- Department of Integrative Biology and Biodiversity Research, Institute of Zoology, University of Natural Resources and Life Sciences, Vienna, Austria
Glyphosate-based herbicide formulations are broadly used in agriculture, silviculture, horticulture as well as in private gardens all over the world, thus posing the risk of potential contamination of nearby aquatic bodies inhabited by amphibians. Concurrently, climate change can be expected to alter the temperature of amphibian breeding sites. However, while either glyphosate-based herbicides or temperature have been shown to separately affect the development of amphibians, very little is known on possible interactive effects. We studied the impact of herbicide concentrations and temperature on growth and development of eggs and tadpoles of the Common toad (Bufo bufo L.). We hypothesized that (i) eggs would be better protected against herbicides than tadpoles because of their jelly coating, (ii) that higher temperatures would reduce potential herbicide effects because of an accelerated growth and a lower sensitivity of larger specimens. We conducted one experiment starting with eggs (Gosner stage, GS 8) and another experiment starting with tadpoles (GS 21–24) using a full factorial design with 5 concentrations of the herbicide formulation Roundup® LB Plus (0.0 mg acid equivalent L−1, 0.5, 1.0, or 1.5 mg a.e. L−1 and a pulse treatment with 3 times (egg experiment) or 5 times (tadpole experiment) addition of 0.5 a.e. mg L−1 over the course of several weeks) and two temperature levels (15 and 20°C). Contrary to our expectation, our results showed that toad eggs are more sensitive to herbicides than tadpoles leading to an averaged 31% increase in total length, tail length, and body length compared to the herbicide-free control. Tadpole morphology, development, or mortality was not influenced by herbicides. There was no correlation between herbicide concentration and the effect strength on eggs or tadpoles. Higher temperature accelerated growth of both eggs and tadpoles. As one of the first we also observed interactive effects between herbicide concentrations and temperature especially for egg development resulting in more pronounced herbicide effects at lower temperatures than at higher temperatures. This is quite remarkable as ecotoxicologial risk assessment studies are usually conducted at a constant temperature, thereby perhaps not adequately examining non-target effects at natural conditions. more pronounced herbicide effects at lower temperatures than at higher temperatures. This is quite remarkable as ecotoxicologial risk assessment studies are usually conducted at a constant temperature, thereby perhaps not adequately examining non-target effects at natural conditions.
Introduction
Glyphosate-based herbicides are the most often used pesticides worldwide utilized in agriculture, viticulture, horticulture, municipalities, on railroad tracks as well as in private gardens and aquatic environments (Baylis, 2000). Since its introduction in 1974 glyphosate use in the agricultural sector rose 300-fold in 40 years (Benbrook, 2016). The active ingredient glyphosate is taken up by the leaves affecting the shikimate pathway of plants furthermore constraining the synthesis of the aromatic amino acids phenylalanine, tyrosine, and tryptophan as a consequence of which the plants die (Steinrücken and Amrhein, 1980; Franz et al., 1997). Because glyphosates' mode of action is suggested to affect only enzymes present in plants and some microorganisms (Duke et al., 2012), potential effects on animals are believed to be small (Giesy et al., 2000). However, in recent years studies reporting detrimental side-effects on amphibians exposed to glyphosate-based herbicide formulations are increasing (e.g., Relyea, 2005; Jones et al., 2010; Williams and Semlitsch, 2010; Berger et al., 2013; Wagner et al., 2013). Moreover, not declared surfactants (e.g., polyethoxylated tallow amine) in these formulations are another crucial point to consider as they might be more toxic than the active ingredient itself (Moore et al., 2012; Cuhra et al., 2016; Mullin et al., 2016).
Globally, 32% of amphibian species are identified as threatened and 44% are affected by population declines mainly called forth by climate change, environmental pollution and land use changes (IUCN, 2004; Stuart et al., 2004). Amphibians are considered good bio-indicators for several reasons (Chovanec and Grillitsch, 1994): First, they live in a complex environment, spending their juvenile stadium in water and their life after metamorphosis in terrestrial ecosystems with an action radius of a couple of kilometers. Second, their skin is highly permeable and very sensitive against environmental pollutants (Quaranta et al., 2009). Third, amphibian larvae filter water or rasp surfaces for food (algae, dead organic matter etc.). Pollutants accumulating on and in those substances can lead to a contamination of tadpoles even if pollutant levels in the water are below the level of detection.
Studies conducted with North-American or Australian amphibian species have shown that glyphosate-based herbicides can indeed affect their growth and mortality (Mann and Bidwell, 1999; Relyea, 2004, 2012; Fuentes et al., 2011; Moore et al., 2012; Lanctôt et al., 2014). However, to what extent European amphibian species are affected by herbicides has only rarely been investigated (Brühl et al., 2013; Wagner et al., 2014; Ujszegi et al., 2015). Another, even less investigated aspect is, whether a climate-change induced change in temperature will alter pesticide effects on non-target organisms (Broomhall, 2004; IPCC, 2013). It has been suggested that climate change itself does not affect amphibians, but rather will act in combination with biotic and abiotic factors increasing their effects (López-Alcaide and Macip-Ríos, 2011). Asian amphibian species have shown temperature-dependent toxicity patterns with the pesticide methomyl (Lau et al., 2015). However, Lötters et al. (2014) state that a phenological shift of certain amphibian species toward earlier reproduction due to climate change might expose them less to glyphosate-based herbicides compared to those which do not show a shift in reproduction time. In Europe, the Common toad (B. bufo) is the most widespread amphibian species, living in habitats from lowland up to mountainous areas over 2000 m above sea-level (Cabela et al., 2001; Arnold and Ovenden, 2002). Spawning ponds used by Common toads are often located in agriculturally used landscapes, therefore prone to contamination by agrochemicals applied on the nearby fields.
The current experiment focused on the aquatic phase of Common toad development. There are several ways how herbicide formulations can pollute aquatic environments, including, direct overspraying of rivulets, puddles and small ponds, water run-off through heavy rainfall and erosion of herbicide contaminated soil by wind and water (Solomon and Thompson, 2003; Vereecken, 2005; Sasal et al., 2015). Although its molecular properties and high sorption capacity binds glyphosate tightly to soil and organic particles (Hance, 1976), several reports showed that glyphosate and its degradation products (e.g., aminomethylphosphonic acid, AMPA) are leached out of soils (Zaller et al., 2014) and are found in groundwater, rivers, and ponds and that concentration levels can be high (Scribner et al., 2002; Stadlbauer and Fank, 2005; Peruzzo et al., 2008; Battaglin et al., 2009). Furthermore, climate change is thought to increase pesticide application in Europe (Kattwinkel et al., 2011).
