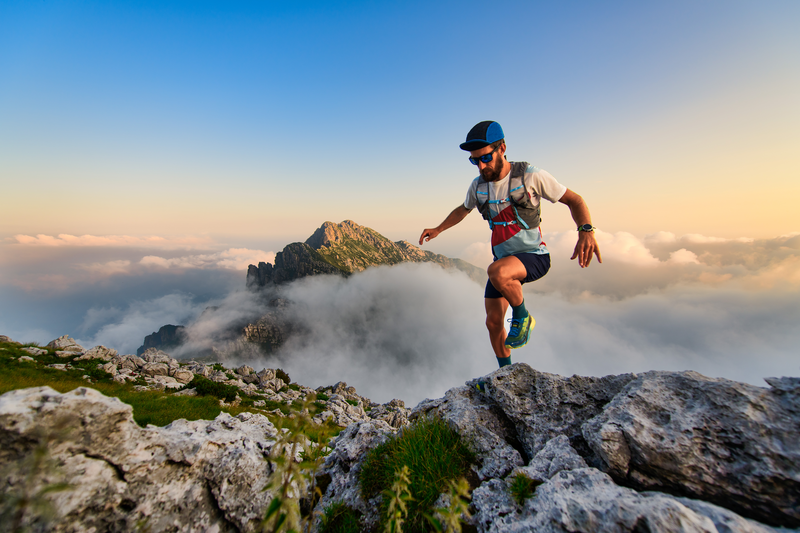
94% of researchers rate our articles as excellent or good
Learn more about the work of our research integrity team to safeguard the quality of each article we publish.
Find out more
HYPOTHESIS AND THEORY article
Front. Environ. Sci. , 30 March 2020
Sec. Soil Processes
Volume 8 - 2020 | https://doi.org/10.3389/fenvs.2020.00028
This article is part of the Research Topic Assessment and Modeling of Soil Functions or Soil-Based Ecosystem Services: Theory and Applications to Practical Problems View all 12 articles
As a critical interface in the environment, soils can provide a wide range of ecosystem services (ES). However, while there is growing demand to assess soil ES from agricultural systems, considering them in land management strategies remains a challenge. Indeed, because of the difficulty in relating soil properties to ES, soil ES are still not fully considered in the territorial planning decision process. Through a comprehensive approach based on soil processes, an assessment framework is proposed to make soil ES understandable and usable by actors of territorial planning. This assessment framework is based on a conceptual model that is then developed into an operational framework. The conceptual model, which is supported by a literature review, relates agricultural soil ES to common socio-economic development challenges. The operational framework is based on the development of soil ES modeling and enables comparison of soil management options, which provides information to help choose among planning scenarios. This soil ES assessment framework, relating soil science to territorial governance, should improve integration of soil ES into decision making in a territorial planning context and support sustainable socio-economic development.
Since the seminal publication about the ecosystem services (ES) concept (Costanza et al., 1997) and its widespread expansion through the Millennium Ecosystem Assessment (MEA, 2005), the ES concept has continually evolved (Costanza et al., 2017). It is recognized that human activities such as agriculture depend on and strongly influence multiple ES (MEA, 2005; Therond et al., 2017; Bommarco et al., 2018). Several ES that influence human well-being (e.g., food production, water flow and/or quality regulation, climate mitigation) have been shown to be directly affected by soil-related ES (Bouma, 2014; McBratney et al., 2014; Adhikari and Hartemink, 2016), referred to here as soil ES. These results have increased policy makers' awareness that ES, especially those involving soil processes and functions, should be explicitly considered in territorial planning (Breure et al., 2012; Albert et al., 2016; Drobnik et al., 2018), i.e., the process developed by public and private entities to influence the distribution of people and activities within territories of various sizes (a city, a county, a watershed, a metropolitan area).
Due to its interest to research and policy communities, there has been much debate on certain critical conceptual and operational issues, such as the following:
1) defining the related key terms, such as ecosystem processes, functions vs. services, goods, benefits vs. contributions (Boyd and Banzhaf, 2007; Wallace, 2007; Fisher et al., 2009)
2) classifying ES through international initiatives (TEEB, 2012; CICES, 2018; IPBES, 2018)
3) understanding and representing relations between ES and human well-being (Dominati et al., 2010; Haines-Young and Potschin, 2010; Potschin-Young et al., 2018)
4) developing assessment methods (Jónsson and Davíðsdóttir, 2016; Burkhard and Maes, 2017; Englund et al., 2017)
5) exploring the accessibility and usefulness of the ES concept to better inform territorial planning policies (de Groot et al., 2010; Hatton MacDonald et al., 2014; Ruckelshaus et al., 2015; Albert et al., 2016; Posner et al., 2016).
As a critical interface in the environment, soils ensure the provision of a wide range of ES (see Adhikari and Hartemink, 2016 and Jónsson and Davíðsdóttir, 2016 for reviews) through complex and highly time- and space-dependent feedbacks and interconnections among above- and below-ground ecosystem components (Dominati et al., 2010; Birgé et al., 2016). The European Commission (EC) (2006) has recognized soils as crucial to support humanity's capacity “to produce food, prevent droughts and flooding, stop biodiversity loss, and tackle climate change.” Nevertheless, soils are highly subject to degradation, including “erosion, organic matter decline, salinization, compaction and landslides.” Among terrestrial ecosystems, agricultural systems are probably the most concerned by these threats. Indeed, they face major societal (e.g., food security) and environmental (e.g., soil security, water security, climate mitigation) pressures (McBratney et al., 2014; Bommarco et al., 2018; FAO, 2018). Human activities, particularly urban expansion and inadequate agricultural practices, negatively impact agricultural land, influencing not only the provisioning services but also the entire range of soil ES (which may include provisioning, regulating and cultural services) (MEA, 2005; Bommarco et al., 2018). As Swinton et al. (2007) discussed, consideration of ES (and by extension soil ES) provided by agricultural systems is dual and must be “viewed in the context of what they replace and what they might be replaced with.” Indeed, while human needs cause either natural land to be transformed to agriculture or agricultural land to be urbanized, sustainably managing soil ES from agricultural systems could meet the objectives of food security, climate mitigation and environmental conservation. Thus, agricultural soils need to be addressed specifically as a critical resource and integrated in decision-support tools for sustainable planning strategies, as emphasized by Robinson et al. (2013) and McBratney et al. (2014).
