- 1Climate Service Center Germany (GERICS), Helmholtz-Zentrum Geesthacht (HZG), Geesthacht, Germany
- 2Faculty of Sustainability, Leuphana University of Lüneburg, Lüneburg, Germany
- 3Centre for Hydrology, University of Saskatchewan, Saskatoon, SK, Canada
- 4Consejo Nacional de Investigaciones Científicas y Técnicas, Centro de Investigaciones del Mar y la Atmpósfera (CIMA), UMI-IFAECI (CNRS), Universidad de Buenos Aires, Buenos Aires, Argentina
- 5Universidad de Buenos Aires, Facultad de Ciencias Exactas y Naturales, Departamento de Ciencias de la Atmósfera y los Océanos, Buenos Aires, Argentina
- 6Tunisia Polytechnic School, University of Carthage, La Marsa, Tunisia
- 7Laboratory of Materials, Measurements and Applications, National Institute of Applied Sciences and Technology, University of Carthage, Tunis, Tunisia
- 8Marine Physics Department, National Institute for Water and Atmospheric Research (NIWA), Wellington, New Zealand
- 9School of Geography and the Environment, University of Oxford, Oxford, United Kingdom
- 10Climate Systems Analysis Group (CSAG), University of Cape Town, Cape Town, South Africa
- 11Centre Eau-Terre-Environnement, Institut National de la Recherche Scientifique (INRS), Quebec, QC, Canada
- 12R&D Observations and Data Technology, Royal Netherlands Meteorological Institute, De Bilt, Netherlands
- 13Network on Climate Change in Bangladesh (NCC, B), Dhaka, Bangladesh
- 14Department of Geoscience, University of Calgary, Calgary, AB, Canada
- 15School of Marine and Atmospheric Sciences, Stony Brook University, Stony Brook, NY, United States
- 16R&D Weather- and Climate models, Royal Netherlands Meteorological Institute, De Bilt, Netherlands
- 17Instituto Argentino de Nivología, Glaciología y Ciencias Ambientales (IANIGLA/CONICET), Mendoza, Argentina
- 18Center for Environmental Management and Control (CEMAC), University of Nigeria, Enugu, Nigeria
- 19Laboratory for Climatology and Remote Sensing, Faculty of Geography, Philipps University of Marburg, Marburg, Germany
- 20Institute for Climate and Atmospheric Science, University of Leeds, Leeds, United Kingdom
- 21Eurasia Institute of Earth Sciences, Istanbul Technical University, Istanbul, Turkey
This paper provides an early career researchers (ECRs) perspective on major challenges and opportunities that arise in the study and understanding of, and the provision of regional information for Climate, Weather and Hydrological (CWH) extreme events. This perspective emerged from the discussions of the early career 3-day Young Earth System Scientists - Young Hydrologic Society (YESS-YHS) workshop, which was conjointly held with the Global Energy and Water Exchanges (GEWEX) Open Science Conference. In this paper we discuss three possible ways forward in the field: a stronger interaction between Earth system scientists and users, a collaborative modeling approach between the different modeling communities, and an increased use of unconventional data sources in scientific studies. This paper also demonstrates the important role of ECRs in embracing the above outlined pathways and addressing the long-standing challenges in the field. YESS and YHS networks encourage the global community to support and strengthen their involvement with ECR communities to advance the field of interdisciplinary Earth system science in the upcoming years to decades.
1. Introduction
Future projections of climate change suggest that Climate, Weather, and Hydrological (CWH) extreme events will become more frequent and more intense (IPCC, 2012; Orlowsky and Seneviratne, 2012; Sillmann et al., 2013). These extremes are typically highly heterogeneous at local scales, with severe and uncertain impacts threatening regional ecosystems and human society. In this context, societal stakeholders are increasingly turning toward scientists to guide their regional-to-local decision-making processes, formulating new sets of questions that require novel approaches and answers. Access to reliable, accurate, timely, and understandable information related to extreme events is vital for the safety and resilience of society. In this regard, prediction and adaptation for extreme events requires regional-to-local information collection and sharing, advanced tools and enhanced understanding of the physical processes to underpin the decision making and planning process.
These pressing demands require the next generation of researchers to ensure the advancement of knowledge toward the provision of science-based regional-to-local CWH information. This paper outlines the main challenges and ways forward on the following three key points, building upon the Earth system science frontiers as defined by Rauser et al. (2017). Firstly, we outline the need to foster close interactions between scientists and users to improve the mutual understanding and consideration of objectives, challenges, and constraints. Secondly, we describe the need for projections/predictions across various spatial and temporal scales to deliver the required regional-to-local information about extreme CWH events. Finally, we assess the current state of observational data to support the understanding and prediction of extreme events, while simultaneously recognizing the opportunities and limitations of new technologies to collect Earth system science data.