The current study was designed to examine whether (i) different concentrations of a glyphosate-based herbicide have different effects on the development of either eggs or tadpoles of the Common toad and whether (ii) water temperature influences potential herbicide effects on eggs or tadpoles. Generally, we expected that higher herbicide concentrations will be more harmful than lower concentrations and that tadpoles would be more susceptible than eggs because the latter are protected with a gelatinous substance which protects them from environmental factors (Mutschmann, 2010). Because of a faster development of amphibians at higher temperatures, we expected herbicide effects to be less pronounced at higher than at lower water temperature.
Materials and Methods
Eggs and tadpoles of B. bufo were collected in March and April 2014 from a small pond located in the outskirts of Vienna, Austria (48.240948 N, 16.275672 E). The pond is surrounded by a beech forest and a meadow; to the best of our knowledge, no herbicide application occurred in the vicinity of the pond in the last couple of years. Bufo spawn strings were hand-collected from different randomly selected clutches, transferred into plastic containers filled with pond water and stored for 2 days in a climate chamber at 10°C. Egg and tadpole development was determined according to Gosner (1960), who used morphological features to classify amphibian eggs and tadpoles starting with egg fertilization (Gosner stage, GS 1) and ending with the tail resorbtion of the fully metamorphosed tadpole (GS 46). Spawns were sampled with permission of the pond owner (City of Vienna, permission no. MA49-808754/2014/3) and of the Vienna Environmental Protection Agency (permission no. MA22-736519/2013).
Experimental Setup
We conducted two experiments between end of March and May 2014 in the laboratory of the Institute of Zoology, University of Natural Resources and Life Sciences, Vienna, Austria. One experiment started with B. bufo eggs (GS 8 “egg experiment”) the other one started with B. bufo tadpoles (GS 22, “tadpole experiment”; more details below). Larvae at GS 8 are egg shaped embryos characterized by midcleavage, larvae at GS 22 are tadpole shaped hatchlings characterized by transparent tail fins and fin circulation. In both experiments we studied the effects of herbicide concentration (5 levels) and water temperature (2 levels) and their interactions.
Experimental units were transparent polypropylene tubs (length: 28 cm, width: 19 cm, height: 14 cm; type SAMLA, IKEA, Leiden, The Netherlands) filled with 4 L of coal-filtered (EHEIM classic 250; Deizisau, Germany) tap water. To establish the herbicide treatment we used a liquid formulation of Roundup® LB Plus (Monsanto Co., St. Louis, Missouri, USA) containing the active ingredient glyphosate (360 g L−1 glyphosate, 486 g L−1 Isopropylamine salt). The herbicide was purchased in a garden shop in a 140 ml bottle (Diwoky GmbH, Vienna, Austria). In Austria, Roundup® LB Plus is registered for use in agriculture, viticulture, forestry, horticulture, municipalities, and private gardens. Although application of this herbicide near water bodies is prohibited, we observed that glyphosate-based herbicides are nevertheless applied near streams for instance to control neophytes. In our experiments we studied effects of the commercially available formulation of the herbicide rather than the pure active ingredient glyphosate in order to assess the real-world situation for amphibians. In the tubs containing the toad eggs we established five different herbicide concentrations: (a) a control treatment containing no herbicide (0.0 mg a.e., acid equivalent L−1), (b) three herbicide treatments where the herbicide was added once, at the beginning of the experiment at 0.5 mg a.e. L−1, 1.0 mg a.e. L−1, or 1.5 mg a.e. L−1 and c) a pulse treatment with additions of 0.5 a.e. mg L−1 on day 0, 3, and 4 after the start when studying egg development (total 1.5 mg a.e. L−1) or 0.5 mg a.e. L−1 pulses on day 0, 4, 8, 12, 17 when studying tadpoles (total 2.5 mg a.e. L−1). These glyphosate concentrations in water are within the range of concentrations used in other studies (Relyea, 2005; Bernal et al., 2009; Relyea and Jones, 2009; Jones et al., 2010) and are reported in studies about pesticide pollution in water bodies within agricultural landscapes (Wagner et al., 2013, 2014).
Temperature treatments were established in two climate chambers (Vötsch Type VPL 400, Weiss Umwelttechnik GesmbH, Vienna, Austria), one maintained at 15°C the other one at 20°C. Under each temperature treatment 5 replicates of the 5 herbicide treatments were established making in total 25 tubs per temperature treatment. The air temperature within the climate chambers was monitored with two data loggers (tiny tag, Gemini Data Loggers, West Sussex, United Kingdom). To monitor water quality in the tubs we measured the dissolved oxygen and temperature at least every third day with an oximeter (WTW, type Oxi 90, Weilheim, Germany). Additionally, we measured the pH-value with a pH-Meter (WTW, type pH-95, Weilheim, Germany).
At the start of each experiment 5 randomly chosen eggs or tadpoles were added into each experimental tub resulting in a total of 250 individuals per experiment (5 individuals × 5 replicates × 5 herbicide concentrations × 2 temperatures). Tadpoles were fed with fish food ad libitum (TetraMin, Tetra GmbH, Melle, Germany). The experiments were conducted with a permission for animal testing from the Austrian Federal Ministry of Science, Research and Economy (BMWFW; permission no. BMWFW-66.016/0011-II/3b/2013). In the case tadpoles would get harmed, injured or obviously poisoned an euthanization with MS-222 (Tricaine mesylate; Sandoz, Basel, Switzerland) was foreseen.
Measurements
The experiment on egg development started with eggs at GS 8 (Gosner, 1960) and lasted 22 days. We took images of the tadpoles 22 days after the start of the experiment using a digital single lens reflex camera (Nikon D200 with an Nikon DX objective—APS Nikkor 18–70 mm; Nikon, Tokyo, Japan) in order to non-destructively assess morphological changes in size and shape.
The experiment on tadpole development started with new randomly selected tadpoles (GS 21–24) from the same pond where the eggs were collected. Tadpoles were stored in the climate chamber (10°C) for 2 days in a plastic container filled with pond water. Then 5 tadpoles were randomly selected and distributed among the experimental units that were filled with 4 L coal-filtered tap water (same type of tubs as used in egg experiment). The tadpole experiment ran for 24 days. Treatment effects on tadpole morphology were assessed on images taken by a digital camera at day 24 (for 20°C tadpoles) and day 42 (for 15°C tadpoles).
