- 1Department IFA-Tulln, Institute for Environmental Biotechnology, University of Natural Resources and Life Sciences, Vienna, Austria
- 2School of Architecture and Civil Engineering, Institute of Foundation Engineering, University of Wuppertal, Waste- and Water-Management, Wuppertal, Germany
- 3Government of Upper Austria, Directorate for Environment and Water Management, Division for Environmental Protection, Linz, Austria
- 4GUT Gruppe Umwelt + Technik GmbH, Linz, Austria
Opaque, viscous tars derived from the carbonization of fossile carbon feedstocks, such coal tars and creosote, are long-term sources of groundwater contamination, predominantly with poly- and heterocyclic aromatic hydrocarbons (PAH). The dissolution, aging and migratory behavior of dense, non-aqueous phase liquid (DNAPL) coal tar blobs and pools forming at the aquitard is not sufficiently understood to estimate the risk and adequately design groundwater treatment measures at a contaminated site. In this study, we investigate the composition and dissolution of a migrated, aged creosote DNAPL, and corresponding experimental and groundwater profiles using comprehensive two-dimensional gas chromatography (GCxGC-MS). GC-FID unresolved compounds were attributed to methylated homocyclic species using GCxGC-MS in the Methylanthracene weight range. Equilibrium concentrations were estimated using Raoult's law, assuming non-ideal behavior. Low molecular weight compounds were found to be prevalent even after decades of weathering, with Naphthalene (8% by mass) representing the most abundant identified compound, contrary to the expected preferential depletion of hydrophilic compounds. Morevoer, dimethylnaphthalenes were relatively more abundant in the aqueous boundary layer than in the DNAPL. DNAPL migration over 400 m with the groundwater flow effected lower viscosity and specific gravity of the migrated phase body in a superposition of weathering, transport, and aquifer chromatography effects. Based on a decomposition of analyzed and estimated constituents using the group contribution approach, reference DNAPL values for activity coefficients γi were used to model aqueous solubilities for selected compounds. Anthracene was close to its theoretical precipitation limit in the bulk DNAPL. While laboratory and modeled DNAPL dissolution behavior agree well, field data imply the presence of specific interfacial in situ processes significantly impacting dissolution processes. Based on aqueous GCxGC-MS profiles over the DNAPL, a hypothetical interfacial in situ film was calculated to be composed primarily of Phenanthrene, with minor contribution by Naphthalene, possibly forming a viscous barrier for the dissolution of lower molecular weight PAH. The main advances and gaps in electron donor DNAPL understanding are discussed regarding our conception of weathered, migrating hydrophobic DNAPL bodies in the aquifer of historic contaminated sites for the adequate treatment of contaminated water.
Introduction
Hydrophobic non-aqueous phase liquids (NAPLs), including light—typically petroleum products, LNAPLs; specific gravity below unity—and dense (DNAPLs) varieties are common forms of hydrocarbons released from industrial processes to the environment. Viscous, opaque, complex, and high molecular weight hydrocarbon mixtures, referred to as tars, were one of the primary waste streams of coal gas, carburetted water gas, and oil gas production until the onset of natural gas production (Harkins et al., 1988; Birak and Miller, 2009). The manufacturing process, especially temperature, determines tar composition to a greater extent than feedstock quality. Coal tar originates from coal gasification and is rich in aromatic compounds, with mono- and poly-cyclic aromatic hydrocarbons and their N, S, and O-substitutions as well as phenols as main compounds of environmental concern, along acids and bases (Rhodes, 1966; Brown et al., 2006), while the latter are mostly absent in carburetted water and oil gas (Harkins et al., 1988). Creosotes are distillation products of tars, lacking the higher molecular weight pitch fraction, and are chemically as heterogeneous as their feedstock. Presently, tar-derived DNAPLs are a source of groundwater contamination at many manufactured gas plant sites, coke plants, and wood impregnation facilities. They may be present as DNAPL blobs or collect as pools on the aquitard, where they may dissolve into and contaminate groundwater over centuries to millennia (Eberhardt and Grathwohl, 2002). Such sites are difficult to manage due to the poor source accessibility and the complexity of factors determining mass transfer controlling groundwater pollution and thus, the quality of associated waste water streams. These are controlling the requirements for water treatment facilities, be it in situ bioremediation or on site, such as in bioreactors or filters employing carbonaceous sorbents. In addition, DNAPLs have been observed to migrate with groundwater flow, but associated phenomena related to physical-chemical properties were not comprehensively analyzed.
Recently, we discussed the stratification of groundwater redox-chemistry, microbial population, electron donor transformation products, and terminal acceptors (TEA) above a coal tar-creosote DNAPL at a former railroad sleeper impregnation site (Scherr et al., 2016). There, we found that the rates of source dissolution into the groundwater vs. naturally occurring biotransformation of coal tar contaminants were balanced to the extent that vertical and horizontal plumes were only expressed on the scale of a few decimeters. However, the magnitude and kinetics of both source dissolution and counteracting transformation processes can commonly not be accurately assessed owing to their complex and varying spatio-temporal nature. However, a reliable estimation of both contaminant mass flux into the aquatic system and the mechanisms of concurrent depletion are essential for site risk assessment. On the sink side, the physical accessibility of solid-phase geogenic TEA, predominantly Fe(III), and Mn(IV) species, is dependent on their crystallinity, which is not commonly analyzed except for sites of research interest (Villatoro-Monzón et al., 2003; Lovley et al., 2004; Scherr et al., 2016). Reduced species may be recharged in the upper aquifer portions, as molecular oxygen infiltrated by rain (Foulquier et al., 2010) or on a discontinuous scale from upgradient regions, such as nitrate from agricultural use. Source dissolution processes are characterized to a better extent for electron acceptor DNAPLs, such as single-component halogenated hydrocarbons (Klenk and Grathwohl, 2002; Guilbeault et al., 2005; Johnston et al., 2014). Electon donor NAPLs, including light petroleum and dense coal tar phases, consist of hundreds or even thousands of different compounds of differing environmental properties, most importantly hydrophobicity, biodegradability, toxicity, and subsurface mobility (Brown et al., 2006; Vasilieva et al., 2012a; Erlacher et al., 2013). Commonly, only a limited range of compounds is considered in modeling approaches, most prominently a selection of 16 EPA-listed PAH, which are among the most abundant constituents (Brown et al., 2006; Vasilieva et al., 2012a,b) at tar oil impacted soils, aquifers and sediments. Moreover, coal tars undergo a variety of aging processes in the decades following their release into the subsurface that further complicate the prediction of their behavior (Liu and Haderlein, 2013). The effect of the genesis of phase-internal gradients within a multicomponent DNAPL due to leaching of well soluble compounds over time, and the development of high-viscosity skins (Alshafie and Ghoshal, 2004) or emulsions (Nelson et al., 1996) at the phase interface on coal tar dissolution have been noted and documented in laboratory studies (Nelson et al., 1996; Alshafie and Ghoshal, 2004) but to our knowledge, not under field conditions. In addition, contaminant transport may be increased by dissolved, possibly colloidal organic matter as carrier (Wehrer et al., 2013).
