- 1Department of Bioscience, Aarhus University, Silkeborg, Denmark
- 2Department of Agroecology, Aarhus University, Tjele, Denmark
Vegetated buffer strips are often established in agricultural landscapes as an “edge of the field” mitigation measure against diffuse nutrient pollution of the aquatic environment. Harvesting of the vegetation has been suggested as a possible management strategy to prevent build-up of excessive amounts of nutrients in buffer soils and, at the same time, harvesting can have a positive effect on the diversity of the vegetation. However, the response of the vegetation to harvesting likely depends on taxonomic and functional characteristics. In the present study, we explored effects of harvesting frequency (by comparing harvesting once, twice, and four times per year to an unharvested control) on the taxonomic and functional trait composition of four different types of plant communities in buffer strips in Denmark. We found that one to two harvests per year mediated an increase in the diversity of the vegetation in low diversity buffer strips dominated by tall and productive herbs (tall herb fringe), whereas the diversity remained unchanged in buffer strips dominated by grasses as well as in more diverse buffer strips with rich fen and wet meadow species. We also found that harvesting changed the compositional patterns in the tall herb fringe community, with an increasing abundance of grasses and a declining abundance of tall herb species, in particular at a high harvesting frequency. Concomitant with these taxonomic changes, we observed changes in the trait composition of the community. The abundance of tall species declined in the tall herb fringe and in the tall grass community. Similarly, the abundance of productive species (as indicated by high Ellenberg N and Ellenberg R values) declined in the tall herb fringe (in areas harvested four times per year). We conclude that low frequency harvesting is a promising management strategy to increase plant diversity in buffer strips with an initially low diversity and high productivity and that harvesting over time can mediate a shift in compositional patterns toward less productive species.
Introduction
Buffer strips, defined as narrow, uncultivated riparian margins between waterways and fields, are often established in agricultural landscapes to reduce nutrient loss from nearby fields to the aquatic environment (Osborne and Kovacic, 1993; Correll, 1996; Stutter et al., 2012). High nutrient concentrations endanger aquatic ecosystems (Carpenter et al., 1995; Correll, 1998) and may hinder fulfillment of ecological goals as defined, for instance, in the European Water Framework Directive (European Parliament and Council, 2000). Buffer strips in contrast to wider buffer zones (“conservation buffers” Lovell and Sullivan, 2006) are rarely implemented with the aim of biodiversity conservation, as they are too narrow and too much impacted by agricultural land-use practices to offer natural habitats. Thus, many buffer soils are enriched in nutrients, especially with phosphorus, as they have previously been part of the agricultural land (Meals et al., 2008). Concentrations may further increase with erosional sediment input as well as temporally when nutrients are being recycled during plant senescence (Syversen and Borch, 2005; Uusi-Kämppä, 2005). The latter process is accelerated with the increased microbial activity found in buffers (Stutter et al., 2009; Roberts et al., 2012; Dodd and Sharpley, 2016). Consequently, buffer strips can be a source of nutrient loss to the aquatic environment.
Harvesting (cutting and removal of biomass) has been suggested as a management method to counteract accumulation of nutrients in buffer soils (Osborne and Kovacic, 1993; Stutter et al., 2009), which will consequently reduce P loss to the aquatic environment (Mander et al., 1997; Mander and Lõhmus, 2006). At the same time, harvesting may affect the compositional patterns of the vegetation in buffer strips. Buffer strips are sometimes attributed a higher biodiversity, compared to the otherwise impoverished agricultural landscape; although they are usually not implemented with the goal of conservation. Their value for at least plant conservation has however often been questioned and, indeed, very few species of conservation value occur in Danish buffer strips (Hille et al., 2018). Harvesting, could present a management practice to improve the plant diversity value of buffer strips.
In grasslands, annual harvesting (mowing) has been found to increase plant diversity and alleviate the negative effect of high nutrient levels on diversity (e.g., Collins et al., 1998; Maron and Jefferies, 2001; Yang et al., 2012). This effect can be related to a trade-off often observed in natural communities between productivity and diversity, with highly productive communities having lower species richness and diversity (e.g., Grime, 1974; Baattrup-Pedersen et al., 2005) reflecting that productive species can exclude many smaller species because of shading. Additionally, productive species have a high litter production that may effectively hinder the establishment of new species (Foster and Gross, 1998; Socher et al., 2012). Harvesting represents a major disturbance to the vegetation since it leads to a decrease in community biomass by reducing the abundance of the most productive species (Huston, 2004). Harvesting can therefore affect the relative abundance of species with different competitive capabilities, while preventing the buildup of large amounts of plant litter.
The frequency of harvesting can affect the amount of nutrients removed; thus, more nutrients may be removed if the biomass is harvested several times during the growing season. However, it has been shown that a high harvesting frequency may have negative effects on the vegetation (Zechmeister et al., 2003; Socher et al., 2012, 2013). An intermediate harvesting frequency, representing an intermediate disturbance (Connell, 1978), might therefore be a compromise between maximizing nutrient removal as well as diversity in buffer strips as it might allow for the co-occurrence of species with divergent traits. Mostly the harvesting disturbance might prevent dominance of competitive species through reduced community biomass (Grime, 1973). The vulnerability of species to disturbance varies with their life strategy (Grime, 2006; Biswas and Mallik, 2010) and the response of the vegetation to harvesting may therefore depend on taxonomic and functional characteristics. For example, harvesting can promote species with basal growth (Belsky, 1992; Socher et al., 2013) because their growth meristem stays intact as opposed to species with apical growth.
In this study, we explored effects of harvesting frequency on the taxonomic and functional trait composition of the vegetation in Danish buffer strips. Plant communities in Danish buffer strips are generally highly productive (Hille et al., 2018) due to the high nutrient status of the soils and the generally high degree of human influence in the catchments (Kronvang et al., 2008). We analyzed structural and functional vegetation properties in four different types of plant communities. Our aim was to identify cross-regional patterns in the response of the vegetation. We hypothesized that the effects of harvesting depend on the characteristics of the vegetation. In particular the following hypotheses were tested: (1) harvesting mediates an increase in species diversity in low-diversity plant communities with predominance of highly productive species; (2) harvesting mediates a shift in species composition, the shift occurring faster with increased harvesting frequency; (3) effects of harvesting can be predicted from plant functional traits related to productivity (leaf mass, leaf dry matter content, specific leaf area, canopy height, abundance of competitors, preference for nutrients and soil acidity).
