- 1Institute for Advanced Sustainability Studies (IASS), Potsdam, Germany
- 2Department of Ecosystem Management, Institute for Natural Resource Conservation, Kiel University, Kiel, Germany
- 3Scripps Institution of Oceanography, UC San Diego, La Jolla, CA, United States
- 4Lamont-Doherty Earth Observatory, Columbia University, Palisades, NY, United States
The Southern Ocean and coastal Antarctica provide a variety of ecosystem services with benefits for humankind that are of regional and global importance. Despite being nearly uninhabited, increasing exploitation of natural resources, a growing human presence, and environmental change threaten the sustained provisioning of these services. Ecosystem service assessments have proven as a suitable tool to understand the relevance of ecosystems for human well-being and guide decision-making, but the fluid and transboundary nature of marine ecosystems poses challenges to analyzing ecosystem services in regions with large marine sections. New methods to objectively assess the supply of ecosystem services for such realms are needed, and this need is exemplified by the Antarctic Peninsula region which encompasses rich marine, coastal, and terrestrial ecosystems but faces growing impacts and needs for taking action. In this study we applied the matrix method, an expert-based approach that employs a tabular matrix of ecosystem services and service providing units (SPUs) to elicit expert knowledge and rate the actual supply of key ecosystems services from the Antarctic Peninsula region. Further, we tested the applicability of this method on conventional definitions of SPUs and on objectively defined physico-chemical seascape units for a subset of the study region. Our results show high variations in the estimated supply of ecosystem services for the Antarctic Peninsula region, both with respect to the applied data models and in terms of the assessed services. While cultural and regulating services received highest supply estimates, provisioning services were regarded less relevant for the study region. Further, experts' supply estimates were much lower for the tested physico-chemical seascape units than for bathymetrically regionalized marine areas. The results suggest that a more explicit elaboration of linkages between ecosystem functions and processes and of the actual supply of ecosystem services is required in order to tap the full potential of such seascape data models in the context of qualitative, expert-based ecosystem service assessments.
Introduction
The world's ocean, coasts and seas have been recognized not only as key components of the Earth system but also as one of the most important natural resources for humankind and contributor to human well-being and development (Costanza, 1999; Visbeck et al., 2014; United Nations, 2015, p. 53 ff, 2016). The Southern Ocean and coastal Antarctica do not stand behind other ocean regions in that regard. Yet, despite its size, the continent has no indigenous human population and is “…in short, for the most part a vast and icy emptiness” (Watts, 1992, p. 3). But irrespective of this remoteness, humanity benefits from ecosystem services (ES) derived from Antarctica and the Southern Ocean. The services Antarctic ecosystems deliver are of regional and global significance and include climate regulation through uptake of CO2 from the atmosphere, provision of fish for human consumption, or cultural services such as aesthetic value or cognitive effects (Grant et al., 2013; Deininger et al., 2016).
Antarctic ecosystems, including the Antarctic Peninsula (AP) region and its surrounding seas (see Figure 1), have been exploited and impacted by human activity since the eighteenth century. Significant economic activities have encompassed sealing, whaling, fishing, and harvesting of birds including penguins (Constable et al., 2000; Kock, 2007). This exploitation led to an alarming over-utilization of living resources in the region, most notably the severe depletion of baleen whales (Leaper and Miller, 2011). The globally recognized need for conservation and sustainable use of Antarctic resources and ecosystems led to the establishment of several international conventions and governance mechanisms, such as the International Convention for the Regulation of Whaling (ICRW) in 1948 and the Antarctic Treaty System (ATS) which includes international agreements such as the Convention for the Conservation of Antarctic Marine Living Resources (CAMLR Convention) besides the Antarctic Treaty itself (Kock, 2007; Nyman, 2018; Secretariat of the Antarctic Treaty, 2019). The CAMLR Convention and the Protocol on Environmental Protection to the Antarctic Treaty, which entered into force in 1982 and 1998, respectively, are the two main pillars of the ATS. Aiming to protect and conserve Antarctica's unique environment for humankind, the treaties request inter alia environmental impact assessments for any kind of activity in the region, prohibit discharge from ships to prevent marine pollution, establish specific forms of protected areas such as Antarctic Specially Protected Areas (ASPAs) and Antarctic Specially Managed Areas (ASMAs), and regulate Antarctic fisheries through ecosystem-based management approaches (Constable et al., 2000; Aronson et al., 2011; Constable, 2011; Hughes et al., 2018; Roura et al., 2018).
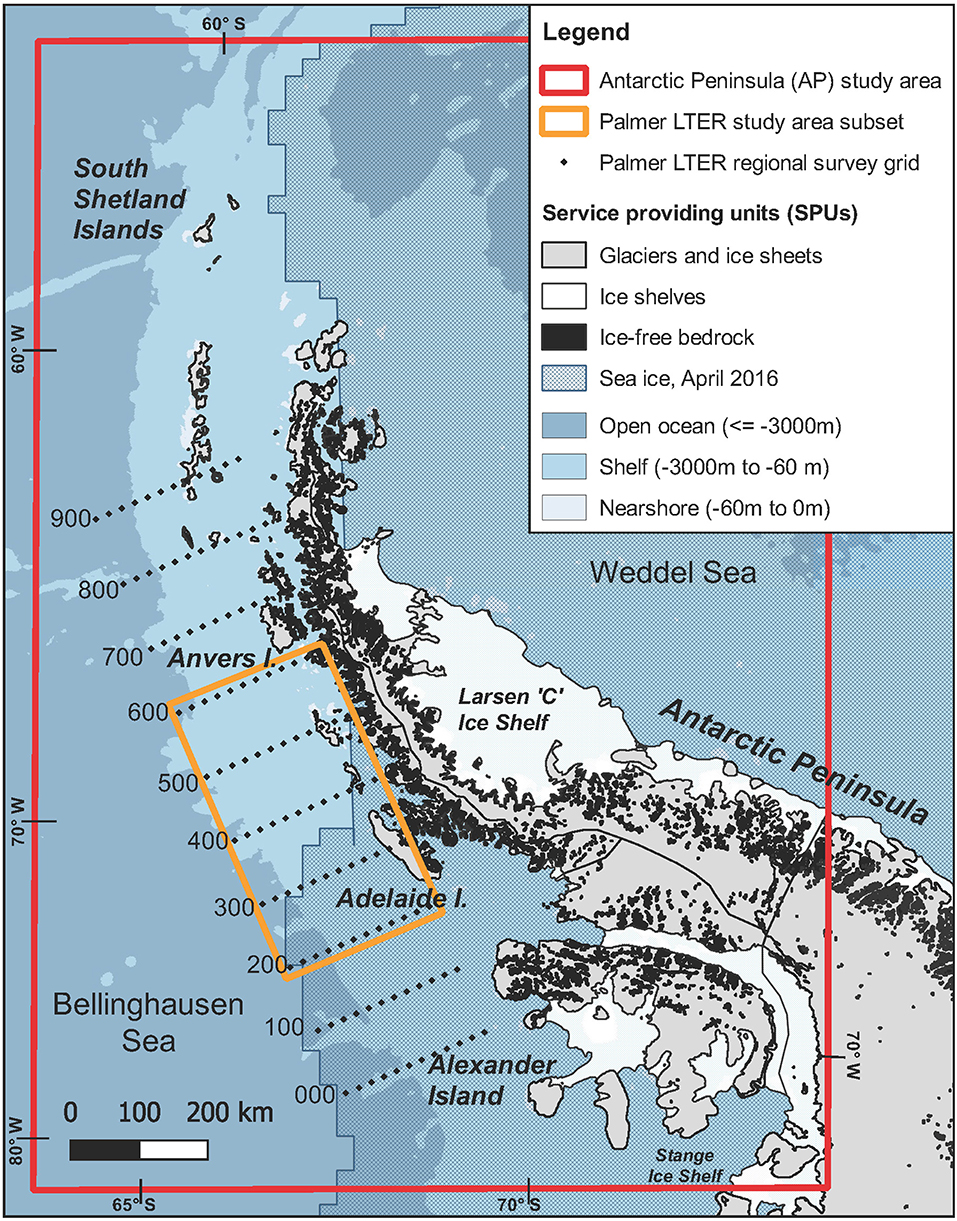
Figure 1. Antarctic Peninsula study area. Data sources: Morgan et al. (2007), Amante and Eakins (2009), Fetterer et al. (2016), Pal LTER (2016). Numbers indicate the sampling lines of the Palmer LTER regional survey grid. Map projection: WGS 84/Antarctic Polar Stereographic.
But despite this comprehensive protection and management framework, human activities continue to affect and change Antarctic ecosystems. On-going exploitation of marine resources, increasing presence and activities of humans in the region (Bender et al., 2016; Brooks et al., 2019), and rapid environmental change including climate change put Antarctica's terrestrial, coastal and marine ecosystems under increasing pressure (Aronson et al., 2011; Ducklow et al., 2013; Constable et al., 2014; Gutt et al., 2015). This threatens the sustained provisioning of ecosystem services from the region (Bölter and Müller, 2016; Longo et al., 2017), and economic or political interests put increasing pressure on the ATS and its comprehensive approach to long-term conservation of Antarctica and its ecosystems and resources (McGee and Liu, 2019).
The AP region is a unique environment due to several specific abiotic, biotic and anthropogenic conditions. Spanning the gradient from Antarctic to sub-Antarctic conditions, the region is a hotspot for avifauna and large marine predators, with large populations of penguins, seals, and whales supported by high rates of primary production (Hassan et al., 2005; Ducklow et al., 2013). These favorable conditions are provided by a complex interplay between sea ice, bathymetry, and ocean circulation. Winter sea ice cover allows the water column to stratify after fall deep-mixing events, setting the stage for the spring phytoplankton bloom (Bowman et al., 2018), while submarine canyons transiting the shelf enable the topographically induced upwelling of nutrient-rich Upper Circumpolar Deep Water (UCDW) (Kavanaugh et al., 2015). This careful balance of conditions is prone to variations in sea ice cover and the strength of the westerly winds that drive surface ocean circulation patterns south of the Polar Front. The AP region has experienced the highest rate of atmospheric warming in the whole Southern Hemisphere (Larsen et al., 2014); since 1950 the winter surface air temperature has risen as much as 6°C (Turner et al., 2009). Furthermore, changes in the extent of sea ice, a retreat of glaciers and the loss of six ice shelves are evidence for ongoing rapid environmental changes in the region (Stammerjohn et al., 2008). In addition to these rapid environmental changes and despite the ATS, the AP region and surrounding oceanic sectors still experience anthropogenic pressures such as the exploitation of living resources from fishing industries (Hassan et al., 2005) and increasing ship traffic and tourism (Aronson et al., 2011; Liggett et al., 2011).
Although the AP region is a nexus for research on the Antarctic environment, major questions remain regarding the trajectory of AP ecosystems and its vulnerability to climate and anthropogenic stress. Recent work emphasizes the importance of deepening the knowledge of Antarctica's natural and anthropogenic systems and their coupling in order to tackle the issues and provide robust advice for conservation and management (Chown et al., 2012; Kennicutt et al., 2015; Bölter and Müller, 2016; Longo et al., 2017; Waller et al., 2017). Particularly, the region's relevance for the global climate system, its significance for human well-being, and the potential for economic exploitation suggests a need for new methods to disentangle these interactions and understand effects and impacts. The ES concept offers a framework to assess “the benefits people obtain from ecosystems” (Millennium Ecosystem Assessment, 2005), and to analyze and understand those connections and interdependencies between the natural and the anthropogenic system at larger scales. Despite criticism and issues of operationalization, the mapping and assessing of ES has been recognized as a suitable tool to inform and support decision making for conservation and sustainable use of the natural resources (Guerry et al., 2012, 2015; Hauck et al., 2013; Maes et al., 2013; Schleyer et al., 2015), and to integrate a diversity of values in environmental governance (Jacobs et al., 2016).