The foundations of this paper were laid during discussions that took place at the 3-day YESS-YHS early career researcher (ECR) workshop, attended by 40 ECR from 23 countries, jointly held with the GEWEX Open Science Conference (Osman et al., 2018; van Oevelen, 2018). In the following sections, we summarize the main converged outcomes of the workshop discussions, consolidated by over half of the attendees, providing an ECR perspective on the main challenges and opportunities that arise in the study and understanding of CWH extreme events and the provision of adequate regional information for decision making.
2. Fostering Interactions
User engagement and knowledge exchange across the scientific-policy-practice communities is essential to deal with complex problems such as the impact of CWH extremes (Hering et al., 2014). The need for this interaction reposes on the importance of understanding how scientific knowledge is applied and used in policy and decision-making contexts (Lemos, 2015). Since large amounts of potentially useful CWH information is currently unused (Lemos et al., 2012; Street, 2015), it is clear that an improved information availability does not automatically lead to a better resilience management.
A classical way to make CWH information more useful and applicable for users and policy-makers is to take an impact-based approach (Hazeleger et al., 2015). For example, to create valuable information and engage societal stakeholders in adaptation and mitigation efforts, scientists can analyze past extreme events, identify their main physical and socio-economic drivers and impacts, and model their evolution under climate change. This impact-based approach can be used to inform users through an estimation of what the future holds, and to advance the scientific knowledge by revealing future changes in the event drivers and their interactions (Zscheischler and Zhang, 2018).
As ECRs, we believe that the interaction with users—policy-makers, government agencies and communities, among others—requires a sustainable iterative communication process toward the co-production of knowledge (Figure 1). This interaction between different stakeholders should pursue the understanding of the capabilities, needs and limitations of each other's field of expertise and the development of a common language. To enable this interaction, it is important that both scientists and users bring their knowledge to the table on an equal footing (Lemos and Morehouse, 2005). In this context, ECRs could take on the role of ‘trust-brokers’, serving as a trusted intermediary between the science and the user. ECRs may facilitate the collaboration between scientists and non-scientists in a more instinctive and cooperative manner, having a larger potential to bridge the gap between different knowledge systems and disciplines. ECRs could play a particularly valuable role, as they bring fresh perspectives and high levels of enthusiasm, both of which are crucial assets to science-policy-practitioner collaborations. Furthermore, many ECRs receive extensive interdisciplinary training and might therefore see the broader context of the science-policy-user nexus. To stimulate the involvement of ECRs in the co-production of knowledge for the management of extreme event impacts, it is important to identify and actively support the skills needed to facilitate this interactive dialogue (Fernàndez, 2016) and to think profoundly about the perception of users, their capabilities and the constraints they face. Collaborations between natural and social scientists in the Earth system science field may play an important role in enhancing the understanding of these interactions, as well as sharing information in multiple directions among users (Weaver et al., 2014).
To ensure that CWH information is accessible and understandable for those involved in decision-making processes, it is important to present it within an adequate context and a useful time frame. One way to achieve these objectives is through the development of storytelling narratives, sometimes referred to as climate risk narratives, where information is introduced as part of a broader story in which the user problems and settings are the main characters (Hazeleger et al., 2015; Lejano et al., 2018; Scott et al., 2018). To create a continuous flow of the latest scientific information to users, these narratives shall have an evolving character and be updated regularly in collaboration with the users. These risk narrative are not a-one-solution-fits-all approach. They shall be tailored to specific users, responding to the different requirements according to their location in the research-to-application spectrum. For example, collaboration with technical users, such as engineers, will results in different narratives than with policy-makers. As possible outsets, we acknowledge the role of decision-making and adaptation tools to assist users to inform their decisions and planning processes. Additionally, we encourage the improvement of the communication and dissemination channels, such as interactive mobile-phone applications, in order to increase the effectiveness and applicability of CWH extreme events information (Cifelli et al., 2005).
The improvement and usability of information, and interactions between scientists and stakeholder is now increasingly on the agenda of many institutions and organizations, though there are some constraints which are important to acknowledge. In this sense, we recognize that the development of user-driven science should not be performed at the expense of fundamental and curiosity-driven science. To advance the field in line with the described ways forward, an additional amount of human and material resources may be required. A way to overcome this hurdle is to engage boundary organizations (e.g., the non-governmental organizations, service providing institutions, or governmental organizations) in the development of information by improving the efficiency of processing and its dissemination (Lemos et al., 2012). The involvement of boundary organization in the co-production of knowledge could be facilitated by the professional presence of ECRs, To allow interdisciplinary science to thrive ECRs face challenges for their career development. Time intensive, co-production based scientific approaches may not fit well within the current scientific evaluation schemes, such as h-index publication scores. New and existing approaches (Lebel and McLean, 2018) to measure scientific impact and excellence need to be further developed to ensure the continuity of scientific advances of this kind, as well as to ensure a stimulating environment for ECRs to pursue their careers in academia, as well as in the boundary organizations.