Despite the recognition of soil management in agroecosystems as a powerful mechanism for addressing environmental challenges (Robertson et al., 2014; Schulte et al., 2014; Ruhl, 2016), few studies have focussed specifically on soil-centered assessment of ES within agroecosystems (Greiner et al., 2017; Vogel et al., 2019) or even in other ecosystems (Dominati et al., 2010; Breure et al., 2012; Bouma, 2014; Grêt-Regamey et al., 2017). Recent publications on soil ES (Dominati et al., 2014; Adhikari and Hartemink, 2016; Birgé et al., 2016; Jónsson and Davíðsdóttir, 2016) highlight the need to develop an assessment framework for soils to be integrated in ES assessment studies.
This article revisits definitions and concepts from both science- and policy-based perspectives to argue for a possible way forward, positing that relevant soil ES assessment needs its own defined framework. Based on well-established frameworks such as the “cascade model” of ES (Haines-Young and Potschin, 2010; Potschin-Young et al., 2018) and the “conceptual framework linking soil to human needs” of Dominati et al. (2010, 2014), the assessment framework we developed is adapted to the objective of enhancing operational implementation of soil ES in decision-making processes. To this end, we first discuss critical assumptions about the perception of soils in an ES assessment context and detail the conceptual model that forms the first part of the assessment framework. An operational model is then developed to assess soil ES, followed by discussion of the relevance and potential added value of this assessment framework in a territorial planning context.
As discussed by Ponge (2012), applying the definition of “ecosystem” to soils is not new and remains an on-going debate. The issue of considering soils either as ecosystems in themselves or only as components of ecosystems needs to be addressed in ES assessment to develop a soil-centered assessment framework. Until recently, the MEA (2005) framework, distinguishing four categories of ES (i.e., provisioning, regulating, cultural and supporting), did not explicitly consider soils as providers of ES, recognizing them only as contributors to ES provision through the “supporting” category, which underlies the others. In contrast, the working group on Mapping and Assessment of Ecosystems and their Services (MAES) (Maes et al., 2018), in line with the CICES (2018) classification, tends to consider soils as providers of ES, merging the “supporting” and “regulating” categories into a “regulating and maintenance” category. Despite this increased consideration of soils in the ES approach, these frameworks still do not view soils as the subject of ES assessment but only as a component of the subject, which remains the ecosystem.
This under-consideration of soils in ES assessment could come from diverging perceptions of their place within ecosystems. To date in ES assessment (i.e., at the ecosystem scale), soils are commonly considered as a physical component that supports activities, which leads to their perception as only an “integrated component” of ecosystems (Ponge, 2012; Bouma, 2014). Otherwise, in line with the MEA (2005), ES are defined as “the goods and services from ecological systems that benefit people.” Thus, for agricultural systems, soils are embedded in the definition of the system, and soil ES are not differentiated explicitly from agricultural ES. In this perception, agricultural ES resulting from both natural and anthropogenic components are aggregated into the contribution to human well-being (Figure 1A). This perception reduces soils to “contributors” rather than “providers” of ES (see Adhikari and Hartemink, 2016 and Greiner et al., 2017 for reviews), which relegates soil ES to the “supporting” category (MEA, 2005). This perception prevents soils from being captured specifically and explicitly (McBratney et al., 2014; Adhikari and Hartemink, 2016) in the complex chain connecting ES to human well-being (Costanza et al., 2017; Potschin-Young et al., 2018).
Figure 1. Two alternative representations of soils in agricultural systems and their outcomes that regard soils as (A) contributors to agricultural ecosystem services or (B) providers of soil ecosystem services.
Ponge (2005, 2012) suggested applying to soils the assumption of interdependence with overlying activities. By assuming that soils interact with their overlying environment (i.e., both influencing their overlying environment and being influenced by it), they can be perceived as an “integral component” of the system considered. Thus, for agricultural systems, soils are one of the elements that define the system. In this perception, soil ES can be differentiated explicitly from agricultural ES and defined as the natural part of the contribution to human well-being, in contrast to the anthropogenic part that results from land use and management practices. In line with some recent literature (Costanza et al., 2017; Therond et al., 2017), the contribution to human well-being results from interactions between the soil component (and ultimately soil ES) and anthropogenic components (Figure 1B). This perception of soils as co-suppliers of the contribution to human well-being can be a solution to correcting the perception of soils by considering them as direct “providers” of ES and as an explicit subject in ES assessment. Furthermore, this perception is in line with recent classifications of ES (CICES, 2018; IPBES, 2018), which promote soil ES to the merged category “regulating and maintenance.”
Finally, beyond these ecosystem concepts lies the need to clarify the perception of soils in ES assessment. Indeed, in line with the relatively recent reintroduction of the “geodiversity approach” [i.e., “the natural range (diversity) of geological, geomorphological and soil features” (Gray, 2008; Gray et al., 2013; Alahuhta et al., 2018)], which emphasizes the role of soil features in providing ES, the concept of soil ES is emerging (Birgé et al., 2016; Jónsson and Davíðsdóttir, 2016; Su et al., 2018). Thus, we suggest splitting the agricultural system into an anthropogenic component as an external driver (including both land use and management practices as land management, and policy action as a societal response) and a soil component as natural assets (including both inherent and dynamic properties) in order to highlight the proper role of soils in ES assessment (Figures 1A,B). This perception is more in line with the recent classification and provides the opportunity to (i) capture soil ES better in the complex chain connecting soil ES, contributions to human well-being and governance and (ii) better assess the role that soils can play in territorial planning processes.
Barnaud and Antona (2014) and Danley and Widmark (2016) reported that a broad range of stakeholders used the ES concept and argued that its wide adoption creates ambiguity in its meaning. Reporting stakeholders' feedback about operationalization of ES, Carmen et al. (2018) and Jax et al. (2018) identified the need to adapt language to each stakeholder as one of the crucial principles to frame and shape action on land management across scales of governance. Considering these findings, we suggest a common lexicon for soil ES (Table 1) based on the ES literature.
Clearly distinguishing the terms “contributions” and “services” is necessary to (i) clarify relations between environmental and anthropocentric spheres and (ii) provide a common support bridging science, social and policy arenas (Figure 2), as emphasized by IPBES (2018) goals and Potschin-Young et al. (2018). First, unlike Potschin-Young et al. (2018), the term “contributions” is preferred to “benefits,” as proposed in the latest discussions of IPBES (Díaz et al., 2018), thus avoiding the trend toward purely economic considerations. In this article, “contributions to human well-being” is defined as the perceived societal expectations (positive and negative) underpinned by soil ES and anthropogenic components when setting up territorial planning (Table 1). Second, in line with Costanza et al. (2017) and Therond et al. (2017), “soil ES” is considered as the share of soils in the “contributions to human well-being.” Defined as contributions to human well-being resulting from direct expression of soil processes (Table 1), “soil ES” meets the need to match the social language with natural features that are usually studied in the research arena (scientific language) (Table 1). Among “soil ES,” we distinguish “current” and “potential” soil ES. “Current soil ES” is defined as the current soil ES provision observed under current agricultural systems, while “potential soil ES” is defined as the potential soil ES provision that could be expected under alternative agricultural systems. Furthermore, according to territorial planning objectives (e.g., balance between urban development and protection of natural landscapes, maintaining human well-being) in a context of urbanization and limited land area, the objective of promoting high soil ES provision is crucial. Thus, to satisfy this need stated by stakeholders, “potential soil ES” is considered as the maximum soil ES that can be obtained in a given context.
