- 1UMR ECOSYS, AgroParisTech-Institut National de la Recherche Agronomique, Université Paris-Saclay, Thiverval-Grignon, France
- 2Research Department, FPS Finance, Brussels, Belgium
- 3Department of Economics, Rensselaer Polytechnic Institute, Troy, NY, USA
Over the last few years, considerable attention has been devoted in the scientific literature and in the media to the concept of “ecosystem” services of soils. The monetary valuation of these services, demanded by many governments and international agencies, is often depicted as a necessary condition for the preservation of the natural capital that soils represent. This focus on soil services is framed in the context of a general interest in ecosystem services that allegedly started in 1997, and took off in earnest after 2005. The careful analysis of the literature proposed in this article shows that, in fact, interest in the multifunctionality of soils emerged already in the mid-60s, at a time when hundreds of researchers worldwide were trying, and largely failing, to figure out how to put price tags meaningfully on “nature's services.” Soil scientists, since, have tried to better understand various functions/services of soils, as well as their possible relation with key soil characteristics, like biodiversity. They have also tried to make progress on the challenging quantification of soil functions/services. However, researchers have manifested very little interest in monetary valuation, undoubtedly in part because it is not clear what economic and financial markets might do with prices of soil functions/services, even if we could somehow come up with such numbers, and because there is no assurance at all, based on neoclassical economic theory, that markets would manage soil resources optimally. Instead of monetary valuation, focus in the literature has been put on decision-making methods that, among other features, do not require the systematic monetization of soil functions/services. Bayesian Belief Networks (BBNs) have the added advantage that they allow the effect of parameter uncertainties to be accounted for, whereas Multi-Criteria Decision Analysis (MCDA) methods easily accommodate deliberative approaches involving a variety of stakeholders. A prerequisite to progress in such public deliberations is that participants be very cognizant of the extreme relevance of soils to many aspects of their daily life. We argue that, as long as this prerequisite is satisfied, the combination of deliberative decision-making methods and of a sound scientific approach to the quantification of soil functions/services (including uncertainties) is a very promising avenue to manage effectively and ethically the priceless heritage that soils constitute.
“The economic function is but one of many vital functions of land. It invests man's life with stability; it is the site of his habitation; it is a condition of his physical safety; it is the landscape and the seasons. We might as well imagine his being born without hands and feet as carrying on his life without land.”
Karl Polanyi (Polanyi, 1944), p.187)
Introduction
Not a day goes by at the moment, it seems, without the publication of some book, one or more editorials, or a flurry of scholarly journal articles referring in one way or another to the many services that soils provide to human populations. The field is quickly acquiring sizeable momentum, and references to the “ecosystem” services of soils are rapidly becoming mandatory indicators of real-world relevance in journal articles or grant proposals. Many international organizations, like the UNEP, as well as governmental agencies in numerous countries, e.g., DEFRA in the UK and EPA in the USA, are increasingly adopting the “ecosystem services framework” (ESF) (Turner and Daily, 2008) as the de facto standard vantage point from which to envisage the conservation of soils and their sustainable use. In recent years, the EU has financed a number of large-scale research and extension projects, including SoilTrEC (Banwart, 2011), SOIL SERVICE, and EcoFINDERS, which devote considerable attention to soil ecosystem services. Undoubtedly, the flurry of activities centered on soils, stemming from the decision of the United Nations to make 2015 the “International Year of Soils,” is fueling this movement even further.
This interest in the services provided by soils is part of a much broader effort to integrate the whole of nature into economic activities. Underlying this larger movement, at its onset in the late 1940s and 50s, was the belief that environmental deterioration, e.g., the loss of habitats and biological species, as well as the depletion of natural resources, stemmed from the fact that, in large measure, the services provided by natural systems had no readily identifiable monetary value and were therefore entirely overlooked in economic and financial transactions. From this perspective, a logical solution consisted of clearly identifying and ranking these services, estimating their values, and translating them into monetary amounts, which could ultimately be used by the financial sector to set up payment or compensation schemes (Boeraeve et al., 2015; Helm, 2015). After a period of enthusiasm for the approach in the 60s and early 70s, during which researchers and practitioners faced, and generally could not resolve, a number of practical challenges, the monetization of nature's services largely disappeared from the radar screen until the mid-90s, when a favorable political climate in the US brought it back into the limelight under the terminology of “ecosystem services.” The field got a major boost after the publication of the Millennium Ecosystem Assessment (2005), and has experienced phenomenal growth since.
The step-by-step process leading from the identification of ecosystem services to their monetization is generally referred to as the “ESF” (Turner and Daily, 2008). Its application to soils has attracted increasing attention from soil scientists over the last two decades. The first step of this framework, involving the cataloging and classification of the many services provided by soils, has been the object of a sustained publication stream. However, the transition to the next step, in which values, and eventually monetary values, are supposed to be assigned to the various services identified, appears to progress extremely slowly. Only a handful of articles to date have attempted to associate any kind of price tag to soil services (Dominati et al., 2010b, 2016; Adhikari and Nadella, 2011; Robinson et al., 2014). All signs are, instead, that soil researchers are actively trying to migrate toward other approaches that do not require monetization, at least not of each and every soil service. Some even advocate methods that avoid the direct valuation of soil services altogether (e.g., Teshome et al., 2014).
In this context, the purpose of the present article is to document this evolution of the research on the ecosystem services of soils over the last two decades, and to critically analyze the key reasons that seem to have motivated its general direction. To frame this analysis properly, it is useful to first recognize that uses of soils by human populations go back to the night of times, and that researchers acknowledged the multi-functionality of soils several decades before the notion of their “ecosystem services” ever surfaced.
Soil Uses in Historical Perspective
When the topic of human populations' dependence on soils is brought up, the event that is most often mentioned is the transition from hunting-gathering to agriculture, about 11,000 years ago (McNeill and Winiwarter, 2004). Yet, there is solid archeological evidence that, in numerous other ways, humans used soils, and materials like clays that are found in soils, already thousands of years before the onset of agriculture. Cave paintings in Spain and Southern France, which have subsisted to this day, were made more than 40,000 years ago with earth pigments, including different types of ochre (iron oxide). Twenty thousand years ago, plastic clay scooped from riverbanks or clay beds was routinely formed into various shapes representing fertility goddesses and other figurines (Salisbury, 2012). At about the same time agriculture started, during the 10th millennium B.C.E., the Jomon peoples in Japan seem to have used mud to make the first pottery vessels, and used them for cooking, food preparation, and storage. Also around 10,000 B.C.E., clay shaped into tokens, stored in clay envelopes, was used in Mesopotamia to represent items of trade or payment in records of commercial transactions. A little closer to us, human populations in various parts of the world seem to have discovered around 7000 B.C.E. that soil can be used to make houses, either as daub on twig structures, as rammed earth, or as mud- or fired bricks (Cammas, 2003; Cellauro and Richaud, 2005; Stiglat, 2012). At about the same time, the Chinchorros, in modern-day southern Peru and northern Chile, started wrapping their mummies in clay, and painted them with various earth pigments, mainly iron, manganese, and copper oxides (Sepúlveda et al., 2014).