3. Crossing Scales and Regionality
To deliver the CWH information needed by users, significant progress in modeling studies and our physical understanding remains critical. Over recent decades, major advancements have been made in understanding the controls and regulators of CWH phenomena across scales. These developments, in part, have been achieved through internationally coordinated model intercomparison projects (e.g., AMIP and CMIP, CMIP6-Endorsed MIPs; Eyring et al., 2016; Gutowski et al., 2016) that have informed global synthesis reports (i.e., IPCC Assessment Reports, SREX) to provide scientists and society with a more complete view of the past, present, and projected future global climate. While this progress is crucial for the scientific understanding of the Earth system and its change, translating this knowledge to regional and local contexts and connecting CWH phenomena remains challenging.
CWH datasets continue to improve and grow in size and must be adequately processed to produce readily accessible and useful information. The effectiveness and utility of this information depends not only on the quality of data and post-processing, but also on the production of meaningful climate indices, which capture consistent and complete scenarios for both technical (e.g., attribution or vulnerability, impacts, and adaptation studies) and non-technical (e.g., collective education, policy, and spreading common knowledge contexts) uses (Street, 2015). Furthermore, interpretation tools and a clear explanation of potential errors and uncertainties must be provided to guide the processing and interpretation of climate series for understanding CHW events. As ECRs, we believe that a priority should be given to amplify the development and application of scenario science (Carter et al., 2001; IPCC, 2013; Huard et al., 2014) and fostering the connections between modeling and impact communities, alongside consolidation of methods for post-processing data into climate information at different scales for projecting extreme events.
There is a rapid movement toward an era in which different modeling scales are merging; numerical models of the atmosphere are run close to the spatial scales of hydrological models and the resolution of global climate models is approaching that of regional models and even numerical weather prediction models. Advances in the simulation of mesoscale to convective-scale processes in the atmosphere are opening new frontiers in modeling hydroclimate and land-atmosphere exchanges of water and energy that are crucial for the understanding of extreme events. The developers of global and regional climate models have been largely separate, a division which is still visible in large international research efforts (CMIP6-EndorsedMIPs; Jacob et al., 2014; Eyring et al., 2016; Gutowski et al., 2016). This division, mainly based on the differences in horizontal resolution, has led to different representation of key climate processes in the models. Global climate modeling typically focused on the accurate simulation of planetary scale processes, while regional modeling sought to resolve climate processes at the regional to local scales. In the new generation of global high-resolution models, however, this division slowly narrows. For example, regional features, such as tropical cyclones and hurricanes, can now be realistically simulated in global models (e.g., Wehner et al., 2014). Simultaneously, the recent developments toward convection-permitting scales in regional models offer promising opportunities for improved local understanding of climate and hydrological processes and impacts (Prein et al., 2015; Clark et al., 2016), especially for complex terrain and cities. This merging of scales of the global and regional modeling communities could foster further development in the scientific understanding of weather and climate extremes at regional scales as well as enhance the climate information available to users, which has traditionally been produced in regional climate modeling communities.
As ECRs, we believe that there is an urgent need to intensify interactions and meaningful scientific efforts between the regional and global climate modeling researchers and established communities. One specific way forward could be to develop a common, potentially process-based, methodological approach to model evaluation at regional scales (e.g., James et al., 2018). In the longer run, a unified rigorous model and data assessment protocol between global and regional climate modeling communities could be developed to support mutually beneficial scientific development.
Furthermore, with increased interaction between the global and regional research communities it would be possible to speed-up scientific progress through mutual learning, save on computational costs, and avoid repetition toward faster and more efficient improvements to dynamical models. Additionally, we believe that through joining forces we will be able to reduce the biases currently shown by model outputs compared to observations. ECRs can play a role in this development by interacting amongst themselves to exchange ideas on global and regional approaches and ensure intensified collaborations between the next generation of CWH scientists, to bring about a better understanding of regional-to-local extreme events.
4. Integrating Unconventional Data Sources
Observational networks to monitor weather, climate, and hydrology are crucial for research and underpin the development of regional information for extreme events. Such networks are formed from a broad range of widely-used, conventional data sources, including in situ and remotely-sensed measurements. ECRs stand with senior scientists in recognizing the crucial need for the continuation of these observational networks and programmes. Simultaneously, we recognize that over the past decade a variety of new, unconventional methods to generate hydro-meteorological observations have been developed. These unconventional data sources can be used to increase the temporal and spatial resolution of observational networks, but also to enhance the public involvement and interest in science.