Figure 2. Conceptual model connecting social, scientific and policy arenas, and anthropocentric and environmental spheres. Solid arrows indicate the effects of causal links and clarify the connections between the two terms “contributions” and “soil natural assets” (i, ii, iii); dotted arrows indicate the stepwise integration of soil ecosystem services in the decision-making process.
As Díaz et al. (2018) discussed, the concepts of “services” and “contributions” can be seen from context-specific (i.e., local scale) to general (i.e., global scale) perspectives and aim to be incorporated into policy and practice. To this end, “governance scale” is defined as the policy scale at which “contributions” could be incorporated and considered in a regulatory way. “Governance” meets the need to match the planning objectives to be achieved (social language) with consistent policy tools at a given territorial scale (policy language).
The definition of soil ES retained (Table 1) and the use of the term “soil processes” is similar to Boyd and Banzhaf's (2007) perception of a service that contributes to human well-being and clarifies somewhat in a territorial planning context the concept of intermediate and final services discussed by Fisher et al. (2009) and Robinson et al. (2013). Thus, aligning largely with previous studies (Dominati et al., 2010; TEEB, 2012; Haines-Young and Potschin, 2013; Robinson et al., 2013), supporting services from the general ES assessment framework (MEA, 2005) are considered as “soil processes” rather than “soil ES.” Consequently, “soil processes” are defined as interactions among soil natural assets underlying soil ES (Table 1). These interactions are classified into four processes (i.e., cycling, storage, transfer and biotic web) (Figure 2) and define the basis of our soil ES operational model, in which processes underlying soil ES are quantified by simulation models or pedotransfer functions. As soil ES can be supported by several soil processes (Figure 2), distinguishing the two terms avoids the problem of double counting (Fu et al., 2011) in subsequent economic valuations.
Finally, although the concept of soils as a natural capital initially defined by Costanza et al. (1997) is broadly embodied in the ES approaches that consider soils (Robinson et al., 2013; Dominati et al., 2014; Smith et al., 2017), the term “soil natural assets” (Table 1) is preferred to “soil natural capital.” This asset also refers to intrinsic characteristics of soils derived from soil formation that are use- and time-invariant at the human scale and to characteristics of soils susceptible to change due to land use and climate change that are use- and time-variant at the human scale (Table 1, Figure 2). To define these soil characteristics, the terminology of Robinson and Lebron (2010) is retained, namely “inherent properties” and “dynamic properties,” which Dominati et al. (2010, 2014) spread widely as a basis for defining “natural capital.” Thus, “soil natural assets” are defined as both inherent and dynamic properties (Table 1), which refer to any soil properties used to define and compare soils.
The science-policy arena, through international initiatives (e.g., TEEB, IPBES, the Convention on Biological Diversity), recognizes the need to produce usable science-based knowledge to move toward sustainable governance of ecosystems (Díaz et al., 2015; Tengö et al., 2017). Despite the progress made on ES definitions and conceptualization, this knowledge still plays a limited role in decision making during planning (Carmen et al., 2018; Saarikoski et al., 2018). This suggests that simply increasing the amount of soil ES knowledge does not always improve understanding or integration of soil ES into decision-making processes. Thus, developing a conceptual model for using soil ES knowledge adapted to multi-stakeholder planning contexts is crucial to perform decision-relevant assessment and support sustainable regional governance at multiple scales.
The conceptual model proposed is divided into three components (or arenas). The policy arena (in the anthropocentric sphere), which refers to governance, includes considerations related to territorial, national or global policy and planning constraints. The social arena (in the anthropocentric sphere) refers to considerations related to human well-being and ES. Lastly, the scientific arena (in the environmental sphere) considers soil processes and soil natural assets. Using this structure as a basis, the model suggests a series of steps to integrate soil ES into the territorial planning process (Figure 2). This model can first be used as a basis for implementing soil ES in a regulatory context. The iterative step from “contributions” to “governance” allows evaluation of the potential to consider contributions by using currently available regulatory tools and thus determine the contributions that could be effectively implemented and the corresponding governance scale. The model can also be used as the basis of a soil ES assessment methodology. The steps from “contributions” to “soil natural assets” clarify the connections between the two terms by answering the following questions: (i) Which soil ES are related to the given “contributions”? (ii) Which model of soil processes can quantify the given soil ES? and (iii) Are the data required to model the steps from soil process to soil natural assets available? These connections constitute the steps to structure the soil ES assessment, thus putting theoretical concepts into operation.
This conceptual model is in accordance with the frameworks of Potschin and Haines-Young (2011, 2016) and Dominati et al. (2010, 2014), as they appear suitable for disentangling relations among soils, soil ES and human well-being. A stepwise “cascade model” is useful for supporting multi-stakeholder understanding of soil ES (Spangenberg et al., 2014) and using related knowledge, which builds an argument for using them in decision making in a structured way.
The conceptual model developed (Figure 2) shows interconnections between science, social and policy arenas, including causal effects among the main elements of our assessment framework (Figure 2). Although “soil ES” has progressively acquired status as a scientific concept (Barnaud and Antona, 2014), the language used is more a social language that places “soil ES” at the interface between the scientific and social arenas. The anthropocentric sphere includes both social and policy arenas, which are connected through “contributions.” These “contributions” bridge a gap in implementation from the social to policy arenas, in line with other authors (Primmer et al., 2015; Bouwma et al., 2018; Dick et al., 2018; Saarikoski et al., 2018), through planning decisions. Also, through “soil ES,” “contributions” bridge the gap in implementation from the social to scientific arenas, in line with reviews of Grêt-Regamey et al. (2017) and Dick et al. (2018). Thus, “contributions” connect, in a practical way, the soil ES concept to its potential degree of integration in planning strategies, defining which governance scale corresponds to the planning objective and which policy tools integrate knowledge about soil ES. Finally, “driving forces,” particularly through human-induced factors, connect the policy and scientific arenas through potential impacts on soil natural assets. “Driving forces” also connect the policy and social arenas as co-suppliers of “contributions” to soil ES (Figure 1). This connection bridges the two knowledge systems (i.e., science-based knowledge as a decision-support tool and policy-based knowledge as a tool to support policy instruments) described by Carmen et al. (2018).