A range of methods, approaches, and guidelines has been developed to conduct ES assessments including biophysical, monetary and socio-cultural methodologies, promoting inclusive valuations and tiered approaches, and advocating for an improved integration of stakeholder knowledge (Jacobs et al., 2014; Gret-Regamey et al., 2015; Bennett, 2016; Potschin et al., 2016; Barton and Harrison, 2017). Progress has also been made in establishing ecosystem service assessments for coastal and marine areas (Barbier, 2012; Drakou et al., 2017a). The matrix method (Burkhard et al., 2009, 2012; Jacobs et al., 2015) is an established expert-based assessment method which has been employed in a range of studies to examine the supply of or demand for ES (Burkhard et al., 2014; Sohel et al., 2015), including for coastal and marine areas (Kaiser et al., 2013; Stoll et al., 2015; Geange et al., 2019). But the assessment of ES for marine areas proves challenging due to the transitionary and fluid character of ecosystems, the complexity of interactions and other issues such as lack of appropriate data (Guerry et al., 2013; Townsend et al., 2014; Drakou et al., 2017a,b), making it difficult to appropriately define marine service providing units (SPUs). The definition of SPUs, geospatial units that are characterized by specific biotic and abiotic ecosystem components from which ecosystem services are delivered (Burkhard et al., 2014), is an essential component in the application of the matrix method. In terrestrials assessments, SPUs are commonly determined from readily available land use/land cover data or other biogeophysical regionalization derived from remote sensing data or land surveys (Burkhard et al., 2012), while the establishment of robust marine SPUs or “seascapes” is more complex due to the specific characteristics of marine ecosystems (Hidalgo et al., 2016; Kavanaugh et al., 2016; Manderson, 2016).
Here we leverage expertise from the Palmer Long Term Ecological Research (LTER) project, an interdisciplinary science project initiated in 1993, to estimate ES along the AP employing an expert-based assessment approach, the matrix method. We employ and compare a novel data model to describe marine SPUs from physico-chemically defined seascape units (SUs) (Bowman et al., 2018) against conventional SPUs, and introduce the application of objectively defined seascape data with the matrix method as a potentially transformative tool for ES valuation in Antarctica, and in marine environments more broadly.
Materials and Methods
The Study Area
The study area we have defined for this assessment is oriented along the AP and extends from ~200 km north of the South Shetland Islands to the Stange Ice Shelf south of Alexander Island (Figure 1). It includes parts of the Bellinghausen Sea in the west and extends into the Weddell Sea in the east, both marginal seas in the greater Southern Ocean system. The AP study area encompasses the largely glaciated Antarctic Peninsula which also features interspersed ice-free bedrock areas and ice shelves extending into the bordering seas. The marine portions of the AP study area are further characterized by sea ice, with permanent sea ice covering large sections of the Weddell Sea and in the Bellinghausen Sea between Adelaide Island and Alexander Island, and seasonal sea ice extending as far north as the South Shetland Islands.
The Palmer LTER regional survey grid (Waters and Smith, 1992; Pal LTER, 2016) is located on the western side of the AP and spans from the continental slope (depth > 3,000 m) to coastal waters (Figure 1). For this part of the AP study area, Bowman et al. (2018) had developed a novel approach to classify seascapes. We employed the derived SUs in this study as an alternative data model to define SPUs for a subset of the Palmer LTER regional survey grid area (see Figure 1), and to test their applicability to the matrix method.
When referring to coastal areas, we follow (Woodroffe, 2002) who defined coastal areas as a transition zone or interface between land and sea in which both terrestrial and marine environments influence each other. For the AP region, we therefore consider coastal areas to encompass near-shore coastal waters to a depth of 60 m, as well as sea ice, ice shelves, ice-free bedrock and glaciers where present along the shoreline.
Expert-Based Assessment of Ecosystem Service Supply
In order to assess the actual supply of ES per SPU for the AP region, we applied the matrix method, a qualitative valuation approach that links spatially explicit SPUs to a defined set of ES and employs expert elicitations (Burkhard et al., 2009, 2012; Jacobs et al., 2015). The matrix method reduces the complexity of human-environmental systems, allows for reproducibility and has proven useful in addressing the urgency-uncertainty dilemma for ES assessments, i.e., to provide best available knowledge where detailed modeling is not feasible or where data gaps obstruct more explicit approaches (Jacobs et al., 2015). Four key procedures are part of a classical matrix method assessment and were implemented in this study as follows: First, a set of key ES was selected and described for the defined study area (see section Selecting and Describing Key Ecosystem Services). Second, SPUs were defined and mapped out; here, we employed and tested two different approaches to classifying marine areas as described in section Classifying Service Providing Units. Third, a tabular matrix of ES and SPUs and additional information material were presented to experts in one-on-one interview sessions; during these sessions, experts were requested to estimate the actual supply of the described ES from each of the classified SPUs (section Conducting Expert Interviews). By ES supply we understand the actual benefits to people provided by ES from the natural system within a given time period, building on the definition of “ecosystem service flows” of Burkhard et al. (2014). Finally, the derived data were analyzed to create summary assessments of ES supply per SPU for the defined study area (section Analyzing the Data From the Expert Interviews).
Selecting and Describing Key Ecosystem Services
An appropriate selection and description of ES is essential for assessments that employ expert elicitations to estimate the intensity of ES supply. As this study aimed to apply the matrix method to the AP region and to test the applicability of a new SPU data model with this method, we worked along two criteria when selecting a suitable set of ES and developing descriptive indicators for these: (i) representativeness of and relevance to the study area, and (ii) methodological constrains. For this purpose, recent studies and literature outlining ES classifications for biodiversity-related assessments (Millennium Ecosystem Assessment, 2003; Burkhard et al., 2012; Haines-Young and Potschin, 2013; Kandziora et al., 2013a), describing ES assessments in marine and coastal areas (Liquete et al., 2013; European Commission, 2014; Charles et al., 2015) and investigating ES for the Southern Ocean and Antarctica (Grant et al., 2013; Deininger et al., 2016) were consulted to identify a suitable set of ES for the AP region. A comparative table listing the various ES classes that were proposed or utilized by the above studies served as source document for the identification of relevant services for the study area (see Supplementary Table 2). The selection of services was based on literature sourced on the various ES topics as well as on own expert judgement. Following criterion (i), potential ES candidates were scrutinized against aspects like the actual occurrence of an ES in the study area and the significance of the respective service for ecosystem functioning, ecosystem integrity, service security and ecosystem management in the study area. ES that had been proposed or utilized by other studies but did not prove relevant for the study area were dismissed (see Supplementary Table 2). In consideration of experience made in earlier qualitative ES assessment studies, and against the defined scope and setup of this study in which two types of ES-SPU combinations should be elicited within 1-h semi-structured interview sessions (see section Conducting Expert Interviews), it was further decided that the final set of ES in this study should not exceed 12–15 ES classes, i.e., cover 4–5 ES per ES category [selection criterion (ii)].
We derived a best estimate in a bundle of 13 key ES across the three main ES categories: provisioning, regulating and cultural services (Table 1). Once the ES selection was completed, two scoping interviews were conducted in advance of the actual expert interviews among the co-authors of this study to further adjust the interview schedule and confirm the usability of the selected set of ES. One main criterion here was whether the selected 13 ES and two sets of ES-SPU combinations could sensibly be evaluated during 1-h one-on-one interview sessions which encompassed several components: besides the elicitation of the actual supply of ES per SPU for the two different SPU models presented in this paper, experts were tasked to describe which of the ES in the AP region they considered most threatened by human activities, to suggest measures to sustain the resilience of ecosystems and maintain the provision of ES for future generations, and to draw on a paper map possible priority sites for conservation. These additional components of the conducted expert interviews are not further discussed here, but since they took time from the allocated 1-h interview slots, we consider it relevant to mention. Finally, in consideration of criterion (i), a total of 13 ES was chosen and considered appropriate for the scope of this study. All of the selected ES were underpinned with descriptive indicators and units of reference, where applicable (Table 1), upon which experts should base their rating of the actual supply of each of the described ES (see also section Conducting Expert Interviews).
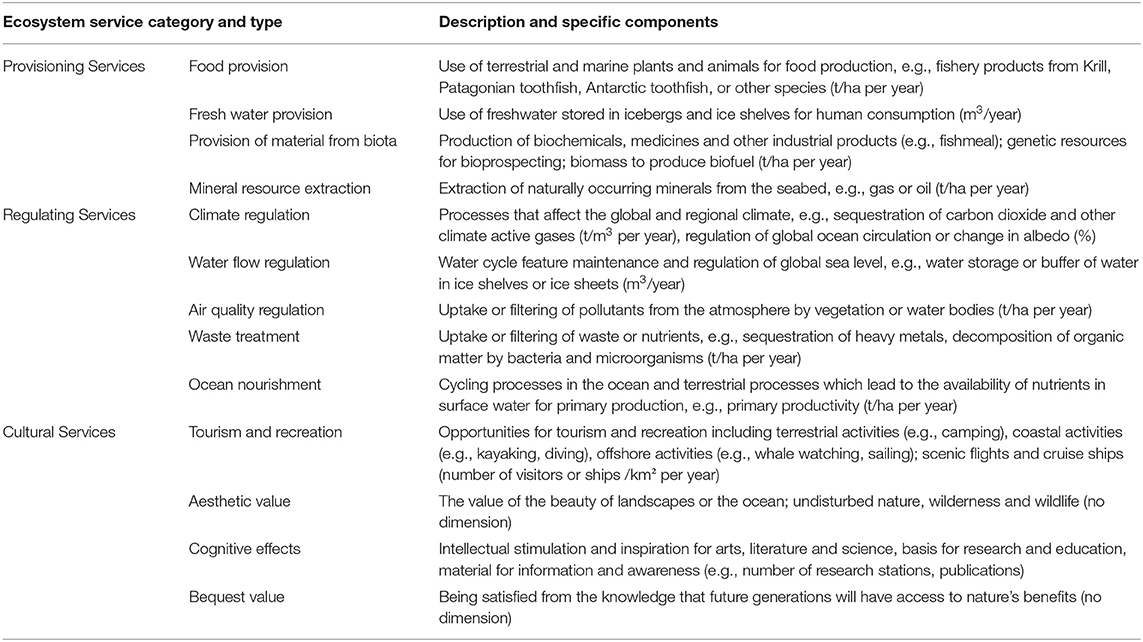
Table 1. Key ecosystem services of the case study region (developed from Millennium Ecosystem Assessment, 2005; Grant et al., 2013; Guerry et al., 2013; Charles et al., 2015; Deininger et al., 2016).
Under provisioning services we considered the provision of food, reflecting the role of the region for certain types of fisheries such as Antarctic krill (Euphausia superba) and Patagonian or Antarctic toothfish (Dissostichus eleginoides and Dissostichus mawsoni, respectively) fisheries (Fallon and Stratford, 2003; Collins et al., 2010; Ainley and Pauly, 2014). In addition, the provision of water, of material or products from biota, and mineral resource extraction were included in this category. The latter was taken into consideration despite current prohibition of commercial mineral resource extraction under the ATS in order to elicit possible non-commercial extraction (Hughes et al., 2016). The service “provision of material or products from biota” refers to industrial resource uses such as krill harvesting for the production of fishmeal or biochemical products, and to bioprospecting for genetic resources as part of marine research (Grant et al., 2013; Furbino et al., 2014; Alvarado-Cuevas et al., 2015).