This long history of soil usage by human populations over thousands of years is well-documented. Nevertheless, in spite of such a protracted usage, a comprehensive outlook on the topic has emerged only recently. Certainly, each of the varied uses of soils has been the object of a sizeable amount of writing over time, but there is very little cross-over from one body of literature to another. For example, none of the many articles and books devoted to rammed-earth construction in the last few centuries (Guillaud, 1997; Cellauro and Richaud, 2005) has dealt even in passing with the fact that using soil to make houses could interfere with other usages. Diderot and D'Alembert (1777–1780) celebrated “Encyclopédie,” besides numerous articles related to agricultural practices, also contains, remarkably, a number of separate entries related to the use of soil in construction (“pisé,” “torchis”), in medicine, or in the production of wool and sugar, yet it offers no synoptic perspective on the different uses of soils.
One could argue that the lack of a comprehensive perspective is due to the fact that until the early to mid-twentieth century, the soil resource was not scarce, and therefore people did not necessarily think of different usages of soils as competing with each other. However, that changed around the 1940s, and concomitantly, a number of mentions of the multifunctionality of the “land” began appearing in the literature. The quotation by Polanyi that starts the present article is an example of one such mention, by a member of the general public (Polanyi was an economist).
Explicit recognition by scientists that soils fulfill many needs of human societies took a little longer to manifest, and is in fact surprisingly recent. Simonson (1966) was apparently the first to discuss in writing the fact that soil resources not only produce food and fiber, but are also important “as construction materials for highways and dams, as foundation for homes, and for waste disposal, to name only a part of the expanding spectrum of uses.” A few years later, in his comprehensive listing of the societal functions of nature, Hueting (1970) included a number of functions that are directly attributable to soils. Shortly thereafter, the Council of Europe (1972) published a soil charter, acknowledging that “soil is a living and dynamic medium [that] supports plant and animal life. It is vital to man's existence as a source of food and raw materials. It is a fundamental part of the biosphere and […] helps regulate the circulation and affects the quality of water. […] Soil may be put to many uses and it is generally exploited according to economic and social necessity. But the use made of it must depend on its properties, its fertility and the socio-economic services [that] it is capable of providing for the world of today and tomorrow.” The same year, Schlichting (1972) introduced the concept of multi-functionality of soils and described how the buffering capacity of soils in different respects is important to a multitude of organisms and to other parts of the landscape.
Categories of Soil Functions
In the 70s and 80s, various soil scientists described several of the traditional functions of soils (Vittoria and Goldberg, 1975; Schroeder and Lamp, 1976; Warkentin and Fletcher, 1977), evincing that the topic was on the radar screen of the soil science community. However, Brümmer (1978) appears to have been the first to propose a classification of soil functions, followed a few years later by Várallyay (1987, 1989). In these texts, the concept of soil “function” is not defined explicitly. The authors seem to consider that there is a wide enough understanding of what the term means, not to require a formal definition. Yet, it is clear that they refer to soil functions as benefits that are derived from soils, not just by human populations, but more generally also by plants and animals.
In 1988, Blum (1988) organized soil functions into five categories, two of which he labeled respectively “socio-economic” and “technical-industrial,” and the remaining three he termed “ecological” (Figure 1). In this classification, as in the previous literature on the same topic, soil functions implicitly encompass uses of soils by human populations as well as by animals and plants. As suggested in Figure 1, the portion of the subsurface that is referred to as “soil” in this context can be very deep, extending past the root zone of tall trees, all the way down to aquifers and even including them, as long as the porous material in which these aquifers are located is made of loose particles. This is in sharp contrast with the traditional, much shallower object of study of pedologists (e.g., Brevik and Arnold, 2015), and, consequently, one should not assume that typical soil maps be necessarily relevant to all soil functions, as will be stressed later on.
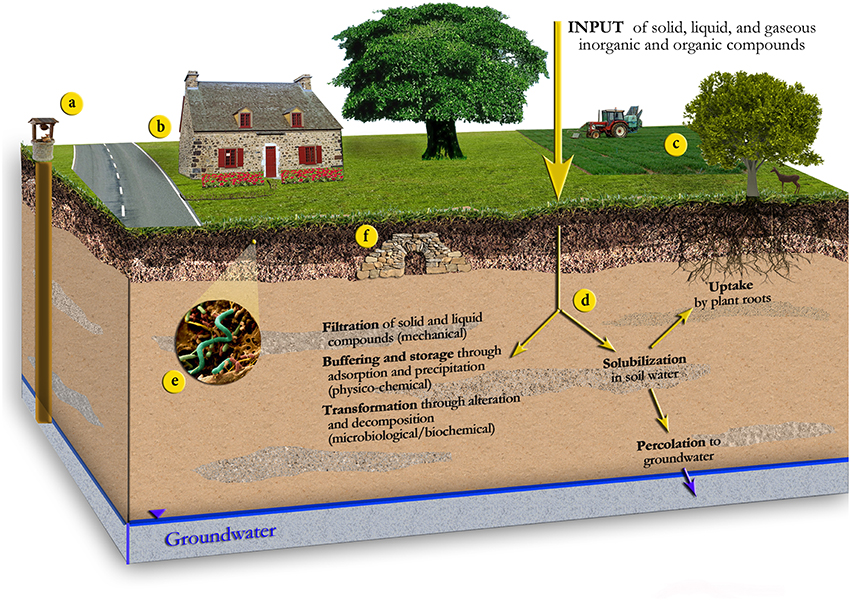
Figure 1. Schematic illustration of the different functions of soils according to Blum's (1988) classification. The six categories of soil functions correspond, respectively, to (a) the extraction of raw materials and water, (b) physically supporting buildings and other man-made structures, (c) the production of biomass, (d) filtration, buffering, storage, and chemical/biochemical transformations, and (e) the preservation of biodiversity or potentially useful genetic material, as well as of geogenic and cultural heritage. (Original drawing by P. Baveye).
The first category of functions, referred to as “socio-economic” (“a” in Figure 1), involves the supply, by soils, of water and of different kinds of raw materials such as clay, sand, gravel, or coal, that are used in a wide range of building, industrial, and manufacturing operations. Many of these functions of soils, by and large, have continued unabated over the millennia and are still relevant today. For example, some authors estimate that as much as half of the world's population currently still live in houses made of dried clay or mud (see, e.g., http://www.motherearthnews.com/fair/2014-washington-building-with-mud.aspx). If one counts fired bricks, the proportion of people whose houses are made of soil material might even be higher (Staubach, 2005). The “technical-industrial” functions (“b” in Figure 1) relate to the fact that soils, generally after being sealed in some way, serve as structural supports for many different types of buildings, roads, sport facilities, as well as landfills. The first of the ecological functions of soils (“c” in Figure 1) relates to the production of biomass, e.g., crops on agricultural fields, or trees in forests. Soils serve as physical support for roots and as a nutritive substrate, supplying air, water, and nutrients required for plant growth. The second ecological function (“d” in Figure 1) relates to the fact that soils are important filters of chemical or biological contaminants, as physical buffers in the global water cycle, and as a medium that fosters biological/biochemical transformations of toxic organic compounds. Finally, the third ecological function in Blum's (1988) classification (“e” in Figure 1) involves the preservation of genetic diversity, in particular the preservation of a myriad of genes that could be of potential use to humans, for example for the production of new types of antibiotics. In addition, this third ecological function also encompasses the preservation of paleontological and archeological treasures of high value for the understanding of the history of human populations and of the earth. In the last few years, Blum's (1988) classification has evolved somewhat, by the morphing of the cultural and historical aspects of the third ecological function into a third “non-ecological” function, labeled “geogenic and cultural heritage” (Blum, 2005; Blum et al., 2006). Also, an additional ecological function has been added, involving the increasingly crucial rôle of soils as carbon pools, in relation especially to global climate change.
