- 1Energy Resources and Environment Program, School of Advanced International Studies, Johns Hopkins University, Washington, DC, United States
- 2Center for Environment and Development, College of Development Studies, Addis Ababa University, Addis Ababa, Ethiopia
Agriculture must raise productivity while addressing climate change in order to ensure the food security of a growing population. Adding fossil fuel energy to the agricultural system can increase productivity through the use of manufactured fertilizer but creates greenhouse gas emissions. This study quantifies an alternative in which energy is added to the agricultural system through a substitution of solar energy for fossil fuel energy by the tree species Faidherbia albida. This substitution can be quantified as an avoided emission of greenhouse gas, a climate benefit. F. albida trees have unusual phenology, leafing out during the dry season and shedding leaves in the rainy season. In agroforestry systems, F. albida adds nutrients and organic matter to the soil through leaf drop, and these are beneficial to the crop growing under the tree canopy. Dormant during the cropping season, they do not compete for light, water or nutrients, and contribute nitrogen to the soil under their canopy. This nitrogen benefit is analyzed in relation to an equivalent quantity of urea fertilizer. This is a substitution of solar energy that the trees use to obtain nitrogen from the atmosphere, for the fossil fuels used in the manufacture and transport of urea fertilizer. This energy contribution by the tree, within the food energy and water system, enhances the food production, and resilience of the system, as soil organic matter increases available water for the plants. This energy contribution to the Ethiopian farming system is estimated as 3.48 GJ ha−1 year−1, based on the nitrogen contribution. Greenhouse gas emissions are avoided by the substitution of solar energy for fossil fuel energy, a climate change mitigation benefit estimated as 0.116 tons CO2 ha−1 year−1. This mitigation is fundamentally different from sequestration of carbon in biomass or soil organic matter. It is a permanently avoided emission of carbon dioxide into the atmosphere, associated with a particular cropping year, and is not reversible, unlike carbon stored in biomass or soil organic matter that could return to the atmosphere. The potential extent of F. albida agroforestry is substantial and its potential climate change mitigation benefits are great.
Introduction
The relationship between energy inputs and agricultural productivity is a critical aspect of the food-energy-water nexus. An agroforestry production system based on the acacia species Faidherbia albida has shown great promise to provide an energy input by adding nitrogen to some agricultural systems. In effect, a F. albida agroforestry production system captures solar energy and converts it to a nitrogen fertilization input, through symbiotic nitrogen fixation in root nodules. This is quantified in the current study as equivalent to an amount of urea nitrogen fertilizer and the energy that would be required to manufacture and transport that amount of fertilizer to the field. The marked increases in crop yield, especially in maize associated with Faidherbia agroforestry (Saka et al., 1994), illustrate a direct link between this tree-based energy input and the potential for enhanced food security, especially since Faidherbia is well-adapted to challenging dryland regions such as the Sahel. F. albida has long been viewed as one of the most important acacia species due to the roles it plays in improving small-holder agriculture in developing countries and in recovering the degraded lands of some of the most difficult dryland landscapes, including in large areas of the Sahel (Barnes and Fagg, 2003). The species is an integral part of a highly successful development strategy known as Evergreen Agriculture (Garrity et al., 2010). The Evergreen Agriculture system is based on the integration of trees, into annual food production and cropping systems, and incorporates mutually supporting strategies that are applied in various combinations at different locations. In addition to cultivating beneficial trees, the Evergreen Agriculture system includes soil conservation structures, water collection, and targeted fertilizer and manure applications. The adoption of Evergreen Agriculture often associated with F. albida is becoming widespread in Africa, with demonstrated success in improving farmer livelihoods and agricultural sustainability in Ethiopia, Zambia, Malawi, Burkina Faso, and Niger (Garrity et al., 2010) and F. albida is one of the principle species incorporated into this approach.
Distribution and Biophysical Characteristics
Faidherbia albida can achieve heights of 30 m, with trunk diameters up to 2 m. It has a taproot that can reach a depth of 20 m to access ground water, pull nutrients from the deeper layers of the soil, and cycle these nutrients to the surface layers of the soil (Barnes and Fagg, 2003; Sileshi, 2016). Additionally, because F. albida pods fall at the end of the dry season, they become nutritious forage for livestock when other pastures and foraging sources are typically scarce (Barnes and Fagg, 2003).
The species has an extremely wide natural distribution across large areas of semi-arid Africa, from Senegal to Ethiopia and south through Kenya and Malawi into the Transvaal (Umar et al., 2013). In Ethiopia, it is predominantly grown in the agroforestry systems for fodder, mulch, soil improvement, and soil conservation.The species is highly unusual in that it exhibits “reverse” phenology, meaning that, unlike most other plant species that grow in areas with a distinct rainy season, F. albida sheds its leaves and goes dormant at the start of the rainy season, and only goes to full leaf during the dry season (Barnes and Fagg, 2003). Reverse phenology is an unusual trait that may have arisen as adaptation to competition from other tree species. Faidherbia is a poor competitor with other species of trees and this restricts its natural occurrence (Barnes and Fagg, 2003). Reverse phenology is a trait that gives the species a competitive advantage in colonizing and growing in alluvial soils on river banks, floodplains or in the river bed itself (Barnes and Fagg, 2003). Faidherbia becomes dormant during periodic flooding and is thus able to survive the inundation that kills other species of trees. This reverse phenology means that the species is out of phase with field crops growing nearby and under its canopy, so it does not compete with these crops for water, light, or nutrients. The tree uses a symbiotic relationship with nitrogen fixing bacteria living in nodules in its roots to pull nitrogen from the atmosphere. A portion of this nitrogen is translocated from the point of fixation in the nodules to the growing leaves in the canopy, throughout the period of leaf growth. This nitrogen enters the agricultural system primarily when the tree drops its leaves. The leaves may be incorporated into the soil and as decomposition proceeds the nitrogen in the leafy biomass enters the soil and becomes available to crops. This process provides numerous benefits to the crops beneath the canopy and within the area of influence of the tree. These benefits include enhanced availability of nitrogen, phosphorus1, and soil moisture, plus increased quantities of soil organic matter in the vicinity of the trees (Hadgu et al., 2009; Sileshi, 2016).
Effect on Crop Yields
These characteristics of the tree frequently increase crop yields and help to account for the popularity of the species with farmers (Wahl and Bland, 2013). Indeed, there is an extensive literature showing significant yield increases associated with F. albida for many crops including maize, millet, sorghum, and groundnut (Barnes and Fagg, 2003). Hadgu et al. (2009) found increases in yields of barley in Tigray Ethiopia tied to the impacts of F. albida. In Malawi, Saka et al. (1994) reported a 280% increase in maize yields under the canopy of these trees. Shitumbanuma (2012) reported consistent increases in yields of maize, soybeans, and groundnuts when these were grown with Faidherbia, but also found concurrently observed decreases in cotton yields. Boffa (1999) reported substantial increases in cereal grain production associated with F. albida, including increases in millet and sorghum that were observed when production under the Faidherbia canopy was compared with production outside of the area of influence of the trees, and these relative increases were often quite pronounced in years with below average rainfall. Faidherbia is easily incorporated into smallholder farming systems in which hand cultivation facilitates tillage activity around the tree's base. It has been incorporated into mechanized systems planted on a grid pattern with spacing measured to allow the passage of tillage equipment.
One of F. albida's greatest benefits is the addition of nitrogen to the soil in the area around the tree, primarily through the addition and subsequent decomposition of leaves to the soil surface (Barnes and Fagg, 2003). This quantity of nitrogen can be substantial and a major factor in the increased crop productivity that occurs in proximity to the tree (Rhoades, 1995; Sileshi, 2016). A recent study by Yengwe et al. (2018), sampled the total addition of nitrogen in leaf fall over two growing seasons and determined the addition on a per hectare basis, for three age classes of Faidherbia trees. The average value for this addition is used in the current study to provide a realistic baseline for determining the energy and climate mitigation contribution of Faidherbia in agricultural systems.
Energy Input to the Farming System
Although the addition of nitrogen and its benefits for soil fertility and crop growth are widely recognized, F. albida's corresponding energy input to the farming systems in which they are integrated, has not yet been explored. A part of this contribution can be quantified through the equivalent nitrogen benefit that the trees provide. This nitrogen benefit can be evaluated as an input equal to the energy expended in manufacturing, transporting, and applying the same amount of urea to the field. Viewing nitrogen fertilizer as a quantifiable energy input to agricultural production is well-established in the context of an energy analysis of agriculture, in the United States (Patzek, 2005; Pimental, 2009), including Pimental's definition of the term “energy,” but that analysis has neither been applied to the nitrogen contribution of F. albida nor been performed in relation to an energy equivalent substitution for urea fertilizer, prior to the current study, based on a review of the literature.