Soil ES that can be provided by agricultural soils were selected (Table 2) based on studies related to soil ES, including global reviews (Adhikari and Hartemink, 2016; Jónsson and Davíðsdóttir, 2016; Greiner et al., 2017), methodological frameworks (Robinson et al., 2013; Dominati et al., 2014; Lescourret et al., 2015; Birgé et al., 2016; Calzolari et al., 2016) and existing ES typologies (MEA, 2005; COM, 2006; Zhang et al., 2007; Ruhl, 2008; CICES, 2018; Díaz et al., 2018). Within agricultural systems, soils and their interactions with other ecosystem components (e.g., land use, management practices, climate, hydrology) can provide a set of ES, as conceptualized by Dominati et al. (2010, 2014).
Table 2. Soil ecosystem services (soil ES) developed in the assessment framework, underpinning soil processes, indicators, governance scales, and parallels with common ecosystem services (ES) frameworks.
Twelve soil ES (Table 2) were identified in seven IPBES (2018) ES categories (excluding cultural ES), corresponding to nine CICES (2018) ES classes. These soil ES support certain major challenges addressed in territorial planning and their scale of governance (i.e., local, national or global) (Table 2). This list relates soil ES to underlying soil processes and suggests indicators derived from model outputs used to quantify soil ES.
We start from a conceptual model of soil ES (Figure 2) that focusses on agricultural systems and intends to estimate soil ES. The associated operational model to assess soil ES (Figure 3) focusses on agricultural system management, particularly on the relation between soils and land management. This allows one, using dedicated models, to (i) estimate impacts of land management on soil processes and thus on soil ES and (ii) predict the evolution of multiple soil ES. Results from this modeling framework will indicate which agricultural system provides both the largest contribution to human well-being, given the objectives of territorial planning, and the lowest environmental impacts. Applying this operational model requires three successive steps and is illustrated by an example in which two soil types and three land management types are considered.
Figure 3. Workflow of the operational model for assessing agricultural soil ecosystem services (ES).
As shown previously, the agricultural system is split into two components: (i) soils, with their physical, chemical and biological properties, and (ii) land management, which includes land use (i.e., cropping or grassland) and management practices (e.g., crop rotation, fertilization, grazing density, and timing) (Figure 1) observed at the spatial extent of the planning area. Soil natural assets (Table 1) have inherent properties, which may vary spatially, and dynamic properties, which vary greatly both spatially and temporally, due to disturbance or changes caused by agricultural land management, which modify soil processes and subsequently the soil ES they provide (Groffman et al., 2009; Burkhard et al., 2012; McFero Grace and Skaggs, 2013; Durán et al., 2017).
For the operational model, agricultural systems are described by all possible pairs of soil types (i.e., observed in the field or clustered into groups) and land management types (i.e., commonly observed in the field) in the planning area. Soil types and their associated properties (i.e., inherent and current dynamic properties, which are initial input data of the models considered) in the planning area are extracted from a soil database. Land management types may be (i) existing types, identified by analysis of agricultural censuses or specific surveys, or (ii) alternative types, selected or designed in the territorial planning procedure. Combinations of soil type and land use and management type define either existing or alternative agricultural systems (Figure 4).
Figure 4. An example of application of the operational model to consider soil ecosystem services (ES) as decision support information in territorial planning.
To estimate the “current state” of soil ES provision, only currently existing agricultural systems are considered, and soil ES prediction models are selected to quantify them (Figure 4). The “potential state” of soil ES provision is estimated by the maximum of ES modeling predictions for all existing or alternative agricultural systems (Figure 4). This assessment enables evaluation of possible gains and/or losses in overall soil ES provision under several planning scenarios.
Models that simulate carbon, nitrogen, water and energy flows at the field scale (not described in this article) are used to predict soil ES values. Using agricultural system characteristics as inputs, model predictions are processed to calculate one indicator value per soil ES for each agricultural system. Two key classes of indicators can then be defined: “current soil ES” (soil ES indicator value provided by a real pair of soil type and land management type) and “potential soil ES” (maximum soil ES indicator value provided by a real pair or by an alternative pair corresponding to the same soil type combined with another land management type observed at the planning scale).
Subsequent analysis of these modeling results allows one to (i) determine potential soil ES, (ii) compare current and potential soil ES indicator values, (iii) evaluate the multiservice provision ability of each agricultural system defined and (iv) display these results in a map (GIS data processing).
Mapping ES can be a useful and powerful tool for raising awareness and support decision making (Grêt-Regamey et al., 2017; Maes et al., 2018). For territorial planning purposes, stakeholders may require both qualitative and quantitative knowledge: simple qualitative information may capture strengths and weaknesses of a given area better, while more quantitative information is needed to evaluate expected results from alternative agricultural systems.
The last step of the operational model is therefore to develop a sequential mapping approach that describes at the territory scale both current and potential soil ES states (Figure 4). First, a map showing the current soil ES value can be drawn using semi-quantitative classes to identify areas with low and high provision of soil ES. Second, a map showing the difference between the current soil ES value and the potential soil ES state may identify areas with high expectations of increased soil ES if actions are implemented. Finally, a map showing the potential soil ES enables the potential provision of soil ES and the existing soil ES demand to be compared within the territory.
Finally, full implementation of the operational model would enable two potential assessment perspectives:
• Assessment of the current state of provision of a given soil ES. Here, soil ES assessment aims to answer the following questions: What are the values of soil ES at various planning scales? How are these values spatially distributed (areas of low or high provision)? and How does this spatial distribution correspond to social needs?
• Assessment of different territorial planning scenarios in which gains or losses of soil ES are compared. Here, soil ES assessment aims to answer the following questions: Under different conditions (i.e., land-use change scenarios), what gains or losses of soil ES provision can be expected? and Which soils are best suited to provide a given soil ES and under which land management conditions?
In a planning context, the soil-centered assessment framework and the associated conceptual (Figure 2) and operational models (Figure 3) raise four key methodological issues:
• Data availability. Values of soil natural assets underpinning current and potential soil ES can be obtained in three ways: (i) direct measurements (if no data exist), (ii) soil databases (as minimum data set providers) and (iii) modeling (i.e., predicted from soil properties).
• Data homogeneity. As soil ES assessment is highly time dependent, it must consider both land management and climatic conditions. To do so, soil ES values must be integrated over cropping periods to consider interactions between land use and soils properly. If soil ES are modeled, simulation periods must capture climatic variability; so, simulations of several years or decades are recommended, depending on the agronomic and pedoclimatic contexts.
