- 1Department of Agricultural Soil Science, University of Göettingen, Göttingen, Germany
- 2Leibniz Centre for Agricultural Landscape Research (ZALF), Group of Hydropedology, Müncheberg, Germany
- 3Division of Soil Physics, University of Bayreuth, Bayreuth, Germany
Mucilage is receiving increasing attention because of its putative effects on plant growth, but so far no method is available to measure its spatial distribution in the rhizosphere. We tested whether the C-H signal related to mucilage fatty acids is detectable by infrared spectroscopy and if this method can be used to determine the spatial distribution of mucilage in the rhizosphere. Maize plants were grown in rhizoboxes filled with soil free of organic matter. Infrared measurements were carried out along transects perpendicular as well as axially to the root channels. The perpendicular gradients of the C-H proportions showed a decrease of C-H with increasing distance: 0.8 mm apart from the root center the C-H signals achieved a level near zero. The measured concentrations of mucilage were comparable with results obtained in previous studies, which encourages the use of infrared spectroscopy to quantitatively image mucilage in the rhizosphere.
Introduction
The rhizosphere is the soil volume around the roots and plays a crucial role for nutrient and water acquisition by plants (Gregory, 2006; Neumann et al., 2009). Organic compounds (rhizodeposits) are released through the root into the rhizosphere in different chemical forms and they may have positive effects on plant growth (Jones et al., 2009). Mucilage is one of those compounds and makes up 2–12% of total rhizodeposition (Dennis et al., 2010 and references therein). Mucilage is a gel-like substance and is released from the root-cap cells (Figure 1). Its main components are polysaccharides (~ 94%), proteins (1–5%) and phospholipids (Oades, 1978; Bacic et al., 1986; Read et al., 2003; Jones et al., 2009; Carminati and Vetterlein, 2013).
Mucilage provides several benefits for plant growth, such as the lubrication during root penetration (Iijima et al., 2004) or the stabilization of aggregates (Morel et al., 1991; Traoré et al., 2000). Recently it has been shown that mucilage increases rhizosphere water content (Carminati et al., 2010) due to the high water-holding capacity of the contained polysaccharides (McCully and Boyer, 1997) and it may therefore facilitate root water uptake (Ahmed et al., 2014). The extent of such benefits depends on the spatial distribution of mucilage around roots. To date, there is no experimental method to non-invasively and quantitatively image mucilage in soils and our knowledge of mucilage spatial distribution remains largely speculative.
Among the many effects of mucilage on soil properties, one spectacular effect is the rhizosphere water repellency (Hallett, 2003; Whalley et al., 2004; Moradi et al., 2012). While the polysaccharides contained in mucilage have a very high water holding capacity (McCully and Boyer, 1997), the lipids which are also contained (Read et al., 2003), can have a reverse effect when mucilage dries. It is therefore likely that mucilage is responsible for the observed rhizosphere hydrophobicity (Carminati, 2013; Ahmed et al., 2015; Benard et al., 2017). Fatty acids are composed of a hydrophilic and a hydrophobic tail of nonpolar fatty acid chains (C-H groups). Upon soil drying these hydrophobic tails probably turn outwards. Hereby they reduce soil wettability and cause a hydrophobic region around the root as indicated in Figure 2.
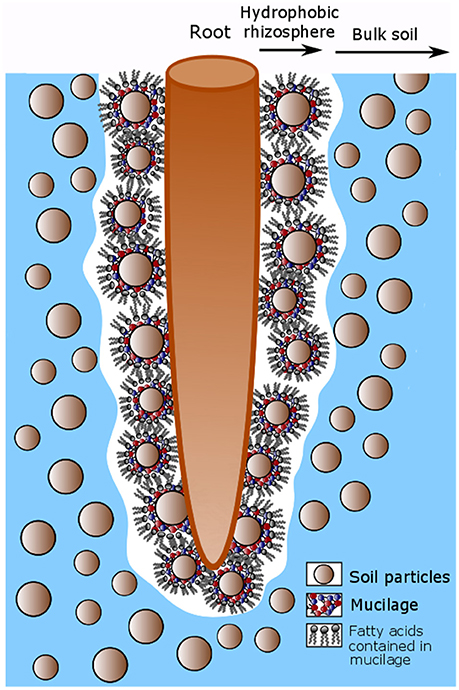
Figure 2. Conceptual model describing the hydrophobicity around root after soil drying caused by fatty acids contained in mucilage. Mucilage is released by the root tip and accumulates around soil particles in the rhizosphere. Upon soil drying the fatty acids contained in mucilage turn their hydrophobic tails outwards so that the soil around the root becomes hydrophobic.