Methods
Study Sites and Experimental Set-Up
This study was conducted between 2014 and 2016 at two locations (Sillerup and Spjald) with differing soil types in Denmark. Sillerup is located in southern Jutland and receives on average 795 mm precipitation per year. The soil consists of clay and silt deposits. Spjald is located in western Jutland and receives on average 833 mm precipitation per year. The soil consists of glacial sand and gravel deposits. The experimental years 2014 and 2016 can be considered normal years with an average precipitation amount of 16% more and 7% less, respectively, than in a typical meteorological year, while 2015 was a wet year with 25% more precipitation than in a typical meteorological year (Danmarks Meteorologiske Institut, 2017).
We established 112 experimental plots (1 m2) distributed over 3 different sites per location, comprising 24 control plots (C, unharvested reference) and 88 treatment plots. Experimental plots within the same site were situated in close proximity to each other. The treatment plots were harvested (cutting and removal of plant material) with different frequencies. The vegetation was cut ~5 cm above the soil surface. A total of 24 plots were harvested once a year (H1) and four times a year (H4), respectively, and 40 plots were harvested twice a year (H2). Harvests were carried out in May (H4), June (H1, H2 and H4), July (H4), and August (H2 and H4). Plant species cover was registered by placing a frame (25 × 25 cm), divided into 16 sub-quadrats, in the center of each plot. Registrations were carried out in June (H1 and H2), July (C and H3), and August (H2). Species abundance in each plot was calculated as the sum of cover from all sub-quadrants divided by 16 (total number of sub-quadrants). Species were identified according to Mossberg and Stenberg (2014).
Data Analysis
Vegetation Types
We performed an initial Detrended Correspondence Analysis (DCA) in R (version 3.3.3; R Core Team, 2017) using species abundance data from the first assessment in July 2014 (function decorana, package “vegan”; Oksanen et al., 2017) in order to extract the major floristic gradients in the data set as ordination axes. From DCA scores 1–4, we calculated an Euclidean distance matrix (function vegdist, method “euclidean,” package “vegan”) for use in a hierarchical clustering (Ward's minimum variance clustering, function hclust, method “ward.D2,” package “stats”), which minimizes the within-group sum of squares (linear model criterion of least squares) to identify existing vegetation types in the experimental sites. When using DCA site scores to calculate an Euclidean distance matrix for the clustering instead of using the raw abundance data, a certain independence from the “double zero problem” (two sites being judged “similar” because both are lacking certain species) is achieved as DCA uses χ2 distance, which is not influenced by double zeros (Borcard et al., 2011). We chose four interpretable clusters by visual examination as these offered the most equal distribution of plots over clusters, while simultaneously representing ecological meaningful vegetation types (Borcard et al., 2011), although treatment H1 was not present in one of the clusters. We also determined indicator species for each vegetation type (R, function “indval,” package “labdsv”; Roberts, 2016; Appendix Table 1 in Supplementary Material).
Diversity Parameters
We calculated Shannon Diversity Index (H) and species richness (S) in R (function diversity, method “Shannon” and function specnumber, package “vegan”) to describe community diversity for each vegetation type and used these parameters as response variables to test for effects of harvesting.
Community Composition Parameters
We performed DCAs for each vegetation type identified by the cluster analysis using DCA1-3 site scores to describe changes in plant community composition over time. We proceeded by plotting the development in DCA1 site scores over time to visualize effects of harvesting over time. Additionally, we plotted species with DCA1 species scores >1 and <-1 as these were the most important for the change in DCA1 site scores. We furthermore used the DCA1-3 site scores as response variables to analyze the effect of harvesting on compositional patterns.
Plant Traits
Traits reflecting the productivity of the plant community, inferred from life-history traits, were: canopy height, specific leaf area, leaf dry mass and leaf mass. Trait information was downloaded as a subset of the LEDA trait database (Kleyer et al., 2008). Furthermore we used the abundance of competitive species (being one of Grime's life strategies), as these species exhibit high primary production due to effective use of resources (Grime, 1974). Finally we used the ecological preference of the community in terms of soil fertility (Ellenberg N) and soil pH (Ellenberg R; Hill and Carey, 1997; Schaffers and Sýkora, 2000) as traits related to productivity of the plants. Traits of the mentioned sources were recorded for northwestern Europe but we judged that the climatic conditions were similar enough to allow use of these traits in our study.
Community weighted means (CWM) were calculated for all traits as the mean trait expression multiplied by the abundance of species present (based on percent cover) within an experimental plot. Trait CWM were used as response variables to analyze effects of harvesting on the plant communities.
Test for Initial Differences in Response Variables Between Vegetation Types
We performed non-parametric, permutation-based pairwise tests (Nemenyi-Damico-Wolfe-Dunn test) in R (Hollander et al., 2013; package coin; Hothorn et al., 2008) to identify initial differences in response variables between vegetation types by using CWM from all experimental plots (control as well as treatments; see Appendix Table 2 in Supplementary Material for number of samples). The Nemenyi-Damico-Wolfe-Dunn test is an independence test based on Monte Carlo permutations with 50,000 iterations and includes a Tukey post-hoc test. We used this method since variance homogeneity of the residuals was not fulfilled.
Evaluation of Treatment Effects on Response Variables
In total, we had 422 observations of the 112 experimental plots over three summers (Appendix Table 2 in Supplementary Material shows the number of plots studied per vegetation type and treatment within a year) and encountered a total of 91 plant taxa. We square root transformed species richness for a better fit with normal distribution. We calculated single Linear Mixed Effects Models (LMEMs) for each vegetation type and year on the response variables (Shannon diversity, species richness, DCA site scores 1–3, plant traits related to productivity as described above) in SAS (version 9.4) to test for effects of harvesting on plant diversity, plant community composition, and functional trait expression. We used location (Sillerup and Spjald) and site (3 sites per location) as random effects and treatment (C, H1, H2, and H4) as fixed effect. The model used for calculating the effect on each response variable being:
where Y is the vector of the response variable, μ is the vector of the effects of treatment, Z is the design matrix for the random effects [location*site(treatment)], u is the vector of the random effects and ε is the vector of the random errors. The LMEMs used in this study implemented a likelihood-based method (REML) to estimate the covariance structure, which accounts for missing data and the slightly unbalanced sampling design in this study (as derived from the SAS/STAT 9.2 users guide; Rubin, 1976; Little, 1995).