We selected five regulating services of high regional and global relevance: climate regulation, water regulation, air quality regulation, waste treatment, and ocean nourishment (Table 1). For example, the deep ocean water masses of the Southern Ocean play an important role for the uptake and transport of heat and CO2, and Antarctic Bottom Water, produced in key spots around the Antarctic continent, is a major driver of global thermohaline circulation (Ducklow et al., 2012; Purkey and Johnson, 2013). Antarctic ice shelves and glaciers act as storage and buffer of water (Turner and Overland, 2009) with potentially large global effects if changes occur to the intensity of the related ES due to climate change impacts (Nicholls et al., 2011). Marine and coastal ecosystems in the AP region also have important effects on regional and global air quality (Charles et al., 2015), and provide waste treatment through decomposition of organic matter or sequestration of heavy metals (Guerry et al., 2013). Ocean nourishment, supported by nutrient cycling as well as by exogenous nutrient inputs from terrestrial processes (Charles et al., 2015), reflects another regulating ES in the AP region. A complex interplay between wind and sea ice can lead to high concentrations of nutrients in the photic zone and to high levels of primary production (Ducklow et al., 2012).
Antarctica and the AP region further deliver important cultural services by providing opportunities for tourism and recreation (Liggett et al., 2011; Bender et al., 2016; Vila et al., 2016). This augments the supply of considerable cognitive effects such as scientific resources, resulting scientific research on the Antarctic continent and its surrounding seas (Kennicutt et al., 2015; Hughes et al., 2018; Brooks et al., 2019), and of important other non-use values such as aesthetic and bequest values, which we also included this assessment (Table 1).
Classifying Service Providing Units
Two approaches to defining SPUs, i.e., characteristic spatio-temporal units from which ES are supplied, were employed and tested in this study. First, we considered a classical approach that defines SPUs on the basis of geospatial land and ice cover, and relief data; this was done for the entire AP study area. Second, we considered a novel classification of recurrent SUs which builds on physico-chemical parameters; this dataset had been developed by Bowman et al. (2018) for a subset of the Palmer LTER regional survey grid (see Figure 1).
The classical approach to defining and mapping SPUs was conducted for the greater AP study area. It builds on three geospatial datasets (Table 2): So-called environmental domains as developed by Morgan et al. (2007) for Antarctica from abiotic environmental variables, a global relief model (Amante and Eakins, 2009), and a dataset showing the median extent of sea ice for April 2016 (Fetterer et al., 2016). These datasets were processed with ArcGIS® and QGIS, Geographic Information System (GIS) software, to create a thematic map of the AP study area featuring the defined SPUs (see Figure 1). For this purpose, a geospatial shapefile of environmental domains of Antarctica (Morgan et al., 2007) was reclassified for the AP study area along principal land cover characteristics into glaciers (includes ice sheets and glaciers), ice-free bedrock, and ice shelves (Table 3). The bathymetric reclassification of the global relief model for the marine section of the study area into open ocean, shelf incl. shelf break, and near shore follows Ducklow et al. (2007) who suggest that these units can be linked to specific ocean dynamics in the AP region. As the observation time for this study was set to cover the Antarctic summer months, the margin of the median sea ice extent as recorded for April 2016 by Fetterer et al. (2016) was used to differentiate ice-free from ice-covered marine areas. Sea ice plays an important role for the polar ecosystem of the AP region which is influenced by strong seasonal and inter-annual sea ice dynamics (Ross et al., 2008; Stammerjohn et al., 2008; Ducklow et al., 2013). All of these SPUs present fixed units, but they may change slowly with time due to processes such as sea level rise or the melting of ice shelves and glaciers.
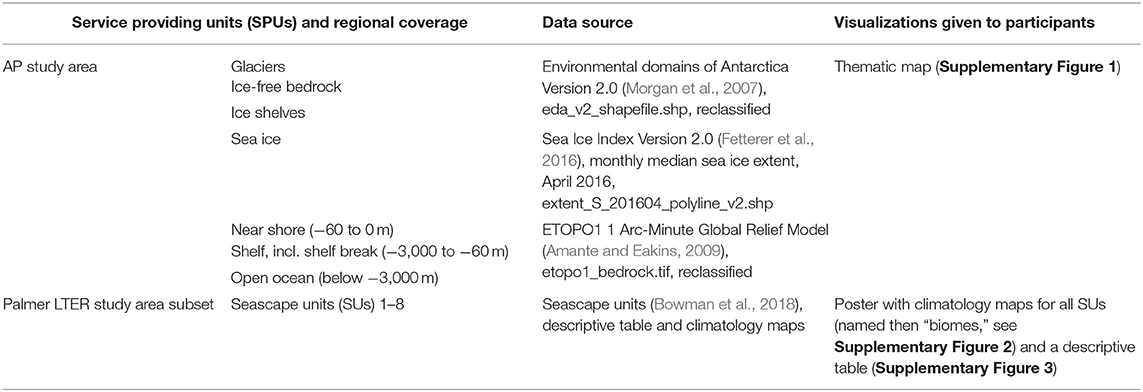
Table 2. Overview of ecosystem service providing units (SPUs) used for the valuation of ecosystem services (ES) in the Antarctic Peninsula (AP) region.
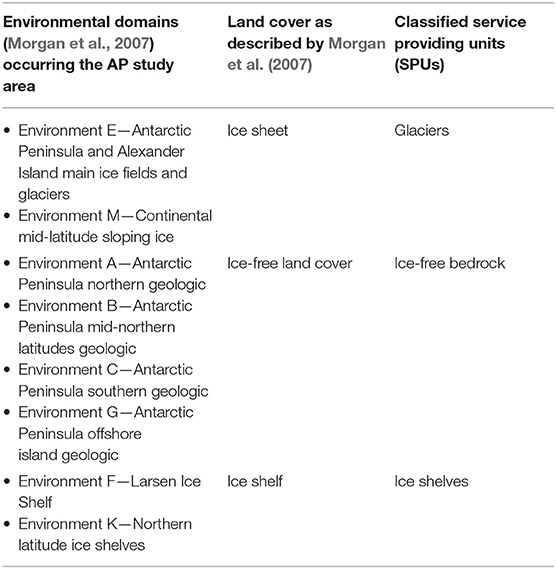
Table 3. Reclassification of the environmental domains of Antarctica dataset into SPU definitions for the AP study area.
For the Palmer LTER study area subset, a novel data model describing recurrent SUs as developed by Bowman et al. (2018) was employed to explore an alternative concept of SPU definition for coastal and open ocean waters. Similar to terrestrial environments, marine areas can be described as a three-dimensional mosaic of different habitats or “seascapes” with distinct characteristics reflecting their physical, chemical, and biological nature and occurrence in space and time (Hidalgo et al., 2016; Kavanaugh et al., 2016; Manderson, 2016). Employing a self-organizing map (SOM) approach to classify spatially interpolated data at selected depths, Bowman et al. (2018) had defined eight SUs from key physico-chemical parameters (temperature, salinity, phosphate, silicate, nitrate, nitrite, and chlorophyll a) collected from 21 Palmer LTER research cruise datasets between 1993 and 2014, covering the area between line 200 and line 600 of the Palmer LTER regional survey grid. Table 4 gives an overview of these SUs with a short description of their physico-chemical and spatio-temporal characteristics. In order to visualize the summed occurrence of the classified SUs for the period 1993 to 2014 at depths of 5 m, 20 m, and 80 m, a set of climatology maps was developed for each SU. For instance, SU 1 occurred onshore and offshore over the whole Palmer LTER study area subset, but generally occurred more often offshore and was only rarely observed onshore (Figure 2). Another characteristic of SU 1 is that it occurred more frequently in surface water than in deeper water bodies. Further, SU 1 is comparatively low in salinity and macronutrients, and it is characterized by elevated temperature while chlorophyll is moderately low; this combination suggests water that has already been depleted in nutrients by phytoplankton growth. In contrast, SU 2, which is found below the photic zone, has been rarely observed and does not occur offshore. Its water is significantly colder than that of other SUs, and it shows intermediate salinity and elevated nutrient contents while chlorophyll is rather low. These are recognizable characteristics of remnant “winter water” which has the potential to support a phytoplankton bloom if it reaches the surface ocean.
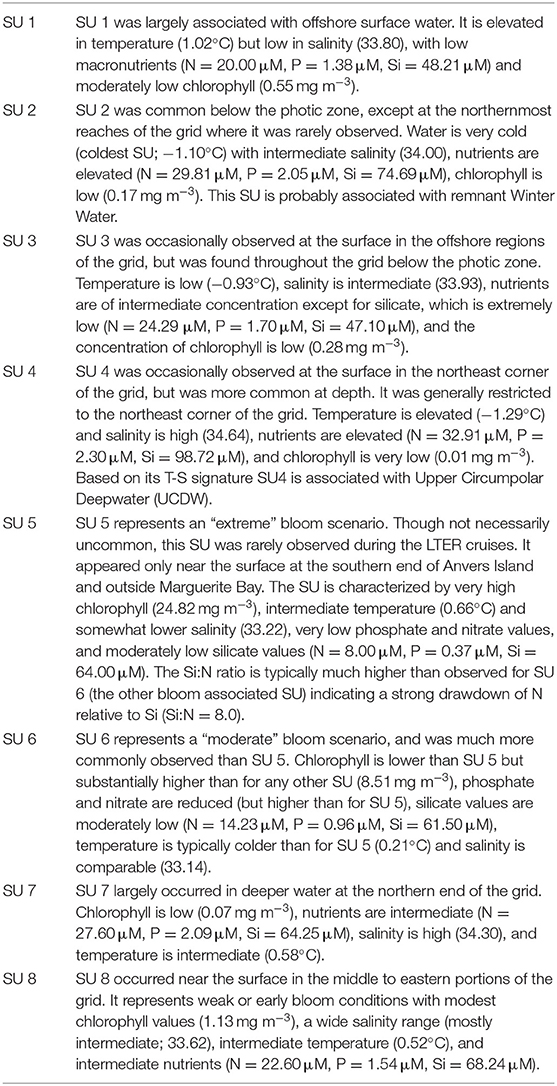
Table 4. Description of the physico-chemically defined seascape units (SUs) developed for Palmer LTER study area subset (see also Bowman et al., 2018).
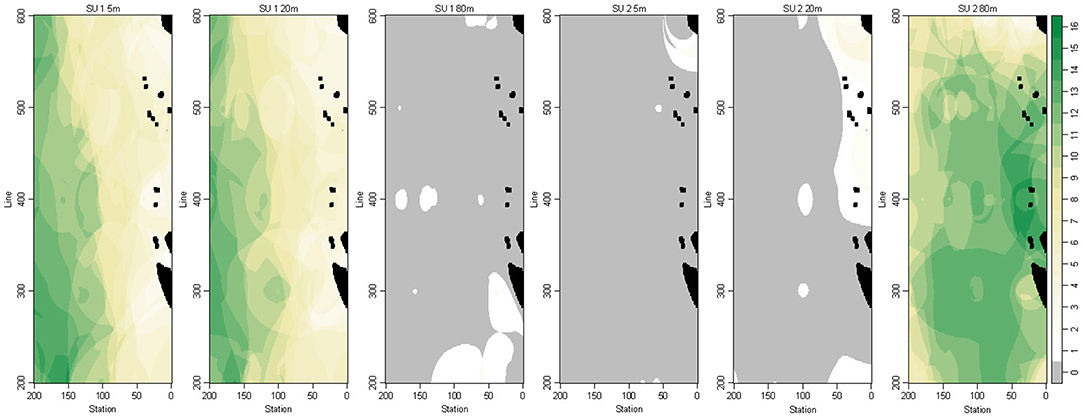
Figure 2. Physico-chemical seascape units (SUs) 1 and 2 in a water depth of 5 m, 20 m and 80 m visualized as number of occurrences between 1993 and 2014 (after Bowman et al., 2018, modified). “Station” refers to the sampling station within a sampling line of the Palmer LTER regional survey grid.