Diffuse reflectance infrared Fourier transform (DRIFT) spectroscopy can be used to map functional groups such as C-H groups at intact soil surfaces at the mm-scale (Leue et al., 2010, 2015). In combination with microscopic techniques, DRIFT spectroscopy can be used to determine organic functional groups also at the μm-scale. The aim of this study was to use the C-H groups of the fatty acids contained in mucilage as a proxy for mucilage distribution in the rhizosphere. Although the main compound of mucilage is polysaccharides, we used fatty acids as a proxy for mucilage because the C-H groups are specific for mucilage, while no functional group specifically for polysaccharides exists. Although C = O or O-H groups can be measured by IR spectroscopy, those groups are not specifically for polysaccharides because they are also present in sugar monomers or amino acids, which are present in the rhizosphere as they are released as root exudates.
We used DRIFT spectroscopy and Fourier-transform infrared spectroscopy (FTIR) microscopy to map the distribution of fatty acids from mucilage in soil. Maize plants were grown in rhizoboxes and the signal of the C-H groups was recorded with a high spatial resolution along the roots as well as perpendicular to the roots.
Materials and Methods
Plant Growth and Mucilage Extraction
Quartz material consisting of 70% quartz sand [collected from Duingen (Germany), particle size: 0.1–0.2 mm] and 30% quartz powder (Carl Roth, particle size: < 0.125 mm) was used for the calibration and as a growth medium free of soil organic matter. For the calibration, maize root mucilage was extracted as described in Zickenrott et al. (2016) in a slightly modified way. A mesh of stainless steel (2 mm diameter mesh size) was hanged into a plastic box 15 cm above surface of the box. The box had a size of 30 × 40 × 30 cm and was filled with water to a height of approximately 6 cm (Figure 3A). An aquarium diffuser was placed into the water in the box and connected to a plastic tube which was plugged to pressurized air. The air was circulated though the diffuser which creates tiny air bubbles which increased the humidity in the box. Maize seeds were sterilized in 10% H2O2 solution for 10 min and placed on the mesh and the box was closed with a plastic lid to maintain high air humidity. After 3–4 days root tips were growing trough the mesh and showed small drops of hydrated mucilage at the tips due to the high humidity in the box (Figure 3A). The mucilage was collected from the tips once a day till the roots reached the water surface after approximately 3 days. The collection of mucilage was done with a syringe by applying negative pressure. Per box and day we collected approximately 6 mg of dry mucilage.
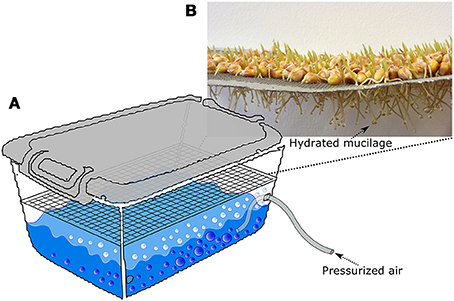
Figure 3. Sketch, describing the procedure of mucilage collection. A tray covered with maize seeds is placed in a closed plastic box. The box is partly filled with deionized water. An aquarium diffuser is placed into the water and pressurized air is pumped through it so that tiny bubbles are produced (A). Hereby the humidity of the air is increased so that the mucilage stays hydrates and forms small drops around the roots tips (B). The mucilage drops are once a day with a syringe by applying negative pressure.