Results
We identified four different vegetation types based on the initial clustering of the DCA scores of species recorded in the experimental plots in the studied buffer strips: a tall herb fringe, a tall grass community, a ryegrass-dominated lower herb/grass community and a rich fen/wet meadow community (Appendix Image 1 in Supplementary Material). Indicator species for each vegetation type are given in Appendix Table 1 (Supplementary Material). Although the soil type varied, revealed the cluster analysis that vegetation types were more similar between than within location, indicating that soil type was less important for the vegetation types than other factors i.e., soil nutrient content (Appendix Table 3 in Supplementary Material).
Productivity and diversity varied significantly among the four vegetation types at the beginning of the experiment. The tall herb fringe was the most productive as inferred from CWM for soil fertility (mean Ellenberg N 7.06, Appendix Table 3 in Supplementary Material), soil pH (Ellenberg R 6.77), as well as canopy height (1.42 m) and from the abundance of competitive species (68%). The tall grass community had intermediate productivity, while the rich fen/wet meadow and the ryegrass-dominated lower herb/grass community were the least productive. The rich fen/wet meadow was the most diverse based on both Shannon Diversity (mean 2.16, Appendix Table 3 in Supplementary Material) and species richness (12.85), the tall grass community and the tall herb fringe had intermediate diversity (H = 1.68 and 1.63, S = 7.04 and 7.25, respectively) and the ryegrass-dominated lower herb/grass community was the least diverse (H = 1.09 and S = 5.96).
Harvesting of the vegetation mediated an increase in Shannon diversity in the tall herb fringe (Figure 1, LMEM, adj. p < 0.05, Appendix Table 4 in Supplementary Material) but not in the tall grass community (LMEM, adj. p > 0.05), the ryegrass-dominated lower herb/grass community, LMEM, adj. p > 0.05) or in the rich fen/wet meadow (LMEM, adj. p > 0.05). The increase in Shannon diversity in the tall herb fringe was significant after 2 years of harvesting in plots harvested twice per year and after 3 years of harvesting in plots harvested once per year (Figure 1, LMEM, adj. p < 0.05, Appendix Table 4 in Supplementary Material). Similarly, species richness increased in the tall herb fringe after 2 years in plots harvested twice per year and after 3 years in plots harvested once per year (Figure 2, LMEM, adj. p < 0.05, Appendix Table 4 in Supplementary Material) but did not change in the other vegetation types studied. In contrast, no significant effect of harvesting on Shannon diversity or species richness was found in plots harvested four times per year (Figures 1, 2, LMEM, adj. p > 0.05).
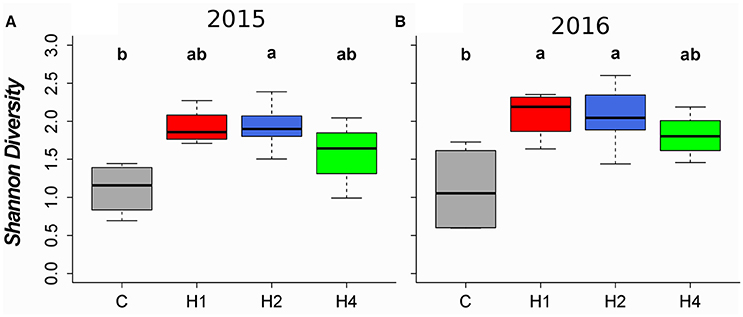
Figure 1. Boxplots of Shannon diversity for the second and third experimental year for the tall herb fringe community. Horizontal lines indicate the median, boxes the interquartile range and whiskers the 95% interval of the data. (A) 2015, (B) 2016. Treatments: C, control; H1, harvest once per year; H2, harvest twice per year; H4, harvest four times per year. Different letters indicate significant difference (p < 0.05) tested by linear mixed effects model with post-hoc test for pairwise comparisons (for details, see Appendix Table 4 in Supplementary Material). Community-weighted medians (min, max) after the first experimental year (non-significant, LMEM, see Appendix Table 5 in Supplementary Material) as well as boxplots for the other tested community types (non-significant, LMEM) can be found in Appendix Image 2 (Supplementary Material).
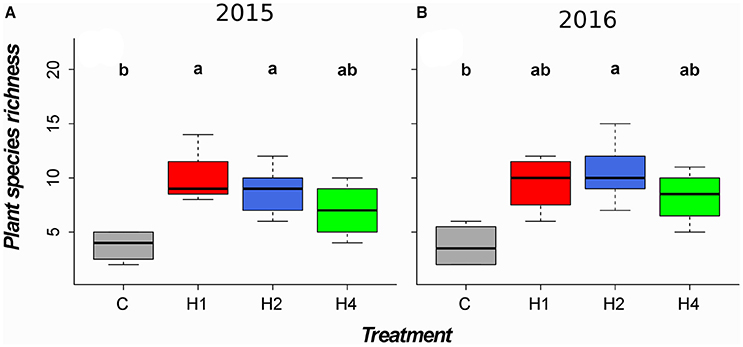
Figure 2. Boxplots of plant species richness for the second and third experimental year for the tall herb fringe community. Horizontal lines indicate the median, boxes the interquartile range and whiskers the 95% interval of the data. Panel and treatment designation as in Figure 1. Different letters indicate significant difference (p < 0.05) tested by linear mixed effects model with post-hoc test for pairwise comparisons (for details, see Appendix Table 4 in Supplementary Material). Community-weighted medians (min, max) after the first experimental year (non-significant, LMEM, see Appendix Table 5 in Supplementary Material) as well as boxplots for the other tested community types (non-significant, LMEM) can be found in Appendix Image 3 (Supplementary Material). (A) 2015, (B) 2016.
A significant shift in species composition occurred in the tall herb fringe (although only significant after 2 years; Figure 3; LMEM, adj. p < 0.05, Appendix Table 4 in Supplementary Material) but not in the other vegetation types studied (LMEM, adj. p > 0.05). The species shift occurred more rapidly in plots harvested four times per year compared with those harvested once or twice per year (Figure 3; LMEM, adj. p < 0.05, Appendix Table 4 in Supplementary Material). Harvesting affected herbs and grasses differently (Figure 3). This is clearly illustrated by a decline in the abundance of many tall herb species in response to harvesting (e.g., Urtica dioica L., Epilobium hirsutum L., Valeriana sambucifolia J.C. MIKAN EX POHL), while the abundance of several grass species (e.g., Alopecurus geniculatus L., Holcus lanatus L., Poa pratensis L.) and some smaller herbs (e.g., Taraxacum sp. F.H.WIGG., Cerastium fontanum BAUMG., Galeopsis sp. L.) increased.