The current study performs these analyses and is part of an ongoing research effort examining the likely response of Ethiopian agricultural to climate change and potential climate shocks (Bakker et al., 2018). The current framework of this research examines the potential responses of the Ethiopian agricultural system in the interconnected Food-Energy-Water (FEWS) meta-system (Leck et al., 2015) in which it is embedded. The current study looks at one energy component of this meta-system and uses an Ethiopian case study as the starting point, consistent with the other aspects of the research effort.
Agriculture in Ethiopia
Agriculture in Ethiopia is a core component of the economy. Productivity in this sector is increasingly challenged by depletion of soil nutrients, high levels of livestock grazing pressure, loss of forest cover, population increases, and land use practices that do not ensure long term sustainability [IFDC (International Fertilizer Development Center), 2012]. The wide ranges of topographic, climatic factors, parent material, and land use have resulted in extreme variability of soils in Ethiopia. F. albida's contribution to Ethiopian agriculture needs to be understood in the context of Ethiopian soil nutrient status and nutrient depletion. Assessments of nutrient status of Ethiopian soils indicate ranges of 0.9–2.9 g N kg−1 soil and 0.4–1.10 g P kg−1 soil. The calculated national nutrient balances were on average: 47 kg N ha−1, 15 kg P205 ha−1, and 38 kg K2O ha−1 for the year 2000 (Haileslassie et al., 2005). At the national level, full nutrient balance results indicate a depletion rate of 122 kg N ha−1 yr−1, 13 kg P ha−1 yr−1, and 82 kg K ha−1 yr−1. The soil nutrient stocks are decreasing except in areas under permanent and vegetable crops. These challenges are exacerbated by the semi-arid climate of the region, which is subject to periodic droughts. The combined impact of these factors compromises Ethiopia's economic productivity and puts parts of the Ethiopian population at risk of food insecurity, malnutrition, and poor health outcomes [IFDC (International Fertilizer Development Center), 2012]. Reversing these trends, halting soil degradation, and boosting soil fertility, are top priorities of the Government of Ethiopia [IFDC (International Fertilizer Development Center), 2012].
Ethiopian farmers primarily use urea and diammonium phosphate (DAP) as fertilizers, but only 30–40% of Ethiopian small farmers apply fertilizers to their fields and often at rates below the recommended rates of application (Abdulkadi et al., 2017). Other sources of added plant nutrition may also be limited as, in some communities, 80% of animal manure is used as cooking fuel (Abdulkadi et al., 2017). F. albida, which is native to Ethiopia, can help reverse these trends in soil depletion by providing additional plant nutrition and soil amendment benefits to smallholder agricultural systems. The distribution of F. albida populations in Ethiopia is not uniform as F. albida tree populations are lower in the Northern regions of Tigray and Gonder than in the central highlands. The highest populations of F. albida trees are commonly found along Southern Ethiopia's Great Rift Valley lakes and Awassa, Koka, and Arba Minch drainage systems, as well as in the heavy vertisols around Debre Zeit (Barnes and Fagg, 2003).
Determination of Energy Savings of Faidherbia albida in Fertilizer Equivalents
Faidherbia albida makes significant additions of nitrogen to the soil annually and these additions can be expressed in terms of an equivalent quantity of urea fertilizer [CTFT (Centre Technique Forestier Tropical), 1989]. This nitrogen addition is valuable both as a nutrient and as an energy contribution, because the nitrogen provided by the trees substitutes for the energy required to manufacture and transport an equal amount of urea to the field where it would be applied. Yengwe et al. (2018) collected data on the total leaf fall added to the soil surface in maize plots in Zambia and analyzed the nitrogen content over two annual growing seasons. This study converts their value for nitrogen addition to an equivalent amount of nitrogen in the form of urea and uses this value as the basis for calculating both the energy input to the system and the climate mitigation benefit (Figures 1, 2).
Energetics of Fertilizer Manufacture
To determine this energy equivalent, it is first necessary to calculate the energy required to manufacture the ammonia, as ammonia is a necessary precursor to manufactured urea. A study by the U.S. Environmental Protection Agency [EPA (United States Environmental Protection Agency), 2017], indicated that producing each metric ton of ammonia in a typical fertilizer plant required between 30.9 GJ and 39.1 GJ of natural gas. Taking the average of these two values as representative, this study uses 35.0 GJ as the energy used to produce ammonia.
The ammonia produced is then used as a feedstock to produce urea. According to the same EPA study [EPA (United States Environmental Protection Agency), 2017], the energy intensity of urea production in a conventional total recycling plant, can be estimated as 6.0 GJ per ton of urea produced. The lower energy requirement for the production of urea as compared with that for ammonia is due, in part, to the energy required to break the triple chemical bond of atmospheric dinitrogen, during the production of ammonia.
To determine the total energy requirement of urea manufacture, the energy intensity of the urea production is added to the energy required to produce the ammonia feedstock used to manufacture urea. This ammonia energy input must be adjusted for the stoichiometric amount of ammonia used in the production of urea, which is 0.567 tons ammonia per ton of urea (Fertilizer Europe, 2000). The total energy used to produce urea is then the sum of the energy used in the production of urea and the energy used in the production of the ammonia feedstock (Equation 1).
Nitrogen Contribution and Urea Energy Equivalence
This energy value needs to be related to the nitrogen contribution of F. albida, so it can be appropriately adjusted to capture the energy contribution of the trees to the agricultural system. Yengwe et al. (2018) sampled leaf litter drop in three age classes of Faidherbia trees over two cropping seasons and then used this to determine the litter fall on a per hectare basis. By analyzing the nitrogen content of the leaf litter, they were able to estimate the nitrogen addition in the leaf drop (Table 1). The authors of the study discuss the possibility that variability in litterfall across seasons and age classes could be due to differences in rainfall distribution and amount, but they were not able to confirm this.
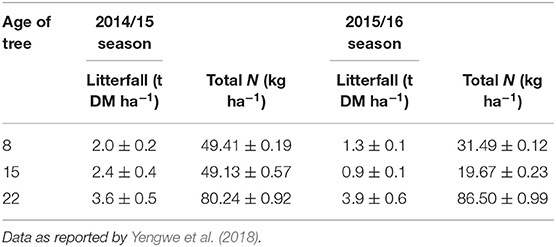
Table 1. Foliar litterfall quantity and nitrogen return for three age classes of Faidherbia albida trees for two growing seasons (average ± SE; n = 3).
The current study uses the averages of these values to determine the average amount of nitrogen returned to the soil in a typical growing season. Using the average of the nitrogen returned by the three age classes, the average Faidherbia Albida tree returned 59.59 total N (kg ha−1) for the 2014/15 season and 45.92 total N (kg ha−1) for the 2015/16. The average of these two values is 52.76 kg total N per hectare and this value is used in the current study as the representative value of nitrogen returned by F. albida trees to the soil in an annual growing season.
To obtain an equivalent amount of urea fertilizer, the current study used a stoichiometric value of 46% percent nitrogen in urea (CH4N2O)2 to convert the nitrogen value of 52.76 kg N ha−1 to a mass-based value of urea fertilizer. Equation (2) below, multiplies the nitrogen value by a factor of 2.17 (1/0.46) to obtain an equivalent amount of urea fertilizer equal to 114.48 Urea (kg ha−1).
To calculate the energy that would be required to manufacture this same amount of urea, Equation (3) below, multiplies this calculated urea equivalent in Equation (2) by the energy required to produce manufactured urea from Equation (1). The energy equivalent of the F. albida contribution in terms of the manufacture of urea is calculated as 2.91 GJ/ha-year (Equation 3).
A realistic estimate of the energy equivalent contribution of the F. albida trees with respect to urea also needs to take into account that the nitrogen from the trees is applied directly to the farm field, but manufactured urea must be transported to the farm for application. This study assumes that the urea will be applied in Ethiopia, in which urea fertilizer is applied at significant rates [IFDC (International Fertilizer Development Center), 2012] and F. albida is deeply integrated into some of its agricultural system (Hadgu et al., 2011). China is a primary source for urea shipped to Ethiopia [IFDC/AFAP (International Fertilizer Development Center/African Fertilizer Agribusiness Partnership), 2015], and typically, the urea is offloaded at the port of Djibouti and trucked to distribution warehouses in Ethiopia [IFDC (International Fertilizer Development Center), 2012].
Energetics of Urea Transport
To calculate the energy required to transport manufactured urea, this study assumes that the fertilizer is transported first by ship from the Chinese port of Shanghai to the port of Djibouti and next driven by truck from the port of Djibouti to a distribution warehouse in Addis Ababa. Further distribution within Ethiopia is not included in the calculation of the distribution from the warehouse to the individual fields would be prohibitive. Although other fertilizer routes and sources are possible, this route is realistic and captures a significant portion of the fertilizer import supply chain, allowing for a realistic and conservative calculation of the energy used to transport urea from China to Ethiopia.
The online shipping distance calculating tool found at sea-distances.org, estimates this ocean voyageto be 5,973 nautical miles. Although routes may vary, this estimate is within ~50 nautical miles of those obtained from other online commercial shipping distance calculating tools. For example, MarineTraffic.com estimated the most direct route of this voyage to be 6,000 nautical miles.