For the calibration, dried quartz material was mixed with different amounts of previously collected mucilage at concentrations of 0.0, 0.025, 0.05, 0.1, 0.25, 0.5, and 1 mg/g (gram of dry mucilage per gram of dry soil). The mixtures were applied on object slides in four replicates and were air-dried. For the mapping of the spatial distribution of mucilage across root channels, maize (Zea mays L.) plants (KWS 2376) were grown in rhizoboxes with an inner size of 10 × 20 × 1.5 cm filled with the same quartz substrate as used for the calibration. Before germination, maize seeds were immersed in a 10% H2O2 solution for 10 min to avoid seed-borne diseases. Prior to plant growth the following nutrients were added per kg of soil: NH4NO3-N: 0.3 g, Ca(H2PO4)2-P: 0.06 g, K2SO4-K: 0.03 g, CaSO4-Ca: 0.05 g, MgSO4-Mg: 0.05 g, H3BO4-B: 2 mg, CuSO4-Cu: 0.03 mg, MnSO4-Mn: 1.5 mg, (NH4)2MoO4-Mo: 0.03 mg, ZnCl2-Zn: 1.2 mg, FeNaEDTA-Fe: 3.6 mg. During plant growth, the water content was adjusted to 20%. Soil water content was checked gravimetrically each day and lost water was added from the top with a syringe. The rhizoboxes were kept at an angle of approximately 55° to make sure that the roots were growing close to the lower side of the rhizoboxes. The temperature in the climate chamber was 25°C during day and 22°C during night, the photoperiod was 14 h and the light intensity was 300 μmol m−2 s−1. After 4 weeks of growth, plant shoots were cut and the soil samples were dried at 35°C. A low temperature of 35°C was chosen for drying to allow for a drying process close to natural conditions and to avoid physical and chemical changes of mucilage. After drying, the roots were removed from the soil manually so that the channel were root had been growing in remained as a depression in the otherwise even soil surface (Figure 4).
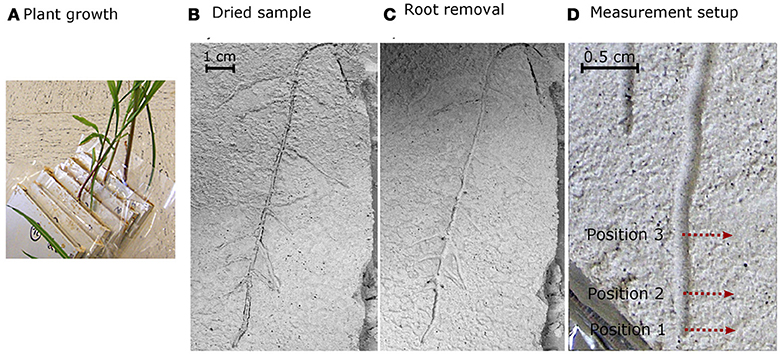
Figure 4. Plant growth (A) and rhizobox with dried soil and plants before (B) and after (C) the roots were excavated from the soil. Measurements were conducted after removing the roots at three position (0, 1, and 3 cm behind the tip) perpendicular to the root channel as indicated by the red arrows (D).
Diffuse Reflectance Infrared Spectroscopy
Diffuse reflectance infrared spectroscopy (DRIFT) measurements in the mid-infrared range (wavelength: 2.5–25 μm, wave numbers (WN): 4,000–400 cm−1) was applied to the calibration samples at the object slides using a BioRad® FTS 135 spectrometer (Cambridge, Massachusetts, USA). Each DRIFT spectrum was recorded by 16 co-added scans with a spectral resolution of 4 cm−1 against a gold background (Leue et al., 2015). At the intact root channels and surrounding substrate, DRIFT spectra were conducted using an Agilent Cary series 600 FTIR microscope (Agilent Cary series 600, Agilent Technologies, Inc., Santa Clara, CA, USA). The measurements were done at three positions, (a) directly at the root tip, 1 cm behind the root tip and 3 cm behind the root tip. Measurement at each position started in the center of the root channel. From there, IR spectra were recorded each 100 μm perpendicular to the root channel till a distance of 13 mm from the root channel center was reached (Figures 4, 5). The measurements within the root channel (soil that had been in direct contact with the root) are referred to as “root channel” while measurements beyond the root channel border are referred to as “rhizosphere.” The spectra were recorded as 64 co-added scans at a spectral resolution of 4 cm−1 in steps of 100 μm from sample areas of 0.1 × 0.1 mm. For the rhizosphere samples, one root was chosen per plant and spectra were measured at three positions along each root (0, 1, and 3 cm behind the tip). After preprocessing of the spectra (Ellerbrock et al., 2009; Leue et al., 2010), the local peak heights of the C-H bands were normalized for the absolute peak height of the SiO2 band at WN 1,350 cm−1. The relationship between mucilage content and C-H signal intensities obtained from the calibration samples was used to quantify the mucilage content in the rhizosphere of the maize roots. After calibration, the background signal (i.e., the average signal in the bulk soil where no mucilage was expected) was subtracted from each measured value.
