- 1Dipartimento di Scienze e Tecnologie, Università degli Studi di Napoli “Parthenope”, CoNISMa, Naples, Italy
- 2Istituto di Scienze dell'Atmosfera e del Clima, CNR, UOS Roma, Rome, Italy
Concern about marine litter has been rising in the last decades, triggered by the discovery of the great mid-ocean garbage patches. The Mediterranean Sea is strongly affected by the presence of floating litter, as it has a very high amount of waste generated annually per person that eventually ends up in its waters, with plastic objects accounting for a large percentage of all manmade debris. In principle, the basin looks very vulnerable to possible accumulation of floating debris, since its dynamics is characterized by an inward surface flow of water from the Atlantic hampering surface floating items from being flushed out. Yet, no evidence of permanent litter accumulation areas has been reported so far in the Mediterranean. In this paper we utilized the largest available set of historical Lagrangian data gathered in the Mediterranean Sea to estimate the probability of debris particles to reach different subareas of the basin, with the main objective of singling out possible retention areas. Climatological reconstructions of the time evolution of litter distribution in the basin carried out on the basis of observed Lagrangian displacements suggest a general tendency of floating matter to collect in the southern portion of the basin, and in particular a long term accumulation in the southern and southeastern Levantine basin, areas not yet sampled by marine litter observation campaigns, whose targeted organization we strongly recommend at the end of this paper.
Introduction
Marine litter in the Mediterranean Sea, which only recently became the object of extensive studies, is a very sensitive issue from several standpoints (ecological, social, and economic, see Galgani et al., 2014). Concern about marine litter has been rising in the last decades, triggered by the studies on the great mid-ocean garbage patches (Moore et al., 2001; Law et al., 2010; Lebreton et al., 2012; Maximenko et al., 2012; van Sebille et al., 2012; Eriksen et al., 2014; Cózar et al., 2015; van Sebille et al., 2015).
The current state of knowledge of this issue, with a particular focus on the Mediterranean Sea, was assessed in a dedicated workshop in June 2014 organized by the Mediterranean Science Commission (or CIESM, i.e., Commission Internationale pour l'Exploration Scientifique de la Méditerranée), resuming previous knowledge and encouraging new studies. The aspects treated in the workshop were sources, composition and abundance of litter and its degradation and transformation, particle transport by marine circulation, impacts on marine life and legal instruments (CIESM, 2014).
The Mediterranean region is one of the world areas characterized by the highest amount of man-generated solid waste (Galgani et al., 2014). In particular, the Mediterranean Sea is vulnerable to plastics (van Sebille et al., 2015): while they typically do not constitute a high percentage of discarded waste, they are the most important part of marine litter, constituting over 80% of floating items (Galgani, 2014; Suaria and Aliani, 2014). This particular pollutant is ubiquitous—due to its extensive worldwide use—and extremely persistent, degrading slowly into tiny pieces (Barnes et al., 2009). It can travel very far from its sources, because time scales of degradation and transformation of plastics are much longer than the scales of transport (Barnes et al., 2009; Andrady, 2011). It harms marine species and ecosystems: threating biodiversity, favoring the spreading of invasive alien species and entering the food chain—through which it is transferred to larger predators including humans (Deudero and Alomar, 2015). Attempts to quantify plastic debris in the Mediterranean date back to 1980, when a quantitative visual survey reported around 1300 plastic items per square kilometer in a central region of the basin (Morris, 1980).
The Mediterranean Sea (Figure 1) is not only surrounded by a massively waste-generating coastal zone and inland, subject to a heavy anthropic, urban, and industrial pressure; the structure of its circulation, as well as its being embedded in the global ocean conveyor belt, may promote retention of floating material inside the basin. The Mediterranean circulation (Malanotte-Rizzoli et al, 1999; Robinson et al., 2001) is characterized by an inward surface flow of waters from the Atlantic Ocean, with no significant outward flow anywhere along its coastline; the return flow into the Atlantic happens at the subsurface, thus hampering surface floating items from being expelled from the basin and destinating them to accumulating within it. At the global ocean level, the Mediterranean's possible sink role for floating particles of global origin was shown by Lebreton et al. (2012), who used a global numerical model simulating 30 years of input, transport, and accumulation of debris in the world ocean; their estimate is that the Mediterranean potentially retained between 6 and 8% of all particles introduced into the model, thus achieving one of the highest concentrations of marine litter in the world ocean. Yet, no evidence of permanent litter accumulation areas (“garbage patches”) has been reported so far in the Mediterranean, as discussed by Cózar et al. (2015; see Section Conclusions on this point).
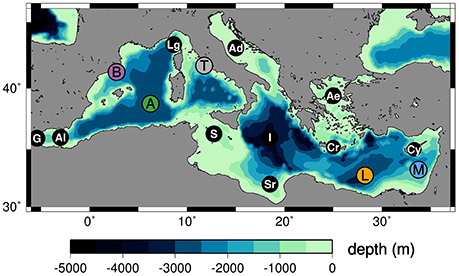
Figure 1. Bathymetric map of the Mediterranean. Black circles correspond to key places (subbasins, straits, islands) explicitely mentioned in the paper (Ad, Adriatic Sea; Ae, Aegean Sea; Al, Alboran Sea; I, Ionian Sea; Lg, Ligurian Sea; Sr, Gulf of Sirte; G, Strait of Gibraltar; S, Sicily Strait; Cr, Crete Island; Cy, Cyprus Island). Colored circles indicate key places mentioned in the paper and specific locations sampled to build time evolution of local tracer concentration, as shown in Figure 12 (A, Algerian Basin; B, Balearic Sea; L, Levantine Basin; M, Middle Eastern coast; T, Tyrrhenian Sea). Bathymetry is based on the ETOPO5 5-min gridded elevation data (Edwards, 1989) provided in the Generic Mapping Tools package (Wessel et al., 2013). For a map of the main features of the Mediterranean surface circulation we refer readers to Figure 12 of the paper by Poulain et al. (2012).
Zambianchi et al. (2014) provided a review of previous studies on transport and dispersion of debris due to marine circulation, the main methods used as well as findings at large and regional scales and in the Mediterranean Sea. Transport of particles in the ocean is generally investigated making use of Lagrangian particle models that describe the flow as formed by two components: a large scale adjective mean field and a smaller scale irregular field. Numerical models or velocity data from drifters draw the former component with high confidence, while the latter is much more complicated to investigate and quantify, and thus typically parameterized in various ways (e.g., Zambianchi and Griffa, 1994; Haza et al., 2012). Previous works employed numerical models to simulate both ocean circulation and the spread of particles (Lebreton et al., 2012; Mansui et al., 2015), or statistical methods that use observed surface drifter trajectories as input to spread particles forward in time (Maximenko et al., 2012; van Sebille et al., 2012), which is the approach we also selected.
Regional studies on litter transport are very few compared to large scale ones; they are limited to the East Asian marginal seas and the ocean surrounding Hawaiian Islands (among the most recent, respectively, Carson et al., 2013; Kako et al., 2014). In the Mediterranean, several studies investigated the abundance of debris (see again CIESM, 2014, for comprehensive reviews), while studies on its transport are scarce. Aliani et al. (2003) and Ramirez-Llodra et al. (2013) gave hints about the possible transport mechanisms that could have led to the observed debris density, i.e., remote and local three dimensional oceanographic conditions. More recently, Mansui et al. (2015) used numerical models and simulations of particle spreading, identifying possible retention areas in the Mediterranean.
In the present paper, along the lines drawn by Maximenko et al. (2012) for the global ocean, we utilized the largest available set of historical Lagrangian data gathered in the Mediterranean Sea to estimate the probability of floating litter to reach different subareas of the basin, with the main objective of pointing out possible retention areas. As will be seen, our results suggest marine debris accumulation in the southern and southeastern Levantine basin. However, it should be noted that our study is fairly idealized, and that in reality the plastic distribution might be modulated by the basin inter- and intraseasonal variability which we do not resolve.
The method is illustrated in Section Lagrangian Data Analysis, while results are presented in Section Results and Discussion, along with their comparison with direct litter observations in the basin. Conclusions and recommendations for future investigations follow in Section Conclusions.
Lagrangian Data Analysis
Preliminary Data Treatment
The data utilized in this work come from the Mediterranean Drifter Data Base, which gathers virtually all near-surface drifters deployed in the Mediterranean in the last 30 years in the framework of the most diverse experiments (e.g., Poulain et al., 2012). We used drifter data spanning from 1987 to 2014 for a total of 1429 drifters. Drifters are of three types, listed here from the more to the less abundant: Surface Velocity Program (SVP), Coastal Ocean Dynamics Experiment (CODE), and Compact Meteorological and Oceanographic Drifters (CMOD).
SVP drifters are equipped with a holey-sock drogue centered at a 15 m depth (Lumpkin and Pazos, 2007). CODE and CODE-modified drifters are 70 cm to 1 m long plastic or aluminum cylinders with four perpendicular sails, whose vertical alignment is provided by four small floaters (Davis, 1985; for their water following characteristics see, e.g., Pisano et al., 2016 and references therein). CMOD drifters are 60 cm long aluminum tubes with a 35 cm-diameter buoyancy collar, drogued with a tether of variable length (4–100 m, the latter case being much more frequent, Selsor, 1993).
