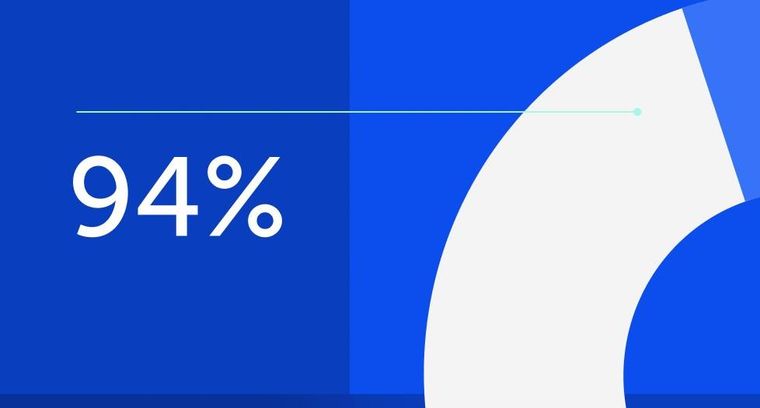
94% of researchers rate our articles as excellent or good
Learn more about the work of our research integrity team to safeguard the quality of each article we publish.
Find out more
ORIGINAL RESEARCH article
Front. Environ. Sci., 26 November 2020
Sec. Conservation and Restoration Ecology
Volume 8 - 2020 | https://doi.org/10.3389/fenvs.2020.556942
This article is part of the Research TopicProceedings of the 29th International Congress for Conservation Biology (ICCB 2019)View all 9 articles
Heterogeneous landscapes harboring mosaics of natural habitat and agriculture are increasingly gaining conservation focus in the tropics. While research on cash crops such as coffee, rubber, and oil palm has led to an understanding of their role as supplementary habitats, such has remained limited in cashew plantations despite it being a cash crop of global significance. We conducted a study to understand the occurrence of terrestrial mammal species in a mixed forest–cashew landscape in the northern Western Ghats, India. During January to April 2016, we used trail cameras to sample a total area of 25 km2 divided into 100 grid cells of 0.25 km2 each. We deployed six trail cameras for a 24 h period in each grid cell and obtained photo-captures of 11 species in forests, of which nine were detected in cashew plantations, but most species showed low capture rates. For three of these species—Indian crested porcupine, sambar, and wild pig—we modeled habitat use as a function of site-specific attributes. Wild pig showed a higher probability of use of cashew than forest, while porcupine and sambar did not show any pronounced differences between the two land uses. The probability of habitat use by sambar and porcupine was positively influenced by undergrowth but not for the wild pig. Wild pig habitat use was positively related to increased proximity of human settlements and increasing distance to forests, but the pattern was unreliable for sambar and porcupine. Our preliminary study demonstrates that a subset of terrestrial mammals in the forest makes use of cashew plantations and highlights the need for further research in forest–cashew landscapes to assess conservation opportunities.
Degradation and conversion of tropical forests to agriculture, plantations, or secondary forests reduce their biodiversity values considerably (Gibson et al., 2011). However, increasing demands on land resources means that there is limited scope for expanding terrestrial protected area coverage on privately owned land (Jenkins and Joppa, 2009; Venter et al., 2014). Thus, the long-term persistence of many mammalian species depends on their capacity to survive in modified landscapes and the ability of human societies to coexist with wildlife in such heterogeneous landscapes (Perfecto et al., 1997; Rosenzweig, 2003; Bhagwat et al., 2008; Gardner et al., 2009). Improved management of agro-plantation landscapes, especially when in proximity to native forests, is urgently required in the tropics to enhance their conservation value for native biodiversity (Azhar et al., 2011, 2013; Mone et al., 2014). Agro-forestry plantations could play a supplementary role in conservation if managed within a mosaic of natural habitats (Chazdon, 2008; Chazdon et al., 2009).
Occurrence and habitat use of wild mammals in tropical agro-plantations can vary significantly depending on factors such as proximity to remnant forest patches, native vegetation, and extent of human management or disturbance. Coffee (Coffea sp.) plantations in proximity to forests and under natural shade harbor a large subset of the diversity that occurs in adjoining forests (Bali et al., 2007; Bhagwat et al., 2008; Caudill et al., 2015). Palm oil (Elaeis guineensis) generally fails to support mammalian diversity (Yue et al., 2015), but the retention of riparian corridors, proximity to forested habitat, and undergrowth supported mammalian habitat use to some extent in oil palm (Pardo et al., 2019). Intensively managed rubber plantations (Hevea brasiliensis) also support much smaller subsets of mammalian diversity than forests (Phommexay et al., 2011; Warren-Thomas et al., 2015). This gives the impression that animal responses vary based on management regimes used within production landscapes.
Cashew (Anacardium occidantale) is a globally dominant crop type that has not received adequate research attention in terms of supplementary habitat. Cashew trees are perennial and drought resistant, growing to adult heights of 5–14 m (Dendena and Corsi, 2014) in 7–10 years. Cashew plantations often have a layer of understory bushy growth, which is periodically weeded out to make cashew nut harvesting easier. There is a crucial need to examine the role of cashew plantations as supplementary habitat, since the crop is grown in highly biodiverse regions across the world with unquantified impacts on vertebrate species (Phalan et al., 2011; Vasconcelos et al., 2015). Cashew crop is native to Brazil (Johnson, 1973) but is now widely cultivated in 33 countries across the tropics (Food and Agriculture Organization [FAO], 2019). Vietnam and India are the top producers of cashew nuts (Food and Agriculture Organization [FAO], 2019). Over 10,000 km2 of land area in India is under cashew cultivation, with the states of Maharashtra, Andhra Pradesh, and Odisha having the largest land areas under cashew cultivation (Nayak and Paled, 2018; Food and Agriculture Organization [FAO], 2019).