The climatology maps for all eight SUs were collated into an informative poster together with further information on the data set, and shared with the experts as information material at the interview sessions (see also section Conducting Expert Interviews). When conducting the expert interviews in 2016, an earlier version of SU climatology maps had been used; these were showing the same data for the defined SUs but which, at the time, were termed “biomes” (see Supplementary Figure 2).
Conducting Expert Interviews
To assess the supply of key ES from the different SPUs defined for the AP region, researchers from the Palmer LTER project were approached and invited to participate in structured expert interviews on the occasion of the annual science meeting of the Palmer LTER project. The meeting, which took place from 26 to 30 September 2016 at Rutgers University in Brunswick, NJ USA, offered the opportunity to arrange interview sessions with these experts during 4 days of the meeting. Thirteen experts had been identified and contacted by the co-authors working on the Palmer LTER project prior to the meeting; criteria for selection were their involvement in research in the AP region, familiarity with the study area and appropriate expertise in marine sciences to ensure that the experts were capable of understanding and interpreting the novel seascape data model applied in this study. Their expertise and backgrounds covered biological and physical oceanography, polar ecology with specifications into penguins, seabirds, marine mammals, conservation ecology, polar microbial and zooplankton ecology, biogeochemistry, and climate. Their work experience in the AP region varied from 3 to 42 years (see Supplementary Table 1). This specific pool of experts had been targeted because the novel data model employed in this study to elicit ES for marine SPUs through recurrent SUs had been developed by Bowman et al. (2018) for the Palmer LTER regional survey grid the area. By engaging with experts that have been engaged in the Palmer station and Palmer LTER research project, we hoped to cover a wide range of expertise while ensuring that the selected experts would be in a good position to understand and interpret this new data model towards the actual supply of ES. However, some relevant fields of expertise were missing from the elicited pool of experts, such as geology or glaciology, which may have contributed to recorded uncertainties in responses as discussed in section Implications and Uncertainties of the Employed Methods and Data Models.
The pre-scheduled face-to-face interviews were conducted between the 27 and 30 September 2016 at the sidelines of the Palmer LTER project's annual science meeting. In advance of the interviews, all interviewees had been informed about the study and its aims through a participant information sheet which was sent together with the invitations (see Supplementary Figures 20, 21). They received further briefing on the study during a joint workshop session at the beginning of the Palmer LTER annual science meeting in which extensive information on the data model and conceptual approach was shared with the experts before the actual interviews were conducted. Thorough information of the experts before the interviews was considered essential for them to understand the general approach of the assessment, the ES concept, and the SPU models employed, but also to reduce possible bias and uncertainties (Jacobs et al., 2015). Materials provided to each expert during the interviews included a map of the AP study area showing the spatial distribution of classical SPUs for the AP region and the extent of the Palmer LTER regional survey grid (Supplementary Figure 1); a descriptive table on the defined SUs (Supplementary Figure 3) and a poster visualizing the spatio-temporal occurrence of each SU for depths of 5 m, 20 m, and 80 m (Supplementary Figure 2); a document explaining the selected ES through descriptive indicators (Supplementary Figure 4); and a blank ES-SPU matrix (Supplementary Figure 5) which was used to guide and record the experts' ratings of ES supply for the different SPUs during the interviews.
At the beginning of each interview, personal information such as profession, field of expertise, duration of engagement with the Palmer LTER project or the Palmer station as such, and age was collected from the participant. Recording such information is a suitable standard practice to understand and reduce possible bias when interpreting the interview data in this kind of studies (Jacobs et al., 2015). Besides briefly outlining the scope of the interviews in reference to the joint workshop held earlier, definitions of terminology such as ES, ES supply, and SPU were provided and the presented material was explained (Supplementary Figure 6). Participants were then asked to estimate the actual supply of each ES per SPU using a Likert-type rating scale (Table 5). The rating scale allowed experts to choose values running from 0 (no relevant supply) to 5 (very high relevant supply) for a relative rating of the actual ES supply per SPU, as described by Burkhard et al. (2012) and Kandziora et al. (2013b). Experts were asked to make their estimates for summer months and relate to the recent year, i.e., to October 2015 to April 2016 (see section Conducting Expert Interviews). The estimates were manually recorded by the interviewer on the ES-SPU matrix. Estimates made present relative judgements of ES supply along each SPU and across all ES-SPU combinations for the AP study area and the Palmer LTER study area subset. All interviews were audio-recorded for control-purposes and anonymized for the analysis.
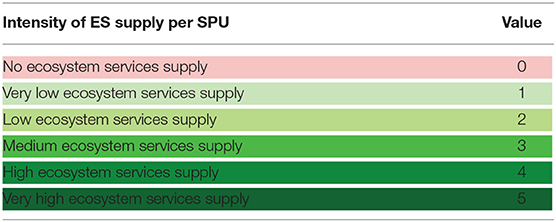
Table 5. Estimate scale applied for the valuation of intensity of ecosystem service (ES) supply from service providing units (SPUs).
Analyzing the Data From the Expert Interviews
From the recorded responses of estimated ES supply per SPU, five-number summaries of median, upper and lower quartiles, and minimum and maximum values were taken after an initial exclusion of outliers (see Supplementary Figures 7–19). Outliers were accounted for and excluded following the Tukey industry standard (Hoaglin, 2003), which defines outliers as points which lie 1.5 times the length of the interquartile range away from the limit of the upper or lower quartile. Outlier values were additionally cross-checked against recorded explanations given by participants during the interviews when assigning values to ES-SPU combinations. All outlier decisions could be interpreted as reasonable and final outlier exclusion remained unchanged against the statistical pre-test. Across all datasets, 62 data points from the classical SPU estimates and one data point from the SU estimates were excluded before conducting further data analyses. The integer median of ES supply estimates was used to summarize the interview data in a results matrix (Table 6). In four cases where the median did not show an even number, the mode was used for a final adjustment of the median results for the results matrix. In these cases, the numbers were brought up or down to a round figure, respectively. The results matrix of median expert-rated ES supply was color-coded along the color scheme of the applied estimate scale (Table 5) to visualize the intensity of ES supply for each SPU (see Table 6).
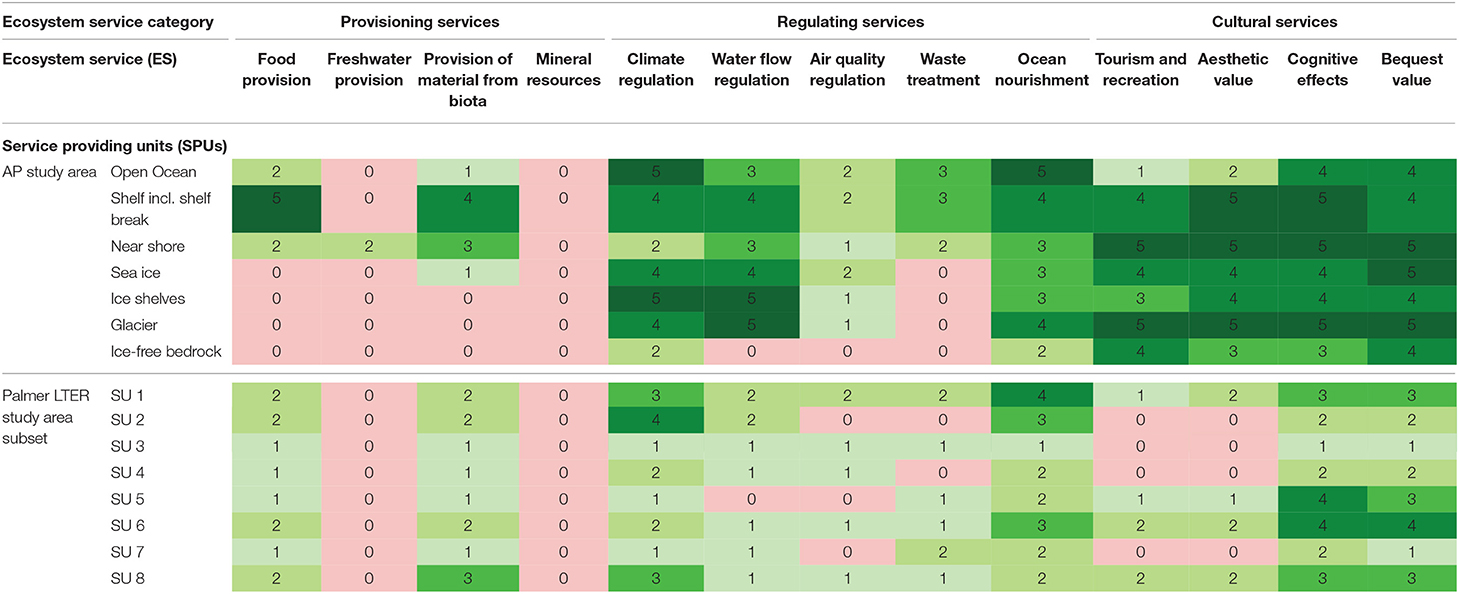
Table 6. Result matrix of median ecosystem service (ES) supply from service providing units (SPUs) in the Antarctic Peninsula (AP) study area and the Palmer LTER study area subset (See Table 5 for meaning of values assigned).
Commonly, geospatial maps are created from median ES supply estimates per SPU to visualize the results. But due to the 4-dimensional nature of the employed SUs and incompatibility of the different data types employed and produced, the derived ES supply estimates for the eight SUs could not be transferred back onto the climatology maps or processed into GIS maps. Therefore, comparative cross-analysis of the results from both SPU approaches was conducted from the results matrix and graphs presented in this paper (Table 6, Figures 3, 4, and Supplementary Figures 7–19).
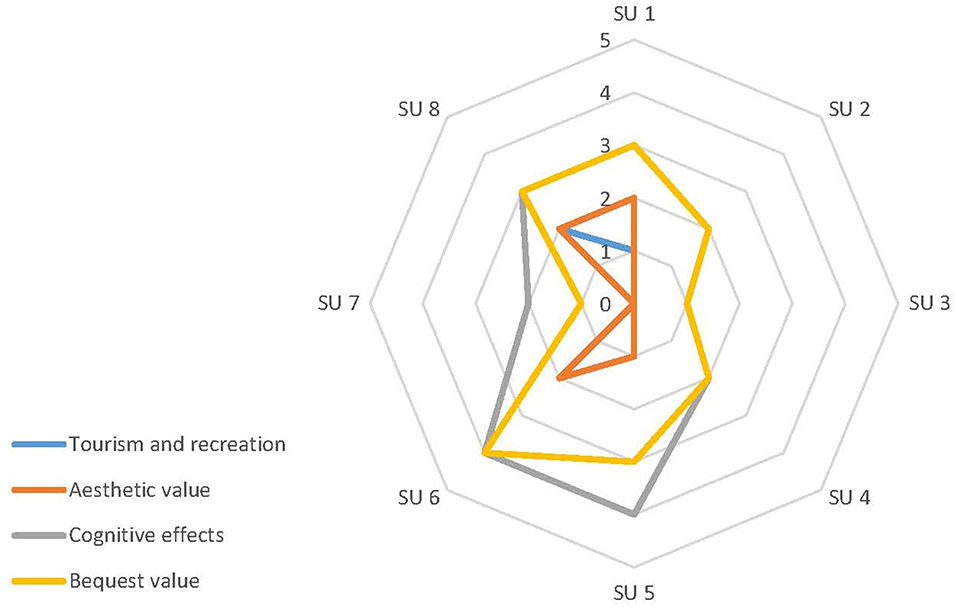
Figure 4. Median supply of cultural ES for seascape units (SUs) in the Palmer LTER study area subset.
