snirh.gov.br/hidroweb/, and https://drive.google.com/drive/folders/1Z-PmTJ9MYNlzkkfhQkYaMjJ8MQdb1nMQ?usp=sharing.
Author Contributions
PJ: assembling and modeling the MGB model, results generation, statistics calculation, results interpretation, and generating figures and tables, writing. MM: assembling and modeling the SIRIPLAN model, results generation, and results interpretation. LR: generating IHA statistics, writing and interpretation of the results. AP: assembling and modeling the SIRIPLAN model, results generation, results interpretation. WC: assembling and modeling the MGB model, results generation and interpretation, and writing. All authors contributed to the article and approved the submitted version.
Funding
This study was financed in part by the Coordenação de Aperfeiçoamento de Pessoal de Nível Superior – Brazil (CAPES) – Finance Code 001. PJ, MM, and LR were supported by CAPES. WC and AP were supported by the Brazilian Agency CNPq (Conselho Nacional de Desenvolvimento Científico e Tecnologico) grants.
Conflict of Interest
The authors declare that the research was conducted in the absence of any commercial or financial relationships that could be construed as a potential conflict of interest.
References
Acreman, M. (2016). Environmental flows—basics for novices. Wiley Interdiscip. Rev. Water 3, 622–628. doi: 10.1002/wat2.1160
Agência Nacional da Água [ANA] (2011). ANA (Agência Nacional da Água). Qualificação de Dados Hidrológicos e Reconstituição de Vazões Naturais no País. Brasília: Agência Nacional de Águas.
Agência Nacional das Águas (2004). Error! Hyperlink Reference Not Valid. ANA (Agência Nacional das Águas). Implementação de Práticas de Gerenciamento. (Integrado)de Bacia Hidrográfica Para o Pantanal e Bacia Do Alto Paraguai NA/GEF/PNUMA/OEA: Programa de Ações Estratégicas Para o Gerenciamento Integrado do Pantanal e Bacia do Alto Paraguai. Brasília: Agência Nacional de Águas.
Agostinho, A. A., Gomes, L. C., Santos, N. C. L., Ortega, J. C. G., and Pelicice, F. M. (2016). Fish assemblages in Neotropical reservoirs: colonization patterns, impacts and management. Fish. Res. 173, 26–36. doi: 10.1016/j.fishres.2015.04.006
Agostinho, A. A., Marques, E. E., Agostinho, C. S., Almeida, D. A. D., Oliveira, R. J. D., and Melo, J. R. B. D. (2007). Fish ladder of Lajeado Dam: migrations on one-way routes? Neotrop. Ichthyol. 5, 121–130. doi: 10.1590/s1679-62252007000200005
Agostinho, A. A., Thomaz, S. M., and Gomes, L. C. (2004). Threats for biodiversity in the floodplain of the Upper Paraná River: effects of hydrological regulation by dams. Ecohydrol. Hydrobiol. 4, 255–268.
Anderson, E. P., Jenkins, C. N., Heilpern, S., Maldonado-Ocampo, J. A., Carvajal-Vallejos, F. M., Encalada, A. C., et al. (2018). Fragmentation of Andes-to-Amazon connectivity by hydropower dams. Sci. Adv. 4:eaao1642. doi: 10.1126/sciadv.aao1642
Assine, M. L., Merino, E. R., Pupim, F. N., Warren, L. V., Guerreiro, R. L., and McGlue, M. M. (2015). “Geology and geomorphology of the Pantanal basin,” in Dynamics of the Pantanal Wetland in South America, eds I. Bergier and M. Assine (Cham: Springer), 23–50. doi: 10.1007/698_2015_349
Bailly, D., Agostinho, A. A., and Suzuki, H. I. (2008). Influence of the flood regime on the reproduction of fish species with different reproductive strategies in the Cuiabá River, Upper Pantanal, Brazil. River Res. Appl. 24, 1218–1229. doi: 10.1002/rra.1147
Barletta, M., Jaureguizar, A. J., Baigun, C., Fontoura, N. F., Agostinho, A. A., Almeida-Val, V. M. F., et al. (2010). Fish and aquatic habitat conservation in South America: a continental overview with emphasis on neotropical systems. J. Fish Biol. 76, 2118–2176. doi: 10.1111/j.1095-8649.2010.02684.x