Egg development was on average assessed every third day and tadpole development every sixth day according to Gosner (1960). Therefore, all tadpoles were collected from each tub, transferred into a petri dish with deionized water and examined under a binocular at 6x magnification (NIKON, type SMZ 645, Leuven, Belgium). To assess tadpole development we took photos of all tadpoles at the end of each experiment. Tadpoles were put in a petri dish (diameter 10 cm) with deionized water and photographs of each tadpole were taken using a digital reflex camera (Nikon D200 with an Nikon DX objective—APS Nikkor 18–70 mm; Nikon, Tokyo, Japan) mounted on a tripod. On these images total length (body + tail length), tail length, body length, and body width were measured on all surviving individuals per experimental unit using the open source image analysis software ImageJ (version 1.48v, National Institute of Health, USA).
Statistical Analyses
All data were tested for homogeneity of variance and normal distribution with the Kolmogorov-Smirnov and the Levene test, respectively. Effects of the herbicide and the temperature treatments and treatment interactions on tadpole morphology, development, and mortality as well as on water pH and water oxygen were tested with two-way analyses of variance (ANOVAs). Tukey tests were used for multiple comparisons between the different concentrations within each temperature treatment. Statistical results at P < 0.05 were considered significant. All statistical tests were performed using the software SPSS (Version 20, IBM©, Armonk, New York, U.S.).
Results
Egg Growth and Development
Herbicide concentration significantly affected body length, tail length, and total length (Table 1, Figure 1). No significant effects of herbicide concentration on body width, mortality, or development were found (Table 1, Figure 1). Temperature significantly affected all growth parameters, development and mortality of eggs (Table 1, Figure 1). On average, body length, body width, tail length and total length of tadpoles at 20°C was increased by 63, 57, 86, and 76% compared to 15°C. Temperature accelerated the development, 22 days after the first herbicide application, the development stage at 20°C was GS 36 while tadpoles at 15°C had only reached GS 28; herbicide concentrations had no significant effect on egg development (Table 1, Figure 3A). Significant herbicide × temperature interactions for body length, body width, tail length and total length resulted in an increased body length of herbicide treated tadpoles by 26%, body width by 19%, tail length by 35%, and total length by 31% at 15°C compared to the no-herbicide control. In contrast, at 20°C body length, tail length, and total length of herbicide treated tadpoles increased by about 3% and body width was decreased by 2% compared to no-herbicide control. In total, 12.4% (31 out of 250 individuals) of the eggs/tadpoles died or had to be euthanized during the course of the experiment. Mortality was significantly affected by temperature but not by herbicide concentrations or temperature-herbicide, with a total of 18.4% (23 ind.) dead eggs/tadpoles at 15°C compared to 6.4% (8 ind.) dead eggs/tadpoles at 20°C averaged across all herbicide concentrations (Table 1, Figure 4). Water pH was significantly influenced by herbicide concentration and temperature. Across herbicide concentrations pH values measured were 8.31 ± 0.02 vs. 8.14 ± 0.07 at 15 vs. 20°C, respectively; water oxygen at 15°C was 10.07 ± 0.11 mg L−1, vs. 8.85 ± 0.68 mg L−1 at 20°C, respectively (Tables 1, 2). No significant influence of herbicide concentration on water oxygen and herbicide by temperature interaction on water oxygen and pH were found (Tables 1, 2).
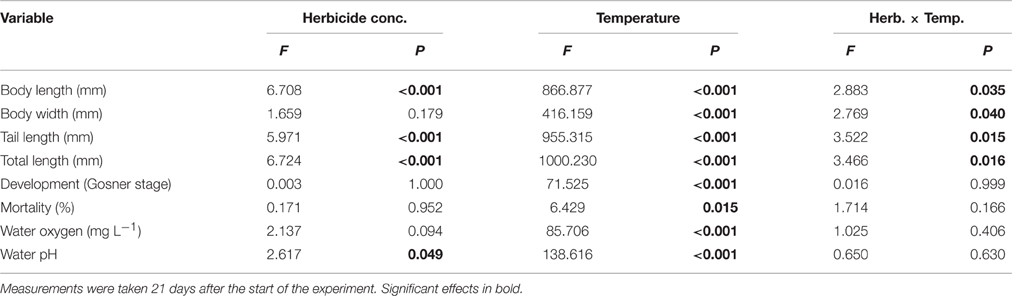
Table 1. Egg experiment–Results of a 2-way ANOVA on the effect of a herbicide treatment (5 levels) and a temperature treatment (2 levels) on growth and mortality of the Common toad as well as on water oxygen and pH.
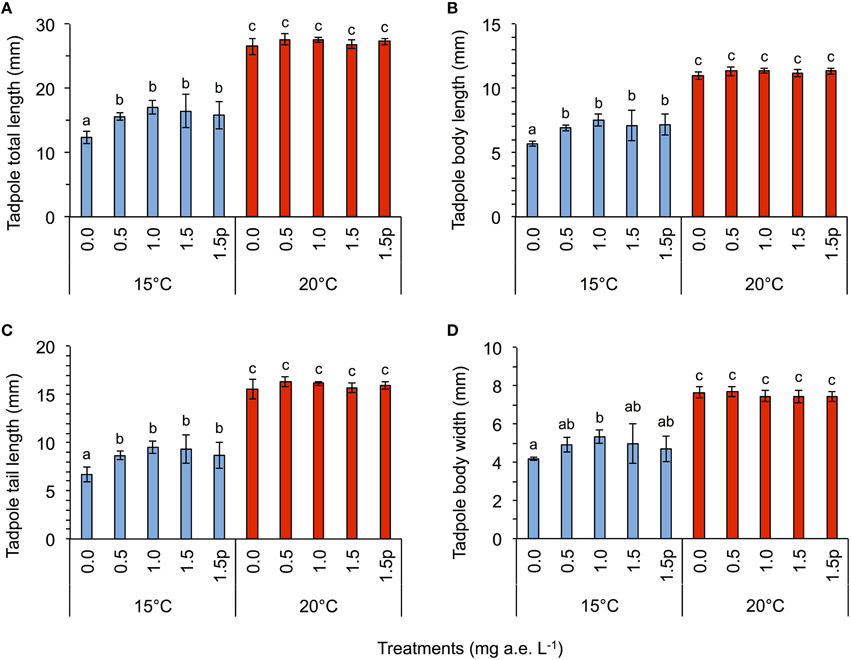
Figure 1. Egg experiment-Average tadpole total length (A), body length (B), tail length (C), and body width (D) in response to five herbicide concentrations (0.0, 0.5, 1.0, 1.5 a.e. mg L−1 and a 1.5p a.e. mg L−1 pulse treatment) at two temperatures (15 vs. 20°C) at the end of the experiment. Means ± SD; n = 5. Different letters indicate significant differences between means (Tukey's HSD, p < 0.05).