These three types of drifters have different water-following capabilities, the CMOD being more affected by windage as well as SVP drifters that happen to lose their drogue (Pazan, 1996). Such a variety of drifter designs could facilitate, in principle, the simulation of different kinds of debris dragged by winds and currents in various ways. However, the relative paucity of the available data did not allow us to distinguish among different kinds of behavior in a statistically reliable way, and for this reason we used all data together, as a bulk of undifferentiated items.
The Mediterranean drifter data are processed and archived by the Istituto Nazionale di Oceanografia e di Geofisica Sperimentale in Trieste, Italy, and are accessible through the MedSVP website (http://nettuno.ogs.trieste.it/sire/medsvp). They were filtered to remove high frequencies; positions and velocities are subsampled every 6 h (see Poulain et al., 2012, for further details about dataset and data treatment).
As to the temporal and spatial distributions of the data, drifter days were <500 before 1990, nearly 2000 from 1990 to 1995, and doubled on average thereafter (not considering the years 2000, 2001, and 2014 when they were again <500). Figure 2A shows the 6-hourly data density within the basin. The maximum concentration of drifters is in the Adriatic Sea and in the northern areas of the western basin. Other regions of high data density are the central Tyrrhenian Sea, the Sicily Strait, the Aegean Sea, and the sea area south of Cyprus. Figure 2B reports a histogram of the drifter lifetime in the Mediterranean; the average lifetime is 72 days. Figure 2C shows the time evolution of drifter lifetime averaged over five-year periods. Besides intrinsic failures, the main reasons why drifters “died” (Lumpkin et al., 2012) are stranding and being picked up by fishing boats; however, on the basis of the limited average lifetime shown by earlier drifters, after the turn of the millennium several investigators started to limit the programmed transmission time to around 90 days, in order to avoid wasteful expenditures in case of instruments being trapped on land.
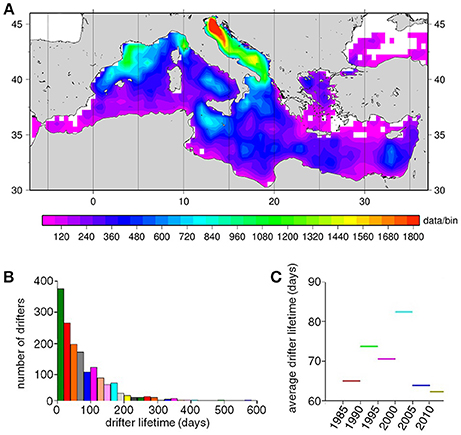
Figure 2. Six hourly data density for the whole drifter dataset (A); histogram of drifter lifetime (B); drifter lifetime averaged over 5-year periods (C).
Data are not sufficient to look at inter-annual variability, but they allow the study of seasonal variations. A first step to study seasonality has been to consider four seasons of the same duration: autumn (October–December), winter (January–March), spring (April–June), and summer (July–September). The total amount of data in each season is approximately the same; however, data density in the eastern basin is very low in summer, with <30 observations in ½° longitude by ½° latitude subregions for the most part of the area, while in the western basin it is lower during winter, especially in the Alboran, Algerian, and Tyrrhenian Sea. As a consequence, a winter and a summer season of 6 months each were considered a better choice to ensure statistical significance of the results. In previous studies of the Mediterranean Sea circulation using drifter data two extended seasons were also considered, choosing two opposite periods so as to maximize the differences between the resulting fields (Poulain and Zambianchi, 2007; Rinaldi et al., 2010; Poulain et al., 2012). In particular, the choice of the starting and ending times of the seasons was here optimized considering two different configurations: one with an extended summer starting in May and ending in October and another one starting in June and ending in November. In both cases differences between extended winter and summer spatial distributions of drifter density for each configuration are larger than between the two configurations of the same season (Figures 3A–D). This implies that the choice of the starting and ending time of the two seasons will likely not have a large impact on subsequent analysis in terms of statistical significance. The mean velocity field and the eddy kinetic energy (EKE) pattern obtained using the two configurations are also almost identical; however, the total amount of data per season is more evenly distributed using the second configuration and therefore only those obtained using the second configuration (i.e., with an extended summer between June and November and an extended winter between December and May) are presented in this paper.
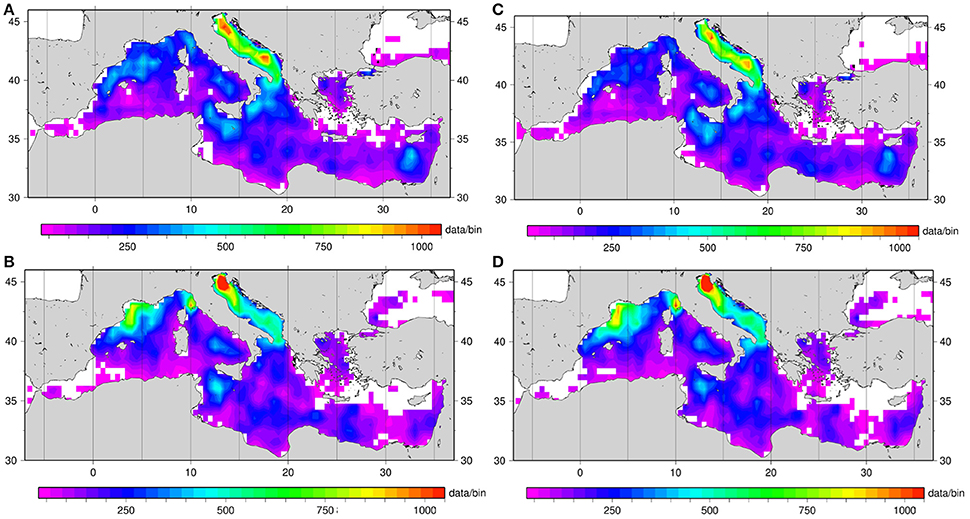
Figure 3. Data density for extended winter (A) and summer (B) defined from October to March and from April to September; for extended winter (C) and summer (D) defined from November to April and from May to October.
The description of the near-surface circulation in the Mediterranean as derived from the drifter data was carried out using the so-called pseudoeulerian description (e.g., Falco and Zambianchi, 2011): the basin was sudivided into ½° longitude by ½° latitude subregions (bins) and the flow field was described in terms of velocity averages and residuals computed on all drifter passes within each individual bin (on more sophisticated ways to build such statistics see Lumpkin, 2003; Lumpkin and Garraffo, 2005; Peng et al., 2015; Lumpkin et al., 2017, and references therein). The bin size was chosen so as to be able to effectively separate the mean circulation from its variable portion while retaining a sufficient number of data points in each bin, thus ensuring a statistical significance of the results.
In particular, for each bin we estimated the mean flow, defined as the average velocity vector, the kinetic energy of the mean flow per unit mass (mean kinetic energy, or MKE), the mean kinetic energy of the velocity residuals per unit mass (eddy kinetic energy, or EKE). For a formal definition of these statistical quantities see Trani et al. (2014). They are displayed in Figure 4 for the whole data set, and in Figures 5, 6 for the two extended seasons.
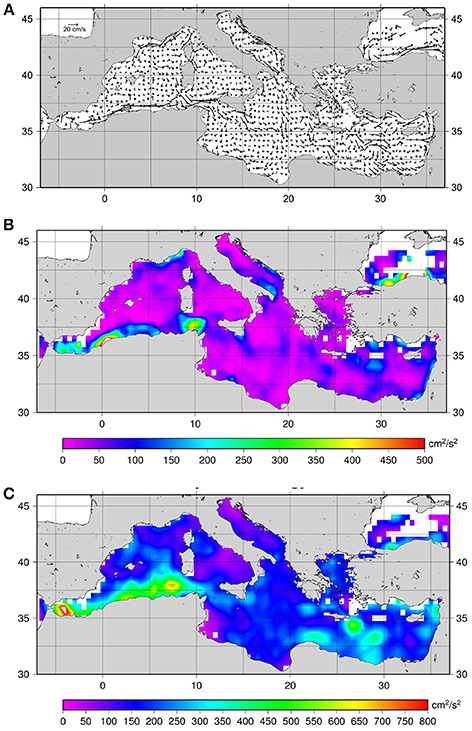
Figure 4. Pseudoeulerian climatological fields of mean velocity (A), MKE (B), and EKE (C) obtained from the drifter data set.