Barros, V., Chamorro, L., Coronel, G., and Baez, J. (2004). The major discharge events in the Paraguay River: magnitudes, source regions, and climate forcings. J. Hydrometeorol. 5, 1161–1170. doi: 10.1175/jhm-378.1
Barzotto, E., and Mateus, L. (2017). Reproductive biology of the migratory freshwater fish Salminus brasiliensis (Cuvier, 1816) in the Cuiabá River basin, Brazil. J. Appl. Ichthyol. 33, 415–422. doi: 10.1111/jai.13262
Bates, P. D., Horritt, M. S., and Fewtrell, T. J. (2010). A simple inertial formulation of the shallow water equations for efficient two-dimensional flood inundation modelling. J. Hydrol. 387, 33–45. doi: 10.1016/j.jhydrol.2010.03.027
Bejarano, M. D., Jansson, R., and Nilsson, C. (2018). The effects of hydropeaking on riverine plants: a review. Biol. Rev. 93, 658–673. doi: 10.1111/brv.12362
Bergier, I. (2013). Effects of highland land-use over lowlands of the Brazilian Pantanal. Sci. Total Environ. 463, 1060–1066. doi: 10.1016/j.scitotenv.2013.06.036
Bravo, J. M., Allasia, D., Paz, A. R., Collischonn, W., and Tucci, C. E. M. (2012). Coupled hydrologic-hydraulic modeling of the Upper Paraguay River basin. J. Hydrol. Eng. 17, 635–646. doi: 10.1061/(asce)he.1943-5584.0000494
Brismar, A. (2002). River systems as providers of goods and services: a basis for comparing desired and undesired effects of large dam projects. Environ. Manag. 29, 598–609. doi: 10.1007/s00267-001-0058-3
Casas-Mulet, R., Alfredsen, K., Hamududu, B., and Timalsina, N. P. (2015). The effects of hydropeaking on hyporheic interactions based on field experiments. Hydrol. Process. 29, 1370–1384. doi: 10.1002/hyp.10264
Catella, A. C., and Petrere, M. Jr. (1996). Feeding patterns in a fish community of Baia da Onça, a floodplain lake of the Aquidauana River, Pantanal, Brazil. Fish. Manag. Ecol. 3, 229–237. doi: 10.1111/j.1365-2400.1996.tb00150.x
Coelho da Silva, A. C., Fantin-Cruz, I., Marques, de Lima, Z., Maimoni, and de Figueiredo, D. (2019). Cumulative changes in water quality caused by six cascading hydroelectric dams on the Jauru River, tributary of the Pantanal floodplain. Braz. J. Water Resour. 24:e18.
Collischonn, W., Allasia, D., Da Silva, B. C., and Tucci, C. E. M. (2007). The MGB-IPH model for large-scale rainfall—runoff modelling. Hydrol. Sci. J. 52, 878–895. doi: 10.1623/hysj.52.5.878
Collischonn, W., Bravo, J. M., and Pontes, P. R. M. (2016). “Paraná (Rio de la Plata) river basin,” in Handbook of Applied Hydrology, ed. V. P. Singh (New York, NY: McGraw-Hill Education).
Collischonn, W., Tucci, C. E. M., and Clarke, R. T. (2001). Further evidence of changes in the hydrological regime of the River Paraguay: part of a wider phenomenon of climate change? J. Hydrol. 245, 218–238. doi: 10.1016/s0022-1694(01)00348-1
Costa, R. M. R. D., and Mateus, L. A. D. F. (2009). Reproductive biology of pacu Piaractus mesopotamicus (Holmberg, 1887) (Teleostei: Characidae) in the Cuiabá River Basin, Mato Grosso, Brazil. Neotrop. Ichthyol. 7, 447–458. doi: 10.1590/s1679-62252009000300012
Couto, T. B., and Olden, J. D. (2018). Global proliferation of small hydropower plants–science and policy. Front. Ecol. Environ. 16, 91–100. doi: 10.1002/fee.1746
CPRM (2011). Levantamento da Geodiversidade: Projeto Atlas Pluviométrico do Brasil, Isoietas Anuais Médias – Período 1977 a 2006. Brasília: SERVIÇO GEOLÓGICO DO BRASIL. 2.
da Rocha, M., de Assis, R. L., Piedade, M. T. F., Feitosa, Y. O., Householder, J. E., Lobo, G. D. S., et al. (2019). Thirty years after Balbina Dam: diversity and floristic composition of the downstream floodplain forest, Central Amazon, Brazil. Ecohydrology 12:e2144.