Results
The results matrix (Table 6) shows the median supply of key ES as rated by experts for the different SPUs (i) across the entire AP study area, and (ii) for the Palmer LTER study area subset. On average, SU 1 to SU 8 received lower estimates than the conventional SPUs of open ocean, shelf including shelf break, and near shore, despite a spatial overlap between the conventional and the physico-chemically defined SPUs along the west coast of the AP. This discrepancy becomes especially obvious for cultural ES which were scored with up to 5 (very high ES supply) for several conventional SPUs, partially across all cultural ES, while SUs were scored with 4 (high ES supply) as highest estimates. Overall, ES supply estimates for SUs never received a rating higher than 4, while six of the seven conventional SPUs received ratings of 5 across eight of the thirteen described ES.
Provisioning ES generally received the lowest values in comparison to the regulating and cultural ES. Here, food provision and provision of materials from biota were estimated as very low (1) to low (2) across SUs with one exception, the provision of materials from biota for SU 8 which was rated as providing a medium supply (3). SU 8 occurs near the surface in the central to eastern section of the Palmer LTER study area subset and represents weak or early bloom conditions. Freshwater provision and mineral resources were both estimated to have an ES supply of 0 (no ES supply) in most SPUs with the exception of freshwater provision from near shore waters which received a median rating of 2 (low ES supply). In contrast to that, experts rated the supply of provisioning ES for shelf areas, i.e., marine areas between −60 m and −3,000 m depth, as high (4) for food provision and very high (5) for provision of material from biota when referring to conventionally defined marine SPUs.
Under the category of regulating services, climate regulation and ocean nourishment received high estimates for SU 1, which is largely associated with offshore surface waters that are low in salinity, macronutrients and moderately low in chlorophyll, and for SU 2, a rarely observed water mass occurring below the photic zone with elevated nutrient contents. In comparison, the bathymetrically defined marine SPUs (open ocean; shelf incl. shelf break; near shore) were generally estimated to provide much higher climate regulation, water flow regulation or ocean nourishment services, with values ranging between 2 (low ES supply) for near shore SPUs and 5 (very high ES supply) for open ocean SPUs. The non-marine coastal and terrestrial SPUs as well as sea ice were assumed to supply regulating ES at a relatively high level, with ice shelves and glaciers estimated to deliver high (4) to very high (5) climate and water flow regulation services. The lowest median ES supply in the category of regulating services was assigned to ice-free bedrock; this SPU was further estimated to supply no water flow regulation, air quality regulation, and waste treatment services.
The cultural ES examined in this study received high values across all conventional SPUs, while supply estimates were lower (cognitive effects; bequest value) to significantly lower (tourism and recreation; aesthetic value) for the physico-chemically defined SUs than for the conventionally described units. Glaciers, near shore marine areas and shelf incl. shelf break but also sea-ice were among the highest rated SPUs for cultural ES while open ocean was attributed a very low (1) to low (2) supply of touristic and recreational ES, and of aesthetic ES (see also Figures 3, 4). All conventional SPUs were rated as contributing to the delivery of cultural ES while four SUs received a rating of 0 (no ES supply); all of these SUs occurred below the photic zone or even deeper. Overall, highest values were assigned to ES of cognitive effects and bequest value across conventional SPUs and SUs, but differences between all four cultural ES are marginal.
From all conventional SPUs, ice-free bedrock was considered as the least relevant provider of ES except for cultural ES, while shelf incl. shelf break received the highest estimates across all ES. From the eight seascape units, SU 3, SU 4, and SU 7 received lowest estimates of ES supply across all categories.
Discussion
In the following, we highlight some interesting—and partially surprising—results that we gained through this qualitative, expert-based ES assessment for the AP region. Further we discuss lessons learned, uncertainties relating to the applied methods and summarize the main findings of this study.
Relevance of Regulating and Cultural Ecosystems Services
In general, this expert-based assessment confirmed our assumptions and results from other studies (Grant et al., 2013; Bölter and Müller, 2016; Deininger et al., 2016) suggesting that the AP region provides ES of regional and global importance: Regulating services (climate regulation, water flow regulation, and ocean nourishment) were estimated to be supplied at medium to very high rates from marine waters and ice-covered areas in the AP region; these estimates reflect the general importance that is assigned to ocean areas in the Earth system (Stocker, 2015). The supply of cultural services was rated as high to very high for the AP region for ocean, coastal and terrestrial features, except for open ocean areas and water masses found at greater depths as represented by seascape units SU 2, SU 3, SU 4, and SU 7. The biophysically SPUs SU 1–SU 8 received significantly lower scores for tourism and recreation and for aesthetic value than the classical SPUs, with zero ratings given to those four SUs that represent deeper water masses. These results likely reflect the fact that touristic activities in the AP region are mostly related to ship-based cruises with landings along the AP coastline (Liggett et al., 2011; Deininger et al., 2016), which results in physical or cognitive confrontation that this is mostly linked to coastal ocean and land surfaces, but less so to deeper ocean waters. The general high rating of cultural services for the AP region's classical SPUs meets with observations of growing tourism activities around Antarctica and the AP region (Liggett et al., 2011; Bender et al., 2016; Walton, 2018), but also with observations on humans perceptions of wilderness, and of aesthetic, cognitive and intrinsic values of Antarctica (Liggett et al., 2011; Summerson and Bishop, 2011; Hemmings, 2012; Bastmeijer and Tin, 2014). Interestingly, ice-free bedrock was not estimated to be of high relevance for most ES except for cultural services, which could be linked to the known preference of Adélie, gentoo, and chinstrap penguin colonies for these habitats as breeding and nesting sites, forming a major tourism attraction (Dunn et al., 2019). Similarly, when asked to rate the presented SPUs for cognitive effects and bequest value, experts assigned high to very high ES supply values to the classical SPUs while the physico-chemically defined SUs received much lower values even when occurring at the surface or presenting ecologically relevant water masses (SU1, SU5, SU6, SU8). SUs that occurred predominantly at greater depth and below the photic zone were not rated highly by experts. The recorded responses suggest that experts do not consider these units relevant for intellectual stimulation including science and research, or for future generations, which is surprising. Given the relevance of also nutrient poor waters and deeper water masses for the marine system, we rather assume that these low ratings fall back on uncertainties as discussed below and in section Implications and Uncertainties of the Employed Methods and Data Models.
The overall high rating of cultural services over regulating and provisional services across all conventional SPUs is remarkable when considering the remoteness of the AP region and the still relatively small numbers of visitors. This estimate could be influenced by a potential bias of the interviewed experts that have partially visited the AP region over decades, spent ample time there for their research and possibly highly value their workplace and study objects. On the other hand, a study by Summerson (2011, p. 40 ff) on perceptions of wilderness and aesthetic values on Antarctica did not show a significant difference between people that had experienced Antarctica through national research programs and those that had come as touristic visitors, nor were their aesthetic values significantly different from people that had never visited Antarctica. This suggests that despite the high relevance of Antarctica and the Southern Ocean for global climate regulation or certain types of fisheries, people value the region even more so for its cultural services.
On the part of uncertainties, the higher ratings across cultural and regulating service categories against provisioning services likely also reflect the following aspects: The set-up of the expert interviews had originally foreseen to elicit the actual supply against the potential supply, i.e., the “the hypothetical maximum yield of selected ecosystem services” (Burkhard et al., 2012, 2014). Therefore, also services such as mineral resource extraction or freshwater provisioning had been included in the list of elicited ES, both services with a large potential supply against a rather low actual use, i.e., supply. The extraction of mineral resources is at current prohibited under the Antarctic Treaty, but there is concern among experts that commercial interests in Antarctic mineral resources might lead to changes to the current prohibition of such activities in the medium term (Hemmings, 2012). However, due to a lack of time during most interviews, the planned assessment of potential against current ES supplies could not be completed and was therefore not included in this study. The zero estimates made by expert regarding the actual supply of mineral resources from the study area confirm the situation with the Antarctic Treaty prohibiting extraction. Similarly, we had expected that freshwater provision would likely have played higher role, too, when eliciting the potential supply of ES in addition to the actual supply. The ice masses of Antarctica bear a large potential for driving freshwater to use as drinking water or process water but which, at current and as estimated by the experts, seems to be tapped only in limited amounts by research stations or other semi-permanent human settlements in the AP region.
Implications and Uncertainties of the Employed Methods and Data Models
Estimates of ES supply derived through this study are quite different for the marine part of the AP study area when comparing the two data models that were employed to define SPUs. For example, the physico-chemically defined SUs received generally lower estimates than the conventional SPUs, especially for cultural ES. Although, from an ecological point of view, such a 4-dimensional classification of seascapes provides a much more detailed picture of the ecological conditions upon which ES such as food provision or climate regulation build, experts seemed to find it difficult to relate the presented ES to these features. But also for ES that have a more direct link to ecosystem structures and processes such as climate regulation or waste treatment, experts seemed to have difficulty in translating the physico-chemical characteristics of the classified SUs into ES. For example, we had expected that SU 5 and SU 6, representing two bloom scenarios, would be rated significantly higher for food provisioning as experts actually did.
The ES concept and assessment studies employing methods such as the matrix approach imply a certain simplification of ecological processes. This study presents a specific case in that the employed seascape data model requires experts to interpret the presented physico-chemical information into ecosystem functions, and from that into ES, which is possibly demanding too much from experts in the chosen interview setting. We therefore suggest that in future assessments, the linkages between ecosystem functions and processes, and ES should be presented more explicitly to experts when employing novel seascape data models such as the ones developed by Bowman et al. (2018) with the matrix method or other expert-based approaches. As Hou et al. (2013) point out, there is a range of uncertainties related to landscape modeling, which likely holds true for seascape modeling and should be taken into account when employing such data in ES assessments. Major issues are, from their perspective, (i) uncertainties relating to the complexity of ecosystem and the establishing of links between ecosystems and ES, (ii) uncertainties due to a potential inadequacy of participants' knowledge or experience for subjective judgements on ES, and (iii) technical uncertainties relating to the methods employed. These uncertainties have possibly featured in our study, although we had addressed issues (i) and (ii) by carefully preparing, conducting and documenting the expert interviews (section Conducting Expert Interviews), by excluding outliers possibly resulting from misinterpretation of the material presented to the experts (section Analyzing the Data from the Expert Interviews), and by due consideration of the specific methodological setup of this study when interpreting the results. Uncertainties might further be a matter of the diversity of marine science backgrounds of the interviewed experts falling short of some specific expertise (see section Conducting Expert Interviews), or of the wide amplitude of years of experience of the elicited experts. The selection of a group of specialized experts is common in such studies (Jacobs et al., 2015) but also bears uncertainties with respect to the rating of ES supply across the different ES categories.
