- 1Department of Sociology, Social Work, and Anthropology, Utah State University, Logan, UT, United States
- 2State of Washington Water Research Center, Washington State University, Pullman, WA, United States
- 3Center for Environmental Research, Education & Outreach, Washington State University, Pullman, WA, United States
- 4Department of Civil and Environmental Engineering, Washington State University, Pullman, WA, United States
- 5Water Resources Graduate Program, University of Idaho, Moscow, ID, United States
- 6College of Law and Waters of the West Program, University of Idaho, Moscow, ID, United States
In the face of climate change, achieving resilience of desirable aspects of food-energy-water (FEW) systems already strained by competing multi-scalar social objectives requires interdisciplinary approaches. This study is part of a larger effort exploring “Innovations in the Food-Energy-Water Nexus (INFEWS)” in the Columbia River Basin (CRB) through coordinated modeling and simulated management scenarios. Here, we focus on a case study and conceptual mapping of the Yakima River Basin (YRB), a sub-basin of the CRB. Previous research on FEW system management and resilience includes some attention to social dynamics (e.g., economic and governance systems); however, more attention to social drivers and outcomes is needed. Our goals are to identify several underutilized ways to incorporate social science perspectives into FEW nexus research and to explore how this interdisciplinary endeavor alters how we assess innovations and resilience in FEW systems. First, we investigate insights on FEW nexus resilience from the social sciences. Next, we delineate strategies for further incorporation of social considerations into FEW nexus research, including the use of social science perspectives and frameworks such as socio-ecological resilience and community capitals. Then, we examine a case study of the YRB, focusing on the historical development of the FEW nexus and innovations. We find that a resilience focus applied to the FEW nexus can inadvertently emphasize a status quo imposed by those already in power. Incorporating perspectives from the social sciences, which highlight issues related to inequality, power, and social justice, can address these shortcomings and inform future innovations. Finally, we use causal loop diagrams to explore the role of the social in the FEW nexus, and we suggest ways to incorporate social aspects into an existing stock and flow object-oriented modeling system. This project represents a starting point for a continued research agenda that incorporates social dynamics into FEW system resilience modeling and management in the CRB.
Introduction
The Columbia River Basin (CRB) in the Pacific Northwest Region of the United States is home to a network of food production systems, hydroelectric dams, and tributaries and watersheds that shape the food-energy-water (FEW) nexus. The region is currently attempting to plan for projected changes in climate and precipitation, extremes in flood, and the renegotiation of the Columbia River Treaty (Cosens, 2010, 2016; Vano et al., 2010; Cosens and Fremier, 2018). Within the CRB, the Yakima River Basin (YRB) in particular provides more than $3 billion in agricultural products (Yoder et al., 2017), $3.4 million in power generation to local and regional grids (USBR, 2011a,b), and instream flows for endangered salmon and steelhead (Cosens et al., 2018). Over the past century the YRB has experienced a veritable food-energy-water transformation, from one dominated by Native American tribes and fishing, to a multicultural, highly managed, highly profitable agricultural center. This transformation was made possible by innovative developments and massive investments in infrastructure for irrigated agriculture, reservoir storage, railroad and highway connectivity, and fish ladders and screens (Meinig, 1962; Jarosz and Qazi, 2000; Vano et al., 2010; 98 Stat. 1333, Public Law 98–381; 98 Stat. 1379, Public Law 98–396). Not all transformations have been beneficial, nor have the benefits and costs been distributed evenly. For example, health concerns for farmworkers and consumers due to pesticide usage (Jarosz and Qazi, 2000), civil unrest over wage disputes (McMahon, 2002), protests over rangeland parcelization (Olson, 1980), and decades of litigation over declining fish resources available for Yakama Nation communities (Cosens et al., 2018) are inexorably tied to the development of the FEW nexus in this region.
In order to address FEW nexus sustainability in the YRB, CRB, and beyond, we need research that incorporates not only biophysical feedbacks and tradeoffs into proposals for innovation, but also socio-cultural drivers and outcomes of these interactions. According to the (World Commission on Environment Development, 1987), “a world in which poverty is endemic will always be prone to ecological and other disasters” (Lockie, 2016). However, divides between social and natural sciences sometimes hinder interdisciplinary work, in part because disciplines have developed independently with their own methodologies, epistemologies (Miller et al., 2008; Stuart, 2016), and ontologies. To address FEW nexus sustainability, we draw upon the concept of resilience. This offers one promising way forward, but it is not without issues. Numerous scholars address why resilience as a concept is unappealing to social sciences (Olsson et al., 2015) or how it has important limitations (Davidson, 2010; Cote and Nightingale, 2012; Hatt, 2013). Many of these issues are also relevant to discussions of sustainability. The puzzle we tackle in this paper is how to address these issues and incorporate considerations of the social, including political, economic, and cultural issues, into FEW nexus resilience research. We argue that incorporating considerations of social-ecological resilience along with other insights, frameworks, and indicators from the social sciences will improve research by making it more just, more accurate, and more likely to produce desired advances, such as innovation adoption, and avoid unintended negative consequences.
In what follows, we first discuss several issues in bringing social science, and social considerations, into FEW nexus resilience research. We then discuss opportunities in this area and identify a set of possibly useful frameworks and indicators, while also acknowledging limitations and data needs. The strategies we suggest for incorporating the social into FEW nexus resilience emphasize process, metrics, and modeling. After this, to provide real-world context, we examine a case study of the YRB focusing on the historical development of the FEW nexus and current FEW nexus challenges and innovations. We also use this case study to explore some of the issues and opportunities that arise when incorporating social considerations into FEW nexus research. Finally, we discuss our conceptual mapping approach, the goal of which is to advance incorporation of the social into FEW nexus resilience research and facilitate later efforts at system dynamic (SD) modeling. We examine how social insights inform research based on an object-oriented river basin modeling system already in use (RiverWare™, Carron et al., 2000; Zagona et al., 2001), and we discuss implications for future research. This paper represents an interdisciplinary approach—while engineers may not be aware of some of the social science frameworks we discuss, sociologists may not be as familiar with system dynamics modeling—therefore our work aims to facilitate collaboration between diverse audiences.
Bringing Social Science Into FEW Nexus Resilience Research
Growing concern has been expressed over food, energy, water resources, and their deep connection to income inequality, economic instability, and urban expansion (Middleton et al., 2015; Howarth and Monasterolo, 2016). Importantly, researchers are questioning how FEW nexus frameworks can be operationalized (Liu et al., 2017), whether critical issues such as labor, human capital, health, and welfare are absent (Wichelns, 2017), and what perspectives/institutions should be prioritized for integration (Al-Saidi and Elagib, 2017). Here we discuss concepts and issues related to these questions in an effort to bring social science perspectives more explicitly into FEW nexus resilience research.
Concepts
Many have studied the water-energy-food (WEF) nexus and have used this specific abbreviation to investigate the complex interactions between these resources (Lawford et al., 2013; Biggs et al., 2015; Middleton et al., 2015; Larcom and van Gevelt, 2017; Liu et al., 2017). We use the FEW (food-energy-water) abbreviation for consistency with recent initiatives and projects funded by the National Science Foundation (2015) and Mohtar and Lawford (2016). These projects emphasize a systems-based approach to understanding Innovations at the Nexus of Food, Energy, and Water Systems (INFEWS). D'Odorico et al. (2018) recently discuss these emerging initiatives and parallel growing literatures in their thorough review of the Global Food-Energy-Water nexus. Synergies among variations in WEF focus, which tend to emphasize global resource security and development, and FEW nexus research themes such as fundamental understanding and basic research, need to continually be explored, especially with further considerations for how they define ultimate goals (National Science Foundation, 2015; Albrecht et al., 2018; D'Odorico et al., 2018). Despite differences in terminology, we utilize insights from both of these complementary bodies of research.
Sustainability and resilience are also related concepts. Davidson (2010: p. 1136) defines sustainability as a “systemic state of indefinite equilibrium, in which levels of anthropogenic material consumption and waste production remain below threshold productive and absorptive capacities of the ecological system, while at the same time ensuring a quality of life that is considered acceptable by current and future members of that social system.” The most common definition of sustainable development comes from the World Commission on Environment Development (1987) report Our Common Future, which defines sustainable development as “development that meets the needs of the present without compromising the ability of future generations to meet their own needs.”
We understand resilience generally to refer to the ability of systems to absorb and adapt to change without the dissolution or transformation of the system (Gunderson, 2000); we expand on this in more detail below. In line with others, we consider resilience in the face of change to be a component of larger sustainability aims (Summers et al., 2017). Yet we recognize resilience is not a synonym for sustainability. Resilience is not always desirable (Fernandez et al., 2016); even when it is desirable it may not be a priority for sustainability, it is not required for or an outcome of sustainable development, and it has limitations when applied to the social realm (Lockie, 2016).
Issues With Incorporating the Social Into FEW Nexus Resilience Research
Social scientists have been critical of work attempting to include social factors in studies of resilience because the concept is often seen as incompatible with social systems (Davidson, 2010; Olsson et al., 2015; Stuart, 2016). Here, we present five areas of complexity when integrating the social into biophysical FEW nexus resilience research. This is useful because understanding the complexity of interdisciplinary integration is necessary in order to move past previous limitations. In highlighting these issues, we provide insights as to how incorporating social considerations can advance understanding of FEW nexus resilience.
Defining Resilience Across Disciplines
A first issue is variation in the concept of resilience across disciplines. “It is evident that the dominant worldview in resource and environmental management of “systems in equilibrium” is incompatible with observations of the complex dynamics of social and ecological systems” (Berkes et al., 2003: p. xi). In engineering and some branches of ecology, resilience is commonly understood as a natural balance, or a steady state to which a system returns after a stress or perturbation. These understandings often reference the ball and cup analogy of returning to a state of equilibrium, or possibly transitioning to a new state with a new equilibrium. Applying a social science lens to the FEW system draws attention to limitations of the engineering definition of resilience and highlights the need to understand ecological and social system realities more explicitly represented in the ecological definition of resilience. While in engineering, resilience may be operationalized as time to return to equilibrium, in ecology, resilience might be measured as the magnitude of change that can be absorbed before transitioning to a new state (Berkes et al., 2003: p. 35; see Davidson et al., 2016 and Quinlan et al., 2016 for reviews of definitions across disciplines). In socio-ecological and other interdisciplinary research, resilience refers to the ability of systems to resist or adapt to changes or shocks and persist, or to move into an alternative, possibly more desirable state (Folke, 2006; Olsson et al., 2015). Addressing conceptual variations in understandings of resilience, and subsequent differences in operationalization and measurement, is a first step in incorporating the social into FEW nexus resilience research (Chuang et al., 2018).
Social Justice Considerations—Resilience for Whom and of What?
A second related consideration when bringing the social into FEW nexus resilience research is the question of whether resilience is normative. Resilience researchers tend to understand resilience as “good” (Olsson et al., 2015). However, social scientists are likely to question this assumption, encouraging resilience researchers to take a more critical approach by asking “resilience for whom?” and “resilience of what?” (Cote and Nightingale, 2012; Olsson et al., 2015)1.
In terms of “for whom,” social scientists, especially sociologists, are likely to highlight the roles of inequality and power in systems (Roberts and Parks, 2006; Jorgenson and Clark, 2009; Jorgenson, 2016; Givens, 2018). Lockie (2015: p. 139) writes, “the pollution that makes some people wealthy makes other people sick, threatens their livelihoods, and increases their risk of injury and displacement.” Yet despite knowledge that social problems make societies and systems more vulnerable, resilience research does not tend to prioritize poverty alleviation or address social injustice (Lockie, 2016). Cote and Nightingale (2012: p. 484–485, 479) portray FEW nexus resilience as “a power-laden framework that creates certain windows of visibility on the processes of change while obscuring others.” Resilience research has also tended to treat social groups as homogeneous and static (Stuart, 2016). FEW nexus research would be improved by giving attention not only to tradeoffs between sectors (Endo et al., 2017; Larcom and van Gevelt, 2017) but also to conflict, conflicting interests, and power dynamics within sectors and nexus stakeholders (e.g., Huszar et al., 1978; Jarosz and Qazi, 2000; Diver, 2018). Instances of mutually beneficial cooperation and exchange can also be examined and may serve as models.