Current observational networks do not usually meet the desired climate information needs for regional and global studies, especially in low-density populations areas (Kaspar et al., 2015; Mason et al., 2015) and developing countries (WMO, 2007; Aldrian et al., 2010; Koutsouris et al., 2015; Akinsanola et al., 2017; Dike et al., 2018). The lack of a spatially and temporally dense network of observations propagates uncertainties and errors in datasets and model outputs (Sun et al., 2018), diminishing our capacity to advance the understanding of extreme events (New et al., 2006; Omondi et al., 2014; Alexander, 2016; Menang, 2017). Unconventional data sources can provide part of the solution to this problem by increasing the spatial and temporal coverage of hydrometeorological datasets. Specifically, emerging technologies, such as single-board computers, for example Raspberry Pi and Arduino (León et al., 2017), or small, low cost, satellites such as CubeSats (Woellert et al., 2011) allows the production of a wide range of observational instruments at low cost (Hut, 2013) and makes it more affordable to cover extensive areas or areas with a high risk of loss of instruments. Another emerging technology rapidly gaining attention is the use of unmanned aerial vehicles (UAV), with applications ranging from creating high-resolution digital elevation models to sampling hard-to-access environments and validating computational models (Kelleher et al., 2018). A different approach is to encourage the participation of society in the generation of new scientific knowledge in the form of crowdsourcing data collection. Some hydrological and climate studies have already integrated crowd-sourced data into their analysis; for example, smartphone applications to collect information on local weather conditions provide data that can be used to evaluate models performance and track the evolution of specific high impact events (Sosko and Dalyot, 2017), pictures of stream stage gauges can be used to record streamflow (Lowry and Fienen, 2013), social media messages can be used to improve streamflow simulations for flood monitoring (Restrepo-Estrada et al., 2018) and images can be analyzed to retrieve rainfall intensity (i.e., Allamano et al., 2015; Yang and Ng, 2017).
As we work toward the integration of unconventional data sources into scientific projects, opportunities arise to address other ongoing data issues. Firstly, there is a need for the standardization and improvement of metadata, not only from the initial observation gathering but throughout the processing chain, for both rapidly developing unconventional data sources and established datasets. An interactive metadata cycle is encouraged, whereby anyone adding value to a dataset keeps detailed metadata which is shared via feedback with the data provider, as well as forwarded to the next user. There is also a need for improved technical documentation of datasets, specifically geared toward multidisciplinary next-users. Finally, a key ongoing issue with CWH sciences is open access data. Without freely and easily available data, science is unable to move forward. We suggest that collectors of unconventional data sources aim to further the cause of open-access by sharing the generated datasets. We believe ECR networking can play a large role in promoting the generation and dissemination of data on national and international levels, in particular involving developing countries, where cheaper and unconventional data sources could have an important role to play.
For the science community to accept unconventional data sources as valid and reliable datasets, a strong quality control must first be conducted, and a methodology for efficient inclusion in the data gathering process must be developed (Buytaert et al., 2014; Coz et al., 2016; Paul et al., 2018). We suggest that ECRs could take a leading role in performing this groundwork, and any ongoing development, especially since ECRs will be the ones developing and using this data in the years ahead. We aim for more national and international projects pushing for the development, expansion, and maintenance of unconventional data networks.
Citizen science, where amateurs or volunteers participate in research activities and assist scientists, can be used as an awareness and outreach tool to build an ongoing relationship between scientists and users, expanding the possibilities for ECRs to act as trust-brokers through developing relationships with the users in a way that is beneficial to both sides (Paul et al., 2018). Crowdsourcing initiatives need to have a strong collaboration with the community throughout the scientific project to keep motivation and participation levels high, for continued data collection. This can be done by sharing the outcomes of the research on a regular basis, both in terms of datasets and information, with the relevant communities. This iterative process provides an opportunity to move forward into the co-generation of knowledge and the development of valuable CWH information.
5. Outlook
We have outlined major challenges and opportunities that arise in the study and understanding of extreme events, a topic which is gaining societal relevance as the occurrence and impact of such events increases in the changing climate. As ECRs, we suggest three main ways forward in the field: a stronger interaction between users and scientists, a collaborative modeling approach between the different modeling communities, and an assessment, and possible inclusion, of unconventional data sources in scientific studies (Figure 2). By making strides in the three domains, the scientific community can gain improved quantification and prediction of extreme events, and be able to deliver more useful and relevant regional information to users.