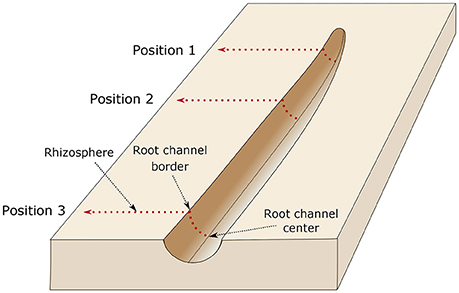
Figure 5. Illustration of the measurement setup. The dried roots were carefully removed from the sample and IR measurements were done at three positions, directly at the root tip (position 1), 1 cm behind the root tip (position 2), and 3 cm behind the root tip (position 3). Measurement at each position started in the center of the root channel. From there, IR spectra were recorded each 100 μm perpendicular to the root channel till a distance of 13 mm from the root channel center was reached. The border of the root channel is defined as the position were the intact soil sample (i.e., rhizosphere) starts.
Statistics
To test for differences between soil regions and root positions we applied a one-way ANOVA (p < 0.05) followed by a post-hoc test. Statistical analysis were done in R 3.3.1.
Results
The calibration measurements on the object slides revealed a linear relationship between mucilage concentration and the C-H/SiO2 ratio The fit of the linear equation war good and resulted in an R2 of 0.99 (Figure 6). The calibration equation was applied to the IR measurements at the root channels to obtain mucilage concentration (mg/g).
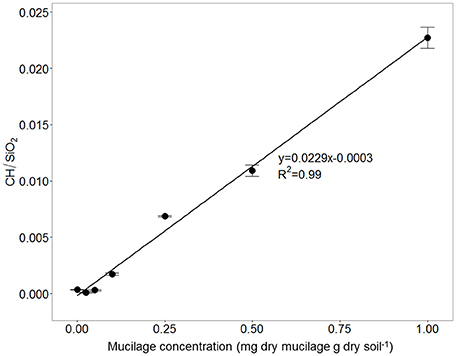
Figure 6. Ratio between CH and SiO2 signal for soil mixed with different maize-mucilage concentrations collected from maize seedlings ranging from 0 to 1 mg dry mucilage per g dry soil.
The IR measurements at the 3 root positions showed that mucilage concentration slightly increased with increasing distance from the root tip (i.e., increasing root age), however, differences between the positions were not statistically significant (Figure 7). Mucilage concentration in the rhizosphere was lower compared to the root channel for all 3 positions but these differences were likewise not statistically different probably due to very high variation between the samples.
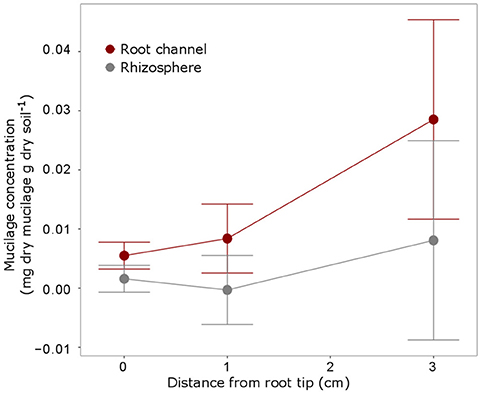
Figure 7. Mucilage concentration (mg dry mucilage per g dry soil) for the 3 positions were measurements were taken i.e., at the root tip, 1 cm behind the tip and 3 cm behind the tip as indicated in Figure 4. The measurements within the root channel (soil that had been in direct contact with the root) were averaged and compared to the measurements beyond the root channel with was defined as rhizosphere soil [10 measurements (= 1 mm) beyond the root channel border, corresponding to the region defined as “rhizosphere” in Figure 5]. The displayed values are the means of 5 replicates (i.e., plants). Variation is given as standard error. Differences between the positions and the soil regions were not significant (p < 0.05).