With respect to “resilience of what,” social scientists may question the desirability of FEW system resilience, which presupposes the value of maintaining the system, rather than aiming for system change. In the social sciences, especially sociology, theoretical perspectives emphasize conflict, inequality, and power, see order as determined and maintained by dominant groups, and highlight conflict between groups as a source of change. Therefore, change could be seen as needing to be transformative and involve the redistribution of power. Without a social science perspective, change may be depoliticized (Olsson et al., 2015: p. 5).
Achieving Resilience—What End Goals?
A third related question in research that aims for innovations in FEW nexus resilience is, what are the goals? If the desirability of maintaining the system as a whole is questioned, identifying system functions may be an alternate way to identify what is desirable to sustain and what is meant by adaptation vs. transformation. However, focusing on a system's function tends to ignore inequality and conflict in the system by not attending to who gets to identify what functions are valued and benefit most from valued functions (Hatt, 2013; Olsson et al., 2015: p. 5). Different individuals, groups, and sectors obtain different functions from the FEW nexus. Focusing on system function may also obscure the desirability of system change for some. If adopted unquestioningly, focusing on system functions risks placing the focus on positive functions and obscuring negative and unintended outcomes. For example, increasing the number of salmon in the CRB might come at the expense of irrigation water for farmers, or improving the resilience of per capita economic growth could increase environmental damage. Simply focusing on the function(s) of a system also ignores the possibility of substitutability, that a different system could provide some of the same identified functions in coupled human and natural systems (Chen et al., 2012). Finally, this raises further issues regarding ethical considerations of the “function” of an ecosystem and the consequences for not only humans, but also non-human species and the ecosystem itself. Identifying goals for resilience still represents a productive way forward, as long as an equity dimension is part of conceptualizing FEW nexus resilience.
Conceptualizing and Operationalizing Resilience and Change
Fourth, and especially in order to model FEW nexus resilience, we need to consider how we conceptualize and operationalize adaptation, change, and system change in FEW nexus resilience, especially when it comes to the social. Social relations may not be able to be adequately characterized by the terms either resilience researchers or systems modelers use to describe system interactions. Resilience researchers' differentiation between disturbances vs. deliberate efforts for system change and system modeler's conceptualizations of stocks, flows, and feedback loops may all be inadequate in accounting for variations caused by human agency and in the contexts of norms, cultures, and power inequalities (Olsson et al., 2015). For example, the choice by agricultural workers in one region to strike, the decision by some tribal groups to bring lawsuits that result in the removal of dams, or the election of a president which creates uncertainty about future environmental regulations all represent examples of human agency that vary by context and are difficult to model at the system level. Further, what do we mean by “persist” vs. adapt and change, and how do we give these precise conceptual and operational definitions? Is transformation part of resilience or something different? How do we know when a system has adapted, vs. transformed? In other words, there is ambiguity in resilience research regarding operationalizing and modeling the role of change and transformation in perpetuating the system and making it more resilient vs. shifting to a new system, which is further complicated by the addition of the social (Olsson et al., 2015). Further, a new system may or may not be more sustainable or resilient.
Boundaries and Scale
Fifth, studying the resilience of FEW systems requires delineating boundaries of the system and considering scale. This involves designating factors as exogenous or endogenous to the system, conceptualizing boundaries across space and time, and thinking about multilevel processes across scales. These considerations become even more complex with incorporation of the social, as systems operate at different units or levels. Dietz (2017) offers one useful typology as an illustration, as follows. Ecological systems may be seen as including the biosphere, biotic province, landscape, watershed/airshed, community population, and individual, whereas the social system may be thought of as the world system, nation, culture, political subdivisions, community, household, and individual. Political boundaries, from national to tribal to state to county overlap with natural features. “Human system boundaries and ecological system boundaries are nearly always different” (Dietz, 2017: p. 199).
Social relations also connect actors, institutions, and structures across and beyond the system (Olsson et al., 2015). Adger et al. (2005: p. 1037) find in an increasingly globally connected world, “the resilience (or conversely vulnerability) of coastal societies is more tightly linked to larger-scale processes today than in the past.” In a review of research on the WEF nexus, Endo et al. (2017) find stakeholders across boundaries and at various scales, representing research, science-policy interfaces, funders, governments, development organizations, business and industry, civil society (NGOs), and media. Perrone and Hornberger (2014) emphasize the role of boundary crossing trade on FEW nexus security. Peterson (2000) tackles resilience and transformation across scales in interacting natural and social systems in a case study of salmon in the CRB, while Jarosz and Qazi (2000) examine global forces that shape the apple industry in Washington. Such approaches highlight the complexities of boundaries and scale when social system considerations are included; they also demonstrate the necessity of including social considerations for an accurate understanding of FEW system dynamics.
Unequal power dynamics also shape the delineation of boundaries, with important social implications. An emphasis on resilience at local scales may detract from the need for collective responsibility at larger scales to address inequality and risk (Lockie, 2016). Furthermore, what we label as resilient could just be a short-term condition, or a condition enabled by exploitation elsewhere in space or time (Hornborg, 2006; Davidson, 2010). Many studies of sustainability examine one place and do not attend to interactions across boundaries and impacts on sustainability in multiple places; in response Liu et al. (2013) propose an integrated framework based on telecoupling, a concept that draws attention to socioeconomic and environmental interactions over distances.
In sum, social science perspectives help us consider in more depth what we mean by resilience, inequality and power in driving processes and outcomes; goals of the FEW nexus; strategies for adaptation vs. system change; and boundaries of coupled human and natural systems at various scales and across space and time. Consideration of the social helps us reflexively assess “whose environments and livelihoods we seek to protect and why” (Cote and Nightingale, 2012) and who has voice in that process. Such considerations will help advance FEW nexus research by more fully capturing FEW nexus realities and by attending to issues of justice and equity, especially in the context of environmental changes and proposed innovations.
Incorporating social science perspectives into FEW nexus research also highlights data needs: data that capture social inequalities between and within stakeholder groups, data that are compatible to both social and ecological systems at various scales, and data collected over time. We need data that relate to processes, drivers, outcomes, and goals. Next, we turn to some specific strategies for incorporating social considerations and available data into FEW nexus research.
Strategies for Incorporating the Social Into FEW Nexus Resilience Research
There are several theoretical and methodological approaches well-suited to addressing some of the issues highlighted above and enabling incorporating the social into FEW nexus resilience research. Here, we briefly discuss several approaches that we put into categories relating to processes, metrics, or modeling.
Processes
Process-based approaches focus on the participants in the resilience process and assist in developing generalizable frameworks for understanding complex FEW systems. The resilience framework for social-ecological systems analysis is one of the most common process-based approaches for integrating the social into resilience research. This conceptual framework draws on complex systems theory to analyze how human societies interact with ecological change and build capacity to adapt. Resilient social-ecological systems are ones that can adapt to change—both respond to change and shape change—in a way that does not limit future options and actually enhances capacity to adapt (Berkes et al., 2003). Scholars working in this area bring together ecological, social, and economic elements, conceptualize resilience as a dynamic process rather than a state variable, and focus on adaptive capacity, complex adaptive systems, and panarchy, a concept specifically emphasizing systems analysis, multi-scale and temporal interactions, and interdependencies (Gunderson and Holling, 2002; Folke, 2006; Curtin and Parker, 2014; Chaffin et al., 2016; Cosens and Gunderson, 2018; Cosens et al., 2018). Humans are increasingly seen as the cause of system changes and this perspective can provide insights as to why conventional scientific and technological approaches to resource and ecosystem management sometimes make problems worse (Berkes et al., 2003; Folke, 2006).
Much of the research on socio-ecological systems draws from a related and overlapping body of research on governing the commons and managing common pool resources, Ostrom (1990, 2009) also emphasizes process and focuses on self-governance of resources by users to achieve sustainability. Challenging the assumptions that resource users are not able to engage in sustainable self-governance and that market control, i.e., privatization, or state control of resources are the only ways to avoid a “tragedy of the commons,” predicted by Garrett Hardin (Hardin, 1968: p. 1243), Ostrom (1990, 2009) documented self-organization as a successful alternative in her empirical research. This research identifies a variety of factors that encourage the likelihood of collective social and institutional organization to sustainably manage social-ecological systems, which makes system collapse less likely. Ostrom (2009) in her article, “A General Framework for Analyzing Sustainability of Socio-Ecological Systems” finds system collapse is more likely in systems that are large, highly valuable, open access, and made up of diverse resource users who do not communicate and fail to develop rules and norms for managing the resource (Ostrom, 2009). Furthermore, examples of locally evolved self-governance, sustained over time but disrupted when outside factors impinge, demonstrate the need to analyze complex systems at different spatial and temporal scales and portray sustainability as an ongoing struggle in the face of increasing resource demands driven by interactions between population, consumption, and advanced technologies that increase resource use (Dietz et al., 2003). In addition to influencing the literature discussed above on socio-ecological system resilience, Ostrom's work has spawned a large body of research on commons governance, some of which engages with FEW issues. For example, Villamayor-Tomas et al. (2015) analyze the role of institutions on environmental outcomes of the FEW nexus. Ostrom's work has also inspired multiple other research areas (see Arrow et al., 2012 for a review). Two relevant examples include work on human drivers of environmental change (Rosa and Dietz, 2012; Dietz, 2017) and work on Coupled Human and Natural Systems (CHANS) (Liu et al., 2007a,b). Within the CHANS framework, Chen et al. (2012) explore systems in which human and natural aspects interact and the production of human, animal, and ecosystem well-being and sustainability.
Metrics
Issues related to equitable access to resources, power, and agency have often been neglected from FEW nexus evaluation (Biggs et al., 2015), but several frameworks of indicators from the social sciences facilitate their incorporation. The Livelihoods Framework (LF) puts people and their needs at the center of the analysis and emphasizes the needs of the poorest and most vulnerable (Tanner et al., 2015). Livelihoods provide a link between the FEW nexus, resilience, and human well-being (Biggs and Watmough, 2012; Biggs et al., 2015). Researchers using the LF often examine local, context-specific livelihoods in rural contexts in less developed countries, and link social outcomes of the FEW nexus to the UN Sustainable Development Goals (Biggs et al., 2015; Rasul, 2016). The multidimensional Livelihoods index includes human, physical, social, financial, and natural metrics and enables measuring and monitoring of outcomes (Donohue and Biggs, 2015).
We are especially concerned with environmental change because of its expected impacts on human well-being (Dietz, 2017). Social science perspectives would likely conceptualize the purpose of the FEW system to be improving human well-being2. The theory of structural human ecology draws attention to the use of natural, human, and manufactured resources, and the role of social structure in producing well-being (York et al., 2002; Dietz, 2015). In the past, economic measures of wealth or affluence were used as proxies for well-being, leading to an emphasis on economic growth. There was also an overreliance on “technological fix” approaches to deal with environmental and resource issues. Together, the emphasis on growth and technology, and the assumption that focusing on these would also solve other problems, led to de-prioritization of directly addressing issues of inequality (Dietz, 2015). While the GINI coefficient is one useful and widely used metric of economic inequality, the structural human ecology of well-being perspective addresses relationships between inequality, human well-being, and the environment and employs measures such as life expectancy and subjective well-being. Such approaches and metrics of well-being are particularly well-suited to quantify a variety of system inequalities. Metrics include the environmental efficiency of well-being (Dietz et al., 2009; Knight and Rosa, 2011), environmental or ecological intensity of well-being (Dietz et al., 2012; Jorgenson and Dietz, 2015), energy intensity of well-being (Jorgenson et al., 2014), and carbon intensity of well-being (CIWB) (Jorgenson, 2014; Jorgenson and Givens, 2015; Givens, 2017, 2018). While these metrics are comparable across large geographic areas, there are many efforts to develop well-being focused indicators and indices at various scales. Smith et al. (2013) review ~20 different international well-being indices including the UNDP's Human Development Index, based on the capabilities approach of Sen and Nussbaum (Nussbaum and Sen, 1993). In the US, more nuanced measures may be especially pertinent for evaluating social aspects of FEW nexus resilience. The County Health Rankings and Roadmaps (CHR&R) measures community health at the county level (http://www.countyhealthrankings.org), the Center for Disease Control and Prevention's Social Vulnerability Index (SVI), is measure of community resilience based on US Census Data (https://svi.cdc.gov), and the Environmental Protection Agency's Climate Resilience Screening Index (Summers et al., 2017) incorporates social and environmental variables.