Of the limited studies on mammalian use of cashew plantations, chimpanzees in Africa and bats in India are perceived positively by cashew farmers, since these species only consume cashew pedicels, thereby dropping cashew nuts onto the ground and making collection easier (Hockings and Sousa, 2012; Deshpande and Kelkar, 2015). Where cashew plantations occur in proximity to forests, they may host a subset of mammals found in forests, as the plantations are relatively undisturbed except during the cashew nut harvest period of about 3 months (Vasconcelos et al., 2015). Although practices vary across regions, it appears that the general lack of intensive management could allow elements of biodiversity to persist in cashew monocultures. An improved understanding of the use of cashew monocultures as wildlife habitat could result in management guidelines to enhance wildlife persistence and may also benefit land owners through reward-based certification programs.
The aim of this study was to understand the use of cashew plantations by terrestrial wild mammals from adjoining forests in a mixed forest–cashew landscape. Specifically, the questions were: (1) Which terrestrial mammals are present in the mixed forest–cashew landscape? (2) What subset of the species in the forests also use cashew? (3) How does land cover, undergrowth, distance to the forest edge, and distance to the nearest human settlement influence the probability of habitat use by terrestrial mammals? While we initially intended to answer the latter question for all the species detected, due to limitations of data, we only used the three most-photographed species (Indian porcupine Hystrix indica, sambar Rusa unicolor, and wild pig Sus scrofa) to assess the effect of these factors on species habitat use.
The study was conducted in an area of 25 km2 comprising of mixed forest–cashew plantation habitat in the Tillari region of Dodamarg tehsil, Sindhudurg district, Maharashtra, India. We chose a study site within Sindhudurg district since it has the highest land area under cashew cultivation in Maharashtra at 344 km2, accounting for 41.1% of Maharashtra’s cashew cultivation area (Sengar et al., 2012). The study area is located in the northern part of the Western Ghats and Sri Lanka global biodiversity hotspot (Myers et al., 2000). The elevation ranges from 50 to 1,030 m above sea level (Punjabi and Rao, 2017). The vegetation comprises mainly of moist deciduous forests with semi-evergreen forests along riparian patches (Champion and Seth, 1968). The dominant tree species include Tectona grandis, Garcinia gummi-gutta, Sterculia urens, Schleichera oleosa, and riparian species such as Hydnocarpus pentandra, Homonoia riparia, and the liana Entada rheedii (Jog, 2009). The mean annual rainfall is 3,000 mm (Gathade, 2013), and mean annual temperatures vary from 16°C to 35°C (Punjabi and Rao, 2017). The study region falls on a large mammal corridor between three protected areas: Kali Tiger Reserve in the state of Karnataka, Mhadei Wildlife Sanctuary in the state of Goa, and the Radhanagari Wildlife Sanctuary in Maharashtra (Punjabi and Rao, 2017).
The area we sampled included eight villages with a total population of 5,500 people (Census of India Ministry of Home Affairs Government of India, 2011). The major land covers included forest and cashew, with relatively smaller holdings of rubber, pineapple, paddy, coconut, banana, and areca nut, which are mostly surrounded homesteads (Patil, 2010). The local population depends on forests for grazing livestock and resource extraction of firewood and medicinal plants.
We conducted camera trapping across 100 grid cells of 0.25 km2 each from January to April 2016. Each grid cell was a sampling unit, and the small size of the grid cell enabled sampling at a finer spatial scale than the species’ home range, which allowed us to draw inferences on habitat use relationships (MacKenzie, 2006). The sampling design was guided by general advice on occupancy studies (MacKenzie and Royle, 2005), which states that for rare species, it is more efficient to survey more sampling units less intensively. We assumed that since the study region is a human-dominated landscape, most terrestrial wildlife would be rare. Therefore, we surveyed more sampling units (n = 100) less intensively (24 h period). We sampled a total of 305 locations across 51 grid cells in cashew plantations and 289 locations across 49 grid cells in forests (one camera trap per location). For 48 grid cells, which contained both forest and cashew, we considered the spatially dominant land use as representative of that grid cell. In these grid cells, camera traps were also placed in the dominant land use.
We deployed six motion sensor camera traps (model Moultrie M880C White-LED or Cuddeback C2 IR) along two rows with three locations per row, each location being 125 m apart. The two rows were separated by approximately 165 m distance (Figure 1). Camera trap locations were generated using Quantum GIS (Version 2.14.2, QGIS Development Team, 2016) to ensure even spatial coverage within the cell. Each camera trap was tied to a tree at about 35 cm from the ground and directed at the nearest animal trail. Locations in proximity to roads and houses were avoided, in which case we shifted the location by a few meters to avoid camera theft. While a bare minimum of three replicates are suggested when detection probability is high (>0.5; MacKenzie and Royle, 2005), we had no prior knowledge of detection probability of wildlife. Nevertheless, we accounted for this limitation by having six camera traps in a grid cell to enhance detection and divided our data into four temporal sampling occasions. A 24 h trapping session per grid cell was divided into four temporal replicates of 6 h each. We pooled detection/non-detection data from all six camera traps in a grid cell for each replicate. The replicates consisting “0600–1159 h” and “1200–1759 h” represented daytime replicates, and those consisting “1800–2359 h” and “0000–0559 h” represented nighttime replicates. All the camera trap images were visually examined to identify animals. Capture rates were calculated as the number of photo sequences per night for both forest and cashew land uses.