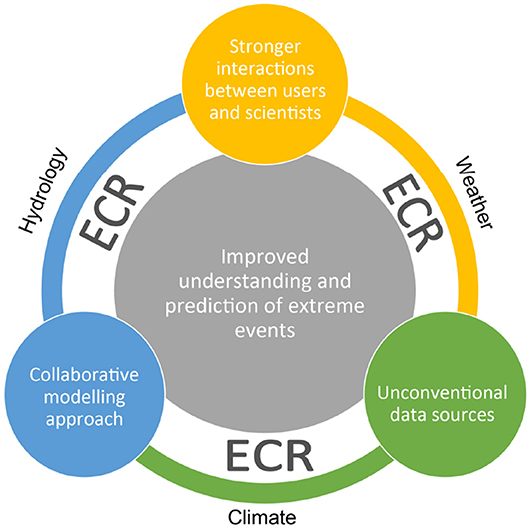
Figure 2. Ways forward toward regional information for improved understanding and prediction of CWH extreme events.
The challenges outlined above also provide great opportunities for ECRs to take a leading role in moving CWH sciences forward. By doing so, distinct challenges arise for ECRs, particularly to develop their careers in this highly interdisciplinary environment. Alternative approaches are required to evaluate scientific impact and excellence that correspond to the research needs of our generation. ECR networks provide an excellent platform to support ECRs in their leading roles by involving and empowering ECRs within the global community through interactions and collaborations. These networks bring together the fresh and diverse perspectives and enthusiasm from the global ECR community, assets that are crucial to making breakthroughs in the challenges outlined in this paper (Rauser et al., 2017; Dike et al., 2018; Swart et al., 2018). We encourage the global community to support and strengthen their involvement with ECR communities for further advancement in the field of interdisciplinary Earth system science in the upcoming years to decades.
Author Contributions
All authors participated in the discussion leading to the paper. GSL, CA-W, MO, and CG compiled the workshop discussions into the initial draft and acted as principal editors during the manuscript redaction. FA-B, EB, AB, NH, VSI, SI, EvdL, NM, KR, KAR, NR, JR, RR, BUU, JPW, DPW, BJW, and YY participated in writing the subsequent manuscript redaction.
Conflict of Interest Statement
The authors declare that the research was conducted in the absence of any commercial or financial relationships that could be construed as a potential conflict of interest.
Acknowledgments
We wish to thank the Global Energy and Water Exchanges project (GEWEX), as well as the World Climate Research Programme (WCRP), the World Weather Research Programme (WWRP) and the Global Atmosphere Watch (GAW)—the research arms of the World Meteorological Organisation (WMO), who supported the participation of the majority of the co-authors of this paper, as well as the organization of the Young Earth System Scientists—Young Hydrologic Society (YESS-YHS) workshop, which was conjointly held with the GEWEX Open Science Conference in May 2018. We also acknowledge the role of the YESS community and YHS for bringing ECRs together to facilitate research dialogues and write-ups of such kind. We would also like to thank Kristoffer Aalstad, Christina Aragon, Furrukh Bashir, Christopher Beale, Imme Benedict, Cassiano Bortolozo, Felicia Chang, Mia Gross, Laurie Huning, Dambaru Kettel, Patrici Lawson, Charlotte Love, Shailendra Mandal, Regula Muelchi, Luana Pampuch, Izidine Pinto, Maria Mercedes Poggi, Matilde Ungerovich, Sara Vallejo-Bernal for their participation to the YESS-YHS workshop and sharing their views on the topics.
References
Akinsanola, A. A., Ogunjobi, K. O., Ajayi, V. O., Adefisan, E. A., Omotosho, J. A., and Sanogo, S. (2017). Comparison of five gridded precipitation products at climatological scales over West Africa. Meteorol. Atmos. Phys. 129, 669–689. doi: 10.1007/s00703-016-0493-6
Aldrian, E., Oludhe, C., Garanganga, B., Pahalad, J., Corradi, M. R., Boulahya, M., et al. (2010). Regional climate information for risk management. Proc. Environ. Sci. 1, 369–383. doi: 10.1016/j.proenv.2010.09.024
Alexander, L. V. (2016). Global observed long-term changes in temperature and precipitation extremes: a review of progress and limitations in ipcc assessments and beyond. Weather Clim. Extremes 11, 4–16. doi: 10.1016/j.wace.2015.10.007
Allamano, P., Croci, A., and Laio, F. (2015). Toward the camera rain gauge. Water Resour. Res. 51, 1744–1757. doi: 10.1002/2014WR016298
Buytaert, W., Zulkafli, Z., Grainger, S., Acosta, L., Alemie, T. C., Bastiaensen, J., et al. (2014). Citizen science in hydrology and water resources: opportunities for knowledge generation, ecosystem service management, and sustainable development. Front. Earth Sci. 2:26. doi: 10.3389/feart.2014.00026
Carter, T. R., La Rovere, E. L., Jones, R. N., Leemans, R., Mearns, L. O., Nakicenovic, N., et al. (2001). “Developing and applying scenarios,” in Climate Change 2001: Impacts, Adaptation, and Vulnerability. Contribution of Working Group II to the Third Assessment Report of the Intergovernmental Panel on Climate Change, eds J. J. McCarthy, O. F. Canziani, N. A. Leary, D. J. Dokken, and K. S. White (Cambridge; New York, NY: Cambridge University Press), 145–190.