The juxtaposition of different functions of soils in Figure 1 helps to visualize several of their fundamental features, in particular their interdependence. Indeed, it is clear from that diagram that the fulfillment of a function by a soil will, in general, affect the extent to which it is capable of fulfilling others. For example, on a given piece of land, a farmer's decision to change the land use or its management, e.g., by planting a forest instead of having a field or a pasture, on which livestock feeds (Renison et al., 2010), or by switching from industrial agricultural practices to a form of conservation agriculture or organic farming, will likely have a marked effect on the percolation of water down the soil profile, which in turn will affect the recharge of groundwater and/or the filtration of chemicals. Another, more extreme, example, is if a soil is covered with an impervious surface, as in a parking lot. In this case, several other services, including the soil reserve of genetic material, are more than likely to be severely decreased, if not virtually eliminated. This interdependence of functions is a key property to keep in mind in later discussions about their measurement and evaluation, or when envisaging trade-offs and possible complementarities among functions/services (Reed et al., 2013; Setala et al., 2014).
As detailed as Blum's classification is already, it is clear in hindsight that it does not include a number of soil processes that we now acknowledge to be important. For example, not all transformations are microbiological/biochemical; some may be entirely catalyzed abiotically by the solid phase. Nor do all physico-chemical reactions reduce to sorption or precipitation; additional reactions include hydrolysis, hydration, clay formation, and transformation. The infiltration of water into soil is significant not just in terms of its filtration and ultimate recharge of groundwater or uptake by plants, but also because water that infiltrates does not runoff above ground. This function is extremely significant in relation with the hydrology of the regions in which soils are located, and in particular in terms of the response of watersheds to precipitation events and of associated risks of flooding downstream (Lehmann and Stahr, 2010). Blum's classification also includes no other “out” arrows besides those related to plant uptake and percolation to groundwater. Yet, we know that the release of massive amounts of CO2, methane, and other greenhouse gases by soils is a very significant process, with (negative) planetary consequences that threaten the well-being of human populations. Because of that, “climate regulation” has been added to a more recent classification and graphical depiction of soil functions (Figure 2), along with carbon sequestration, which has a potentially positive influence on climate change. In addition to these omissions, Blum's classification also does not list spiritual, affective, functions of soils. Nor does it mention the kind of aesthetic appeal that Daily et al. (1997) are alluding to, when they write lyrically about “breathtaking landscapes that have captured the imagination of artists for centuries.”
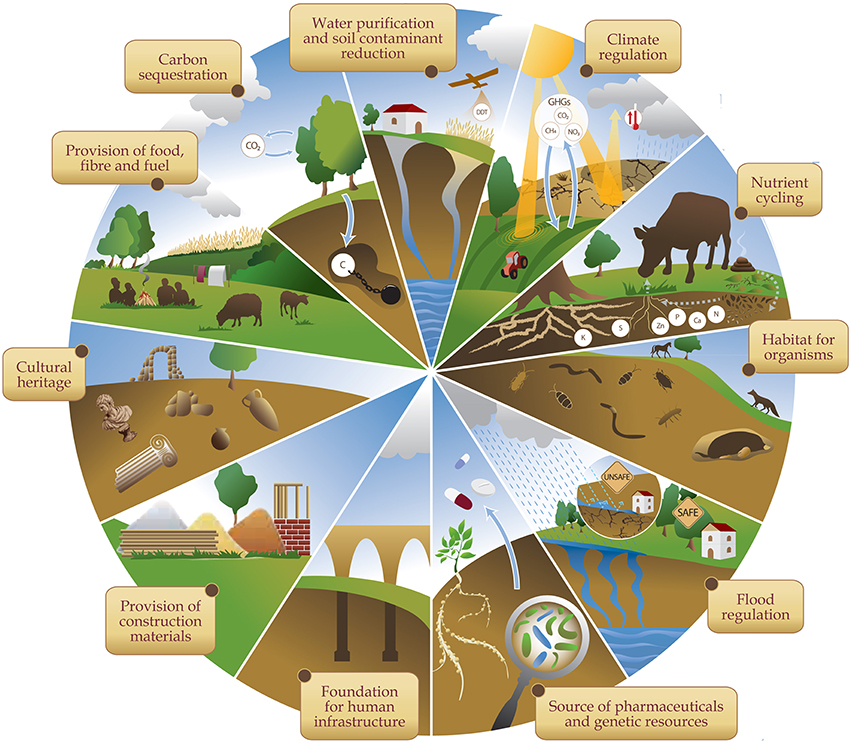
Figure 2. Schematic diagram of soil functions. This diagram is part of an “infographics” on soil functions put together by the FAO, with the subtitle “Soils deliver ecosystem services that enable life on earth” (Adapted from http://www.fao.org/resources/infographics/infographics-details/en/c/284478/).
In spite of its relative incompleteness (at least, with the benefit of hindsight), Blum's (1988) classification of soil functions has been very influential in policy-making circles in Europe, where it has served in the 90s as a conceptual foundation for several soil protection legislations, e.g., in Germany (Lehmann and Stahr, 2010; Liang et al., 2014) and England (Tzilivakis et al., 2005), as well as at the broader scale of the EU (Blum, 1990, 1993; Glaesner et al., 2014). A “Thematic Strategy for Soil Protection” (Blum et al., 2004; Rodrigues et al., 2009), published in Brussels in 2002 and based on a classification of soil functions inspired by Blum's, led a few years later to a proposal by the European Communities of a “Soil Directive” (Commission of the European Communities, 2006), which has been under negotiation among EU member countries between 2006 and 2014. Its key objective was “the establishment of a common framework to protect soil on the basis of the principles of preservation of soil functions, prevention of soil degradation, mitigation of its effects, [and] restoration of degraded soils” (Commission of the European Communities, 2006). In other parts of the world as well, like China (Liang et al., 2014), and at international agencies like the Food and Agriculture Organization (Figure 2), the multi-functionality of soils has progressively become a key feature in policy-making related to land use planning (Schulte et al., 2014).
This sustained interest has created a demand for detailed scientific information on soil functions, and have given rise to a steady stream of publications on the subject over the years (e.g., Kovda et al., 1990; Lavelle et al., 1995; Lavelle, 1996, 2000; Karl, 1997; Schouten et al., 1997; Rampazzo et al., 1998; Yaalon and Arnold, 2000; Barancikova and Madaras, 2003; Calvet, 2003; Blume et al., 2010, 2016; Buchan, 2010; Bujnovsky and Vilcek, 2012; Glenk et al., 2012; Madena et al., 2012; Morel et al., 2014; O'sullivan et al., 2015). In particular, soil functions have become a cornerstone in the long-standing debate on the somewhat vague concepts of “soil quality” and “soil health,” various authors arguing for the existence of absolute values of the notions for any given soil, whereas a growing number of researchers consider that their evaluation makes sense only in relation to specific soil functions (e.g., Sojka and Upchurch, 1999; Bouma, 2002, 2010, 2014; Nortcliff, 2002; Letey et al., 2003; Robert, 2005; Kibblewhite et al., 2008; Keller et al., 2012; Thomsen et al., 2012; Volchko et al., 2014).
Early Interest of Ecologists in Nature's Services
At about the same time soil scientists started to classify and investigate the different functions of soils, several ecologists, and ecological economists, predominantly in the US, also dealt with the concept of function, although they did so from another vantage point, developing an alternative terminology, and generally adopting a different perspective on human populations' relationship to nature.