Figure 1. The study area within the Tillari region of India showing major land use types and the gridded sampling design. Major land use types include forests (green), cashew plantations (yellow), rubber plantations (light orange), and built-up rural human populated areas (purple). The inset map shows the location of Tillari (box with red outline) within the state of Maharashtra (shaded in gray). The enlarged grid cell diagram on the left-hand side depicts the placement of camera traps in each grid cell.
Site-specific covariates recorded for each grid cell included percentage undergrowth cover, measured as one value of visual percentage estimation of bushy understory cover within a 3 m radius of each camera trap location. This was averaged across the six camera trap locations to obtain one value per grid cell. We also noted the dominant land use type (forest or cashew plantation) after physically surveying a grid cell. Remotely derived landscape attributes included distance to the nearest village and distance to the nearest natural forest edge (Version 2.14.2, QGIS Development Team, 2016; Grass Development Team, 2016). These were computed from the centroid of each grid cell to the boundaries of the nearest settlement/nearest forest edge from a land cover map. The land cover map was made using Google Earth Pro and verified using field observations.
Besides site-specific covariates, we also considered daytime/nighttime as a covariate for detection probability of terrestrial mammals. To do so, we obtained the time of each detection (photo capture) from the camera trap images and assigned them to either “daytime” (0600–1759 h) or “nighttime” (1800–0559 h). We also obtained the moon phase of each sampling day from Google CalendarTM (Google CalenderTM, 2016) to model detection probability of porcupines, since moonlight influences their activity (Alkon and Mitrani, 1988). A summary of all covariates has been provided in Table 1.
Table 1. Covariates used to model habitat use of porcupine, sambar, and wild pig in Tillari region from January to April 2016.
We used the single-species occupancy model (MacKenzie et al., 2002) to assess habitat use of the study species. The model estimates parameters Ψ, which represents the probability that a grid cell was used as habitat by the study species, and p, which is the probability of detection of the study species conditional on the grid cell being occupied and used (MacKenzie, 2006). The beta coefficients relate to site and survey-specific covariates for Ψ and p. Covariates that were highly correlated (Pearson’s correlation coefficient | r| > 0.7) were not included in the same model to avoid problems of multicollinearity (Dormann et al., 2013).
We constructed a set of a priori hypotheses for species with sufficient camera trap captures to model habitat use (i.e., detected in more than 25 grid cells out of 100, thus with a naive occupancy ≥ 0.25; Supplementary Materials 1, 2). We then constructed a set of a priori candidate models that specified covariate effects on both probability of habitat use (Ψ) and detection probability (p). We modeled detection probability of the study species using the time of the day (day or night) or proportion of visible moonlight (only for porcupine). Site-specific covariates were also examined for possible effects as they could influence species-level p directly (by affecting the detectability of individual animals) or indirectly (by affecting abundances and hence detectability). The covariates “habitat type” and “distance from forest edge” were correlated positively (Pearson correlation coefficient | r| = 0.78) and were therefore never included in the same model. We modeled site-specific probabilities of habitat use as linear functions of the measured covariates using the logit link function (MacKenzie et al., 2002).
We used the package “unmarked” (Fiske and Chandler, 2011) in the statistical software program R (Version 1.1.383, R Development Core Team, 2015) to fit models, which were ranked based on Akaike’s Information Criteria (Burnham and Anderson, 2004). Since no model was highly ranked over others, probability of habitat use was averaged across models that ranked higher than the null model for each species (Supplementary Material 3), weighted by each model’s Akaike weights.
From a total of 309 photo captures (175 in forests and 134 in cashew plantations), we captured a total of 11 terrestrial mammal species in the study (Supplementary Figure S1). All species were detected in the forest, and nine of these mammal species were detected in cashew plantations. Indian chevrotain and barking deer were exclusively detected in forests (Table 2). Capture rates for all species except Indian crested porcupine, Indian gray mongoose, Indian hare, and gaur were higher in forest than in cashew plantations. We had sufficient camera trap detections to determine the influence of land use type, proximity to forest edge and village, and undergrowth on the probability of habitat use by Indian crested porcupine, sambar, and wild pig. All other animals had naive occupancy <0.1.
Table 2. Camera trap capture statistics of terrestrial mammal species surveyed in Tillari region from January to April 2016.
The average probability of habitat use was ψ (SE) = 0.71 (0.02), ranging from 0.17 (0.15) to 0.91 (0.18). Average detection probability was p (SE) = 0.15 (0.02). The mean probability of habitat use in forests was slightly higher than cashew plantations [ψ (SE) in forests = 0.76 (0.03) and cashew plantations = 0.66 (0.03)]. There was a narrow range of habitat use in forest with several outliers and a wider range in cashew (Figure 2A). Habitat use increased sharply with an increase in proportion of undergrowth initially, before reaching an asymptote (Figure 2B). The effects of distance from nearest forest edge and distance from nearest human settlement were unreliable (Supplementary Figure S2).