Following Raoult's law, the vapor pressure of a compound in mixture is calculated as the product of the pure compound's vapor pressure and its molar fraction in the mixture. Its modification for liquids (ci, sat = si·γi·fi, o) is commonly used to calculate the aqueous equilibrium saturation concentration, ci, sat, of individual constituents i in multi-component NAPL mixtures. As given above, the saturation concentration is a product of a compound i's pure aqueous solubility if the compound is present in liquid (si, w) state, or its subcooled solubility (si, sub) if in solid state at the environmental conditions of interest. Moreover, the activity coefficient γi and the compound's mole fraction in the organic phase, fi, o are included in the calculation. The latter may simplified to their mass fraction (Eberhardt and Grathwohl, 2002), which is often more readily available than a DNAPL's average molecular weight due to considerable uncertainties in the determination of the composition of the substance mixture.
Non-ideal behavior, with γi deviating from unity is suggested to be taken into account by some authors (Luthy, 1993; Peters et al., 1997; Liu et al., 2012). For the estimation of γi for constitutents i in complex mixtures, including light, and dense hydrophobic NAPLs, the semi-empirical UNIFAC (Universal Quasichemical Functional Group Activity Coefficient (Fredenslund et al., 1977) method is applied (Lee et al., 1992; Peters et al., 1999). Based on the decomposition of phase constituents into their functional groups, the degree of non-ideality resulting from a diversity of molecular interactions, driven by size, shape, and molecular structure, in the hydrophobic liquid can be inferred. This assessment of relative contributions of constituent functional groups theoretically requires comprehensive qualitative compositional information, which is rarely available for coal tar DNAPLs (Birak and Miller, 2009). The deviation from ideality (γi = 1) has been found to be sufficiently small (Mukherji et al., 1997; Eberhardt and Grathwohl, 2002) for ideality to be assumed in field estimations. In contrast, γQuinoline was 0.1 and γEthylbenzene up to 1.3 in several tar oils (Peters et al., 1999). Based on the UNIFAC approach, deviation from ideality obviously increases for compounds with increasingly dissimilar properties from mixture average, such as molecular weight or predominance of functional groups. These relations may increase in relevance in a long perspective, since DNAPL composition is expected to change with coal tar weathering, associated with a depletion of low molecular weight compounds which is adjoined by the relative enrichment of hydrophobic constituents within the residual DNAPL. This has been observed to lead up to the solidification of coal tar in model aquifers (Liu et al., 2009), while the dominance of Naphthalene in weathered aquifer samples remains unexplained to date (Birak and Miller, 2009).
Based on our previous site investigations on the attentuation and microbial transformation of coal-tar creosote impacted groundwater (Scherr et al., 2016), i.e., focusing on possible contaminant sinks or drains, this study comprises an in-depth investigation of complementary source dissolution processes, and a comparison with field measurements. A combination of conventional and comprehensive two dimensional gas chromagrography analysis methods of DNAPL and groundwater samples enables to fill compositional gaps and to infer the composition of a theoretical interfacial skin. We hypothesize that, in comparison to details in Raoult's law application, the more prominent factor of uncertainty regarding DNAPL dissolution processes lies in the physical architecture of an aged DNAPL, i.e., in the formation of interfacial films and diffusion processes within a bulk DNAPL filling porous media.
Materials and Methods
For details regarding the contaminated site, sampling and analysis methods we refer to our recent publication (Scherr et al., 2016). They are briefly summarized in the following.
Site Description
The contaminated site is located in Upper Austria in proximity of the river Danube. Between 1896 and 1972, a railroad sleeper impregnation plant, encompassing approximately 11 hectar, was in operation. Sleepers, poles, and other wooden materials were impregnated using distilled coal tar, zinc chloride, and other heavy metal salts in mobile (up to approximately 1900) and fixed tank and steam pressure impregnation (in the following) facilities. Documents from the early operation period indicate that spent creosote was disposed of in groundwater wells, i.e., without passage through the vadose zone. Cooling water was drained close to the impregnation facilities, possibly entailing increased contaminant mobility. Local surface contamination arose from losses during manipulation at the site of impregnation and creosote storage facilities and storage of recently treated material. Following site closure in the 1970s, the area is continuously used for industrial purposes. Contaminated soil was partly disposed of, and a large part of the surface is now sealed and poorly accessible for investigations. At the time of risk recognition in the 1990s, the creosote had formed a DNAPL at the site and in the groundwater downstream region following the aquitard relief approximately 15 below surface, spreading over an area of approximately 30.000 m2, and with a thickness of up to 100 cm. Maximum length and width are about 450 and 40 m, totalling a volume of 2 million liters of creosote. The groundwater flow velocity is approximately 0.8–1 m/d, at a table inclination of 1.0–1.5 permille, and a permeability of the aquifer sandy gravel of 9 × 10−4−9 × 10−3 m/s. A detailed description is available elsewhere (UBA, 2013). Our recent publication provides information about aqueous contamination, iron mineral availability, bacterial, and archaeal microbiome, aquifer stratification and describes natural attenuation processes (Scherr et al., 2016).
Field Sampling
Groundwater samples were collected from deep, i.e., DNAPL-containing, and shallow wells above the DNAPL and in up- and downstream regions, and analyzed for their content of coal tar derived constituents. The DNAPL sample, approximately 2 L, was collected from a central downstream deep well using a disposable plastic pump that was carefully immersed approximately 20 cm below the DNAPL/water interface, and stored in a glass bottle at 4°C in the dark until further analytical and experimental treatment.
