- Department of Microbiology and Plant Pathology, University of California, Riverside, Riverside, CA, United States
Agriculture production in California is negatively impacted by soilborne fungi, such as Verticillium dahliae, and limited water availability for irrigation. Some regions have adapted the use of recycling wastewater, i.e., reclaimed water, to supplement the potable water supply. Wastewater purification is not fully efficient at removing all contaminants and small amounts of pharmaceutical products, known as chemicals of emerging concern (CECs), remain. Acetaminophen, trimethoprim, sulfamethoxazole and gemfibrozil are some of the most common CECs found in treated wastewater and were therefore used in this study. These CECs were evaluated for their potential to interact with microorganisms directly, or for their ability to alter the development of Verticillium wilt disease in eggplants. The microorganisms Verticillium dahliae, Fusarium oxysporum f. sp. lycopersici, Piriformospora indica, Phytopthora capsici, and Bradyrhizobium japonicum were used for in vitro growth assays in the presence of CECs. CECs induced varying responses in strains of the same fungi by promoting growth of one strain while inhibiting growth of the other. CECs influenced spore germination of V. dahliae and F. oxysporum. Greenhouse experiments in which Solanum melongena (eggplants) were inoculated with V. dahliae and irrigated with CECs were used to evaluate the impacts of these chemicals on disease development. Overall our results found that most of the organisms we tested were sensitive to the CECs. P. capsici was found to be the most sensitive microorganism, while B. japonicum growth was unaffected by the CECs at the concentrations used. The greenhouse assays results indicated that plant disease severity may be influenced by given CECs at certain stages of plant growth. Overall the results of this study indicate that the concentrations of CECs found in reclaimed water are occurring at biologically relevant concentrations.
Introduction
Extended periods of drought and the presence of soilborne plant pathogens jeopardize California's agricultural production, which contributes a significant portion to the United States food supply. Recent drought conditions and continued population growth in Southern California have place great demands on the region's limited potable water supply (AghaKouchak et al., 2015; Howitt et al., 2015). This valuable resource is consumed by the population directly or indirectly through irrigation of crops and landscape vegetation. Since potable water is in such high demand, California has taken steps to conserve this limited resource. Recycling wastewater has been successfully employed to protect the supply of potable water to satiate the growing demands for clean water (Parsons et al., 2010; Schulte, 2011; Cooley and Phurisamban, 2016; Warsinger et al., 2018). Normally wastewater is treated to a limited capacity and allowed to exit the potable water supply and re-enter the natural water cycle. Alternatively, wastewater can be treated to a far greater extent, and used to supplement potable water supplies as reclaimed water (Figure S1; Warsinger et al., 2018). Reclaimed water can be used to recharge potable water supplies directly by injecting it into ground water reservoirs, or allowing it to mix with potable water aquifers (Warsinger et al., 2018). Reclaimed water can also be used to irrigate crops or landscape vegetation, thereby preserving potable water supplies for direct human consumption (Warsinger et al., 2018).
Chemicals of emerging concern (CECs) such as pharmaceuticals, personal care products, detergents, nanoparticles, etc. continue to be introduced in the environment, and have potential to impact human and aquatic life that would otherwise not be exposed to them (Kinney et al., 2006; Gros et al., 2010; EPA, 2019) Unfortunately, even after extensive treatment, CECs remain in the treated water (Kinney et al., 2006; Gros et al., 2010). The past consensus with these chemicals is that they do not pose much danger for human and environmental health since they occur in such low concentrations, and in the case of pharmaceuticals, well below therapeutic doses used for humans (Boxall et al., 2006; Wu et al., 2013; Zimmermann and Curtis, 2017). However, this notion has been eroded by the observations that sub-therapeutic concentrations of certain pharmaceuticals can impact microbial, plant, and insect life (Wang and Gunsch, 2011; Pennington et al., 2017, 2018). Additionally, some CECs have been observed to accumulate in soils upon repeated irrigation with reclaimed water containing the compounds (Wu et al., 2015). Therefore, the concentrations of CECs found in reclaimed water can be at biologically relevant concentrations or can be raised to that level with repeated irrigation or in the presence of chemicals that can increase their potency.
Given that CECs accumulate in plants (Wu et al., 2015), they may impact plant physiology, potentially altering a plants ability to interact with microorganisms (pathogenic or beneficial). Exacerbation of already prevalent soilborne fungi (or plant pathogens), and consequently plant diseases, found in agricultural soils is one potential outcome of chemical interference from the CECs that can impact plants, and/or the microbial community that is associated with plants. CECs may interact with soil microbes directly by stimulating or inhibiting their growth, thus impacting their ability to colonize or infect a host. CECs may alter microbial population equilibrium by promoting some, while inhibiting others and can thereby increase pathogen populations by reducing their competitors or antagonist in the soil (Mulligan et al., 1982; De Vries-Hospers et al., 1991; Azevedo et al., 2015).
Verticillium dahliae, the causative agent of Verticillium wilt, is a soilborne pathogen that may benefit from the anthropogenic inputs of CECs from reclaimed water. V. dahliae has a wide host range for many crops that are important to California's agriculture industry including bell peppers, eggplants, strawberries, tomatoes, and watermelon (Pegg, 1984; Aguiar et al., 1998; Bhat and Subbarao, 1999; Klosterman et al., 2009). California leads the nation in the production of the above mentioned crops (California Department of Food Agriculture, 2018) and major reductions in their yields could have devastating consequences to food supply and income. Plant diseases already reduce California's agriculture output and increase cost of production, and they may be exacerbated by anthropogenic input of CECs into agriculture soils.
Acetaminophen (APAP), trimethoprim (TMP), sulfamethoxazole (SMX), and gemfibrozil (GEM) are synthetic pharmaceutical products that are commonly found in recycled wastewater. APAP is an antipyretic pain killer that has been observed to have antimicrobial properties at concentrations above therapeutic use (Zimmermann and Curtis, 2017), and observed to have growth promoting effects on microorganism at therapeutic concentrations (Carvalho et al., 2010). TMP and SMX are antibiotics that are commonly taken in combination, but exhibit antimicrobial effects independently (Reeves and Wilkinson, 1979; Hida et al., 2005; Tunali et al., 2012). GEM is a medication used to treat high blood pressure, but has been observed to increase the antimicrobial potency of some pharmaceuticals (Rudin et al., 1992; Bulatova and Darwish, 2008) and to have direct impacts on plant growth (D'Abrosca et al., 2008; Pino et al., 2016). These four CECs are found consistently in wastewater treatment plant effluent such as reclaimed water, and in soils irrigated with recycled water in the ng/L and μg/L range (Kinney et al., 2006; Batt et al., 2007; Erickson et al., 2014; Wu et al., 2015).
Extended periods of drought conditions are common in arid regions such as Southern California, which makes water conservation efforts such as reclaimed water use a necessity (Brown et al., 2013). Despite the presence of chemicals remaining in the treated wastewater, reclaimed water has done much to alleviate water demands and California plans to increase its use (California State Water Resources Control Board, 2010). Therefore, it is critical for us to understand the direct impacts CECs have on plants and their associated soil microbiome to prevent elevation of plant losses from microorganisms. In our study we subject plants and microorganisms to concentrations similar to those found in treated wastewater effluent, or reclaimed water. A number of previous studies have found direct phytotoxic effects of various CECs, however these studies used concentrations that are higher than those found in typically treated wastewater effluent (D'Abrosca et al., 2008; Liu et al., 2009a; Pino et al., 2016; Madikizela et al., 2018). Some studies found phytotoxic effects to developing plants, but did not grow the plants in soil (D'Abrosca et al., 2008; Pino et al., 2016), which can impact plants uptake of chemicals through the roots (Pan and Chu, 2017). Use of higher concentrations of CECs and not growing the plants in soil means that the plants are exposed to unusually high concentrations of the compounds. This under states the potential severity of microbial community disturbances and phototoxic effects of in situ CEC concentrations found in recycled water. Therefore, the direct impacts of CECs on eggplants grown in soil were tested using concentrations within the range of CECs detected in recycled water or wastewater treatment plant effluent. The impacts of CECs on the growth of microorganisms—fungi, oomycetes, and bacteria that are known to be beneficial or deleterious to plants were also tested using CECs concentrations that are relative to in situ concentrations. We hypothesized that the CECs will not impact microbial growth, since we are using relatively low concentrations of CECs that are well below therapeutic doses. We also hypothesized that CECs would not have any impact on disease development in eggplants inoculated with the plant pathogen Verticillium dahliae. Therefore, objective of this study was to determine the impact of CECs on microbial growth and disease development in eggplants.
Materials and Methods
In total 4 CEC's -acetaminophen (APAP), trimethoprim (TMP), sulfamethoxazole (SMX), and gemfibrozil (GEM)—were evaluated to determine their impacts on the microbial growth and development of Verticillium wilt in eggplants. Each CEC was tested at a high concentration (H) and a low concentration (L) as specified in Table 1 below. The concentrations used mimic the range of concentrations of the respective CECs that have been found in the final effluent of wastewater treatment plants or in soils irrigated with reclaimed water (Vanderford and Snyder, 2006; Batt et al., 2007; Stackelberg et al., 2007; Dia-Cruz and Barcelo, 2008; Fram and Belitz, 2011). These compounds at the concentrations listed in Table 1 were used in all assays described below.
Media Preparation
Czapek-dox, buffered 10% V-8, potato dextrose agar (PDA), and Luria Bertani (LB) broth were used to cultivate the different microorganisms. Czapek-Dox agar was prepared as the “originally proposed” version described in Thom (1930) and Smith (1941). Briefly, 30.0 g of sucrose, 3.0 g of sodium nitrate, 1.0 g of dipotassium phosphate, 0.5 g of magnesium sulfate, 0.5 g of potassium chloride, and 0.01 g of ferrous sulfate per 1 L of sterile ddH2O were used. The buffered 10% V-8 agar was made by adding 10% volume of V8 juice and 0.2 % (W/V) of calcium carbonate to sterile ddH2O. The V8 calcium carbonate solution was clarified by centrifuging it at 3,000 × g for 10 min, and the supernatant was added to the required amount of ddH2O to make a final concentration of 10% V8. To make solid media for petri dishes, 10% agar (W/V) was added. LB broth and PDA were prepared based on the manufacturer's instructions. PDA amended with 0.833 μM of bromocresol purple sodium salt (BCP) for a growth assay described below (Masachis et al., 2016).