Figure 2. Model-averaged estimates of habitat use of Indian crested porcupine as a function of (A) habitat type and (B) proportion of undergrowth.
The average probability of habitat use was ψ (SE) = 0.67 (0.01), ranging from 0.36 (0.30) to 0.79 (0.21). The average detection probability was p (SE) = 0.18 (0.01). The probabilities of habitat use were similar in forests and cashew plantations [ψ (SE) in forests = 0.70 (0.01) and cashew plantations = 0.64 (0.02); Figure 3A]. An increase in undergrowth corresponded to an initial increase in habitat use, after which it was not affected (Figure 3B). Habitat use increased with increasing distance to the nearest human settlement of up to 1 km, after which it did not change much (Figure 3C). Distance from the forest edge was unreliable in explaining habitat use of sambar (Supplementary Figure S3).
Figure 3. Model-averaged estimates of habitat use of sambar as a function of (A) habitat type, (B) proportion of undergrowth, and (C) distance to nearest human settlement.
The average probability of habitat use was ψ (SE) = 0.57 (0.03) with a range from 0.11 (0.15) to 0.96 (0.08). The average detection probability was p (SE) = 0.16 (0.07). The mean probability of habitat use was higher in cashew plantations than in forests ψ (SE) in forests = 0.45 (0.04) and cashew plantations = 0.69 (0.04); Figure 4A]. Habitat use by wild pig increased with distance from the forest edge (Figure 4B) and decreased with distance from human settlements (Figure 4C). The effect of undergrowth on habitat use of wild pig was unreliable (Supplementary Figure S4).
Figure 4. Model-averaged estimates of habitat use of wild pig as a function of (A) habitat type (B), distance from nearest forest edge, and (C) distance to nearest human settlement.
Individual species responses to human modification of habitat are complex, undermining the simplistic notion that the “matrix” is non-habitat for all species (Magioli et al., 2019). Matrix habitat quality and structural complexity determine how animals use it for movement, dispersal, or resource supplementation, thereby affecting species richness in human-modified landscapes (Brady et al., 2011). Our study reveals that nine of the 11 terrestrial mammal species that were detected in the adjoining forests made use of cashew plantations. Mammalian presence in cashew plantations could be partly attributed to the rescue effect, since the cashew plantations occurred in proximity to forests (Daily et al., 2003). The farthest distances from forest edge at which porcupine, sambar, and wild pig were detected were 0.51, 0.66, and 0.71 km, respectively. All mammal species other than porcupine, sambar, and wild pig had very low capture rates, suggesting that only few generalist species thrive in such human-modified landscapes. We discuss our findings for each of these species and what it means for forest–cashew landscapes in the developing tropics.
Porcupine showed slightly higher probability of using forests over cashew plantations, while sambar deer showed comparable probabilities of using both forests and cashew plantations. In the study area, villagers reported that both species fed on the cashew fruit, which may partly explain the use of cashew plantations by these species. The probability of habitat use by porcupine also increased with undergrowth. Porcupines being herbivorous rodents likely prefer a higher proportion of undergrowth to either avoid predation or maximize foraging benefits (Sharma, 2001). For sambar, we envisaged higher undergrowth to provide foraging benefits and cover from predators, such as tigers, dholes, and leopards, all of which are present in the study site (Punjabi and Rao, 2017). However, we observed a weak positive relationship with undergrowth for sambar. Nevertheless, preserving undergrowth in cashew plantations appears to be a positive influence on both species, as the proportion of undergrowth was similar in cashew plantations (0.42 ± 0.01) and forests (0.39 ± 0.008) during the study period.
The habitat use by sambar increased with increasing distance to human settlements, corroborating findings that sambar is sensitive to disturbance (Kushwaha et al., 2004). We did not observe any reliable patterns for the covariate “distance from nearest forest edge” at the scale of this study. Other smaller-sized deer species such as Indian chevrotain and muntjac were detected only in forests with very low naive occupancy of 0.05 and 0.02, respectively. Chevrotain habitat use is heavily dependent on understory complexity and the availability of refuges such as fallen logs, boulders, and bushy undergrowth (Sridhara et al., 2013). Chevrotain had the lowest capture rates in coffee plantations adjoining Bhadra Tiger Reserve of south India, with only five records out of nearly 1,900 records of signs and sightings of 28 mammalian species (Bali et al., 2007). Likewise, muntjac has been reported in coffee plantations in two sites of the Western Ghats in low abundances (Bali et al., 2007; Sridhar et al., 2008). The lack of detections of chevrotain and muntjac in cashew plantations could perhaps be attributed to their forest specificity or low abundances in modified land uses due to hunting.