Flora et al. (2004; see also Flora et al., 1997) developed the Community Capitals Framework (CCF) to understand community development. The CCF offers a third way to conceptualize and operationalizing the social in the FEW nexus. The framework consists of seven types of capital: natural, cultural, human, social, political, financial, and built and attends to creating development, wealth, and self-sufficiency, improving leadership, and reducing poverty. In order to experience successful economic development, communities are advised to focus on types of capital and interactions among them (Emery and Flora, 2006). The capitals are seen as building upon each other; investment in some capitals impact other capitals, with the goal being “spiraling up” (Emery and Flora, 2006). The CCF encourages research from a systems perspective, and allows the analysis of stocks and flows, conceptualized as stocks of the various forms of capital and flows as types of capital invested. Capitals are operationalized with some of the same metrics mentioned above, such as census, voting, or survey data, although data availability over time and at various scales remains an issue3. While livelihoods and well-being are often conceptualized as outcomes of the FEW nexus, CCF explicitly frames capitals as both drivers and outcomes. For example, Schirmer et al. (2015) operationalize the capitals and interpret the capitals as determinants of human well-being, while Rijkhoff et al. (2017) and Martinkus et al. (2017) utilize the CCF to inform decisions about site selection for bio-fuel development. Donoghue and Sturtevant (2007) describe how various projects have used community capitals indicators to estimate community capacity and resilience in towns and cities of the western United States (for related work see Haynes et al., 1996; Harris et al., 2000).
Modeling
Conceptual maps are useful tools for representing complex system dynamics, including social aspects (Heemskerk et al., 2003). They enable capturing, organizing, and refining understanding of key feedback structures and outcomes within a particular system of interest. Conceptual mapping exercises, by nature, are creative processes in that they seek only to identify those important concepts or structures (whether physical or social) and the relationships that link them. Linkages in these exercises convey basic information, such as directionality or positive/negative impacts, rather than specific mathematical relationships. As such, these general, visual representations of a system are particularly useful for developing and building consensus around how a system is thought to behave (Stave, 2003), and bridging divides in interdisciplinary research (Stuart, 2016). Conceptual maps have been used to bring more explicit attention to social, community, and ecosystem service factors and to highlight composition and competition of communities (Nandalal and Simonovic, 2003), limitations and constraints due to environmental resources (Ghashghaie et al., 2014), co-dependent FEW security needs (Ericksen, 2008), and considerations related to governance, labor, poverty, and population fluctuations (Flora et al., 2004). Conceptual mapping can be a first step toward more precise modeling efforts.
A more rigorous methodology for representing complex system dynamics is system dynamics (SD) modeling. SD modeling is a structured framework for simplifying and simulating the complex feedback dynamics and time delays on the accumulation, movement, transformation, and reduction of key resource stocks (Luna-Reyes and Andersen, 2003). SD models tend to be computationally efficient and relatively flexible, particularly in comparison to the physically-based, mechanistic models often used for biophysical assessments. Models can have any number of feedbacks represented (from several to thousands) that when coupled integrate these myriad interactions into a set of non-linear expressions of accumulation and delayed response- a major strength of SD modeling for systems understanding. While SD modeling efforts span a diversity of disciplines and systems of interest, all center on the assumption that the structure of the system determines the observable behavior (Sterman, 2001). This assumption may be incompatible with social analysis because it minimizes the ability to incorporate human agency.
A second problem is that while some of the feedbacks and processes are quantifiable, many important social components are difficult to quantify adequately. For instance, it is relatively easy to find high-quality, quantitative data on economic or biophysical processes (e.g., yearly accumulated rainfall, annual gross domestic product), but reliable quantitative data for many social processes that are important drivers of system behavior (e.g., social capital, trust) are not widely available at varying geographic and time scales (Castelletti and Soncini-Sessa, 2007; Pahl-Wostl, 2007). Efforts to integrate quantitative and qualitative social data into SD models is ongoing. Many SD models make use of qualitative social data in the conceptual mapping and causal diagramming phases of model construction. In these early stages of model development, such data can be extremely useful for identifying appropriate policies, key structures, and decision-making logic that underlie the structure of the system and its behavior (Coyle, 2000). One promising approach regarding data is offered by current research on interoperable data, data that are compatible at different scales and units of analysis and that can integrate both social and biophysical components. Scholars working in this area highlight the potential for such data collection at scales ranging from the community to networks of environmental observatories, and the need for such data to improve our understanding of complex environmental problems and linked human and natural systems (Alessa et al., 2018; Bourgeron et al., 2018; Griffith et al., 2018).
The Yakima River Basin (YRB)
In this section, we turn to a case study to flesh out some of the social and FEW nexus dynamics at play in the YRB. We then link examples from the YRB back to the complexities and strategies addressed above for seeing the social as both driver and outcome in FEW systems.
The YRB, located in south central Washington (Figure 1), spans four counties, and supports a diverse array of economic activities, wildlife habitat, and cultural heritages. Today, the YRB is perhaps best known for its agriculture, but this area has had a long and complicated history of food, energy, and water development, both from a biophysical and social perspective, that continues to influence FEW management and policy in the basin. Currently, the YRB is one of the most agriculturally-productive regions in the state (Vano et al., 2010). In 2012, crop and livestock production in Yakima County alone generated over $1.6 billion in market sales (USDA, 2012). With approximately one-third of the farmland in the basin currently under irrigation, this area is one of the state's top producers of apples, wine grapes, milk, and hops (USDA, 2012). Estimates in 1978 valued agricultural products to be worth $180 million annually (Huszar et al., 1978); today it is over $3 billion (Yoder et al., 2017). This agricultural success is tied tightly to the development and proliferation of irrigation in the basin, and arguably embodies the heart of FEW nexus issues in the YRB. Given the importance of this basin to the state economy, regional wildlife, and local culture, the YRB has been heavily studied and thus provides a rich body of information and science from which to examine the FEW nexus through time. Here, we identify important technological and social innovations or changes that have shaped FEW nexus development in the basin over the past 150 years to provide a richer understanding of how the FEW nexus developed in interaction with the social context.
The Co-evolution of FEW Systems in the YRB
Before the nineteenth century, this basin was home to the Yakama and other Native American Tribes. These indigenous communities had cultures that entwined tribal traditions and livelihoods with the land, water, and wildlife. Most indigenous people in this area were subsistence-based and relied on root crops and the large populations of anadromous fish, including salmon and steelhead that would enter the Yakima River to spawn (Hunn, 1990). Records from the 1880's suggest runs of up to 790,000 fish per year (McIntosh et al., 1994; USBR and State of Washington Department of Ecology, 2012). The importance of these fish populations to the Yakama people were both tangible, with salmon accounting for upwards of 40% of a tribal person's daily caloric intake, and intangible (Gunnier, 2008). Salmon were honored in ceremonies and places of worship and were deeply integrated into social and cultural heritage (Montag et al., 2014).
In the early nineteenth century, Euro-American explorers arrived, displacing many Native American Tribes and creating the foundations of the agricultural and ranching industry that now dominate the basin (Figure 2). Critical to the success of this colonization was the installation of irrigation systems to support production in an otherwise semi-arid environment. The mid-1800's saw a rapid growth of private irrigation systems- series of canals and ditches drawing water from the Yakima River- and railroad development that spurred cattle and sheep ranching as well as irrigated wheat, alfalfa and fruit tree production (Kuhler, 1940). By 1900, more than 700 miles of canals and laterals extended throughout the basin and this number increased by orders of magnitude when Yakima was selected as the first “intervention area” by the Federal Reclamation Act of 1902 to subsidize agriculture through low-cost or free irrigation construction and infrastructure (Sheller, 1997). The culmination of this intervention was the Yakima Irrigation Project, initially constructed between 1905 and 1917, now providing water for nearly 470,000 acres of farmland, and comprising five reservoirs on the Yakima River system, six irrigation divisions, and about 2 million acre-feet of water entitlements (Yoder et al., 2014). By the 1950s, dam construction on the Yakima and Columbia Rivers had blocked a majority of waterways historically used by anadromous fish for passage to upstream breeding grounds, and consequently fish populations declined dramatically. Several species, including the sockeye, summer chinook, coho, steelhead, and bull trout are now listed as threatened under the Federal Endangered Species Act (State of Washington Dept of Ecology, 2012).
Meanwhile, the ability to irrigate large areas of the basin made this location particularly well-suited to food production, but required a large amount of farm labor to plant, tend, harvest, and process goods. This need for labor in the Yakima increased substantially with the onset of World War II (WWII), when many of the farm workers needed to produce food were sent to war. By 1941, there was a critical labor shortage in the YRB and throughout the Pacific Northwest (Gamboa and Leonard, 1990). Women, children, members of Native American Tribes, prisoners were recruited to help bring in harvests, in addition to migrant workers from Mexico under the federally negotiated Mexican Farm Labor (MFL) Program of 1942 (Jarosz and Qazi, 2000; Darian, 2006). While Mexicans had been present in small numbers in the Yakima basin since at least the 1850's, the MFL program brought in nearly 5 million workers to the US, many to the Northwest, in the 22 years the program existed (Darian, 2006). When WWII ended, the labor shortage also subsided, except in Washington. The completion of the Columbia Basin Irrigation Project in 1948 created a continued need for agricultural and food processing labor allowing many migrant workers year-round employment. Between 1950 and 1960, Hispanic populations in the Yakima Valley, and elsewhere in Washington, grew rapidly. Immigration was facilitated by two changes to federal law- the Immigration and National Act Amendments of 1965, which created a preference system for immigrants with relatives in the US, and the Immigration Reform and Control Act of 1986, which granted amnesty to immigrants who could prove they had been living in the US since 1982 (Darian, 2006). Since the 1960's, the Hispanic population in the Pacific states continued to rise, partially driven by the continued need for farm labor, but also because of the well-established Hispanic communities that facilitate integration for new migrants (Darian, 2006).
Hydropower development has also occurred in the YRB. The first power plant was built in Prosser in 1932 in the Kennewick Division and was later incorporated into the larger Chandler Power Plant in 1955. The Roza Power Plant was built in 1958. Today, these plants primarily generate hydropower for the agricultural sector, with an installed generating capacity of 24,900 kW. Surplus is marketed by the Bonneville Power Administration (BPA), which was established as a regional scale entity in 1937 and is now part of the federal Department of Energy. The BPA is responsible for coordinating the sale of hydropower from federal dams in the Pacific Northwest (Cosens et al., 2018). The hydropower economy of the YRB is smaller than the agricultural sector, with two divisions (Kennewick and Roza) using water for irrigation and power. In recent decades, these power plants have been subject to subordination to augment the streamflow and improve the aquatic habitats in the Yakima River as authorized by Title XII of the Act of October 31, 1994 (108 Stat. 4550, Public Law 103-434). There are concerns that further subordination could lead to hydropower deficiency in the agricultural sector.
Current FEW Issues in the YRB
Today, agriculture accounts for nearly 28% of the labor force in Yakima County. Demographics in this county reflect the mixed heritage of the YRB, with 48% of residents claiming Hispanic or Latino descent, 44% registering as White, and 6.2% as American Indian/Alaskan Native (Meseck, 2017). Despite the constant need for field and food processing in the Yakima, many of the jobs in this basin are seasonal, and thus the per capita income in Yakima County ($38,527) is significantly lower than the state average ($51,898), and the poverty rate is higher (19.1%- Yakima, 12.2%- state average) (Meseck, 2017).
Additional FEW issues in the Yakima link to climate change and a long-term trend in increasing water demands that have created tensions between water users in the basin. Inequality is characteristic of water rights in the YRB. The prior appropriation doctrine that guides water rights in this basin favors early water users, or senior water rights holders, over those who acquired water rights later. These later users, or junior water rights holders, may be prorated each season and may only receive water after the senior water allocations have been met. A mechanism for determining water allocations to YRB irrigators in years of shortage was developed with the 1945 Consent Decree [US District Court, 1945] along with a strategy for forecasting drought (and thus how much water junior rights holders could expect to have in a given season). Miscalculations associated with drought forecasting can, and have had, serious economic and societal ramifications for individual farmers in the YRB (e.g., Glantz, 1982; Vano et al., 2010). The issue of the “haves” and “have nots” is amplified as state water permitting agencies (Washington Administrative Code 173 539A-WAC) are forced to recognize and deal with the growing problem of groundwater use impacting surface water user rights, and the need to continue to provide, and in some cases augment, necessary in-stream flows for endangered fish (Gendaszek et al., 2014).