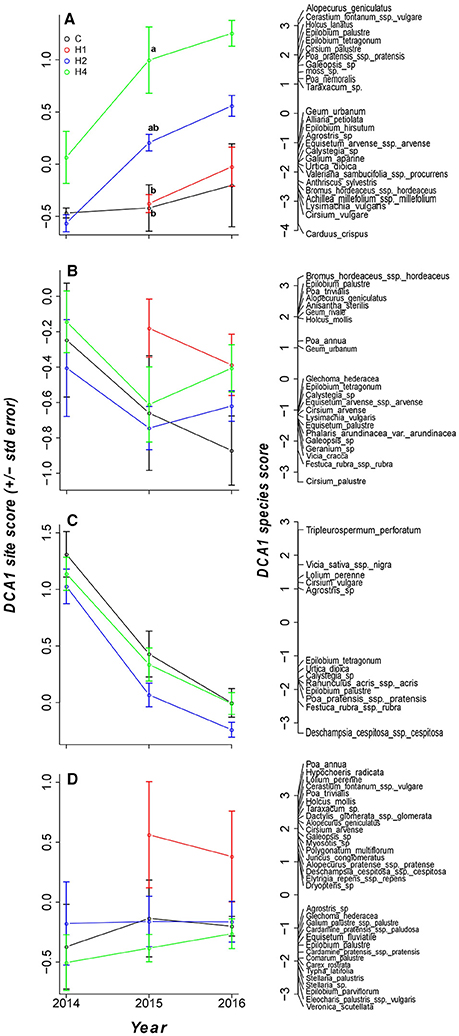
Figure 3. Mean (standard error) of DCA 1 site scores of all vegetation types plotted over time with DCA1 species scores for each vegetation type. Treatment designation as in Figure 1. Different letters indicate significant difference (p < 0.05) tested by linear mixed effects model with post-hoc test for pairwise comparisons (for details, see Appendix Table 4 in Supplementary Material). Only species with scores < -1 or >1 are plotted. (A) Tall herb fringe, (B) Tall grass community, (C) Ryegrass-dominated lower herb/grass community, (D) Rich fen/wet meadow.
In contrast to the observed increase in diversity and the species shift in the tall herb fringe, harvesting mediated a significant decrease in productivity-related traits, including canopy height (Figure 4; LMEM, adj. p < 0.05, Appendix Table 4 in Supplementary Material), preference for soil fertility (Figure 5; LMEM, adj. p < 0.05, Appendix Table 4 in Supplementary Material) and preference for soil pH (not shown; LMEM, adj. p < 0.05, Appendix Table 4 in Supplementary Material) after 3 years in plots harvested four times per year. In the tall grass community, canopy height decreased after 3 years in plots harvested two and four times per year (Figure 4; LMEM, adj. p < 0.05, Appendix Table 4 in Supplementary Material), but preferences for soil fertility and soil pH did not change (LMEM, adj. p > 0.05). In the ryegrass-dominated lower herb/grass community as well as in the rich fen/wet meadow, we found no significant effect of harvesting on productivity-related traits (LMEM, adj. p > 0.05).
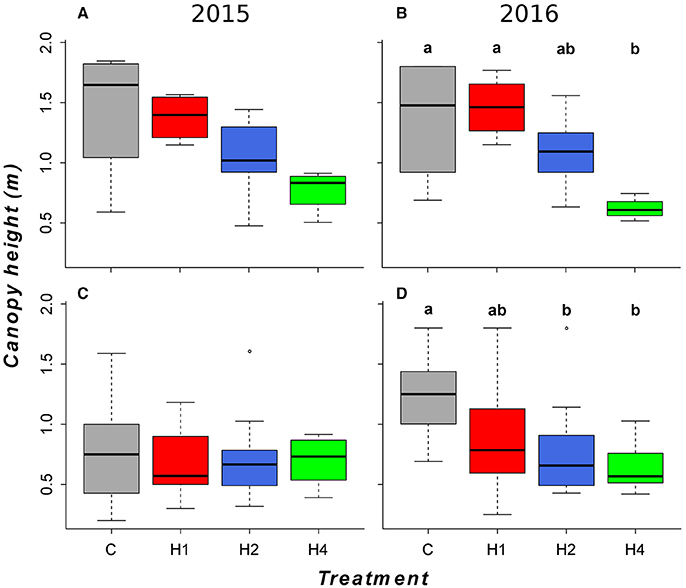
Figure 4. Boxplots of community canopy height for the second and third experimental year for the tall herb fringe (A,B) and the tall grass communities (C,D). (A,C) 2015, (B,D) 2016. Horizontal lines indicate the median, boxes the interquartile range and whiskers the 95% interval of the data. Treatment designation as in Figure 1. Different letters indicate significant difference (p < 0.05) tested by linear mixed effects model with post-hoc test for pairwise comparisons (for details, see Appendix Table 4 in Supplementary Material. Community-weighted medians (min, max) after the first experimental year (non-significant, LMEM, see Appendix Table 5 in Supplementary Material) as well as boxplots for the other tested community types (non-significant, LMEM) can be found in Appendix Image 4 (Supplementary Material).
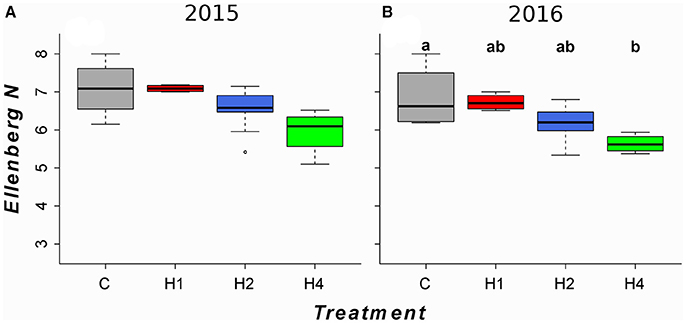
Figure 5. Boxplots of community-weighted preference for soil fertility (Ellenberg N) for the second and third experimental year for the tall herb fringe community. Horizontal lines indicate the median, boxes the interquartile range and whiskers the 95% interval of the data. Panel and treatment designation as in Figure 1. Different letters indicate significant difference (p < 0.05) tested by linear mixed effects model with post-hoc test for pairwise comparisons (for details, see Appendix Table 4 in Supplementary Material). Community-weighted medians (min, max) after the first experimental year (non-significant, LMEM, see Appendix Table 5 in Supplementary Material) as well as boxplots for the other tested community types (non-significant, LMEM) can be found in Appendix Image 5 (Supplementary Material). (A) 2015, (B) 2016.