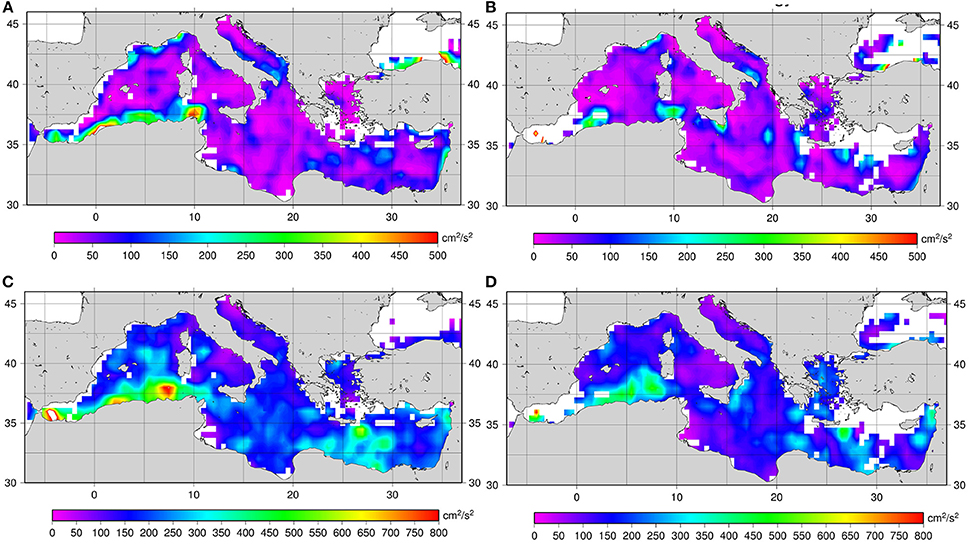
Figure 6. Pseudoeulerian kinetic energies: extended winter MKE (A) and EKE (B); extended summer MKE (C) and EKE (D).
Methodology Used to Study Transport
In this study, we implemented a method proposed by Maximenko et al. (2012) to investigate litter transport on the basis of the Mediterranean drifter dataset. Starting from a prescribed initial distribution, this method reconstructs the evolution of the density of a passive tracer representing an adimensional proxy for floating litter (indicated as FLP, i.e., floating litter proxy, from now on), using a probability density function obtained from the drifter trajectories.
The process consists of two phases: first, the existing drifter data are utilized to estimate the probability for them to move between two bins in a fixed time; then the probability is used to estimate the evolution of litter over the basin in the same fixed time.
In particular, the number of times that a drifter moves from one bin to another within a determined time span (hereafter defined “travel time”), once normalized over all switches, is assumed to represent the probability for a drifter to move between the two bins. This allows to build a probability density function of particle displacement over different travel times.
For simplicity, the calculation of the probability for a drifter to move between two points in a fixed time assumes drifter spreading as a statistically stationary process, so that probability depends only on travel time and not on the start time.
In practical terms, we gridded the domain spanned by the 1429 drifter trajectories into ½° latitude by ½° longitude bins. Then we used all pairs of 6-hourly locations along the same trajectory separated by the chosen travel time to compute the probability for a drifter to move between bin pairs. We tested five different travel times: 3 days, that is the typical Mediterranean Lagrangian time scale (Garcia-Olivares et al., 2007); 5 days, the time used by Maximenko et al. (2012); and 2, 4, and 6 days were also tested. Travel time has to be slightly higher than the Lagrangian time scale, but close to the time taken by a drifter to travel a distance close to the bin size (this is to limit cases of a “false” zero probability in bins that drifters cross without reaching them at exactly the travel time). The travel time values were tested counting the bins with a non-zero probability: results proved that 4 days was the best choice, with a number of non-zero bins very close to that obtained with 3 days where the maximum number is achieved.
It is worth underlining that, as explained by Maximenko et al. (2012, Appendix A), the qualitative outcome of our reconstruction in terms of FLP distribution in correspondence of its maxima is not crucially affected by the initial one, but is mainly due by the local flow regime at different scales.
The probability distribution obtained from the drifter trajectories was then used to initialize a procedure that iteratively computes a reconstructed evolution of FLP density in each bin over 4 days: it sums up in each arrival bin the densities of the starting bins at the previous time multiplied by the relative probabilities of reaching that bin in 4 days (see again Maximenko et al., 2012; Equation 2). Bins where at least one drifter ends and from which no drifters exit are considered as sinks. Thus we obtained an estimated FLP density in each bin every 4 days; these multiples of the 4-day travel times were chosen on basis of the characteristics of the Mediterranean Sea circulation and eddy structures (e.g., Rio et al., 2007; Poulain et al., 2013). Here we show the resulting distributions at different nominal target times, namely after a week, a month, 3 months, 1, 3, and 10 years (since target times have to be multiples of the 4-day travel time, the exact target times are 8, 28, 100, 364, 1096, 3648 days).
Two input scenarios have been considered, with two different initial distributions. Initial conditions were: a homogeneous distribution of FLP density = 1 per bin (the homogeneous initial distribution is indicated as HID from now on), and a distribution localized along the coasts based on the amount of coastal population (coastal initial distribution, or CID), the latter choice being motivated by the fact that main sources of litter in the basin are land-based (Galgani, 2014). Data of coastal population along the Mediterranean coasts have been extracted from the dataset provided by NCEAS Ocean Health Index project (Halpern et al., 2008). Raw data were provided in the Mollweide WGS84 symmetric projection therefore they were reprojected to longitude and latitude in the WGS84. Subsequently, all values in bins of size ½° latitude by ½° longitude were summed together and normalized to express each value in a bin as a fraction of the total.
The total (non-dimensional) density of our FLP in the HID case was 1174, corresponding to the number of bins populated by the drifters utilized to build the probability matrix; in the CID case it was 1556, i.e., as close as the original drifter number as allowed by the constraint of following the coastal population. These values are given here for the sake of completeness, but we want to reiterate that our FLP density is an adimensional quantity, thus they do not have to be misinterpreted as the number of litter items floating in the basin, which is orders of magnitude larger (see references in the Introduction).
The probability distributions and the resulting FLP displacement reconstructions were obtained using the whole drifter dataset—hereafter called climatological pattern—and the seasonal, i.e., the extended summer and winter, data.
Our ultimate goal in this paper is to identify possible areas of litter accumulation. Different definitions of such a process are available in the literature, typically related with tracer particle Lagrangian displacement described as a stochastic process, and spanning through quite different dispersion scenarios: e.g., particle trapping in porous media (e.g., Koch and Brady, 1985), in convective cells (e.g., Guyon et al., 1987), in anomalous diffusion situations (Young, 1988). In oceanographic flows, particle retention has been assessed with tools derived from dynamical systems theory (e.g., Castiglione et al., 1999; Cencini et al., 1999); the attractive properties of Lagrangian coherent structures may play an important trapping role (Peacock and Haller, 2013; for a Mediterranean application see Rossi et al., 2014). Froyland et al. (2014) quantitatively assessed the location and effectiveness of particle attraction areas in the world ocean on the basis of synthetic Lagrangian trajectories interpreted in a Markovian approximation. Here we will limit ourselves to the most straightforward accumulation definition, i.e., a medium- and/or long-term local increase of FLP density (see also below, Section Lagrangian Transport Reconstruction).
Results and Discussion
Traits of the Mediterranean Near-Surface Circulation Reconstructed by the Drifter Data
Figure 4A shows the pseudoeulerian mean velocity derived from the drifter data, averaged over the whole period (climatological). The binned current field displays the main, well-known currents, and semi-permanent eddy structures, consistently with the biased pseudoeulerian statistics by Poulain et al. (2012), who used a subset of the same drifter dataset, limited to 2010 data. Our analysis clearly shows the surface, eastward branch of the Mediterranean circulation (Malanotte-Rizzoli et al, 1999; Robinson et al., 2001), taking Modified Atlantic Water from the Strait of Gibraltar all the way to the Middle East Mediterranean coasts. The most evident feature is the swift current represented by the subsequence of the Algerian Current, of the Atlantic Ionian Stream, of the articulated and variable Mid-Ionian Jet and of the Mid-Mediterranean Jet, from which a number of side branches depart: the cyclonic circulations in the Algero-Provencal basin and in the Tyrrhenian Sea, which merge in the Northern Current, flowing in the Ligurian Sea and along the northern coasts of the Western Mediterranean; a series of alternating anticyclonic and cyclonic eddies from the south of the Peloponnese to the southeast of Crete and all the way to the middle eastern Mediterranean coasts; a system of strong coastal currents flowing cyclonically along the coasts of Egypt, Israel, Lebanon, Syria, and Turkey. The predominant mean flow is shown in the map of near-surface MKE drawn from the drifter data, where the inflow of Atlantic waters through the Alboran Sea and its first circulation branch along the Algerian coasts (Algerian Current) reaching the central Mediterranean is particularly evident, as its MKE-values are much higher than for the rest of the basin interior circulation (Figure 4B). The distribution of the EKE suggests that the Mediterranean circulation is strongly affected by variability, as EKE maxima faithfully retrace the main near-surface branch of the Mediterranean circulation, from the Strait of Gibraltar eastwards, clearly mirroring the Algerian Current, the Atlantic Ionian Stream, the Mid Ionian Jet, while in the Levantine basin the variability is strongly prevalent in correspondence of the major, persistent, well documented recirculations such as the Ierapetra, Rhodes, Mersa-Matruh gyres, and the Shikmona eddy (Figure 4C).