Issues over water rights are reflected not only at the individual scale, but can be seen in sectoral conflicts as well. As streamflow timing and availability shift with climate change, irrigation, hydropower and municipal water users are increasingly coming into conflict as the volume of water available no longer matches when water is needed (and permitted for). This is exacerbated by the subordination of hydropower production in the YRB over the last 20 years in favor of meeting water demands for agricultural production and in-stream flow requirements for endangered fish. Other concerns include agribusiness runoff affecting water quality and lawn watering systems putting heavy demand on available water (Yakama Nation, 2016). Today, the Yakima Basin Integrated Plan (YBIP) aims to balance competing needs for the river (USBR and State of Washington Department of Ecology, 2012). The YBIP contains elements related to improving fish passage and habitat, increasing and enhancing surface and groundwater storage, implementing market-based water reallocation programs, and promoting water conservation (State of Washington Department of Ecology, 2013; S. 714 amendment to Public Law 103–434). The plan while contentious due in part to concerns by tribes about the impacts and benefits of additional infrastructural development on fish survivability and cultural heritage sites, has progressed into the first of three phases for the $3.8 billion, 30-year Plan (State of Washington Department of Ecology., 2018). Some of the YBIP's main aims are to assure water reliability for the Yakama Nation and to create a consensus-based management approach that provides an alternative to litigation (S. 714 amendment to Public Law 103–434).
Integrating the Social Into the FEW Nexus and Modeling
The YRB case demonstrates the value of considering how the social is integrated into FEW nexus research. Rather than thinking of the resilience of this system as characterized by a return to equilibrium, the social component represents ongoing change over time. Furthermore, inequalities shape the system and inequality is an outcome. In terms of social justice and “resilience for whom,” system change had negative ramifications for tribal groups and non-human species such as the salmon, while others prospered, albeit unevenly, from agricultural revenues. The question “resilience of what,” or questions of system function, highlights inequalities in power to shape the prioritization of valued functions. For example, we can see the role that powerful agricultural played in policy development and passage of laws regarding multiple aspects of the FEW system- migration to meet labor needs, the building of dams for irrigation and energy, or in the designation of water rights.
In addition to encouraging a deeper consideration of how resilience is defined, an incorporation of the social allows researchers to explore questions such as: Do these unequal outcomes mean the FEW nexus is less resilient in certain aspects? Would the system as a whole be more resilient if there was less inequality in process and/or outcome? Further, are these changes over time accurately characterized as innovations to increase resilience? Also, when should they be considered adaptations vs. system changes? For example, some affected parties might characterize YRB FEW nexus changes as adaptation, while others, such as the tribal groups who experienced drastic alterations in their lives and cultures, might perceive this as system change. External forces, including colonization, war, and market pressures, also highlight the complexity of delineating system boundaries and forces across scales.
The YRB case study also demonstrates the social is both driver and outcome of the FEW nexus, shaping how we conceptualize and model FEW nexus resilience and sustainability. A social science lens allows researchers to ask, how do these inequalities shape our assessment of FEW nexus resilience? The CCF literature highlights how livelihoods, well-being, and the various capitals are not only outcomes, but also drivers that shape future options (Emery and Flora, 2006). Clearly, social forces were key in driving the development of the FEW nexus in this region and they continue to shape the region today. The presence or absence of adaptive governance practices, emphasized in the socio-ecological resilience literature (Folke et al., 2005), or common pool resource governance practices, described by Ostrom (1990), draw attention to inequality in process and how it affects uneven outcomes in terms of resource access. Examples include access to fish species and water resources (Cosens et al., 2018). Additionally, these processes link to uneven outcomes in terms of livelihoods and well-being for some groups in the region. Negative social ramifications are especially important to consider when designing innovations for improving FEW nexus resilience or modeling innovation outcomes, in order to make interventions more just and accurate and to help avoid unintended consequences of proposed changes.
This case study provides a structure from which we can start parsing the social and biophysical together into a unified modeling effort. While the modeling effort is on-going, below we highlight how such social frameworks and indicators may be integrated to model the FEW nexus.
Conceptual Mapping the Evolution of the FEW Nexus Over Time
Building on the information presented in the Yakima case study, we use a type of conceptual map (causal loop diagrams) to show how YRB socio-ecological systems have developed over time and what factors dominate. A timeline (Figure 2) highlights the introduction of innovations, especially government policies, in which affected groups had unequal access to shape processes and changes had lasting societal impacts. Figure 3 shows the development of linkages and feedbacks in the Yakima River Basin. In this figure, a series of successive conceptual maps depict simplified linkages and feedbacks related to these trends which demonstrate a (A) pre-1900s era, (B) 1900-1950s era, and (C) 1950s-2000s era management of the water available. The initial social-ecological system in Figure 3A revolves around the boundaries and scale of the Yakama Nations in the YRB. The local FEW system was defined by the water availability that contributed to habitats for large populations of anadromous fish, including salmon and steelhead, which produced local food security and social and cultural heritage (Montag et al., 2014). Resilience of this food resource benefited the tribes' and bands' well-being in 4.6 million ha of the Lower CRB. This food system's resilience and change is illustrated in the subsequent eras (Figures 3B,C).
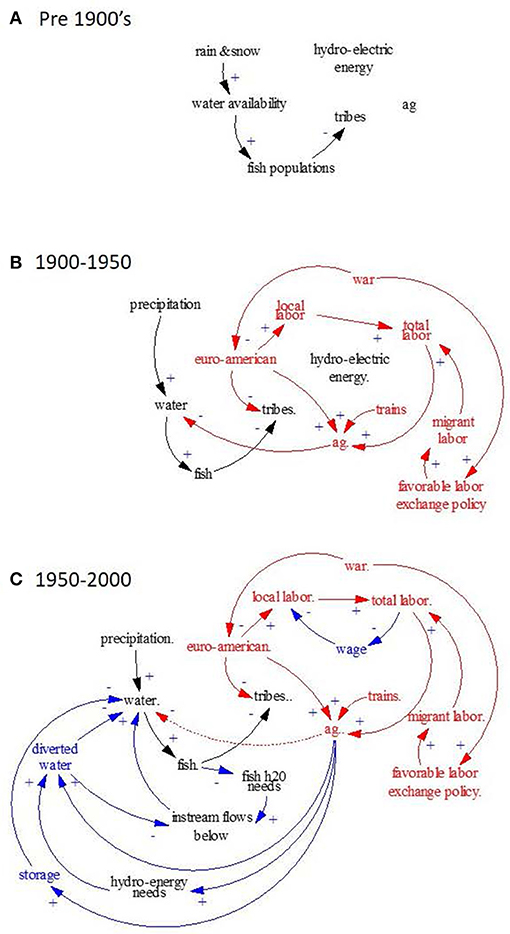
Figure 3. Development of linkages and feedbacks in the Yakima River Basin. (A) Pre 1990, (B) 1900-1950, and (C) 1950-2000.
After ceding 90% of this land to the U.S. government and with the increase in Euro-American settlers into the region (Figure 3B), a new FEW system began intensely affecting the previous one with the rising dominance of irrigated agriculture. Washington apple production in particular begin to cause rising tensions related to social justice and conflicting goals for food systems. Federal policies and actions privileged the production of apples for shipment to Hong Kong, Honolulu, and Europe by 1900 (Jarosz and Qazi, 2000) effectively exporting water to global consumers and in contrast to the previous food system developed for local consumption. Similarly, many other regional agricultural products were produced for national and international markets. This caused regional social shifts, such as decreasing the presence and power of the Yakama nations and increasing Euro-American settlers' influence, and demographic shifts, such as increasing the Mexican migrant labor population. Salmon populations significantly decreased and global shocks begin to have more direct impact via war-time policies and international agricultural market competition.
The 1950s to 2000s era (Figure 3C) saw further interaction between the local FEW system and the export-driven FEW system. Hydropower and diversion rules that decreased the supply and quality of instream flows were established, however legal measures also became available to require the water needed for endangered anadromous fish.
Figure 3 demonstrates that throughout these three periods, the questions of “resilience for whom?” and “of what?” shifted from a focus on local residents' sustenance to export-driven economic development and finally to more emphasis on market forces, management of resources, and an attention to the incorporation of multiple stakeholders enabled through legal mechanisms4. We do not mean to suggest that this is either a desirable or undesirable change, nor that it necessarily results in a decline in overall local resilience. We simply aim to suggest that modeling resilience in this way can incorporate social factors, and that the best conceptualizations of resilience take into account the uneven impacts, impacts at various scales and across boundaries, and multiple definitions of and desired pathways to resilient situations for different groups.
Conceptual Maps of the FEW System That Further Incorporate the Social
The timeline conceptual maps in Figure 3 show how conflicting goals for multiscale FEW systems can shape which groups dominate both processes and outcomes, and how these have lasting impacts by shaping future opportunities. Next, we incorporate more components, both biophysical and social, into a map of the current YRB FEW system (Figure 4). Figure 4 is a conceptual map of FEW system demands and allocations based on distribution between users that prioritize water for development (D) and users that prioritize water for fish and ecosystem function (EF). To create this we build on several modeling efforts that address various components. For example, Ericksen (2008) models aspects of food systems that contribute to food security; this can be adapted to include energy and water security and considerations of livelihoods, well-being, and elements of the CCF. Nandalal and Simonovic (2003) create a conceptualization where the power with which a group of stakeholders is able to employ to pursue their interests results in a decreased allocation for the neighboring stakeholder. Loops are negative when the variable dynamics balance each other or positive when they are reinforcing and leading to positive feedbacks. This model assumes an ultimate equilibrium dependent on the aspirations and the weight of the fight in each group (in what can be seen as the balancing loops of B2 and B3, for ecosystem function and development priorities, respectively). While built upon clearly limiting assumptions, this serves to highlight the fundamental social interactions that are at the basis of the FEW nexus. Other modeling efforts show that while increased water utilization could encourage the development and growth of a community (Loops R1, R2, R3), this is limited by resource availability (Ghashghaie et al., 2014) which in this case is demonstrated through the reservoir water balancing loop (B1). Figure 4 represents our attempt to build on these previous models and incorporate attention to the social as both input and output of FEW system mapping. For example, in Figure 4 one section of the conceptual map indicates how governance plays a role in introducing policies that ultimately negotiate and prioritize FEW security of various stakeholders as well as how their satisfaction and aspirations (e.g., livelihood, well-being) create feedbacks. Starting with archetypes of resource allocation (Loops B1, R1,R2,R3) and combining with resource conflict (Loops B2, B3) demonstrates a adjustable, specific, and reproducible methodology that has been called for in nexus assessment (Ghashghaie et al., 2014; Albrecht et al., 2018).
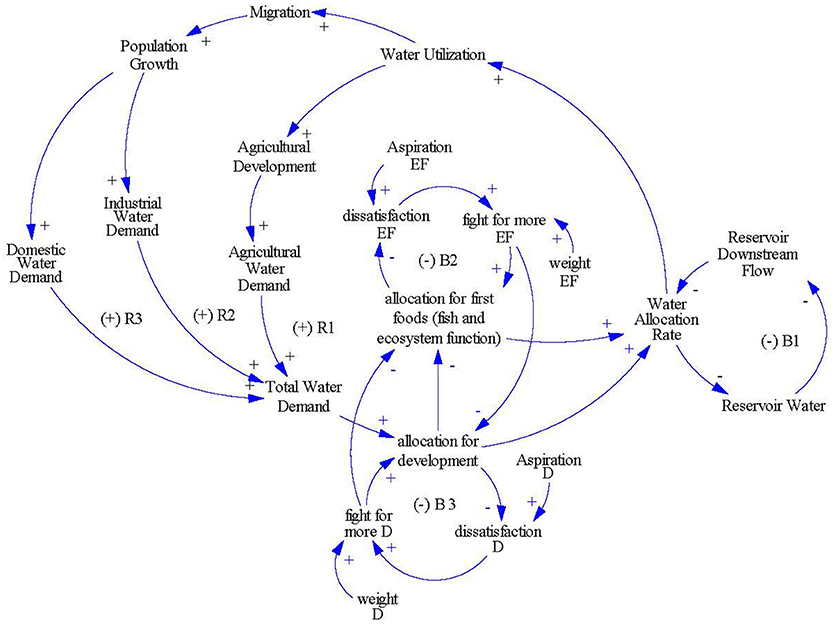
Figure 4. Conceptual map of FEW system demands and allocations based on distribution between users that prioritize water for development (D) and users that prioritize water for fish and ecosystem function (EF). R and B represent positive and negative feedback loops.