The highest mucilage concentrations of 0.02 mg/g were found in the center of the root channel (Figure 8). Toward the border of the root channel this concentration decreased to around 0.01 mg/g. The radial gradients of mucilage from the center of the root channel toward rhizosphere dropped rapidly and decreased to approximately 0 mg/g at a distance of around 0.8 mm from the center of the root channel (Figure 8). Averaging the mucilage concentration in the root channel for all 3 measured root positions resulted in a mucilage concentration of 0.017 mg/g. The rhizosphere reached on average a much lower concentration of 0.003 mg/g mucilage (Figure 9). Differences between both measurements were not different statistically, presumably because of the high variation in the root channel measurements.
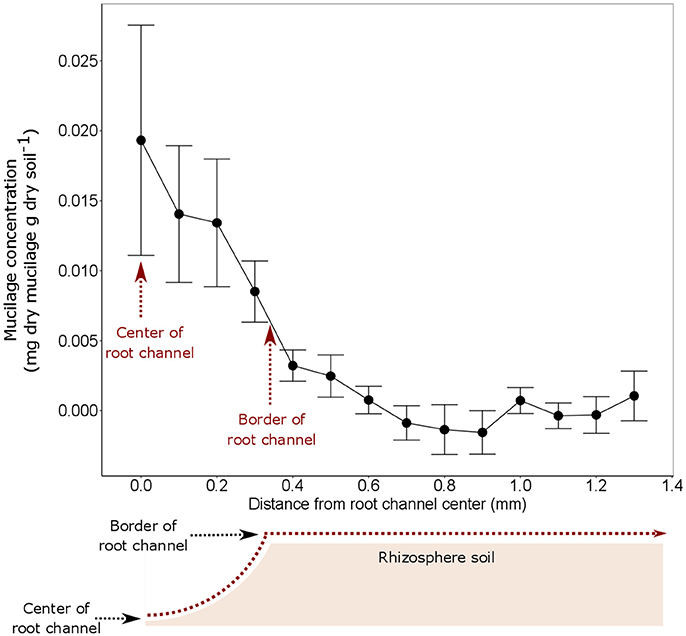
Figure 8. Mucilage concentration (mg dry mucilage per g dry soil) starting from the root channel center in radial direction toward the bulk soil. The measurements from the 3 different root positions were averaged because they did not reveal any statistical difference (Figure 6). The displayed values are the means of 5 replicates (i.e., plants). Variation is given as standard error. x = 0 equals to the position of the center of the root channel as indicated in the sketch below the figure. The average extend of the root channel (r = 0.34 mm) is indicated as border of root channel.
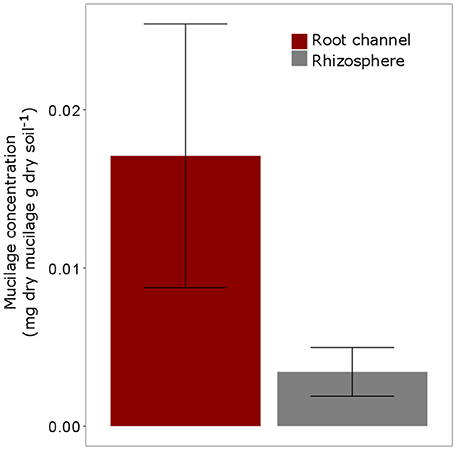
Figure 9. Average Mucilage concentration (mg dry mucilage per g dry soil) for all measured positions (= 3) and all plants (5 replicates). “Root channel” refers to the measurements within the root channel (soil that had been in direct contact with the root). “Rhizosphere” refers to the soil 1 mm (= 10 measurements) beyond the root channel border, corresponding to the region defined as “rhizosphere” in Figure 3). Variation is given as standard error. Differences between Root channel and rhizosphere were not statistically different (p < 0.05).
Discussion
This pilot experiment showed that infrared spectroscopy can in principle be applied to detect gradients of mucilage around roots grown in soil. Although not significant, the measurements of mucilage at different root positions showed an increase in C-H groups (i.e., hydrophobicity) with increasing root age (Figure 7). This is in agreement with Carminati (2013) who found that rhizosphere hydrophobicity was enhanced with increasing root age. This observation was explained by an increased stiffening of mucilage either due to (a) repeated drying and rewetting, (b) interaction between mucilage and solutes such as Ca2+ present in soil solution or (c) microbial decomposition of mucilage (Carminati, 2013; Carminati and Vetterlein, 2013). The first two processes cannot explain an increase in C-H groups that we observed. However, it is possible that mucilage was exposed to microbial decomposition. This could cause a change in the ratio of fatty acids to other compounds such as polysaccharides or may increase the amount of fatty acids. A change in mucilage composition due to aging would be problematic for the interpretation of our results. As the measurements were calibrated with maize root mucilage, a prerequisite for this approach is that the chemical composition of mucilage used in the calibration is similar to those found in the soil around roots.