Winter and summer velocity fields and the relative MKE and EKE patterns showed differences in some areas that are worth considering (Figures 5, 6). During winter, the eddy structures are more energetic than in summer, the two branches of the mid-Ionian current are weaker and the Algerian current is stronger. Poulain et al. (2012) noticed a scarce seasonal variability among the whole basin, with the exception of the Sicily Strait and the outflow toward the Levantine basin; however, it must be considered that they only refer to the geostrophic portion of the near-surface circulation. Poulain and Zambianchi (2007) also found a strong seasonal variability of the circulation in the Sicily Strait, furthermore stressing the crucial role of local wind forcing. Since the purpose of this work is to study transport of objects at the surface, the role of the wind-driven part of the currents and of its variability is extremely important, thus seasonality is expected to play some role, at least in the transient behavior.
Lagrangian Transport Reconstruction
Seasonal Transport Patterns
Before using drifter trajectories to study transport and dispersion properties of the mean and eddy current fields briefly described in the former section, maps of the starting and ending points of trajectories were produced, and are shown in Figure 7; they clearly suggested that the effect of the near-surface velocity field was stronger than that of the drifter initial deployment distribution, and moreover gave indications of a noticeable seasonal behavior. The majority of drifters were deployed in the northern sub-basins and in the Sicily Strait. The tendency of trajectories is to end along the coasts, but there is also a higher collection in the Ionian Sea and Balearic Sea with respect to the number of releases, while very few drifters end at the Cretan Passage and in the Levantine basin center despite the relatively high number of releases.
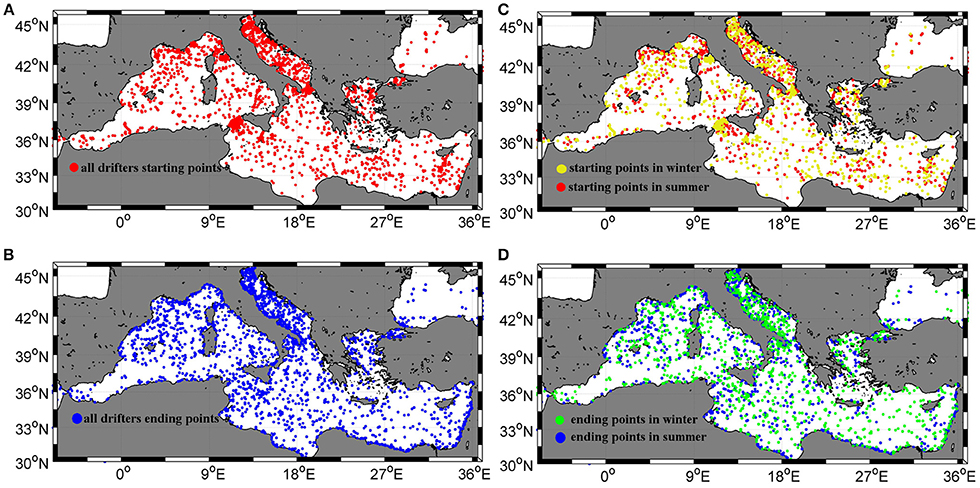
Figure 7. Starting (A) and ending (B) points of all drifters; starting points of winter trajectories (light brown dots) and of summer trajectories (red dots, C); ending points of winter trajectories (green dots) and summer trajectories (blue dots, D).
The comparison of the seasonal maps of starting and ending points confirmed the appropriateness of the choice of using two seasons of 6 months rather than four. Maps obtained for the four seasons showed two, rather than four, different distributions of ending points despite the release locations were similar in all seasons (data not shown). Ending points were quite homogeneously distributed in all the Mediterranean in autumn and winter, while they crowded the coasts in spring and summer. No significant differences were observed between autumn and winter nor between spring and summer distributions.
Even though the deployment point distribution was similar in both extended seasons, the pattern of ending points resulted quite different. As can be seen, in the eastern Mediterranean, and in particular in the Levantine basin, the distribution of ending points during summer was markedly different from winter ones. Namely, a higher tendency of drifters to group toward the coast in summer was evident. In the western basin, such difference was less clear, though some regions showed a similar behavior, namely the Ligurian and the Balearic Sea. On the contrary, in winter the distribution of ending points was sensibly more homogeneous throughout the entire Mediterranean. Such an important seasonal character was also displayed by the transport reconstruction results.
This is confirmed by a first glance at the evolution of reconstructed FLP density after the three target times of 1 week, 1 month, and 3 months, shown in Figures 8, 9. On both figures left panels refer to results obtained with the HID, right panels with the CID.
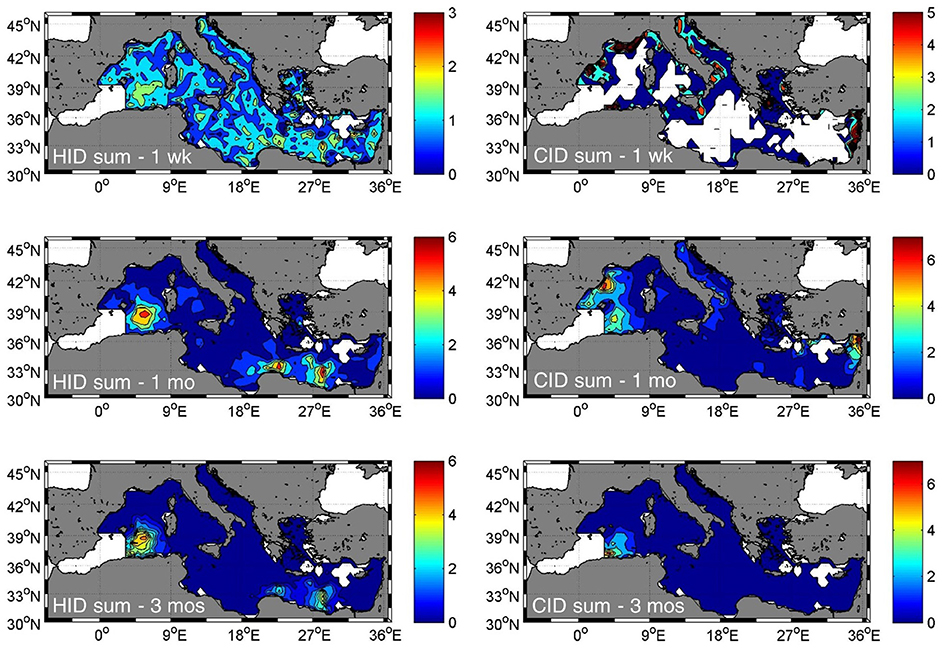
Figure 8. FLP density distribution for the homogeneous initial distribution (HID, left panels) and the coastal population initial distribution (CID, right panels) at different target times in the extended summer season.
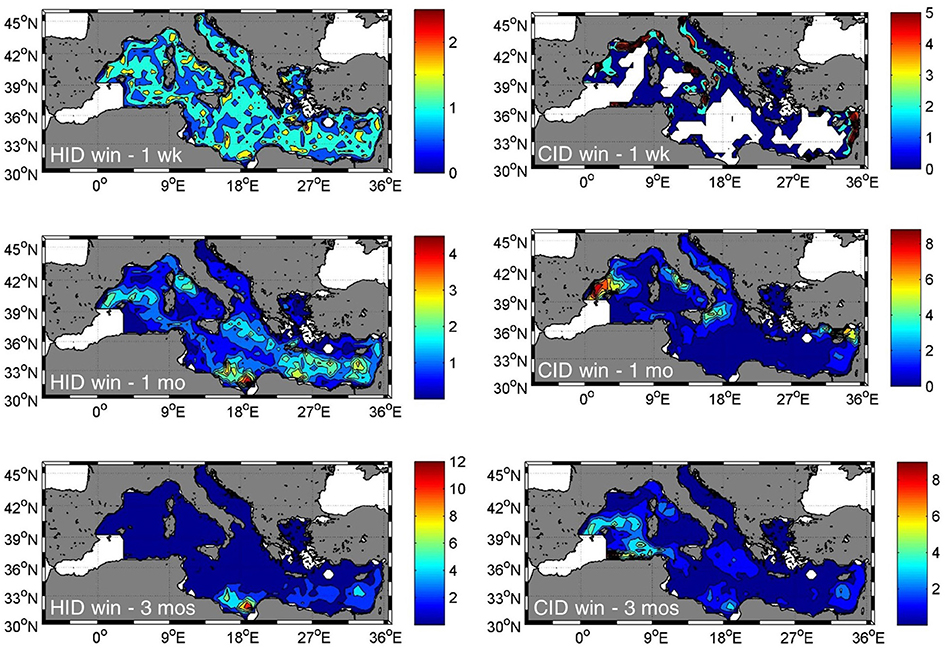
Figure 9. Same as in Figure 8 for the extended winter season.
The subsequent FLP distributions for those target times resulted quite different from one another, showing a strong transient character of accumulation zones in the initial period. A combination of some of these characteristics was also found in the climatological reconstructions, as will be discussed in the next section.
In the summer reconstructions, maxima of FLP density were located in the Algerian basin and off the Libyan and Egyptian coasts for the HID case, while in the CID case they were confined very close to the coast, with a maximum transiting cyclonically in the Western Mediterranean and another maximum briefly appearing in the northeastern corner of the Levantine basin. The winter situation for the HID was similar to the summer one, while the CID case was characterized by an accumulation in the Gulf of Sirte, which also appears in the HID case at 3 months, and persists for both initial conditions at least up to 1 year (not shown: for the seasonal cases obviously one-year long reconstructions are not meaningful).