Evans, T. L., Costa, M., Telmer, K., and Silva, T. S. (2010). Using ALOS/PALSAR and RADARSAT-2 to map land cover and seasonal inundation in the Brazilian Pantanal. IEEE J. Sel. Top. Appl. Earth Obs. Remote Sens. 3, 560–575. doi: 10.1109/jstars.2010.2089042
Fan, F. M., Schwanenberg, D., Collischonn, W., and Weerts, A. (2015). Verification of inflow into hydropower reservoirs using ensemble forecasts of the TIGGE database for large scale basins in Brazil. J. Hydrol. Reg. Stud. 4, 196–227. doi: 10.1016/j.ejrh.2015.05.012
Fantin-Cruz, I., Pedrollo, O., Girard, P., Zeilhofer, P., and Hamilton, S. K. (2015). Effects of a diversion hydropower facility on the hydrological regime of the Correntes River, a tributary to the Pantanal floodplain, Brazil. J. Hydrol. 531, 810–820. doi: 10.1016/j.jhydrol.2015.10.045
Fearnside, P. M. (1989). Brazil’s Balbina Dam: environment versus the legacy of the pharaohs in Amazonia. Environ. Manag. 13, 401–423. doi: 10.1007/bf01867675
Finer, M., and Jenkins, C. N. (2012). Proliferation of hydroelectric dams in the Andean Amazon and implications for Andes-Amazon connectivity. PLoS One 7:e35126. doi: 10.1371/journal.pone.0035126
FitzHugh, T. W., and Vogel, R. M. (2011). The impact of dams on flood flows in the United States. River Res. Appl. 27, 1192–1215. doi: 10.1002/rra.1417
Fleischmann, A., Collischonn, W., Paiva, R., and Tucci, C. E. (2019). Modeling the role of reservoirs versus floodplains on large-scale river hydrodynamics. Nat. Hazards 99, 1075–1104. doi: 10.1007/s11069-019-03797-9
Fleischmann, A., Siqueira, V., Paris, A., Collischonn, W., Paiva, R., Pontes, P., et al. (2018). Modelling hydrologic and hydrodynamic processes in basins with large semi-arid wetlands. J. Hydrol. 561, 943–959. doi: 10.1016/j.jhydrol.2018.04.041
Forsberg, B. R., Melack, J. M., Dunne, T., Barthem, R. B., Goulding, M., Paiva, R. C., et al. (2017). The potential impact of new Andean dams on Amazon fluvial ecosystems. PLoS One 12:e0182254. doi: 10.1371/journal.pone.0182254
Fraser, B. (2018). Dams nudge Amazon’s ecosystems off-kilter. Science 359, 508–509. doi: 10.1126/science.359.6375.508
Furnas Centrais Elétricas, S. A. (2002). Aproveitamento Múltiplo Manso – Memória Técnica. Rio de Janeiro: Furnas Centrais Elétricas, 1070.
Girard, P., Fantin-Cruz, I., De Oliveira, S. M. L., and Hamilton, S. K. (2010). Small-scale spatial variation of inundation dynamics in a floodplain of the Pantanal (Brazil). Hydrobiologia 638, 223–233. doi: 10.1007/s10750-009-0046-9
Godinho, A. L., Kynard, B., and Martinez, C. B. (2007). Supplemental water releases for fisheries restoration in a Brazilian floodplain river: a conceptual model. River Res. Appl. 23, 947–962. doi: 10.1002/rra.1018
Gorgoglione, A., Crisci, M., Kayser, R. H., Chreties, C., and Collischonn, W. (2019). A new scenario-based framework for conflict resolution in water allocation in transboundary watersheds. Water 11:1174. doi: 10.3390/w11061174
Graf, W. L. (2006). Downstream hydrologic and geomorphic effects of large dams on American rivers. Geomorphology 79, 336–360. doi: 10.1016/j.geomorph.2006.06.022
Grill, G., Lehner, B., Thieme, M., Geenen, B., Tickner, D., Antonelli, F., et al. (2019). Mapping the world’s free-flowing rivers. Nature 569, 215–221.