The fact that we found a trend of increasing C-H signal with increasing root age but no statistical differences, can have two reasons. First, natural variability between plants may be high. This could be solved by a higher number of replicates. Second, the measured root channel segments at distances of 0, 1, and 3 cm behind the tip may have been too young to show differences in mucilage concentration and distribution. Probably longer time intervals (i.e., older root segments) would be necessary to detect changes in mucilage quantity or quality due to decomposition.
The radial gradients of mucilage were relatively steep: at 0.6 mm distance from the border of the root channel, mucilage content decreased below the limit of detection. The extent of the rhizosphere affected by mucilage (0.6 mm) was smaller compared to results obtained from 14C imaging analyses. Holz et al. (2017) showed that overall root exudates diffused up to 1 mm into the bulk soil. The fact that mucilage did not move as far from the root surface as compared to overall exudates may be explained by the higher viscosity of mucilage compared to root exudates (Read and Gregory, 1997) and reduced diffusion coefficient of mucilage compared to root exudates.
We found contents of up to 0.02 mg dry mucilage per g dry soil in the center of the root channel and average values of around 0.017 mg dry mucilage per g dry soil in the root channel (Figures 8, 9). Based on the literature, we can expect a mucilage exudation rate of 15 μg d−1 per root tip (Chaboud, 1983). Assuming a maximum diffusion length of 1 mm into the soil, a mean root radius of 0.5 mm, a soil bulk density of 1.43 g cm−3 as for our samples and a root elongation of 3 cm d−1 we expect a mucilage concentration in the rhizosphere of 0.056 mg dry mucilage per g dry soil. This theoretical value is 3-times higher than the values obtained by DRIFT spectroscopy; however, they still appear comparable.
One opportunity for increasing the precision of the DRIFT measurements could be the use of the same DRIFT spectrometer (here: FTIR microscope) for both, the calibration and intact sample measurements. The use of a finer substrate to optimize the application of the DRIFT technique could improve the spectral analysis of the mucilage and the identification resolution. However, plant growth may be difficult in even finer substrates.
Conclusions
The results suggest that DRIFT spectroscopy and FTIR microscopy can be applied to quantify the spatial distribution of mucilage in the rhizosphere. The measured mucilage contents and the spatial extent of the mucilage-affected rhizosphere were slightly lower but comparable to calculated and published values. This technique can in principle be applied to any other plant species that produces mucilage.
A few aspects have to be considered when applying the method:
- Our results showed a high variability which resulted in a lack of statistical differences even though trends of increasing mucilage with root age were observed (Figure 7). The high standard error is probably caused by a high variability between the plants. An increase in sample size would probably solve this problem.
- The IR measurements have to be calibrated to obtain the mucilage content in soil. As such, it needs to be ensured that the chemical composition of mucilage used for the calibration is similar to mucilage around roots in soil. This may be true for mucilage close to the root tip but may be problematic at older root sections where mucilage was exposed to microbial decomposition which might have changed its chemical composition.
- This approach is restricted to dried samples and soil materials with no organic matter because any C-H signal from native soil organic matter would overlay the comparatively low C-H signal of mucilage. Therefore, the transfer of results to natural conditions is limited. While the texture of the material we used is comparable to a natural soil, chemical and biological conditions may differ.
Bearing those aspects in mind, IR spectroscopy can be applied to measure the spatial distribution of mucilage in soil. Future studies could investigate the effect of factors such as: root hairs, root age, root type, plant species, soil texture, and soil water content on the spatial distribution of mucilage in the rhizosphere.
Author Contributions
MH, ML: planning and conducting the experiment, data analysis, writing manuscript; MA, PB: planning and conducting experiment; HG: planning experiment, providing facilities for IR measurements; AC: planning experiment, providing funding.