In these short-term seasonal reconstructions the initial condition is clearly bound to play an important role; this is why both CID reconstructions return similar results. On the other hand, the HID accumulation in the Gulf of Sirte in winter and off the Algerian coasts in summer can be interpreted looking at the mean current and EKE fields. In winter, strong currents and high EKE along the Algerian coast can contrast accumulation; in summer, currents are weak even though the EKE maintains a certain intensity. In the Gulf of Sirte, the surface current enters from the north in the summer and proceeds westward along the Libyan coast, preventing retention of FLP there; in winter the current from the north is weak, an eastward current along the Libyan coast enters the gulf and, in addition, the EKE is very low, causing retention of FLP.
Asymptotic Accumulation Patterns
Figures 10, 11 show results obtained with the climatological pattern for the two initial conditions: Figure 10 displays the evolution from the HID and Figure 11 the evolution from the CID at target times of a week, a month, 3 months, 1, 3, and 10 years. Just like in the seasonal case, the color scale indicates the FLP density. The same color range is used in all snapshots, stretched so as to cover the different intervals of FLP densities spanned at different target times. The changing maxima provide an indication of the increasing FLP density in the accumulation regions, further illustrated in Figure 12.
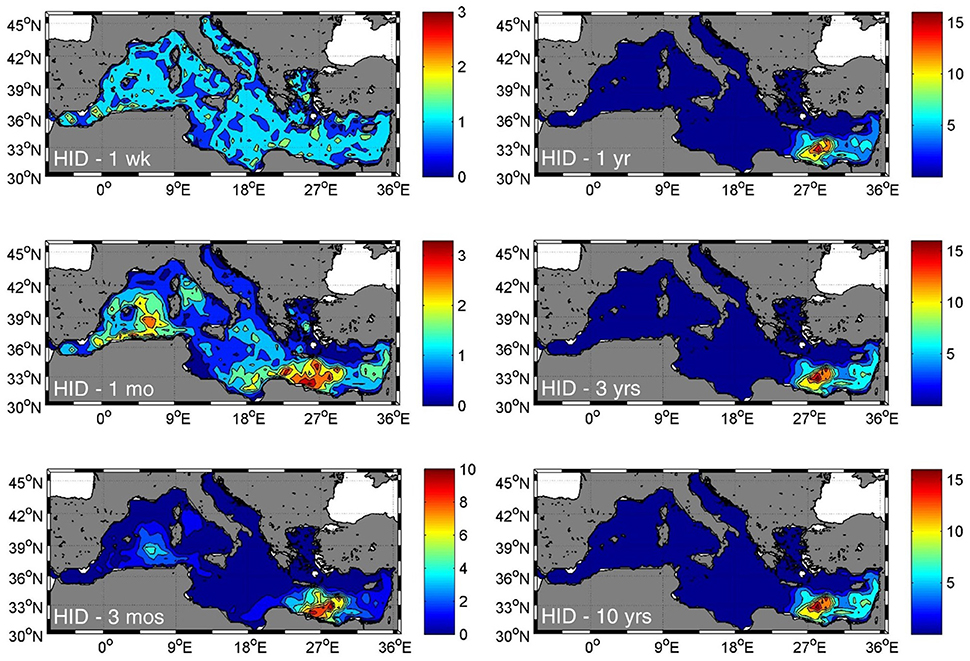
Figure 10. FLP density distribution for the climatological pattern with the homogeneous initial distribution (HID) at different target times.
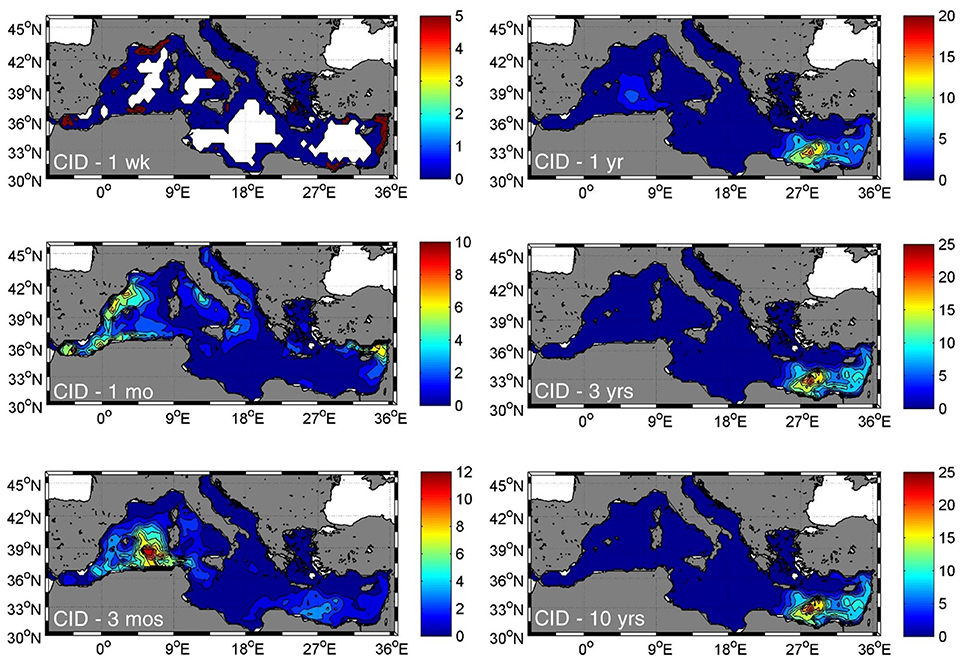
Figure 11. Same as in Figure 10 with the coastal population initial distribution (CID).
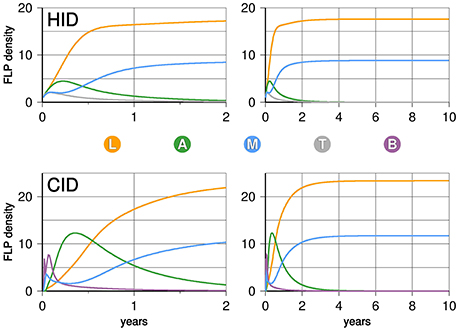
Figure 12. Time history of reconstructed FLP density in different key areas defined in Figure 1.
This allowed us to describe the transient initial displacement (up to 3 months) and the subsequent, asymptotic FLP behavior. The initial behavior is different in the two cases (as it was also in the seasonal cases, see above): while HID FLP by definition populated the interior of the Mediterranean right after deployment, the CID FLP distribution after 7 days still showed large gaps; after a month, the HID case showed a relatively widespread FLP aggregation pattern, with maxima in the Algerian basin, in the northern Tyrrhenian Sea and, more importantly, in the southern Ionian and Levantine basin. The two former relative maxima faded in the turn of another 2 months, showing a weak increase of the FLP density with respect to the background, while the maximum in the Levantine basin was at this point well defined, off the eastern Libyan and western Egyptian coasts. In the CID case, the FLP distribution after 1 month was still affected by specific source locations, combined with the current field: this was the case of the westernmost portion of the western Mediterranean subbasin and of the easternmost part of the Levantine basin; after 3 months a maximum in the Algerian basin was still present, while another, weak relative maximum off the Lybian/Egyptian coasts started to appear.
One year after deployments, both HID and CID FLP distributions showed a similar behavior, which proved to be an asymptotic tendency to accumulate in the southern and southeastern Levantine basin, with an absolute maximum off the eastern Libyan and western Egyptian coasts and a further relative maximum widespread off the entire Middle Eastern coast. This is further illustrated by Figure 12, which shows the time evolution of the local FLP density in different locations of the Mediterranean: colored circles indicate key places mentioned in the paper and specific locations sampled to build the time evolution of local tracer concentration, as shown in Figure 1 [A, Algerian Basin; B, Balearic Sea; L, Levantine Basin; M, Middle Eastern coast; T, Tyrrhenian Sea].
Upper panels refer to the HID initial conditions, lower panels to the CID one; left panels show the evolution over the first 2 years, right panels the evolution over 10 years, clearly displaying its asymptotic character. In particular, the southern/southeastern Levantine basin (L in the figure) shows a monotonic increase of FLP density which reaches its asymptotic values after five-six years.
Figure 12 displays different, typical FLP density trends revealed by our analysis: the southern and southeastern Levantine basin (L in the figure) is characterized by a monotonic increase followed by an asymptotic plateau reached after 5 to 6 years, with the absolute maximum FLP density in the whole Mediterranean; the FLP density off the Middle Eastern coast (M in the figure) steadily increases in the HID case, while in the CID case it reaches its absolute maximum after the very first few weeks, as may be expected since it is a coastal location, then decreases and later increases again, reaching after 6 years its asymptotic value, of the order of one half of the absolute maximum attained in L. The presence of a transient maximum at different initial times is also noticeable for the Algerian basin (A in the figure) where, however, the FLP density vanishes at asymptotic times. In addition to locations L, M and A, presented for both the HID and CID cases, Figure 12 shows the time evolution of the FLP density in the Tyrrhenian Sea for the former initial condition, and in the Balearic Sea for the latter (T and B in the figure). With the HID, the Tyrrhenian behavior is quite similar to the Algerian one, even though the FLP density range is more confined; with the CID, the FLP density in the Balearic attains two subsequent initial relative maxima, as a consequence of its coastal location in a reconstruction dominated at short time scales by coastal population effects, and then vanishes at asymptotic times.