Hahn, L., Agostinho, A. A., English, K. K., Carosfeld, J., Da Camara, L. F., and Cooke, S. J. (2011). Use of radiotelemetry to track threatened dorados Salminus brasiliensis in the upper Uruguay River, Brazil. Endanger. Species Res. 15, 103–114. doi: 10.3354/esr00363
Hamilton, S. K., Sippel, S. J., and Melack, J. M. (2002). Comparison of inundation patterns among major South American floodplains. J. Geophys. Res. Atmospheres 107, LBA 5-1–LBA 5-14.
Junk, W. J., Bayley, P. B., and Sparks, R. E. (1989). The flood pulse concept in river-floodplain systems. Can. J. Fish. Aquat. Sci. 106, 110–127.
Junk, W. J., and de Cunha, C. N. (2005). Pantanal: a large South American wetland at a crossroads. Ecol. Eng. 24, 391–401. doi: 10.1016/j.ecoleng.2004.11.012
Junk, W. J., de Cunha, C. N., Wantzen, K. M., Petermann, P., Strüssmann, C., Marques, M. I., et al. (2006). Biodiversity and its conservation in the Pantanal of Mato Grosso, Brazil. Aquat. Sci. 68, 278–309.
Kennen, J. G., Kauffman, L. J., Ayers, M. A., Wolock, D. M., and Colarullo, S. J. (2008). Use of an integrated flow model to estimate ecologically relevant hydrologic characteristics at stream biomonitoring sites. Ecol. Modell. 211, 57–76. doi: 10.1016/j.ecolmodel.2007.08.014
Latrubesse, E. M., Arima, E. Y., Dunne, T., Park, E., Baker, V. R., d’Horta, F. M., et al. (2017). Damming the rivers of the Amazon basin. Nature 546, 363–369. doi: 10.1038/nature22333
Lourenço, L. S., Fernandes, I. M., Penha, J., and Mateus, L. A. (2012). Persistence and stability of cichlid assemblages in neotropical floodplain lagoons. Environ. Biol. Fish. 93, 427–437. doi: 10.1007/s10641-011-9933-9
Marchese, M. R., Wantzen, K. M., and De Drago, I. E. (2005). Benthic invertebrate assemblages and species diversity patterns of the Upper Paraguay River. River Res. Appl. 21, 485–499. doi: 10.1002/rra.814
Mateus, L., Penha, J. M., and Petrere, M. (2004). Fishing resources in the rio Cuiabá basin, Pantanal do Mato Grosso, Brazil. Neotrop. Ichthyol. 2, 217–227. doi: 10.1590/s1679-62252004000400004
Medinas de Campos, M., Tritico, H. M., Girard, P., Mo, P., Hamilton, S. K., and Fantin-Cruz, I. (2020). Predicted impacts of proposed hydroelectric facilities on fish migration routes upstream from the Pantanal wetland (Brazil). River Res. Appl. 36, 452–464. doi: 10.1002/rra.3588
Mihalicz, J. E., Jardine, T. D., Baulch, H. M., and Phillips, I. D. (2019). Seasonal effects of a hydropeaking dam on a downstream benthic macroinvertebrate community. River Res. Appl. 35, 714–724.