Cultivation of Organisms
The impacts of CECs on the growth of several microorganisms were tested. Organisms covering a wide range of classifications and common plant pathogens in regions where reclaimed water is used were also included in the study. Two Verticillium dahliae strains (0048 and 0049), two Fusarium oxysporum lycopersici strains (CS-3 and CS-5), Phytophthora capsici, Piriformospora indica, and Bradyrhizobium japonicum IRAT FA3 were tested. Two strains of Verticillium and Fusarium were used to assess the impacts of CECs on closely related organisms. V. dahliae strains were cultured on Czapek-Dox plates with 1% agar, F. oxysporum and P. indica were cultured on PDA and B. japonicum was cultured in LB broth. During growth rate assays, each organism was grown on their respective culturing media. The media was amended with the required volume of a given CEC working stock solution to reach the concentrations indicated in Table 1, after the base media was autoclaved and cooled, but before solidifying. An additional treatment containing no CECs was used as a control for each organism. Two strains of V. dahliae and F. oxysporum were tested to find out the response of closely related organism to the same CECs.
Verification of Organism Identity
DNA from V. dahliae and F. oxysporum f. sp. lycopersici strains were extracted using the DNeasy Plant Mini Kit (Qiagen) following the manufacture's protocol. Extracted DNA was used in PCR assays to confirm the identity of the fungal isolates used in this study. V. dahliae isolates were confirmed following the procedure and commonly used primers described in Inderbitzin et al. (2013). Briefly, each PCR reactions consisted of 12.5 μL of 2 × Dream taq green master mix (Thermo Scientific), 1 μL of forward and reverse primer, 1 μL of extracted DNA (i.e., DNA template), and 9.5 μL of nuclease free water. All primers used in this assay were purchased from Integrated DNA Technologies. PCR reactions were carried out in a thermocycler with an initial denaturation step for 2 min (min) at 94°C. This was followed by 35 cycles of denaturation 94°C for 10 s (s), Annealing for 20 s, and elongation for 1 min at 72°C. Then, a final elongation step for 7 min at 72°C was used (Figure S2). Identification of F. oxysporum f. sp. lycopersici was confirmed in a similar fashion using a PCR approach described in Hirano and Arie (2006). The same PCR reaction mixture described above for identifying V. dahliae. To conduct the PCR, the mixtures went through 50 cycles of denaturation at 94°C for 1 min, Annealing at 62°C for 1 min, and elongation at 72 °C for 2 min. The F. oxysporum sample was screen using the Unif/r, Sp13f/r, Sp23f/r, and the sprlf/r primer sets described in Hirano and Arie (2006) to positively identify the F. oxysporum strains used in this study (Figure S2).
Growth Rate Assays
Every fungal and oomycete growth assay had 5 replicates for each treatment, while bacterial growth assays had 3 replicates. For fungal organisms, a 5 mm diameter plug taken from the edge of an actively growing colony was placed in the center of the agar plate. Growth of the organism was monitored by measuring the diameter across the colony. Two diameter measurements were taken from two different angles and averaged together per replicate V. dahliae strains were measured 3, 7, 14, and 21 days post inoculation (dpi). F. oxysporum strains were measured 3, 4, 5, 6, and 7 dpi, P. indica was measured 3, 7, 11, 12, 14 dpi, and P. capsici was measured 1, 2, 3, and 4 dpi (Figures 1A–C). A growth assay carried out on PDA plates amended with the pH indicator bromocresol purple was used to detect if nutrients were consumed beyond the growing mycelium tips in the solid media (Figure 1A).
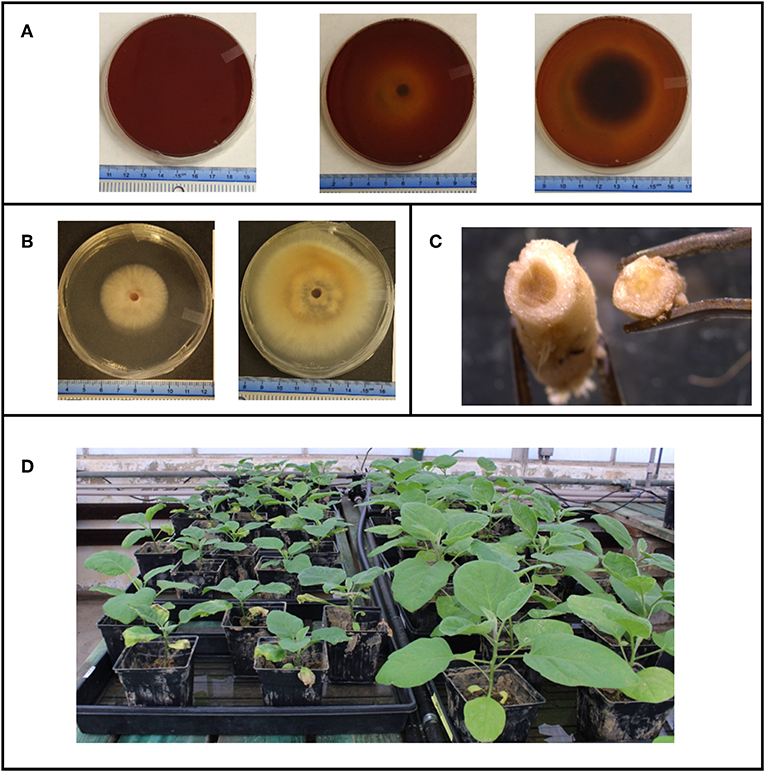
Figure 1. (A) Fusarium oxysporium grown on media containing the pH indicator bromocresol purple to indicate how far beyond the visible mycelial tips, nutrients are being uptaken by the mycelia. (B) Typical growth of Verticillium dahliae on Czepeck dox agar. (C) Stem cross section of eggplants inoculated with V. dahliae (left) and an uninoculated (right). (D) Overall set up of eggplants in the green house with a side by side comparison of inoculated eggplants (left) to uninoculated eggplants (right).
A spectrophotometric assay was done to measure the growth rate of B. japonicum in LB broth. A culture of B. japonicum in exponential phase was used for the assay. The starting concentration was adjusted to OD600 0.05. Using a spectrophotometer, readings (OD600) were taken at 0, 4, 8, 12, 24, 36, 48, 60, and 72 h post inoculation (hpi). An additional specific plate count assay was done at the 18 h mark to verify spectrophotometer results. This was done by plating 10−6, 10−7, and 10−8 serial dilutions of the growing culture on to LB agar for each treatment. Colonies formed on the plate were counted as CFUs. All organisms were grown at their optimal temperatures for cultivation and for the growth rate assays. Growth on solid media was used to calculate the allelopathic index (RI) (i.e., relative index) described (Liu et al., 2009a).
Spore germination assays were conducted on V. dahliae 0049 and F. oxysporum CS-5 similarly as described by Williams et al. (2002) with a few exceptions. Organisms were grown on solid media until they covered the entire surface of a 15 mm × 100 mm petri dish. Spores were harvested from the plate using 0.17 M sterile sucrose solution. Spore suspensions containing CECs listed in Table 1 were adjusted to 1.80 × 105 and 1.92 × 106 spores/ mL using a hemocytometer for V. dahliae 0049 and F. oxysporum CS-5 assays respectively. All final solutions contained 0.085 M of sucrose to make them have an osmotic potential of −2 bars or lower to ensure optimal spore germination as described in Ioannou et al. (1977). The spore solutions were allowed to germinate for 12 h. At this point, 0.2% Aniline blue in lactophenol blue (final concentration) was applied to solutions to prevent further germination (Williams et al., 2002). The number of spores germinated was enumerated using a compound microscope, each treatment had 3 replicates (n = 3).
Cultivation of Eggplants
The Patio Baby variety of eggplants from Johnny's seeds (Fairfiled, Maine, USA) was used for all eggplants in this study. A group of five eggplants (n = 5) were treated with a given CEC at a concentration specified in Table 1. An equivalent group of eggplants was treated in a similar fashion, except they were inoculated with Verticillium dahliae strain 0049 during transplanting (discussed below). The eggplant assay was carried out in two separate sets. Set one (set I) consisted of the acetaminophen, trimethoprim, and associated no CEC control treatments. The sulfamethoxazole, gemfibrozil, and associated no CEC control treatments were carried out on the second set of eggplants (set II).
Eggplant seeds were germinated in a growth room at 22°C. All plants were allowed to reach the 2–4 leaf stage before transplanting them into eggplant field soil obtained from farm lands in Bakersfield, California. This field is not irrigated with reclaimed water nor treated with fungicide because it is only used for organic farming. Eggplants were grown in this field, thus this soil was ideal for mimicking in situ agricultural soil conditions. During transplanting, the inoculated sets of eggplants were exposed to V. dahliae 0049 using the dipping method described by Bhat and Subbarao (1999). Plants were inoculated with 1.85 × 107 spores/ L. Tap water was used for the uninoculated controls. All seeds and plants were watered as needed using tap water for up to 1 week after transplanting to allow them to acclimatize. CEC treatments were applied to plants a week after transplanting. CEC were dissolved into the tap water to reach the final concentration listed in Table 1. The no CEC treatments were watered with tap water only. All treatments received 1L of the solution that contained the respective concentration of CEC per watering event. Watering was done 2–3 times a week as needed. All the plants for a given treatment (n = 5) were kept in the same tray. CEC solutions for the respective treatments were poured into the trays to ensure that all plants were receiving equal amounts of the solution (Figure 1D). This experiment was repeated 2 times.
Plant Measurements and Soil Collection
Stem height measurements of plants and disease assessments were taken weekly starting the week of transplantation. Disease assessments relied on observations of external symptoms to avoid destructive sampling. Disease severity assessments were based on a 0–5 scale (Liu et al., 2009b). Briefly: 0 represented no wilted leaves; 1 ≤ 25% wilted leaves; 2 = 25–50% wilted leaves; 3 = 50–75% wilted leaves; 4 = 75–100% wilted leaves; and 5 = dead plant. Upon the final sampling, eggplants were cut at the base of the stem and the fresh weight of shoots was determined for each plant. Disease index was calculated using the disease severity assessment values as described in Liu et al. (2009b).