Our next conceptual map shown in Figure 5 then incorporates the CCF, including financial, built, political, social, human, cultural, and natural capital (Flora et al., 2004, 2005). In this conceptual map that incorporates the capitals framework into FEW system mapping, green text highlights ecosystem functions and blue text indicates development aspects within the Yakima River Basin. Pink text demonstrates where community capitals influence interactions in the FEW nexus, and red text shows areas that can influence intensification or alleviation of frictions. In conceptualizing the structure of the system, this map can assist researchers in evaluating what shapes the resilience of components of the system. This set of feedbacks and loops is an initial visualization of where certain policies have potential to increase or decrease frictions between diverse stakeholder groups within the FEW nexus. It also helps inform what dynamics are behind incentives to compete or cooperate. Modeling social-ecological system complexities in a way that allows us to form multiple testable hypotheses is vital to evaluating resilience (Gunderson, 2003). Figure 5 demonstrates how the CCF can be integrated into a model that allows for testing of multiple hypotheses, especially related to proposed system innovations. For instance, if aquifer storage and recovery is developed within the Yakama Nation (S. 714 amendment to Public Law 103–434), this might allow natural capital to be amassed, leading to a decreased need to fight for more water. If this innovation combines with a shift in norms in the YRB away from landscaping requiring watering this could further alleviate water demand and conflict.
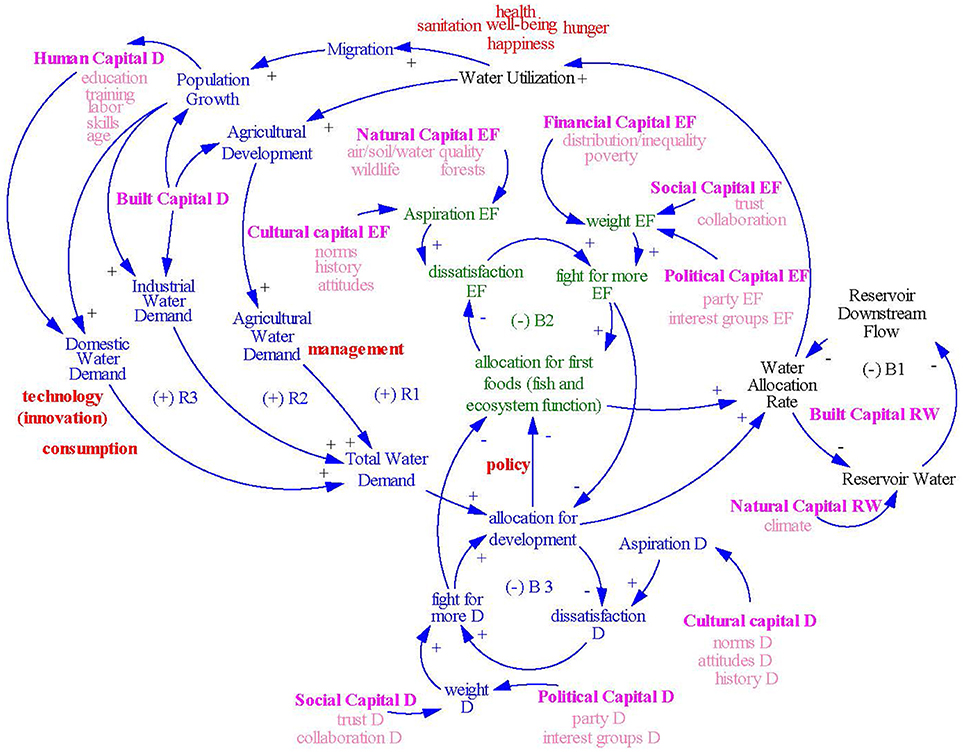
Figure 5. Conceptual map of incorporated capitals into FEW systems with green text highlighting ecosystem functions and blue text indicating development aspects within the Yakima River Basin. Pink text demonstrates where community capitals influence interactions in the FEW nexus and red text shows areas that can influence intensification or alleviation of frictions.
Integrated Modeling for Improved Management of the YRB FEW Nexus
After expanding our understanding of social aspects of the FEW nexus through conceptual mapping, we incorporate these insights into an existing object-oriented river basin modeling system. Figure 6 shows a conceptual map of interaction among FEW systems and society in the YRB. This conceptualization lays the groundwork and provides a roadmap toward developing a simulation tool that can answer more profound questions related to FEW-society interactions. The YAK-RW (left panel) has been developed and used to simulate the river system processes (FEW systems) over the YRB (Carron et al., 2000; Zagona et al., 2001); in the right panel, examples of the community capital frameworks that have been presented in past studies (e.g., Emery and Flora, 2006) are included to build upon work discussing the characteristics of connections between FEW and society (D'Odorico et al., 2018). YAK-RW (Yakima RiverWare) is a case-specific implementation built on the RiverWare generic platform (Zagona et al., 2001), a platform system that, similar to System Dynamics, simulates river system processes through stocks and flows such as streamflow movement, dam operations, irrigation and municipal diversions, and surface-ground water interaction (Figure 6, left side). YAK-RW has been widely used to explore how stressors such as climate change and farmers' adaptation decisions such as farm-level water conservation (Malek et al., 2016) modify the FEW nexus. The model has also been used to assess how infrastructural developments such as building a new dam [USBR (US Bureau of Reclamation), 2008; USBR, 2012, 2018; Yoder et al., 2017] can affect the economy of FEW sectors including agriculture, hydropower, and fisheries. Below, we draw upon YAK-RW's recent application in the YRB to highlight salient social impacts and feedbacks related to water-saving technologies. Other research could incorporate social considerations into similarly developed SD models.
The YRB has been historically sensitive to droughts and is projected to experience more frequent and severe droughts in the future (Elsner et al., 2010; Vano et al., 2010; Malek et al., 2018). Malek et al. (Forthcoming) explore how future droughts provide financial incentives for farmers to switch to more-efficient irrigation systems. The authors used YAK-RW to model the compound impacts of climate change and farm-level irrigation decisions in the YRB. Results indicate the economy of the agricultural sector improves under more-efficient irrigation scenarios and the agricultural sector as a whole will be more resilient to droughts. Moreover, streamflow increases in some of vulnerable areas of the YRB. The increase in streamflow facilitates salmonid outmigration. However, while the economy of the energy sector is smaller than other sectors, it declines when irrigation systems are more efficient. This suggests that irrigation technology innovations have conflicting impacts across FEW sectors and may create cross sector conflicts.
Ongoing research (e.g., Malek et al., Forthcoming) also addresses the impacts of FEW-related changes in the YRB such as farm-level investment in greater irrigation efficiency, by identifying the “winners and losers” within the agricultural sector and rural communities. Results indicate that growers of high-value crops (e.g., grapes, cherries, apples) and multiple-cutting crops (e.g., alfalfa) benefit the most from the way their yields respond to the compound effects of climate change and improvements in water use efficiency. However, investment is not viable for growers of annual crops (e.g., corn, potatoes, and wheat) which currently make up about 23% of total farmed areas in the YRB (Malek et al., Forthcoming). Furthermore, farmers' decisions depend on several factors, such as age, education, risk appetite, gender, and familiarity with new systems (Gardebroek and Lansink, 2004; Crane et al., 2011; Viscusi et al., 2011; Taylor and Zilberman, 2017). For example, older farmers might be more reluctant to invest because it could be more challenging for them to adopt new techniques, or the investment horizon might be longer than their perception of their own lifespans (Wang and Hanna, 1998). Moreover, efficient irrigation systems that are installed usually degrade the quality of return flow, which is a key component of water supply for downstream users (Bliesner et al., 1977; Causapé et al., 2004). Therefore, efficient irrigation systems might lead to additional socio-economic complications through lower quality of return flow.
This research on irrigation efficiency and the broader social implications demonstrates that although efficient irrigation systems cause an overall improvement in the agricultural economy of the YRB and make the economy of the basin more resilient to water stress (i.e., droughts) other interests are affected and need to be considered. This includes asking the questions: resilience for whom and of what? For example, a social science approach allows us to ask how labor demand is affected, since research shows that reduction in labor demand can lead to adverse outcomes for rural economy (Jobbins et al., 2015). We also see that a single economic indicator (e.g., improvement in overall or sectoral economy of FEW systems) oversimplifies the conflicting dynamics, such as those between instream stakeholders (e.g., tribes) and out-of-stream stakeholders (e.g., farmers) or even between farmers of different types of crops. Furthermore, local socioeconomic and biophysical interactions are complex and generalizations across time and space must be completed carefully. No single best management practice (e.g. farm-level water conservation) that benefits all parties may exist, but an inclusive and transparent policy-making process can lead to a mutually understandable plan that minimizes conflicts and eventually creates a lasting self-organized and self-governed system (Ostrom, 2009).
Our conceptual maps and integrated modeling efforts are useful in the planning phases of large decision-making processes. The YAK-RW YRB example (Figure 6, left side) shows how the components of the FEW system are represented in the YAK-RW. Despite its strength simulating FEW system components, though, YAK-RW cannot capture the ways that society interacts with FEW systems. The middle panel of Figure 6 shows several socioeconomic factors that could be used to characterize the feedback process between FEW systems and society. The right panel shows components of the CCF that could be integrated. The implementation of these factors, however, is still cumbersome and hindered by data issues. Our model presented in Figure 6 represents progress to date in capturing how shifts in FEW systems affect society and vice versa, this can inform YAK-RW and other modeling moving forward.
Conclusions
FEW nexus research is a complex and highly interdisciplinary endeavor. This paper demonstrates the complexity, but also the importance, of considering social factors when examining FEW nexus resilience. We find, via historical case analysis and conceptual modeling over time, that FEW management decisions in the YRB led to starkly unequal outcomes. This demonstrates that a resilience focus that does not incorporate insights from the social sciences may unintentionally privilege a status quo created by those in power. This structures unequal opportunities, limits diverse understandings of resilience, and may have real impacts on FEW nexus sustainability. Considerations of the social enable us to ask questions about inequality, power, and multiple indicators of resilience, and may productively shift how we conceptualize FEW nexus sustainability. To encourage the incorporation of social factors, we highlight complexities to address. We then provide examples of multiple approaches to incorporating the social, including ones that focus on participatory processes and others that focus on indicators for incorporation, as both drivers and outcomes, in conceptualizations and modeling of systems at multiple scales. We then use these in conceptual and system dynamics modeling. While our research focuses on the YRB within the CRB, it is applicable to other FEW systems around the world. Including considerations of social drivers and social outcomes improves research by making it more accurate in that it is (1) a more holistic conceptualization of reality, (2) more likely to produce desired advances, such as innovation adoption, and avoid unintended negative consequences, and (3) more just, by taking into account inequalities in the FEW system.
While some research methodologies such as the case study approach facilitate considering the social, conceptual mapping and attempts at bringing the social into a modeling system such as RiverWare highlight complexities and data needs. We need data that enable us to explore questions such as how do inequalities in power to shape process and outcomes matter to various conceptualizations of resilience and FEW system outcomes? And, how do system inequalities affect different groups and various aspect of resilience and sustainability? Modeling allows us to explore these relationships. While conceptual mapping does not require data, it, along with suggested framework and indicators, draws attention to important concepts to consider and thus it points to data needs. We need data at multiple scales and time points and data that are compatible across biophysical and social systems. For example, in the YRB better data at multiple scales, collected over time, on water rights allocations, prorationing, litigation, and cultural damages would allow us to explore how inequalities (e.g., cultural or between senior and junior water users) develop and evolve, and how different innovations may directly or indirectly impact these relationships. This dearth of data is a challenge to accurately modeling socio-ecological systems. Despite these difficulties, we maintain that the social science frameworks presented, including those that capture livelihoods, well-being, and community capitals, offer productive ways forward to incorporate important social components into FEW nexus research.
Author Contributions
JG, JP, CG, and KM contributed to the conception and design of the study. JG wrote the first draft of the manuscript, and took the lead in overall organization, on all subsequent drafts and revisions, and on writing the sections that pertain to the social science aspects of the research. JP and CG took the lead on the YRB case study section and JP, CG, and KM took the lead on the modeling sections. All authors contributed to manuscript revision and read and approved the submitted version.