Cifelli, R., Doesken, N., Kennedy, P., Carey, L. D., Rutledge, S. A., Gimmestad, C., et al. (2005). The community collaborative rain, hail, and snow network: informal education for scientists and citizens. Bull. Am. Meteorol. Soc. 86, 1069–1078. doi: 10.1175/BAMS-86-8-1069
Clark, P., Roberts, N., Lean, H., Ballard, S. P., and Charlton-Perez, C. (2016). Convection-permitting models: a step-change in rainfall forecasting. Meteorol. Appl. 23, 165–181. doi: 10.1002/met.1538
Coz, J. L., Patalano, A., Collins, D., Guillén, N. F., García, C. M., Smart, G. M., et al. (2016). Crowdsourced data for flood hydrology: Feedback from recent citizen science projects in Argentina, France and New Zealand. J. Hydrol. 541, 766–777. doi: 10.1016/j.jhydrol.2016.07.036
Dike, V. N., Addi, M., Andang'o, H. A., Attig Bahar, F., Barimalala, R., Diasso, U. J., et al. (2018). Obstacles facing Africa's young climate scientists. Nat. Clim. Change 8, 447–449. doi: 10.1038/s41558-018-0178-x
Eyring, V., Bony, S., Meehl, G. A., Senior, C. A., Stevens, B., Stouffer, R. J., et al. (2016). Overview of the coupled model intercomparison project phase 6 (cmip6) experimental design and organization. Geosci. Model Dev. 9, 1937–1958. doi: 10.5194/gmd-9-1937-2016
Fernàndez, R. J. (2016). How to be a more effective environmental scientist in management and policy contexts. Environ. Sci. Policy 64, 171–176. doi: 10.1016/j.envsci.2016.07.006
Gutowski, W. J. Jr., Giorgi, F., Timbal, B., Frigon, A., Jacob, D., Kang, H.-S., et al. (2016). WCRP COordinated regional downscaling experiment (cordex): a diagnostic MIP for CMIP6. Geosci. Model Dev. 9, 4087–4095. doi: 10.5194/gmd-9-4087-2016
Hazeleger, W., Van den Hurk, B., Min, E., Van Oldenborgh, G., Petersen, A., Stainforth, D., et al. (2015). Tales of future weather. Nat. Clim. Change 5, 107–113. doi: 10.1038/nclimate2450
Hering, J. G., Dzombak, D. A., Green, S. A., Luthy, R. G., and Swackhamer, D. (2014). Engagement at the science-policy interface. Environ. Sci. Technol. 48, 11031–11033. doi: 10.1021/es504225t
Huard, D., Chaumont, D., Logan, T., Sottile, M.-F., Brown, R. D., St-Denis, B. G., et al. (2014). A decade of climate scenarios: the ouranos consortium modus operandi. Bull. Am. Meteorol. Soc. 95, 1213–1225. doi: 10.1175/BAMS-D-12-00163.1
Hut, R. W. (2013). New Observational Tools and Datasources for Hydrology: Hydrological data Unlocked by Tinkering. Ph.D. thesis, Faculty of Civil Engineering and Geosciences, Department of Water Management. Delft University of Technology.
IPCC (2012). Managing the Risks of Extreme Events and Disasters to Advance Climate Change Adaptation. A special report of Working Groups I and II of the Intergovernmental Panel on Climate Change, eds C. B. Field, V. Barros, T. F. Stocker, D. Qin, D. J. Dokken, K. L. Ebi, et al. (Cambridge, UK; New York, NY: Cambridge University Press).
IPCC (2013). Annex III: Glossary [Planton, S. (ed.)]. In: Climate Change 2013: The Physical Science Basis. Contribution of Working Group I to the Fifth Assessment Report of the Intergovernmental Panel on Climate Change, eds T. F. Stocker, D. Qin, G.-K. Plattner, M. Tignor, S.K. Allen, J. Boschung (Cambridge, UK; New York, NY: Cambridge University Press).