Wild pig used cashew more than forests, away from forest edges and near human settlements. Wild pigs are known to use human-modified spaces (Madhusudan, 2003; Chauhan et al., 2009; Thurfjell et al., 2009) and raid agricultural fields for food (Massei et al., 1997; Keuling et al., 2008; Thurfjell, 2011). At the same time, wild pigs may also choose areas close to human settlements to avoid predation (Muhly et al., 2011). Tigers hunt wild pigs, but they are sensitive to changes in habitat and avoid human-disturbed areas (Bhattacharjee et al., 2015). In Southeast Asia, wild pigs can become hyper-abundant in oil palm plantations due to food availability and low predator densities (Luskin et al., 2017). Punjabi and Rao (2017) also found higher abundances of wild pig near agricultural areas in the Tillari landscape. Wild pigs likely use cashew plantations near human settlements and away from forests for the dual benefits of access to cashew pedicels and nuts as a food source and to avoid predation by large carnivores.
Detection probability for all study species was comparable across daytime and nighttime (Supplementary Material 4). Porcupine is a nocturnal species (Sharma, 2001), while sambar and wild pig are not strictly nocturnal, and wild pig shows cathemeral activity in disturbed patches (van Schaik and Griffiths, 1996; Keuling et al., 2008). The study species in our study showed mostly crepuscular and nocturnal activity, although we captured them during daytime as well. Mammals outside forested spaces may alter activity times to avoid predation and interactions with humans (Shamoon et al., 2018).
Despite low capture rates, it is evident that the mammal species make use of cashew plantations adjoining forests. One caveat is that our sampling period (January to April) coincided with the cashew fruiting season, possibly resulting in higher captures of the three focal species that reportedly consume cashew pedicels and nuts. Future studies can examine seasonal habitat use of mammals across fruiting and non-fruiting seasons for a comprehensive understanding of cashew as supplementary habitat. Combining such information with data on crop loss trends across seasons can aid in prioritizing seasonal crop protection efforts.
Grown across biodiverse regions, cashew is an income source for small land holders in many tropical countries. Given limited research on this subject, our study provides evidence of terrestrial mammals using cashew plantations adjoining forests. To a small extent, our study demonstrates the potential of cashew plantations as supplementary habitat along remnant native forests. The importance of conserving forest patches within modified landscapes to ensure persistence of mammalian diversity is undeniable (Bali et al., 2007; Pardo et al., 2019); however, benefit-sharing mechanisms that enable biodiversity conservation through livelihood sustenance can foster improved coexistence. Similar studies conducted across taxa in landscapes harboring mixed forest–cashew land uses are needed to develop better management mechanisms and develop certification schemes for shared landscapes.
The raw data supporting the conclusions of this article will be made available by the authors without undue reservation upon request.
Permits for this study were issued (No. Desk-5/wildlife/15-16/1489) by the Office of the Chief Conservator of Forests (Territorial), Kolhapur, Maharashtra Forest Department, India. The data collected was purely observational and did not use any invasive sampling techniques.
AR, GP, and AK contributed to the study conceptualization and design. AR contributed to the fieldwork and data collection. AR, GP, DJ, and AK contributed to the data analysis and interpretation and manuscript writing. All authors contributed to the article and approved the submitted version.
Funding for this work was provided by core grants to NCBS-TIFR by the Department of Atomic Energy (India) and a program grant from the Tata Trusts. AR received support from the Tier 1 project grant: Singaporean Ministry of Education Tier 1 RG145/19. This work comprises EOS contribution number 339.
The authors declare that the research was conducted in the absence of any commercial or financial relationships that could be construed as a potential conflict of interest.
We wish to thank the Maharashtra Forest Department for research permit (No. Desk-5/wildlife/15-16/1489) and Tillari Biodiversity Research Trust for logistic support. AR wishes to thank Mr. Narayan Desai, Mr. Tanaji Desai and family, and all members of Hewale village for field assistance and immense logistic support. Fieldwork was supported by M. Naik, P. Desai, A. Karnik, P. Agarwal, A. Sutar, T. Desai, and Pradeep R. We thank J. Ratnam, M. Gangal, N. Prakash, C. Home, R. Bhalla, C. Desai, and A. Borker for the support extended throughout. AR thanks S. W. Smith and J. S. H. Lee for comments that improved the manuscript. GP was supported by the Wildlife Research Conservation Society, Pune, and the Rufford Foundation during data collection. We thank the reviewers whose suggestions greatly improved this manuscript.