Conflict of Interest Statement
The authors declare that the research was conducted in the absence of any commercial or financial relationships that could be construed as a potential conflict of interest.
Acknowledgments
We would like to thank the Columbia River FEW team composed of researchers from Washington State University, University of Idaho, Utah State University, and Pacific Northwest National Laboratories for their collaboration, research support, and helpful discussions in formulating conceptualizations of FEW systems and resilience. Funding for this work was provided by: NSF EAR #1639458 and USDA #2017-67004-26131.
Footnotes
1. ^Resilience researchers occasionally acknowledge that there is not necessarily an implication that resilience is good, because it could get in the way of replacing an undesirable system (Folke, 2006) and sometimes change is desired (Walker et al., 2004). Despite such acknowledgments, much of the writing about resilience assumes it is desirable.
2. ^Related research calls attention to well-being of nonhuman species and the ecosystem as a whole; for example, the Coupled Human and Natural Systems (CHANS) literature sets forth a research agenda on systems in which human and natural aspects interact (Liu et al., 2007a,b).
3. ^There are some issues with the capitals framework. First, Dietz (2015: p. 134) expresses a preference to “reserve the term capital for resources that are used with the expectation of a positive return on investment—a profit” and recommends use of the term resources unless there is a specific intention to use the resource to generate exchange value. Second, Bourdieu's initial conceptualization in the 1980s of the multiple forms of capital highlighted their role in producing and reproducing inequality (Bourdieu, 1986; Schor et al., 2016).
4. ^Key features of governance in the region are dominated by legislation and acts of Congress that enable feasibility studies for enhancement projects (93 Stat. 1241, Public Law 96-162) and later funding to implement mitigation (phase 1; 98 Stat. 1333, Public Law 98-381; 98 Stat. 1379, Public Law 98-396) and conservation (phase 2; Title XII 108 Stat. 4550, Public Law 103-434) (USBR, 2018). While legal recognition and facilitation is enabling the space for reconciliation of highly developed river systems with ecosystem functions (Cosens et al., 2018), significant effort is needed to continue to promote adaptive governance that includes effects on stakeholders' well-being (Montag et al., 2014). With phase 3 of the Yakima River Basin Enhancement Project being authorized in 2017 (S. 714 amendment to Public Law 103–434), $3.8 billion has been allotted toward collaboration that enhances long-term resiliency.
References
Adger, W. N., Hughes, T. P., Folke, C., Carpenter, S. R., and Rockström, J. (2005). Social-ecological resilience to coastal disasters. Science 309, 1036–1039. doi: 10.1126/science.1112122
Albrecht, T. R., Crootof, A., and Scott, C. A. (2018). The Water-Energy-Food Nexus: a systematic review of methods for nexus assessment. Environ. Res. Lett. 13:043002. doi: 10.1088/1748-9326/aaa9c6
Alessa, L., Kliskey, A., Gosz, J., Griffith, D., and Ziegler, A. (2018). MtnSEON and social–ecological systems science in complex mountain landscapes. Front. Ecol. Environ. 16, S4–S10. doi: 10.1002/fee.1753
Al-Saidi, M., and Elagib, N. A. (2017). Towards understanding the integrative approach of the water, energy and food nexus. Sci. Tot. Environ. 574, 1131–1139. doi: 10.1016/j.scitotenv.2016.09.046
Arrow, K. J., Keohane, R. O., and Levin, S. A. (2012). Elinor Ostrom: an uncommon woman for the commons. Proc. Natl. Acad. Sci. U.S.A. 109, 13135–13136. doi: 10.1073/pnas.1210827109
Berkes, F., Colding, J., and Folke, C. (eds). (2003). Navigating Social-Ecological Systems. Cambridge: Cambridge University Press.
Biggs, E. M., Bruce, E., Boruff, B., Duncan, J. M., Horsley, J., Pauli, N., et al. (2015). Sustainable development and the water–energy–food nexus: a perspective on livelihoods. Environ. Sci. Policy 54, 389–397. doi: 10.1016/j.envsci.2015.08.002
Biggs, E. M., and Watmough, G. R. (2012). A community-level assessment of factors affecting livelihoods in Nawalparasi District, Nepal. J. Int. Dev. 24, 255–263. doi: 10.1002/jid.1844
Bliesner, R. D., Hanks, R. J., King, L. G., and Willardson, L. S. (1977). Effects of irrigation management on the quality of irrigation return flow in Ashley Valley, Utah. Soil Sci. Soc. Am. J. 41, 424–428. doi: 10.2136/sssaj1977.03615995004100020051x
Bourdieu, P. (1986). “The forms of capital,” in Handbook of Theory and Research for the Sociology of Education, ed J. G. Richardson (New York, NY: Greenwood), 241–258.
Bourgeron, P., Kliskey, A., Alessa, L., Loescher, H., Krauze, K., Virapongse, A., et al. (2018). Understanding large-scale, complex, human–environmental processes: a framework for social–ecological observatories. Front. Ecol. Environ. 16, S52–S66. doi: 10.1002/fee.1797
Carron, J. C., Zagona, E. A., and Fulp, T. J. (2000). Uncertainty Modeling in RiverWare. In Watershed Management and Operations Management 2000, 1–11. American Society of Civil Engineers. Available online at: http://ascelibrary.org/doi/abs/10.1061/40499%282000%2958.
Castelletti, A., and Soncini-Sessa, R. (2007). Bayesian Networks and participatory modelling in water resource management. Environ. Model. Softw. 22, 1075–1088. doi: 10.1016/j.envsoft.2006.06.003
Causapé, J., Quílez, D., and Aragüés, R. (2004). Irrigation efficiency and quality of irrigation return flows in the Ebro river basin: an overview. Environ. Monit. Assess. 117, 451–461. doi: 10.1007/s10661-006-0763-8
Chaffin, B. C., Garmestani, A. S., Gunderson, L. H., Benson, M. H., Angeler, D. G., Arnold, C. A., et al. (2016). Transformative environmental governance. Annu. Rev. Environ. Resour. 41, 399–423. doi: 10.1146/annurev-environ-110615-085817
Chen, X., Frank, K. A., Dietz, T., and Liu, J. (2012). Weak ties, labor migration, and environmental impacts: toward a sociology of sustainability. Org. Environ. 25, 3–24. doi: 10.1177/1086026611436216
Chuang, W. C., Garmestani, A., Eason, T. N., Spanbauer, T. L., Fried-Petersen, H. B., Roberts, C. P., et al. (2018). Enhancing quantitative approaches for assessing community resilience. J. Environ. Manage. 213, 353–362. doi: 10.1016/j.jenvman.2018.01.083
Cosens, B. (2010). Transboundary River governance in the face of uncertainty: resilience theory and the Columbia River Treaty. Univ. Utah J. Land Resour. Environ. Law 30, 229–265.
Cosens, B. (2016). The Columbia River Treaty: an opportunity for modernization of basin governance. Colorado Nat. Res. Energy Environ. Law Rev. 27, 1–19.
Cosens, B., and Fremier, A. (2018). “Social-ecological resilience in the columbia river basin: the role of law and governance,” in Practical Panarchy for Adaptive Water Governance eds B. Cosens and L. Gunderson (Cham: Springer).
Cosens, B., and Gunderson, L. (eds.). (2018). Practical Panarchy for Adaptive Water Governance. Cham: Springer International.
Cosens, B., McKinney, M., Paisley, R., and Wolf, A. T. (2018). Reconciliation of development and ecosystems: the ecology of governance in the International Columbia River Basin. Reg. Environ. Change. 18, 1679–1692. doi: 10.1007/s10113-018-1355-1
Cote, M., and Nightingale, A. J. (2012). Resilience thinking meets social theory: situating social change in socio-ecological systems (SES) research. Prog. Hum. Geogr. 36, 475–489. doi: 10.1177/0309132511425708
Coyle, G. (2000). Qualitative and quantitative modelling in system dynamics: some research questions. System Dynamics Rev. 16, 225–244. doi: 10.1002/1099-1727(200023)16:3<225::AID-SDR195>3.0.CO;2-D
Crane, T. A., Roncoli, C., and Hoogenboom, G. (2011). Adaptation to climate change and climate variability: the importance of understanding agriculture as performance. NJAS - Wagening. J. Life Sci. 57, 179–185. doi: 10.1016/j.njas.2010.11.002
Curtin, C. G., and Parker, J. P. (2014). Foundations of resilience thinking. Conserv. Biol. 28, 912–923. doi: 10.1111/cobi.12321
Darian, L. (2006). The emerging Hispanic homeland of the Pacific Northwest- A case study of Yakima Valley, Washington. Thesis, Bozeman, MT: Montana State University.
Davidson, D. J. (2010). The applicability of the concept of resilience to social systems: some sources of optimism and nagging doubts. Soc. Nat. Res. 23, 1135–1149. doi: 10.1080/08941921003652940
Davidson, J., Jacobson, C., Lyth, A., Dedekorkut-Howes, A., Baldwin, C., Ellison, J., et al. (2016). Interrogating resilience: toward a typology to improve its operationalization. Ecol. Soc. 21:27. doi: 10.5751/ES-08450-210227
Dietz, T. (2015). Prolegomenon to a structural human ecology of human well-being. Soc. Dev. 1, 123–148. doi: 10.1525/sod.2015.1.1.123
Dietz, T. (2017). Drivers of human stress on the environment in the twenty-first century. Annu. Rev. Environ. Res. 42, 189–213. doi: 10.1146/annurev-environ-110615-085440
Dietz, T., Ostrom, E., and Stern, P. C. (2003). The struggle to govern the commons. Science 302, 1907–1912. doi: 10.1126/science.1091015
Dietz, T., Rosa, E. A., and York, R. (2009). Environmentally efficient well-being: rethinking sustainability as the relationship between human well-being and environmental impacts. Hum. Ecol. Rev. 16, 114–123. Available online at: https://www.jstor.org/stable/24707742
Dietz, T., Rosa, E. A., and York, R. (2012). Environmentally efficient well-being: Is there a Kuznets curve? Appl. Geogr. 32, 21–28. doi: 10.1016/j.apgeog.2010.10.011
Diver, S. (2018). Native water protection flows through self-determination: understanding tribal water quality standards and “treatment as a state”. J. Contemp. Water Res. Educ. 163, 6–30. doi: 10.1111/j.1936-704X.2018.03267.x
D'Odorico, P., Davis, K. F., Rosa, L., Carr, J. A., Chiarelli, D., Dell'Angelo, J., et al. (2018). The global food-energy-water nexus. Rev. Geophys. 56, 1–76. doi: 10.1029/2017RG000591
Donoghue, E. M., and Sturtevant, V. E. (2007). Social science constructs in ecosystem assessments: revisiting community capacity and community resiliency. Soc. Nat. Resour. 20, 899–912. doi: 10.1080/08941920701561114
Donohue, C., and Biggs, E. (2015). Monitoring socio-environmental change for sustainable development: developing a Multidimensional Livelihoods Index (MLI). Appl. Geogr. 62, 391–403. doi: 10.1016/j.apgeog.2015.05.006
Elsner, M. M., Cuo, L., Voisin, N., Deems, J. S., Hamlet, A. F., Vano, J. A., et al. (2010). Implications of 21st century climate change for the hydrology of Washington State. Clim. Change 102, 225–260. doi: 10.1007/s10584-010-9855-0
Emery, M., and Flora, C. (2006). Spiraling-up: mapping community transformation with community capitals framework. Commun. Dev. 37, 19–35. doi: 10.1080/15575330609490152
Endo, A., Tsurita, I., Burnett, K., and Orencio, P. M. (2017). A review of the current state of research on the water, energy, and food nexus. J. Hydrol. Reg. Stud. 11, 20–30. doi: 10.1016/j.ejrh.2015.11.010
Ericksen, P. J. (2008). Conceptualizing food systems for global environmental change research. Global Environ. Chang. 18, 234–245. doi: 10.1016/j.gloenvcha.2007.09.002
Fernandez, I. C., Manuel-Navarete, D., and Torres-Salinas, R. (2016). Breaking resilient patterns of inequality in Santiago de Chile: challenges to navigate towards a more sustainable city. Sustainability 8, 820. doi: 10.3390/su8080820
Flora, C., Flora, J., and Fey, S. (2004). Rural Communities: Legacy and Change, 2nd Edn. Boulder, CO: Westview Press.