Statistical Analyses
All parametric ANOVA and GLiM statistical analyses were done using SPSS software (ver. 24.0; SPSS; IBM, Somers, New York, USA). For the green house experiments repeated measures ANOVA was done to test for interactions between time and treatments. Repeated measures Friedman Ranks ANOVA were done using R software v. 3.5.3 (R Core Team, 2018) package npIntFactRep (Feys, 2015, 2016) when ANOVA assumptions could not be met. In both approaches, the Greenhouse-Geisser correction was applied when sphericity was not achieved. Post-hoc ANOVA were used when significant interactions with time were detected and the data satisfied all ANOVA assumptions. In cases were the assumptions could not be met, the best fitting generalized linear model (GLiM) was used as determined by the model with the lowest Akaike's information criterion (AIC). In all cases this model was found to be the normal model with identity link function. Tukey was used for all post-hoc pairwise analyses when ANOVA was valid. In the cases were GLiM analyses were done, the post-hoc, pairwise analyses were carried out using the GLiM model with sequential Bonferroni correction for multiple comparisons. All post-hoc pairwise comparisons considered to be significantly different when P < 0.05. The two eggplant sets were treated as two separate experiments, in which statistical comparisons were only made within the respective sets. Comparisons to the no CEC controls or uninoculated plants were used to assess the impacts of CECs, disease, or the combination of both. The non-repeated measures ANOVA and GLiM procedures were also used to evaluate treatment effects in microbial growth assays.
Results
Identification of Microorganisms
The PCR assay generated results that positively identified the V. dahliae and F. oxysporum strains. In both cases, positive amplification of their template DNA occurred with primer pairs specific to their respective identities (Figure S2). Specifically, we saw positive amplification for V. dahliae with the Df/Dr primer pair only, and positive amplification with the Unif/Unir and Sp13f/Sp13r primer sets for F. oxysporum f. sp. lycopersici (Hirano and Arie, 2006; Inderbitzin et al., 2013).
Impact of CECS on Growth of Microorganisms
Overall, colony diameter measurements of organisms grown on solid media demonstrated that the fungi tested were sensitive to most of the CECs used in this study (Figures S3A, S4). This is greatly exemplified with the allelopathic index (RI) calculations that are based on colony growth. The RI calculations indicate that some of these chemicals promoted growth, hindered growth or had no effect on a given organism (Figure 2 and Figure S3B). Positive RI values indicated that an organism's growth was promoted by the CECs, while a negative showed that its growth was inhibited. At 7 days post inoculation (dpi) V. dahliae strain 0049 grown with low concentrations of GEM (GEM-L) had a negative RI value that was significantly lower than the control (Figure 3B) (GLiM: = 44.754, P < 0.001). By 14 dpi the inhibitory effects of CECs on V. dahliae 0049 growth were more apparent. Five treatments had significantly lower RI values than the control (Figure 3B) (GLiM: = 247.137, P < 0.001). GEM-L and high concentrations of SMX (SMX-H) treatments had the lowest RI values for 0049, which were significantly lower than all other treatments except for the high concentration of TMP (TMP-H) (GLiM: = 247.137, P < 0.001). Colony diameter growth of the other V. dahliae strain 0048 (Figure S4A), did not follow the same trends. CECs either benefited or had no effect on V. dahliae 0048 mycelial growth. By 7 dpi three treatments had significantly higher RI values than the control (TMP-L, SMX-H, and APAP-H) (GLiM: = 83.749, P < 0.001). By 14 dpi all treatments for V. dahliae 0048 had significantly higher RI values than the control (GLiM: = 76.092, P < 0.001) (Figure 2A).
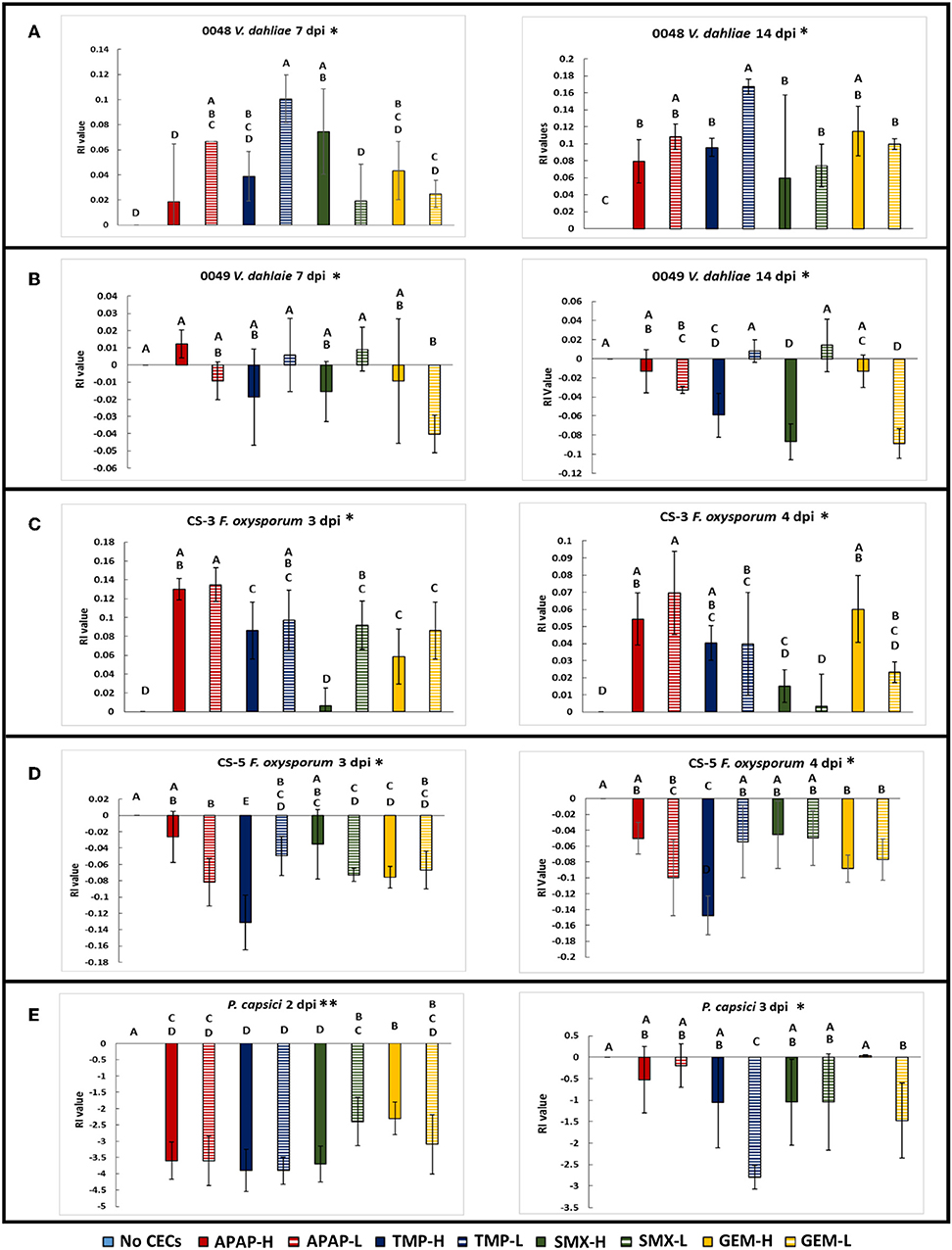
Figure 2. Comparing the Allelopathic index values (RI) of the plant pathogens used in this study. (A) Verticillium dahliae strain 0048 measured at 7 and 14 dpi, (B) Verticillium dahliae strain 0049 measured at 7 and 14 dpi. (C) Fusarium oxysporum f.sp lycopersici strain CS-3 measured at 3 and 4 dpi. (D) Fusarium oxysporum f.sp lycopersici strain CS-5 measured at 3 and 4 dpi. (E) Phytopthora capsici measured at 2 and 3 dpi. *Samples compared statistically using GLiM with normal distribution and identity link function, pairwise analyses with sequential Bonferroni pairwise correction for multiple comparisons (P < 0.05). **ANOVA used for statistical analyses, post-hoc Tukey test used for pairwise analyses (P < 0.05).
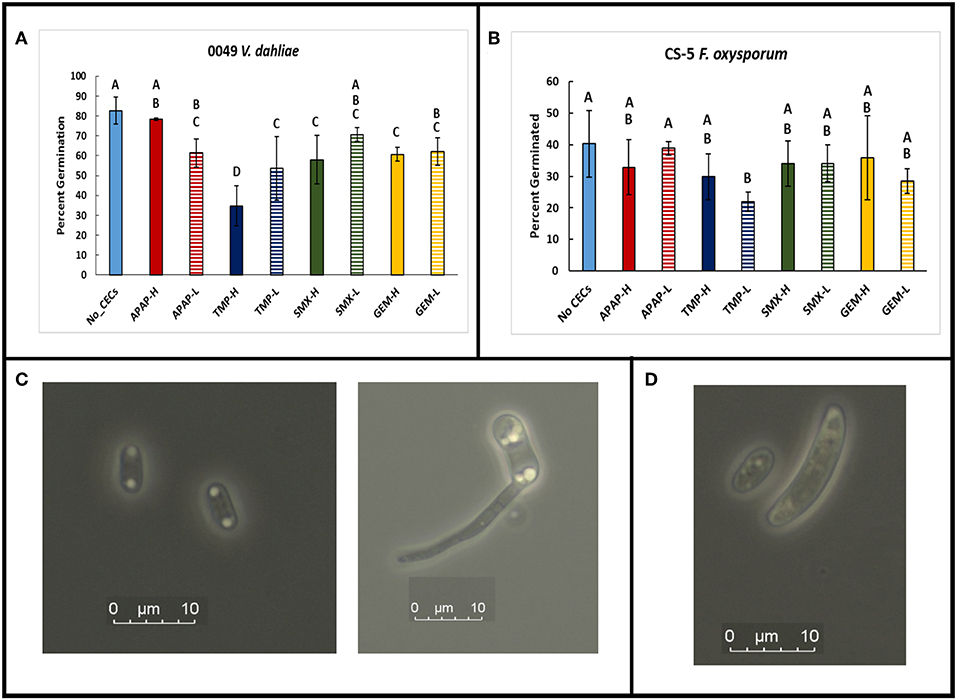
Figure 3. Spore germination assays done in a mild sucrose solution. GLiM with normal distribution and identity link function used for all statistical comparisons. Samples that do not share the same letter are significantly different as determined by a post-hoc, pairwise GLiM analyses done with sequential Bonferroni correction for multiple comparisons (all P's < 0.05). (A) Spore germination results for Verticillium dahliae after 12 h of incubation in a 0.085 M sucrose solution. (B) Spore germination results for Fusarium oxysporum f.sp lycopersici after 12 h of incubation in 0.085 M sucrose solution. (C) Photographs of Verticillium dahliae spores at 1,000× total magnification. Ungerminated spore (left), germinating spore (right) (D) Photograph of small and large Fusarium oxysporum f.sp lycopersici spores at 1,000× magnification.