For purposes of calculation, 5,973 nautical miles was converted to 11,062 km and used in Equation (4) to calculate the energy expended to ship the 0.114 ton of urea equivalent provided by F. albida. Simonsen and Walnum (2011) estimate that 0.172 MJ/ton-km is the energy expended per ton-km of cargo sent by ocean shipping routes.
Equation (5) calculates the energy required to transport the manufactured urea by road from the port of Djibouti to distribution warehouses in Ethiopia. For overland transport by truck, the distance from the Djibouti Container Terminal to Addis Ababa, was estimated, using the online distance estimator Distanceto.com, to be 891 km. The International Energy Agency (IEA) (2009) has estimated the energy required for truck road freight transport in Africa at slightly more than 3.5 MJ ton-km−1. This study uses a conservative value of 3.5 MJ ton-km−1
The total energy of transportation is the sum of the energy used for marine and road transport (Equation 6).
Total Energy Contribution of F. albida
Thus, the equivalent energy contribution of F. albida to each hectare of the agricultural system, can be calculated by summing the energy used to manufacture urea fertilizer and the energy used to transport that fertilizer (Equation 7). Equation (7) estimates the total energy equivalent of F. albida fertilizer contribution to be 3.48 GJ per hectare per year.
Calculation of Emissions From Manufacture of Urea
The contribution of F. albida can also be quantified in terms of avoided CO2 emissions, or the emissions that would have occurred in manufacturing and transporting the equivalent urea fertilizer (Figure 2). With respect to the CO2 emissions involved in the manufacture of urea, it must be acknowledged that carbon dioxide is a feedstock for the manufacturing process, so some offsetting of CO2 occurs. On a stoichiometric basis, each ton of urea produced consumes 0.733 tons of CO2 (Fertilizer Europe, 2000). The emissions per Giga Joule are 56.0 kg CO2 [EPA (United States Environmental Protection Agency), 2017] and the adjusted emissions from a year of manufacturing are then the total emissions minus the CO2 recovered as feedstock (Equation 8) or 0.079 ton CO2 per ha-year.
Calculation of Emissions From Transportation of Urea
To obtain the total avoided CO2 emissions, the emissions from manufacturing must be added to the emissions produced by transporting the fertilizer. A corresponding estimate of the per-km emissions from deep water ocean transport (Equation 9) is 8.4 g CO2 per ton-km (McKinnon and Piecyk, 2010), and the total emissions from shipping 0.114 tons of urea from China to Djibouti are calculated in Equation (9). Equation (10) calculates the total emissions of trucking 0.114 tons of urea from the Port of Djibouti to the warehouses in Ethiopia. Transport of that quantity of urea for that distance is 101.5 ton-km, while the International Energy Agency (IEA) (2009) value for road freight energy usage of 3.5 MJ/ton-km, and Edwards et al. (2004) estimate that the value to relate energy expenditure for diesel fuel to CO2 emissions is 73.54 kg CO2/MJ. Thus, as shown in Equation (11), the total CO2 emissions from transporting the F. albida equivalent quantity of urea fertilizer is the sum of emissions from marine and road.
Calculation of Total Emissions
Finally, the emissions avoided by gaining the nitrogen input from the Faidherbia trees rather than from the application of manufactured urea can be calculated by adding the emissions from the urea manufacturing process to the emissions from transporting the urea. The annual total avoided emissions from the nitrogen provided by F. albida is 0.116 tons CO2 per hectare, as shown in Equation (12).
Discussion
On a per hectare basis through the contribution of nitrogen alone, F. albida could make an annual equivalent energy contribution of 3.48 GJ/ha in Ethiopia. Such a contribution can facilitate significant improvements in agricultural development such as enhanced productivity, enhanced crop nutrition, and adaptation to climate change for farming systems, as well as a contribution to climate change mitigation. These aspects are discussed further below. It is important to note that in different regions and under different climate and environmental circumstances, there may be significant variation in the nitrogen additions of F. albida to cropping systems. The values arrived at in this study, while based on sound research, and likely to have broad application, should not be regarded as precisely prescriptive in each instance, inside or outside of Ethiopia. Future research can build on the principles described in the current study to arrive at accurate assessments of the energy and climate contributions of F. albida in other regions and circumstances.
Soil Nitrogen Additions
A crucial factor for this study is the quantity of nitrogen added to the soil by the leaf litter from the F. albida trees. Earlier research has provided estimates that were far higher than the value used in the current study. One such estimate by the Centre Technique Forestier Tropical [CTFT (Centre Technique Forestier Tropical), 1989] estimated an annual addition of 480 Kg N ha−1 and linked this to an equivalent addition of 1.15 tons of urea nitrogen fertilizer. (Dancette and Poulain, 1969), cited in Umar et al. (2013) provide an estimate of 300 kg N ha−1 added by Faidherbia trees annually. A study in Zambia by Yengwe et al. (2017) estimated that, over a 35-year time span, a F. albida tree contributed between 61 and 78 kg N ha−1 year −1, with mature trees contributing an estimated 100 Kg N ha−1 year −1. By contrast, Umar et al. (2013), in another study in Zambia, estimated that a Faidherbia tree would provide 39 kg N ha−1 annually. Umar et al. (2013) also stated that, for small holders, this nitrogen addition could be viewed as a direct substitute for purchased mineral fertilizer. The value used for calculation in the current study is a conservative estimate that is consistent with the range of values reported in recent work on nitrogen additions from Faidherbia trees and based on data from direct measurements using more recent investigative techniques.
Manufacturing Energy Calculations
The current study uses the values set out by the EPA (United States Environmental Protection Agency) (2017) to calculate the energy expended to manufacture urea. The range of values for the production of ammonia was 30.9–39.1 GJ/ ton of ammonia depending on the age and practices of the manufacturing facility. The current study used the average of this range, 35.0 GJ/ton of ammonia as representative on the recommendation of one of the EPA study's authors (EPA (United States Environmental Protection Agency), 2017). The same EPA (United States Environmental Protection Agency) (2017) study provided a value of 6.0 GJ per ton of urea produced that is used in the current study. Earlier studies of energy consumption for ammonia production cited by Patzek (2005) found a value of 34.5 GJ/ton for modern NH3 production, and Patzek (2005) found values ranging from 35.6 to 58 GJ/ton reported in the pre-1986 literature with values as high as 65 GJ/ton reported in the literature of small pre-1969 plants. The energy required to produce urea, citing the values of 35.6 GJ/ton value for the production of ammonia and 7.5 GJ/ton for urea from the same source, is reported in the existing literature as 42 GJ/ton of urea. However, this assumes that on a per ton basis the energy expenditures are directly additive and does not account for the stoichiometric relationship that occurs in the actual consumption of ammonia in the urea manufacturing process.
The current study, however, accounts for this stoichiometric relationship by adjusting the energy consumed in urea production by 0.567 ton of ammonia, which is the ammonia consumed per ton of urea produced (Fertilizer Europe, 2000; EPA (United States Environmental Protection Agency), 2017). When this relationship is included in calculations based on the values presented by Patzek, the energy value for the ammonia used in the production of a ton of urea is 20.2 GJ/ton urea. When this 20.2 GH/ton urea is added to the 7.5 GJ/ton urea used in the manufacturing process, this study obtains a value of 27.7 GJ/ton urea manufactured. This calculated value is much closer to the value of 25.49 GJ/ton derived from the values reported by EPA (United States Environmental Protection Agency) (2017).
Transportation Energy Calculations
The values used in this study to calculate the energy used in transporting urea fertilizer are not identical to those found in other sources. Standard values for energy consumption for freight transport were reported by Deutsche Bahn (2017). For truck freight energy consumption, the value of 1.31 MJ/ton-km closely matches the value of 1.33 MJ/ton-km published by Simonsen and Walnum (2011) but both are far below the >3.5 MJ/ton-km for Africa found in the International Energy Agency (IEA) (2009). The International Energy Agency (IEA) (2009) value was specific to Africa and it was used in the current study.
The value for ocean freight provided by Deutsche Bahn was 0.09 MJ/ton-km and substantially lower than the 0.172 MJ/ton-km published by Simonsen and Walnum (2011). Values reported in Gleick et al. (2012) are substantially higher than either of these values, and Gleick cites DOE and Natural Resources Canada with values of 0.37 MJ/ton-km for ocean freight transport and 3.5 MJ/ton-km for heavy truck transport (also consistent with the International Energy Agency (IEA) (2009). In this study we use the values of Simonsen and Walnum (2011) because they are more conservative than those cited by Gleick and likely to underestimate, not overestimate, the equivalent energy input benefit to the farming system. Further, these values were selected over the more conservative values of Deutsche Bahn because sourcing from Simonsen and Walnum includes explicit external peer review. Evidently further research on this energy expenditure will be needed in the future, focused specifically on the transportation of urea fertilizer and specifying both the typical type and displacement of the ship transport and the likely energy expenditure of the typical trucking journey from the port of Djibouti to Addis Ababa.