Conflict of Interest Statement
The authors declare that the research was conducted in the absence of any commercial or financial relationships that could be construed as a potential conflict of interest.
Acknowledgments
We acknowledge the DFG for funding (Projects CA 921/3-1, KU 1184/33-1, and LE3177/1-2) and ev. Studienwerk Villigst for funding the position of MH. We thank KWS for providing the seeds for the experiment and we acknowledge support by the Open Access Funds of the University of Göttingen.
References
Ahmed, M. A., Holz, M., Woche, S. K., Bachman, J., and Carmintati, A. (2015). Effect of soil drying on mucilage exudation and its water repellency: a new method to collect mucilage. J. Plant Nutr. Soil Sci. 178, 1–4. doi: 10.1002/jpln.201500177
Ahmed, M. A. A., Kröner, E., Holz, M., Zarebanadkouki, M., and Carminati, A. (2014). Mucilage exudation facilitates root water uptake in dry soils. J. Funct. Plant Biol. 41, 1129–1137. doi: 10.1071/FP13330
Bacic, A., Moody, S. F., and Clarke, A. E. (1986). Structural analysis of secreted root slime from maize (Zea mays L.). Plant Physiol. 80, 771–777. doi: 10.1104/pp.80.3.771
Benard, P., Zarebanadkouki, M., Hedwig, C., Holz, M., Ahmed, M. A., and Carmintati, A. (2017). Pore-scale distribution of mucilage affecting water repellency in the rhizosphere. Vadose Zo. J. 7:170013. doi: 10.2136/vzj2017.01.0013
Carminati, A. (2013). Rhizosphere wettability decreases with root age: a problem or a strategy to increase water uptake of young roots? Front. Plant Sci. 4:298. doi: 10.3389/fpls.2013.00298
Carminati, A., Moradi, A. B., Vetterlein, D., Vontobel, P., Lehmann, E., Weller, U., et al. (2010). Dynamics of soil water content in the rhizosphere. Plant Soil 332, 163–176. doi: 10.1007/s11104-010-0283-8
Carminati, A., and Vetterlein, D. (2013). Plasticity of rhizosphere hydraulic properties as a key for efficient utilization of scarce resources. Ann. Bot. 112, 277–290. doi: 10.1093/aob/mcs262
Chaboud, A. (1983). Isolation, purification and chemical composition of maize root cap slime. Plant 73, 395–402. doi: 10.1007/BF02184316
Dennis, P. G., Miller, A. J., and Hirsch, P. R. (2010). Are root exudates more important than other sources of rhizodeposits in structuring rhizosphere bacterial communities? FEMS Microbiol. Ecol. 72, 313–327. doi: 10.1111/j.1574-6941.2010.00860.x
Ellerbrock, R. H., Gerke, H. H., and Böhm, C. (2009). In situ drift characterization of organic matter composition on soil structural surfaces. Soil Sci. Soc. Am. J. 73, 531–540. doi: 10.2136/sssaj2008.0103
Gregory, P. J. (2006). Roots, rhizosphere and soil: the route to a better understanding of soil science? Eur. J. Soil Sci. 57, 2–12. doi: 10.1111/j.1365-2389.2005.00778.x
Hallett, P. (2003). Plant influence on rhizosphere hydraulic properties: direct measurements using a miniaturized infiltrometer. New Phytol. 157, 597–603. doi: 10.1046/j.1469-8137.2003.00690.x
Holz, M., Zarebanadkouki, M., Kuzyakov, Y., Pausch, J., and Carminati, A. (2017). Root hairs increase rhizosphere extension and carbon input to soil. Ann. Bot. 121, 61–69. doi: 10.1093/aob/mcx127
Iijima, M., Higuchi, T., and Barlow, P. W. (2004). Contribution of root cap mucilage and presence of an intact root cap in maize (zea mays) to the reduction of soil mechanical impedance. Ann. Bot. 94, 473–477. doi: 10.1093/aob/mch166
Jones, D. L., Nguyen, C., and Finlay, R. D. (2009). Carbon flow in the rhizosphere: carbon trading at the soil–root interface. Plant Soil 321, 5–33. doi: 10.1007/s11104-009-9925-0