The other plant pathogens examined, two F oxysporum strains, were also sensitive to the CECs tested. However, like V. dahliae, the CECs impacted the two F. oxysporum strains (CS-5 and CS-3) differently. The CECs appear to either have no impact, inhibit growth for one strain (CS-5), or enhance growth of the other strain (CS-3). By 3 dpi the RI values for all of the CEC treatments for F. oxysporum CS-5 were below the control, though, only 6 (APAP-L, TMP-H, TMP-L, SMX-L, GEM-H, and GEM–L) were significantly different (GLiM: = 105.467, P < 0.001) (Figure 3D). This trend continued into the following day at 4 dpi in which all treatments still had lower RI values than the control, though only 4 (APAP-L, TMP-H, GEM-H, and GEM-L) were significant (GLiM: = 82.84, P < 0.001). At this point the faster growing mycelia were approaching the edges of the agar plate. This most likely inhibited their growth, allowing slower growing mycelia to catch up and reduce differences found among treatments. Growth of F. oxysporum on PDA plates amended with BCP indicated that the fungal mycelia used up the nutrients in the media beyond the reach of the mycelia. This was shown by a color change in the media before the mycelia occupied it (Figure 1A). Treatment with high concentrations of TMP appeared to have the most dramatic impact on CS-5. At both time points TMP-H had the lowest RI value and at 4 dpi its RI value was significantly lower than all treatments (Figure 2D) (GLiM = 82.84, P < 0.001) except for APAP-L. Despite growth inhibiting effects seen for strain CS-5, growth promoting effects of CECs were observed on CS-3 (Figure 2C and Figure S4B). For instance, by 3 dpi the no CEC control had the smallest colony diameter (Figure S4B). The diameter was significantly smaller than all but two treatments, SMX-H and GEM-H (GLiM: = 66.502, P < 0.01) (Figure S4B). By the next day at 4 dpi growth approached the edges of the plate and no significant differences in colony sizes were observed among any of the treatments. Growth of the plant pathogen P. capsici was inhibited by all of the CECs used in this study. At 2 dpi all CEC treatments had smaller diameter than the control, while only two of these treatments, SMX-L and GEM-H, were not significantly lower (Figure S4E) (ANOVA: F8 = 7.496, P < 0.001). The RI values for each treatment were negative and significantly lower than the control (Figure 2E) (ANOVA: F8 = 20.796, P < 0.001). The RI values were also much smaller than any of the other organisms tested, indicating that this strain is particularly sensitive. By 3 dpi only TMP-L treatment had a significantly smaller diameter than the control (Figure S4E) (GLiM: = 35.136, P < 0.001). TMP-L and GEM-L treatments had significantly lower RI values than the control at this time point as well (Figure 2E) (GLiM: = 69.872, P < 0.001). Again, this is most likely due to declining growth rates as the colonies approach the edge of the plate allowing the slower growing organisms time to catch up in size.
Testing the impacts of CECs on the growth of the plant symbiont, Piriformospora indica, yielded mixed results. In this case there were CECs that either had no impact, inhibited, or enhanced the growth of this organism (Figures S3A,B). At 8 dpi, for example, only one treatment had significantly less growth than the no CEC control, SMX-L (GLiM: = 52.329, P < 0.001), while the rest of the treatments were not significantly different from the control. At 10 dpi only the TMP-H treatment was significantly larger than the no CEC control (GLiM: = 48.169 P < 0.001). Furthermore, the RI values calculated using colony diameter reinforce these trends (Figure S3B). At 8 dpi both the low and high concentrations of SMX had negative RI values that were below the no CEC control, but only the low concentration was significantly different (GLIM: = 58.997, P < 0.001). At 10 dpi the TMP-H treatment had a significantly higher RI value than the no CEC control, while none of the other treatments are significantly different (GLiM: = . 52.512, P < 0.001). Overall, only two treatments were significantly different than the control, suggesting that this organism was not very sensitive to the CECs being tested.
Another plant beneficial organism, B. japonicum, was found to be insensitive to the CECs tested. This plant growth promoting rhizobacteria (PGPR) did not exhibit any signs of sensitivity to the CECs during its growth rate assay carried out in liquid media. B. japonicum remained in the exponential growth phase from 0 to about 24 h post inoculation (hpi) (Figures S3C–F). During this time, no significant differences in optical density were detected among different treatments within a given sampling time point. Therefore, it appears that B. japonicum is not sensitive to the CECs at the concentrations tested. To confirm this result an additional specific plate count assay of cells at 18 hpi was carried out. There were no significant differences among cell counts from different treatments (ANOVA: F8 = 1.203; P = 0.36) (data not shown).
Besides impacting mycelial growth of fungal organisms, CECs impacted spore germination of V. dahliae 0049 and F. oxysporum CS-5 (Figure 3A). At 12 hpi, the V. dahliae no CEC control had significantly greater spore germination than all but two treatments, APAP-H and SMX-L (GLIM: = 95.648, P < 0.01; post-hoc pairwise, P < 0.05). TMP-H treatment had the least number of germinated of spores compared to the other treatments (GLIM: = 95.648, P < 0.001). TMP also impacted spore germination of F. oxysporum CS-5. Both TMP treatments had lower spore germination than the control. TMP-L had the lowest spore germination out of all treatments and was significantly lower than the control and APAP-L (Figure 3B) (GLiM: = 19.076, P = 0.014).
Impact of CECS on Plant Growth and Development
A greenhouse assay was carried out to evaluate the direct impacts of CECs on plant growth, monitor for changes in Verticillium wilt disease progression and severity using eggplants and V. dahliae strain 0049. Overall, the greenhouse assay indicated that the CECs studied did not have major effects on the growth and development of plants. Repeated ANOVA analyses showed that there was a significant interaction between time and treatments for stem heights. (set I: F25.137, 111.721 = 3.901, P ≤ 0.001; set II: F33.43, 148.577 = 9.704, P ≤ 0.001). As expected though, inoculation with V. dahliae proved to have drastic effects on plant growth. Major differences in stem height and other plant metrics discussed below occurred between inoculated and uninoculated eggplants. No significant differences in stem height occurred among plants treated with different CECs, or a different concentration of the same CEC within the set of inoculated or uninoculated plants (P > 0.05 for all post hoc comparisons). However, all of the inoculated plants had significantly lower stem height than their uninoculated counter parts by week 6 and later (P's <0.05 for all post hoc tests) (Figures 1D, 4A–D).
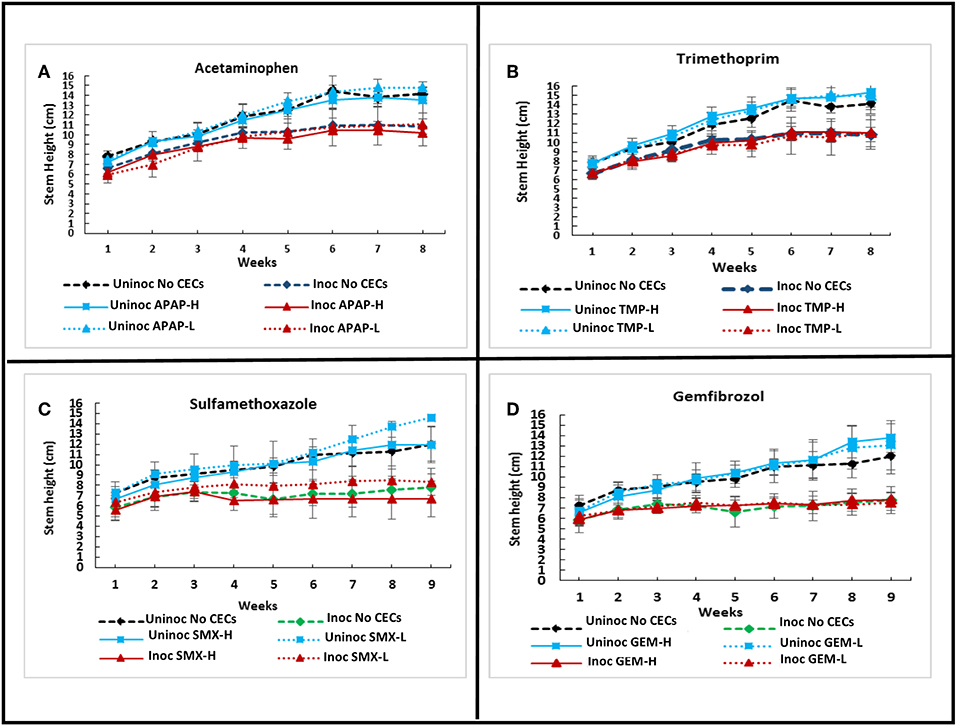
Figure 4. Effects of chemicals of emerging concern (CECs) overtime on stem height of inoculated and uninoculated eggplants grown in the greenhouse. Error bars represent standard deviation. (A) Eggplants treated with acetaminophen (APAP) and a no CEC control. (B) Eggplants treated with trimethoprim (TMP) and the no CEC control. (C) Eggplants treated with sulfamethoxazole (SMX) and a no CEC control. (D) Eggplants treated with gemfibrozil (GEM) and a no CEC control. The same no CEC controls were used for (A,B), while (C,D) have the same no CEC controls. For each CEC two concentrations were used high (H) and low (L).