• Data operability. Fully integrating soil ES assessment in planning processes requires tools that are accessible and compatible. Mapping soil ES at the territory scale appears to be an appropriate tool that territorial planners and stakeholders can easily understand to help compare scenarios and identify land management that enhances soil ES.
• Data transferability. The range of potential soil ES values defines the validity domain (i.e., ranges of spatial scale and soil types) of the assessment. Depending mainly on the resolution of available data, transferability of one assessment to another also depends on boundary values of potential soil ES.
There is growing demand to assess ES from agricultural systems for the purpose of territorial planning (Birgé et al., 2016; Ruhl, 2016). Planning strategies can involve spatial distribution of different land uses (e.g., definition of urban, agricultural or natural protection areas) and, for agricultural areas, be based on a variety of land management options, such as “land sparing” (separate areas of high-intensity agriculture and wilderness) or “land sharing” [low-intensity agriculture interspersed with natural features (e.g., hedgerows, ponds, wetlands)] (Legras et al., 2018). Consequently, the assessment framework proposed provides a basis for integrating the soil ES concept into the land management decision making process, mainly for the following points.
By combining biophysical approaches and connecting environmental and anthropocentric terms, the assessment framework tends to provide the holism required by Primmer et al. (2015), Schleyer et al. (2015), and Loft et al. (2015) in the ES approach, integrating multiple modes (i.e., hierarchical, scientific-technical and adaptive collaborative) and scales (i.e., vertical and horizontal knowledge production, sharing and policy integration) for governance of ES.
Because it is soil-based, the framework contributes to emerging knowledge about how ES provided by agricultural systems depend upon soil characteristics by assessing the testable hypothesis that optimal soil/land use combinations that maximize soil ES do exist. Scientific understanding of assessment framework components (Table 1, Figure 2) and how they emphasize soil ES may encourage decision makers to follow the soil ES approach in land planning (Swinton et al., 2007). In addition, its combined biophysical approach provides a decision-support tool that allows estimation of potential provision of soil ES due to land planning strategies and differentiation of land management options and the soil ES they may provide (i.e., trade-offs and synergies) (Loft et al., 2015; Ruhl, 2016; Bommarco et al., 2018; Kim and Arnhold, 2018).
As a process-based assessment, the workflow of the operational model (Figure 3), can help define a monitoring dataset that decision makers can use to assess the effectiveness of land management strategies on soil ES provision and thus the success or failure of policy instruments (Loft et al., 2015; Rabot et al., 2017). Feedback from this monitoring could help inform policy design and encourage decision makers to implement a particular land management option (Primmer et al., 2015; Baveye, 2017; Legras et al., 2018).
Finally, these insights address the new paradigm of a shift from conserving nature to using it sustainably (Loft et al., 2015; Legras et al., 2018). This change in perception involves considering both societal needs (i.e., demand for soil ES) and conservation of natural assets (i.e., supply of soil ES). Because it does so by producing knowledge, this soil ES assessment framework supports the nature-based solutions approach, which aims to conciliate socio-economic development goals with beneficial outcomes for both society and the environment (European Commission (EC), 2015; Faivre et al., 2017; Lafortezza et al., 2017). As an agricultural soil-based assessment, the framework includes a soil security dimension (McBratney et al., 2014) by considering the resilience and sustainable use of soils (i.e., conserving soil natural assets) and provides a tool that addresses soil ES trade-offs (Kim and Arnhold, 2018) through the ability to arbitrate land management strategies effectively (e.g., supply vs. demand, land sharing vs. land sparing).
Agroecosystems provide services that must respond to both human needs and environmental constraints. Because they lie at a critical interface in the biosphere, soils contribute greatly to provision of these services. To address the increasing demand for consideration of these services in territorial planning, the evaluation framework proposed takes into account scientific, social and policy considerations. This assessment framework is based on both a conceptual model and an operational model. In this framework, soils are considered as providers of ES, and are therefore better positioned in the chain of decision. On this basis, the operational framework proposed allows identification of land use and management practices that optimize soil ES. Further development and applications have already begun to (i) define soil-indicator thresholds using both empirical data and modeling and (ii) improve the soil ES model's operability (i.e., in its tool forms, as maps and matrices) to improve usability and acceptance through interdisciplinary work among soil scientists, urban planners, decision makers and economists.
All datasets generated for this study are included in the article/supplementary material.
MF: substantial contributions to the conception of the work and drafting the work. Agree to be accountable for all aspects of the work. DA, CC, CG-O, and CW: substantial contributions to the conception of the work and revising it critically for important intellectual content. CBu, CBe, PD, AJ, and GP: provide approval for publication of the content.
CBe was employed by company SCE, Groupe Keran.
The remaining authors declare that the research was conducted in the absence of any commercial or financial relationships that could be construed as a potential conflict of interest.
This study was performed as part of a collaborative research project (Multi-scale assessment of soil ecosystem services in agroecosystems) within the framework of the SOILSERV project (ANR-16-CE32-0005-01) funded by the French National Research Agency (ANR).