Paes, R. P., and Brandão, J. L. B. (2013). Flood control in the Cuiabá River Basin, Brazil, with multipurpose reservoir operation. Water Resour. Manag. 27, 3929–3944. doi: 10.1007/s11269-013-0388-y
Paiva, R. C., Collischonn, W., and Tucci, C. E. M. (2011). Large scale hydrologic and hydrodynamic modeling using limited data and a GIS based approach. J. Hydrol. 406, 170–181. doi: 10.1016/j.jhydrol.2011.06.007
Pavanato, H. J., Melo-Santos, G., Lima, D. S., Portocarrero-Aya, M., Paschoalini, M., Mosquera, F., et al. (2016). Risks of dam construction for South American river dolphins: a case study of the Tapajós River. Endanger. Species Res. 31, 47–60. doi: 10.3354/esr00751
Paz, A. R., Bravo, J. M., Allasia, D., Collischonn, W., and Tucci, C. E. M. (2010). Large-scale hydrodynamic modeling of a complex river network and floodplains. J. Hydrol. Eng. 15, 152–165. doi: 10.1061/(asce)he.1943-5584.0000162
Paz, A. R., Collischonn, W., Bravo, J. M., Bates, P. D., and Baugh, C. (2014). The influence of vertical water balance on modelling Pantanal (Brazil) spatio-temporal inundation dynamics. Hydrol. Process. 28, 3539–3553. doi: 10.1002/hyp.9897
Paz, A. R., Collischonn, W., Tucci, C. E. M., and Padovani, C. R. (2011). Large-scale modelling of channel flow and floodplain inundation dynamics and its application to the Pantanal (Brazil). Hydrol. Process. 25, 1498–1516. doi: 10.1002/hyp.7926
Penha, J., Landeiro, V. L., Ortega, J. C., and Mateus, L. (2017). Interchange between flooding and drying, and spatial connectivity control the fish metacommunity structure in lakes of the Pantanal wetland. Hydrobiologia 797, 115–126. doi: 10.1007/s10750-017-3164-9
Penha, J., Mateus, L., and Lobón-Cerviá, J. (2015). Population regulation in a Neotropical seasonal wetland fish. Environ. Biol. Fish. 98, 1023–1034. doi: 10.1007/s10641-014-0336-6
Pereira, M. J., and Súarez, Y. R. (2018). Reproductive ecology of Otocinclus vittatus (Regan, 1904) in the Pantanal floodplain, upper Paraguay River basin. Braz. J. Biol. 79, 735–741. doi: 10.1590/1519-6984.191560
Petrere, M. Jr., Agostinho, A. A., Okada, E. K., and Júlio, H. F. Jr. (2002). “Review of the fisheries in the Brazilian portion of the Paraná/Pantanal basin,” in Management and Ecology of Lake and Reservoir Fisheries, ed. I. G. Cowx (Hoboken, NJ: Wiley-Blackwell), 123–143. doi: 10.1002/9780470995679.ch11
Pinho, J. B., and Marini, M. A. (2012). Using birds to set conservation priorities for Pantanal wetland forests, Brazil. Bird Conserv. Int. 22, 155–169. doi: 10.1017/s0959270911000207
Poff, N. L., Allan, J. D., Bain, M. B., Karr, J. R., Prestegaard, K. L., Richter, B. D., et al. (1997). The natural flow regime. BioScience 47, 769–784.
Poff, N. L., Richter, B. D., Arthington, A. H., Bunn, S. E., Naiman, R. J., Kendy, E., et al. (2010). The ecological limits of hydrologic alteration (ELOHA): a new framework for developing regional environmental flow standards. Freshw. Biol. 55, 147–170. doi: 10.1111/j.1365-2427.2009.02204.x
Poff, N. L., and Zimmerman, J. K. (2010). Ecological responses to altered flow regimes: a literature review to inform the science and management of environmental flows. Freshw. Biol. 55, 194–205. doi: 10.1111/j.1365-2427.2009.02272.x
Pompeu, P. D. S., and Godinho, H. P. (2006). Effects of extended absence of flooding on the fish assemblages of three floodplain lagoons in the middle São Francisco River, Brazil. Neotrop. Ichthyol. 4, 427–433. doi: 10.1590/s1679-62252006000400006
Pontes, P. R. M., Fan, F. M., Fleischmann, A. S., de Paiva, R. C. D., Buarque, D. C., Siqueira, V. A., et al. (2017). MGB-IPH model for hydrological and hydraulic simulation of large floodplain river systems coupled with open source GIS. Environ. Modell. Softw. 94, 1–20. doi: 10.1016/j.envsoft.2017.03.029
Reid, A. J., Carlson, A. K., Creed, I. F., Eliason, E. J., Gell, P. A., Johnson, P. T., et al. (2019). Emerging threats and persistent conservation challenges for freshwater biodiversity. Biol. Rev. 94, 849–873.