The background of some of this work was provided by a movement in the 40s and 50s, concerned that if no monetary value could be associated with, e.g., national parks and nature conservation in general, economic and financial markets would entirely ignore the subject and take decisions that would be damaging to the environment. This perspective led to the development of a number of practical valuation methods. In particular, Hotelling's (1949) discussion of use of travel costs to estimate the value of parks stimulated the development of several revealed preference approaches, like the travel cost valuation method, formally proposed by Clawson (1959) a decade later, and hedonic pricing methods (Ridker and Henning, 1967). Similarly, suggestions by Ciriacy-Wantrup (1947) eventually led to the use of stated preference techniques, like contingent valuation (Davis, 1963). Krutilla (1967) specifically focused on values associated with cultural services of nature.
In the wake of such methodological developments, a very large body of work was carried out in the 60s and 70s on what was at the time referred to as “ecosystem functions” (Odum, 1959), “environmental goods and services” (Vatn and Bromley, 1994), or “environmental amenities” (Adamowicz, 1991). The Williamstown Study of Critical Environmental Problems (SCEP, 1970), generally credited to be one of the starting points of this movement, listed a number of “environmental services” like pollination, fisheries, climate regulation, flood control, and soil erosion prevention. In a comprehensive review of the state of the art of evaluating intangible benefits and costs associated with the use of the environment, Coomber and Biswas (1973) list around 300 articles, books, and reports. A few years later, an extensive annotated bibliography assembled by Leitch and Scott (1977) comprises no < 691 articles, reports, theses, and other publications, dealing solely with the economic values of fish and wildlife and their habitats.
These early attempts to value nature were quickly followed by detailed analyses of the shortcomings of the approaches used. In a seminal article on “nature's services,” which has been seldom cited (compared in particular to the work of Costanza and Daily) but nevertheless appears in retrospect to be one of the most thoughtful articles on the topic, Westman (1977) analyzed several of these services in detail, including the ability of soils to absorb air pollutants, which he viewed as a useful example of “service of an ecosystem.” He also inquired about the possibilities there might be to associate monetary values with nature's services, and he came to conclusions that were not optimistic in this regard. He expressed the view that it is both “sobering and important to recognize” that, even in the long run, quantitative estimates of the worth of nature to man are likely to be akin to estimates of the worth of a flower to a poet: “What is the value to societies, present and future, of the inspirations that flowed from Wordsworth's poetry, and indirectly from nature?”
Be it or not a consequence of Westman's reservations and those of other authors who have expressed similar views (Hines, 1991), the question of the valuation of nature's services received little further attention during the following two decades. Nevertheless, the terminology evolved somewhat, and eventually denoted a stronger emphasis than had been the case until then on ecosystems as the most appropriate perspective from which to envisage nature. Two ecologists (Ehrlich and Ehrlich, 1981) coined the now emblematic expression of “ecosystem services” in a book concerned with the “dependence of human civilization on the services provided by ecosystems” and illustrated the concept with a number of examples, including a few involving soils, but they did not propose a comprehensive analysis of the variety of ecosystem services of soils. A few authors adopted the notion of ecosystem services afterwards (Cairns and Niederlehner, 1994; Brown and MacLeod, 1996), but for a while, the field failed to acquire any kind of momentum.
Development of the Ecosystem Services Framework
Quite a few years after the introduction of the concept of ecosystem services, it was not any particular breakthrough in science, but politics in Washington that propelled the topic to prominence and led to the elaboration of the ESF (Baveye, 2014). Soon after Republicans gained control of both the US house and senate in 1994 for the first time in more than 40 years, what by all accounts appears like a frontal neoliberal attack was launched on environmental regulations of all kinds. Shortly thereafter, the President's Council on Sustainable Development, a high-level stakeholder advisory committee assembled by President Bill Clinton, produced a consensus report (President's Council for Sustainable Development, 1996) recommending a reliance on market forces in environmental policy and in the drive to sustainable development. The 170-page report is predicated on the belief, among others, that “[e]conomic growth based on technological innovation, improved efficiency, and expanding global markets is essential for progress toward greater prosperity, equity, and environmental quality.” The text of the report itself is replete with references to “market-based” or “market-related” incentives and mechanisms to better manage environmental issues, as well as encouragements for businesses to couch “their environmental strategies in the financial terms that Wall Street can understand and reward.”
Even though none of the doubts expressed eloquently almost 2 decades earlier by Westman (1977) had been adequately answered by then, the new political climate in Washington provided impetus to some researchers to suggest once again that ecosystem services should be evaluated “in terms comparable to economic services and manufactured capital” (Costanza et al., 1997). Several workshops and conferences took place, like the workshop on “Human activity and ecosystem function: Reconciling economics and ecology,” organized by the Renewable Natural Resources Foundation in 1995, where the concepts of ecosystem functions and services, as well as their valuation, were often hotly debated.
The books (e.g., Simpson and Christensen, 1997) and numerous articles (Cairns and Niederlehner, 1994; Ryszkowski, 1995; Toman, 1998) that resulted directly or indirectly from these meetings show clearly that consensus among researchers in the field could not be reached on the merits of an economic approach to ecosystem services or on the definition of the various concepts involved (Fisher et al., 2009). In particular, ecosystem services were defined in very different ways, alternatively as the “conditions and processes associated with natural ecosystems that confer some benefits to humanity” (van Wilgen et al., 1996; Daily, 1997), or as the “benefits human populations derive, directly or indirectly, from ecosystem functions,” where ecosystem functions refer “variously to the habitat, biological or system properties or processes of ecosystems” (Costanza et al., 1997). In the first definition, services encompass functions and processes, in the second they do not. As a result of this dissonance, agreement remained elusive as well on suitable methods to associate prices with ecosystem services in a meaningful way.
A consensus, at least on the definition of the key terms, was reached almost a decade later, as part of the massive international effort of the Millenium Ecosystem Assessment (MEA), a multi-year, multi-million dollar international undertaking involving 1300 scientists from around the world to assess the consequences of ecosystem change, and consequent alterations in the flow of ecosystem services. Some have argued that U.S. researchers influenced other participants significantly in the negotiations leading to the final MEA report (Pesche, 2013). Nevertheless, in this report, ecosystem services are defined as the “benefits people obtain from ecosystems.” Aside from clarifying the meaning of this terminology, the MEA, largely inspired by a number of earlier attempts (de Groot et al., 2002), also proposed a clear nomenclature for ecosystem services that rapidly became the norm in the field. It distinguishes among four broad categories of ecosystem services (Figure 3): supporting services that are necessary for the production of all other ecosystem services, provisioning services encompassing all products obtained from ecosystems, regulating services including all the benefits obtained from the regulation of ecosystem processes, and, finally, a “potpourri of intangible benefits” (Orenstein, 2013) or “intangible dimensions” (Setten et al., 2012), referred to as “cultural” services and involving the nonmaterial benefits people obtain from ecosystems through spiritual enrichment, cognitive development, reflection, recreation, and aesthetic experiences (Chan et al., 2012a,b; Milcu et al., 2013).