The maxima of the color scale of Figures 10, 11 and the time history of FLP densities of Figure 12 show that the southern/southeastern Levantine basin is indeed a place of sustained enhanced local increase of FLP. Maxima, however, do not exceed a 25-fold increase of the initial value over 10 years. Such a figure is of the same order found by Maximenko et al. (2012) for the northern Atlantic and the southern Indian ocean, but far from the values attained in the Pacific. Whether these high FLP density areas may be considered as potential “garbage patches” depends on patch definition, really. Since the FLP initial density was set to 1, values attained by its distribution at different target times roughly correspond to the Tracer Amplification Factor (TAF) introduced by van Sebille et al. (2012); however, their threshold value TAF >2 to define patches would most likely not be appropriate for the Mediterranean, as at least in the short-term transient we see strong positive and negative variations of features with a FLP density larger than 5 or 10. This leads us to consider the latter, i.e., a 10-fold increase of FLP density for the long-term reconstruction, as a valid threshold value for the Mediterranean.
Comparison with In Situ Litter Observations
The comparison of our outcomes with the results of in situ litter observations is far from straightforward; this is mainly due to the sporadic character of observations, strongly constrained by research vessel time and routes, and inevitably not synoptic (see, e.g., the review of observations provided by Deudero and Alomar (2015), which displays the presence of large gaps in the coverage of the Mediterranean). A proper validation would call for systematic in space and repeated in time measurement campaigns that are currently not even planned (we will return to this point in the conclusions).
In particular, our results suggest the presence of asymptotic accumulation in the southeastern portion of the Levantine basin. Unfortunately, gathering detailed in situ data along the southern coasts of the Mediterranean is very difficult for a number of reasons, particularly in specific areas. This causes observations in those regions to be episodic and with coarse resolution; however, a strong presence of floating debris off the southern Mediterranean coasts is suggested by the most overarching recent observational work in the Mediterranean, by Cózar et al. (2015), which shows high concentrations south-east off Crete, in a position roughly corresponding to our HID and CID climatological long-term reconstruction maxima.
Eriksen et al. (2014) report results from 24 expeditions (2007–2013) across the world ocean, including coastal Australia, the Bay of Bengal and the Mediterranean Sea, and use those data to calibrate a Lagrangian debris dispersion model in the same areas. Their perspective is global and much coarser than ours, but a clear zonation of debris in the Mediterranean Sea is visible, and their results agree with the outcomes of our analysis, showing relative maxima in the southern portion of the basin, both in the western and in the eastern Mediterranean. Their long-term predictions nicely mirror, in an integrated manner, our asymptotic distributions, as well as our intermediate (1-month) ones.
Again at a coarser resolution, van Sebille et al. (2015) use three different, pre-existing global ocean circulation models to spatially interpolate a global dataset of plastic marine debris observations, after a careful preprocessing. Their outcomes, in particular the microplastic distributions based on the Lebreton et al. (2012) and the (van Sebille et al., 2012; van Sebille, 2014) models, appear clearly compatible with our results in terms of an asymptotic increased density of FLP in the southeastern Levantine basin.
Finally, but in terms of model-only results, it is worth underlining that the southern coastal strip of the eastern Mediterranean was identified by Mansui et al. (2015), if not as an accumulation area, as a preferential beaching destination.
As far as the other regions, characterized by transient accumulation according to our reconstruction, for the already mentioned logistic reasons we cannot find any observational confirmation of the high debris concentration expected in the Gulf of Sirte, clearly shown to be a likely retention area in the drifter data by Poulain and Zambianchi (2007), contained in the dataset utilized in this work, and representing one of three major retention areas resulting from the modeling study by Mansui et al. (2015).
Floating debris have been observed in the Algero Provencal basin by all recent samplings: see the above mentioned study by Cózar et al. (2015), in the Sardinia Channel and south of the Balearic Island, with further high concentration areas in the north, associated with the Northern Current or with the northward propagation of Algerian eddies (Millot, 1999; Salas et al., 2001, 2002; Salas, 2003; Isern-Fontanet et al., 2004; for a very recent census of eddies in the Mediterranean see Le Vu et al., 2016).
Other findings in the Sardinia Channel, along with observations in the southern portion of the Algerian basin, are reported by Suaria and Aliani (2014) and discussed by Zambianchi et al. (2014), and resemble very closely our results obtained for the case of litter sources located in correspondence of the most populated coastal areas, in particular the 1-month distribution for both extended seasons and for the climatological reconstructions.
A short-term FLP-populated area appears in our results in the northern Tyrrhenian Sea, but does not represent an accumulation area, as its signal is present only up to 1 month and disappears in the longer reconstructions. Observations in the Tyrrhenian rather suggest more abundance in the southern portion of the basin, characterized by very slow, basically stagnating dynamics (Rinaldi et al., 2010; for a discussion see Zambianchi et al., 2014; this is also in agreement with the modeling results by Mansui et al., 2015) and in the Corsica Channel (Suaria and Aliani, 2014; Cózar et al., 2015). The former is not to be seen in our reconstruction, while the latter is compatible with the 1-month results for the CID FLP distribution (Figure 10). It has to be underlined that the Corsica Channel represents the chokepoint for waters passing from the Tyrrhenian to the Ligurian Sea, thus possibly inducing an enhanced concentration of floating material transiting through, but not accumulating in, the strait.
Further indications can be provided by observations of the bottom distribution of debris. However, also in this case possible comparisons are constrained by the strongly uneven sampling, as shown by the maps displayed in the papers by Ramirez-Llodra et al. (2013, see their Figure 4), Pham et al. (2014, see their Figure 1), Tubau et al. (2015, see their Figure 12 and the studies summarized in it).
The results of the comparison are quite interesting: obviously, the observed distribution at the sea bottom is largely influenced by the vertical detail of the Mediterranean circulation, that in our study based on near-surface drifters cannot be accounted for, at least in terms of asymptotic zonation. However, on one hand observations confirm a relatively stronger long-term presence of litter in the southern Algerian basin and southeast off Crete. At the same time, our short-term distribution agrees, e.g., with observations in areas characterized by bottom litter abundance corresponding to the presence of canyons and other areas influenced by strong sinking patterns, such as the Gulf of Lyons. This is shown by Figure 10 for the CID case at 1 week and 1 month for the northern Ligurian Sea and Algero Provencal basin, in which we clearly observe the signature of the Northern Current which might be at least partly at the origin of the coincident bottom abundance. In other words, in such an area the asymptotic distribution is the result of horizontal advection and sinking, and the final destiny of debris is at the sea bottom.
Conclusions
The largest available near-surface drifter dataset for the area has been utilized to study transport of marine litter in the Mediterranean Sea. The drifter data have been first processed to provide Lagrangian displacement probabilities; from these probabilities we have then drawn estimates of the evolution throughout the basin of the density of a passive tracer, considered as a proxy of floating litter. Two kinds of sources have been considered, an initial homogeneous distribution and a more realistic one, simulating land-based coastal sources of pollution. The distribution of our floating litter proxy has been reconstructed for different travel times, in the climatological case and for two opposite extended seasons, a 6-month summer and a 6-month winter.
Decadal reconstructions of Lagrangian trajectories for the climatological case showed a quite clear asymptotic pattern of accumulation of our litter proxy (in terms of a local increase of density) in the southern and southeastern Levantine basin. This was true for both initial distributions (homogeneous over the basin vs. based on coastal population), the asymptotic behavior being achieved from 5 to 6 years on.
As is well known, no evidence of localized permanent debris accumulation (“garbage patch”) has been reported so far in the Mediterranean. However, as pointed out by Cózar et al. (2015) and van Sebille (2015), the term “garbage patch” itself might be misleading, as it suggests accumulation visible by the naked eye, which is not the case even in the most plastic-crowded regions of the global ocean. Moreover, no targeted litter observation campaigns have been yet carried out in the areas that our reconstructions identified as subject to asymptotic density enhancement of our litter proxy, that anyway found some agreement with the very few available data.
It is worth adding that our seasonal recontructions showed transient accumulation in locations varying over time and different from one season to another. This suggests that the surface flow temporal variability might play a role in shaping litter accumulation pattern; the structure of our experiment does not allow to assess such effects in the long term. The latter argument calls for some caution in considering the outcomes of an investigation like ours, carried out on a climatological, i.e., long-term averaged, data set. However, we want to underline that our shorter-term results, both seasonal and climatological, were found in good agreement with existing sightings of marine litter at the surface as well as at depth in selected locations.
Our results clearly indicate the need for a systematic floating litter observation activity, that may shed light on the likely accumulation in the southern and southeastern Levantine basin; the outcomes of our study lead us to strongly recommend the organization of regular, synoptic campaigns enabling the assessment of marine litter distribution over the whole Mediterranean Sea.