Energy Analysis of the Fertilizer Contribution to the Farming System
One of the factors that separates agriculture in the developing world from agriculture in the industrialized world is the difference in energy inputted to the farming systems and the crop yields, per unit of energy invested, from each system. Using corn as a comparison crop, Pimental (2009) found that corn production in India and Indonesia had an energy input to output ratio of 1:1.08 but corn production in the United States had an energy input to output ratio of 1:4.11 with a corresponding 5-fold increase in crop yield. The energy input, occurring through mechanization and the very large application of fertilizers in the United States, is responsible for much of this massive increase in crop yield (Warren, 1998). In the energetics of this study, part of the increase in yield comes from what is essentially an increase in efficiency, where energy in the form of animal traction and human labor that is less productive in cultivating and harvesting, is substituted for by more productive mechanical and fossil fuel energy, in the United States. However, with respect to nutrient inputs, the energy contribution of nitrogen virtually doubles between the two systems, with the energy value of nitrogen in India and Indonesia reported as 1,200 kCal × 1,000 ha−1and as 2,480 kCal × 1,000 ha−1for the United States (Pimental, 2009). This is an energy input increase achieved through significant additions of fossil fuel, in the form of natural gas, used to produce nitrogen fertilizer.
While a corresponding input of fertilizer energy would probably also increase yields in the developing world, there are several obstacles to realizing this benefit. One such obstacle is the lack of farmer financial resources, or sometimes shortages of foreign exchange on a national level, to buy fertilizer. This is compounded by the logistical difficulty in transporting and distributing fertilizer to the small farmers who need it. In addition, the heavy reliance of nitrogen fertilizer production on fossil fuel, which is a primary energy input into this production system, means that there will be significant emissions of greenhouse gases, which have negative consequences for the earth's climate. Given the energetics of breaking the triple atomic bond of diatomic Nitrogen, it is currently not cost-effective to use renewable energy, and not natural gas, in the nitrogen fertilizer production system (Pimental, 2009).
Nevertheless, the agricultural benefits to be gained from adding nitrogen fertilization are so substantial that an alternative method of bringing this energy into the Ethiopian agricultural system would be quite useful. This study shows that incorporating F. albida into the Ethiopian farming systems can provide this energy input in a manner that is effective and environmentally beneficial, from a climate change point of view. Due to its reverse phenology, Faidherbia is out of phase with the planted crops, so is able to harvest what would otherwise be agriculturally unused photosynthetically active radiation incident from the cropping area during the unplanted dry season and use parts of this energy to fix nitrogen from the atmosphere. This occurs as Faidherbia provides part of the resulting photosynthate to the symbiotic bacteria in the roots. These roots then break the energy-intensive nitrogen triple bond in ammonia, without using fossil fuels and provide the resulting nitrogen to the tree. The tree is not unique in this ability to obtain nitrogen from the atmosphere but is unique in that it can take advantage of this nitrogen fixation in areas where alternatives such as “green manure,” or nitrogen-fixing crops would be infeasible because these methods can provide nitrogen by depleting the soil of moisture and sacrificing an entire cropping cycle. F. albida trees, conversely, are able to expand the net quantity of energy entering the system by converting it into a usable form, rather than letting it escape from the system as radiant heat. This increase in energy input is equivalent to the additional energy of fertilizer that is often made available through large investment of fossil fuel, but without the expense or climate consequences of fossil fuel consumption.
A process of adding energy to the agricultural system through the addition of nitrogen via biological nitrogen fixation, through the integration of F. albida into the agricultural system, could increase yields in large areas of Africa (and elsewhere) in the developing world but without the need to develop corresponding manufacturing and transportation infrastructure to support this increase and without the corresponding greenhouse gas emissions. This increase might also be enhanced by the development of other components of the agricultural system, such as the development of locally adapted, dryland crop varieties that could withstand the restricted quantity and variability of rainfall, while taking advantage of the increased nutrient availability due to introduction of this agroforestry system. The combination of more productive and adaptive varieties could be further enhanced by the adoption of water capture and soil conservation methods (Reij et al., 2009a), as well as highly targeted, locally-appropriate fertilizer application technologies for other limiting nutrients, once the fundamental nutrient base for crop production has been provided, by the Faidherbia trees. In these ways there is the potential for F. albida to provide the energy/nutrient foundation for a marked, discontinuous increase in crop yields.
The Energy Substitution of Faidherbia Produces Additional Benefits Beyond N Fertilization
In addition to the avoided emissions, there are significant differences between the nitrogen energy expenditures that provide manufactured nitrogen fertilizer to the agricultural system and those used by F. albida to add nitrogen to the system. The nitrogen from Faidherbia enters the agricultural system when the trees lose their leaves at the beginning of the rainy season and the decomposing leaves deposit both the fixed nitrogen that was used to grow the leaves and the leaves' carbon component into the soil, leading to increases in soil organic matter in proximity to the tree (Hadgu et al., 2009; Sileshi, 2016). This increase in soil organic matter has significant implications for crop productivity, agricultural system food security and climate change adaptation. The net increase in organic matter, especially in agricultural systems from which it has been depleted, both greatly enhances the effectiveness of added nitrogen fertilizer (Marenya and Barret, 2009) and improves other soil properties, such as plant available water and cation exchange capacity. The increase in soil organic matter also favors increased plant productivity and crop resistance to drought (Bot and Benites, 2005), both of which are critical components to maintaining sustainable yields for food security and to facilitating adaptation to climate change, which is projected to result in greater variability in rainfall and increased instances of drought (Kandji et al., 2006).
The energy input of the Faidherbia tree thus provides multiple system-wide benefits3 beyond the addition of nitrogen that cannot be matched by the equivalent energy input provided by chemical nitrogen fertilizer. These additional systemic benefits are more difficult to quantify than the fertilizer energy use equivalence but should be considered when assessing the potential of F. albida to improve agricultural systems in the context of rural development.
The Fertilizer Substitution of Faidherbia Provides Climate Change Mitigation Benefits
As illustrated in Equations (8)–(12), above, there are significant avoided carbon dioxide emissions associated with using nitrogen provided by Faidherbia instead of nitrogen from manufactured urea. This study calculates avoided CO2 emissions of 0.116 tons per hectare per year. Where large-scale integration of Faidherbia takes place as a strategy for agricultural development, this contribution to climate change mitigation could be significant. For example, the late Prime Minister Meles Zenawi of Ethiopia announced at the Durban Climate Change Convention in December 2011, that the Ethiopian government would work to establish a 100 million F. albida trees Ethiopia through engagement with smallholder agriculture (https://allafrica.com/stories/201211190086.html, site accessed 8/15/2018). Achieving this level of integration would result in real contributions to Ethiopia's pledge in its Nationally Determined Contribution to reduce emissions by 64% below business-as-usual projections by 2030. Such a contribution would be significant in both ensuring agricultural productivity continues to rise to meet a growing human population and in showing that climate mitigation can also support the achievement other national development benefits.
The increasing yields achieved in industrialized agriculture have come at a price of increased emissions of greenhouse gases, especially CO2 and methane (CH4), making agriculture a major contributor to climate change [IPCC (Intergovernmental Panel on Climate Change), 2014]. Nitrogen fixation by human chemical means has now altered the global nitrogen cycle (Steffen et al., 2005). At the same time, there is a need to increase agricultural productivity to feed the growing human population in an environmentally sustainable way in part by closing the yield gap, between the potential productivity of the agricultural system and its current productivity (Foley et al., 2011). F. albida can help accomplish these dual goals, as it provides nitrogen fertilization, without any additions of heat-trapping gases to the atmosphere. As such, F. albida provides an alternative development pathway to closing of the yield gap, while having a positive effect on the climate. As this closing would otherwise need to be accomplished, in part, by the addition of fossil fuel produced nitrogen fertilizer, the contribution of Faidherbia effectively substitutes for the chemical fertilizer input (Umar et al., 2013). Thus, the emissions associated with the manufacture and transportation of the fertilizer to the point of application can legitimately be counted as an avoided emission that helps mitigate climate change in combination with the carbon sequestered in the trees' biomass and in the increases in soil organic matter associated in the trees' areas of influence. Indeed, increases in carbon sequestered above and below ground in Evergreen Agriculture systems that include Faidherbia, or other tree species that provide a fertilizer benefit, can be as much as an order of magnitude greater than that of conservation farming alone (Garrity et al., 2010).
Faidherbia also sequesters carbon in its biomass and increases the levels of soil organic matter within its area of influence. These benefits of sequestration are fundamentally different from avoided emissions, as carbon sequestered in soil is released if the tree is cut or burned or if the soil brought under more intensive tillage. Conversely, the avoided emissions resulting from fertilizer substitution are permanent, as they are associated with a specific fertilizer application in a specific cropping cycle in a particular year. They are thus equivalent to avoided emissions on par with an increase in energy efficiency at a factory that reduces emissions in a given year. As such any tradable carbon credits associated with the trees' avoided emissions should be considered as permanent, fully fungible credits and thus different from other land use credits.