The Supplementary Material for this article can be found online at: https://www.frontiersin.org/articles/10.3389/fenvs.2020.556942/full#supplementary-material
Alkon, P. U., and Mitrani, D. S. (1988). Influence of season and moonlight on temporal-activity patterns of Indian crested porcupines (Hystrix indica). J. Mammal. 69, 71–80. doi: 10.2307/1381749
Azhar, B., Lindenmayer, D., Wood, J., Fischer, J., Manning, A., McElhinny, C., et al. (2013). Contribution of illegal hunting, culling of pest species, road accident and feral dogs to biodiversity loss in established oil-palm landscapes. Wildl. Res. 40, 1–9. doi: 10.1071/wr12036
Azhar, B., Lindenmayer, D. B., Wood, J., Fischer, J., Manning, A., McElhinny, C., et al. (2011). The conservation value of oil palm plantation estates, smallholdings and logged peat swamp forest for birds. For. Ecol. Manage. 262, 2306–2315. doi: 10.1016/j.foreco.2011.08.026
Bali, A., Kumar, A., and Krishnaswamy, J. (2007). The mammalian communities in coffee plantations around a protected area in the Western Ghats, India. Biol. Conserv. 139, 93–102. doi: 10.1016/j.biocon.2007.06.017
Bhagwat, S. A., Willis, K. J., Birks, H. J. B., and Whittaker, R. J. (2008). Agroforestry: a refuge for tropical biodiversity? Trends Ecol. Evol. 23, 261–267. doi: 10.1016/j.tree.2008.01.005
Bhattacharjee, S., Kumar, V., Chandrasekhar, M., Malviya, M., Ganswindt, A., Ramesh, K., et al. (2015). Glucocorticoid stress responses of reintroduced tigers in relation to anthropogenic disturbance in Sariska tiger reserve in India. PLoS One 10:e0127626. doi: 10.1371/journal.pone.0127626
Brady, M. J., McAlpine, C. A., Possingham, H. P., Miller, C. J., and Baxter, G. S. (2011). Matrix is important for mammals in landscapes with small amounts of native forest habitat. Landsc. Ecol. 26, 617–628. doi: 10.1007/s10980-011-9602-6
Burnham, K. P., and Anderson, R. P. (2004). Multimodel inference: understanding AIC and BIC in model selection. Sociol. Methods Res. 33, 261–304. doi: 10.1177/0049124104268644
Caudill, S. A., DeClerck, F. J. A., and Husband, T. P. (2015). Connecting sustainable agriculture and wildlife conservation: does shade coffee provide habitat for mammals? Agric. Ecosyst. Environ. 199, 85–93. doi: 10.1016/j.agee.2014.08.023
Census of India Ministry of Home Affairs Government of India (2011). Dodamarg Taluka-Sindhudurg. http://www.census2011.co.in/data/subdistrict/4282-dodamarg-sindhudurg-maharashtra.html (accessed October 27, 2018).
Champion, H. G., and Seth, S. K. (1968). A Revised Survey of the Forest Types of India. New Delhi: Manager of Publications.
Chauhan, N. P. S., Barwal, K. S., and Kumar, D. (2009). Human-wild pig conflict in selected states in India and mitigation strategies. Acta Silvatica Lignaria Hungarica 5, 189–197.
Chazdon, R. L. (2008). Beyond deforestation: restoring forests and ecosystem services on degraded lands. Science 320, 1458–1460. doi: 10.1126/science.1155365
Chazdon, R. L., Harvey, C. A., Komar, O., Griffith, D. M., Ferguson, B. G., Martínez−Ramos, M., et al. (2009). Beyond reserves: a research agenda for conserving biodiversity in human−modified tropical landscapes. Biotropica 41, 142–153. doi: 10.1111/j.1744-7429.2008.00471.x
Daily, G. C., Ceballos, G., Pacheco, J., Suzán, G., and Sánchez−Azofeifa, A. (2003). Countryside biogeography of neotropical mammals: conservation opportunities in agricultural landscapes of Costa Rica. Conserv. Biol. 17, 1814–1826. doi: 10.1111/j.1523-1739.2003.00298.x
Dendena, B., and Corsi, S. (2014). Cashew, from seed to market-a review. Agron. Sustain. Dev. 34, 753–772. doi: 10.1007/s13593-014-0240-7
Deshpande, K., and Kelkar, N. (2015). How do fruit bat seed shadows benefit agroforestry? Insights from local perceptions in Kerala, India. Biotropica 47, 654–659. doi: 10.1111/btp.12275
Dormann, C. F., Elith, J., Bacher, S., Buchmann, C., Carl, G., Carré, G., et al. (2013). Collinearity: a review of methods to deal with it and a simulation study evaluating their performance. Ecography 36, 27–46. doi: 10.1111/j.1600-0587.2012.07348.x
Fiske, I., and Chandler, R. (2011). Unmarked: an R package for fitting hierarchical models of wildlife occurrence and abundance. J. Stat. Softw. 43, 1–23.
Food and Agriculture Organization [FAO] (2019). FAOSTAT. Available online at: http://faostat.fao.org (accessed March 1, 2020).
Gardner, T. A., Barlow, J., Chazdon, R., Ewers, R. M., Harvey, C. A., Peres, C. A., et al. (2009). Prospects for tropical forest biodiversity in a human−modified world. Ecol. Lett. 12, 561–582. doi: 10.1111/j.1461-0248.2009.01294.x
Gathade, P. D. (2013). Tourism Development in Dodamarg Taluka-A Geographical Analysis. Ph. D thesis, Tilak Maharashtra Vidyapeeth, Pune.
Gibson, L., Lee, T. M., Koh, L. P., Brook, B. W., Gardner, T. A., Barlow, J., et al. (2011). Primary forests are irreplaceable for sustaining tropical biodiversity. Nature 478, 378–381. doi: 10.1038/nature10425
Google CalenderTM (2016). Available online at: https://calendar.google.com/calendar (accessed June 1, 2016).
Grass Development Team (2016). Geographic Resources Analysis Support System (GRASS). Software, Version 7.0.4. Open Source Geospatial Foundation. Electronic document. Available online at: http://grass.osgeo.org (accessed May 1, 2016).