Laboratory Dissolution Experiments
Equilibration experiments using site DNAPL and groundwater samples to obtain ci, sat, lab (Table 1) were conducted in the laboratory at 20°C in the dark as described earlier (Scherr et al., 2016). Briefly, approximately each 10 g of DNAPL were weighed into triplicate 2 L Pyrex bottles which were carefully filled with 1 L of site groundwater. Following gentle mixing on an orbital shaker (10 rpm) for 24 h, avoiding DNAPL dispersion, the bottles were left to rest for 1 week. Aqueous samples from different levels were withdrawn using a volumetric pipette in regular intervals to determine the point of equilibrium.
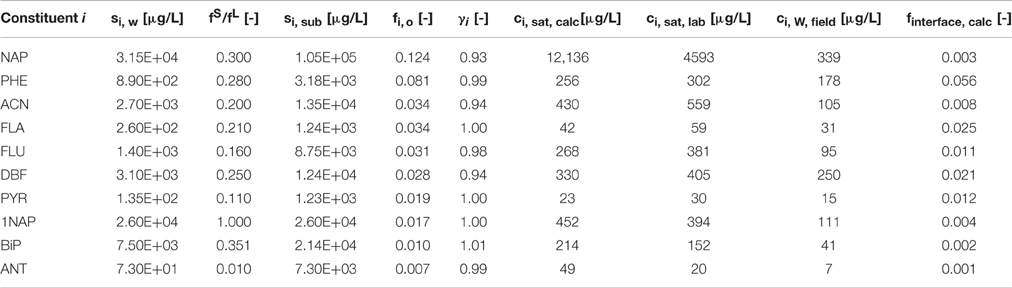
Table 1. Aqueous equilibrium concentrations of some abundant coal tar constitutents, based on Raoul'ts law using aqueous solubilities si, w (from Eberhardt and Grathwohl, 2002) and solid/liquid fugacity ratios at 25°C fS/fL (from Peters and Luthy, 1993) to calculate subcooled liquid solubilities (si, sub), activity coefficients γi (adopted from Peters et al., 1999), molar DNAPL fractions fi, o, Raoult's law (ci, sat, calc), measured ci, sat, lab aqueous equilibrium concentrations, groundwater data (ci, w) and theoretical molar fractions of a DNAPL/groundwater interface (finterface, calc).
Gas Chromatographic Analysis of Phase and Dissolved PAH
Extraction of aqueous and DNAPL samples was conducted following ultrasound-assisted (1 h) dissolution in heptane and dichloromethane (Scherr et al., 2016). Light and dense solvent and corresponding aqueous residues were separated in separation funnels, and gently evaporated if required. Analysis of extracted water, groundwater, and tar DNAPL samples using gas chromatography coupled to flame ionization detection (GC-FID) was perfomed in a modification of ISO DIN 16703 (Scherr K. et al., 2007). Unidimensional gas chromatographic (GC-MS) and comprehensive two-dimensional gas chromatography coupled to mass spectrometry (GCxGC-MS) analysis was conducted as described earlier (Hasinger et al., 2012; Vasilieva et al., 2012a,b). GCxGC-MS analysis is efficiently applied for the qualitative analysis of complex hydrocarbon mixtures (Rowland et al., 2012; Wilde et al., 2015). Compound identification must be carefully performed via library searches, requiring differentiation from those not included in the library, which is supported by the use of authentic standards similar to unidimensional GC. Equivalent carbon numbers on GC-FID were identified via comparison with authentic standards of normal alkanes purchased from Sigma. The presented data are averages of triplicate extractions. For quantified compounds, the following abbreviations are used: NAP Naphthalene, PHE Phenanthrene, FLA Fluoranthene, CAN Acenaphthene, FLU Fluorene, DBF Dibenzofuran, DBT Dibenzothiophene, CAR Carbazol, PYR Pyrene, 1NAP 1-Methylnaphthalene, 2NAP 2-Methylnaphthalene, BiP Biphenyl, ANT Anthracene, BaA Benzo(a)anthracene, CHR Chrysene, 26DN 2,6-Dimethylnaphthalene, BT Benzothiophene, IDA Indan, IDE Inden, 2ANT 2-Methylanthracene, ACY Acenaphtylene, BhQ Benzo(h)quinolin, BbF Benzo(b)fluoranthene, BaP Benzo(a)pyrene, 1PNA 1-Phenylnaphthalene, PHO Phenanthridinone, BkF Benzo(k)fluoranthene, ACR Acridine, and PHI Phenanthridine.
Results and Discussion
Effect of Migration on Physical Creosote Properties
The original DNAPL composition on the present site is not known, and has likely undergone substantial changes over extended time of plant operation and the following period of weathering in the aquifer. Moreover, technical advances in coal gasification led to different tar qualities used over time, which vary especially in the content of acids and heterocyclic compounds (Adam, 1932; Birak and Miller, 2009). The DNAPL sample of concern was sampled from a deep groundwater well approximately 250 m in groundwater flow direction from the downstream border of the original site. The DNAPLs's low specific gravity of 1.01, but also low dynamic viscosity (around 15 mPa·s) (UBA, 2013) are unusual even for lighter and distilled tars (Brown et al., 2006; Birak and Miller, 2009). Earlier investigations (UBA, 2013) have noted a decline of viscosity and specific gravity along the direction of migration along the aquitard relief, with 40 mPa·s and a specific gravity of 1.1 in the center of the site, suggesting an impact of transport in the composition of migratory DNAPL. Subsurface chromatography effects have been observed for high molecular weight hydrocarbons in crude oil reservoirs (Bastow et al., 2007), and a similar depletion may have occurred during DNAPL migration presently.