Not surprisingly, a similar trend is revealed when measuring the number of leaves retained by the eggplants during the growing season (Figure 5A). There was a significant interaction between time and treatment for leaves remaining on plants (repeated measures Friedman Ranks ANOVA: setI: F32.856, 146.027 = 2.207, P ≤ 0.001; set II: F38.308, 170.257 = 8.825, P ≤ 0.001). Inoculation with V. dahliae led to higher leaf loss than the uninoculated controls in all treatments (Figures 1D, 5A). From the 6th week to the end of the experiment all of the inoculated plants retained significantly less leaves than their uninoculated counterparts (All P's < 0.005). Some differences with leaf retention did occur between treatments and their respective controls, but only with inoculated plants. These differences occurred in weeks 4 (inoc APAP-H, inoc TMX-H, inoc SMX-L, and inoc GEM-L), 5 (inoc APAP-H and inoc SMX-L), 6 (inoc SMX-H), and 8 (inoc APAP-H) (post hoc comparisons all P's < 0.05)
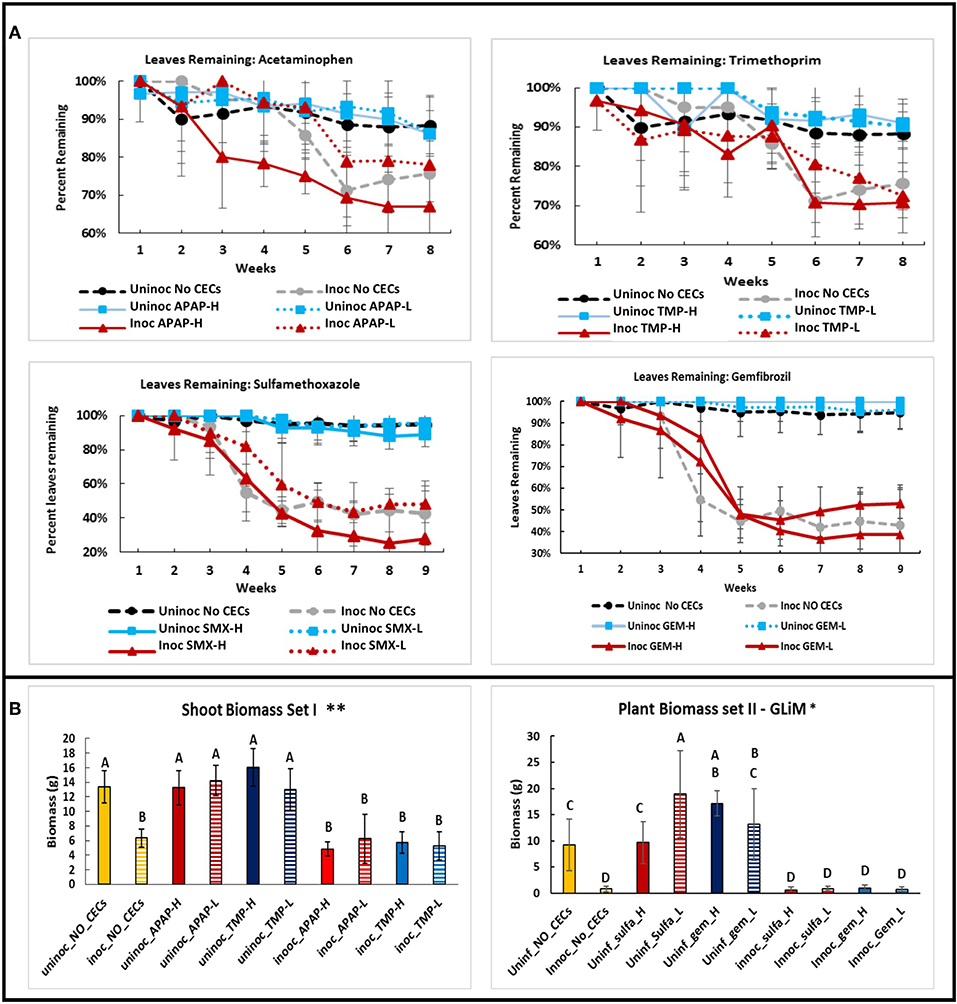
Figure 5. Comparisons of plant health metrics of eggplants grown in the green house among different chemicals of emerging concern (CECs) treatments. (A) Line graphs of leaves remaining on plants over time for each CEC. (B) Above ground biomass of both sets of eggplants at the end of the experiment. The CECs used were acetaminophen (APAP), trimethoprim (TMP), sulfamethoxazole (SMX) and gemfibrozil (GEM). For each CEC two concentrations were used high (H) and low (L). *Samples compared statistically using GLiM with normal distribution and identity link function, pairwise analyses with sequential Bonferroni pairwise correction for multiple comparisons (P < 0.05). **ANOVA used for statistical analyses, post-hoc Tukey test used for pairwise analyses (P < 0.05).
Shoot fresh weight followed the same trend as stem height and leaf loss discussed above except for 2 treatments. Among the uninoculated plants, both SMX-L and GEM-H had significantly higher shoot weight than their associated uninoculated no CEC control treatment (GLiM: = 186.179, P < 0.001). Major differences among inoculated and uninoculated samples were observed, with uninoculated samples having significantly higher shoot fresh weight than their inoculated counterparts (Set I plants: ANOVA: F9 = 20.281, P < 0.001; Set II plants: GLiM: = 186.179, P < 0.001 (Figure 5B).
Impact of CECs on Disease Severity
Inoculation of eggplants with V. dahliae caused Verticillium wilt disease. Disease severity was calculated based on the disease scoring and disease severity index according to Liu et al. (2009b) (Figure 6). There was a significant interaction of disease severity between treatments and time (repeated measures Friedman Ranks ANOVA: set I: F29.304, 130.240 = 1.140, P ≤ 0.001; set II: F30.609, 136.038 = 25.0123, P ≤ 0.001). Disease severity varied with the type of CEC and the growth phase of the plants. Most treatments reached their disease index maximum by 5 weeks and had the fastest increase between week 3 and 5. Some of the plant treatments still increased in severity after week 5, while most remained steady. Plants treated with TMP had a higher, but not significant disease severity index earlier in the season (between week 2 and 5) compared to the other CECs and the no CECs control (Figures 6A,B). However, SMX and GEM had lower disease index than the control between week 2 and 5, but after that their disease index was above the control although it was not significant (Figures 6C,D). Both inoculated TMP-H and inoculated no CECs had steady increases in disease severity after 5 weeks (Figures 6A,B). APAP-L and TMP-L plateaued at low disease severity between week 5 and 7, but TMP-L plants sharply increased in severity near the end of the experiment. APAP-L, however, maintained relatively low levels of disease severity to the end of the experiment, week 8. Plants treated with TMP (high or low) increased in disease severity index between week 7 and 8, while the disease index of the control plants reduced in this time. APAP-L had significantly lower disease severity than the inoculated TMP-H and the inoculated no CEC treatment by week 8 (GLiM: = 15.536, P = 0.004) (Figure 6A). The disease severity of the eggplants was used to calculate the disease index. As expected, the disease index results paralleled the disease severity results (Figures 6B,D).
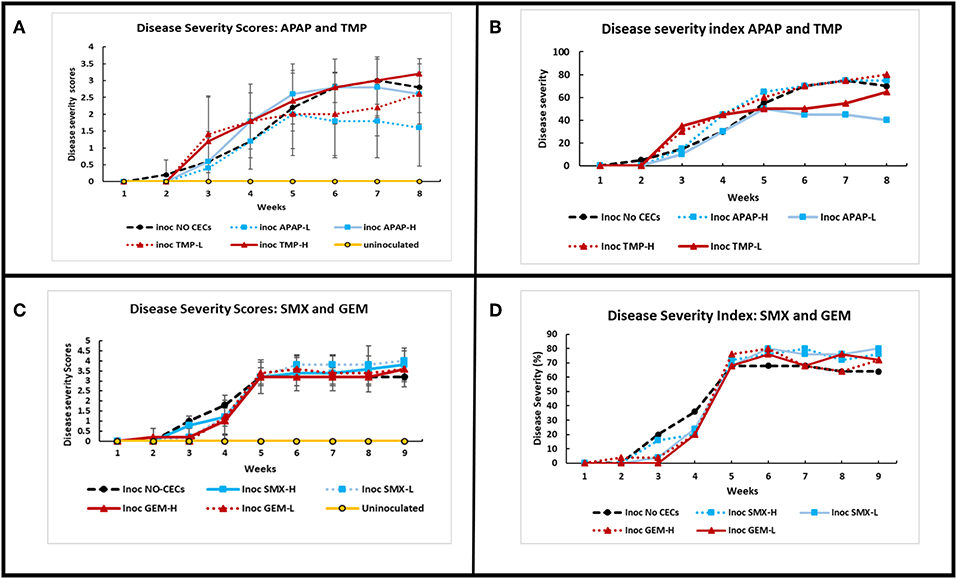
Figure 6. Comparison of disease severity and disease severity index of greenhouse grown eggplants inoculated with Verticillum dahliae and watered with or without the addition of chemicals of emerging concern. Calculations to determine the disease severity index (DSI) are described in Liu et al. (2009b) and Chiang et al. (2017). All error bars shown represent standard deviation. (A) Disease severity of inoculated and uninoculated eggplants for acetaminophen (APAP) and trimethoprim (TMP), treatments. (B) Comparing DSI values for APAP and TMP treatments over time. (C) Disease severity of inoculated and uninoculated eggplants for sulfamethoxazole (SMX) and gemfibrozil (GEM)treatments over time. (D) Comparing DSI values for SMX and GEM treatments over time.
Discussion
In this study we investigated the impacts of CECs at concentrations found in reclaimed water on plant microbes and Verticillium wilt disease severity on eggplants. Microorganism growth assays done in vitro showed that the tested microorganisms were differentially affected by CECs. Even strains of the same fungus responded differently to the same CEC. In the greenhouse experiments, plant disease index varied with the stage of plant growth and the CEC that was applied. APAP and TMP had stronger impacts on disease development early in the season, while SMX and GEM appeared to have less impact on disease development early in the season. APAP and TMP had a stronger influence of disease development at higher concentrations than lower concentrations, but the concentrations of SMX and GEM used did not differently impact in disease development. Inoculation with the pathogen V. dahliae had the strongest impact on disease development than any CEC treatment.
Impact of CECs on Microorganism Growth
In this study we investigated the impact of CECs on growth of microorganism in vitro. We used growth rates to calculate the allelopathic index, which is normally used to evaluate true allelopathy of chemicals excreted by organisms directly into the environment (Williamson and Richardson, 1988; Liu et al., 2009b). By applying this concept to extrinsically derived chemicals we can compare the impacts of anthropogenic CECs to naturally formed compounds used by the producing organism to influence other organisms. Originally the concept of allelopathy was used to refer to any chemical involved with positive or negative plant-plant interactions (Patrick, 1986). Over time this concept has evolved to include chemicals involved between plant-plant or plant-microbe interactions, but only in the negative, or inhibitory sense (Patrick, 1986). Lower, negative RI values have been associated with reduction of disease severity in plants in some experiments (Liu et al., 2009a; Zhou et al., 2011). Although, in the study by Liu et al. (2009b) significantly lower RI values of root exudates did not always associate with reduced disease severity or incidence. Suggesting that growth can only partially explain disease progression or infectivity. It is likely that there are many other factors involved in the interaction between hosts, CECs, and pathogenicity. Environmental factors such as pH (Zimmermann and Curtis, 2017) or carbon substrate availability (Hida et al., 2005) can influence potency of some CECs. Environmental factors can also influence a microorganism's ability to infect a given host (Jarosz and Burdon, 1988). The interactions of CECs with plants and microbes are multifaceted.