An indication whether those uncertainties have played a role in our study could be the range of answers that was given by experts when rating the different ES-SPU combinations (see Supplementary Figures 7–19). This range is particularly broad for cultural ES in relation to SUs, but also for some regulating services such climate regulation. To better understand such uncertainties, we recommend that confidence scorings, as suggested by Jacobs et al. (2015) and tested by Campagne et al. (2017), or psychometric instruments or are included in future assessments that employ such methods and novel data models, which was unfortunately beyond the scope of this study. Alternatively, focus group settings could be introduced for such studies to account for and reduce possible bias and overconfidence of experts, a phenomenon that has been described by Singh et al. (2017) for expert interviews. However, also group elicitations bring about challenges, as Singh et al. (2017) write, and thus consensus rounds in addition to individual one-on-one interviews, as proposed by Jacobs et al. (2015), or a combination of individual interviews and focus groups as tested by Kaplowitz and Hoehn (2001) might deliver less biased results.
The notion that human society is directly dependent on nature and on the services it provides was put forth by Daily et al. (Daily, 1997; Daily et al., 2000), and has been taken up not only by scientists, but also by decision-makers and the civil society—though not yet to its full potential. In recent years the scientific community has drawn much attention to the opportunities and challenges of the ES concept and its application in assessments, increasingly also in the context of policy and decision making (Jacobs et al., 2016; Pandeya et al., 2016; Posner S. et al., 2016; Posner S. M. et al., 2016). With his study we hope to advance the assessment of ES for marine ecosystems and regions with large marine sections, including Antarctica and its surrounding ocean and seas. For example, an application of similar data models such as the global map of ecological marine units by Sayre et al. (2017) with the matrix method, and in consideration of the experiences made in this study, would be highly interesting in this context.
Conclusions
This study, which employed a qualitative ES assessment method while introducing a novel data model for the description of SPUs and testing against conventional land- and seascape units for the Antarctic Peninsula region, brought several innovations:
As first application of the matrix method to Antarctica on the case of the AP region, the assessment provides a first-order assessment of key ES supply from the region and on the basis of this method, emphasizing the relevance of cultural and regulating services in the AP region. Second, by engaging with a novel definition of seascapes, the study reveals the potential as well as uncertainties of different types of spatial regionalization methods for the definition of SPUs, and indicates needs for advancing objectively defined seascape definitions and their application in ES assessments. And last but not least, this expert-based study highlights the relevance of this special environment for humanity and the Earth System, and the need for strengthened conservation efforts. Tourism and fishing are expected to further intensify along the Antarctic Peninsula with potentially increasing pressures on the ecological system. The regulation of human activities at regional and global scales as well as appropriate area-based management and conservation efforts will thus be crucial to sustain the AP region's ES for current and future generations (Aronson et al., 2011; Liggett et al., 2011; Hughes and Grant, 2017).
Data Availability Statement
The raw data supporting the conclusions of this manuscript will be made available by the authors, without undue reservation, to any qualified researcher.
Ethics Statement
From the part of the German research institution at which the main part of the study was conducted, Kiel University, the approval of an ethics committee was not required for conducting expert interviews. However, the study employed a rigorous ethical approach to contacting and informing participants in advance of the study; receiving consent from participants to participate in one-on-one interviews; and granting anonymity to participants. Participants were informed about the background and purpose of the study, methods employed and demands, and possible risks in advance of the interviews. All participants gave their written consent to take part in one-on-one expert interviews and in the interview being audio recorded.
Author Contributions
BN, AM, JB, HD, and FM contributed conception and design of the study and wrote sections of the manuscript. BN, AM, and JB designed the expert elicitations and prepared the interview materials. AM conducted and analyzed the interview data. AM and BN performed the analysis and visualization of the results. BN and AM developed the first draft of the manuscript. All authors contributed to manuscript revision, and read and approved the submitted version.
Funding
Funding was provided to BN and AM by the Cluster of Excellence 80 The Future Ocean which is funded within the framework of the Excellence Initiative by the German Research Foundation (DFG) on behalf of the German Federal and State governments. The work of BN was further supported by the German Federal Ministry of Education and Research (BMBF) through its Research for Sustainable Development program (FONA), and the Federal State of Brandenburg. Funding was also provided by NSF-OPP 1641019 to JB, a Simons Foundation Early Career Marine Microbial Investigator award to JB, a grant from the World Surf League PURE initiative to HD, NSF-OPP 1440435 to HD and a gift from the Vetlesen Foundation to HD.
Conflict of Interest
The authors declare that the research was conducted in the absence of any commercial or financial relationships that could be construed as a potential conflict of interest.
Acknowledgments
We would like to thank all interview participants for their contributions and interest in the study, our donors for their support of this research, and the Cluster of Excellence The Future Ocean in Kiel, Germany, for match-making across disciplines and allowing our ideas for this study to develop. Furthermore, we would like to thank the reviewers of this article for their valuable feedback and input.
Supplementary Material
The Supplementary Material for this article can be found online at: https://www.frontiersin.org/articles/10.3389/fenvs.2019.00157/full#supplementary-material
References
Ainley, D. G., and Pauly, D. (2014). Fishing down the food web of the Antarctic continental shelf and slope. Polar Record 50, 92–107. doi: 10.1017/S0032247412000757
Alvarado-Cuevas, Z. D., López-Hidalgo, A. M., Ordoñez, L. G., Oceguera-Contreras, E., Ornelas-Salas, J. T., and De León-Rodríguez, A. (2015). Biohydrogen production using psychrophilic bacteria isolated from Antarctica. Int. J. Hydrogen Energy 40, 7586–7592. doi: 10.1016/j.ijhydene.2014.10.063
Amante, C., and Eakins, B. W. (2009). “ETOPO1 1 arc-minute global relief model: procedures, data sources and analysis,” in NOAA Technical Memorandum NESDIS NGDC-24, ed NOAA, National Geophysical Data Center (Boulder: National Geophysical Data Center, Marine Geology and Geophysics Division)
Aronson, R. B., Thatje, S., McClintock, J. B., and Hughes, K. A. (2011). Anthropogenic impacts on marine ecosystems in Antarctica. Ann. N. Y. Acad. Sci. 1223, 82–107. doi: 10.1111/j.1749-6632.2010.05926.x
Barbier, E. B. (2012). Progress and challenges in valuing coastal and marine ecosystem services. Rev. Environ. Econ. Policy 6, 1–19. doi: 10.1093/reep/rer017
Barton, D. N., and Harrison, P. A. (2017). Integrated Valuation of ecosystem services. Guidelines and Experiences. EU FP7 OpenNESS Project Deliverable 33-44. European Commission FP7.
Bastmeijer, K., and Tin, T. (2014). “Antarctica – a wilderness continent for science: the ‘public's dream' as a mission impossible?” in The Yearbook of Polar Law, eds. G. Alfredsson, T. Koivurova, and H. Ó. Ágústsson (Boston, MA; Leiden: Brill/Martinus Nijhoff Publishers). doi: 10.1163/1876-8814_020
Bender, N. A., Crosbie, K., and Lynch, H. J. (2016). Patterns of tourism in the Antarctic Peninsula region: a 20-year analysis. Antarctic Sci. 28, 194–203. doi: 10.1017/S0954102016000031
Bennett, E. M. (2016). Research frontiers in ecosystem service science. Ecosystems 20, 31–37. doi: 10.1007/s10021-016-0049-0
Bölter, M., and Müller, F. (2016). Resilience in polar ecosystems: from drivers to impacts and changes. Polar Sci. 10, 52–59. doi: 10.1016/j.polar.2015.09.002
Bowman, J. S., Kavanaugh, M. T., Doney, S. C., and Ducklow, H. W. (2018). Recurrent seascape units identify key ecological processes along the western Antarctic Peninsula. Glob. Chang Biol. 24, 3065–3078. doi: 10.1111/gcb.14161
Brooks, S. T., Jabour, J., Van Den Hoff, J., and Bergstrom, D. M. (2019). Our footprint on Antarctica competes with nature for rare ice-free land. Nat. Sustain. 2, 185–190. doi: 10.1038/s41893-019-0237-y
Burkhard, B., Kandziora, M., Hou, Y., and Müller, F. (2014). Ecosystem service potentials, flows and demands – concepts for spatial localisation, indication and quantification. Landscape Online 34, 1–32. doi: 10.3097/LO.201434
Burkhard, B., Kroll, F., Müller, F., and Windhorst, W. (2009). Landscapes' capacities to provide ecosystem services–a concept for land-cover based assessments. Landscape Online 15:22. doi: 10.3097/LO.200915
Burkhard, B., Kroll, F., Nedkov, S., and Müller, F. (2012). Mapping ecosystem service supply, demand and budgets. Ecol. Indicat. 21, 17–29. doi: 10.1016/j.ecolind.2011.06.019
Campagne, C. S., Roche, P., Gosselin, F., Tschanz, L., and Tatoni, T. (2017). Expert-based ecosystem services capacity matrices: dealing with scoring variability. Ecol. Indicat. 79, 63–72. doi: 10.1016/j.ecolind.2017.03.043
Charles, M., Mongruel, R., Beaumont, N., Hooper, T. L., Levrel, H., Thiébaut, E., et al. (2015). “Towards effective ecosystem services assessment in marine and coastal management,” in Routledge Handbook of Ecosystem Services, eds. M. Potschin, R. Haines-Young, R. Fish, and R. K. Turner (New York, NY: Routledge), 359–373. doi: 10.4324/9781315775302-31
Chown, S. L., Lee, J. E., Hughes, K. A., Barnes, J., Barrett, P. J., Bergstrom, D. M., et al. (2012). Challenges to the future conservation of the Antarctic. Science 337, 158–159. doi: 10.1126/science.1222821
Collins, M. A., Brickle, P., Brown, J., and Belchier, M. (2010). “Chapter 4: The patagonian toothfish: biology, ecology and fishery,” in Advances in Marine Biology, ed M. Lesser (Academic Press), 227–300. doi: 10.1016/B978-0-12-381015-1.00004-6
Constable, A. J. (2011). Lessons from CCAMLR on the implementation of the ecosystem approach to managing fisheries. Fish Fish. 12, 138–151. doi: 10.1111/j.1467-2979.2011.00410.x
Constable, A. J., De La Mare, W. K., Agnew, D. J., Everson, I., and Miller, D. (2000). Managing fisheries to conserve the Antarctic marine ecosystem: practical implementation of the Convention on the Conservation of Antarctic Marine Living Resources (CCAMLR). ICES J. Mar. Sci. 57, 778–791. doi: 10.1006/jmsc.2000.0725
Constable, A. J., Melbourne-Thomas, J., Corney, S. P., Arrigo, K. R., Barbraud, C., Barnes, D. K. A., et al. (2014). Climate change and Southern Ocean ecosystems I: how changes in physical habitats directly affect marine biota. Glob. Change Biol. 20, 3004–3025. doi: 10.1111/gcb.12623
Costanza, R. (1999). The ecological, economic, and social importance of the oceans. Ecol. Econ. 31, 199–213. doi: 10.1016/S0921-8009(99)00079-8
Daily, G. C. (ed.). (1997). Nature's Services: Societal Dependence on Natural Ecosystems. Washington, DC: Island Press.