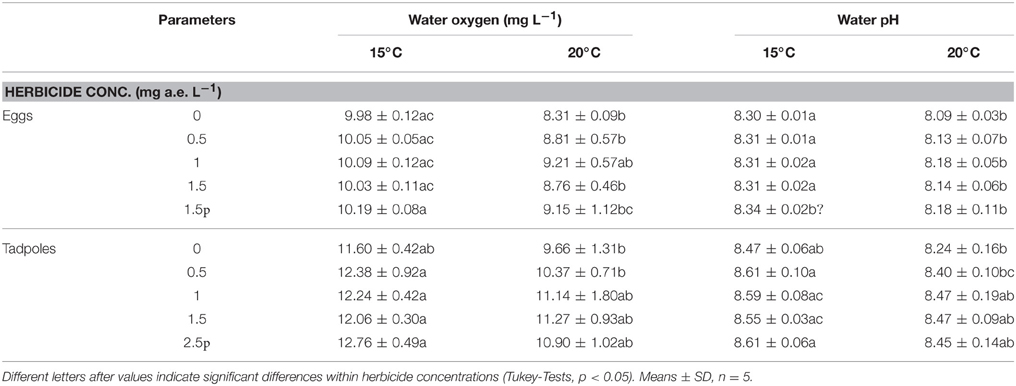
Table 2. Water oxygen and pH in the egg and tadpole experiment investigating the effects of herbicide concentrations and temperature on Common toad growth and development.
Tadpole Growth and Development
Herbicide concentrations had no effect on tadpole growth, development or mortality (Table 3, Figures 2, 3). However, temperature highly significantly affected tadpole growth and development but not mortality (Table 3, Figure 2). Body length, body width, tail length, and total length of tadpoles at 20°C was on average increased by 12, 8, 22, and 18%, respectively, compared to 15°C. Only for tadpole development a significant interaction of herbicide concentration and temperature was found: larvae at 15°C reached on average GS 31, whereas at 20°C an averaged GS 39 was reached 24 days after the first herbicide application (Table 3, Figure 3B). In total, 14% (35 out of 250) of the tadpoles died or had to be euthanized during this experiment, however neither herbicide concentration nor temperature affected tadpole mortality (Table 3, Figure 4). During the tadpole experiment, water pH was significantly influenced by herbicide concentration and temperature, whereas water oxygen was significantly influenced by temperature only (Tables 2, 3). Across herbicide concentrations, water pH was 8.57 ± 0.08 vs. 8.41 ± 0.16 at 15 vs. 20°C, water oxygen was 12.21 ± 0.64 mg L−1 vs. 10.67 ± 1.26 mg L−1 at 15 vs. 20°C, respectively. No significant interaction of herbicide concentration and temperature on water oxygen and pH was observed (Table 3).
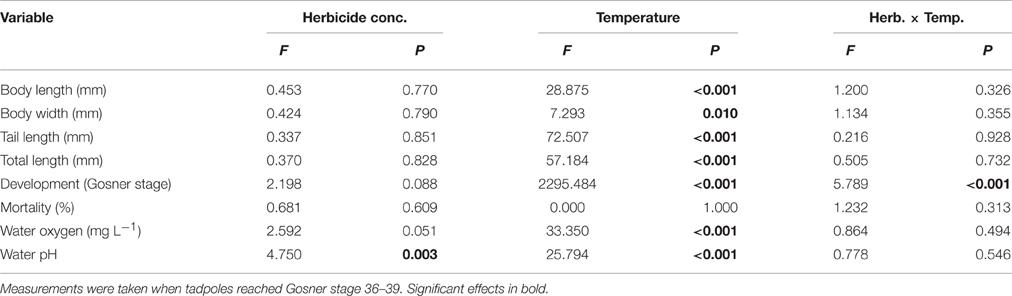
Table 3. Tadpole experiment–Results of a 2-way ANOVA on the effect of a herbicide treatment (5 levels) and a temperature treatment (2 levels) on growth and mortality of the Common toad as well as on water oxygen and pH.
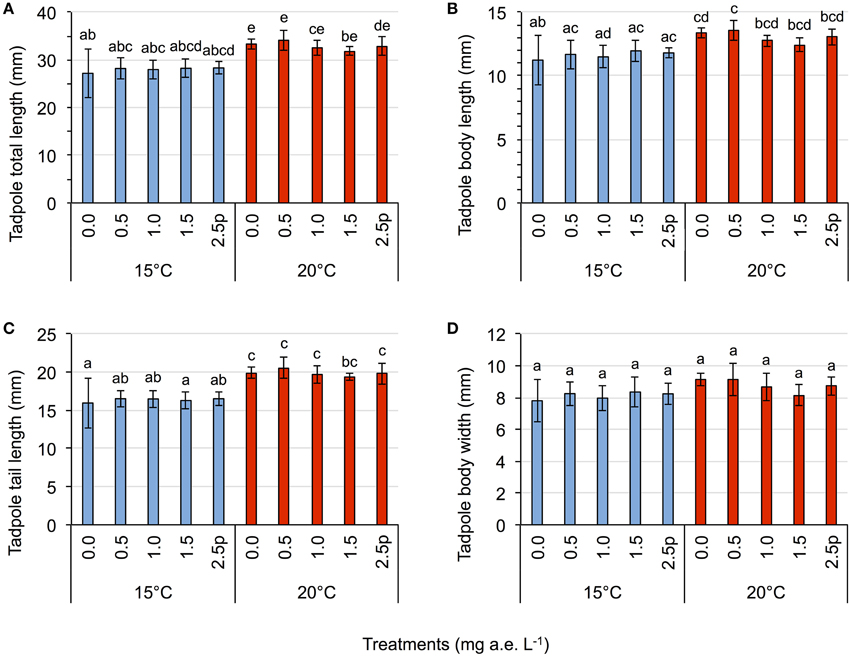
Figure 2. Tadpole experiment–Average tadpole length (A), body length (B), tail length (C), and body width (D) in response to five herbicide concentrations (0.0, 0.5, 1.0, 1.5 a.e. mg L−1 and a 2.5p a.e. mg L−1 pulse treatment) at two temperatures (15 vs. 20°C) at the end of the experiment. Means ± SD, n = 5. Different letters indicate significant differences between means (Tukey's HSD, p < 0.05).