As a general remark, it has to be pointed out that the lack of solid observations, so far, of localized accumulation shall not anyway suggest a lack of an environmental problem, and should not decrease our concern: among the marginal basins of the world ocean the Mediterranean is one of the most affected by marine litter and plastics, and a strong and continuous effort is required to contain the deployment of garbage into its waters.
Author Contributions
EZ: Contribution to the main structure and contents of all sections, experiment design, result analysis, drafting, review, and final approval of the submitted version. MT: Contribution to the main structure and contents of all sections, experiment design and execution, result analysis, drafting, review, and final approval of the submitted version. PF: Contribution to the main structure and contents of all sections, result analysis, drafting, review, and final approval of the submitted version.
Conflict of Interest Statement
The authors declare that the research was conducted in the absence of any commercial or financial relationships that could be construed as a potential conflict of interest.
Acknowledgments
The authors are indebted to Erik van Sebille and Laurent Lebreton for very useful and constructive criticisms on this paper. We thank Marco Uttieri for useful comments on the manuscript. This work was partly funded by the Flagship Project RItMare (The Italian Research for the Sea), by the EU 7th Framework Programme project COCONET (Grant agreement No. 287844, http://www.coconet-fp7.eu/), by the Parthenope University Individual Research fund (DR 727/2015 and 954/2016) and by the Italian Ministry of the Environment in the framework of the implementation of the Marine Strategy Framework Directive.
References
Aliani, S., Griffa, A., and Molcard, A. (2003). Floating debris in the Ligurian Sea, northwestern Mediterranean. Mar. Pollut. Bull. 46, 1142–1149. doi: 10.1016/S0025-326X(03)00192-9
Andrady, A. L. (2011). Microplastic in the marine environment. Mar. Pollut. Bull. 62, 1596–1605. doi: 10.1016/j.marpolbul.2011.05.030
Barnes, D. K., Galgani, F., Thompson, R. C., and Barlaz, M. (2009). Accumulation and fragmentation of plastic debris in global environments. Philos. Trans. R. Soc. B 364, 1985–1998. doi: 10.1098/rstb.2008.0205
Carson, H. S., Lamson, M. R., Nakashima, D., Toloumu, D., Hafner, J., Maximenko, N., et al. (2013). Tracking the sources and sinks of local marine debris in Hawaii. Mar. Environ. Res. 84, 76–83. doi: 10.1016/j.marenvres.2012.12.002
Castiglione, P., Cencini, M., Vulpiani, A., and Zambianchi, E. (1999). Transport in finite size systems: an exit time approach. Chaos 9, 871–879. doi: 10.1063/1.166459
Cencini, M., Lacorata, G., Vulpiani, A., and Zambianchi, E. (1999). Mixing in a Meandering Jet: a markovian approximation. J. Phys. Oceanogr. 29, 2578–2594. doi: 10.1175/1520-0485(1999)029<2578:MIAMJA>2.0.CO;2
Cózar, A., Sanz-Martín, M., Martí, E., González-Gordillo, J., Ubeda, B., Gálvez, J., et al. (2015). Plastic accumulation in the Mediterranean Sea. PLoS ONE 10:e0121762. doi: 10.1371/journal.pone.0121762
Davis, R. E. (1985). Drifter observations of coastal currents during CODE: the method and descriptive view. J. Geophys. Res. 90, 4741–4755. doi: 10.1029/jc090ic03p04741
Deudero, S., and Alomar, C. (2015). Mediterranean marine biodiversity under threat: reviewing influence of marine litter on species. Mar. Pollut. Bull. 98, 58–68. doi: 10.1016/j.marpolbul.2015.07.012
Edwards, M. O. (1989). Global Gridded Elevation and Bathymetry on 5-minute Geographic Grid (ETOPO5). Boulder, CO: NOAA, National Geophysical Data Center.
Eriksen, M., Lebreton, L. C. M., Carson, H. S., Thiel, M., Moore, C. J., Borrero, J. C., et al. (2014). Plastic pollution in the world's oceans: more than 5 trillion plastic pieces weighing over 250,000 Tons Afloat at Sea. PLoS ONE 9:e111913. doi: 10.1371/journal.pone.0111913
Falco, P., and Zambianchi, E. (2011). Near-surface structure of the Antarctic Circumpolar Current derived from World Ocean Circulation Experiment drifter data. J. Geophys. Res. 116:C05003. doi: 10.1029/2010jc006349
Froyland, G., Stuart, R. M., and van Sebille, E. (2014). How well-connected is the surface of the global ocean? Chaos 24:033126. doi: 10.1063/1.4892530
Galgani, F. (2014). “Distribution, composition and abundance of marine litter in the Mediterranean and Black Seas,” in CIESM Workshop Monograph 46: Marine Litter in the Mediterranean and Black Seas, ed F. Briand (Monaco, CIESM Publisher), 23–30.
Galgani, F., Barnes, D. K. A., Deudero, S., Fossi, M. C., Ghiglione, J. F., Hema, T., et al. (2014). “Executive Summary,” in CIESM Workshop Monograph 46: Marine Litter in the Mediterranean and Black Seas, ed F. Briand (Monaco, CIESM Publisher), 7–20.
Garcia-Olivares, A., Isern-Fontanet, J., and García-Ladona, E. (2007). Dispersion of passive tracers and finite-scale Lyapunov exponents in the Western Mediterranean Sea. Deep Sea Res. I 54, 253–268. doi: 10.1016/j.dsr.2006.10.009
Guyon, E., Pomeau, Y., Hulin, J. P., and Baudet, C. (1987). Dispersion in the presence of recirculation zones. Nucl. Phys. B-Proc. 2, 271–280. doi: 10.1016/0920-5632(87)90023-5
Halpern, B. S., Walbridge, S., Selkoe, K. A., Kappel, C. V., Micheli, F., D'Agrosa, C., et al. (2008). A global map of human impact on marine ecosystems. Science 319, 948–952. doi: 10.1126/science.1149345
Haza, A. C., Özgökmen, T. M., Griffa, A., Garraffo, Z. D., and Piterbarg, L. (2012). Parameterization of particle transport at submesoscales in the Gulf Stream region using Lagrangian subgridscale models. Ocean Modell. 42, 31–49. doi: 10.1016/j.ocemod.2011.11.005
Isern-Fontanet, J., Font, J., García-Ladona, E., Emelianova, M., Millot, C., and Taupier-Letage, I. (2004). Spatial structure of anticyclonic eddies in the Algerian basin (Mediterranean Sea) analyzed using the Okubo–Weiss parameter. Deep Sea Res. II 51, 3009–3028. doi: 10.1016/j.dsr2.2004.09.013
Kako, S. I., Isobe, A., Kataoka, T., and Hinata, H. (2014). A decadal prediction of the quantity of plastic marine debris littered on beaches of the East Asian marginal seas. Mar. Pollut. Bull. 81, 174–184. doi: 10.1016/j.marpolbul.2014.01.057
Koch, D. L., and Brady, J. F. (1985). Dispersion in fixed beds. J. Fluid Mech. 154, 399–427. doi: 10.1017/S0022112085001598
Law, K. L., Morét-Ferguson, S., Maximenko, N. A., Proskurowski, G., Peacock, E. E., Hafner, J., et al. (2010). Plastic accumulation in the North Atlantic subtropical gyre. Science 329, 1185–1188. doi: 10.1126/science.1192321
Lebreton, L. C. M., Greer, S. D., and Borrero, J. C. (2012). Numerical modeling of floating debris in the world's oceans. Mar. Pollut. Bull. 64, 653–661. doi: 10.1016/j.marpolbul.2011.10.027
Le Vu, B., Stegner, A., and, T., Arsouze (2016). Atlas of eddies in Mediterranean sea from satellite imagery. Rapp. Comm. Int. Mer. Médit. 41:73.
Lumpkin, R. (2003). Decomposition of surface drifter observations in the Atlantic Ocean. Geophys. Res. Lett. 30:1753. doi: 10.1029/2003GL017519
Lumpkin, R., and Garraffo, Z. (2005). Evaluating the decomposition of tropical Atlantic drifter observations. J. Atmos. Oceanic Technol. 22, 1403–1415. doi: 10.1175/JTECH1793.1
Lumpkin, R., Maximenko, N., and Pazos, M. (2012). Evaluating where and why drifters die. J. Atmos. Oceanic Technol. 29, 300–308. doi: 10.1175/JTECH-D-11-00100.1
Lumpkin, R., and Pazos, M. (2007). “Measuring surface currents with Surface Velocity Program drifters: the instrument, its data, and some recent results,” in Lagrangian Analysis and Prediction of Coastal and Ocean Dynamics, eds A. Griffa, A. D. Kirwan A. J. Jr., Mariano, T. Özgökmen, and H. T. Rossby (Cambridge, UK: Cambridge University Press), 39–67.