Daily, G. C., Söderqvist, T., Aniyar, S., Arrow, K., Dasgupta, P., Ehrlich, P. R., et al. (2000). The value of nature and the nature of value. Science 289, 395–396. doi: 10.1126/science.289.5478.395
Deininger, M., Koellner, T., Brey, T., and Teschke, K. (2016). Towards mapping and assessing antarctic marine ecosystem services – The weddell sea case study. Ecosyst. Serv. 22(Pt A), 174–192. doi: 10.1016/j.ecoser.2016.11.001
Drakou, E. G., Kermagoret, C., Liquete, C., Ruiz-Frau, A., Burkhard, K., Lillebø, A. I., et al. (2017a). Marine and coastal ecosystem services on the science–policy–practice nexus: challenges and opportunities from 11 European case studies. Int. J. Biodivers. Sci. Ecosyst. Serv. Manage. 13, 51–67. doi: 10.1080/21513732.2017.1417330
Drakou, E. G., Pendleton, L., Effron, M., Ingram, J. C., and Teneva, L. (2017b). When ecosystems and their services are not co-located: oceans and coasts. ICES J. Mar. Sci. 74, 1531–1539. doi: 10.1093/icesjms/fsx026
Ducklow, H., Clarke, A., Dickhut, R., Doney, S. C., Geisz, H., Huang, K., et al. (2012). “The marine system of the Western Antarctic Peninsula,” in Antarctic Ecosystems: An Extreme Environment in a Changing World, eds A. D. Rogers, N. M. Johnston, E. J. Murphy, and A. Clarke (Chichester: John Wiley & Sons, Ltd), 121–159. doi: 10.1002/9781444347241.ch5
Ducklow, H. W., Baker, K., Martinson, D. G., Quetin, L. B., Ross, R. M., Smith, R. C., et al. (2007). Marine pelagic ecosystems: the West Antarctic Peninsula. Philos. Trans. R. Soc. B Biol. Sci. 362, 67–94. doi: 10.1098/rstb.2006.1955
Ducklow, H. W., Fraser, W. R., Meredith, M. P., Stammerjohn, S. E., Doney, S. C., Martinson, D. G., et al. (2013). West Antarctic Peninsula: an ice-dependent coastal marine ecosystem in transition. Oceanography 26, 190–203. doi: 10.5670/oceanog.2013.62
Dunn, M. J., Forcada, J., Jackson, J. A., Waluda, C. M., Nichol, C., and Trathan, P. N. (2019). A long-term study of gentoo penguin (Pygoscelis papua) population trends at a major Antarctic tourist site, Goudier Island, Port Lockroy. Biodivers. Conserv. 28, 37–53. doi: 10.1007/s10531-018-1635-6
European Commission (2014). Mapping and Assessment of Ecosystems and their Services. Indicators for Ecosystem Assessments Under Action 5 of the EU Biodiversity Strategy to 2020. Luxemburg: European Union.
Fallon, L., and Stratford, E. (2003). Issues of Sustainability in the Southern Ocean Fisheries – the Case of the Patagonian Toothfish. Hamburg: Lighthouse Foundation.
Fetterer, F., Knowles, K., Meier, W., Savoie, M., and Windnagel, A. K. (2016). “Monthly median sea ice extent, August, 1981-2010” in Sea Ice Index, Version 2, ed National Snow & Ice Data Center (Boulder, CO). doi: 10.7265/N5736NV7 (accessed October 03, 2017).
Furbino, L. E., Godinho, V. M., Santiago, I. F., Pellizari, F. M., Alves, T. M. A., Zani, C. L., et al. (2014). Diversity patterns, ecology and biological activities of fungal communities associated with the endemic macroalgae across the Antarctic Peninsula. Microb. Ecol. 67, 775–787. doi: 10.1007/s00248-014-0374-9
Geange, S., Townsend, M., Clark, D., Ellis, J. I., and Lohrer, A. M. (2019). Communicating the value of marine conservation using an ecosystem service matrix approach. Ecosyst. Serv. 35, 150–163. doi: 10.1016/j.ecoser.2018.12.004
Grant, S. M., Hill, S. L., Trathan, P. N., and Murphy, E. J. (2013). Ecosystem services of the Southern Ocean: trade-offs in decision-making. Antarctic Sci. 25, 603–617. doi: 10.1017/S0954102013000308
Gret-Regamey, A., Weibel, B., Kienast, F., Rabe, S. E., and Zulian, G. (2015). A tiered approach for mapping ecosystem services. Ecosyst. Serv. 13, 16–27. doi: 10.1016/j.ecoser.2014.10.008
Guerry, A. D., Polasky, S., Lubchenco, J., Chaplin-Kramer, R., Daily, G. C., Griffin, R., et al. (2015). Natural capital and ecosystem services informing decisions: from promise to practice. Proc. Natl. Acad. Sci. U.S.A. 112, 7348–7355. doi: 10.1073/pnas.1503751112
Guerry, A. D., Ruckelshaus, M. H., Arkema, K. K., Bernhardt, J. R., Guannel, G., Kim, C.-K., et al. (2012). Modeling benefits from nature: using ecosystem services to inform coastal and marine spatial planning. Int. J. Biodivers. Sci. Ecosyst. Serv. Manage. 8, 107–121. doi: 10.1080/21513732.2011.647835
Guerry, A. D., Ruckelshaus, M. H., Plummer, M. L., and Holland, D. (2013). “Modeling marine ecosystem services,” in Encyclopedia of Biodiversity, ed A. L. Simon (Waltham, MA: Academic Press), 329–346. doi: 10.1016/B978-0-12-384719-5.00333-6
Gutt, J., Bertler, N., Bracegirdle, T. J., Buschmann, A., Comiso, J., Hosie, G., et al. (2015). The Southern Ocean ecosystem under multiple climate change stresses - an integrated circumpolar assessment. Glob. Change Biol. 21, 1434–1453. doi: 10.1111/gcb.12794
Haines-Young, R., and Potschin, M. (2013). Common International Classification of Ecosystem Services (CICES): Consultation on Version 4. EEA Framework Contract No EEA/IEA/09/003.
Hassan, R. M., Scholes, R., and Ash, N. (eds.). (2005). Ecosystems and Human Well-being: Current State and Trends: Findings of the Condition and Trends Working Group. Washington, DC: Island Press.
Hauck, J., Schweppe-Kraft, B., Albert, C., Görg, C., Jax, K., Jensen, R., et al. (2013). The promise of the ecosystem services concept for planning and decision-making. GAIA Ecol. Perspect. Sci. Soc. 22, 232–236. doi: 10.14512/gaia.22.4.6
Hemmings, A. D. (2012). Considerable values in Antarctica. Polar J. 2, 139–156. doi: 10.1080/2154896X.2012.679565
Hidalgo, M., Secor, D. H., and Browman, H. I. (2016). Observing and managing seascapes: linking synoptic oceanography, ecological processes, and geospatial modelling. ICES J. Mar. Sci. J. 73, 1825–1830. doi: 10.1093/icesjms/fsw079
Hoaglin, D. C. (2003). John W. Tukey and data analysis. Stat. Sci. 18, 311–318. doi: 10.1214/ss/1076102418
Hou, Y., Burkhard, B., and Müller, F. (2013). Uncertainties in landscape analysis and ecosystem service assessment. J. Environ. Manage. 127(Suppl.), S117–S131. doi: 10.1016/j.jenvman.2012.12.002
Hughes, K. A., Constable, A., Frenot, Y., López-Martínez, J., McIvor, E., Njåstad, B., et al. (2018). Antarctic environmental protection: Strengthening the links between science and governance. Environ. Sci. Policy 83, 86–95. doi: 10.1016/j.envsci.2018.02.006
Hughes, K. A., and Grant, S. M. (2017). The spatial distribution of Antarctica's protected areas: a product of pragmatism, geopolitics or conservation need? Environ. Sci. Policy 72, 41–51. doi: 10.1016/j.envsci.2017.02.009
Hughes, K. A., López-Martínez, J., Francis, J. E., Crame, J. A., Carcavilla, L., Shiraishi, K., et al. (2016). Antarctic geoconservation: a review of current systems and practices. Environ. Conserv. 43, 97–108. doi: 10.1017/S0376892915000387
Jacobs, S., Burkhard, B., Van Daele, T., Staes, J., and Schneiders, A. (2015). ‘The Matrix Reloaded': a review of expert knowledge use for mapping ecosystem services. Ecol. Modell. 295, 21–30. doi: 10.1016/j.ecolmodel.2014.08.024
Jacobs, S., Dendoncker, N., and Keune, H. (2014). Ecosystem Services: Global Issues, Local Practices. San Diego, CA; Waltham, MA: Elsevier. doi: 10.1016/B978-0-12-419964-4.02002-3
Jacobs, S., Dendoncker, N., Martín-López, B., Barton, D. N., Gomez-Baggethun, E., Boeraeve, F., et al. (2016). A new valuation school: Integrating diverse values of nature in resource and land use decisions. Ecosyst. Serv. 22, 213–220. doi: 10.1016/j.ecoser.2016.11.007
Kaiser, G., Burkhard, B., Römer, H., Sangkaew, S., Graterol, R., Haitook, T., et al. (2013). Mapping tsunami impacts on land cover and related ecosystem service supply in Phang Nga, Thailand. Nat. Hazards Earth Syst. Sci. 13, 3095–3111. doi: 10.5194/nhess-13-3095-2013
Kandziora, M., Burkhard, B., and Müller, F. (2013a). Interactions of ecosystem properties, ecosystem integrity and ecosystem service indicators—A theoretical matrix exercise. Ecol. Indicat. 28, 54–78. doi: 10.1016/j.ecolind.2012.09.006
Kandziora, M., Burkhard, B., and Müller, F. (2013b). Mapping provisioning ecosystem services at the local scale using data of varying spatial and temporal resolution. Ecosyst. Serv. 4, 47–59. doi: 10.1016/j.ecoser.2013.04.001
Kaplowitz, M. D., and Hoehn, J. P. (2001). Do focus groups and individual interviews reveal the same information for natural resource valuation? Ecol. Econ. 36, 237–247. doi: 10.1016/S0921-8009(00)00226-3
Kavanaugh, M. T., Abdala, F. N., Ducklow, H., Glover, D., Fraser, W., Martinson, D., et al. (2015). Effect of continental shelf canyons on phytoplankton biomass and community composition along the western Antarctic Peninsula. Mar. Ecol. Prog. Ser. 524, 11–26. doi: 10.3354/meps11189
Kavanaugh, M. T., Oliver, M. J., Chavez, F. P., Letelier, R. M., Muller-Karger, F. E., and Doney, S. C. (2016). Seascapes as a new vernacular for pelagic ocean monitoring, management and conservation. ICES J. Mar. Sci. J. 73, 1839–1850. doi: 10.1093/icesjms/fsw086
Kennicutt, M. C. I., Chown, S. L., Cassano, J. J., Liggett, D., Peck, L. S., Massom, R., et al. (2015). A roadmap for Antarctic and Southern Ocean science for the next two decades and beyond. Antarctic Sci. 27, 3–18. doi: 10.1017/S0954102014000674
Kock, K.-H. (2007). Antarctic marine living resources - exploitation and its management in the Southern Ocean. Antarctic Sci. 19, 231–238. doi: 10.1017/S0954102007000302
Larsen, J. N., Anisimov, O. A., Constable, A., Hollowed, A. B., Maynard, N., Prestrud, P., et al. (2014). “Polar regions,” in Climate Change 2014: Impacts, Adaptation, and Vulnerability. Part B: Regional Aspects. Contribution of Working Group II to the Fifth Assessment Report of the Intergovernmental Panel of Climate Change, eds V. R. Barros, C. B. Field, D. J. Dokken, M. D. Mastrandrea, K. J. Mach, T. E. Bilir, M. Chatterjee, K. L. Ebi, Y. O. Estrada, R. C. Genova, B. Girma, E. S. Kissel, A. N. Levy, S. Maccracken, P. R. Mastrandrea, and L. L. White (Cambridge, UK; New York, NY: Cambridge University Press), 1567–1612.