Chemical DNAPL Composition
Figures 1-3 show the results of qualitative and quantitative DNAPL hydrocarbon analysis via GC-FID, GCxGC-MS and a synopsis of GC-MS and GC-FID analysis, respectively. Despite the long exposure time—40–140 years, under consideration of site operation time—hydrophilic compounds such as Indene and Indane (aqueous solubility >100 mg/L) and NAP are abundant inside the DNAPL body, indicating little impact of weathering. Other studies have found groundwater well DNAPL samples to be less weathered than those from the vadose zone, also with NAP remaining the dominant compound (Brown et al., 2006; Birak and Miller, 2009). In contrast, DNAPL solidification, adjoined by depletion of low molecular weight compounds has been observed in simulated aquifers within only a few years (Liu et al., 2009). The investigated samples are from a depth of approximately 20 cm below the groundwater/DNAPL interface. Based on the identified compounds, 37% of DNAPL molecules contain at least one aromatic ring (Figure 3, corresponding to 40% by weight), and homocyclic molecules dominate over heterocyclic ones. The residual, poorly resolved mixture in the GC-FID chromatogram (Figure 1) is referred to in the following as uncharacterized hydrocarbons (UHC), and represents a substantial coal tar mass fraction (Figure 3), although of unknown composition. The coal tar visibly dissolved without residue in dichloromethane or n-heptane upon sonication, with a recovery between 94.7 and 95.3%, respectively, eluting between n-C10 and n-C40 on GC-FID. Solubilisation in saturated solvents reflects the low abundance of asphaltenes (Kharrat et al., 2007), and may be attributed to the migratory character of the sampled DNAPL and the distillation process. BTEX and phenols were not detectable (Scherr et al., 2016), and inorganic contaminants, typically Arsenic, Chromium, Lead, and Cyanides associated to coal tar, were not within the focus of the present study.
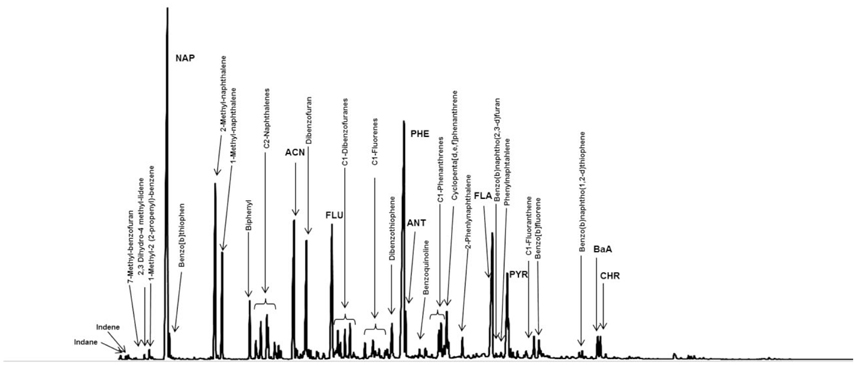
Figure 1. GC-FID Chromatogram of coal tar DNAPL sample from well foot. Some compounds are identified based on their retention time and mass spectra determined via analysis of the same sample using GC-MS. For abbreviations, see Materials and Methods section. Modified from Scherr et al. (2016), reused with permission from Elsevier.
Representation of Uncharacterized Hydrocarbon (UHC) Constitutents
Coal tars and creosotes consist of up to 10.000 of individual constituents with the molecular weight of pitch range constituents exceeding 10,000 Da (Millan et al., 2005; Herod et al., 2007), rendering a comprehensive compositional assessment difficult, especially for high molecular weight tars. This requires the use of indirect methods to assess DNAPL average molecular weight and functional group chemistry. The traditional method for the determination of the average molecular weight, vapor pressure osmometry, has been criticized for its dependence on sample properties (Brown et al., 2005), and was not available in the period of data compilation, prompting for an alternative method of assessment. Previously (Vasilieva et al., 2012a,b; Scherr et al., 2016), we used high-resolution two dimensional gas chromatography coupled to quadrupole (GCxGC-MS) and time-of-flight mass spectrometry (GCxGC-TOF-MS) for the identification of coal tar oil constituents. Figure 2 presents an annotated GCxGC-MS chromatogram of a DNAPL sample. Beside those identified based on comparison of mass spectra and retention behavior with authentic standards on GC-MS (see Figure 3), a variety of additional DNAPL constituents were identified based on their mass spectral library match; thus, most isomers are only tentatively assigned. The presently used GC-FID system is set up for the quantification up to 563 Da, or ECN-40 (n-tetracontane) and qualification compounds of a boiling point of up to 550°C (Scherr K. E. et al., 2007; Erlacher et al., 2013), encompassing typical creosote distillation ranges. Beyond 6-ring PAH, neither GC-FID nor GCxGC-MS analysis of fully dissolved coal tar gave profound signals. Up to 90% of coal tar mass is known to be aromatic (Morgan et al., 2008). Mid-molecular weight compounds, predominantly methylated homocyclic compounds were identified on GCxGC-MS (Figure 2), and are also visible as unresolved mixture in the corresponding GC-FID chromatogram (Figure 1), with an equivalent carbon number (ECN) below 30. The unresolved mixture's centroid is located around ECN-15, indicative of the average carbon number of compounds comprising the UHC. Together with the aromatic, alkylated nature of the uncalibrated compounds, methylanthracene (C15H15) was chosen as a model compound for the stoichiometric conversion of UHC range hydrocarbons (Figure 3). Trimethyl- and ethylmethylbenzenes add to to the light fraction, but were not quantified, as indicated in Figure 2. Consequently, the coal tar DNAPL has an average molecular weight (MW) of approximately 180 g/mole, representing an untypically light DNAPL (Brown et al., 2006), moreover in the light the weathering period, where light components are expected to be preferentially depleted. This may also be indicative of migratory effects, where higher molecular weight compounds may be separated in subsurface chromatographic effects during the movement through the aquifer. In addition, high molecular weight compounds such as asphaltenes, if present originally, may have been held in solution by saturated constituents, and were precipitated from the unstable DNAPL following alkane dissolution during weathering. Precipitation is ideally to be expected when the mole fraction of a compound exceeds its solid/liquid fugacity ratio (Peters et al., 1997), which is nearly the case for anthracene in the present DNAPL (Table 1).