Our microbial growth assays showed that nearly all the microbes tested were sensitive to the CECs used in this study. However, the specific effects of the CECs varied, among the organisms. CECs have been observed to exhibit growth promoting effects on microbes (Carvalho et al., 2010; Zimmermann and Curtis, 2017), while growth inhibition by these chemicals typically occurs at higher concentrations (Koch and Burchall, 1971; Kabbash et al., 2004; Argyropoulou et al., 2009; Al-Janabi, 2010). For these reasons we predicted that the microorganisms would not be affected the presence of CECs. We observed increased growth in the presence of CECs with two fungi, V. dahliae 0048 and F. oxysporum CS-3. Both of these strains exhibited significantly more growth and higher RI values compared to their controls, suggesting that these CECs have growth promoting effects on these particular organisms. In contrast, other strains of the same fungi V. dahliae 0049 and F. oxysporum CS-5 had nearly an exact opposite trend in which the CECs either had no effect or were inhibitory to mycelial growth, suggesting that our hypothesis was partially correct. Their RI values correspond well to values found for root exudate of resistant tomato plant roots (−0.155 to −0.020) (Liu et al., 2009b). Having similar RI values to root exudates that exhibit antimicrobial effects also suggests that the in situ levels of CECs are at biologically relevant concentrations. Our results concurred with another study that showed that closely related fungi can respond differently to some CECs, in particular, sulfamethoxazole (Hida et al., 2005).
Not all the microorganisms evaluated were equally sensitive to all the CECs. P. indica was only affected by two CEC treatments in opposing ways at two separate time points, while the growth of the bacterium B. japonicum was not affected by any CEC. These CECs usually exhibit antimicrobial effects at equal or greater concentrations of those found in human blood plasma levels corresponding to therapeutic doses. Typical therapeutic plasma levels of these CECs fall within the ranges of 10,000–20,000 μg/L; 3,000–8,000 μg/L; 80,000–100,000 μg/L, and 19,000–45,000 μg/L for APAP, TMP, SMX, and GEM, respectively (Nolte and Buettner, 1974; Reeves and Wilkinson, 1979; Spence et al., 1995; Kyrklund et al., 2003; Niemi et al., 2003; Stuart et al., 2004; Clajus et al., 2013; Zimmermann and Curtis, 2017), which are about 10,000–100,000 times more than those used in our study (Table 1). However, these studies were conducted on human pathogens and not soilborne organisms. CECs have been shown to disrupt the functions of soilborne nitrogen cycling bacteria when tested at concentrations higher than therapeutic concentrations (Colloff et al., 2008) and at levels found in wastewater treatment plant effluent (Wang and Gunsch, 2011).
Taken together our results and those of others indicate that many more plant or soil associated organisms may be sensitive to CECs at the concentrations found in reclaimed water. Thus, additional studies on the impacts of CECs on free living and plant associated microorganisms are necessary to fully understand the impacts of these chemicals on disease severity especially in fields where reclaimed water has been used to irrigate plants for years. We observed that fungal strains were sensitive to CECs concentrations well below typical therapeutic doses. Our microbial growth rate assays demonstrated that these particular CECs are capable of impacting microbial growth at in situ concentrations and suggesting the potential to disrupt plant- pathogen dynamics.
Spore Germination of Microorganisms
The spore germination assays demonstrated that the CECs used in this study can affect fungal spore germination in addition to mycelial growth discussed above. In spore germination assays we used sucrose solutions to decrease the osmotic potential of the solution to optimal spore germination conditions as described for V. dahliae in Ioannou et al. (1977). We used the same sucrose solutions for the F. oxysporum CS-5 assay to optimize spore germination as well. When we used sterile water, the germination rate was only 5%. This is similar to results obtained by Steinkellner et al. (2005), who also obtained 5% germination rate of F. oxysporum in sterile water. The control (sucrose only solution) for our V. dahliae spore germination assay reached over 80%, which is comparable to the amount of spore germination in a previous study that uses similar methods (Ioannou et al., 1977). All of the CEC treatments had lower spore germination than the control, although two (APAP-H and SMX-L) were not significantly lower. TMP-H treatment had the greatest impact on V. dahliae 0049 spore germination and had significantly less germination than all treatments. This combined with the results of the mycelium growth assay, discussed above, suggest that this particular strain of V. dahliae is sensitive to TMP. Although spore germination did not get as high in the F. oxysporum CS-5 assay, the sucrose solution was successful with increasing spore germination rates. The average spore germination for the F. oxysporum assay reached above 40% for the controls, which is higher than the about 5% germination amounts generated in the water only (Steinkellner et al., 2005). Over all, spore germination of F. oxysporum CS-5 exhibited less sensitivity to the CECs, but TMP had an effect of decreasing spore germination. The effects of TMP in reducing spore germination and mycelial growth did not translate to reduced disease severity as discussed below. The combined results of our spore germination assay suggest that soilborne microbes may be altered by CECs found in reclaimed water, which may impact plant-microbe interactions and plant disease severity.
Impact of CECS on Plant Growth and Severity of Verticillium Wilt of Eggplants
Eggplants were grown in a greenhouse at the University of California, Riverside to evaluate the impacts of CECs on host-pathogen dynamics. Plants were watered with the range of concentrations of the respective CECs to simulate agriculture conditions in which plants are being irrigated with reclaimed water (Table 1) (Kinney et al., 2006; Vanderford and Snyder, 2006; Batt et al., 2007; Fram and Belitz, 2011). We predicted that disease severity would increase because the CECs would most likely enhance V. dahliae growth (Carvalho et al., 2010). Antibacterial compounds can displace microbes that are antagonistic toward plant pathogens, allowing them to increase in numbers and therefore have a higher chance to establish an infection (Mulligan et al., 1982; De Vries-Hospers et al., 1991; Azevedo et al., 2015). Previous studies have shown that certain pharmaceutical products can have detrimental impacts on plants (Liu et al., 2009a; Madikizela et al., 2018). The impacts of CECs on disease severity or disease severity index varied with the CEC: TMP-H had a higher severity index earlier in the season (week 2–4), while SMX at both concentrations had a greater impact later in the season (week 5–9). APAP-L and TMP-L had the least effects on disease index between week 5 and 7. However, these differences were not statistically significant from the associated controls. Our result indicated that APAP-L and TMP-L were beneficial to the eggplant in some capacity. However, the exact interaction or mechanism is not known and requires additional study.
Besides examining disease severity, plant growth metrics of stem height, leaf loss, and shoot fresh weight were used to assess if the CECs directly impacted plant growth, or if there were any interactions between plant growth, disease severity and CECs. As expected, both stem height and final shoot fresh weight were significantly lower in the V. dahliae inoculated plants than in the uninoculated ones. Inoculated plants also lost significantly more leaves. Uninoculated plants did not have any indications of Verticillium wilt. By the end of the experiment, there were no significant differences found for stem heights or percentage of leaf loss between the different CEC treatments for inoculated or uninoculated plants. Plant growth was not impacted in any visible way by the concentrations of the CECs we used. This fits well with other studies that indicate that larger, more complex organisms, such as developed plants, will not be impacted directly by CECs at low concentrations (Wu et al., 2013). Although, other studies indicate that plants take up these compounds and that these CECs can be found in tissues of plants that have been irrigated with water containing these CECs (Dodgen et al., 2013; Wu et al., 2013, 2015). Most of these studies have only been conducted within a single growing season. However, these chemicals can accumulate into tissues over time (Wu et al., 2010) thus longer studies are needed given that these compounds are impacting microbial and insect life at low concentrations (Pennington et al., 2018). Other studies have also found direct impacts to plants by anthropogenic compounds. The studies by D'Abrosca et al. (2008) and Pino et al. (2016) observed growth inhibition of plants when they were exposed to low levels of anthropogenic chemicals, including gemfibrozil. Inhibition of seed germination by CECs was also observed in the study D'Abrosca et al. (2008). Together these studies suggest that seeds and seedlings are more vulnerable to CECs, and the chemicals may have direct impacts on developed plants once they reach higher concentrations. In our study the plants used were at the 4th leaf stage and therefore had time to develop beyond the seedling stage before being exposed to CECs, which partially explains why there was not a great impact to their stem growth, or leaf production. In addition, some studies found that not all plants are impacted equally by a given CEC (D'Abrosca et al., 2008), and some plant tissues such as roots, maybe more sensitive to CECs than other parts of the plant (Pino et al., 2016).
By the end of the experiment we observe that two treatments had effects on shoot fresh weight. The uninoculated SMX-L treatment had significantly greater biomass than the associated uninoculated control. This result suggests that at low concentrations SMX may be beneficial for plant growth. Perhaps the plants are able to utilize the sulfur component of sulfamethoxazole. We also saw significant shoot fresh weight in plants treated with GEM-L than the associated uninocualted no CEC control. Other studies conducted on plants at the seed stage have indicated that even low concentrations of GEM can have deleterious impacts on growth (D'Abrosca et al., 2008; Pino et al., 2016). However, these studies were conducted on seeds. Developed plants appear to be more resistant to chemical interference. The study Wu et al. (2013) found no phytotoxic effects of the CECs they tested on mature plants at low concentrations. It has been observed that small amounts of harmful substances can actually stimulate plant growth in a concept known as hormesis (Pan and Chu, 2017). Hormesis may at least partially explain the increased shoot biomass observed in the SMX-L and GEM-L treatments (Pan and Chu, 2017). The two studies that found GEM to be harmful were also done in the absence of soil, while our plants were grown directly in soil. GEM was most likely broken down or partially absorbed by the soil, thus further reducing the amount of the chemical the eggplants were exposed to Pan and Chu (2017). A lower amount of GEM may not have phytotoxic effects and instead have stimulatory effects on a given plant due to hormesis. However, the concept of hormesis was not formally tested in this experiment and should be investigated further.
Data Availability Statement
All datasets generated for this study are included in the manuscript/Supplementary Files.
Author Contributions
EG conceived and planned the study. NM carried out the experimental work and data analyses. EG and NM interpreted the results and wrote the manuscript.
Funding
This work was supported by the University of California, Riverside Startup funds to EG.