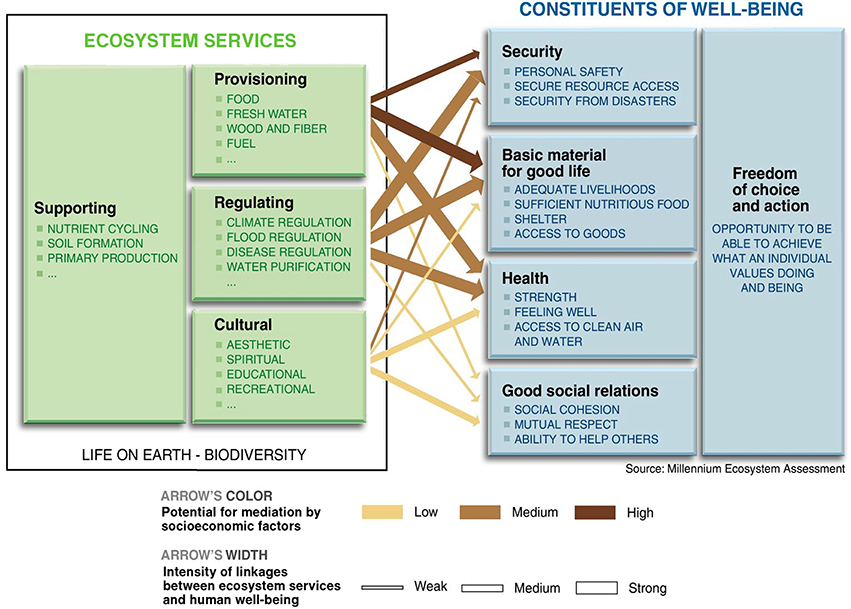
Figure 3. Ecosystem services as defined by the Millenium Ecosystem Assessment (Modified from MEA, 2005).
Divergences of opinion about what constitutes a suitable classification of ecosystem services did not die down completely after the publication of the MEA (e.g., Boyd and Banzhaf, 2007; Wallace, 2007; Costanza, 2008), and various proposals were made to introduce changes. In particular, another international committee, “The Economics of Ecosystems and Biodiversity” (TEEB), suggested a number of revisions to the MEA classification in order to synthesize recent research and prevent double counting in ecosystem services audits (Kumar, 2010; Kumar et al., 2013). The category of “Supporting Services” was removed and split into “Habitat Services,” and “Ecosystem Functions,” the latter defined “as a subset of the interactions between ecosystem structure and processes that underpin the capacity of an ecosystem to provide goods and services.” The most recent attempt to standardize the definition of ecosystem services has resulted from work on environmental accounting undertaken by the European Environment Agency (EEA). Developed since 2009 and known as the “Common International Classification of Ecosystem Services” (CICES, website: http://cices.eu), this latest scheme involves three main categories of ecosystem services (provisioning, regulation/maintenance, and cultural). Its classification relies on a five-level hierarchical structure proposed as a way to handle some of the challenges that arise in relation to the specific spatial and thematic scales used in different applications. This hierarchy consists, from the top down, of sections, divisions, groups, classes, and class types.
Soils Initially Left Out of the Ecosystem Services Framework
In the soil functions framework, no matter how large of a geographical area is considered, the soil is viewed as a complete system in itself, with a high degree of spatial heterogeneity, open to, and interacting in complex ways with, its surroundings. By contrast, the ESF apprehends nature a single ecosystem (e.g., wetland, tundra, forest, estuary) at a time, or as a collection or mosaic of juxtaposed ecosystems, as is also the case in many landscape management practices (Burkhard et al., 2009; Willemen et al., 2012; van Wensem, 2013; Hodder et al., 2014).
O'Neill (2001) argues that, in ecology, such ecosystems have often tended to be viewed tacitly as stable, closed, spatially homogeneous, and self-regulating systems to which humans are considered external (except in the special case of agroecosystems). Ecological methods developed to analyze the properties of ecosystems have been adapted to systems that meet these basic assumptions, and at a very practical level, the ecosystem perspective presents the advantage that approximate information about the spatial distribution of specific ecosystems over a landscape can often be obtained from remotely-sensed (e.g., satellite-based) observations. In some very unique cases, the assumption of a mosaic of stable environments may be a good approximation of reality, as in the traditional satoyama landscape systems in Japan, whose distinct environments (e.g., water bodies, woodlands, paddy fields) have often been maintained and managed sustainably for many centuries (Takeuchi, 2003), and therefore have reached a virtual steady state. However, in the overwhelming majority of situations, the applicability of the traditional ecosystem construct has been the focus of intense skepticism. Indeed, over the last 2 decades, it has been increasingly criticized from different directions, and many authors have argued that it cannot be reconciled with the current understanding of ecological systems as metastable adaptive systems that usually operate far from equilibrium (Blandin and Bergandi, 2000; O'Neill, 2001; Gignoux et al., 2012; Tassin, 2012; Silvertown, 2015).
Nevertheless, the implicit focus on the traditional ecosystem paradigm within the ESF has led to significant simplifications. Among others, it has rendered unnecessary an explicit account of the response of ecosystem components or subsystems. Indeed, from this viewpoint, one can refer to the role played, e.g., by forest ecosystems in nutrient cycling or in water retention, without necessarily having to break it down into the contributions of the various components of forests. Sometimes, the focus is on “biomes,” i.e., on biotic communities (Costanza et al., 1997; Palm et al., 2007), with abiotic components, the climate and soils among them (Binkley, 2006) acting as shaping factors of the biome. This emphasis on the biotic side of things is so strong in some cases that in terms of provisioning services, various researchers have argued that for the provision of raw materials to be considered an ecosystem service, the raw materials in question have to be renewable and biotic (e.g., de Groot et al., 2002; Dominati E. et al., 2014; McBratney et al., 2014); non-renewable, abiotic resources like sand or clay cannot be included.
In this overall context, it is hardly surprising that until just a few years ago, articles on ecosystem services tended to devote very little attention to soils, and insofar as they did, they often left out many of their common uses. For example, in their highly controversial attempt to estimate the total monetary value of all ecosystem services on earth, Costanza et al. (1997) listed 17 ecosystem services emanating from 16 primary categories of biomes, but only two of these ecosystem services (“erosion control and sediment retention” and “soil formation”) refer to soils explicitly. Worse yet, “cropland” biomes, in which one would expect soils to play significant roles, are considered not to provide at all a number of functions or services usually associated with soils, like gas-, climate-, or water regulation, water supply, soil formation, nutrient cycling, raw materials, genetic resources, recreation, or cultural services. Instead, croplands are assumed to supply services associated only with pollination, biological control, and food production. Even in this very limited context, Costanza et al. (1997) apparently did not deem it necessary to account explicitly for the role of soils in the provision of any of these services. Neither did Costanza et al. (2014) in a recent update of the 1997 study.
Similarly, 8 years later, the final MEA report did not mention soils very much either (Giger, 2006; Dominati et al., 2010b). It adopted from the onset a large-scale ecosystem approach and envisaged all environmental issues from that vantage point. The focus was on the status and trends of the services provided by a selected number of ecosystems. The soil component in those ecosystems received little or no attention. Soil issues were taken up mainly in relation to the assessment of nutrient cycles, soil formation, erosion regulation, water regulation, and natural hazard regulation. Again, services provided by soils in terms of, e.g., raw materials extraction, spiritual, or aesthetic aspects, or genetic resources, were neglected. This generally low visibility of soils was maintained in later classification efforts. For example in the CICES, soils are mentioned under regulation/maintenance at the group level (3rd level down in the 5-level hierarchy) in connection with soil formation and composition; at the class type level (lowest level of the hierarchy) in relation to biomass provisioning and the mediation of wastes, toxics, and other nuisances; and finally as an example of a locus for the preservation of cultural heritage.