Richter, B., Baumgartner, J., Powell, J., and Braun, D. (1996). A method for assessing hydrological alteration within ecosystems. Conserv. Biol. 10, 1163–1174. doi: 10.1046/j.1523-1739.1996.10041163.x
Richter, B., Baumgartner, J., Wigington, R., and Braun, D. (1997). How much water does a river need? Freshw. Biol. 37, 231–249. doi: 10.1046/j.1365-2427.1997.00153.x
Ryo, M., Iwasaki, Y., Yoshimura, C., Oliver, C., and Saadvedra, V. (2015). Evaluation of spatial pattern of altered flow regimes on a river network using a distributed hydrological model. PLoS One 10:e0133833. doi: 10.1371/journal.pone.0133833
Santana, C. A., Tondato, K. K., and Súarez, Y. R. (2019). Reproductive biology of Hyphessobrycon eques (Characiformes: Characidae) in Southern Pantanal, Brazil. Braz. J. Biol. 79, 70–79. doi: 10.1590/1519-6984.176273
Santos, H. D. A., dos Santos Pompeu, P., and Kenji, D. O. L. (2012). Changes in the flood regime of São Francisco River (Brazil) from 1940 to 2006. Reg. Environ. Change 12, 123–132. doi: 10.1007/s10113-011-0240-y
Santos, R. E., Pinto-Coelho, R. M., Fonseca, R., Simões, N. R., and Zanchi, F. B. (2018). The decline of fisheries on the Madeira River, Brazil: the high cost of the hydroelectric dams in the Amazon Basin. Fish. Manag. Ecol. 25, 380–391. doi: 10.1111/fme.12305
Schmutz, S., and Moog, O. (2018). “Dams: ecological impacts and management,” in Riverine Ecosystem Management, eds S. Schmutz and J. Sendzimir (Cham: Springer), 111–127.
Schulz, C., Whitney, B. S., Rossetto, O. C., Neves, D. M., Crabb, L., de Oliveira, E. C., et al. (2019). Physical, ecological and human dimensions of environmental change in Brazil’s Pantanal wetland: synthesis and research agenda. Sci. Total Environ. 687, 1011–1027. doi: 10.1016/j.scitotenv.2019.06.023
Shimabukuro-Dias, C. K., Costa Silva, G. J. D., Ashikaga, F. Y., Foresti, F., and Oliveira, C. (2017). Molecular identification of the fish fauna from the Pantanal flood plain area in Brazil. Mitochondrial DNA Part A 28, 588–592. doi: 10.3109/24701394.2016.1149826
Shirashi, F. K. (2003). Avaliação dos Efeitos da Construção do APM Manso no Controle das Cheias nas Áreas Urbanas das Cidades de Cuiabá e Várzea Grande. Tese de Mestrado: UFRJ Rio de Janeiro, 149.
Siqueira, V. A., Fleischmann, A., Jardim, P. F., Fan, F. M., and Collischonn, W. (2016). IPH-Hydro Tools: a GIS coupled tool for watershed topology acquisition in an open-source environment. Braz. Water Resour. J. 21, 274–287. doi: 10.21168/rbrh.v21n1.p274-287
Siqueira, V. A., Paiva, R. C. D., Fleischmann, A. S., Fan, F. M., Ruhoff, A. L., Pontes, P. R. M., et al. (2018). Toward continental hydrologic–hydrodynamic modeling in South America. Hydrol. Earth Syst. Sci. 22, 4815–4842. doi: 10.5194/hess-22-4815-2018
Sorribas, M. V., Paiva, R. C., Melack, J. M., Bravo, J. M., Jones, C., Carvalho, L., et al. (2016). Projections of climate change effects on discharge and inundation in the Amazon basin. Clim. Change 136, 555–570. doi: 10.1007/s10584-016-1640-2
Souza, C. F., Collischonn, W., Tucci, C. E., and Arthington, A. H. (2009). “Preliminary assessment of dam hydrological effects in the Brazilian Pantanal,” in Proceedings of the International Symposium on Ecohydraulics, 7 (Concepcion: IAHR).
Súarez, Y. R., and Scanferla, A. F. (2016). Flood pulse are the main determinant of feeding dynamics and composition of Odontostilbe pequira (Characiformes: Characidae) in southern Pantanal, Brazil. Acta Limnol. Brasiliensia 28:e19.