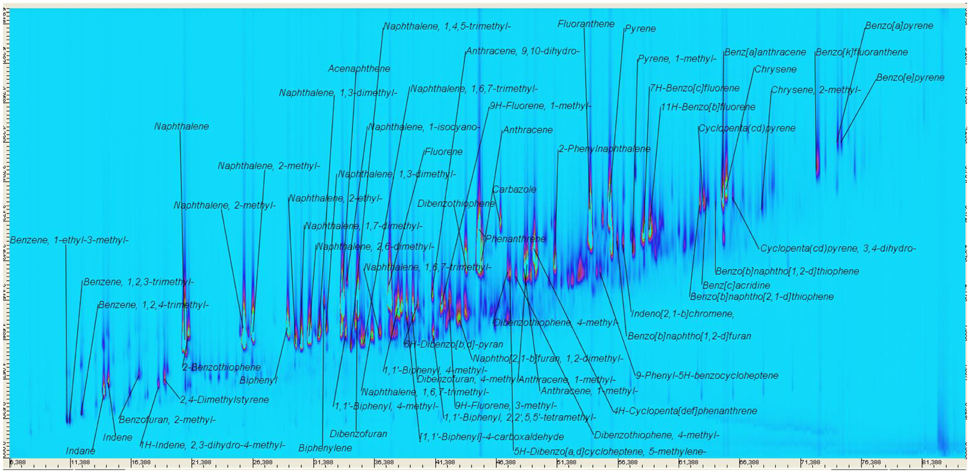
Figure 2. GCxGC-MS Chromatogram of the coal tar DNAPL. Some compounds are identified based on their spectral library match (match factor at least 850), some isomers were not unambiguously identified. Modified from Scherr et al. (2016), reused with permission from Elsevier.
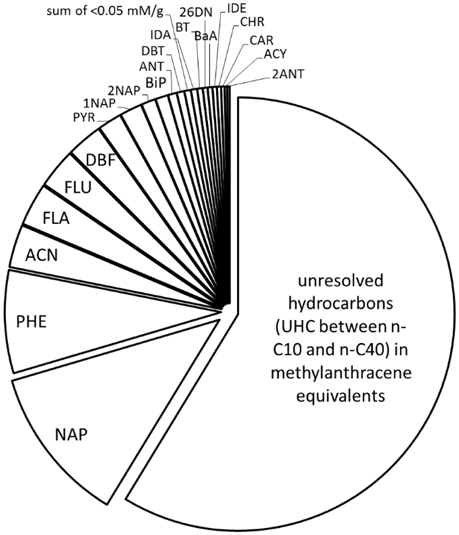
Figure 3. Stoichiometric DNAPL composition, based on quantitation via GC-MS and GC-FID. Molar fractions of 1PNA, BhQ, BbF, BaP, PHO, PHI, BkF, and ACR were below 0.05 mM/g and are displayed as their sum, and unresolved hydrocarbons eluting between n-C10 and n-C40 were converted to equivalents of methylanthracene.
DNAPL Functional Group Chemistry
Following Raoult's law, ideality in hydrophobic mixtures, with an activity coefficient γi = 1 can be assumed for all constitutents i provided their similarity in size, structure or shape and functional group chemistry (Peters et al., 1999; Eberhardt and Grathwohl, 2002; Klenk and Grathwohl, 2002). However, if molecular interaction or arrangement deviates substantially from that of a pure, unmixed liquid, excess enthalpy or entropy become nozero, respectively; if this is the case for hydrophobic mixtures, is not known a priori, owing to the complexity of possible coal tar dense or light NAPLs, and their degree of post-release alteration, i.e., weathering (Brown et al., 2006; Birak and Miller, 2009). Compositional changes during DNAPL weathering, with a continuous depletion of hydrophilic compounds, further contribute to the dynamic character of phase composition, relative abundance constituent properties, and thus, individual activity. Activity coefficients exceeding unity suggest self-association tendencies, and those below 1 to have molecular properties resulting in excess solvation tendencies. Structural decomposition using the group contribution method for UNIFAC (Universal Quasichemical Functional Group Activity Coefficient (Fredenslund et al., 1977) modeling indicate the most abundant structures, including methylanthracene as model UHC compound, in the present DNAPL to be ACH (aromatic carbon with one hydrogen atom attached, 69%), AC (aromatic carbon, 29%) and, to a lesser extent, ACCH3 (methylated aromatic carbon, 0.5%), similar to comparable light coal tars (Peters et al., 1999). Heteroaromatic group fractions (C4S, C5H3N, and C-O) each contribute two orders of magnitude less.
Estimation of Activity Coefficients γi Using the UNIFAC Model
Peters et al. (1999) provided estimations of γi based on the UNIFAC group contribution method for 43 tar oil components, which ranged between 0.14 and 1.13 for quinoline and ethylbenzene, respectively, in the two light DNAPLs of comparable molecular composition (average MW around 250 g/mol), suggesting excess solvation tendencies. Based on the similarity of MW, functional group distribution and molecular composition, we adopted the activity coefficients γi provided there. Values for γi averaged (n = 2), for the presently most abundant compounds, to 1.00, 0.99, and 0.98 for FLA, PHE, and FLU, respectively, and 0.93 and 0.94 for NAP and ACN, while the two heavier DNAPLs had slightly higher activity coefficients (e.g., γPHE = 1.02, γNAP = 0.99; Table 1). More condensed PAH such as BaA and heavier congeners, showed γi only slightly exceeding unity. Generally, alkylated PAH tend to have γi > 1, and N-heterocyclic and hydrophilic compounds γi < 1.
Calculated Equilibrium Concentrations over DNAPL
Table 1 shows calculated aqueous equilibrium concentrations (ci, sat, calc) for some abundant DNAPL constituents, incorporating subcooled liquid solubilities and activity coefficients in extension of data presented earlier (Scherr et al., 2016), and comparison with experimental (ci, sat, lab) and groundwater concentration data in proximity of the DNAPL surface (ci, w, field). Equilibrium concentrations are significantly higher than those presented earlier (Scherr et al., 2016) when using a simplified version of Raoult's law, owing predominantly to the consideration of fugacity ratios than to the consideration of activity coefficients, deviating largely unsubstantially from unity (Table 1). They are in good agreement with those obtained from our laboratory equilibrium experiments (ci, sat, lab), with the exception of Naphthalene, which is overpredicted by a factor of 2.6.
Field Groundwater Contaminant Profile
Taking qualitative groundwater concentration data into account (Table 1), Figure 4 presents a GCxGC-MS profile of a low-flow groundwater sample taken approximately 20 cm above the groundwater/DNAPL interface in a well, i.e., above the open DNAPL surface. The profile is substantially different from that of the DNAPL and presents a limited range of hydrophilic compounds, encompassing a variety of dimethylnaphthalenes, which are underrepresented in the DNAPL. Previous calculations (Scherr et al., 2016) indicated the thickness of the theoretical boundary layer in the aquifer above the interface, where equilibrium concentrations (i.e., ci, sat, Table 1) are expected to prevail, to be at least 80 cm for the case of NAP, and even greater over a free DNAPL surface inside the well. There, any depletive, anaerobic natural attenuation processes are expected to occurr at a lower rate than DNAPL dissolution (Eberhardt and Grathwohl, 2002; Lee, 2004; Scherr et al., 2016). Thus, the groundwater sample can be assumed to be in equilibrium with the DNAPL. However, while laboratory data, using a freshly sampled DNAPL, agree well with equilibrium assumptions, significantly lower concentrations were recovered throughout the field tests (additional groundwater data presented in Scherr et al., 2016), indicating interfacial alterations during weathering.