Flora, C. B., Emery, M., Fey, S., and Bregendahl, C. (2005). Community Capitals: A Tool for Evaluating Strategic Interventions and Projects. Ames, IA: North Central Regional Center for Rural Development. Available online at: https://naaee.org/sites/default/files/204.2-handout-community-capitals.pdf
Flora, J. L., Sharp, J., Flora, C., and Newlon, B. (1997). Entrepreneurial social infrastructure and locally initiated economic development in the nonmetropolitan united states. Sociol. Q. 38, 623–645.
Folke, C. (2006). Resilience: the emergence of a perspective for social-ecological systems analyses. Glob. Environ. Change 16, 253–267. doi: 10.1016/j.gloenvcha.2006.04.002
Folke, C., Hahn, T., Olsson, P., and Norberg, J. (2005). Adaptive governance of social-ecological systems. Annu. Rev. Environ. Resour. 30, 441–473. doi: 10.1146/annurev.energy.30.050504.144511
Gamboa, E., and Leonard, K. (1990). Mexican Labor and World War II: Braceros in the Pacific Northwest 1942-1947. Austin, TX: University of Texas Press.
Gardebroek, C., and Lansink, A. G. J. M. O. (2004). Farm-specific adjustment costs in Dutch pig farming. J. Agric. Econ. 55, 3–24. doi: 10.1111/j.1477-9552.2004.tb00076.x
Gendaszek, A. S., Ely, D. M., Hinkle, S. R., Kahle, S. C., and Welch, W. B. (2014). Hydrogeologic framework and groundwater/surface-water interactions of the upper Yakima River Basin, Kittitas County, central Washington (USGS Numbered Series No. 2014–5119). Scientific Investigations Report. U.S. Geological Survey, Reston, VA.
Ghashghaie, M., Marofi, S., and Marofi, H. (2014). Using system dynamics method to determine the effect of water demand priorities on downstream flow. Water Resour. Manage. 28, 5055–5072. doi: 10.1007/s11269-014-0791-z
Givens, J. E. (2017). World society, world polity, and the carbon intensity of well-being, 1990–2011. Soc. Dev. 3, 403–435. doi: 10.1525/sod.2017.3.4.403
Givens, J. E. (2018). Ecologically unequal exchange and the carbon intensity of well-being, 1990-2011. Environ. Soc. 4, 1–14. doi: 10.1080/23251042.2018.1436878
Glantz, M. H. (1982). Consequences and responsibilities in drought forecasting: the case of Yakima, 1977. Water Resour. Res. 18, 3–13.
Griffith, D. L., Alessa, L., and Kliskey, A. (2018). Community-based observing for social–ecological science: lessons from the Arctic. Front. Ecol. Environ. 16, S44–S51. doi: 10.1002/fee.1798
Gunderson, L. H. (2000). Ecological resilience—In theory and application. Annu. Rev. Ecol. Syst. 31, 425–439. doi: 10.1146/annurev.ecolsys.31.1.425
Gunderson, L. H. (2003). “Adaptive dancing: interactions between social resilience and ecological crises,” in Navigating Social-Ecological Systems, eds F. Berkes, J. Colding, and C. Folke (Cambridge: Cambridge University Press), 33–52.
Gunderson, L. H., and Holling, C. S. (2002). Panarchy: Understanding Transformations in Systems of Humans and Nature. Washington, DC: Island Press.
Gunnier, M. (2008). The History and Contemporary Role of the Lyle Falls Dip net Fishery in Yakama Economy and Culture. Thesis, Central Washington University.
Harris, C., McLaughlin, W., Brown, G., and Becker, D. R. (2000). Rural Communities in the Inland Northwest: An Assessment of Small Rural Communities in the Interior and Upper Columbia River Basins. Gen. Tech. Rep. PNW-GTR-477. USDA Forest Service, Pacific Northwest Research Station, and USDI Bureau of Land Management, Portland, OR.
Hatt, K. (2013). Social attractors: a proposal to enhance “resilience thinking” about the social. Soc. Nat. Resour. 26, 30–43. doi: 10.1080/08941920.2012.695859
Haynes, R. W., Graham, R. T., and Quigley, T. M. (1996). A Framework for Ecosystem Management in the Interior Columbia Basin Including Portions of the Klamath and Great Basins. Gen. Tech. Rep. PNW-GTR-374. USDA Forest Service, Pacific Northwest Research Station, Portland, OR.
Heemskerk, M., Wilson, K., and Pavao-Zuckerman, M. (2003). Conceptual models as tools for communication across disciplines. Conserv. Ecol. 7:8. doi: 10.5751/ES-00554-070308
Hornborg, A. (2006). Footprints in the cotton fields: the Industrial Revolution as time–space appropriation and environmental load displacement. Ecol. Econ. 59, 74–81. doi: 10.1016/j.ecolecon.2005.10.009
Howarth, C., and Monasterolo, I. (2016). Understanding barriers to decision making in the UK energy-food-water nexus: the added value of interdisciplinary approaches. Environ. Sci. Policy 61, 53–60. doi: 10.1016/j.envsci.2016.03.014
Hunn, E. S. (1990). Nci'i-Wana. “The Big River”: mid-Columbia Indians and their land. Seattle, WA: University of Washington Press.
Huszar, P. C., Radosevich, G. E., Skogerboe, G. V., Trock, W. L., and Vlachos, E. C. (1978). Socio-Economic and Institutional Factors in Irrigation Return Flow Quality Control. Vol. 2 Yakima Valley Case Study. U.S. Environmental Protection Agency, EPA-600/2-78-174b.
Jarosz, L., and Qazi, J. (2000). The geography of Washington's world apple: global expressions in a local landscape. J. Rural Stud. 16, 1–11. doi: 10.1016/S0743-0167(99)00028-5
Jobbins, G., Kalpakian, J., Chriyaa, A., Legrouri, A., and El Mzouri, E. H. (2015). To what end? Drip irrigation and the water–energy–food nexus in Morocco. Int. J. Water Resour. Dev. 31, 393–406. doi: 10.1080/07900627.2015.1020146
Jorgenson, A. K. (2014). Economic development and the carbon intensity of human well-being. Nat. Climate Change. 4, 186. doi: 10.1038/nclimate2110
Jorgenson, A. K. (2016). Environment, development, and ecologically unequal exchange. Sustainability 8, 227. doi: 10.3390/su8030227
Jorgenson, A. K., Alekseyko, A., and Giedraitis, V. (2014). Energy consumption, human well-being and economic development in central and eastern European nations: A cautionary tale of sustainability. Energy Policy 66, 419–427. doi: 10.1016/j.enpol.2013.11.020
Jorgenson, A. K., and Clark, B. (2009). The economy, military, and ecologically unequal exchange relationships in comparative perspective: a panel study of the ecological footprints of nations, 1975−2000. Soc. Prob. 56, 621–646. doi: 10.1525/sp.2009.56.4.621
Jorgenson, A. K., and Dietz, T. (2015). Economic growth does not reduce the ecological intensity of human well-being. Sustain. Sci. 10, 149–156. doi: 10.1007/s11625-014-0264-6
Jorgenson, A. K., and Givens, J. (2015). The changing effect of economic development on the consumption-based carbon intensity of well-being, 1990–2008. PLoS ONE 10:e0123920. doi: 10.1371/journal.pone.0123920
Knight, K. W., and Rosa, E. A. (2011). The environmental efficiency of well-being: A cross-national analysis. Soc. Sci. Res. 40, 931–949. doi: 10.1016/j.ssresearch.2010.11.002
Kuhler, J. (1940). A History of Agriculture in the Yakima Valley, Washington, from 1880-1900. University of Washington, Seattle, WA.
Larcom, S., and van Gevelt, T. (2017). Regulating the water-energy-food nexus: Interdependencies, transaction costs and procedural justice. Environ. Sci. Policy 72, 55–64. doi: 10.1016/j.envsci.2017.03.003
Lawford, R., Bogardi, J., Marx, S., Jain, S., Pahl Wostl, C., Knüppe, K., et al. (2013). Basin perspectives on the Water–Energy–Food Security Nexus. Curr. Opin. Environ. Sustain. 5, 607–616. doi: 10.1016/j.cosust.2013.11.005
Liu, J., Dietz, T., Carpenter, S. R., Alberti, M., Folke, C., Moran, E., et al. (2007a). Complexity of coupled human and natural systems. Science 317, 1513–1516. doi: 10.1126/science.1144004
Liu, J., Dietz, T., Carpenter, S. R., Folke, C., Alberti, M., Redman, C. L., et al. (2007b). Coupled human and natural systems. AMBIO J. Hum. Environ. 36, 639–649. doi: 10.1579/0044-7447(2007)36[639:CHANS]2.0.CO;2
Liu, J., Hull, V., Batistella, M., DeFries, R., Dietz, T., Fu, F., et al. (2013). Framing sustainability in a telecoupled world. Ecol. Soc. 18:26. doi: 10.5751/ES-05873-180226
Liu, J., Yang, H., Cudennec, C., Gain, A. K., Hoff, H., Lawford, R., et al. (2017). Challenges in operationalizing the water–energy–food nexus. Hydrol. Sci. J. 62, 1714–1720. doi: 10.1080/02626667.2017.1353695
Lockie, S. (2015). What is environmental sociology? Environ. Soc. 1, 139–142. doi: 10.1080/23251042.2015.1066084
Lockie, S. (2016). Beyond resilience and systems theory: reclaiming justice in sustainability discourse. Environ. Soc. 2, 115–117. doi: 10.1080/23251042.2016.1182308
Luna-Reyes, L. F., and Andersen, D. L. (2003). Collecting and analyzing qualitative data for system dynamics: methods and models. Syst. Dyn. Rev. 19, 271–296. doi: 10.1002/sdr.280
Malek, K., Adam, J., Stockle, C., and Brady, M. (2016). What Are the Downstream Water Availability Consequences of Switching to More Efficient Irrigation Systems? American Geophysical Union, San Francisco, CA. Available online at: http://adsabs.harvard.edu/abs/2016AGUFM.H51H1592M
Malek, K., Adam, J., Stockle, C., Brady, M., and Rajagopalan, K. (Forthcoming). When should irrigators invest in more water-efficient technologies as an adaptation to climate change? Water Resour. Res.
Malek, K., Adam, J. C., Stöckle, C. O., and Peters, T. P. (2018). Climate change reduces water availability for agriculture by decreasing non-evaporative irrigation losses. J. Hydrol. 561, 444–460. doi: 10.1016/j.jhydrol.2017.11.046
Martinkus, N., Rijkhoff, S. A., Hoard, S. A., Shi, W., Smith, P., Gaffney, M., et al. (2017). Biorefinery site selection using a stepwise biogeophysical and social analysis approach. Biomass Bioenergy 97, 139–148. doi: 10.1016/j.biombioe.2016.12.022
McIntosh, B. A., Sedell, J. R., Smith, J. E., Wissmar, R. C., Clarke, S. E., Reeves, G. H., et al. (1994). Management History of Eastside Ecosystems: Changes in Fish Habitat Over 50 Years, 1935-1992. Eastside Forest Ecosystem Health Assessment. Vol. 3. Gen. Tech. Rep. PNW321. U.S.D.A. Forest Service, Portland, OR.
McMahon, J. (2002). Case studies of violations of workers' freedom of association: migrant agricultural workers: human rights watch. Int. J. Health Services 32, 443–465. doi: 10.2190/3W5P-Q5C5-YVQH-YM08
Meinig, D. (1962). A comparative historical geography of two railnets: Columbia Basin and South Australia. Ann. Assoc. Am. Geogr. 52, 394–413. doi: 10.1111/j.1467-8306.1962.tb00421.x
Meseck, D. (2017). Yakima County Profile. Washington State Employment Security Department Olympia, WA. Available online at: https://esd.wa.gov/labormarketinfo/county-profiles/yakima#labor (Last Accessed June, 2018).
Middleton, C., Allouche, J., Gyawali, D., and Allen, S. (2015). The rise and implications of the water-energy-food nexus in Southeast Asia through an environmental justice lens. Water Alt. 8, 627–654.