Case Studies in Niger and Zambia
Farmer Managed Natural Regeneration (FMNR)4, is a highly successful approach to the regeneration of degraded land and to generating productivity increases centered on the cultivation of trees in small-holder agricultural systems (Reij et al., 2009a). The set of methods was introduced and promoted in Niger in the 1970s and 1980s with key adoption occurring during the drought of 1983–1984 (Reij et al., 2009b). By 2009 the methods had spread widely in areas of Niger, largely by spontaneous adoption among farmers and communities. Since F. albida is a key species used in this approach, the area of FMNR on a landscape can provide some insight into the potential scale that could be achieved through the integration of F. albida into smallholder agricultural landscapes. Based on the analysis of high-resolution, remote sensing imagery for the Maradi-Zinder region of Niger, Reij et al. (2009a) estimate that FMNR was practiced over 4,828,500 ha. Using this figure as a starting point in a thought experiment, it is assumed, conservatively, that Faidherbia is present in half of this area and that the Faidherbia tree cover is half of that of the Yengwe et al. (2018) study. The energy for transportation will likely be greater to this area than for typical areas of Ethiopia, but for purposes of this thought experiment, the same value is used to produce a conservative estimate. Based on the calculations suggested in the current study, F. albida covering half the FMNR land used in Niger would result in an annual injection of energy equal to 4.2 million GJ and 0.28 million tons of avoided CO2 emissions.
The avoided emissions are at a significant scale such that they could help Niger fulfill the pledges in its Nationally Determined Contribution plan to both reduce GHG emissions by at least 3.5% by 2030 and to scale up sustainable land management practices over all agroecological areas5 (INDC, Niger, 2015). At the scale of adoption of F. albida that is already occurring in large areas of the Sahel and elsewhere in Sub-Saharan Africa, it seems reasonable to propose that the avoided emissions produced by the trees could also potentially make contributions to the climate mitigation efforts of some countries including, Ethiopia.
Similarly, the Zambian Conservation Farming Unit (CFU) estimates that F. albida is now cultivated on 300,000 ha of farmland with recommended planting rates likely providing full canopy cover (Garrity et al., 2010). As in the Niger example, the energy for transportation will likely be greater to this area than for Ethiopia, but to be consistent, the same value is used to produce a conservative estimate. Based on the current study figures, the energy input of the F. albida trees cultivated on the 300,000 ha in Zambia is estimated in this thought experiment to be 1.04 million GJ per year and the avoided emissions to be 50,400 tons of CO2 per year.
Further, the Faidherbia nitrogen is estimated to substitute for 34,200 tons of manufactured urea annually. A full economic analysis of this substitution is beyond the scope of the current study, however a simple price comparison is provided to show the possible scale of the monetary value of the substitution. It is assumed that Faidherbia nitrogen is directly interconvertible with manufactured urea nitrogen and that Faidherbia nitrogen substitutes directly for urea nitrogen. The price is for urea as purchased in bulk from a distributor and does not include transportation, handling, or application costs. The addition of these costs could potentially raise the value of the substitution. At a price urea fertilizer of $2006 per ton of urea fertilizer the Faidherbia leads to savings of $6.8 million USD in fertilizer that would not need to be purchased.
The Contribution of Faidherbia albida Would Also Have Multiple Economic Benefits
Finally, it is important to note that adding nitrogen from F. albida is likely to have multiple economic benefits, some of which are specific to integrating trees into the agricultural system. In addition to the positive impacts on crop productivity, food security, and decreases in vulnerability to drought impacts that are discussed throughout this study, F. albida provides tangible financial savings to the farmers, governments, and other stakeholders in the economic system. Governments in Sub-Saharan Africa sometimes subsidize manufactured fertilizer, so procuring the nitrogen fertilizer by cultivating F. albida instead of from imported manufactured urea would reduce government's foreign exchange expenditures. The use of the trees would also avoid an unstable economic dynamic in which farmers are dependent on government subsidies to use nitrogen fertilizers to a degree such that the curtailment of said subsidies would lead to abrupt drop in fertilizer use, crop productivity, and potentially, a severe economic shock. By contrast, Faidherbia trees are long lived, provide a consistent and reliable source of nutrients, and are decoupled from both the world price of fertilizer and the economic policies of the government. Malawi has adopted policies that reflect the benefits of substituting tree-based sources of nitrogen for subsidized chemical fertilizer (Garrity et al., 2010) and other governments in the region may also recognize these benefits.
Relevance and Applicability to Other Areas in Africa
The native range of Faidherbia in Africa extends over vast areas of Africa (Figure 3) and includes over 20 African countries. In addition to its current application in Ethiopia (Hadgu et al., 2009), Faidherbia has demonstrated potential to contribute to agriculture in Zambia (Yengwe et al., 2017), Malawi (Beedy et al., 2016), Niger (Kho et al., 2001), and Burkina Faso (Reij et al., 2009b). The benefits of F. albida for crop production include the addition of nitrogen to the soil (Yengwe et al., 2017), increasing soil organic matter (Kamara and Haque, 1992), and improving crop micro-climate (Sida et al., 2017). These benefits are associated with the tree species and are not specific to a particular crop. It is very likely that the potential beneficial effects of the tree are available for many crops. Yield increases associated with Faidherbia have been reported for millet, maize, sorghum, teff grown with wheat, sunflower (Barnes and Fagg, 2003), wheat alone (Sida et al., 2018), and barely (Hadgu et al., 2009). All of these crops have benefited when grown in association with Faidherbia and their production systems have received an energy input. To the extent that Faidherbia nitrogen inputs substitute for manufactured fertilizer these systems can also provide quantified avoided emissions as a climate change benefit and where they are implemented at scale could contribute to African countries meeting their Nationally Determined Contributions under the international climate accords. The methods described here and associated with Faidherbia agroforestry have wide application beyond Ethiopia in many countries and landscapes.
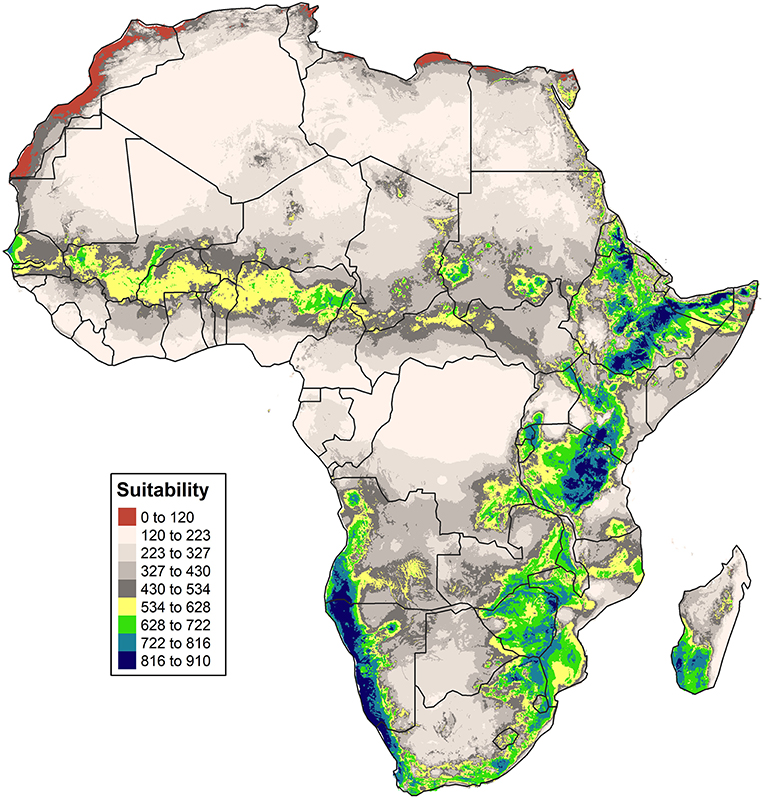
Figure 3. Estimated distribution of Faidherbia albida in Africa, obtained by ensemble suitability modeling with BiodiversityR (Kindt, 2018). In the map, the green-blue colors show where the species is expected to be suitable, the gray areas where the species is not suitable and the orange areas where the species was predicted to be suitable but where those latter areas were outside a buffer around the known presence observations. Suitability values above 534 correspond to likely presence of the species with increasing values associated with higher likelihood of species presence. The suitability index is unitless can be understood as the probability of the species occurring at a given location (de Sousa et al., 2019). Country boundaries were obtained from the tmap package [data(World), (Tennekes, 2018; de Sousa et al., 2019)].