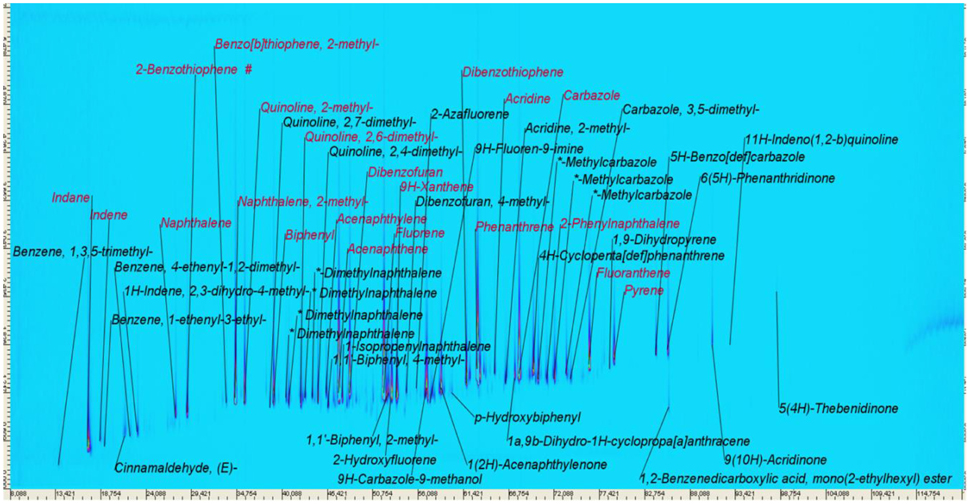
Figure 4. GCxGC-MS Chromatogram of a groundwater sample taken approximately 0.3m above the groundwater/DNAPL interface. Some compounds are identified based on their spectral library match (match factor at least 850), some isomers were not unambiguously identified.
Theoretical Interface Composition or Selectivity for DNAPL Dissolution
At the DNAPL-groundwater interface, a variety of effects are expected to occur. Alshafie and Ghoshal (2004) reported the formation of viscous, semi-rigid films, providing significant resistance to solute diffusion into the water phase, resulting in a reduced mass transfer from the DNAPL to the aqueous phase. There, depletion of hydrophobic compounds, bulk DNAPL diffusion effects and changed wetting properties were ruled out as driving factors. After 1 year of weathering, Nelson et al. (1996) found no enrichment, depletion or polymerization at the interface, but the formation of a semigelatinous film formed by weakly bonded water in an emulsified interface. However, the formation of a skin could not be verified due to the in situ character of the study. Based on field PAH concentrations, a hypothetical representation of the interfacial composition or selectivity of any film or skin for PAH solubilization from the bulk DNAPL, finterface, calc can be calculated based on Raoult's law (Table 1, Figure 5—compare to bulk DNAPL, Figure 3). Using this approach, NAP and ACN are overproportionally depleted, or selectively kept from release, possibly due to emulsification, with the mid-molecular weight PHE becoming the dominant compound.
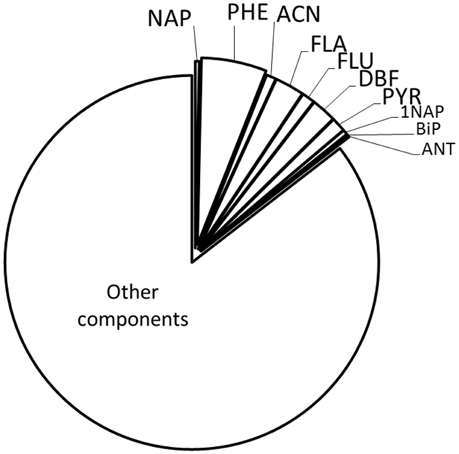
Figure 5. Molar composition (finterface, calc) or selectivity for PAH solubilisation of a theoretical film at the DNAPL/water interface, based on Table 1. “Other components” were not incorporated into the calculation.
Conclusion—Aging and Migration of Hydrocarbon DNAPLs
There is little information available on how tar DNAPLs in movement might change spatially across a given site in terms of composition and physico-chemical properties. Presently, some aspects determining contaminant release from aged, migrating coal tar or creosote DNAPLs were discussed and tentatively approached using simple models. Contrary to both models and laboratory observations, low molecular weight compounds remain prominent DNAPL constituents even after decades of weathering. Our calculations indicate that the composition of the water/DNAPL interface may be substantially different, i.e., composed of higher molecular weight components, from the bulk DNAPL composition. Possibly, a more viscous, higher molecular weight film may form a barrier on the interface under in situ conditions, protecting low molecular weight PAH from dissolution. An improved understanding of DNAPL processes can be obtained by the application of high resolution, two dimensional gas chromatographic methods. However, little remains known on the long term vertical diffusion and lateral (de)mixing processes within a moving bulk DNAPL undergoing weathering, or the chromatographic effect of DNAPL migration through an aquifer. The further elucidation of the effect of the water/DNAPL interface on contaminant release with a focus on in situ studies will help to increase the efficiency of modeling and risk assessment efforts on electron donor DNAPLs.
Author Contributions
KS designed the study, performed modeling, and wrote the manuscript. VV performed GCxGC-MS analysis, and analyzed the data. WL designed field experiments and discussed the data. MN designed the study, provided field data, and discussed the data.
Conflict of Interest Statement
The authors declare that the research was conducted in the absence of any commercial or financial relationships that could be construed as a potential conflict of interest.
Acknowledgments
This study was funded by The Austrian Federal Ministry of Agriculture, Forestry, Environment and Water Management under contract number B020003 and the Government of Upper Austria, contract number US 170-045. Laboratory assistance by M. Sumetzberger-Hasinger and G. Kadlec, and field assistance by M. Rothberger and I. Kaiser is thankfully acknowledged.