We did not find any significant effects of harvesting on the abundance of competitive species, specific leaf area, leaf dry matter content or leaf mass in any of the four vegetation types (LMEM, adj. p > 0.05), nor did we find any significant effects of harvest on any of the response variables (diversity parameters, DCA site scores, productivity related plant traits) after one year of harvesting (see Appendix Table 5 in Supplementary Material for CWM).
Discussion
Effect of Harvesting on Species Diversity
In accordance with our first hypothesis, we found an increase in diversity in the tall herb fringe in response to harvesting but not in the tall grass community, the ryegrass-dominated lower herb/grass community or the rich fen/wet meadow. The tall herb fringe was the most productive community initially in comparison to the other communities. At the same time, the tall herb fringe displayed intermediate diversity, whereas the rich fen/wet meadow and the ryegrass-dominated lower herb/grass community displayed higher and lower diversity, respectively. We did not observe an increase in the diversity of the tall grass community in response to harvesting even though the diversity of this community was as low as in the tall herb fringe. This finding indicates that productivity rather than diversity determined the response of the vegetation to harvesting.
Species richness increased in response to both one and two annual harvests after 2 years and the increase became even more pronounced following two harvests after 3 years. This finding indicates that harvesting facilitated the establishment of new species in the community (Burke and Grime, 1996). In particular, we observed that, for instance, Poa nemoralis L. (in year 2), Epilobium tetragonum L., and Galeopsis sp. (both in year 3) established in the area. Shannon diversity also increased in plots harvested twice per year after 2 years and increased both in plots harvested once and twice per year after 3 years of harvesting, further indicating that the species that entered the community became established and even proliferated, creating a more even distribution after the third year.
In accordance with our findings, it has been observed that one or two harvests a year resulted in the highest plant diversity in grassland experiments (Socher et al., 2013), while higher harvesting frequency affected plant diversity negatively (Zechmeister et al., 2003; Socher et al., 2012). In the before mentioned studies, as well as in the four harvests per year treatment in our study, more frequent harvesting also meant harvesting earlier in the season. This might have led to discrimination against species unable to reproduce vegetatively as well as species flowering late in the season by preventing their reproduction (Smith et al., 2000). An extensive harvest of one cut conducted later in the year might have yielded higher diversity in the grass-dominated vegetation types (tall grass community and ryegrass-dominated lower herb/grass community) as shown by others (e.g., Smith et al., 2000; Knop et al., 2006).
Effect of Harvesting on Species Composition
In accordance with the second hypothesis, we also observed a shift in species composition in the tall herb fringe (highest deviation in species composition between control and treatments) but not in the other vegetation types studied. Harvesting led to a shift from a community dominated by tall herbs to a community where smaller herbs and grasses were more evenly distributed. For example, we observed a shift in Epilobium species from the highly productive and tall E. hirsutum toward the smaller E. tetragonum and E. palustre. This effect was not found in the more diverse rich fen/wet meadow, which comprised less productive species from the beginning and thus was more stable and more resilient to disturbance (Tilman and Downing, 1994).
We also observed that four harvests a year led to a decline in the abundance of tall herbs and an increase in the abundance of grasses in the tall herb fringe, which is consistent with the results of other studies testing the effect of disturbances on plant species with different morphologies (Belsky, 1992; Socher et al., 2013). More space due to disturbance allows for more light to filter through the canopy and diminishes competitive exclusion of less productive species by highly productive species (Huston and DeAngelis, 1994). Even though we did not find a significant decline in the abundance of competitive species in response to harvesting, we observed that some tall species were replaced with less tall species. We found that the highly productive and tallest species (like E. hirsutum and U. dioica) were negatively affected and that species with smaller growth forms (e.g., rosettes, like Taraxacum sp.) were positively affected by harvesting. Similarly, harvesting reduced competition since the abundance of tall species was more strongly reduced compared to that of smaller species (Norman, 1960), indicating that shorter species benefitted from improved light conditions (Belsky, 1992). By reducing the abundance of tall species, harvesting yielded more space for species to coexist in a small area (Parr and Way, 1988). This has been shown before for grasses in relation to the removal of litter in connection with harvesting (Fynn et al., 2004).
Grasses are better adapted to disturbances such as cutting than herbs, especially the rhizomatous grass species H. lanatus L. and P. pratensis L, since grazing has been a major driver in their evolution (Mack and Thompson, 1982), which makes them effective in spreading vegetatively and persisting under stress. This might also explain why we did not find an effect of harvesting on species composition or diversity in the less productive tall grass and ryegrass-dominated lower herb/grass communities, which contained proportionally more grasses and smaller species and thus were less likely to be affected by harvesting.
Significant effects on species composition were found after 2 years but not after 3 years of the experiment in the tall herb fringe. We have no explanation for this, but this might reflect that the variation in compositional patterns in the control plots increased. Thus, we observed that species composition in the controls diverged after 3 years similar to what was observed for the harvest treatments (as inferred from DCA scores, Figure 3). Longer studies than ours are required to analyze the natural variation in species composition in buffer strips.
Effect of Harvesting on Trait Expression
In accordance with the third hypothesis, the effect of harvesting was clearly related to the productivity level of the plant communities. The preferences for soil fertility and soil reaction, as well as mean community canopy height, were significantly reduced following four annual harvests after the third year. This reduction in productivity-related traits clearly followed the change in species composition. Canopy height was also affected in the tall grass community by two and four harvests in the third year; however, there was no significant change in species composition in this community, probably due to high variability in the data.
The species we found to benefit from low frequency harvesting were smaller herbs (e.g., Galeopsis sp., Taraxacum sp.) and grasses (e.g., P. nemoralis, P. pratensis) that can form a dense and rapidly repairing leave canopy close to the ground (Grime, 2002). We propose that the change in species community over time can lead to communities that are less competitive, a trend that—albeit not significant—is clearly visible in the tall herb fringe and to a lesser extent in the tall grass community after the third year (Appendix Image 6 in Supplementary Material); thus, the shift from tall species toward less tall species. This has also been described by Grime (1977) who identified ruderals and not competitors as being the best adapted to high stress in productive habitats. We acknowledge that our experiment might have been too short to capture the whole extent of the effect that harvest can have on buffer plant communities, since the communities' response to disturbance is determined by both the length of time that individual species require to react to disturbances, the interaction of species in response to disturbances and, finally, to temporally varying environmental conditions during the time of the disturbance (Belsky, 1992). Thus, a continued harvesting over several years at our study sites may lead to a significant increase in diversity and a decrease in traits related to community productivity also in the tall grass community. This also implies that the potential of removing nutrients by harvesting buffer vegetation may diminish over time (shown for at least N; Tanner, 1996).