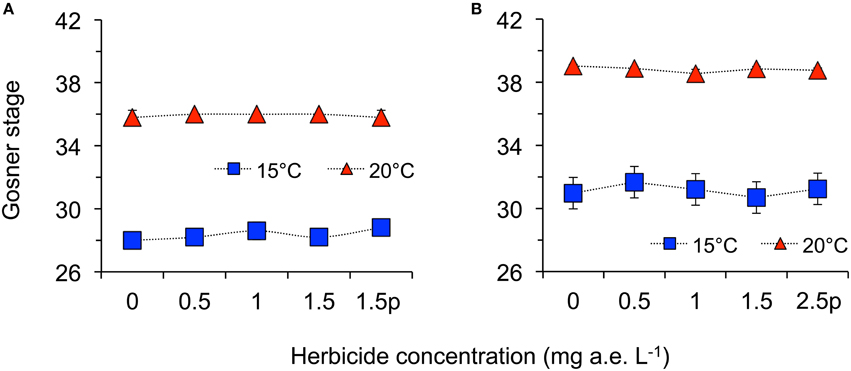
Figure 3. Average development stage according to Gosner (1960) of eggs (A) and tadpoles (B) of the Common toad in response to five herbicide concentrations (0.0, 0.5, 1.0, 1.5 a.e. mg L−1 and a 1.5p/2.5p a.e. mg L−1 pulse treatment) at two temperatures (15 vs. 20°C) at the end of the experiments; n = 5.
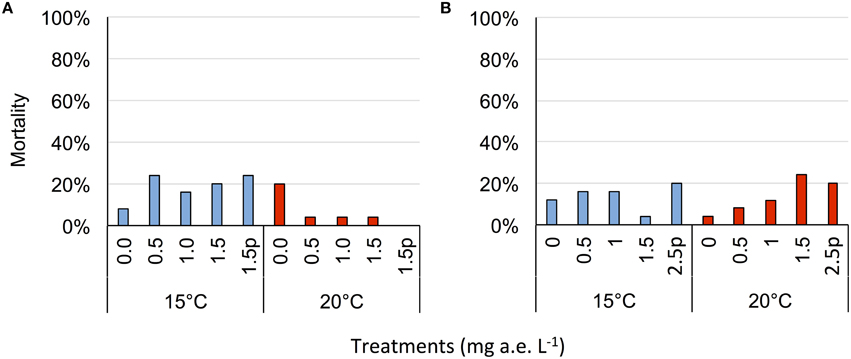
Figure 4. Average mortality (% of total) of eggs (A) and tadpoles (B) of the Common toad in response to five herbicide concentrations (0.0, 0.5, 1.0, 1.5 a.e. mg L−1 and a 1.5p/2.5p a.e. mg L−1 pulse treatment) at two temperatures (15 vs. 20°C) at the end of the experiment; n = 5.
Discussion
To the best of our knowledge, this study shows for the first time that the globally most widely used herbicide Roundup® affects the growth and development of aquatic stages of Common toads. We expected that egg growth and development would be less sensitive to herbicide concentrations than tadpoles due to the protective jelly coating of the eggs. Indeed, other studies showed that jelly coatings protected embryos of North American amphibian species against endosulfan or tebufenozide pesticides (Berrill et al., 1998; Pauli et al., 1999). A decreased hatching success was also observed for eggs of a European frog species (Rana arvalis) that were exposed to the insecticide α-cypermethrin (Greulich and Pflugmacher, 2003); however, no comparison to tadpole development was made in that study. In contrast, in our experiment eggs were actually more sensitive to herbicide concentrations than tadpoles. Since no other study looked into effects of glyphosate-based herbicides on Common toad eggs it remains to be investigated if toad eggs are either especially sensitive or if glyphosate-based herbicides are especially harmful to egg stages of amphibians. Overall, a potential contamination by glyphosate-based herbicides of toad spawning ponds located in agriculturally used landscapes can be expected both for egg and tadpole stages as timing of pesticide application has been shown to match the life cycle of amphibians (Berger et al., 2013; Brühl et al., 2013). Clearly, more studies including more amphibian species are needed to estimate whether this effect is species-specific or applicable more widely.
Our experiment showed a significant increase in body length, tail length, and total length at 15°C after herbicide application which we interpret as a consequence of a further nutrient addition by the herbicide. In contrast to our results, Lanctôt et al. (2014) found that snout to vent length of tadpoles decreased by 11.3 and 5.8% when exposed to two different glyphosate-based herbicide formulations, either two pulses of 0.21 mg a.e. L−1 Roundup WeatherMax® or 2.89 mg a.e. L−1 Vision®, respectively. However, comparisons with our results have to be made with caution as (i) different glyphosate-based herbicide formulations were used containing different adjuvants with potentially ecotoxicological side-effects (Mullin et al., 2016), (ii) different amphibian species (Rana sylvaticus) at a different temperature (21.4°C) have been investigated, and (iii) even the active ingredient glyphosate is not a single chemical, but rather a family of compounds with different chemical, physical, and toxicological properties making comparisons between different glyphosate-based formulations even more tricky (Cuhra et al., 2016).
Another hypothesis of our study was that higher herbicide concentrations would show more pronounced growth effects than lower concentrations. Based on the egg and tadpole experiment we can reject this hypothesis as no correlation between herbicide concentration and the strength of the effect on growth, development, or mortality was observed. Reportedly, glyphosate has a half-life of 7–14 days in water (Franz et al., 1997; Giesy et al., 2000). Hence, our finding suggests that even low concentrations affected the growth of toad eggs and tadpoles and that the higher concentrated active ingredient might had already disappeared when the growth measurements were conducted. Again, not-declared adjuvants of glyphosate-based herbicide formulations with a higher toxicity than the glyphosate active ingredient itself (Perkins et al., 2000; Edginton et al., 2004; Howe et al., 2004; Peixoto, 2005; Wagner et al., 2013) or their properties as as endocrine disruptors (Gasnier et al., 2009; Defarge et al., 2016) might have contributed to the observed effects. Observed growth stimulations of toad larvae at low herbicide concentrations could perhaps be due to endocrine disruptors as they generally lack a dose-effect relationship (Vandenberg et al., 2012; Vandenberg, 2014). However, the design of our study precludes a substantiation of this explanation. In any case our findings suggest that agrochemical risk assessments that take into account only pesticide active ingredients without their adjuvants commonly used in their comercial formulations will likely miss important toxicological effects (Mullin et al., 2016).