Leue, M., Ellerbrock, R. H., and Gerke, H. H. (2010). DRIFT mapping of organic matter composition at intact soil aggregate surfaces. Vadose Zo. J. 9, 317–324. doi: 10.2136/vzj2009.0101
Leue, M., Gerke, H. H., and Godow, S. C. (2015). Droplet infiltration and organic matter composition of intact crack and biopore surfaces from clay-illuvial horizons. J. Plant Nutr. Soil Sci. 178, 250–260. doi: 10.1002/jpln.201400209
McCully, M. E., and Boyer, J. S. (1997). The expansion of maize root-cap mucilage during hydration. 3. changes in water potential and water content. Physiol. Plant. 99, 169–177. doi: 10.1111/j.1399-3054.1997.tb03445.x
Moradi, A. B., Carminati, A., Lamparter, A., Woche, S. K., Bachmann, J., Vetterlein, D., et al. (2012). Is the rhizosphere temporarily water repellent? Vadose Zone J. 11. doi: 10.2136/vzj2011.0120
Morel, J. L., Habib, L., Plantureux, S., and Guckert, A. (1991). Influence of maize root mucilage on soil aggregate stability. Plant Soil 136, 111–119. doi: 10.1007/BF02465226
Neumann, G., George, T. S., and Plassard, C. (2009). Strategies and methods for studying the rhizosphere-the plant science toolbox. Plant Soil 321, 431–456. doi: 10.1007/s11104-009-9953-9
Oades, J. M. (1978). Mucilages at the root surface. J. Soil Sci. 29, 1–16. doi: 10.1111/j.1365-2389.1978.tb02025.x
Read, D. B., Bengough, A. G., Gregory, P. J., Crawford, J. W., Robinson, D., Scrimgeour, C. M., et al. (2003). Plant roots release phospholipid surfactants that modify the physical and chemical properties of soil. New Phytol. 157, 315–326. doi: 10.1046/j.1469-8137.2003.00665.x
Read, D. B., and Gregory, P. J. (1997). Surface tension and viscosity of axenic maize and lupin root mucilages. New Phytol. 137, 623–628. doi: 10.1046/j.1469-8137.1997.00859.x
Traoré, O., Groleau-Renaud, V., Plantureux, S., Tubeileh, A., and Boeuf-Tremblay, V. (2000). Effect of root mucilage and modelled root exudates on soil structure. Eur. J. Soil Sci. 51, 575–581. doi: 10.1046/j.1365-2389.2000.00348.x
Whalley, W., Leeds-Harrison, P., Leech, P., Riseley, B., and Bird, N. (2004). The hydraulic properties of soil at root-soil interface. Soil Sci. 2, 90–99. doi: 10.1097/01.ss.0000117790.98510.e6
Zickenrott, I.-M., Woche, S. K., Bachmann, J., Ahmed, M. A., and Vetterlein, D. (2016). An efficient method for the collection of root mucilage from different plant species–a case study on the effect of mucilage on soil water repellency. J. Plant Nutr. Soil Sci. 179, 294–302. doi: 10.1002/jpln.201500511
Keywords: rhizosphere extension, root mucilage, DRIFT spectroscopy, maize (Zea mays L.), soil hydrophobicity
Citation: Holz M, Leue M, Ahmed MA, Benard P, Gerke HH and Carminati A (2018) Spatial Distribution of Mucilage in the Rhizosphere Measured With Infrared Spectroscopy. Front. Environ. Sci. 6:87. doi: 10.3389/fenvs.2018.00087
Received: 31 January 2018; Accepted: 20 July 2018;
Published: 17 August 2018.
Edited by:
Luiz Fernando Wurdig Roesch, Federal University of Pampa, BrazilReviewed by:
Akifumi Sugiyama, Kyoto University, JapanPaul Knox, University of Leeds, United Kingdom
Copyright © 2018 Holz, Leue, Ahmed, Benard, Gerke and Carminati. This is an open-access article distributed under the terms of the Creative Commons Attribution License (CC BY). The use, distribution or reproduction in other forums is permitted, provided the original author(s) and the copyright owner(s) are credited and that the original publication in this journal is cited, in accordance with accepted academic practice. No use, distribution or reproduction is permitted which does not comply with these terms.
*Correspondence: Maire Holz, maire.holz@forst.uni-goettingen.de