Timpe, K., and Kaplan, D. (2017). The changing hydrology of a dammed Amazon. Sci. Adv. 3:e1700611. doi: 10.1126/sciadv.1700611
Tondato, K. K., Súarez, Y. R., de Fátima Mateus, L. A., Vicentin, W., and Fialho, C. B. (2018). Life history characteristics and recruitment of fish under the effect of different hydrological regimes in a tropical floodplain. Environ. Biol. Fish. 101, 1369–1384. doi: 10.1007/s10641-018-0784-5
Tucci, C. E. M. (1978). Hydraulic and Water Quality Model for a River Network. Ph.D. thesis, Colorado State University, Fort Collins, CO, 218.
Tundisi, J. G., Goldemberg, J., Matsumura-Tundisi, T., and Saraiva, A. C. (2014). How many more dams in the Amazon? Energy Policy 74, 703–708. doi: 10.1016/j.enpol.2014.07.013
Vehanen, T., Louhi, P., Huusko, A., Mäki-Petäys, A., van der Meer, O., Orell, P., et al. (2020). Behaviour of upstream migrating adult salmon (Salmo salar L.) in the tailrace channels of hydropeaking hydropower plants. Fish. Manag. Ecol. 27, 41–51. doi: 10.1111/fme.12383
Wantzen, K. M., Marchese, M. R., Marques, M. I., and Battirola, L. D. (2016). “Invertebrates in neotropical floodplains,” in Invertebrates in Freshwater Wetlands, eds D. Batzer and D. Boix (Cham: Springer), 493–524. doi: 10.1007/978-3-319-24978-0_14
Winemiller, K. O., Mcintyre, P. B., Castello, L., Fluet-Chouinard, E., Giarrizzo, T., Nam, S., et al. (2016). Balancing hydropower and biodiversity in the Amazon, Congo, and Mekong. Science 351, 128–129.
Wongchuig, S. C., de Paiva, R. C. D., Siqueira, V., and Collischonn, W. (2019). Hydrological reanalysis across the 20th century: a case study of the Amazon Basin. J. Hydrol. 570, 755–773. doi: 10.1016/j.jhydrol.2019.01.025
Zarfl, C., Lumsdon, A. E., Berlekamp, J., Tydecks, L., and Tockner, K. (2015). A global boom in hydropower dam construction. Aquat. Sci. 77, 161–170. doi: 10.1007/s00027-014-0377-0
Zeilhofer, P., and de Moura, R. M. (2009). Hydrological changes in the northern Pantanal caused by the Manso dam: impact analysis and suggestions for mitigation. Ecol. Eng. 35, 105–117. doi: 10.1016/j.ecoleng.2008.09.011
Ziober, S. R., Bialetzki, A., and Mateus, L. A. D. F. (2012). Effect of abiotic variables on fish eggs and larvae distribution in headwaters of Cuiabá River, Mato Grosso State, Brazil. Neotrop. Ichthyol. 10, 123–132. doi: 10.1590/s1679-62252012000100012
Keywords: Manso dam, indicators of hydrologic alteration, hydrological modeling, MGB, SIRIPLAN, Pantanal
Citation: Jardim PF, Melo MMM, Ribeiro LC, Collischonn W and Paz AR (2020) A Modeling Assessment of Large-Scale Hydrologic Alteration in South American Pantanal Due to Upstream Dam Operation. Front. Environ. Sci. 8:567450. doi: 10.3389/fenvs.2020.567450
Received: 29 May 2020; Accepted: 22 September 2020;
Published: 19 October 2020.
Edited by:
Vinicius Fortes Farjalla, Federal University of Rio de Janeiro, BrazilReviewed by:
Marcos Callisto, Federal University of Minas Gerais, BrazilSharad Kumar Jain, Indian Institute of Technology Roorkee, India
Copyright © 2020 Jardim, Melo, Ribeiro, Collischonn and Paz. This is an open-access article distributed under the terms of the Creative Commons Attribution License (CC BY). The use, distribution or reproduction in other forums is permitted, provided the original author(s) and the copyright owner(s) are credited and that the original publication in this journal is cited, in accordance with accepted academic practice. No use, distribution or reproduction is permitted which does not comply with these terms.
*Correspondence: Pedro Frediani Jardim, cGVkcm8uZmphckBnbWFpbC5jb20=