Leaper, R., and Miller, C. (2011). Management of Antarctic baleen whales amid past exploitation, current threats and complex marine ecosystems. Antarctic Sci. 23, 503–529. doi: 10.1017/S0954102011000708
Liggett, D., McIntosh, A., Thompson, A., Gilbert, N., and Storey, B. (2011). From frozen continent to tourism hotspot? Five decades of Antarctic tourism development and management, and a glimpse into the future. Tour. Manage. 32, 357–366. doi: 10.1016/j.tourman.2010.03.005
Liquete, C., Piroddi, C., Drakou, E., Gurney, L., Katsanevakis, S., Charef, A., et al. (2013). Current status and future prospects for the assessment of marine and coastal ecosystem services: a systematic review. PLoS ONE 8:e67737. doi: 10.1371/journal.pone.0067737
Longo, C. S., Frazier, M., Doney, S. C., Rheuban, J. E., Humberstone, J. M., and Halpern, B. S. (2017). Using the ocean health index to identify opportunities and challenges to improving southern ocean ecosystem health. Front. Mar. Sci. 4:20. doi: 10.3389/fmars.2017.00020
Maes, J., Hauck, J., Paracchini, M. L., Ratamaki, O., Hutchins, M., Termansen, M., et al. (2013). Mainstreaming ecosystem services into EU policy. Curr. Opin. Environ. Sustain. 5, 128–134. doi: 10.1016/j.cosust.2013.01.002
Manderson, J. P. (2016). Seascapes are not landscapes: an analysis performed using Bernhard Riemann's rules: Table 1. ICES J. Mar. Sci. J. 73, 1831–1838. doi: 10.1093/icesjms/fsw069
McGee, J., and Liu, N. (2019). The challenges for antarctic governance in the early twenty-first century. Austral. J. Maritime Ocean Affairs 11, 73–77. doi: 10.1080/18366503.2019.1634940
Millennium Ecosystem Assessment (2003). Ecosystems and Human Well-Being: A Framework for Assessment. Washington, DC; Covelo, CA; London: Island Press.
Millennium Ecosystem Assessment (2005). Ecosystems & Human Well-Being: Synthesis. Washington, DC: Island Press.
Morgan, F., Barker, G., Briggs, C., Price, R., and Keys, H. (2007). Environmental Domains of Antarctica Version 2.0. Final Report. Manaaki Whenua Landcare Research New Zealand Ltd.
Nicholls, R. J., Marinova, N., Lowe, J. A., Brown, S., Vellinga, P., De Gusmao, D., et al. (2011). Sea-level rise and its possible impacts given a ‘beyond 4 degrees C world' in the twenty-first century. Philos. Trans. A Math. Phys. Eng. Sci. 369, 161–181. doi: 10.1098/rsta.2010.0291
Nyman, E. (2018). Protecting the poles: Marine living resource conservation approaches in the Arctic and Antarctic. Ocean Coast. Manage. 151, 193–200. doi: 10.1016/j.ocecoaman.2016.11.006
Pal LTER (2016). Basic Western Antarctic Peninsula Survey Grid. Pal LTER. Available online at: https://oceaninformatics.ucsd.edu/datazoo/catalogs/pallter/datasets/120
Pandeya, B., Buytaert, W., Zulkafli, Z., Karpouzoglou, T., Mao, F., and Hannah, D. M. (2016). A comparative analysis of ecosystem services valuation approaches for application at the local scale and in data scarce regions. Ecosyst. Serv. 22, 250–259. doi: 10.1016/j.ecoser.2016.10.015
Posner, S., Getz, C., and Ricketts, T. (2016). Evaluating the impact of ecosystem service assessments on decision-makers. Environ. Sci. Policy 64, 30–37. doi: 10.1016/j.envsci.2016.06.003
Posner, S. M., McKenzie, E., and Ricketts, T. H. (2016). Policy impacts of ecosystem services knowledge. Proc. Natl. Acad. Sci. U.S.A. 113, 1760–1765. doi: 10.1073/pnas.1502452113
Potschin, M., Haines-Young, R., Fish, R., and Turner, R. K. (eds.). (2016). Routledge Handbook of Ecosystem Services. New York, NY: Routledge. doi: 10.4324/9781315775302
Purkey, S. G., and Johnson, G. C. (2013). Antarctic bottom water warming and freshening: contributions to sea level rise, ocean freshwater budgets, and global heat gain. J. Climate 26, 6105–6122. doi: 10.1175/JCLI-D-12-00834.1
Ross, R. M., Quetin, L. B., Martinson, D. G., Iannuzzi, R. A., Stammerjohn, S. E., and Smith, R. C. (2008). Palmer LTER: Patterns of distribution of five dominant zooplankton species in the epipelagic zone west of the Antarctic Peninsula, 1993–2004. Deep Sea Res. II Top. Stud. Oceanogr. 55, 2086–2105. doi: 10.1016/j.dsr2.2008.04.037
Roura, R. M., Steenhuisen, F., and Bastmeijer, K. (2018). The shore is the limit: marine spatial protection in Antarctica under Annex V of the Environmental Protocol to the Antarctic Treaty. Polar J. 8, 289–314. doi: 10.1080/2154896X.2018.1541549
Sayre, R., Dangermond, J., Wright, D., Breyer, S., Bulter, K., Graafeiland, K. V., et al. (2017). A New Map of Global Ecological Marine Units-an Environmental Stratification Approach. Washington, DC: American Association of Geographers.
Schleyer, C., Gorg, C., Hauck, J., and Winkler, K. J. (2015). Opportunities and challenges for mainstreaming the ecosystem services concept in the multi-level policy-making within the EU. Ecosyst. Serv. 16, 174–181. doi: 10.1016/j.ecoser.2015.10.014
Secretariat of the Antarctic Treaty (2019). The Antarctic Treaty. Available online at: https://www.ats.aq/e/antarctictreaty.html (accessed October 09, 2019).
Singh, G. G., Sinner, J., Ellis, J., Kandlikar, M., Halpern, B. S., Satterfield, T., et al. (2017). Group elicitations yield more consistent, yet more uncertain experts in understanding risks to ecosystem services in New Zealand bays. PLoS ONE 12:e0182233. doi: 10.1371/journal.pone.0190326
Sohel, M. S. I., Ahmed Mukul, S., and Burkhard, B. (2015). Landscape?s capacities to supply ecosystem services in Bangladesh: a mapping assessment for Lawachara National Park. Ecosyst. Serv. 12, 128–135. doi: 10.1016/j.ecoser.2014.11.015
Stammerjohn, S. E., Martinson, D. G., Smith, R. C., and Iannuzzi, R. A. (2008). Sea ice in the western Antarctic Peninsula region: Spatio-temporal variability from ecological and climate change perspectives. Deep Sea Res. II Top. Stud. Oceanogr. 55, 2041–2058. doi: 10.1016/j.dsr2.2008.04.026
Stocker, T. F. (2015). The silent services of the world ocean. Science 350, 764–765. doi: 10.1126/science.aac8720
Stoll, S., Frenzel, M., Burkhard, B., Adamescu, M., Augustaitis, A., Baeßler, C., et al. (2015). Assessment of ecosystem integrity and service gradients across Europe using the LTER Europe network. Ecol. Modell. 295, 75–87. doi: 10.1016/j.ecolmodel.2014.06.019
Summerson, R. (2011). “Wilderness and aesthetic values of Antarctica,” in Exploring Antarctic values. Proceedings of the Workshop: Exploring Linkages Between Environmental Management and Value Systems: the Case of Antarctica, held at the University of Canterbury, Christchurch, New Zealand eds D. Liggett and A. D. Hemmings (Christchurch: Gateway Antarctica, Centre for Antarctic Studies and Research, University of Canterbury), 22–47.
Summerson, R., and Bishop, I. D. (2011). Aesthetic value in Antarctica: beautiful or sublime? Polar J. 1, 225–250. doi: 10.1080/2154896X.2011.626626
Townsend, M., Thrush, S. F., Lohrer, A. M., Hewitt, J. E., Lundquist, C. J., Carbines, M., et al. (2014). Overcoming the challenges of data scarcity in mapping marine ecosystem service potential. Ecosyst. Serv. 8, 44–55. doi: 10.1016/j.ecoser.2014.02.002
Turner, J., Bindschadler, R., Convey, P., Di Prisco, G., Fahrbach, E., Gutt, J., et al. (2009). Antarctic Climate Change and the Environment. Cambridge: Scientific Committee on Antarctic Research.
Turner, J., and Overland, J. (2009). Contrasting climate change in the two polar regions. Polar Res. 28, 146–164. doi: 10.1111/j.1751-8369.2009.00128.x
United Nations (2015). Global Sustainable Development Report. 2015 Edition. Advance Unedited Version. New York, NY: United Nations.
Vila, M., Costa, G., Angulo-Preckler, C., Sarda, R., and Avila, C. (2016). Contrasting views on Antarctic tourism: ‘last chance tourism' or ‘ambassadorship' in the last of the wild. J. Cleaner Product. 111, 451–460. doi: 10.1016/j.jclepro.2014.12.061
Visbeck, M., Kronfeld-Goharani, U., Neumann, B., Rickels, W., Schmidt, J., Van Doorn, E., et al. (2014). Securing blue wealth: The need for a special sustainable development goal for the ocean and coasts. Mar. Policy 48, 184–191. doi: 10.1016/j.marpol.2014.03.005
Waller, C. L., Griffiths, H. J., Waluda, C. M., Thorpe, S. E., Loaiza, I., Moreno, B., et al. (2017). Microplastics in the Antarctic marine system: an emerging area of research. Sci. Tot. Environ. 598, 220–227. doi: 10.1016/j.scitotenv.2017.03.283
Walton, D. W. H. (2018). Tourism in the Antarctic. Antarctic Sci. 30, 147–147. doi: 10.1017/S0954102018000160
Waters, K. J., and Smith, R. C. (1992). Palmer LTER: a sampling grid for the Palmer LTER program. Antarctic J. U. S. 27, 236–239.
Watts, A. (1992). International Law and the Antarctic Treaty System. Cambridge: Grotius Publications.
Keywords: ecosystem services, service providing units, seascapes, matrix method, expert-based assessments, Antarctic Peninsula
Citation: Neumann B, Mikoleit A, Bowman JS, Ducklow HW and Müller F (2019) Ecosystem Service Supply in the Antarctic Peninsula Region: Evaluating an Expert-Based Assessment Approach and a Novel Seascape Data Model. Front. Environ. Sci. 7:157. doi: 10.3389/fenvs.2019.00157
Received: 13 January 2019; Accepted: 30 September 2019;
Published: 23 October 2019.
Edited by:
Juan Jose Alava, University of British Columbia, CanadaReviewed by:
Julian Gutt, Alfred Wegener Institute Helmholtz Centre for Polar and Marine Research (AWI), GermanyMercy J. Borbor-Cordova, Escuela Superior Politecnica del Litoral (ESPOL), Ecuador
Copyright © 2019 Neumann, Mikoleit, Bowman, Ducklow and Müller. This is an open-access article distributed under the terms of the Creative Commons Attribution License (CC BY). The use, distribution or reproduction in other forums is permitted, provided the original author(s) and the copyright owner(s) are credited and that the original publication in this journal is cited, in accordance with accepted academic practice. No use, distribution or reproduction is permitted which does not comply with these terms.
*Correspondence: Barbara Neumann, barbara.neumann@iass-potsdam.de