Consequences for Floristic Quality of Buffer Strips
Overall, the increase in grasses and the decrease in tall herbs made the studied vegetation types more similar following 3 years of applying the most intensive harvesting treatment; a homogenization of the vegetation that has been noted before for land-use intensification in the form of enhanced mowing frequency (Zechmeister et al., 2003). In this study, we used plant species diversity and richness to assess the plant community in buffer strips, but we acknowledge that these features give little indication about the floristic quality of the vegetation (Bowers and Boutin, 2008), and even though we found an increase in diversity due to the treatments in the tall herb fringe, we found no rare species entering the community.
Once species become rare in an area, the soil seed bank and the seed rain become insufficient to create a viable community. Additionally, seedlings of rare species have a high mortality (Poschlod et al., 1998), which is most likely linked to unfavorable abiotic conditions such as, for instance, elevated nutrient status of the soils (Bakker and Berendse, 1999). Other studies have found that for conservation purposes and establishment of species-rich communities, a reduction in soil nutrient levels (mainly phosphorus and nitrogen) is compulsory (Gough and Marrs, 1990; Pallett et al., 2016). Besides having a high nutrient status, soils in buffer strips are frequently drier than natural riparian areas (Hille et al., 2018) due to the high degree of drainage and channelization of streams in agricultural areas, which have probably turned them into unsuitable habitats for rare wetland species. Harvesting, which leads to removal of nutrients from the buffer soil, could in the long term create more favorable conditions for rare species, maybe in combination with sowing, to help establish viable populations. However, for the re-establishment of riparian or wetland species and to improve the floristic quality of buffer strips, an additional reconnection of the stream with the riparian zone through, for instance, widening of stream channels and buffers (Dybkjær et al., 2012) might also be necessary.
Nevertheless, the improved plant diversity due to low frequency harvesting with a concomitant increase of not only grasses but also of smaller herbs can be viewed as a desirable development—with the reduction of the most competitive species in the tall herb fringe studied, this vegetation community type could over time develop into a hydrophilous tall herb fringe (Natura 2000 code: 6430; Fredshavn et al., 2014), which is a protected habitat according to the EU Habitats Directive.
Conclusions
Since the buffer vegetation is highly productive, we strongly recommend harvesting (cutting and removal) of the vegetation on a regular basis as a management practice to remove nutrients and to improve diversity. In our study, we found that one or two harvests per year had a positive effect on plant diversity in communities with initially low to intermediate diversity and a high productivity dominated by tall species. A higher harvest frequency did not affect diversity but might have an undesirable homogenizing effect on the vegetation.
In conclusion, we suggest that a twofold benefit can be obtained by introducing low frequency harvesting in buffer strips. Firstly, a diversity increase with a concomitant reduction in the frequency of highly productive species and, secondly, a steady removal of nutrients from the buffer strip soil, which over time may induce even higher plant diversity. Harvesting over time, however, can mediate a change in the vegetation toward dominance of less productive species. Since species productivity can influence nutrient removal, harvesting could lead to less removal if the vegetation changes, a possibility that requires further research.
Author Contributions
SH, AB-P, and GR conceived the study and wrote the manuscript. SL analyzed the data and contributed to the manuscript writing. BK revised the manuscript.
Funding
The work is supported by the Strategic Research Foundation/Innovation Fund Denmark project BUFFERTECH—Optimization of Ecosystem Services Provided by Buffer Strips Using Novel Technological Methods' (Grant No. 1305-00017B).
Conflict of Interest Statement
The authors declare that the research was conducted in the absence of any commercial or financial relationships that could be construed as a potential conflict of interest.
Acknowledgments
We thank Henrik Stenholt and Tommy Silberg for field work assistance and Anne Mette Poulsen for linguistic improvements of the manuscript.
Supplementary Material
The Supplementary Material for this article can be found online at: https://www.frontiersin.org/articles/10.3389/fenvs.2018.00058/full#supplementary-material
References
Baattrup-Pedersen, A., Friberg, N., Larsen, S. E., and Riis, T. (2005). The influence of channelisation on riparian plant assemblages. Freshw. Biol. 50, 1248–1261. doi: 10.1111/j.1365-2427.2005.01383.x
Bakker, J. P., and Berendse, F. (1999). Constraints in the restoration of ecological diversity in grassland and heathland communities. Trends Ecol. Evol. 14, 63–68. doi: 10.1016/S0169-5347(98)01544-4
Belsky, A. J. (1992). Effects of grazing, competition, disturbance and fire on species composition and diversity in grassland communities. J. Veg. Sci. 3, 187–200. doi: 10.2307/3235679
Biswas, S. R., and Mallik, A. U. (2010). Disturbance effects on species diversity and functional diversity in riparian and upland plant communities. Ecology 91, 28–35. doi: 10.1890/08-0887.1
Bowers, K., and Boutin, C. (2008). Evaluating the relationship between floristic quality and measures of plant biodiversity along stream bank habitats. Ecol. Indic. 8, 466–475. doi: 10.1016/j.ecolind.2007.05.001
Burke, M. J. W., and Grime, J. P. (1996). An experimental study of plant community invasibility. Ecology 77, 776–790. doi: 10.2307/2265501
Carpenter, S. R., Chisholm, S. W., Krebs, C. J., Schindler, D. W., and Wright, R. F. (1995). Ecosystem experiments. Science 269, 324–327. doi: 10.1126/science.269.5222.324
Collins, S. L., Knapp, A. K., Riggs, J. M. B., Blair, J. M., and Steinauer, E. M. (1998). Modulation of diversity by grazing and mowing in native tallgrass prairie. Science 280, 745–747. doi: 10.1126/science.280.5364.745
Connell, J. H. (1978). Diversity in tropical rain forests and coral reefs. Science 199, 1302–1310. doi: 10.1126/science.199.4335.1302
Correll, D. L. (1996). “Buffer zones and water quality protection: general principles,” in Buffer Zones: Their Processes and Potential in Water Protection Conference Handbook (Harpenden: Quest Environmental), 7–20. Available online at: https://extension.umd.edu/sites/default/files/_docs/programs/riparianbuffers/correll.pdf (Accessed March 18, 2016).