Large Scale Impact Scenarios
To illustrate the potential large-scale contribution of the avoided emissions provided by F. albida in an Ethiopian context, the following scenarios are provided. It is well understood that this is an illustrative exercise and that actual implementation would be far more complex and could produce different results. The aggregated suitability analysis which integrates the environmental factors determining the range of Faidherbia indicates that very large areas of Ethiopia are highly suitable for Faidherbia adoption (Figure 3), other factors that could influence adoption include the presence of existing viable rootstock and potential policy interventions such as provision of seedlings, extension support and secure land tenure. However, while taking the uncertainty of these factors into account, this analysis does provide an initial comparison, at scale, of selection between policy alternatives that include large-scale adoption of F. albida agroforestry on uncultivated and unproductive lands, no action or intervention to improve productivity on uncultivated and unproductive lands, and the addition of an equivalent amount of nitrogen in the form of urea fertilizer to increase productivity on uncultivated and unproductive lands. F. albida has been a key part of the Farmer Managed Natural Regeneration (FMNR) approach which has been adopted over millions of hectares of Niger and Burkina Faso and has been successful in reversing land degradation and rehabilitating barren agricultural landscapes, bringing them to high levels of agricultural production (Reij et al., 2009b).
In the first scenario F. albida is used to fully transform that land in Ethiopia which has been degraded or is currently unproductive, into productive agricultural land, following some aspects of the Sahelian FMNR model including cultivation from existing rootstock and cultivation from seedlings. As of 2013 unproductive land in Ethiopia accounted for 12,457,975 ha, while for uncultivated land accounted for 1,400,565 ha (Table 2, Figure 4).
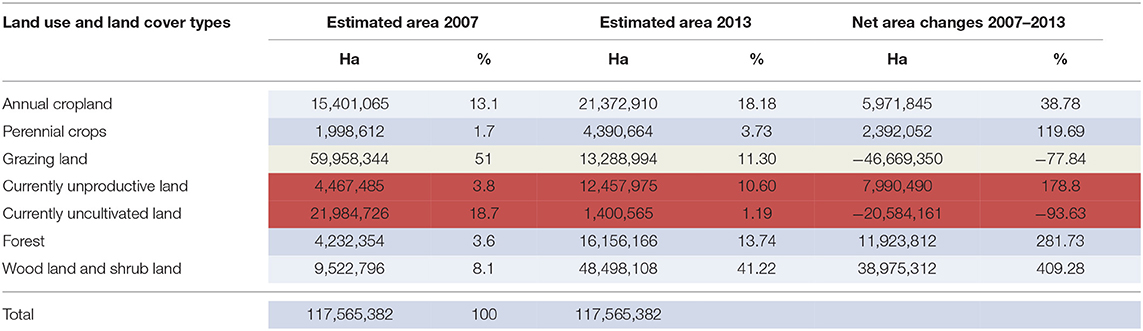
Table 2. Estimated area and net area change of land use land cover types (Simane Personal Communication, 2019).
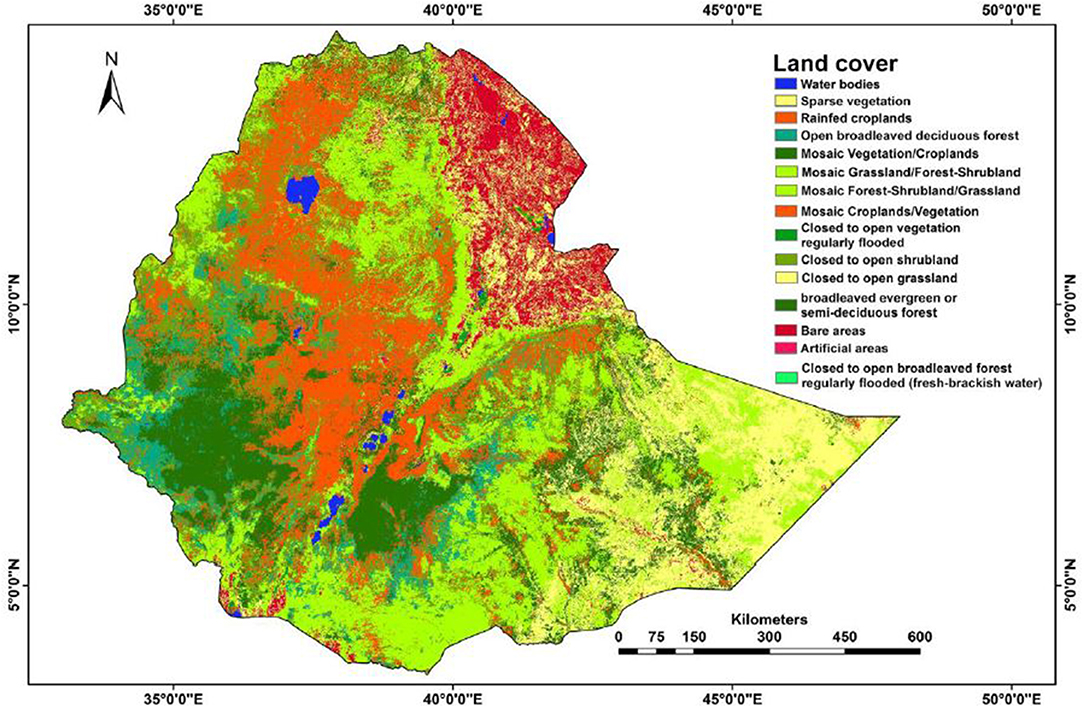
Figure 4. Landcover map of Ethiopia (FAO, 2009 cited in Hailu et al., 2015).
The area available for F. albida agroforestry crop production, without changing practices on land currently in production, was the sum of the unproductive and uncultivated land, 13,858,540 ha. Based on the value of 0.116 t CO2e/ha/yr, if this area was converted to F. albida based production, the avoided emissions would be 1.6 Mt CO2e per year or 104 Mt CO2e over the 65-year productive life of the trees. The Intended National Determined Contribution (INDC) plan presented by Ethiopia, specifies emissions reductions for all major sectors of the economy. The proposed annual emission reduction for the building sector is 5 Mt CO2e per year. In this scenario avoided emissions gained from F. albida agroforestry would be equivalent to achieving ~32% of this goal.
For comparison two business—as—usual scenarios are presented. In the first business—as—usual scenario there is no cultivation or promotion of Faidherbia and no effort to bring uncultivated or unproductive lands into cultivation. This would mean forgoing the Faidherbia mediated productive capacity of this land area, which could be substantial. Sida et al. (2018) reports average wheat yield under Faidherbia in the rift valley of Ethiopia, as 6.7 t/ha, yield levels of this kind, over the scenario area, could mean a foregoing access to 94 Mt of wheat annually. Alternatively, in the second business as usual scenario, if the scenario area was brought under cultivation using additions of urea fertilizer this would mean additional emissions of 1.6 Mt CO2. As this would be a net addition to existing emissions levels, under this business—as—usual scenario Ethiopia would have to decrease emissions by 3.2 Mt CO2e per year to achieve the same levels of emissions produced by the 1.6 Mt CO2e avoided emissions provided by the first Faidherbia scenario. It should be noted that in the chemical fertilizer scenario, the organic matter additions provided by Faidherbia do not occur. On the weathered soils of Africa this is a critical deficiency because the without organic matter in the soil the yield increases obtained by the addition of chemical fertilizer cannot be maintained and yields begin to fall off despite ongoing additions of chemical fertilizer (Pieri, 1992). Ultimately this business—as—usual scenario is most likely to produce declining yields without a climate benefit and with emissions that add to the reductions needed to attain the INDC.
A second scenario with a somewhat more conservative approach is as follows. Between 2007 and 2013 the estimated area of unproductive land went from 4,467,485 to 12,457,975 ha, a net increase of 7,990,490 ha. As productive land is at a premium, it is assumed that this increase in unproductive land in this brief period of time was due largely land degradation. The primary cause of land degradation is soil erosion driven by excessive cultivation using poor tillage practices, excessive livestock grazing and stocking rates, and deforestation (Taddese, 2001). If Faidherbia agroforestry were used to bring all of this land back into production the avoided emissions per year would be, again based on a value of 0.116 t CO2e/ha/yr, 0.93 Mt CO2e per year. This would equivalent to achieving ~18% of the emissions reductions proposed for the building sector in Ethiopia's INDC. As with the scenario covering both unproductive and uncultivated land, there are two corresponding business—as—usual scenarios for this area. The first business as usual scenario involves leaving the land in an unproductive state and forgoes the potential productivity achieved through Faidherbia agroforestry. In this instance that could include substantial harvests of wheat, again at the level of 6.7 t/ha and forgoing 53 Mt of wheat annually. In the second business—as—usual scenario the nitrogen benefit of Faidherbia is replaced by equivalent additions of urea. As in the large area scenario, this would be a net addition to emissions and would require net emission reductions of 1.9 Mt CO2e to achieve the same level of emissions as that provided by the 0.93 Mt CO2e contributed by the Faidherbia scenario. Ultimately, as with the large area scenario, fertilizer additions in the absence of soil organic matter maintenance will result in declining yields while emissions remain constant an increasing carbon footprint per unit of yield.