Conflict of Interest
The authors declare that the research was conducted in the absence of any commercial or financial relationships that could be construed as a potential conflict of interest.
Acknowledgments
We would like to acknowledge the University of California, Riverside for start-up funds to EG. We would like to thank Dr. Alex Putman for donating the Verticillium dahliae strains, Dr. Soumaila Sanogo for donating Phytophthora capsici, and Dr. Ralf Oelmueller for donating the Piriformospora indica strain. We would also like to thank Rochelle Medel for her help with data collection.
Supplementary Material
The Supplementary Material for this article can be found online at: https://www.frontiersin.org/articles/10.3389/fenvs.2019.00156/full#supplementary-material
References
AghaKouchak, A., Feldman, D., Hoerling, M., Huxman, T., and Lund, J. (2015). Water and climate: recognize anthropogenic drought. Nature 524, 409–411. doi: 10.1038/524409a
Aguiar, J., Molinar, R., and Valencia, J. (1998). Eggplant Production in California. doi: 10.3733/ucanr.7235
Al-Janabi, A. A. H. (2010). In vitro antibacterial activity of ibuprofen and acetaminophen. J. Glob. Infect. Dis. 2, 105–108. doi: 10.4103/0974-777X.62880
Argyropoulou, I., Geronikaki, A., Vicini, P., and Zani, F. (2009). Synthesis and biological evaluation of sulfonamide thiazole and benzothiazole derivatives as antimicrobial agents. Arkivoc 2009, 89–102. doi: 10.3998/ark.5550190.0010.611
Azevedo, M. M., Teixeira-Santos, R., Silva, A. P., Cruz, L., Ricardo, E., Pina-Vaz, C., et al. (2015). The effect of antibacterial and non-antibacterial compounds alone or associated with antifugals upon fungi. Front. Microbiol. 6:669. doi: 10.3389/fmicb.2015.00669
Batt, A. L., Kim, S., and Aga, D. S. (2007). Comparison of the occurrence of antibiotics in four full-scale wastewater treatment plants with varying designs and operations. Chemosphere 68, 428–435. doi: 10.1016/j.chemosphere.2007.01.008
Bhat, R. G., and Subbarao, K. V. (1999). Host range specificity in Verticillium dahliae. Am. Phytopathol. Soc. 89, 1218–1225. doi: 10.1094/PHYTO.1999.89.12.1218
Boxall, A. B. A., Johnson, P., Smith, E. J., Sinclair, C. J., Stutt, E., and Levy, L. S. (2006). Uptake of veterinary medicines from soils into plants. J. Agric. Food Chem. 54, 2288–2297. doi: 10.1021/jf053041t
Brown, E., Rodriquez, M., Marcus, F., Spivy-Webber, F., Doduc, T., and Moore, S. (2013). Policy for water quality control for recycled water (recycled water policy). Calif. State Water Resour. Control Board 1–14. Available online at: https://www.waterboards.ca.gov/water_issues/programs/water_recycling_policy/docs/recycledwaterpolicy_approved.pdf
Bulatova, N. R., and Darwish, R. M. (2008). Effect of chemosensitizers on minimum inhibitory concentrations of fluconazole in Candida albicans. Med. Princ. Pract. 17, 117–121. doi: 10.1159/000112964
California Department of Food Agriculture (2018). California Agricultural Statistics Review 2017-2018. Sacromento, CA. Available online at: www.cdfa.ca.gov/statistics
California State Water Resources Control Board (2010). Final Report (Draft for Public Comments) Monitoring Strategies for Chemicals of Emerging Recommendations of a Science Advisory Panel Panel Members. Sacromento, CA. Available online at: http://www.santacruzwatersupply.com/sites/default/files/resource-files/CECMonitoringInCARecycledWater_FinalReport.pdf
Carvalho, A. P., Gursky, L. C., Rosa, R. T., Rymovicz, A. U. M., Campelo, P. M. S., Grégio, A. M. T., et al. (2010). Non-steroidal anti-inflammatory drugs may modulate the protease activity of Candida albicans. Microb. Pathog. 49, 315–322. doi: 10.1016/j.micpath.2010.07.007
Chiang, K. S., Liu, H. I., and Bock, C. H. (2017). A discussion on disease severity index values. Part I: warning on inherent errors and suggestions to maximise accuracy. Ann. Appl. Biol. 171, 139–154. doi: 10.1111/aab.12362
Clajus, C., Kuhn-Velten, W. N., Schmidt, J. J., Lorenzen, J. M., Pietsch, D., Beutel, G., et al. (2013). Cotrimoxazole plasma levels, dialyzer clearance and total removal by extended dialysis in a patient with acute kidney injury: risk of under-dosing using current dosing recommendations. BMC Pharmacol. Toxicol. 14:19. doi: 10.1186/2050-6511-14-19
Colloff, M. J., Wakelin, S. A., Gomez, D., and Rogers, S. L. (2008). Detection of nitrogen cycle genes in soils for measuring the effects of changes in land use and management. Soil Biol. Biochem. 40, 1637–1645. doi: 10.1016/j.soilbio.2008.01.019
Cooley, H., and Phurisamban, R. (2016). The Cost of Alternative Water Supply and Efficiency Options in California. Oakland, CA: Pacific Institute.
D'Abrosca, B., Fiorentino, A., Izzo, A., Cefarelli, G., Pascarella, M. T., Uzzo, P., et al. (2008). Phytotoxicity evaluation of five pharmaceutical pollutants detected in surface water on germination and growth of cultivated and spontaneous plants. J. Environ. Sci. Heal. A 43, 285–294. doi: 10.1080/10934520701792803
De Vries-Hospers, H. G., Tonk, R. H. J., and Van Der Waaij, D. (1991). Effect of intramuscular ceftriaxone on aerobic oral and faecal flora of 11 healthy volunteers. Scand. J. Infect. Dis. 23, 625–633. doi: 10.3109/00365549109105188
Dia-Cruz, M. S., and Barcelo, D. (2008). Trace organic chemicals contamination in ground water recharge. Chemosphere 72, 333–342. doi: 10.1016/j.chemosphere.2008.02.031
Dodgen, L. K., Li, J., Parker, D., and Gan, J. J. (2013). Uptake and accumulation of four PPCP/EDCs in two leafy vegetables. Environ. Pollut. 182, 150–156. doi: 10.1016/j.envpol.2013.06.038
EPA (2019). Contaminants of Emerging Concern including Pharmaceuticals and Personal Care Products. www.epa.gov. Available online at: https://www.epa.gov/wqc/contaminants-emerging-concern-including-pharmaceuticals-and-personal-care-products
Erickson, M. L., Langer, S. K., Roth, J. L., and Kroening, S. E. (2014). Contaminants of Emerging Concern in Ambient Groundwater in Urbanized Areas of Minnesota, 2009–12 (ver. 1.2, September 2014): U.S. Geological Survey Scientific Investigations Report 2014–5096, 38, with appendix. doi: 10.3133/sir20145096
Feys, J. (2015). npIntFactRep: Nonparametric Interaction Tests for Factorial Designs With Repeated Measures. Available online at: https://cran.r-project.org/web/packages/npIntFactRep/npIntFactRep.pdf
Feys, J. (2016). Nonparametric tests for the interaction in two-way factorial designs using R. R J. 8, 367–378. doi: 10.32614/RJ-2016-027
Fram, M. S., and Belitz, K. (2011). Occurrence and concentrations of pharmaceutical compounds in groundwater used for public drinking-water supply in California. Sci. Total Environ. 409, 3409–3417. doi: 10.1016/j.scitotenv.2011.05.053
Gros, M., Petrovi, M., Ginebreda, A., and Barceló, D. (2010). Removal of pharmaceuticals during wastewater treatment and environmental risk assessment using hazard indexes. Environ. Int. 36, 15–26. doi: 10.1016/j.envint.2009.09.002
Hida, S., Yoshida, M., Nakabayashi, I., Miura, N. N., Adachi, Y., and Ohno, N. (2005). Anti-fungal activity of sulfamethoxazole toward Aspergillus Species. Biol. Pharm. Bull. 28, 773–778. doi: 10.1248/bpb.28.773
Hirano, Y., and Arie, T. (2006). PCR-based differentiation of Fusarium oxysporum ff. sp. lycopersici and radicis-lycopersici and races of F. oxysporum f. sp. lycopersici. J. Gen. Plant. Pathol. 72, 273–283. doi: 10.1007/s10327-006-0287-7
Howitt, R., Macewan, D., Medellín-azuara, J., Lund, J. R., and Sumner, D. (2015). Economic Analysis of the 2014 Drought for California Agriculture. Davis, CA: Center for Watershed Sciences. University of California. Available online at: http://watershed.ucdavis.edu
Inderbitzin, P., Davis, R. M., Bostock, R. M., and Subbarao, K. V. (2013). Identification and differentiation of Verticillium species and V. longisporum lineages by simplex and multiplex PCR assays. PLoS ONE 8:e65990. doi: 10.1371/journal.pone.0065990
Ioannou, N., Schneider, R. W., Grogan, R. G., and Duniway, J. M. (1977). Effect of water potential and temperature on growth, sporulation, and production of microsclerotia by Verticillium dahliae. Phytopathology 67, 637–644. doi: 10.1094/Phyto-67-637
Jarosz, A. M., and Burdon, J. J. (1988). The effect of small-scale environmental changes on disease incidence and severity in a natural plant-pathogen interaction. Oecologia 75, 278–281. doi: 10.1007/BF00378609
Kabbash, C., Shuman, H. A., Silverstein, S. C., and Della-Latta, P. (2004). Antimicrobial Activity of Gemfibrozil. U.S. Patent No 6,713,043.