Jacob, D., Petersen, J., Eggert, B., Alias, A., Christensen, O., Bouwer, L., et al. (2014). Euro-cordex: new high-resolution climate change projections for european impact research. Region. Environ. Change 14, 563–578. doi: 10.1007/s10113-013-0499-2
James, R., Washington, R., Abiodun, B., Kay, G., Mutemi, J., Pokam, W., et al. (2018). Evaluating climate models with an African lens. Bull. Am. Meteorol. Soc. 99, 313–336. doi: 10.1175/BAMS-D-16-0090.1
Kaspar, F., Helmschrot, J., Mhanda, A., Butale, M., de Clercq, W., Kanyanga, J. K., et al. (2015). The sasscal contribution to climate observation, climate data management and data rescue in southern Africa. Adv. Sci. Res. 12, 171–177. doi: 10.5194/asr-12-171-2015
Kelleher, C., Scholz, C. A., Condon, L., and Reardon, M. (2018). Drones in geoscience research: the sky is the only limit. Eos 99. doi: 10.1029/2018EO092269
Koutsouris, A., Chen, D., and Lyon, S. (2015). Comparing global precipitation data sets in eastern Africa: a case study of Kilombero Valley, Tanzania. Int. J. Climatol. 30, 2000–2014. doi: 10.1002/joc.4476
Lebel, J., and McLean, R. (2018). A better measure of research from the global south. Nature 559, 23–26. doi: 10.1038/d41586-018-05581-4
Lejano, R. P., Casas, E. V., Montes, R. B., and Lengwa, L. P. (2018). Weather, climate, and narrative: a relational model for democratizing risk communication. Weather Clim. Soc. 10, 579–594. doi: 10.1175/WCAS-D-17-0050.1
Lemos, M. C. (2015). Usable climate knowledge for adaptive and co-managed water governance. Curr. Opin. Environ. Sustain. 12, 48–52. doi: 10.1016/j.cosust.2014.09.005
Lemos, M. C., Kirchhoff, C. J., and Ramprasad, V. (2012). Narrowing the climate information usability gap. Nat. Clim. Change 2, 789–794. doi: 10.1038/nclimate1614
Lemos, M. C., and Morehouse, B. J. (2005). The co-production of science and policy in integrated climate assessments. Global Environ. Change 15, 57–68. doi: 10.1016/j.gloenvcha.2004.09.004
León, J. R. C., Martínez-González, R. F., Medina, A. M., and Peralta-Pelaez, L. A. (2017). Raspberry pi and arduino uno working together as a basic meteorological station. arXiv:1711.09750.
Lowry, C. S., and Fienen, M. N. (2013). Crowdhydrology: Crowdsourcing hydrologic data and engaging citizen scientists. Groundwater 51, 151–156. doi: 10.1111/j.1745-6584.2012.00956.x
Mason, S., Kruczkiewicz, A., and Ceccato, P. A. C. (2015). Accessing and using climate data and information in fragile, data-poor states. Int. Instit. Sustain. Dev. 1–27.
Menang, K. P. (2017). Climate extreme indices derived from observed daily precipitation and temperature data over cameroon: the need for further assessments. Meteorol. Appl. 24, 167–171. doi: 10.1002/met.1628
New, M., Hewitson, B., Stephenson, D. B., Tsiga, A., Kruger, A., Manhique, A., et al. (2006). Evidence of trends in daily climate extremes over southern and west Africa. J. Geophys. Res. Atmos. 111, 1–11. doi: 10.1029/2005JD006289
Omondi, P. A., Awange, J. L., Forootan, E., Ogallo, L. A., Barakiza, R., Girmaw, G. B., et al. (2014). Changes in temperature and precipitation extremes over the greater horn of africa region from 1961 to 2010. Int. J. Climatol. 34, 1262–1277. doi: 10.1002/joc.3763
Orlowsky, B., and Seneviratne, S. I. (2012). Global changes in extreme events: regional and seasonal dimension. Clim. Change 110, 669–696. doi: 10.1007/s10584-011-0122-9
Osman, M., Aubry-Wake, C., Gulizia, C., and Langendijk, G. (2018). 2018 early career researcher workshop at the gewex open science conference. GEWEX Newslett. 28, 3–4.