This quick overview of the literature on the ESF would not be complete without mentioning that a number of authors have opted in recent years to distance themselves from the emphasis on ecosystems, and have preferred to talk about “landscape services” (Frede et al., 2002; Ungaro et al., 2014b) or “services of multi-functional landscapes” (Garcia-Llorente et al., 2012) instead. The key motivations are that process-pattern relationships are better understood at the landscape scale, and that local stakeholders seem to have a more acute perception of the concept of “landscape” than of “ecosystem” (Setten et al., 2012). For the same reason, especially in wine-producing regions of the world, it may make sense to use the common concept of “terroir” (Douguet and O'Connor, 2003; Deloire et al., 2005; Rouvellac, 2008), to which the public relates readily. Like the term of “Landschaft” used in German-speaking countries, “terroir” encompasses both the landscape and the people who inhabit it, and also to some extent the “character of the land” and the bond between nature and people. In terms of areal extent, it is interesting to note that these landscape, terroir, or landschaft concepts, like the ESF, focus on relatively large-scale areas. For example, in their work on landscape services, Ungaro et al. (2014b) consider a region of 576 km2.
Increasing Focus on Soils
Whereas almost all publications on ecosystem services in the period of 1997–2005 adopted a large-scale ecosystem perspective, leading to a relative neglect of soils, a few publications by ecologists and economists are notable exceptions and adopt a different approach. Apparently unaware of Blum's classification, Daily et al. (1997) review in detail a number of services provided by soils, namely buffering and moderation of the hydrological cycle, physical support of plants, retention and delivery of nutrients to plants, disposal of wastes and dead organic matter, renewal of soil fertility, and regulation of major element cycles. Some of these services correspond to soil functions listed by Blum (Figure 1), but a number of the other functions he identified are clearly missing, in particular the technical-industrial functions, and the preservation of genetic diversity or historical artifacts. Also, there is no mention of cultural or spiritual aspects of soils, nor of the effect of soils on global climate change. Also apparently unaware of the work done on soil functions, Pimentel et al. (1997) propose the same year an overview of the economic and environmental benefits of biodiversity. They focus on the “vital services that are provided by all biota (biodiversity), including their genes and biomass, to humans and to the environment,” and they refer explicitly to the role of soil biota in terms of topsoil formation, nitrogen fixation by soil-borne diazotrophic bacteria, and the bioremediation or biotreatment of highly polluted soils. Their emphasis on biological species, however, prevents them from looking at the full range of soil services. Finally, in a later series of articles citing Daily et al. (1997) and discussing the economic valuation of soil functions, Fromm and Bruggemann (1999) distinguish among functions related to “control” (of water and biogeochemical cycles), “habitat” (for microorganisms and fauna), and “production” (of crops), but do not include cultural aspects of soils, climate regulation, carbon sequestration, or the role of soils as reserves of biodiversity, for example.
Starting in 2000, the terminology of ecosystem services slowly made inroads within the soil science literature. Initially, the motivation behind the adoption of the concept by soil scientists was manifestly to take advantage of a topical framework that enjoyed growing popularity and afforded significant funding potential. The intent was to try to boost specific research topics in soil science, e.g., the activity of soil fauna (Lavelle, 2000; Wall and Virginia, 2000; Wall et al., 2004; Lavelle et al., 2006; Barrios, 2007; Ritz et al., 2009), soil organic matter management (Collard and Zammit, 2006), or health issues associated with acid sulfate soils (Ljung et al., 2009). Given the appeal that, by then, ecosystem services had for governmental agencies in various countries, even a cursory mention of related buzzwords in one's writing was indeed, and still is, perceived likely to give it the appearance of societal relevance and legitimacy (Cardona, 2012; Hellec et al., 2015). However, in all of these early contributions to the soil science literature, and in those, more recent, that appear to still be inspired by the same mindset (Haygarth and Ritz, 2009; Mulder et al., 2011; Brussaard, 2012; Brussaard et al., 2012; Foudi, 2012; Hedlund and Harris, 2012; Wall, 2012; Pascual et al., 2015), the framework of ecosystem services seems to be little more than a vague context in which to pursue one's traditional pet research topics, related particularly to the “major groups” of soil fauna (Briones, 2014) or to the fate of soil organic matter (e.g., Lal et al., 2013), and, most frequently, focused on single services (Blouin et al., 2013).
After about 2009, though, a steadily growing number of authors (Robinson et al., 2009; Bennett et al., 2010; Bristow et al., 2010; Dominati et al., 2010a,b; Robinson and Lebron, 2010; Martins and Angers, 2015) manifested increasing interest in determining how a reflection in terms of the services of soils to human populations could help society address a number of pressing questions causing public concern. With few exceptions, this research effort has not been framed at all in the context of the various ecosystems or biomes envisaged by ecologists, or in any landscape context either, but has focused instead exclusively on soils.
Pragmatically, this emphasis makes eminent sense for a number of reasons. Land-based ecosystems are made up of many small pieces of land, each belonging to a different landowner. As pointed out, e.g., by Vejre et al. (2015), “the mismatch between legal units and ecological phenomena” causes practical difficulties when one is trying to deal with the management of many ecosystem services. In particular, if one ever got to the point of paying individual landowners directly for the services provided by their land, it would be necessary to figure out what each parcel or “cadastral unit” of land is contributing, i.e., what each owner is entitled to receive. A relatively simple solution would consist of paying everyone in a given ecological region or agroecosystem the same amount of money. However, that would ignore completely the fact that adjacent pieces of land can have very contrasted properties and therefore capacities to provide services, if only because of differences in topography. To account adequately for the spatial heterogeneity of land qualities, one needs to consider each field or each pasture as a special case, and in that context, it becomes unavoidable to consider soil characteristics explicitly, as farmers do on a daily basis.
Similarly, if one is trying to determine within a given region where it would be the least ecologically damaging to build a shopping mall and the vast expense of parking lots that are typically associated with it, again the problem focuses on individual parcels of land, and the analysis of ecosystem services once more becomes local in nature. In some cases, the scale is so local that the soil compartment is overwhelming (Figure 4). In these situations, it is not meaningful to deal with changes in land use as a transition from one broad ecosystem to another, and an approach centered on the services rendered directly by soils is expected to be far more helpful.
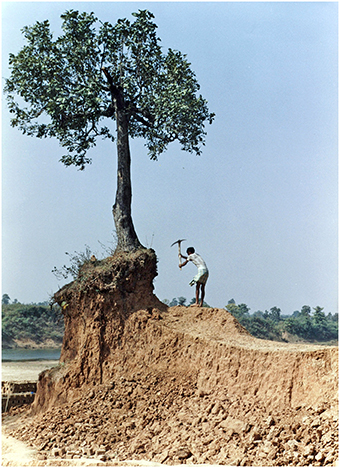
Figure 4. Illustration of a local situation involving competing soil uses, where reasoning in terms of a transition from one traditional type of stable, large-scale ecosystem to another appears ill-adapted (Modified from http://www.unep.org/depi/Ecosystemservicesandeconomics/tabid/6389/Default.aspx).