Adhikari, K., and Hartemink, A. E. (2016). Linking soils to ecosystem services — a global review. Geoderma 262, 101–111. doi: 10.1016/j.geoderma.2015.08.009
Alahuhta, J., Ala-Hulkko, T., Tukiainen, H., Purola, L., Akujärvi, A., Lampinen, R., et al. (2018). The role of geodiversity in providing ecosystem services at broad scales. Ecol. Indic. 91, 47–56. doi: 10.1016/j.ecolind.2018.03.068
Albert, C., Galler, C., Hermes, J., Neuendorf, F., von Haaren, C., and Lovett, A. (2016). Applying ecosystem services indicators in landscape planning and management: the ES-in-Planning framework. Ecol. Indic. 61, 100–113. doi: 10.1016/j.ecolind.2015.03.029
Barnaud, C., and Antona, M. (2014). Deconstructing ecosystem services: uncertainties and controversies around a socially constructed concept. Geoforum 56, 113–123. doi: 10.1016/j.geoforum.2014.07.003
Baveye, P. C. (2017). Quantification of ecosystem services: beyond all the “guesstimates”, how do we get real data? Ecosyst. Serv. 24, 47–49. doi: 10.1016/j.ecoser.2017.02.006
Birgé, H. E., Bevans, R. A., Allen, C. R., Angeler, D. G., Baer, S. G., and Wall, D. H. (2016). Adaptive management for soil ecosystem services. J. Environ. Manage. 183, 371–378. doi: 10.1016/j.jenvman.2016.06.024
Bommarco, R., Vico, G., and Hallin, S. (2018). Exploiting ecosystem services in agriculture for increased food security. Glob. Food Secur. 17, 57–63. doi: 10.1016/j.gfs.2018.04.001
Bouma, J. (2014). Soil science contributions towards sustainable development goals and their implementation: linking soil functions with ecosystem services. J. Plant Nutr. Soil Sci. 177, 111–120. doi: 10.1002/jpln.201300646
Bouwma, I., Schleyer, C., Primmer, E., Winkler, K. J., Berry, P., Young, J., et al. (2018). Adoption of the ecosystem services concept in EU policies. Ecosys. Serv. 29, 213–222. doi: 10.1016/j.ecoser.2017.02.014
Boyd, J., and Banzhaf, S. (2007). What are ecosystem services? the need for standardized environmental accounting units. Ecol. Econ. 63, 616–626. doi: 10.1016/j.ecolecon.2007.01.002
Breure, A. M., De Deyn, G. B., Dominati, E., Eglin, T., Hedlund, K., Van Orshoven, J., et al. (2012). Ecosystem services: a useful concept for soil policy making! Curr. Opin. Environ. Sustain. 4, 578–585. doi: 10.1016/j.cosust.2012.10.010
Burkhard, B., Krolol, F., Nedkov, S., and Müller, F. (2012). Mapping ecosystem service supply, demand and budgets. Ecol. Indic. 21, 17–29. doi: 10.1016/j.ecolind.2011.06.019
Calzolari, C., Ungaro, F., Filippi, N., Guermandi, M., Malucelli, F., Marchi, N., et al. (2016). A methodological framework to assess the multiple contributions of soils to ecosystem services delivery at regional scale. Geoderma 261, 190–203. doi: 10.1016/j.geoderma.2015.07.013
Carmen, E., Watt, A., Carvalho, L., Dick, J., Fazey, I., Garcia-Blanco, G., et al. (2018). Knowledge needs for the operationalisation of the concept of ecosystem services. Ecosys. Serv. 29, 441–451. doi: 10.1016/j.ecoser.2017.10.012
CICES (2018). The Common International Classification of Ecosystem Services, V5.1. Available online at: https://cices.eu/resources/ (accessed April 06, 2019).
COM (2006). Communication de la Commission au Conseil, au Parlement Européen, au Comité Economique et Social Européen et au Comité des Régions, Stratégie Thématique en Faveur d ela Protection des sols. COM.
Costanza, R., d'Arge, R., de Groot, R., Farber, S., Grasso, M., Hannon, B., et al. (1997). The value of the world's ecosystem services and natural capital. Nature 387, 253–260. doi: 10.1038/387253a0
Costanza, R., de Groot, R., Braat, L., Kubiszewski, I., Fioramonti, L., Sutton, P., et al. (2017). Twenty years of ecosystem services: How far have we come and how far do we still need to go? Ecosys. Serv. 28, 1–16. doi: 10.1016/j.ecoser.2017.09.008
Danley, B., and Widmark, C. (2016). Evaluating conceptual definitions of ecosystem services and their implications. Ecol. Econ. 126, 132–138. doi: 10.1016/j.ecolecon.2016.04.003
de Groot, R. S., Alkemade, R., Braat, L., Hein, L., and Willemen, L. (2010). Challenges in integrating the concept of ecosystem services and values in landscape planning, management and decision making. Ecol. Complex. 7, 260–272. doi: 10.1016/j.ecocom.2009.10.006
Díaz, S., Pascual, U., Stenseke, M., Martin-Lopez, B., Watson, R. T., Molnar, Z., et al. (2018). Assessing nature's contributions to people. Science 359, 270–272. doi: 10.1126/science.aap8826
Díaz, S., Demissew, S., Carabias, J., Joly, C., Lonsdlae, M., Ash, N., et al. (2015). The IPBES Conceptual Framework — connecting nature and people. Curr. Opin. Environ. Sustain. 14, 1–16. doi: 10.1016/j.cosust.2014.11.002
Dick, J., Turkelboom, F., Woods, H., Iniesta-Arandia, I., Primmer, E., Saarela, S. R., et al. (2018). Stakeholders' perspectives on the operationalisation of the ecosystem service concept: Results from 27 case studies. Ecosys. Serv. 29, 552–565. doi: 10.1016/j.ecoser.2017.09.015
Dominati, E., Mackay, A., Green, S., and Patterson, M. (2014). A soil change-based methodology for the quantification and valuation of ecosystem services from agro-ecosystems: a case study of pastoral agriculture in New Zealand. Ecol. Econ. 100, 119–129. doi: 10.1016/j.ecolecon.2014.02.008
Dominati, E., Patterson, M., and Mackay, A. (2010). A framework for classifying and quantifying the natural capital and ecosystem services of soils. Ecol. Econ. 69, 1858–1868. doi: 10.1016/j.ecolecon.2010.05.002
Drobnik, T., Greiner, L., Keller, A., and Grêt-Regamey, A. (2018). Soil quality indicators - from soil functions to ecosystem services. Ecol. Indic. 94, 151–169. doi: 10.1016/j.ecolind.2018.06.052
Durán, J., Morse, J. L., Rodriguez, A., Campbell, J. L., Christenson, L. M., Driscoll, C. T., et al. (2017). Differential sensitivity to climate change of C and N cycling processes across soil horizons in a northern hardwood forest. Soil Biol. Biochem. 107, 77–84. doi: 10.1016/j.soilbio.2016.12.028
Englund, O., Berndes, G., and Cederberg, C. (2017). How to analyse ecosystem services in landscapes—a systematic review. Ecol. Indic. 73, 492–504. doi: 10.1016/j.ecolind.2016.10.009
European Commission (EC) (2006). The Implementation of the Soil Thematic Strategy and Ongoing Activities. Final Report from the Commission to the European Parliament, the Council, the European Economic and Social Committee and the Committee of the Regions. COM.
European Commission (EC) (2015). Nature-Based Solutions & Re-Naturing Cities. Final Report of the Horizon 2020 Expert Group on ‘Nature-Based Solutions and Re-Naturing Cities'. Directorate-General for Research and Innovation–Climate Action, Environment, Resource Efficiency and Raw Materials. 74.