Water temperature showed a consistent strong stimulating effect for both egg and tadpole growth and development. This was expected and has been shown by other studies (Dmitrieva, 2014; Egea-Serrano and Van Buskirk, 2016). Moreover, also mortality was significantly affected by temperature in the egg experiment, where 21% of herbicide treated larvae died at 15°C but only 3% at 20°C. Relyea (2005) determined the lethal concentration where 50% of the population (LC50) of wood frogs (Rana sylvaticus) died to be at 1.32 mg active ingredient (a.i.) L−1 but the LC50 value of the American toad (Bufo americanus) at 2.52 mg a.i. L−1. We assume that the Common toad would react in a similar way, however, it is difficult to compare these studies as different herbicide formulations and different concentrations were used.
To the best of our knowledge, our study is also the first one investigating combined effects of herbicides and temperature on growth and development of a European amphibian species. We found interactive effects of herbicide and temperature on the growth of toad eggs with herbicide effects only seen at 15°C but not at 20°C. This indicates on one hand that toad egg growth benefited from higher temperatures making them less prone to herbicide concentrations at higher temperature. On the other hand, the tadpole experiment showed that interactive effects of herbicide concentration and temperature were only observed for tadpole development but not for their growth. Tadpole growth also benefited from higher temperatures and was generally less sensitive to herbicide concentration. However, regarding tadpole development the herbicide x temperature interaction indicated a reduced development at 15°C when herbicides were used but no change in development at 20°C.
The current study investigated effects of herbicide concentrations and temperature on Common toad growth and development in a controlled environment. Therefore, an inference of these data to the field situation should be made with caution, as the circumstances in the field are much more complex. For example, predators present in natural sites can trigger morphological changes in amphibian larvae and even enhance these changes in combination with herbicide exposure (Jones et al., 2010). Two other studies found glyphosate-based herbicide formulations (Roundup® and Roundup Original MAX®) being more deadly to larvae of wood frogs (Rana sylvatica) and bullfrogs (Rana catesbeiana) under a higher stress level (Relyea, 2005; Jones et al., 2011). Although mortality was not influenced by herbicides in our study, it appears that eggs were more stressed under lower temperatures and therefore more susceptible to herbicide concentrations. However, it also has to be noted that most studies, including ours, are rather short term studies and often do not take into account effects of multiple application of various pesticides, which can be even more deleterious to amphibian larvae (Relyea, 2009).
An important implication of our study is that water temperature can alter potential non-target effects of herbicides on amphibian species. This is remarkable as ecotoxicologial risk assessment studies are often conducted at a standard temperature of 20°C which was the temperature showing fewer effects than lower, perhaps more realistic, temperatures.
Author Contributions
MJ, EG, and JZ planned and conducted the experiment. FB and MJ wrote the first draft and analyzed the data. All authors reviewed and edited the final manuscript.
Conflict of Interest Statement
The authors declare that the research was conducted in the absence of any commercial or financial relationships that could be construed as a potential conflict of interest.
Acknowledgments
We are grateful to Rick Relyea and Carsten Brühl for advice during the planning and carrying out of this experiment. Comments of the reviewers helped to improve an earlier version of this manuscript. This study was partly funded by The Austrian Federal Ministry of Agriculture, Forestry, Environment and Water Management (BMLFUW, project no. 100977/1).
References
Arnold, N., and Ovenden, D. (2002). A Field Guide to the Reptiles and Amphibians of Britain and Europe. London: HarperCollins.
Battaglin, W. A., Rice, K. C., Focazio, M. J., Salmons, S., and Barry, R. X. (2009). The occurrence of glyphosate, atrazine, and other pesticides in vernal pools and adjacent streams in Washington, DC, Maryland, Iowa, and Wyoming, 2005–2006. Environ. Monit. Assess. 155, 281–307. doi: 10.1007/s10661-008-0435-y
Baylis, A. D. (2000). Why glyphosate is a global herbicide: strengths, weaknesses and prospects. Pest Manag. Sci. 56, 299–308. doi: 10.1002/(SICI)1526-4998(200004)56:4<299::AID-PS144>3.0.CO;2-K
Benbrook, C. (2016). Trends in glyphosate herbicide use in the United States and globally. Environ. Sci. Eur. 28, 3. doi: 10.1186/s12302-016-0070-0
Berger, G., Graef, F., and Pfeffer, H. (2013). Glyphosate applications on arable fields considerably coincide with migrating amphibians. Sci. Rep. (Nature) 3:2622. doi: 10.1038/srep02622
Bernal, M. H., Solomon, K. R., and Carrasquilla, G. (2009). Toxicity of formulated Glyphosate (Glyphos) and Cosmo-Flux to larval and juvenile Colombian frogs 2. Field and laboratory microcosm acute toxicity. J. Toxicol. Environ. Health A. 72, 966–973. doi: 10.1080/15287390902929717
Berrill, M., Coulson, D., Mcgillivray, L., and Pauli, B. (1998). Toxicity of endosulfan to aquatic stages of anuran amphibians. Environ. Toxicol. Chem. 17, 1738–1744. doi: 10.1002/etc.5620170914
Broomhall, S. D. (2004). Egg temperature modifies predator avoidance and the effects of the insecticide endosulfan on tadpoles of an Australian frog. J. Appl. Ecol. 41, 105–113. doi: 10.1111/j.1365-2664.2004.00883.x
Brühl, C. A., Schmidt, T., Pieper, S., and Alscher, A. (2013). Terrestrial pesticide exposure of amphibians: an underestimated cause of global decline? Sci. Rep. 3:1135. doi: 10.1038/srep01135
Cabela, A., Grillitsch, H., and Tiedemann, F. (2001). Atlas zur Verbreitung und Ökologie der Amphibien und Reptilien in Österreich: Auswertung der Herpetofaunistischen Datenbank der Herpetologischen Sammlung des Naturhistorischen Museums in Wien. Wien: Umweltbundesamt.
Chovanec, A., and Grillitsch, B. (1994). Amphibien als Bioindikatoren für die Schadstoffbelastung von Kleingewässern. Wien: Umweltbundesamt.