Paul, J., Buytaert, W., Allen, S., Ballesteros-Canovas, J., Bhusal, J., Cieslik, K., et al. (2018). Citizen science for hydrological risk reduction and resilience building. Wiley Interdiscipl. Rev. Water 5:e1262. doi: 10.1002/wat2.1262
Prein, A. F., Langhans, W., Fosser, G., Ferrone, A., Ban, N., Goergen, K., et al. (2015). A review on regional convection-permitting climate modeling: demonstrations, prospects, and challenges. Rev. Geophys. 53, 323–361. doi: 10.1002/2014RG000475
Rauser, F., Alqadi, M., Arowolo, S., Baker, N., Bedard, J., Behrens, E., et al. (2017). Earth system science frontiers: an early career perspective. Bull. Am. Meteorol. Soc. 98, 1120–1127. doi: 10.1175/BAMS-D-16-0025.1
Restrepo-Estrada, C., de Andrade, S. C., Abe, N., Fava, M. C., Mendiondo, E. M., and de Albuquerque, J. P. (2018). Geo-social media as a proxy for hydrometeorological data for streamflow estimation and to improve flood monitoring. Comput. Geosci. 111, 148–158. doi: 10.1016/j.cageo.2017.10.010
Scott, D., Iipinge, K. N., Mfune, J. K. E., Muchadenyika, D., Makuti, O. V., and Ziervogel, G. (2018). The story of water in windhoek: a narrative approach to interpreting a transdisciplinary process. Water 10:1366. doi: 10.3390/w10101366
Sillmann, J., Kharin, V. V., Zwiers, F. W., Zhang, X., and Bronaugh, D. (2013). Climate extremes indices in the cmip5 multimodel ensemble: Part 2. Future climate projections. J. Geophys. Res. Atmos. 118, 2473–2493. doi: 10.1002/jgrd.50188
Sosko, S., and Dalyot, S. (2017). Crowdsourcing user-generated mobile sensor weather data for densifying static geosensor networks. ISPRS Int. J. Geo Inf. 6:61. doi: 10.3390/ijgi6030061
Street, R. B. (2015). Towards a leading role on climate services in Europe: a research and innovation roadmap. Climate Serv. 1, 2–5. doi: 10.1016/j.cliser.2015.12.001
Sun, Q., Miao, C., Duan, Q., Ashouri, H., Sorooshian, S., and Hsu, K.-L. (2018). A review of global precipitation data sets: data sources, estimation, and intercomparisons. Rev. Geophys. 56, 79–107. doi: 10.1002/2017RG000574
Swart, N. C., Busecke, J., Langendijk, G., Reed, K. A., Kang, S. M., Behrens, E., et al. (2018). Reflections on the clivar early career scientists symposium 2016. NPJ Clim. Atmos. Sci. 1:6. doi: 10.1038/s41612-018-0015-y
van Oevelen, P. (2018). Highlights from the 8th gewex open science conference. GEWEX Newslett. 28:2.
Weaver, C., Mooney, S., Allen, D., Beller-Simms, N., Fish, T., Grambsch, A., et al. (2014). From global change science to action with social sciences. Nat. Clim. Change 4, 656–659. doi: 10.1038/nclimate2319
Wehner, M. F., Reed, K. A., Li, F., Bacmeister, J., Chen, C.-T., Paciorek, C., et al. (2014). The effect of horizontal resolution on simulation quality in the community atmospheric model, CAM5.1. J. Adv. Model. Earth Syst. 6, 980–997. doi: 10.1002/2013MS000276
Woellert, K., Ehrenfreund, P., Ricco, A. J., and Hertzfeld, H. (2011). Cubesats: cost-effective science and technology platforms for emerging and developing nations. Adv. Space Res. 47, 663–684. doi: 10.1016/j.asr.2010.10.009
Yang, P., and Ng, T. L. (2017). Gauging through the crowd: a crowd-sourcing approach to urban rainfall measurement and storm water modeling implications. Water Resour. Res. 53, 9462–9478. doi: 10.1002/2017WR020682
Keywords: earth system science, extreme events, regional information, unconventional data sources, modeling, user-driven science, ECRs
Citation: Langendijk GS, Aubry-Wake C, Osman M, Gulizia C, Attig-Bahar F, Behrens E, Bertoncini A, Hart N, Indasi VS, Innocenti S, van der Linden EC, Mamnun N, Rasouli K, Reed KA, Ridder N, Rivera J, Ruscica R, Ukazu BU, Walawender JP, Walker DP, Woodhams BJ and Yılmaz YA (2019) Three Ways Forward to Improve Regional Information for Extreme Events: An Early Career Perspective. Front. Environ. Sci. 7:6. doi: 10.3389/fenvs.2019.00006
Received: 31 October 2018; Accepted: 11 January 2019;
Published: 04 February 2019.
Edited by:
Hayley Jane Fowler, Newcastle University, United KingdomReviewed by:
Scott Steinschneider, Cornell University, United StatesStewart Cohen, Environment and Climate Change Canada, Canada
Copyright © 2019 Langendijk, Aubry-Wake, Osman, Gulizia, Attig-Bahar, Behrens, Bertoncini, Hart, Indasi, Innocenti, van der Linden, Mamnun, Rasouli, Reed, Ridder, Rivera, Ruscica, Ukazu, Walawender, Walker, Woodhams and Yılmaz. This is an open-access article distributed under the terms of the Creative Commons Attribution License (CC BY). The use, distribution or reproduction in other forums is permitted, provided the original author(s) and the copyright owner(s) are credited and that the original publication in this journal is cited, in accordance with accepted academic practice. No use, distribution or reproduction is permitted which does not comply with these terms.
*Correspondence: Gaby S. Langendijk, langendijk.gs@gmail.com