This question of local-vs. broad scale is not the only reason for privileging an approach that gives soils more visibility than in the traditional ESF. Under some circumstances, services provided by nature may depend critically on highly dynamic properties of soils, and these therefore need to be accounted for explicitly if one wants to describe and predict nature's services adequately. For example, the recharge of aquifers used for drinking water supply or the availability of water to crop roots may be severely impacted by the (sometimes very rapid) formation of a crust within the first few millimeters below the soil surface, which makes soils virtually impermeable, and increases surface runoff dramatically (Valentin and Bresson, 1992; Bielders and Baveye, 1995; Bielders et al., 1996). In such instances, a broad-scale ecosystem perspective that does not account explicitly for soil processes is likely to prove unable to describe adequately changes that may occur over time in terms of the services that nature provides in a given region.
“Ecosystem” Services of Soils: A Restrictive Misnomer?
In this context of a focus squarely on soils, one might ask whether the label of “ecosystem” still makes sense. Within the soil science literature, the concept of ecosystem is as controversial as it is in ecology. While references are frequently made in the literature to “soil ecosystems” (Fenton, 1947; Nahmani et al., 2003; Barrett et al., 2008), some authors (di Castri, 1970) argue that the assumptions of stability, closedness, and homogeneity traditionally associated with ecosystems does not seem apt at generating new insight about the nature of the multitude of dynamic processes that occur in these systems, nor about the services provided by soils to humans.
Aside from these conceptual issues, one key objection to the label of “ecosystem” associated with the services of soils is that not all of these services involve plants or organisms. The most obvious in that respect are services grouped under “a” and “b” in Figure 1. When a soil is exploited as a source of sand or clay, or when one is interested in water extraction from aquifers for irrigation purposes, the presence of living organisms in the soil is irrelevant. In terms of the transformation of chemicals in the subsurface, (“d” in Figure 1), exclusion of strictly abiotic transformations (e.g., degradation of pesticides by clay minerals) would be unacceptable in many cases. Therefore, for practical purposes, the expressions of soil ecosystem services or even “ecosystem services supplied by soils” seem unnecessarily restrictive. Even if, as some authors have started doing in oral presentations, one talks instead of the “contributions of soils to ecosystem services,” there is still a risk of restricting the range of contributions that are considered, depending on whether or not organisms are involved.
In this context, one could argue that it makes sense to drop the “ecosystem” qualifier entirely, and to simply refer to the “services of soils,” or, if one interested in larger scales, to the “contributions of soils to landscape services.” From that viewpoint, one could say that the only difference between the young soil services literature and the older, parallel literature on soil functions, is merely one of terminology, and that the two concepts are in fact equivalent for all practical purposes (Robinson and Lebron, 2010; Cardona, 2012). In numerous documents or websites (like that, at the F.A.O., where the “infographics” of Figure 2 is found), the terms of function and service of soils are indeed used interchangeably. Nevertheless, further examination reveals that things are not quite as simple, that both terms suffer in fact from distinct conceptual drawbacks, that they are not synonymous, and as a matter of fact, that they should both be kept.
Soil Functions or Services, or Both?
One argument occasionally advanced in the ecology literature in favor of the term of service is that it is far less ambiguous or philosophically laden than that of “function.” Indeed, several authors have indicated that the concept of function encompasses a confusing number of distinct meanings, and raises significant philosophical questions (Nagel, 1961; Millikan, 1989). Jax (2005) describes four common acceptions of the term, respectively (1) as a state change in time (more or less synonymous to “process”), (2) as a shorthand notation for “functioning” (referring to some state or trajectory of a given system, and to the sum of the processes that sustain the system), (3) as the specific role of parts of the system in the different processes they are engaged in, and, finally, (4) as a “service” provided to humans and possibly also other living beings (plants or animals). Aside from this multiplicity of meanings, another problem is that, implicit in the term of function itself, is the teleological connotation of a design or purpose associated with natural phenomena, which has led some authors to argue that the concept comes “with a certain amount of philosophical baggage associated with commitments to holism and the normativity of so-called natural or proper functions” (deLaplante and Picasso, 2011).
These issues are not merely academic. Indeed, the use of “function” in the soil science literature in general is utterly confusing. One can find examples of adoption of each of the possible meanings of the term, causing significant communication issues. Many articles associate “function” with specific processes, like cation exchange, which occur in soils. Various articles collectively aggregate the different “functions,” so defined, into its “functioning” (Freckman et al., 1997; Andrén and Balandreau, 1999; Brussaard, 2012; Semenov et al., 2014), or use the term “function” to refer instead, teleologically, to the activity of specific soil organisms or functional groups of organisms (Lavelle, 1996; Brussaard et al., 1997; Brussaard, 2012) as a component of that functioning. This lack of a clear consensus on what “function” means in general in relation to soils might encourage some to avoid using it.
Another relative downside of the concept of function is related to the fact that not all that soils have to offer to human populations is necessarily beneficial to them (Zhang et al., 2007; Lyytimäki et al., 2008; Redford and Adams, 2009; Bennett et al., 2010; Dunn, 2010; Power, 2012; Ango et al., 2014; Shackleton et al., 2016). Significant negative effects of soils on climate change, through the release of greenhouse gases (Burgin et al., 2013), are acknowledged, as is also the fact that, while they supply nutrients to crops, soils also do the same to countless weeds that eventually require the use of large amounts of herbicides to eliminate them. However, other nefarious effects of soils, for example on human populations (Oliver, 1997; Schenker, 2000; de Silva et al., 2003; Herrmann, 2006; Pepper et al., 2009; Steinnes, 2009; Bardgett and van der Putten, 2014), are far less recognized. Yet, they can be very significant, as vividly illustrated by the case of helminthiasis, a macroparasitic disease of humans and animals transmitted partly through soils. Parasitic worms, known as helminths, typically invade the gastrointestinal tract, but may also burrow into other organs, like the eyes or the brain. Estimates are that more than a billion people in developing countries are hosting at least one species of parasitic worm, leading to numerous health problems and, in many cases, eventually to death. Attempts to control the spread of helminthiasis have relied largely on preventive mass chemotherapeutic treatment of school-age children, who are the highest risk group (Humphries et al., 2012; Figure 5). In recent years, after being neglected for several decades, other health-related disservices of soils have also begun to attract considerable attention, notably in connection with neurodegenerative diseases (Charlet et al., 2012) or the worrisome spread of antibiotic-resistant microbial pathogens (Wellington et al., 2013).
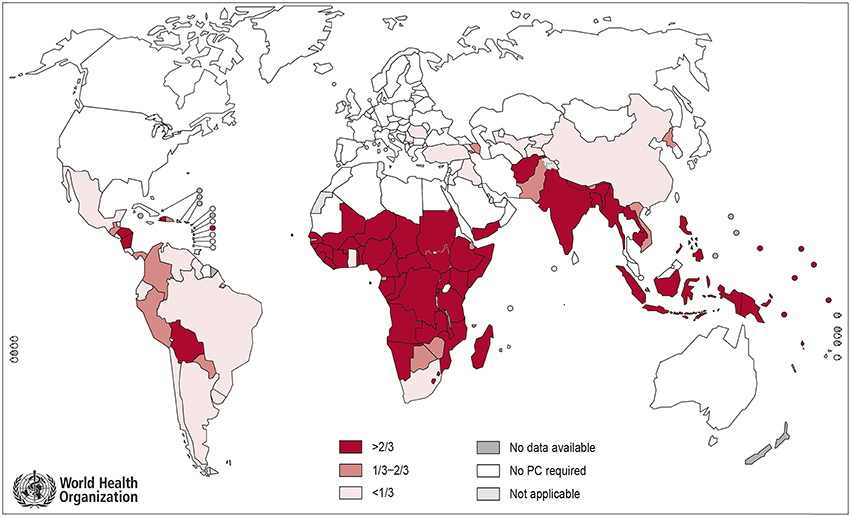
Figure 5. Proportion of children (1–14 years of age) requiring preventive chemotherapy (PC) for soil-transmitted helminthiases, on a per-country basis in 2011. Adapted from World Health Organization, program on Control of Neglected Tropical Diseases (http://gamapserver.who.int/mapLibrary/Files/Maps/STH_2011_global.png).