Cuhra, M., Bøhn, T., and Cuhra, P. (2016). Glyphosate: too much of a good thing? Front. Environ. Sci. 4:28. doi: 10.3389/fenvs.2016.00028
Defarge, N., Takaìcs, E., Lozano, V. L., Mesnage, R., Vendômois, J. S., Séralini, G. E., et al. (2016). Co-formulants in glyphosate-based herbicides disrupt aromatase activity in human cells below toxic levels. Int. J. Environ. Res. Public Health 13:264. doi: 10.3390/ijerph13030264
Dmitrieva, E. V. (2014). Experimental study of the temperature effect on survival and development rates in the early ontogenesis of common toad (Bufo bufo). Moscow Univ. Biol. Sci. Bull. 69, 71–73. doi: 10.3103/S0096392514020023
Duke, S. O., Lydon, J., Koskinen, W. C., Moorman, T. B., Chaney, R. L., and Hammerschmidt, R. (2012). Glyphosate effects on plant mineral nutrition, crop rhizosphere microbiota, and plant disease in glyphosate-resistant crops. J. Agric. Food Chem. 60, 10375–10397. doi: 10.1021/jf302436u
Edginton, A. N., Sheridan, P. M., Stephenson, G. R., Thompson, D. G., and Boermans, H. J. (2004). Comparative effects of pH and Vision herbicide on two life stages of four anuran amphibian species. Environ. Toxicol. Chem. 23, 815–822. doi: 10.1897/03-115
Egea-Serrano, A., and Van Buskirk, J. (2016). Responses to nitrate pollution, warming and density in common frog tadpoles (Rana temporaria). Amphibia-Reptilia 37, 45–54. doi: 10.1163/15685381-00003029
Franz, J. E., Mao, M. K., and Sikorski, J. A. (1997). Glyphosate: A Unique Global Herbicide. Washington, DC: American Chemical Society.
Fuentes, L., Moore, L. J., Rodgers, J. H., Bowerman, W. W., Yarrow, G. K., and Chao, W. Y. (2011). Comparative toxicity of two glyphosate formulations (Original formulation of Roundup® and Roundup Weathermax®) to six North American larval anurans. Environ. Toxicol. Chem. 30, 2756–2761. doi: 10.1002/etc.670
Gasnier, C., Dumont, C., Benachour, N., Clair, E., Chagnon, M.-C., and Séralini, G.-E. (2009). Glyphosate-based herbicides are toxic and endocrine disruptors in human cell lines. Toxicology 262, 184–191. doi: 10.1016/j.tox.2009.06.006
Giesy, J. P., Dobson, S., and Solomon, K. R. (2000). Ecotoxicological risk assessment for Roundup® Herbicide. Rev. Environ. Contam. Toxicol. 167, 35–120. doi: 10.1007/978-1-4612-1156-3_2
Gosner, K. L. (1960). A simplified table for staging anuran embryos and larvae with notes on identification. Herpetologica 16, 183–190.
Greulich, K., and Pflugmacher, S. (2003). Differences in susceptibility of various life stages of amphibians to pesticide exposure. Aquat. Toxicol. 65, 329–336. doi: 10.1016/S0166-445X(03)00153-X
Hance, R. J. (1976). Adsorption of glyphosate by soils. Pestic. Sci. 7, 363–366. doi: 10.1002/ps.2780070407
Howe, C. M., Berrill, M., Pauli, B. D., Helbing, C. C., Werry, K., and Veldhoen, N. (2004). Toxicity of glyphosate-based pesticides to four North American frog species. Environ. Toxicol. Chem. 23, 1928–1938. doi: 10.1897/03-71
IUCN (2004). IUCN Red List of Threatened Species: A Global Species Assessment. Gland; Cambridge: IUCN.
IPCC (2013). Climate Change 2013: The Physical Science Basis. Contribution of Working Group I to the Fifth Assessment Report of the Intergovernmental Panel on Climate Change. Cambridge, UK; New York, NY: Cambridge University Press
Jones, D. K., Hammond, J. I., and Relyea, R. A. (2010). Roundup and amphibians: the importance of concentration, application time, and stratification. Environ. Toxicol. Chem. 29, 2016–2025. doi: 10.1002/etc.240
Jones, D. K., Hammond, J. I., and Relyea, R. A. (2011). Competitive stress can make the herbicide Roundup more deadly to larval amphibians. Environ. Toxicol. Chem. 30, 446–454. doi: 10.1002/etc.384
Kattwinkel, M., Kühne, J. V., Foit, K., and Lies, M. (2011). Climate change, agricultural insecticide exposure, and risk for freshwater communities. Ecol. Appl. 21, 2068–2031. doi: 10.1890/10-1993.1
Lanctôt, C., Navarro-Martín, L., Robertson, C., Park, B., Jackman, P., Pauli, B. D., et al. (2014). Effects of glyphosate-based herbicides on survival, development, growth and sex ratios of wood frog (Lithobates sylvaticus) tadpoles. II: agriculturally relevant exposures to Roundup WeatherMax® and Vision® under laboratory conditions. Aquat. Toxicol. 154, 291–303. doi: 10.1016/j.aquatox.2014.05.025
Lau, E. T., Karraker, N. E., and Leung, K. M. (2015). Temperature-dependent acute toxicity of methomyl pesticide on larvae of three Asian amphibian species. Environ. Toxicol. Chem. 34, 2322–2327. doi: 10.1002/etc.3061
López-Alcaide, S., and Macip-Ríos, R. (2011). “Effects of climate change in amphibians and reptiles,” in Biodiversity Loss in a Changing Planet, ed O. Grillo (Rijeka: InTech), 163–184.