Faivre, N., Fritz, M., Freitas, T., de Boissezon, B., and Vandewoestijne, S. (2017). Nature-based solutions in the EU: innovating with nature to address social, economic and environmental challenges. Environ. Res. 159, 509–518. doi: 10.1016/j.envres.2017.08.032
Fisher, B., Turner, R. K., and Morling, P. (2009). Defining and classifying ecosystem services for decision making. Ecol. Econ. 68, 643–653. doi: 10.1016/j.ecolecon.2008.09.014
Fu, B.-J., Su, C.-H., Wei, Y.-P., Willett, I. R., Lü, Y.-H., and Liu, G.-H. (2011). Double counting in ecosystem services valuation: causes and countermeasures. Ecol. Res. 26, 1–14. doi: 10.1007/s11284-010-0766-3
Grêt-Regamey, A., Siren, E., Brunner, S. H., and Weibel, B. (2017). Review of decision support tools to operationalize the ecosystem services concept. Ecosys. Serv. 26, 306–315. doi: 10.1016/j.ecoser.2016.10.012
Gray, M. (2008). Geodiversity: developing the paradigm. Proc. Geol. Assoc. 119, 287–298. doi: 10.1016/S0016-7878(08)80307-0
Gray, M., Gordon, J. E., and Brown, E. J. (2013). Geodiversity and the ecosystem approach: the contribution of geoscience in delivering integrated environmental management. Proc. Geol. Assoc. 124, 659–673. doi: 10.1016/j.pgeola.2013.01.003
Greiner, L., Keller, A., Grët-Regamey, A., and Papritz, A. (2017). Soil function assessment: review of methods for quantifying the contributions of soils to ecosystem services. Land Use Policy 69, 224–237. doi: 10.1016/j.landusepol.2017.06.025
Groffman, P. M., Hardy, J. P., Fisk, M. C., Fahey, T. J., and Driscoll, C. T. (2009). Climate variation and soil carbon and nitrogen cycling processes in northern hardwood forest. Ecosystems 12, 927–943. doi: 10.1007/s10021-009-9268-y
Haines-Young, R., and Potschin, M. (2010). “The links between biodiversity, ecosystem services and human well-being,” Ecosystem Ecology: A New Synthesis, eds D. Raffaelli and C. Frid (Cambridge: BES Ecological Reviews Series, CUP), 110–139.
Haines-Young, R., and Potschin, M. (2013). Common International Classification of Ecosystem Services (CICES), Version 4.3. Report to the European Environment Agency EEA/BSS/07/007. Retrieved from: www.cices.eu
Hatton MacDonald, D., Bark, R. H., and Coggan, A. (2014). Is ecosystem service research used by decision-makers? A case study of the Murray-Darling Basin, Australia. Landsc. Ecol. 29, 1447–1460. doi: 10.1007/s10980-014-0021-3
IPBES (2018). Intergovernmental Platform on Biodiversity and Ecosystem Services. Retrieved from: https://www.ipbes.net/outcomes (accessed April 05, 2018).
Jax, H., Furman, E., Saarikoski, H., Barton, D. N., Delbaere, B., Dick, J., et al. (2018). Handling a messy world: lessons learned when trying to make the ecosystem services concept operational. Ecosys. Serv. 29, 415–427. doi: 10.1016/j.ecoser.2017.08.001
Jónsson, J. Ö. G., and Davíðsdóttir, B. (2016). Classification and valuation of soil ecosystem services. Agricult. Syst. 145, 24–38. doi: 10.1016/j.agsy.2016.02.010
Kim, I., and Arnhold, S. (2018). Mapping environmental land use conflict potentials and ecosystem services in agricultural watersheds. Sci. Total Environ. 630:827–838. doi: 10.1016/j.scitotenv.2018.02.176
Lafortezza, R., Chen, J., Konijnendijk van der Bosch, C., and Randrup, T. B. (2017). Nature-based solutions for resilient landscapes and cities. Environ. Res. 165, 431–441. doi: 10.1016/j.envres.2017.11.038
Legras, S., Martin, E., and Piguet, V. (2018). Conjunctive implementation of land sparing and land sharing for environmental preservation. Ecol. Econ. 143, 170–187. doi: 10.1016/j.ecolecon.2017.07.006
Lescourret, F., Magda, D., Richard, G., Adam-Blondon, A.-F., Bardy, M., Baudry, J., et al. (2015). A social-ecological approach to managing multiple agro-ecosystem services. Curr. Opin. Environ. Sustain. 14, 68–75. doi: 10.1016/j.cosust.2015.04.001
Loft, L., Mann, C., and Hanjürgens, B. (2015). Challenges in ecosystem services governance: multi-levels, multi-actors, multi-rationalities. Ecosys. Serv. 16, 150–157. doi: 10.1016/j.ecoser.2015.11.002
Maes, J., Teller, A., Erhard, M., Grizzetti, B., Barredo, J. I., Paracchini, M. L., et al. (2018). Mapping and Assessment of Ecosystems and their Services: An Analytical Framework for Ecosystem Condition. Luxembourg: Publications Office of the European Union.
McBratney, A., Field, D. J., and Koch, A. (2014). The dimensions of soil security. Geoderma 213, 203–213. doi: 10.1016/j.geoderma.2013.08.013
McFero Grace, J., and Skaggs, R. W. (2013). “Determination of field-effective soil properties in the tidewater region of North Carolina” in 2013 Proceedings of the 15th biennal southern silvicultural research conference e-Gen. Tech. Resp. SRS-GTR-175, ed J. M. Guldin (Asheville, NC: U.S. Department of Agriculture; Forest Service; Southern Research Station), 411–419.
MEA (2005). Millenium Ecosystem Assessment, Ecosystem and Human Well-being: A framework for Assessment. Washington, DC: Island Press.
Ponge, J.-F. (2005). Emergent properties from organisms to ecosystems: towards a realistic approach. Biol. Rev. Camb. Philos. Soc. 80, 403–411. doi: 10.1017/S146479310500672X
Posner, S., Getz, C., and Ricketts, T. (2016). Evaluating the impact of ecosystem service assessments on decision-makers. Environ. Sci. Policy 64, 30–37. doi: 10.1016/j.envsci.2016.06.003
Potschin, M., and Haines-Young, R. (2016). Defining and Measuring Ecosystem Services. Routledge Handbook of Ecosystem Services. eds M. Potschin, R. Haines-Young, R. Fish, and R. K. Turner (Routledge, London and New York), 25–44.
Potschin, M. B., and Haines-Young, R. H. (2011). Ecosystem services: exploring a geographical perspective. Prog. Phys. Geogr. 35, 575–594. doi: 10.1177/0309133311423172
Potschin-Young, M., Haines-Young, R., Görg, C., Heink, U., Jax, K., and Schleyer, C. (2018). Understanding the role of conceptual frameworks: Reading the ecosystem service cascade. Ecosyst. Serv. 29, 428–440. doi: 10.1016/j.ecoser.2017.05.015
Primmer, E., Jokinen, P., Blicharska, M., Barton, D. N., Bugter, R., and Potschin, M. (2015). Governance of ecosystem services: a framework for empirical analysis. Ecosyst. Serv. 16, 158–166. doi: 10.1016/j.ecoser.2015.05.002
Rabot, E., Keller, C., Ambrosi, J. P., and Robert, S. (2017). Revue des méthodes multiparamétriques pour l'estimation de la qualité des sols dans le cadre de l'aménagement du territoire. Etude et Gestion des Sols 24, 59–72.