Kinney, C. A., Furlong, E. T., Werner, S. L., and Cahill, J. D. (2006). Presence and distribution of wastewater-derived pharmaceuticals in soil irrigated with reclaimed water. Environ. Toxicol. Chem. 25, 317–326. doi: 10.1897/05-187R.1
Klosterman, S. J., Atallah, Z. K., Vallad, G. E., and Subbarao, K. V. (2009). Diversity, pathogenicity, and management of verticillium species. Annu. Rev. Phytopathol. 47, 39–62. doi: 10.1146/annurev-phyto-080508-081748
Koch, A. E., and Burchall, J. J. (1971). Reversal of the antimicrobial activity of trimethoprim by thymidine in commercially prepared media. Appl. Microbiol. 22, 812–7. Available online at: https://aem.asm.org/content/22/5/812.short
Kyrklund, C., Backman, J. T., Neuvonen, M., and Neuvonen, P. J. (2003). Gemfibrozil increases plasma pravastatin concentrations and reduces pravastatin renal clearance. Clin. Pharmacol. Ther. 73, 538–544. doi: 10.1016/S0009-9236(03)00052-3
Liu, F., Ying, G. G., Tao, R., Zhao, J. L., Yang, J. F., and Zhao, L. F. (2009a). Effects of six selected antibiotics on plant growth and soil microbial and enzymatic activities. Environ. Pollut. 157, 1636–1642. doi: 10.1016/j.envpol.2008.12.021
Liu, N., Zhou, B., Zhao, X., Lu, B., Li, Y., and Hao, J. (2009b). Grafting eggplant onto tomato rootstock to suppress verticillium dahliae infection: the effect of root exudates. HortScience 44, 2058–2062. doi: 10.21273/HORTSCI.44.7.2058
Madikizela, L. M., Ncube, S., and Chimuka, L. (2018). Uptake of pharmaceuticals by plants grown under hydroponic conditions and natural occurring plant species: a review. Sci. Total Environ. 636, 477–486. doi: 10.1016/j.scitotenv.2018.04.297
Masachis, S., Segorbe, D., Turrà, D., Leon-ruiz, M., Fürst, U., Ghalid, M., et al. (2016). A fungal pathogen secretes plant alkalinizing peptides to increase infection. Nat. Microbiol. 1, 1–8. doi: 10.1038/nmicrobiol.2016.43
Mulligan, M., Citron, D. M., McNamara, B. T., and Finegold, S. M. (1982). Impact of cefoperazone therapy on fecal flora. Antimicrob. Agents Chemother. 22, 226–230. doi: 10.1128/AAC.22.2.226
Niemi, M., Backman, J. T., Granfors, M., Laitila, J., Neuvonen, M., and Neuvonen, P. J. (2003). Gemfibrozil considerably increases the plasma concentrations of rosiglitazone. Diabetologia 46, 1319–1323. doi: 10.1007/s00125-003-1181-x
Nolte, H., and Buettner, H. (1974). Investigations on plasma levels of sulfamethoxazole in man after single and chronic oral administration alone and in combination with trimethoprim. Chemotherapy 20, 321–330. doi: 10.1159/000221822
Pan, M., and Chu, L. M. (2017). Science of the total environment fate of antibiotics in soil and their uptake by edible crops. Sci. Total Environ. 600, 500–512. doi: 10.1016/j.scitotenv.2017.04.214
Parsons, L. R., Sheikh, B., Holden, R., and York, D. W. (2010). Reclaimed water as an alternative water source for crop irrigation. HortScience 45, 1626–1629. doi: 10.21273/HORTSCI.45.11.1626
Patrick, Z. A. (1986). Allelopathic mechanisms and their exploitation for biological control. Can. J. Plant Pathol. 8, 225–228. doi: 10.1080/07060668609501831
Pegg, G. F. (1984). The Impact of Verticillium diseases in agriculture. Phytopathol. Mediterr. 23, 176–192. Available online at: https://www.jstor.org/stable/pdf/42684709.pdf
Pennington, M. J., Rothman, J. A., Dudley, S. L., Jones, M. B., Mcfrederick, Q. S., and Gan, J. (2017). Contaminants of emerging concern affect Trichoplusia ni growth and development on artificial diets and a key host plant. Proc. Natl. Acad. Sci. U.S.A. 114, 1–9. doi: 10.1073/pnas.1713385114
Pennington, M. J., Rothman, J. A., Jones, M. B., Mcfrederick, Q. S., Gan, J., and Trumble, J. T. (2018). Effects of contaminants of emerging concern on Myzus persicae (Sulzer, Hemiptera : Aphididae) biology and on their host plant, Capsicum annuum. Environ. Monit. Assess. 190, 1–11. doi: 10.1007/s10661-018-6503-z
Pino, M. R., Muñiz, S., Val, J., and Navarro, E. (2016). Phytotoxicity of 15 common pharmaceuticals on the germination of Lactuca sativa and photosynthesis of Chlamydomonas reinhardtii. Environ. Sci. Pollut. Res. 23, 22530–22541. doi: 10.1007/s11356-016-7446-y
R Core Team (2018). R: A Language and Environment for Statistical Computing. R Foundation for Statistical Computing, Vienna.
Reeves, D. S., and Wilkinson, P. J. (1979). The pharmacokinetics of trimethoprim and trimethoprim/sulphonamide combinations, including penetration into body tissues. Infection 7, 330–341. doi: 10.1007/BF01639009
Rudin, D. E., Gao, P. X., Cao, C. X., Neu, H. C., and Silverstein, S. C. (1992). Gemfibrozil enhances the listeriacidal effects of fluoroquinolone antibiotics in J774 macrophages. J. Exp. Med. 176, 1439–1447. doi: 10.1084/jem.176.5.1439
Schulte, P. (2011). Using recycled water on agriculture : sea mist farms and Sonoma County. Pacific Inst. Farm Water Success Stories Recycl. Water Agric. 1–10. Available online at: https://pacinst.org/publication/california-farm-water-success-stories/
Smith, G. (1941). The effect of adding trace elements to czapek-dox medium. Trans. Br. Mycol. Soc. 32, 280–283. doi: 10.1016/S0007-1536(49)80018-0
Spence, J. D., Munoz, C. E., Hendricks, L., Latchinian, L., and Khouri, H. E. (1995). Pharmacokinetlcs of the combination of fluvastatln and gemflbrozll. Am. J. Cardiol. 76, 80–83. doi: 10.1016/S0002-9149(05)80024-4
Stackelberg, P. E., Gibs, J., Furlong, E. T., Meyer, M. T., Zaugg, S. D., and Lippincott, R. L. (2007). Efficiency of conventional drinking-water-treatment processes in removal of pharmaceuticals and other organic compounds. Sci. Total Environ. 377, 255–272. doi: 10.1016/j.scitotenv.2007.01.095
Steinkellner, S., Mammerler, R., and Vierheilig, H. (2005). Microconidia germination of the tomato pathogen Fusarium oxysporum in the presence of root exudates. J. Plant Interact. 1, 23–30. doi: 10.1080/17429140500134334
Stuart, R. K., Braine, H. G., Lietman, P. S., Saral, R., and Fuller, D. J. (2004). Carbenicillin-trimethoprim/sulfamethoxazole versus carbenicillin-gentamicin as empiric therapy of infection in granulocytopenic patients. Am. J. Med. 68, 876–885. doi: 10.1016/0002-9343(80)90217-X
Tunali, Y., Karaca, H., Işcan, G., Demirci, F., and Benkli, K. (2012). Antibacterial and antifungal activities of some trimethoprim salts. Med. Chem. Res. 21, 932–935. doi: 10.1007/s00044-011-9605-5
Vanderford, B. J., and Snyder, S. A. (2006). Analysis of pharmaceuticals in water by isotope dilution liquid chromatography/tandem mass spectrometry. Environ. Sci. Technol. 40, 7312–7320. doi: 10.1021/es0613198
Wang, S., and Gunsch, C. K. (2011). Effects of selected pharmaceutically active compounds on the ammonia oxidizing bacterium Nitrosomonas europaea. Chemosphere 82, 565–572. doi: 10.1016/j.chemosphere.2010.10.007
Warsinger, D. M., Chakraborty, S., Tow, E. W., Plumlee, M. H., Bellona, C., Loutatidou, S., et al. (2018). A review of polymeric membranes and processes for potable water reuse. Prog. Polym. Sci. 81, 209–237. doi: 10.1016/j.progpolymsci.2018.01.004
Williams, J. S., Hall, S. A., Hawkesford, M. J., Beale, M. H., and Cooper, R. M. (2002). Elemental sulfur and thiol accumulation in tomato and defense against a fungal vascular pathogen. Plant Physiol. 128, 150–159. doi: 10.1104/pp.010687
Williamson, B. G., and Richardson, D. (1988). Bioassays for allelopathy: measuring treatment responses with independent controls. J. Chem. Ecol. 14, 181–187. doi: 10.1007/BF01022540
Wu, C., Spongberg, A. L., Witter, J. D., Fang, M., and Czajkowski, K. P. (2010). Uptake of pharmaceutical and personal care products by soybean plants from soils applied with biosolids and irrigated with contaminated water. Environ. Sci. Technol. 44, 6157–6161. doi: 10.1021/es1011115
Wu, X., Dodgen, L. K., Conkle, J. L., and Gan, J. (2015). Plant uptake of pharmaceutical and personal care products from recycled water and biosolids: a review. Sci. Total Environ. 536, 655–666. doi: 10.1016/j.scitotenv.2015.07.129
Wu, X., Ernst, F., Conkle, J. L., and Gan, J. (2013). Comparative uptake and translocation of pharmaceutical and personal care products (PPCPs) by common vegetables. Environ. Int. 60, 15–22. doi: 10.1016/j.envint.2013.07.015
Zhou, B. L., Chen, Z. X., Du, L., Xie, Y. H., Zhang, Q., and Ye, X. L. (2011). Allelopathy of root exudates from different resistant eggplants to Verticillium dahliae and the identification of allelochemicals. African J. Biotechnol. 10, 8284–8290. doi: 10.5897/AJB10.2300
Keywords: pharmaceuticals, reclaimed water, irrigation, soilborne, microorganisms
Citation: McLain NK and Gachomo EW (2019) Chemicals of Emerging Concern in Treated Wastewater Impact Microbial Growth. Front. Environ. Sci. 7:156. doi: 10.3389/fenvs.2019.00156
Received: 06 August 2019; Accepted: 25 September 2019;
Published: 18 October 2019.
Edited by:
Rui C. Martins, University of Coimbra, PortugalReviewed by:
Isaac Dennis Amoah, Durban University of Technology, South AfricaKonstantinos Plakas, Centre for Research and Technology Hellas, Greece
Joana Luísa Pereira, University of Aveiro, Portugal
Copyright © 2019 McLain and Gachomo. This is an open-access article distributed under the terms of the Creative Commons Attribution License (CC BY). The use, distribution or reproduction in other forums is permitted, provided the original author(s) and the copyright owner(s) are credited and that the original publication in this journal is cited, in accordance with accepted academic practice. No use, distribution or reproduction is permitted which does not comply with these terms.
*Correspondence: Emma W. Gachomo, ZW1tYS5nYWNob21vJiN4MDAwNDA7dWNyLmVkdQ==