Correll, D. L. (1998). The role of phosphorus in the eutrophication of receiving waters: a review. J. Environ. Qual. 27, 261–266. doi: 10.2134/jeq1998.00472425002700020004x
Danmarks Meteorologiske Institut (2017). DMI - Vejr Klima Hav. Available online at: http://www.dmi.dk/vejr/arkiver/vejrarkiv/
Dodd, R. J., and Sharpley, A. N. (2016). Conservation practice effectiveness and adoption: unintended consequences and implications for sustainable phosphorus management. Nutr. Cycl. Agroecosyst. 104, 373–392. doi: 10.1007/s10705-015-9748-8
Dybkjær, J. B., Baattrup-Pedersen, A., Kronvang, B., and Thodsen, H. (2012). Diversity and distribution of riparian plant communities in relation to stream size and eutrophication. J. Environ. Qual. 41, 348–354. doi: 10.2134/jeq2010.0422
European Parliament and Council (2000). Establishing a Framework for Community Action in the Field of Water Policy. Available online at: http://eur-lex.europa.eu/legal-content/EN/TXT/?uri=CELEX:32000L0060
Foster, B. L., and Gross, K. L. (1998). Species richness in a successional grassland: effects of nitrogen enrichment and plant litter. Ecology 79, 2593–2602. doi: 10.1890/0012-9658(1998)079[2593:SRIASG]2.0.CO;2
Fredshavn, J., Søgaard, B., Nygaard, B., Sander Johansson, L., Wiberg-Larsen, P., Dahl, K., et al. (2014). Bevaringsstatus for Naturtyper og Arter. Technical Report No 98. Aarhus Universitet, DCE-Nationalt Center for Miljøog Energi. Available online at: http://dce.au.dk/
Fynn, R. W. S., Morris, C. D., Edwards, T. J., and Skarpe, C. (2004). Effect of burning and mowing on grass and forb diversity in a long-term grassland experiment. Appl. Veg. Sci. 7, 1–10. doi: 10.1111/j.1654-109X.2004.tb00589.x
Gough, M. W., and Marrs, R. H. (1990). A comparison of soil fertility between semi-natural and agricultural plant communities: implications for the creations of species-rich grassland on abondoned agricultural land. Biol. Conserv. 51, 83–96. doi: 10.1016/0006-3207(90)90104-W
Grime, J. P. (1973). Competitive exclusion in herbaceous vegetation. Nature 242, 344–347. doi: 10.1038/242344a0
Grime, J. P. (1974). Vegetation classification by reference to strategies. Nature 250, 26–31. doi: 10.1038/250026a0
Grime, J. P. (1977). Evidence for the existence of three primary strategies in plants and its relevance to ecological and evolutionary theory. Am. Nat. 111, 1169–1194. doi: 10.1086/283244
Grime, J. P. (2002). Plant Strategies, Vegetation Processes, and Ecosystem Properties, 2nd Edn. Chichester: John Wiley & Sons.
Grime, J. P. (2006). Trait convergence and trait divergence in herbaceous plant communities: mechanisms and consequences. J. Veg. Sci. 17, 255–260. doi: 10.1111/j.1654-1103.2006.tb02444.x
Hill, M. O., and Carey, P. D. (1997). Prediction of yield in the Rothamsted Park Grass Experiment by Ellenberg indicator values. J. Veg. Sci. 8, 579–586. doi: 10.2307/3237210
Hille, S., Andersen, D. K., Kronvang, B., and Baattrup-Pedersen, A. (2018). Structural and functional characteristics of buffer zone vegetation in an agricultural landscape – high potential for nutrient removal but low potential for plant biodiversity. Sci. Total Environ. 628–629C, 805–814. doi: 10.1016/j.scitotenv.2018.02.117
Hollander, M., Wolfe, D. A., and Chicken, E. (2013). Nonparametric Statistical Methods, 3rd Edn. Weinheim: John Wiley & Sons.
Hothorn, T., Hornik, K., van de Wiel, M. A., and Zeileis, A. (2008). Implementing a class of permutation tests: the coin package. J. Stat. Softw. 1:8. doi: 10.18637/jss.v028.i08
Huston, M. A. (2004). Management strategies for plant invasions: manipulating productivity, disturbance, and competition. Divers. Distrib. 10, 167–178. doi: 10.1111/j.1366-9516.2004.00083.x
Huston, M. A., and DeAngelis, D. L. (1994). Competition and coexistence: the effects of resource transport and supply rates. Am. Nat. 144, 954–977. doi: 10.1086/285720
Kleyer, M., Bekker, R. M., Knevel, I. C., Bakker, J. P., Thompson, K., Sonnenschein, M., et al. (2008). The LEDA Traitbase: a database of life-history traits of the Northwest European flora. J. Ecol. 96, 1266–1274. doi: 10.1111/j.1365-2745.2008.01430.x
Knop, E., Kleijn, D., Herzog, F., and Schmid, B. (2006). Effectiveness of the Swiss agri-environment scheme in promoting biodiversity. J. Appl. Ecol. 43, 120–127. doi: 10.1111/j.1365-2664.2005.01113.x
Kronvang, B., Andersen, H. E., Børgesen, C., Dalgaard, T., Larsen, S. E., Bøgestrand, J., et al. (2008). Effects of policy measures implemented in Denmark on nitrogen pollution of the aquatic environment. Environ. Sci. Policy 11, 144–152. doi: 10.1016/j.envsci.2007.10.007
Little, R. J. A. (1995). Modeling the drop-out mechanism in repeated-measures studies. J. Am. Stat. Assoc. 90, 1112–1121. doi: 10.1080/01621459.1995.10476615
Lovell, S. T., and Sullivan, W. C. (2006). Environmental benefits of conservation buffers in the United States: evidence, promise, and open questions. Agric. Ecosyst. Environ. 112, 249–260. doi: 10.1016/j.agee.2005.08.002
Mack, R. N., and Thompson, J. N. (1982). Evolution in Steppe with few large, hooved mammals. Am. Nat. 119, 757–773. doi: 10.1086/283953
Mander, Ü., and Lõhmus, K. (2006). Dynamics of Nitrogen and Phosphorus Budgets in Riparian Buffer Zones. Tartu.
Mander, Ü., Kuusemets, V., Lõhmus, K., and Mauring, T. (1997). Efficiency and dimensioning of riparian buffer zones in agricultural catchments. Ecol. Eng. 8, 299–324. doi: 10.1016/S0925-8574(97)00025-6
Maron, J. L., and Jefferies, R. L. (2001). Restoring enriched grasslands: effects of mowing on species richness, productivity, and nitrogen retention. Ecol. Appl. 11, 1088–1100. doi: 10.1890/1051-0761(2001)011[1088:REGEOM]2.0.CO;2
Meals, D. W., Cassell, E. A., Hughell, D., Wood, L., Jokela, W. E., and Parsons, R. (2008). Dynamic spatially explicit mass-balance modeling for targeted watershed phosphorus management: II. Model application. Agric. Ecosyst. Environ. 127, 223–233. doi: 10.1016/j.agee.2008.04.005
Mossberg, B., and Stenberg, L. (eds.). (2014). Den Nye Nordiske Flora, 2nd Edn. København K: Gyldendal.