Robertson, G. P., Gross, K. L., Hamilton, S. K., Landis, D. A., Schmidt, T. M., Snapp, S. S., et al. (2014). Farming for ecosystem services: an ecological approach to production agriculture. Bioscience 64, 404–415. doi: 10.1093/biosci/biu037
Robinson, D. A., Hockley, N., Cooper, D. M., Emmett, B. A., Keith, A. M., Lebron, B., et al. (2013). Natural capital and ecosystem services, developing an appropriate soils framework as a basis for valuation. Soil Biol. Biochem. 57, 1023–1033. doi: 10.1016/j.soilbio.2012.09.008
Robinson, D. A., and Lebron, I. (2010). On the natural capital and ecosystem services of soils. Ecol. Econ. 70, 137–138. doi: 10.1016/j.ecolecon.2010.08.012
Ruckelshaus, M., McKenzie, E., Tallis, H., Guerry, A., Daily, G., Kareiva, P., et al. (2015). Notes from the field: lessons from using ecosystem service approaches to inform real-world decisions. Ecol. Econ. 115, 11–21. doi: 10.1016/j.ecolecon.2013.07.009
Ruhl, J. B. (2008). Agriculture and ecosystem services: strategies for state and local governments. NYU Environ. Law J. 17:36.
Ruhl, J. B. (2016). Adaptive management of ecosystem services across different land use regimes. J. Environ. Manage. 183, 418–423. doi: 10.1016/j.jenvman.2016.07.066
Saarikoski, H., Primmer, E., Saarela, S.-R., Antunes, P., Aszalos, R., Baro, F., et al. (2018). Institutional challenges in putting ecosystem service knowledge in practice. Ecosyst. Serv. 29, 579–598. doi: 10.1016/j.ecoser.2017.07.019
Schleyer, C., Görg, C., Hauck, J., and Winkler, K. J. (2015). Opportunities and challenges for mainstreaming the ecosystem services concept in the multi-level policy-making within the EU. Ecosyst. Serv. 16, 174–181. doi: 10.1016/j.ecoser.2015.10.014
Schulte, R. P. O., Creamer, R. E., Donnellan, T., Farrelly, N., Fealy, R., O'Donoghue, C., et al. (2014). Functional land management: a framework for managing soil-based ecosystem services for the sustainable intensification of agriculture. Environ. Sci. Policy 38, 45–58. doi: 10.1016/j.envsci.2013.10.002
Smith, A. C., Harrison, P. A., Pérez-Soba, M., Archaux, F., Blicharska, M., Egoh, B. N., et al. (2017). How natural capital delivers ecosystem services: a typology derived from a systematic review. Ecosyst. Serv. 26, 111–126. doi: 10.1016/j.ecoser.2017.06.006
Spangenberg, J. H., von Haaren, C., and Settele, J. (2014). The ecosystem service cascade: further developing the metaphor. Integrating societal processes to accommodate social processes and planning, and the case of bioenergy. Ecol. Econ.104, 22–32. doi: 10.1016/j.ecolecon.2014.04.025
Su, C., Liu, H., and Wang, S. (2018). A process-based framework for soil ecosystem services study and management. Sci. Total Environ. 627, 282–289. doi: 10.1016/j.scitotenv.2018.01.244
Swinton, S. M., Lupi, F., Robertson, G. P., and Hamilton, S. K. (2007). Ecosystem services and agriculture: cultivating agricultural ecosystems for diverse benefits. Ecol. Econ. 64, 245–252. doi: 10.1016/j.ecolecon.2007.09.020
TEEB (2012). The Economics of Ecosystems and Biodiversity in Local and regional Policy and Management, Edited by Heidi Wittmer and Haripriya Gundimeda. London; Washington, DC: Earthscan.
Tengö, M., Hill, R., Malmer, P., Raymond, C. M., Spierenburg, M., Danielsen, F., et al. (2017). Weaving knowledge systems in IPBES, CBD and beyond—lessons learned for sustainability. Curr. Opin. Environ. Sustain. 26–27, 17–25. doi: 10.1016/j.cosust.2016.12.005
Therond, O., Tichit, M., Tibi, A., Accatino, F., Biju-Duval, L., Bockstaller, C., et al. (2017). Volet 'écosystèmes agricoles' de l'Evaluation Française des Ecosystèmes et des Services Ecosystémiques. Rapport d'étude. INRA, 966. Available online at: https://prodinra.inra.fr/record/432343
Vogel, H. J., Einar, E., Franko, U., Lang, B., Ließ, M, Weller, U., et al. (2019). Quantitative evaluation of soil functions: potential and state. Front. Environ. Sci. 7:164. doi: 10.3389/fenvs.2019.00164
Wallace, K. J. (2007). Classification of ecosystem services: problems and solutions. Biol. Conserv. 139, 235–246. doi: 10.1016/j.biocon.2007.07.015
Keywords: agricultural system, process-based approach, land management, territorial planning, decision support information
Citation: Fossey M, Angers D, Bustany C, Cudennec C, Durand P, Gascuel-Odoux C, Jaffrezic A, Pérès G, Besse C and Walter C (2020) A Framework to Consider Soil Ecosystem Services in Territorial Planning. Front. Environ. Sci. 8:28. doi: 10.3389/fenvs.2020.00028
Received: 31 January 2019; Accepted: 27 February 2020;
Published: 30 March 2020.
Edited by:
Philippe C. Baveye, AgroParisTech Institut des Sciences et Industries du Vivant et de L'environnement, FranceReviewed by:
Pandi Zdruli, Istituto Agronomico Mediterraneo di Bari, ItalyCopyright © 2020 Fossey, Angers, Bustany, Cudennec, Durand, Gascuel-Odoux, Jaffrezic, Pérès, Besse and Walter. This is an open-access article distributed under the terms of the Creative Commons Attribution License (CC BY). The use, distribution or reproduction in other forums is permitted, provided the original author(s) and the copyright owner(s) are credited and that the original publication in this journal is cited, in accordance with accepted academic practice. No use, distribution or reproduction is permitted which does not comply with these terms.
*Correspondence: Christian Walter, Y2hyaXN0aWFuLndhbHRlckBhZ3JvY2FtcHVzLW91ZXN0LmZy
Disclaimer: All claims expressed in this article are solely those of the authors and do not necessarily represent those of their affiliated organizations, or those of the publisher, the editors and the reviewers. Any product that may be evaluated in this article or claim that may be made by its manufacturer is not guaranteed or endorsed by the publisher.
Research integrity at Frontiers
Learn more about the work of our research integrity team to safeguard the quality of each article we publish.