References
Adam, W. (1932). Coal tar Distillation and Its Products and Gas Liquor and Ammonium Sulphate. Vol. 12 of Modern Science Memoirs. London: John Murray.
Alshafie, M., and Ghoshal, S. (2004). The role of interfacial films in the mass transfer of naphthalene from creosotes to water. J. Contam. Hydrol. 74, 283–298. doi: 10.1016/j.jconhyd.2004.03.004
Bastow, T. P., Van Aarssen, B. G. K., and Lang, D. (2007). Rapid small-scale separation of saturate, aromatic and polar components in petroleum. Org. Geochem. 38, 1235–1250. doi: 10.1016/j.orggeochem.2007.03.004
Birak, P. S., and Miller, C. T. (2009). Dense nonaqueous phase liquids at former manufactured gas plants: challenges to modeling and remediation. J. Contam. Hydrol. 105, 81–98. doi: 10.1016/j.jconhyd.2008.12.001
Brown, D. G., Gupta, L., Kim, T. H., Keith Moo-Young, H., and Coleman, A. J. (2006). Comparative assessment of coal tars obtained from 10 former manufactured gas plant sites in the Eastern United States. Chemosphere 65, 1562–1569. doi: 10.1016/j.chemosphere.2006.03.068
Brown, D. G., Gupta, L., Moo-Young, H. K., and Coleman, A. J. (2005). Raoult's law-based method for determination of coal tar average molecular weight. Environ. Toxicol. Chem. 24, 1886–1892. doi: 10.1897/04-470R.1
Eberhardt, C., and Grathwohl, P. (2002). Time scales of organic contaminant dissolution from complex source zones: coal tar pools vs. blobs. J. Contam. Hydrol. 59, 45–66. doi: 10.1016/S0169-7722(02)00075-X
Erlacher, E., Loibner, A., Kendler, R., and Scherr, K. E. (2013). Distillation fraction-specific ecotoxicological evaluation of a paraffin-rich crude oil. Environ. Pollut. 174, 236–243. doi: 10.1016/j.envpol.2012.11.031
Foulquier, A., Malard, F., Mermillod-Blondin, F., Datry, T., Simon, L., Montuelle, B., et al. (2010). Vertical change in dissolved organic carbon and oxygen at the water table region of an aquifer recharged with stormwater: biological uptake or mixing? Biogeochemistry 99, 31–47. doi: 10.1007/s10533-009-9388-7
Fredenslund, A., Gmehling, J., and Rasmussen, P. (1977). Vapor-Liquid Equilibria Using UNIFAC. Amsterdam: Elsevier B.V.
Guilbeault, M. A., Parker, B. L., and Cherry, J. A. (2005). Mass flux distributions from DNAPL Zones in Sandy Aquifers. Groundwater 43, 70–86. doi: 10.1111/j.1745-6584.2005.tb02287.x
Harkins, S. M., Truesdale, R. S., Hill, R., Hoffman, P., and Winters, S. (1988). U.S. Production of Manufactured Gases: Assessment of Past Disposal Practices. Cincinnati, OH: Research Triangle Institute for Hazardous Waste Engineering Research Laboratory, U.S. EPA 600-2-88-012.
Hasinger, M., Scherr, K. E., Lundaa, T., Bräuer, L., Zach, C., and Loibner, A. P. (2012). Changes in iso- and n-alkane distribution during biodegradation of crude oil under nitrate and sulphate reducing conditions. J. Biotechnol. 157, 490–498. doi: 10.1016/j.jbiotec.2011.09.027
Herod, A. A., Bartle, K. D., and Kandiyoti, R. (2007). Characterization of heavy hydrocarbons by chromatographic and mass spectrometric methods: an overview. Energy Fuels 21, 2176–2203. doi: 10.1021/ef060642t
Johnston, C. D., Davis, G. B., Bastow, T. P., Woodbury, R. J., Rao, P. S. C., Annable, M. D., et al. (2014). Mass discharge assessment at a brominated DNAPL site: effects of known DNAPL source mass removal. J. Contam. Hydrol. 164, 100–113. doi: 10.1016/j.jconhyd.2014.05.016
Kharrat, A. M., Zacharia, J., Cherian, V. J., and Anyatonwu, A. (2007). Issues with comparing SARA methodologies. Energy Fuels 21, 3618–3621. doi: 10.1021/ef700393a
Klenk, I. D., and Grathwohl, P. (2002). Transverse vertical dispersion in groundwater and the capillary fringe. J. Contam. Hydrol. 58, 111–128. doi: 10.1016/S0169-7722(02)00011-6
Lee, K. Y. (2004). Modeling long-term transport of contaminants resulting from dissolution of a coal tar pool in saturated porous media. J. Environ. Eng. 130, 1507–1513. doi: 10.1061/(ASCE)0733-9372(2004)130:12(1507)
Lee, L. S., Hagwall, M., Delfino, J. J., and Rao, P. S. C. (1992). Partitioning of polycyclic aromatic hydrocarbons from diesel fuel into water. Environ. Sci. Technol. 26, 2104–2110. doi: 10.1021/es00035a005
Liu, L., Endo, S., Eberhardt, C., Grathwohl, P., and Schmidt, T. C. (2009). Partition behavior of polycyclic aromatic hydrocarbons between aged coal tar and water. Environ. Toxicol. Chem. 28, 1578–1584. doi: 10.1897/08-276.1
Liu, L., and Haderlein, S. (2013). A review on the aging phenomena of organic components and their mass transfer through the NAPL interfacial phase. Chin. J. Geochem. 32, 252–260. doi: 10.1007/s11631-013-0630-6
Liu, L., Maier, U., Grathwohl, P., and Haderlein, S. B. (2012). Contaminant mass transfer from NAPLs to water studied in a continuously stirred flow-through reactor. J. Environ. Eng. 138, 826–832. doi: 10.1061/(ASCE)EE.1943-7870.0000528
Lovley, D. R., Holmes, D. E., and Nevin, K. P. (2004). Dissimilatory Fe(III) and Mn(IV) reduction. Adv. Microb. Physiol. 49, 219–286. doi: 10.1016/S0065-2911(04)49005-5
Luthy, R. G. (1993). Interfacial films in coal tar nonaqueous-phase liquid-water systems. Environ. Sci. Technol. 27, 2914–2918. doi: 10.1021/es00049a035