Hockings, K. J., and Sousa, C. (2012). Differential utilization of cashew—a low-conflict crop—by sympatric humans and chimpanzees. Oryx 46, 375–381. doi: 10.1017/s003060531100130x
Jenkins, C. N., and Joppa, L. (2009). Expansion of the global terrestrial protected area system. Biol. Conserv. 142, 2166–2174. doi: 10.1016/j.biocon.2009.04.016
Jog, S. K. (2009). Sahyadris – Flora and Ethnobotany. Department of Biology, The University of Texas at Tyler. A Report Submitted in Fulfilment of the William L. Brown Fellowship 2007-2008,1-34. Available online at: https://www.researchgate.net/publication/238080269_SAHYADRIS_-_FLORA_AND_ETHNOBOTANY_ (accessed July 10, 2016).
Johnson, D. (1973). The botany, origin, and spread of the cashew Anacardium occidentale L. J. Plant. Crops 1, 1–7.
Keuling, O., Stier, N., and Roth, M. (2008). How does hunting influence activity and spatial usage in wild boar Sus scrofa L.? Eur. J. Wildl. Res. 54, 729–737. doi: 10.1007/s10344-008-0204-9
Kushwaha, S. P. S., Khan, A., Habib, B., Quadri, A., and Singh, A. (2004). Evaluation of sambar and muntjak habitats using geostatistical modelling. Curr. Sci. 86, 1390–1400.
Luskin, M. S., Brashares, J. S., Ickes, K., Sun, I. F., Fletcher, C., Wright, S. J., et al. (2017). Cross-boundary subsidy cascades from oil palm degrade distant tropical forests. Nat. Commun. 8:2213.
MacKenzie, D. I. (2006). Modeling the probability of resource use: the effect of, and dealing with, detecting a species imperfectly. J. Wildl. Manage. 70, 367–374. doi: 10.2193/0022-541x(2006)70[367:mtporu]2.0.co;2
MacKenzie, D. I., Nichols, J. D., Lachman, G. B., Droege, S., Royle, J. A., and Langtimm, C. A. (2002). Estimating site occupancy rates when detection probabilities are less than one. Ecology 83, 2248–2255. doi: 10.1890/0012-9658(2002)083[2248:esorwd]2.0.co;2
MacKenzie, D. I., and Royle, J. A. (2005). Designing occupancy studies: general advice and allocating survey effort. J. Appl. Ecol. 42, 1105–1114. doi: 10.1111/j.1365-2664.2005.01098.x
Madhusudan, M. D. (2003). Living amidst large wildlife: livestock and crop depredation by large mammals in the interior villages of Bhadra Tiger Reserve, South India. Environ. Manage. 31, 466–475. doi: 10.1007/s00267-002-2790-8
Magioli, M., Moreira, M. Z., Fonseca, R. C. B., Ribeiro, M. C., Rodrigues, M. G., and de Barros, K. M. P. M. (2019). Human-modified landscapes alter mammal resource and habitat use and trophic structure. Proc. Natl. Acad. Sci. 116, 18466–18472. doi: 10.1073/pnas.1904384116
Massei, G., Genov, P. V., Staines, B. W., and Gorman, M. L. (1997). Factors influencing home range and activity of wild boar (Sus scrofa) in a Mediterranean coastal area. J. Zool. 242, 411–423. doi: 10.1111/j.1469-7998.1997.tb03845.x
Mone, S., Kusha, K. M., Jathanna, D., Ali, M., and Goel, A. (2014). Comparison of insect biodiversity between organic and conventional plantations in Kodagu. Karnataka, India. J. Threat. Taxa 6, 6186–6194. doi: 10.11609/jott.o3778.6186-94
Muhly, T. B., Semeniuk, C., Massolo, A., Hickman, L., and Musiani, M. (2011). Human activity helps prey win the predator-prey space race. PLoS One 6:e17050. doi: 10.1371/journal.pone.0017050
Myers, N., Mittermeier, R. A., Mittermeier, C. G., da Fonseca, G. A., and Kent, J. (2000). Biodiversity hotspots for conservation priorities. Nature 403, 853–858. doi: 10.1038/35002501
Nayak, M., and Paled, M. (2018). Trends in area, production, yield and export-import of cashew in India an economic analysis. Int. J. Curr. Microbiol. Appl. Sci. 7, 1088–1098. doi: 10.20546/ijcmas.2018.712.135
Pardo, L. E., Campbell, M. J., Cove, M. V., Edwards, W., Clements, G. R., and Laurance, W. F. (2019). Land management strategies can increase oil palm plantation use by some terrestrial mammals in Colombia. Sci. Rep. 9:7812.
Patil, R. (2010). Geographical Analysis of Cashewnut Processing Industry in the Sindhudurg District, Maharashtra. Ph. D thesis, Tilak Maharashtra Vidyapeeth, Pune.
Perfecto, I., Vandermeer, J., Hanson, P., and Cartín, V. (1997). Arthropod biodiversity loss and the transformation of a tropical agro-ecosystem. Biodivers. Conserv. 6, 935–945.
Phalan, B., Onial, M., Balmford, A., and Green, R. E. (2011). Reconciling food production and biodiversity conservation: land sharing and land sparing compared. Science 333, 1289–1291. doi: 10.1126/science.1208742
Phommexay, P., Satasook, C., Bates, P., Pearch, M., and Bumrungsri, S. (2011). The impact of rubber plantations on the diversity and activity of understorey insectivorous bats in southern Thailand. Biodivers. Conserv. 20, 1441–1456. doi: 10.1007/s10531-011-0036-x
Punjabi, G. A., and Rao, M. K. (2017). Large herbivore populations outside protected areas in the human-dominated Western Ghats, India. Mammal. Biol. 87, 27–35. doi: 10.1016/j.mambio.2017.05.004
QGIS Development Team (2016). QGIS Geographic Information System. Open Source Geospatial Foundation Project. Available online at: http://www.qgis.org (accessed May 1, 2016).