Lumpkin, R., Özgökmen, T., and Centurioni, L. (2017). Advances in the applications of surface drifters. Ann. Rev. Mar. Sci. 9, 59–81. doi: 10.1146/annurev-marine-010816-060641
Malanotte-Rizzoli, P., Manca, B., Ribera d'Alcalà, M., Theocharis, A., Brenner, S., Budillon, G., et al. (1999). The eastern Mediterranean in the 80s and in the 90s: the big transition in the intermediate and deep circulations. Dyn. Atmos. Oceans 29, 365–395. doi: 10.1016/s0377-0265(99)00011-1
Mansui, J., Molcard, A., and Ourmieres, Y. (2015). Modelling the transport and accumulation of floating marine debris in the Mediterranean basin. Mar. Pollut. Bull. 91, 249–257. doi: 10.1016/j.marpolbul.2014.11.037
Maximenko, N., Hafner, J., and Niiler, P. (2012). Pathways of marine debris derived from trajectories of Lagrangian drifters. Mar. Pollut. Bull. 65, 51–62. doi: 10.1016/j.marpolbul.2011.04.016
Moore, C. J., Moore, S. L., Leecaster, M. K., and Weisberg, S. B. (2001). A comparison of plastic and plankton in the North Pacific Central Gyre. Mar. Pollut. Bull. 42, 1297–1300. doi: 10.1016/S0025-326X(01)00114-X
Morris, R. J. (1980). Floating plastic debris in the Mediterranean. Mar. Pollut. Bull. 11:125. doi: 10.1016/0025-326x(80)90073-9
Pazan, S. E. (1996). “Intercomparison of drogued and undrogued drift buoys,” in OCEANS'96. MTS/IEEE. Prospects for the 21st Century, Vol. 2, eds C. P. Brancart and A. M. Clark (Fort Lauderdale, FL), 864–872. doi: 10.1109/OCEANS.1996.568343
Peacock, T., and Haller, G. (2013). Lagrangian coherent structures: the hidden skeleton of fluid flows. Physics Today 66:41. doi: 10.1063/PT.3.1886
Peng, S., Qian, Y. K., Lumpkin, R., Li, P., Wang, D., and Du, Y. (2015). Characteristics of the near-surface currents in the Indian Ocean as deduced from satellite-tracked surface drifters. Part II: Lagrangian Statistics. J. Phys. Oceanogr. 45, 459–477. doi: 10.1175/JPO-D-14-0049.1
Pham, C. K., Ramirez-Llodra, E., Alt, C. H. S., Amaro, T., Bergmann, M., Canals, M., et al. (2014). Marine litter distribution and density in European seas, from the shelves to deep basins. PLoS ONE 9:e95839. doi: 10.1371/journal.pone.0095839
Pisano, A., De Dominicis, M., Biamino, W., Bignami, F., Gherardi, S., Colao, et al. (2016). An oceanographic survey for oil spill monitoring and model forecasting validation using remote sensing and in situ data in the Mediterranean Sea. Deep-Sea Res. Part II. 133, 132–145. doi: 10.1016/j.dsr2.2016.02.013
Poulain, P. M., Bussani, A., Gerin, R., Jungwirth, R., Mauri, E., Menna, M., et al. (2013). Mediterranean surface currents measured with drifters. Oceanography 26, 38–47. doi: 10.5670/oceanog.2013.03
Poulain, P. M., Menna, M., and Mauri, E. (2012). Surface geostrophic circulation of the Mediterranean Sea derived from drifter and satellite altimeter data. J. Phys. Oceanogr. 42, 973–990. doi: 10.1175/JPO-D-11-0159.1
Poulain, P. M., and Zambianchi, E. (2007). Surface circulation in the central Mediterranean Sea as deduced from Lagrangian drifters in the 1990s. Cont. Shelf Res. 27, 981–1001. doi: 10.1016/j.csr.2007.01.005
Ramirez-Llodra, E., De Mol, B., Company, J. B., Coll, M., and Sarda, F. (2013). Effects of natural and anthropogenic processes in the distribution of marine litter in the deep Mediterranean Sea. Prog. Oceanogr. 118, 273–287. doi: 10.1016/j.pocean.2013.07.027
Rinaldi, E., Buongiorno Nardelli, B., Zambianchi, E., Santoleri, R., and Poulain, P.-M. (2010). Lagrangian and Eulerian observations of the surface circulation in the Tyrrhenian Sea. J. Geophys. Res. Oceans 115:C4024. doi: 10.1029/2009JC005535
Rio, M. H., Poulain, P. M., Pascual, A., Mauri, E., Larnicol, G., and Santoleri, R. (2007). A mean dynamic topography of the Mediterranean Sea computed from altimetric data, in-situ measurements and a general circulation model. J. Mar. Syst. 65, 484–508. doi: 10.1016/j.jmarsys.2005.02.006
Robinson, A. R., Leslie, W. G., Theocharis, A., and Lascaratos, A. (2001). “Mediterranean Sea circulation,” in Encyclopedia of Ocean Sciences, eds J. H. Steele, K. K. Turekian, and S. A. Thorpe (London: Academic Press), 1689–1706.
Rossi, V., Ser-Giacomi, E., López, C., and Hernández-García, E. (2014). Hydrodynamic provinces and oceanic connectivity from a transport network help designing marine reserves. Geophys. Res. Lett. 41, 2883–2891. doi: 10.1002/2014GL059540
Salas, J. (2003). Evolution of the open-sea eddy ALGERS '98 in the Algerian basin with Lagrangian trajectories and remote sensing observations. J. Mar. Syst. 43, 105–131. doi: 10.1016/j.jmarsys.2003.08.001
Salas, J., Garcıa-Ladona, E., and Font, J. (2001). Statistical analysis of the surface circulation in the Algerian Current using Lagrangian buoys. J. Mar. Syst. 29, 69–85. doi: 10.1016/S0924-7963(01)00010-0
Salas, J., Millot, C., Font, J., and Garcıa-Ladona, E. (2002). Analysis of mesoscale phenomena in the Algerian basin from drifting buoys and infrared images. Deep Sea Res. I 49, 245–266. doi: 10.1016/S0967-0637(01)00052-8
Suaria, G., and Aliani, S. (2014). Floating debris in the Mediterranean Sea. Mar. Pollut. Bull. 86, 494–504. doi: 10.1016/j.marpolbul.2014.06.025
Trani, M., Falco, P., Zambianchi, E., and Sallée, J. B. (2014). Aspects of the Antarctic circumpolar current dynamics investigated with drifter data. Progr. Oceanogr. 125, 1–15. doi: 10.1016/j.pocean.2014.05.001
Tubau, X., Canals, M., Lastras, G., Rayo, X., Rivera, J., and Amblas, D. (2015). Marine litter on the floor of deep submarine canyons of the Northwestern Mediterranean Sea: the role of hydrodynamic processes. Progr. Oceanogr. 134, 379–403. doi: 10.1016/j.pocean.2015.03.013
van Sebille, E. (2014). Adrift.org.au — a free, quick and easy tool to quantitatively study planktonic surface drift in the global ocean. J. Exp. Mar. Biol. Ecol. 461, 317–322. doi: 10.1016/j.jembe.2014.09.002
van Sebille, E. (2015). The oceans' accumulating plastic garbage. Phys. Today 68, 60–61. doi: 10.1063/PT.3.2697
van Sebille, E., England, M. H., and Froyland, G. (2012). Origin, dynamics and evolution of ocean garbage patches from observed surface drifters. Environ. Res. Lett. 7:044040. doi: 10.1088/1748-9326/7/4/044040
van Sebille, E., Wilcox, C., Lebreton, L., Maximenko, N., Hardesty, B. D., Van Franeker, J. A., et al. (2015). A global inventory of small floating plastic debris. Environ. Res. Lett. 10:124006. doi: 10.1088/1748-9326/10/12/124006
Wessel, P., Smith, W. H. F., Scharroo, R., Luis, J. F., and Wobbe, F. (2013). Generic mapping tools: improved version released. EOS Trans. AGU 94, 409–410. doi: 10.1002/2013EO450001
Young, W. R. (1988). Arrested shear dispersion and other models of anomalous diffusion. J. Fluid Mech. 193, 129–149. doi: 10.1017/S0022112088002083
Keywords: near-surface drifters, plastics, marine pollution, Mediterranean, circulation, marine litter, floating debris, garbage patch
Citation: Zambianchi E, Trani M and Falco P (2017) Lagrangian Transport of Marine Litter in the Mediterranean Sea. Front. Environ. Sci. 5:5. doi: 10.3389/fenvs.2017.00005
Received: 28 October 2016; Accepted: 16 January 2017;
Published: 01 February 2017.
Edited by:
Francois Galgani, French Research Institute for Exploitation of the Sea, FranceReviewed by:
Erik Van Sebille, Imperial College London, UKLaurent Lebreton, The Modelling House, New Zealand
Copyright © 2017 Zambianchi, Trani and Falco. This is an open-access article distributed under the terms of the Creative Commons Attribution License (CC BY). The use, distribution or reproduction in other forums is permitted, provided the original author(s) or licensor are credited and that the original publication in this journal is cited, in accordance with accepted academic practice. No use, distribution or reproduction is permitted which does not comply with these terms.
*Correspondence: Enrico Zambianchi, ZW5yaWNvLnphbWJpYW5jaGlAdW5pcGFydGhlbm9wZS5pdA==