Miller, T. R., Baird, T. D., Littlefield, C. M., Kofinas, G., Chapin, F. S. III., and Redman, C. L. (2008). Epistemological pluralism: reorganizing interdisciplinary research. Ecol. Soc. 13:46. doi: 10.5751/ES-02671-130246
Mohtar, R. H., and Lawford, R. (2016). Present and future of the water-energy-food nexus and the role of the community of practice. J. Environ. Stud. Sci. 6, 192–199. doi: 10.1007/s13412-016-0378-5
Montag, J. M., Swan, K., Jenni, K., Nieman, T., Hatten, J., Mesa, M., et al. (2014). Climate change and Yakama Nation tribal well-being. Clim. Change 124, 385–398. doi: 10.1007/s10584-013-1001-3
Nandalal, K. D. W., and Simonovic, S. P. (2003). Resolving conflicts in water sharing: a systemic approach. Water Resour. Res. 39, 1362. doi: 10.1029/2003WR002172
National Science Foundation (2015). Dear Colleague Letter: SEES: Interactions of Food Systems with Water and Energy Systems (NSF 15-040). Available online at: http://www.nsf.gov/pubs/2015/nsf15040/nsf15040.jsp (Accessed May 2018)
Olson, D. A. (1980). The Parcelization of the Open Range, a Conflict in Land Use: Grazing Rights Versus Residential and Recreation Land Sales in Klickitat County, Washington. Thesis, Portland State University.
Olsson, L., Jerneck, A., Thoren, H., Persson, J., and O'Byrne, D. (2015). Why resilience is unappealing to social science: theoretical and empirical investigations of the scientific use of resilience. Sci. Adv. 1:e1400217. doi: 10.1126/sciadv.1400217
Ostrom, E. (1990). Governing the Commons: The Evolution of Institutions for Collective Action. Cambridge, UK: Cambridge University Press.
Ostrom, E. (2009). A general framework for analyzing sustainability of social-ecological systems. Science 325, 419–422. doi: 10.1126/science.1172133
Pahl-Wostl, C. (2007). Transitions towards adaptive management of water facing climate and global change. Water Resour. Manage. 21, 49–62. doi: 10.1007/s11269-006-9040-4
Perrone, D., and Hornberger, G. M. (2014). Water, food, and energy security: scrambling for resources or solutions? Wiley Interdiscipl. Rev. Water 1, 49–68. doi: 10.1002/wat2.1004
Peterson, G. (2000). Political ecology and ecological resilience: an integration of human and ecological dynamics. Ecol. Econ. 35, 323–336. doi: 10.1016/S0921-8009(00)00217-2
Quinlan, A. E., Berbés-Blázquez, M., Haider, L. J., and Peterson, G. D. (2016). Measuring and assessing resilience: broadening understanding through multiple disciplinary perspectives. J. Appl. Ecol. 53, 677–687. doi: 10.1111/1365-2664.12550
Rasul, G. (2016). Managing the food, water, and energy nexus for achieving the Sustainable Development Goals in South Asia. Environ. Dev. 18, 14–25. doi: 10.1016/j.envdev.2015.12.001
Rijkhoff, S. A., Hoard, S. A., Gaffney, M. J., and Smith, P. M. (2017). Communities ready for takeoff: Integrating social assets for biofuel site-selection modeling. Politics Life Sci. 36, 14–26. doi: 10.1017/pls.2017.6
Roberts, J. T., and Parks, B. (2006). A Climate of Injustice: Global Inequality, North-south Politics, and Climate Policy. Cambridge, MA: MIT Press.
Rosa, E. A., and Dietz, T. (2012). Human drivers of national greenhouse-gas emissions. Nat. Climate Change 2, 581–586. Available online at: https://www.nature.com/articles/nclimate1506
Schirmer, J., Mylek, M., Peel, D., and Yabsley, B. (2015). People and Communities the 2014 Regional Wellbeing Survey. Canberra, AU: University of Canberra.
Schor, J. B., Fitzmaurice, C., Carfagna, L. B., Attwood-Charles, W., and Poteat, E. D. (2016). Paradoxes of openness and distinction in the sharing economy. Poetics 54, 66–81. doi: 10.1016/j.poetic.2015.11.001
Sheller, R. (1997). Courage and Water: A Story of Yakima Valley's Sunnyside. Astoria, OR: S Dot S Publishing.
Smith, L. M., Case, J. L., Smith, H. M., Harwell, L. C., and Summers, J. K. (2013). Relating ecoystem services to domains of human well-being: Foundation for a US index. Ecol. Indic. 28, 79–90. doi: 10.1016/j.ecolind.2012.02.032
State of Washington Department of Ecology (2013). Yakima Basin Integrated Water Resource Management Plan (YBIP). Available online at: http://www.ecy.wa.gov/Programs/wr/cwp/YBIP.htm (Accessed December, 23).
State of Washington Department of Ecology (2018). Yakima River Basin Integrated Water Resource Management Plan Implementation Status Report 2017. Pub No. 18-12-005 Available online at: https://fortress.wa.gov/ecy/publications/documents/1812005.pdf (Accessed June 22, 2018).
State of Washington Dept of Ecology (2012). Yakima River Basin Integrated Water Resource Management Plan- Final Programmatic Environmental Impact Statement. State of WA Dept of Ecology, Yakima, WA. Available online at: https://fortress.wa.gov/esd/employmentdata/reports-publications/regional-reports/county-profiles/yakima-county-profile
Stave, K. A. (2003). A systems dynamics model to facilitate public understanding of water management options in Las Vegas, Nevada. J. Environ. Manage. 67, 303–311. doi: 10.1016/S0301-4797(02)00205-0
Sterman, J. D. (2001). System Dynamics Modeling: Tools for Learning in a Complex World. California Management Review, 43, 8–25. doi: 10.2307/41166098
Stuart, D. (2016). Crossing the ‘great divide’ in practice: theoretical approaches for sociology in interdisciplinary environmental research. Environ. Soc. 2, 118–131. doi: 10.1080/23251042.2016.1144242
Summers, J. K., Harwell, L. C., Buck, K. D., Smith, L. M., Vivian, D. N., Bousquin, J. J., et al. (2017). Development of a Climate Resilience Screening Index (CRSI): An Assessment of Resilience to Acute Meteorological Events and Selected Natural Hazards. U.S. Environmental Protection Agency, Washington, DC, EPA/600/R-17/238. Available online at: https://cfpub.epa.gov/si/si_public_record_report.cfm?dirEntryId=337914
Tanner, T., Lewis, D., Wrathall, D., Bronen, R., Cradock-Henry, N., Huq, S., et al. (2015). Livelihood resilience in the face of climate change. Nat. Climate Change. 5, 23. doi: 10.1038/nclimate2431
Taylor, R., and Zilberman, D. (2017). Diffusion of drip irrigation: the case of California. Appl. Econ. Perspect. Policy 39, 16–40. doi: 10.1093/aepp/ppw026
USBR (2011a). The Story of the Yakima Project. Available online at: https://www.usbr.gov/projects/pdf.php?id=220 (Last Accessed June, 2018).
USBR (2011b). Technical Memorandum Yakima River Basin Study – Roza and Chandler Power Plants Subordination and Power Usage Evaluation Tech. Memo. Available online at: https://www.usbr.gov/pn/programs/yrbwep/reports/tm/4-3powsub.pdf
USBR (2012). Yakima River Basin Integrated Water Resource Management Plan [WWW Document]. Available online at: zotero://attachment/967/ (Accessed May, 27 2014).
USBR (2018). The Yakima Project. US Bureau of Reclamation. Available online at: https://www.usbr.gov/projects/index.php?id=400 (Last Accessed June, 2018).
USBR (US Bureau of Reclamation) (2008). Yakima River Basin Water Storage Feasibility Study Planning Report/EIS (Storage Study). Available online at: http://www.usbr.gov/pn/programs/yrbwep/2011integratedplan/ (Accessed May 27, 2014).
USBR and State of Washington Department of Ecology (2012). Yakima River Basin Integrated Water Resource Management Plan Final Programmatic Environmental Impact Statement. Ecology 12-12-002. Benton, Kittitas, Klickitat and Yakima Counties. Available online at: http://www.usbr.gov/pn/programs/yrbwep/reports/FPEIS/fpeis.pdf
USDA (2012). 2012 Census of Agriculture County Profile- Yakima County, Washington.: US Department of Agriculture- National Agricultural Statistics Service. Available online at: https://www.agcensus.usda.gov/Publications/2012/Online_Resources/County_Profiles/Washington/cp53077.pdf
Vano, J. A., Scott, M. J., Voisin, N., Stöckle, C. O., Hamlet, A. F., Mickelson, K. E. B., et al. (2010). Climate change impacts on water management and irrigated agriculture in the Yakima River Basin, Washington, USA. Climatic Change 102, 287–317. doi: 10.1007/s10584-010-9856-z
Villamayor-Tomas, S., Grundmann, P., Epstein, G., Evans, T., and Kimmich, C. (2015). The water-energy-food security nexus through the lenses of the value chain and the Institutional Analysis and Development frameworks. Water Alt. 8, 735–755.
Viscusi, W. K., Phillips, O. R., and Kroll, S. (2011). Risky investment decisions: how are individuals influenced by their groups? J. Risk Uncertain. 43, 81. doi: 10.1007/s11166-011-9123-3
Walker, B., Holling, C. S., Carpenter, S., and Kinzig, A. (2004). Resilience, adaptability and transformability in social–ecological systems. Ecol. Soc. 9:5. doi: 10.5751/ES-00650-090205
Wang, H., and Hanna, S. D. (1998). Does Risk Tolerance Decrease with Age? (SSRN Scholarly Paper No. ID 95489). Social Science Research Network, Rochester, NY.
Wichelns, D. (2017). The water-energy-food nexus: Is the increasing attention warranted, from either a research or policy perspective? Environ. Sci. Policy 69, 113–123. doi: 10.1016/j.envsci.2016.12.018
World Commission on Environment and Development (1987). Our Common Future. Available online at: www.un-documents.net/our-common-future.pdf
Yakama Nation (2016). Climate Adaptation Plan for the Territories of the Yakama Nation. Available online at: http://www.critfc.org/wp-content/uploads/2016/05/Yakama-Nation-Climate-Adaptation-Plan-.pdf (Accessed June 22, 2018).
Yoder, J., Adam, J., Brady, M., Cook, J., and Katz, S. (2014). Benefit-Cost Analysis of the Yakima Basin Integrated Plan Projects (Report to the Washington State Legislature). Pullman, WA: Washington State University.
Yoder, J., Adam, J., Brady, M., Cook, J., Katz, S., Johnston, S., et al. (2017). Benefit-Cost Analysis of Integrated Water Resource Management: Accounting for Interdependence in the Yakima Basin Integrated Plan. J. Am. Water Resour. Assoc. 53, 456–477. doi: 10.1111/1752-1688.12507
York, R., Rosa, E. A., and Dietz, T. (2002). Bridging environmental science with environmental policy: Plasticity of population, affluence, and technology. Soc. Sci. Q. 83, 18–34. doi: 10.1111/1540-6237.00068
Keywords: resilience, sustainability, social science, Food-Energy-Water Nexus, INFEWS, Columbia River Basin, Yakima River Basin
Citation: Givens JE, Padowski J, Guzman CD, Malek K, Witinok-Huber R, Cosens B, Briscoe M, Boll J and Adam J (2018) Incorporating Social System Dynamics in the Columbia River Basin: Food-Energy-Water Resilience and Sustainability Modeling in the Yakima River Basin. Front. Environ. Sci. 6:104. doi: 10.3389/fenvs.2018.00104
Received: 25 June 2018; Accepted: 24 August 2018;
Published: 19 September 2018.
Edited by:
Jill A. Engel-Cox, National Renewable Energy Laboratory (DOE), United StatesReviewed by:
Nidhi Nagabhatla, United Nations University Institute for Water Environment and Health, CanadaRichard Meissner, Council for Scientific and Industrial Research (CSIR), South Africa
Copyright © 2018 Givens, Padowski, Guzman, Malek, Witinok-Huber, Cosens, Briscoe, Boll and Adam. This is an open-access article distributed under the terms of the Creative Commons Attribution License (CC BY). The use, distribution or reproduction in other forums is permitted, provided the original author(s) and the copyright owner(s) are credited and that the original publication in this journal is cited, in accordance with accepted academic practice. No use, distribution or reproduction is permitted which does not comply with these terms.
*Correspondence: Jennifer E. Givens, amVubmlmZXIuZ2l2ZW5zQHVzdS5lZHU=