R Development Core Team (2015). R: A Language and Environment for Statistical Computing. Vienna: R Foundation for Statistical Computing.
Rosenzweig, M. L. (2003). Reconciliation ecology and the future of species diversity. Oryx 37, 194–205. doi: 10.1017/s0030605303000371
Sengar, S. H., Mohod, A. G., Khandetod, Y. P., Shrirame, H. Y., and Deshmukh, A. S. (2012). Performance evaluation of kiln for cashew nut shell carbonization and liquid. Int. J. Energy Eng. 2, 78–85. doi: 10.5923/j.ijee.20120203.04
Shamoon, H., Maor, R., Saltz, D., and Dayan, T. (2018). Increased mammal nocturnality in agricultural landscapes results in fragmentation due to cascading effects. Biol. Conserv. 226, 32–41. doi: 10.1016/j.biocon.2018.07.028
Sharma, D. (2001). Estimating the density of porcupines in semi-arid Sariska valley, western India. J. Bombay Nat. Hist. Soc. 98, 161–168.
Sridhar, H., Raman, T. R. S., and Mudappa, D. (2008). Mammal persistence and abundance in tropical rainforest remnants in the southern Western Ghats, India. Curr. Sci. 94, 748–757.
Sridhara, S., Edgaonkar, A., and Kumar, A. (2013). Understorey structure and refuges from predators influence habitat use by a small ungulate, the Indian chevrotain (Moschiola indica) in Western Ghats, India. Ecol. Res. 28, 427–433. doi: 10.1007/s11284-013-1031-3
Thurfjell, H. (2011). Spatial Behaviour of Wild Boar. Ph. D thesis, Swedish University of Agricultural Sciences, Uppsala.
Thurfjell, H., Ball, J., Åhlén, P.-A., Kornacher, P., Dettki, H., and Sjöberg, K. (2009). Habitat use and spatial patterns of wild boar Sus scrofa (L.): agricultural fields and edges. Eur. J. Wildl. Res. 55, 517–523. doi: 10.1007/s10344-009-0268-1
van Schaik, C. P., and Griffiths, M. (1996). Activity periods of Indonesian rain forest mammals. Biotropica 28, 105–112. doi: 10.2307/2388775
Vasconcelos, S., Rodrigues, P., Palma, L., Mendes, L. F., Palminha, A., Catarino, L., et al. (2015). Through the eye of a butterfly: assessing biodiversity impacts of cashew expansion in West Africa. Biol. Conserv. 191, 779–786. doi: 10.1016/j.biocon.2015.08.032
Venter, O., Fuller, R. A., Segan, D. B., Carwardine, J., Brooks, T., Butchart, S. H., et al. (2014). Targeting global protected area expansion for imperiled biodiversity. PLoS Biol. 12:1001891. doi: 10.1371/journal.pbio.1001891
Warren-Thomas, E., Dolman, P. M., and Edwards, D. P. (2015). Increasing demand for natural rubber necessitates a robust sustainability initiative to mitigate impacts on tropical biodiversity. Conserv. Lett. 8, 230–241. doi: 10.1111/conl.12170
Keywords: camera-trapping, conservation, Hystrix indica, Rusa unicolor, Sus scrofa, occupancy models, tropical forests, Western Ghats
Citation: Rege A, Punjabi GA, Jathanna D and Kumar A (2020) Mammals Make Use of Cashew Plantations in a Mixed Forest–Cashew Landscape. Front. Environ. Sci. 8:556942. doi: 10.3389/fenvs.2020.556942
Received: 29 April 2020; Accepted: 26 October 2020;
Published: 26 November 2020.
Edited by:
John A. Cigliano, Cedar Crest College, United StatesReviewed by:
Phuntsho Thinley, Ugyen Wangchuck Institute for Conservation and Environment (UWICE), BhutanCopyright © 2020 Rege, Punjabi, Jathanna and Kumar. This is an open-access article distributed under the terms of the Creative Commons Attribution License (CC BY). The use, distribution or reproduction in other forums is permitted, provided the original author(s) and the copyright owner(s) are credited and that the original publication in this journal is cited, in accordance with accepted academic practice. No use, distribution or reproduction is permitted which does not comply with these terms.
*Correspondence: Anushka Rege, YW51c2hrYXJlZ2UwMjEwQGdtYWlsLmNvbQ==; cmVnZTAwMDJAZS5udHUuZWR1LnNn
†ORCID: Anushka Rege, orcid.org/0000-0002-8383-0258; Girish Arjun Punjabi, orcid.org/0000-0001-5910-2421; Devcharan Jathanna, orcid.org/0000-0002-7962-4582
Disclaimer: All claims expressed in this article are solely those of the authors and do not necessarily represent those of their affiliated organizations, or those of the publisher, the editors and the reviewers. Any product that may be evaluated in this article or claim that may be made by its manufacturer is not guaranteed or endorsed by the publisher.
Research integrity at Frontiers
Learn more about the work of our research integrity team to safeguard the quality of each article we publish.