The same two scenarios can be used to calculate potential energy inputs to Ethiopian agriculture that Faidherbia could provide in in these areas. With this urea equivalent urea addition there is an input of 3.48 MJ per hectare per year. For the first scenario with 13,858,540 ha, that combines uncultivated and unproductive land, this is equivalent to an input of 48,227,719 GJ/ha/yr. For the second scenario in which the net addition of unproductive land, 7,990,490, is brought under Faidherbia agroforestry, this is equivalent to an input of 27,806,905 GJ/ha/yr. These are equivalent to the energy in 1,645,548 and 948,782 tons of coal, respectively (National Research Council, 2007). Under business—as—usual, if no equivalent urea fertilizer additions are made there is no energy addition made to the agricultural system and no corresponding increase in productivity. In the scenario where an equivalent addition of urea is made without maintaining soil organic matter, there is an initial increase in productivity for an equivalent input of energy to the agricultural system, however over time as yields decline the energy use efficiency, that is, the yield increase per unit of energy input decreases.
Conclusion
F. albida's reverse phenology enables it to provide nitrogen nutrition and soil organic matter additions to farming systems that function as a significant contribution of useable energy to these same systems. The trees' energy input also provides a vehicle to ramp up agricultural productivity, similar to the way in which agriculture productivity increased in the industrialized world through increased energy inputs. In Ethiopia, this additional energy benefit occurs as the trees' decomposing leaves add nitrogen equivalent to the nitrogen contained in a substantial quantity of urea fertilizer and increase water availability to the crops. The development benefits, in terms of increased agricultural productivity and adaptation to climate change, are substantial. Additionally, the trees use solar radiation as their energy input, and this solar energy displaces the natural gas used to both manufacture urea and the fossil fuels used to transport the manufactured fertilizer to the point of use. The trees thus lead to substantial avoided greenhouse gas emissions, meaning that if F. albida trees were cultivated over large areas their avoided emissions could make a significant annual contribution to national efforts to mitigate climate change.
Author Contributions
JH developed the original concepts, elaborated the concepts, performed a literature review, performed the mathematical calculations, wrote language included in the article, and found references supporting the research. BS provided discussion and important analysis of the concepts, provided information from the field that ensured the rigor and correctness of the work, wrote language included in the article, and found references supporting the research. CS provided discussion and important analysis of the concepts, wrote language included in the article, and found references supporting the research.
Funding
This research was support by National Science Foundation award BCS-1639214.
Conflict of Interest
The authors declare that the research was conducted in the absence of any commercial or financial relationships that could be construed as a potential conflict of interest.
Acknowledgments
Benjamin Zaitchik whose encouragement and support helped in execution of the research and the preparation of the manuscript. Roeland Kindt who helped us to access the most current understanding of the range and extent of Faidherbia albida.
Irving Mintzer whose comments and suggestion were a great help in refining and preparing the manuscript.
John Byrne whose feedback and suggestions from the energy point of view were very helpful in putting the work into perspective.
The National Science Foundation whose financial support made this research and scientific collaboration possible.
Footnotes
1. ^Sileshi (2016) proposes that the phosphorus is provided beneath the tree canopy in part by deep capture and recycling of nutrients by Faidherbia albida.
2. ^PubChem at https://pubchem.ncbi.nlm.nih.gov/compound/urea#section=Top
3. ^By increasing the soil organic matter, the tree provides multiple benefits to the cropping system including, increased plant available water, increased fertilizer use efficiency, increased water use efficiency, increased cation exchange capacity, and improved ease of cultivation.
4. ^See Reij et al. (2009a) for a more complete description of the method, adoption and impact of Farmer Managed Natural Regeneration.
5. ^Agriculture Forestry and Other Land Use (AFOLU) is a priority sector for climate mitigation under the Intended Nationally Determined Contribution plan of Niger (INDC, Niger, 2015).
6. ^International Raw Materials Ltd. reported a global granular urea price of $263 per metric ton on July 26, 2018. https://www.irmteam.com/
References
Abdulkadi, B., Kassa, S., Desalegn, T., Tadesse, K., Haileselassie, M., Fana, G., et al. (2017). “Crop response to fertilizer application in Ethiopia: a review,” in A Review of Soil Fertility Management and Crop Response to Fertilizer Application in Ethiopia: Towards Development of Site- and Context-Specific Fertilizer Recommendation, CIAT Publication No. 443 (Cali: Center for International Tropical Agriulture), 2–86.
Bakker, C., Zaitchik, B. F., Siddiqui, S., Hobbs, B. F., Broaddus, E., Neff, R. A., et al. (2018). Shocks, seasonality, and disaggregation: modelling food security through the integration of agricultural, transportation, and economic systems. Agric. Syst. 164, 165–184. doi: 10.1016/j.agsy.2018.04.005
Barnes, R., and Fagg, C. (2003). Faidherbia Albida: Mongraph and Annotated Bibliography, Tropical Forestry Papers. Oxford, UK: Oxford Forestry Institute.
Beedy, T. L., Chanyenga, T. F., Akinnifesi, F. K., Sileshi, G. W., Nyoka, B. I., and Gebrekirstos, A. (2016). Allometric equations for estimating above-ground biomass and carbon stock in Faidherbia albida under contrasting management in Malawi. Agroforestry Syst. 90, 1061–1076. doi: 10.1007/s10457-015-9883-x
Boffa, J.-M. (1999). Agroforestry Parklands in Sub-Saharan Africa, FAO Conservation Guide. Rome: Food and Agriculture Organization of the United Nations.
Bot, A., and Benites, J. (2005). The Importance of Soil Organic Matter: Key to Drought-Resistant Soil and Sustained Food Production, FAO Soils Bulletin. Rome: Food and Agriculture Organization of the United Nations.
CTFT (Centre Technique Forestier Tropical) (1989). Faidherbia albida (Del.) A. Chev. (Synonym Acacia albida el.): A Monograph. Nogent-Sur-Marne: Centre Technique Forestier Tropical and Technical Centre for Agricultural and Rural Cooperation.
Dancette, C., and Poulain, J. F. (1969). Influence of Acacia albida on pedoclimatic factors and crop yields. Afr. Soils 14, 143–184.
de Sousa, K., van Zonneveld, M., Holmgren, M., Kindt, R., and Ordoñez, J. C. (2019). The future of coffee and cocoa agroforestry in a warmer Mesoamerica. Sci Rep. 9:8828. doi: 10.1038/s41598-019-45491-7
Deutsche Bahn (2017). Table: “Specific Primary Energy Consumption by Trips and Transports of DB Group.” Available online at: https://www.deutschebahn.com/en/group/ataglance/facts_figures-1776344 (accessed July 20, 2018).
Edwards, R., Mahieu, V., Griesemann, J.-C., Larivé, J.-F., and Rickeard, D. J. (2004). Well-to-Wheels Analysis of Future Automotive Fuels and Powertrains in the European Cntext. Burssels: European Commission Joint Research Centre.
EPA (United States Environmental Protection Agency) (2017). Energy Efficiency and Cost Saving Opportunities for Ammonia and Nitrogenous Fertilizer Production, eds K. Kermeli, E. Worrell, G. Wina, and M. Corsten. Document Number 430-R-17002.
FAO (2009). Land Cover of Ethiopia-Globcover Regional. Rome. Available online at: http://data.fao.org/ (accessed July, 2014).
Fertilizer Europe (2000). Best Available Techniques for Pollution Prevention and Control in the European Fertilizer Industry. Booklet No. 5 of 8: Production of Urea and Urea Ammonium Nitrate, 2000 Edn. (Brussells: Fertilizer Europe).
Foley, J., Ramankutty, N., Brauman, K. A., Cassidy, E. S., Gerber, J. S., Johnston, M., et al. (2011). Solutions for a cultivated planet. Nature 478, 337–342. doi: 10.1038/nature10452
Garrity, D. P., Akinnifesi, F. K., Ajayi, O. C., Weldesemayat, S. G., Mowo, J. G., Kalinganire, A., et al. (2010). Evergreen agriculture: a robust approach to sustainable food security in Africa. Food Secur. 2, 197–214. doi: 10.1007/s12571-010-0070-7
Gleick, P. H., Allen, L., Christian-Smith, J., Cohen, M. J., Heberger, M., Morrison, J., et al. (2012). The World's Water Volume 7: The Biennial Report on Freshwater Resources. Washington, DC: Island Press.
Hadgu, K. M., Kooistra, L., Rossing, W. A. H., and van Bruggen, A. H. C. (2009). Assessing the effect of Faidherbia albida based land use systems on barley yield at field and regional scale in the highlands of Tigray, Northern Ethiopia. Food Secur. 1, 337–350. doi: 10.1007/s12571-009-0030-2
Hadgu, K. M., Mowo, J., Garrity, D. P., and Sileshi, G. (2011). Current extent of evergreen agriculture and prospects for improving food security and environmental resilience in Ethiopia. Int. J. Agric. Sci. 1:6.