Norman, M. J. T. (1960). The relationship between competition and defoliation in pasture. Grass Forage Sci. 15, 145–149. doi: 10.1111/j.1365-2494.1960.tb00170.x
Oksanen, J., Blanchet, F. G., Kindt, R., Legendre, P., Minchin, P. R., O'Hara, R. B., et al. (2017). vegan: Community Ecology Package. Available online at: http://CRAN.R-project.org/package=vegan
Osborne, L. L., and Kovacic, D. A. (1993). Riparian vegetated buffer strips in water-quality restoration and stream management. Freshw. Biol. 29, 243–258. doi: 10.1111/j.1365-2427.1993.tb00761.x
Pallett, D. W., Pescott, O. L., and Schäfer, S. M. (2016). Changes in plant species richness and productivity in response to decreased nitrogen inputs in grassland in southern England. Ecol. Indic. 68, 73–81. doi: 10.1016/j.ecolind.2015.12.024
Parr, T. W., and Way, J. M. (1988). Management of roadside vegetation: the long-term effects of cutting. J. Appl. Ecol. 25, 1073–1087. doi: 10.2307/2403767
Poschlod, P., Kiefer, S., Tränkle, U., Fischer, S., and Bonn, S. (1998). Plant species richness in calcareous grasslands as affected by dispersability in space and time. Appl. Veg. Sci. 1, 75–91. doi: 10.2307/1479087
R Core Team (2017). R: A Language and Environment for Statistical Computing. Vienna: R Foundation for Statistical Computing. Available online at: http://www.R-project.org/
Roberts, D. W. (2016). labdsv: Ordination and Multivariate Analysis for Ecology. Available online at: https://CRAN.R-project.org/package=labdsv
Roberts, W. M., Stutter, M. I., and Haygarth, P. M. (2012). Phosphorus retention and remobilization in vegetated buffer strips: a review. J. Environ. Qual. 41, 389–399. doi: 10.2134/jeq2010.0543
Rubin, D. B. (1976). Inference and missing data. Biometrika 63, 581–592. doi: 10.1093/biomet/63.3.581
Schaffers, A. P., and Sýkora, K. V. (2000). Reliability of ellenberg indicator values for moisture, nitrogen and soil reaction: a comparison with field measurements. J. Veg. Sci. 11, 225–244. doi: 10.2307/3236802
Smith, R. S., Shiel, R. S., Millward, D., and Corkhill, P. (2000). The interactive effects of management on the productivity and plant community structure of an upland meadow: an 8-year field trial. J. Appl. Ecol. 37, 1029–1043. doi: 10.1046/j.1365-2664.2000.00566.x
Socher, S. A., Prati, D., Boch, S., Müller, J., Baumbach, H., Gockel, S., et al. (2013). Interacting effects of fertilization, mowing and grazing on plant species diversity of 1500 grasslands in Germany differ between regions. Basic Appl. Ecol. 14, 126–136. doi: 10.1016/j.baae.2012.12.003
Socher, S. A., Prati, D., Boch, S., Müller, J., Klaus, V. H., Hölzel, N., et al. (2012). Direct and productivity-mediated indirect effects of fertilization, mowing and grazing on grassland species richness. J. Ecol. 100, 1391–1399. doi: 10.1111/j.1365-2745.2012.02020.x
Stutter, M. I., Chardon, W. J., and Kronvang, B. (2012). Riparian buffer strips as a multifunctional management tool in agricultural landscapes: introduction. J. Environ. Qual. 41, 297. doi: 10.2134/jeq2011.0439
Stutter, M. I., Langan, S. J., and Lumsdon, D. G. (2009). Vegetated buffer strips can lead to increased release of phosphorus to waters: a biogeochemical assessment of the mechanisms. Environ. Sci. Technol. 43, 1858–1863. doi: 10.1021/es8030193
Syversen, N., and Borch, H. (2005). Retention of soil particle fractions and phosphorus in cold-climate buffer zones. Ecol. Eng. 25, 382–394. doi: 10.1016/j.ecoleng.2005.06.005
Tanner, C. C. (1996). Plants for constructed wetland treatment systems — A comparison of the growth and nutrient uptake of eight emergent species. Ecol. Eng. 7, 59–83. doi: 10.1016/0925-8574(95)00066-6
Tilman, D., and Downing, J. A. (1994). Biodiversity and stability in grasslands. Nature 367, 363–365. doi: 10.1038/367363a0
Uusi-Kämppä, J. (2005). Phosphorus purification in buffer zones in cold climates. Ecol. Eng. 24, 491–502. doi: 10.1016/j.ecoleng.2005.01.013
Yang, H., Jiang, L., Li, L., Li, A., Wu, M., and Wan, S. (2012). Diversity-dependent stability under mowing and nutrient addition: evidence from a 7-year grassland experiment. Ecol. Lett. 15, 619–626. doi: 10.1111/j.1461-0248.2012.01778.x
Keywords: buffer strips, harvest, plant traits, productivity, diversity, vegetation types
Citation: Hille S, Larsen SE, Rubæk GH, Kronvang B and Baattrup-Pedersen A (2018) Does Regular Harvesting Increase Plant Diversity in Buffer Strips Separating Agricultural Land and Surface Waters? Front. Environ. Sci. 6:58. doi: 10.3389/fenvs.2018.00058
Received: 14 March 2018; Accepted: 31 May 2018;
Published: 19 June 2018.
Edited by:
Eric O. Young, William H. Miner Agricultural Research Institute, United StatesReviewed by:
Mary Alldred, SUNY Plattsburgh, United StatesJennifer Lesley Silcock, The University of Queensland, Australia
Copyright © 2018 Hille, Larsen, Rubæk, Kronvang and Baattrup-Pedersen. This is an open-access article distributed under the terms of the Creative Commons Attribution License (CC BY). The use, distribution or reproduction in other forums is permitted, provided the original author(s) and the copyright owner are credited and that the original publication in this journal is cited, in accordance with accepted academic practice. No use, distribution or reproduction is permitted which does not comply with these terms.
*Correspondence: Sandra Hille, shi@bios.au.dk