Lötters, S., Filz, K., Wagner, N., Schmidt, B., Emmerling, C., and Veith, M. (2014). Hypothesizing if responses to climate change affect herbicide exposure risk for amphibians. Environ. Sci. Eur. 26, 1–5. doi: 10.1186/s12302-014-0031-4
Mann, R. M., and Bidwell, J. R. (1999). The toxicity of glyphosate and several glyphosate formulations to four species of southwestern Australian frogs. Arch. Environ. Contam. Toxicol. 36, 193–199. doi: 10.1007/s002449900460
Moore, L. J., Fuentes, L., Rodgers, J. H., Bowerman, W. W., Yarrow, G. K., Chao, W. Y., et al. (2012). Relative toxicity of the components of the original formulation of Roundup® to five North American anurans. Ecotoxicol. Environ. Saf. 78, 128–133. doi: 10.1016/j.ecoenv.2011.11.025
Mullin, C. A., Fine, J. D., Reynolds, R. D., and Frazier, M. T. (2016). Toxicological risks of agrochemical spray adjuvants: organosilicone surfactants may not be safe. Front. Public Health 4:92. doi: 10.3389/fpubh.2016.00092
Pauli, B. D., Coulson, D. R., and Berrill, M. (1999). Sensitivity of amphibian embryos and tadpoles to Mimic® 240 LV insecticide following single or double exposures. Environ. Toxicol. Chem. 18, 2538–2544. doi: 10.1002/etc.5620181122
Peixoto, F. (2005). Comparative effects of the Roundup and glyphosate on mitochondrial oxidative phosphorylation. Chemosphere 61, 1115–1122. doi: 10.1016/j.chemosphere.2005.03.044
Perkins, P. J., Boermans, H. J., and Stephenson, G. R. (2000). Toxicity of glyphosate and triclopyr using the frog embryo teratogenesis assay-Xenopus. Environ. Toxicol. Chem. 19, 940–945. doi: 10.1002/etc.5620190422
Peruzzo, P. J., Porta, A. A., and Ronco, A. E. (2008). Levels of glyphosate in surface waters, sediments and soils associated with direct sowing soybean cultivation in north pampasic region of Argentina. Environ. Pollut. 156, 61–66. doi: 10.1016/j.envpol.2008.01.015
Quaranta, A., Bellantuono, V., Cassano, G., and Lippe, C. (2009). Why amphibians are more sensitive than mammals to Xenobiotics. PLoS ONE 4:e7699. doi: 10.1371/journal.pone.0007699
Relyea, R. A. (2004). Growth and survival of five amphibian species exposed to combinations of pesticides. Environ. Toxicol. Chem. 23, 1737–1742. doi: 10.1897/03-493
Relyea, R. A. (2005). The lethal impacts of roundup and predatory stress on six species of North American tadpoles. Arch. Environ. Contam. Toxicol. 48, 351–357. doi: 10.1007/s00244-004-0086-0
Relyea, R. A. (2009). A cocktail of contaminants: how mixtures of pesticides at low concentrations affect aquatic communities. Oecologia 159, 363–376. doi: 10.1007/s00442-008-1213-9
Relyea, R. A. (2012). New effects of Roundup on amphibians: predators reduce herbicide mortality; herbicides induce antipredator morphology. Ecol. Appl. 22, 634–647. doi: 10.1890/11-0189.1
Relyea, R. A., and Jones, D. K. (2009). The toxicity of roundup original max® to 13 species of larval amphibians. Environ. Toxicol. Chem. 28, 2004–2008. doi: 10.1897/09-021.1
Sasal, M. C., Demonte, L., Cislaghi, A., Gabioud, E. A., Oszust, J. D., Wilson, M. G., et al. (2015). Glyphosate loss by runoff and its relationship with phosphorus fertilization. J. Agric. Food Chem. 63, 4444–4448. doi: 10.1021/jf505533r
Scribner, E. A., Battaglin, W. A., Dietze, J. E., and Thurman, E. M. (2002). Reconnaissance Data for Glyphosate, Other Selected Herbicides, Their Degradation Products, and Antibiotics in 51 Streams in Nine Midwestern States, 2002. U.S. Geological Survey Open-File Report 03–217.
Solomon, K. R., and Thompson, D. (2003). Ecological risk assessment for aquatic organisms from over-water uses of glyphosate. J. Toxicol. Environ. Health Part B Crit. Rev. 6, 289–324. doi: 10.1080/10937400306468
Stadlbauer, H., and Fank, J. (2005). Sickerwasserversuche an der Forschungsstation Wagna zur Untersuchung der Verlagerung des Herbizids Glyphosate in der Ungesättigten Bodenzone. Report Umweltbundesamt. Available online at: http://www.literature.at/alo?objid=18684
Steinrücken, H. C., and Amrhein, N. (1980). The herbicide glyphosate is a potent inhibitor of 5-enolpyruvylshikimic acid-3-phosphate synthase. Biochem. Biophys. Res. Commun. 94, 1207–1212. doi: 10.1016/0006-291X(80)90547-1
Stuart, S. N., Chanson, J. S., Cox, N. A., Young, B. E., Rodrigues, A. S. L., Fischman, D. L., et al. (2004). Status and trends of amphibian declines and extinctions worldwide. Science 306, 1783–1786. doi: 10.1126/science.1103538
Ujszegi, J., Gál, Z., Mikó, Z., and Hettyey, A. (2015). No observable effect of a glyphosate-based herbicide on two top predators of temporal water bodies. Environ. Toxicol. Chem. 34, 307–313. doi: 10.1002/etc.2798
Vandenberg, L. N. (2014). Low-dose effects of hormones and endocrine disruptors. Vitam. Horm. 94, 129–165. doi: 10.1016/b978-0-12-800095-3.00005-5
Vandenberg, L. N., Colborn, T., Hayes, T. B., Heindel, J. J., Jacobs, D. R., and Lee, D. H. (2012). Hormones and endocrine-disrupting chemicals: low-dose effects and nonmonotonic dose responses. Endocr. Rev. 33, 378–455. doi: 10.1210/er.2011-1050
Vereecken, H. (2005). Mobility and leaching of glyphosate: a review. Pest Manag. Sci. 61, 1139–1151. doi: 10.1002/ps.1122
Wagner, N., Reichenbecher, W., Teichmann, H., Tappeser, B., and Lötters, S. (2013). Questions concerning the potential impact of glyphosate-based herbicides on amphibians. Environ. Toxicol. Chem. 32, 1688–1700. doi: 10.1002/etc.2268
Wagner, N., Rödder, D., Brühl, C. A., Veith, M., Lenhardt, P. P., and Lötters, S. (2014). Evaluating the risk of pesticide exposure for amphibian species listed in Annex II of the European Union Habitats Directive. Biol. Conserv. 176, 64–70. doi: 10.1016/j.biocon.2014.05.014
Williams, B. K., and Semlitsch, R. D. (2010). Larval responses of three Midwestern anurans to chronic, low-dose exposures of four herbicides. Arch. Environ. Contam. Toxicol. 58, 819–827. doi: 10.1007/s00244-009-9390-z
Keywords: agrochemicals, Roundup, climate change, tadpole, pesticide, non-target effects, amphibia, Bufo bufo
Citation: Baier F, Jedinger M, Gruber E and Zaller JG (2016) Temperature-Dependence of Glyphosate-Based Herbicide's Effects on Egg and Tadpole Growth of Common Toads. Front. Environ. Sci. 4:51. doi: 10.3389/fenvs.2016.00051
Received: 16 May 2016; Accepted: 19 July 2016;
Published: 24 August 2016.
Edited by:
Pankaj Kumar Arora, M. J. P. Rohilkhand University, IndiaReviewed by:
Astrid Rita Taylor, Swedish University of Agricultural Sciences, SwedenFernando José Cebola Lidon, Universidade Nova de Lisboa, Portugal
Copyright © 2016 Baier, Jedinger, Gruber and Zaller. This is an open-access article distributed under the terms of the Creative Commons Attribution License (CC BY). The use, distribution or reproduction in other forums is permitted, provided the original author(s) or licensor are credited and that the original publication in this journal is cited, in accordance with accepted academic practice. No use, distribution or reproduction is permitted which does not comply with these terms.
*Correspondence: Johann G. Zaller, johann.zaller@boku.ac.at