Haileslassie, A., Priess, J., Veldkamp, E., Teketay, D., and Lesschen, J. P. (2005). Assessment of soil nutrient depletion and its spatial variability on smallholders' mixed farming systems in Ethiopia using partial versus full nutrient balances. Agric. Ecosyst. Environ. 108, 1–16. doi: 10.1016/j.agee.2004.12.010
Hailu, B. T., Maeda, E. E., Heiskanen, J., and Pellikka, P. (2015). Reconstructing pre-agricultural expansion vegetation cover of Ethiopia. Appl. Geogr. 62, 357–365. doi: 10.1016/j.apgeog.2015.05.013
IFDC (International Fertilizer Development Center) (2012). Ethiopia Fertilizer Assessment. Muscle Shoals, AL: International Fertilizer Development Center; The African Fertilizer and Agribusiness Partnership.
IFDC/AFAP (International Fertilizer Development Center/African Fertilizer Agribusiness Partnership) (2015). Source Cited in the Preparation of “African Fertilizer Map,” ed A. Harrison. Muscle Shoals, AL: Saudi Arabian Mining Company and International Fertilizer Association; African Fertilizer Agribusiness Partnership; International Fertilizer Development Center and AfricanFertilizer.org.
International Energy Agency (IEA) (2009). Transport Energy and CO2: Moving Towards Sustainability. OECD.
IPCC (Intergovernmental Panel on Climate Change) (2014). “Working group III contribution to the fifth assessment report of the interngovernmental panel on climate change,” in Climate Change 2014: Mitigation of Climate Change, eds O. Edenhofer, R. Pichs-Madruga, and Y. Skona (New York, NY: Cambridge University Press), 111–1248.
Kamara, C. S., and Haque, I. (1992). Faidherbia albida and its effects on Ethiopian highland Vertisols. Agroforestry Syst. 18, 17–29. doi: 10.1007/BF00114814
Kandji, S. T., Verchot, L., and Mackensen, J. (2006). Climate Change and Variability in the Sahel Region: United Nations Environment Program. Nairobi: World Agroforestry Centre.
Kho, R. M., Yacouba, B., Yayé, M., Katkoré, B., Moussa, A., Iktam, A., et al. (2001). Separating the effects of trees on crops: the case of Faidherbia albida and millet in Niger. Agroforestry Syst. 52, 219–238. doi: 10.1023/A:1011820412140
Kindt, R. (2018). Ensemble species distribution modelling with transformed suitability values. Environ. Model. Softw. 100, 136–145. doi: 10.1016/j.envsoft.2017.11.009
Leck, H., Conway, D., Bradshaw, M., and Rees, J. (2015). Tracing the water-energy-food nexus: description, theory and practice: tracing the water-energy-food nexus. Geogr. Compass 9, 445–460. doi: 10.1111/gec3.12222
Marenya, P. P., and Barret, C. P. (2009). State-conditional fertilizer yield response on western Kenyan farms. Am. J. Agric. Econ. 91, 991–1006 doi: 10.1111/j.1467-8276.2009.01313.x
McKinnon, A., and Piecyk, M. (2010). Measuring and Managing CO2 Emissions in European Chemical Transport. Brussels: Cefic. Available online at: https://cefic.org/app/uploads/2018/12/MeasuringAndManagingCO2EmissionOfEuropeanTransport-McKinnon-24.01.2011-REPORT_TRANSPORT_AND_LOGISTICS.pdf
National Research Council (2007). Coal: Research and Development to Support National Energy Policy. Washington, DC: The National Academies Press.
Niger (2015). Intended Nationally Determined Contribution (INDC). Document Submitted to the Registry of Nationally Determined Contribution Documents of the United Nations Framework Convention on Climate Change. Available online at: http://www4.unfccc.int/ndcregistry/Pages/All.aspx (accessed July 25, 2018)
Patzek, T.W. (2005). Thermodynamics of the corn-ethanol biofuel cycle. Crit. Rev. Plant Sci. 23, 519–567. doi: 10.1080/07352680490886905
Pieri, C. J. M. G. (1992). “Items in the organic matter balance of cultivated soil and their evolution,” in Fertility of Soils: A Future for Farming in the West African Savannah, Springer Series in Physical Environment (Berlin: Springer-Verlag), 216–221.
Pimental, D. (2009). Energy inputs in food crop production in developing and developed nations. Energies 2, 1–24. doi: 10.3390/en20100001
Reij, C., Tappan, G., and Smale, M. (2009a). Agroenvironmental transformation in the sahel: another kind of “Green Revolution,” in IFPRI Discussion Paper 00914 (Washington, DC: International Food Policy Research Institue).
Reij, C., Tappan, G., and Smale, M. (2009b). Re-greening the Sahel: Farmer-Led Innovation in Burkina Faso and Niger. IFPRI [WWW Document]. Available online at: http://www.ifpri.org/publication/re-greening-sahel-farmer-led-innovation-burkina-faso-and-niger (accessed May 6, 2018).
Rhoades, C. (1995). Seasonal pattern of nitrogen mineralization and soil moisture beneath Faidherbia albida (syn Acacia albida) in central malawi. Agroforest Syst. 29, 133–145. doi: 10.1007/BF00704882
Saka, A. R., Bunderson, W. T., Itimu, O. A., Phombeya, H. S. K., and Mbekeani, Y. (1994). The effects of Acacia albida on soils and maize grain yields under smallholder farm conditions in Malawi. For. Ecol. Manag. 64, 217–230. doi: 10.1016/0378-1127(94)90296-8
Shitumbanuma, V. (2012). Analyses of Crop Trials Under Faidherbia albida. Results of Crop Yields for the 2010/2011 Agricultural Season and Overall Summary of Yields and Yield Trends From 2008 to 2011. Lusaka: Conservation Farming Unit; Department of Soil Science School of Agricultural Sciences University of Zambia. Available online at: https://conservationagriculture.org/uploads/pdf/CFU-FAIDHERBIA-CROP-TRIALS-Shitumbanuma-Sep-2012.pdf
Sida, T. S., Baudron, F., Kim, H., and Giller, K. E. (2018). Climate-smart agroforestry: Faidherbia albida trees buffer wheat against climatic extremes in the Central Rift Valley of Ethiopia. Agric. For. Meteorol. 248, 339–347. doi: 10.1016/j.agrformet.2017.10.013
Sileshi, G. W. (2016). The magnitude and spatial extent of influence of Faidherbia albida trees on soil properties and primary productivity in drylands. J. Arid Environ. 132, 1–14. doi: 10.1016/j.jaridenv.2016.03.002
Simonsen, M., and Walnum, H. J. (2011). Energy chain analysis of passenger car transport. Energies 4, 324–351 doi: 10.3390/en4020324
Steffen, W., Sanderson, A., Tyson, P. D., Jager, J., Matson, P.A., Morre, B. III, et al. (2005). Global Change and the Earth System: A Planet Under Pressure. Berlin: Springer-Verlag.
Taddese, G. (2001). Land degradation: a challenge to Ethiopia. Environ. Manage. 27, 815–824. doi: 10.1007/s002670010190
Umar, B. B., Aune, J., and Lungu, O. (2013). Effects of Faidherbia albida on the fertility of soil in smallholder conservation agriculture systems in eastern and southern Zambia. Afr. J. Agric. Res. 8, 173–183. doi: 10.5897/AJAR11.2464
Wahl, C. T., and Bland, W. L. (2013). Faidherbia albida on the Tonga Plateau of southern Zambia: an agroecological analysis. Int. J. Agric. Sustain. 11, 382–392. doi: 10.1080/14735903.2013.812607
Warren, G. F. (1998). Spectacular increases in crop yields in the United States in the twentieth century. Weed Technol. 12, 752–760. doi: 10.1017/S0890037X00044663
Yengwe, J., Amalia, O., Lungu, O. I., and De Neve, S. (2018). Quantifying nutrient deposition and yield levels of maize (Zea mays) under Faidherbia albida agroforestry system in Zambia. Eur. J. Agron. 99, 148–155. doi: 10.1016/j.eja.2018.07.004
Keywords: food energy water nexus, climate change adaptation and mitigation, Faidherbia albida, agroforestry, Ethiopia, evergreen agriculture, nitrogen fertilization, avoided emissions
Citation: Haskett JD, Simane B and Smith C (2019) Energy and Climate Change Mitigation Benefits of Faidherbia albida Agroforestry in Ethiopia. Front. Environ. Sci. 7:146. doi: 10.3389/fenvs.2019.00146
Received: 31 August 2018; Accepted: 17 September 2019;
Published: 01 November 2019.
Edited by:
Richard George Lawford, Morgan State University, United StatesReviewed by:
Xander Wang, University of Prince Edward Island, CanadaSushel Unninayar, Morgan State University, United States
Copyright © 2019 Haskett, Simane and Smith. This is an open-access article distributed under the terms of the Creative Commons Attribution License (CC BY). The use, distribution or reproduction in other forums is permitted, provided the original author(s) and the copyright owner(s) are credited and that the original publication in this journal is cited, in accordance with accepted academic practice. No use, distribution or reproduction is permitted which does not comply with these terms.
*Correspondence: Jonathan D. Haskett, Jhaskett314@gmail.com