Millan, M., Morgan, T. J., Behrouzi, M., Karaca, F., Galmes, C., Herod, A. A., et al. (2005). The high-mass component (>m/z 10, 000) of coal tar pitch by matrix-assisted laser desorption/ionisation mass spectrometry and size-exclusion chromatography. Rapid Commun. Mass Spectrom. 19, 1867–1873. doi: 10.1002/rcm.1997
Morgan, T. J., George, A., Davis, D. B., Herod, A. A., and Kandiyoti, R. (2008). Optimization of 1H and 13C NMR methods for structural characterization of acetone and pyridine soluble/insoluble fractions of a coal tar pitch. Energy Fuels 22, 1824–1835. doi: 10.1021/ef700715w
Mukherji, S., Peters, C. A., and Weber, W. J. Jr. (1997). Mass transfer of polynuclear aromatic hydrocarbons from complex DNAPL mixtures. Environ. Sci. Technol. 31, 416–423. doi: 10.1021/es960227n
Nelson, E. C., Ghoshal, S., Edwards, J. C., Marsh, G. X., and Luthy, R. G. (1996). Chemical characterization of coal tar-water interfacial films. Environ. Sci. Technol. 30, 1014–1022. doi: 10.1021/es950482s
Peters, C. A., and Luthy, R. G. (1993). Coal tar dissolution in water-misclble solvents: experimental evaluation. Environ. Sci. Technol. 27, 2831–2843. doi: 10.1021/es00049a025
Peters, C. A., Mukherji, S., Knightes, C. D., and Weber, W. J. Jr. (1997). Phase stability of multicomponent NAPLs containing PAHs. Environ. Sci. Technol. 31, 2540–2546. doi: 10.1021/es960948m
Peters, C. A., Mukherji, S., and Weber, W. J. Jr. (1999). UNIFAC modeling of multicomponent nonaqueous phase liquids containing polycyclic aromatic hydrocarbons. Environ. Toxicol. Chem. 18, 426–429. doi: 10.1002/etc.5620180309
Rhodes, E. (1966). “The History of Coal Tar and Light Oil,” in Bituminous Materials: Asphalts, Tars and Pitches, ed A. J. Hoiberg (New York, NY: John Wiley & Sons).
Rowland, S. J., West, C. E., Scarlett, A. G., Ho, C., and Jones, D. (2012). Differentiation of two industrial oil sands process-affected waters by two-dimensional gas chromatography/mass spectrometry of diamondoid acid profiles. Rapid Commun. Mass Spectrom. 26, 572–576. doi: 10.1002/rcm.6138
Scherr, K., Aichberger, H., Braun, R., and Loibner, A. P. (2007). Influence of soil fractions on microbial degradation behavior of mineral hydrocarbons. Eur. J. Soil Biol. 43, 341–350. doi: 10.1016/j.ejsobi.2007.03.009
Scherr, K. E., Backes, D., Scarlett, A. G., Lantschbauer, W., and Nahold, M. (2016). Biogeochemical gradients above a coal tar DNAPL. Sci. Total Environ. 563–564, 741–754. doi: 10.1016/j.scitotenv.2015.11.036
Scherr, K. E., Braun, R., and Loibner, A. P. (2007). “Long-chain normal alkane biodegradation and ageing in dissimilar soils,” in 9th International In situ and On-Site Bioremediation Symposium 2007, Baltimora, MY; Battelle Press, 356.
UBA (2013). Availble online at: http://www.umweltbundesamt.at/umweltschutz/altlasten/altlasteninfo/altlasten3/oberoesterreich1/o45/ (Accessed February 18, 2015).
Vasilieva, V., Janik, L., Scherr, K., Edelmann, E., and Loibner, A. P. (2012a). Data evaluation of tar oil degradation using comprehensive GC2/MS: individual compounds and principal component analysis. J. Chem. Technol. Biotechnol. 87, 1237–1245. doi: 10.1002/jctb.3838
Vasilieva, V., Scherr, K. E., Edelmann, E., Hasinger, M., and Loibner, A. P. (2012b). Comprehensive GC2/MS for the monitoring of aromatic tar oil constituents during biodegradation in a historically contaminated soil. J. Biotechnol. 157, 460–466. doi: 10.1016/j.jbiotec.2011.08.006
Villatoro-Monzón, W. R., Mesta-Howard, A. M., and Razo-Flores, E. (2003). Anaerobic biodegradation of BTEX using Mn(IV) and Fe(III) as alternative electron acceptors. Water Sci. Technol. 48, 125–131.
Wehrer, M., Rennert, T., and Totsche, K. U. (2013). Kinetic control of contaminant release from NAPLs - Experimental evidence. Environ. Pollut. 179, 315–325. doi: 10.1016/j.envpol.2013.03.041
Wilde, M. J., West, C. E., Scarlett, A. G., Jones, D., Frank, R. A., Hewitt, L. M., et al. (2015). Bicyclic naphthenic acids in oil sands process water: identification by comprehensive multidimensional gas chromatography-mass spectrometry. J. Chromatogr. A, 1378, 74–87. doi: 10.1016/j.chroma.2014.12.008
Keywords: coal tar, contaminated groundwater, groundwater remediation, polycyclic aromatic hydrocarbons (PAH), dense non-aqueous phase liquid (DNAPL), hydrocarbon weathering
Citation: Scherr KE, Vasilieva V, Lantschbauer W and Nahold M (2016) Composition and Dissolution of a Migratory, Weathered Coal Tar Creosote DNAPL. Front. Environ. Sci. 4:61. doi: 10.3389/fenvs.2016.00061
Received: 15 July 2016; Accepted: 02 September 2016;
Published: 16 September 2016.
Edited by:
Ioannis V. Yentekakis, Technical University of Crete, GreeceReviewed by:
Georgios Kyriakou, Aston University, UKMaria Goula, Technological Educational Institute of Western Macedonia, Greece
Copyright © 2016 Scherr, Vasilieva, Lantschbauer and Nahold. This is an open-access article distributed under the terms of the Creative Commons Attribution License (CC BY). The use, distribution or reproduction in other forums is permitted, provided the original author(s) or licensor are credited and that the original publication in this journal is cited, in accordance with accepted academic practice. No use, distribution or reproduction is permitted which does not comply with these terms.
*Correspondence: Kerstin E. Scherr, kerstin.brandstaetter-scherr@boku.ac.at