Unfortunately, the concept of function is ill-equipped to describe these types of negative effects. Indeed, it so intrinsically implies a beneficial outcome that the expression “negative function” seems very awkward. For the same reason, the terms “dysfunction” and “malfunction” convey the meaning of improper functioning of a system, rather than that of negative effects on potential end-users. By contrast, the word “disamenity,” used for example by Simpson (2011), conveys the right meaning and could conceivably be used. The term “amenity” has occasionally been used as a synonym of service, but since it is far less familiar to the general public than either function or service, its opposite, “disamenity” is less likely to foster communication. In this respect, the concept of “service” definitely has the upper hand, because it makes it possible to easily accommodate both positive and negative situations. Indeed, the term of “disservice” (Zhang et al., 2007; Ma and Swinton, 2011; Power, 2012; Bastian et al., 2013; Lele et al., 2013; Raymond et al., 2013; Li et al., 2014; Shackleton et al., 2016) carries unambiguously the suggestion of negative consequences for its recipients. In recent years, however, a number of authors have argued strongly against the use of this notion of disservice. Shapiro and Báldi (2014) claim that the concept of Ecosystem Disservice (EDS) may lead to an exaggeration of the harm caused by nature because this harm is already taken into account by market mechanisms. Villa et al. (2014) argue that the use of the concept of EDS “poses a danger to conservation efforts,” “carries the wrong message for both science and society,” and “adds confusion as nascent efforts emerge to tackle the long-standing goal of understanding and quantifying the dynamic aspects of ecosystem services.” These two articles have given rise to a flurry of counter-arguments, from different quarters (e.g., Sandbrook and Burgess, 2015; Oosterbroek et al., 2016; Shackleton et al., 2016), all assenting with Lyytimaki's (2015) perspective that “the core question of the concept of EDS is not about highlighting the disservices per se, but about putting both ecosystem services and disservices under a common assessment framework. This is required in order to establish a comprehensive overview of the net effects of ecosystem functions for human well-being.” It seems eminently reasonable to adopt the same perspective when dealing with soils.
Aside from these different reasons not to use the term “function,” the fact remains nevertheless that in a narrow and well-defined context, it has been used in connection with soils for over 50 years, and has served as a conceptual foundation for an appreciable body of research and significant policy making, at least in Europe. Indeed, in Blum's (1988) classification of soil functions, it is clear implicitly that this concept refers to ways in which soils result in benefits for the rest of nature, including plants, animals, and humans. Given this tightly circumscribed context (and visual depictions like that of Figure 1), ambiguity has been significantly lessened, and the concept itself seems, as deLaplante and Picasso (2011) put it, “less problematic than might otherwise be.” This in itself should make anyone cautious about eliminating too rapidly the notion of function in connection with soils and replacing it with something else, which initially may not have as much public support.
Another key argument, perhaps even more convincing than the weight of tradition, is that the term “function” does not have, like “service,” a strongly anthropocentric connotation. “Service” conveys the implication that the sole raison d'être of nature, and of soils in particular, is to serve the needs of human populations (Cardona, 2012). In this respect, the concept of service helps perpetuate an attitude that, arguably, has led to the environmental degradation we currently face. As Leopold (1949) once wrote, “We abuse land because we regard it as a commodity belonging to us. When we see land as a community to which we belong, we may begin to use it with love and respect.”
Since, as defined by Blum and his followers, the concept of “function” encompasses benefits delivered not just to human beings but to the rest of nature as well, it is therefore broader and less anthropocentric than the notion of “service.” Therefore, it makes sense to try to retain both “function” and “service” terminologies, as long as they can be articulated carefully, not just relative to each other, but also with respect to soil properties and processes.
From Properties and Processes to Services
One possible way to integrate soil properties and processes with functions and services is as illustrated in Figure 6, which combines a number of elements from the soil science literature. To the right of the diagram in this figure is a schematic representation of the different human needs that soil services can satisfy. This list, inspired by Maslow's (1943) classic study of the so-called “Hierarchy of needs,” was adopted by Dominati et al. (2010b). These authors recognize that this may not be the most complete representation of reality, compared for example to Max-Neef's (1991) far more elaborate “Matrix of needs.” Yet Maslow's simple hierarchy, which is easier to comprehend, already conveys the important message that humans have needs at both physiological and non-physiological levels.
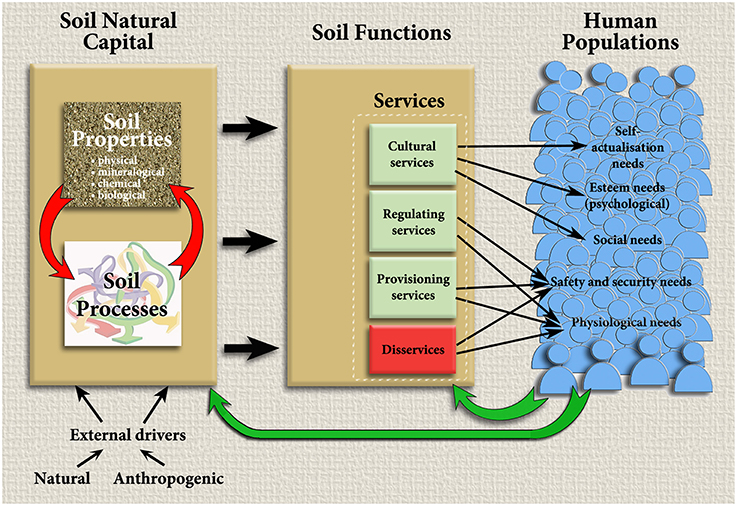
Figure 6. Schematic diagram depicting the relation between soil properties and processes (jointly constituting the soil “capital,” under the influence of a number of external drivers), soil functions (including soil services and disservices as subsets), and human well-being.
In the center in Figure 6, soil functions, defined as services to humans but also to all other living organisms, include as a subset not only the various cultural, regulating, and provisioning services described in detail by a number of authors in the literature Dominati et al. (2010b), but also a number of disservices of soils. These different services and disservices are related to human needs at several levels in Maslow's hierarchy. However, these relationships have not been analyzed in great detail yet, and divergent are likely to emerge about some of them. For example, Dominati et al. (2010b) argue that social and esteem needs cannot be fulfilled by soil services, because these needs are based solely on humans' self-perception of emotionally-based relationships with other human beings (or even animals). However, one could easily argue, as a number of authors have done (Boivin and Owoc, 2004; Wells, 2006; Salisbury, 2012), that, on the contrary, soil cultural services, in and of themselves or as part of a broader connection between humans and land or landscape, contribute strongly to the strengthening of a sense of individual and community identity, as well as of well-being among people (e.g., Tengberg et al., 2012).
The green arrow linking the human needs and soil functions/services components of Figure 6 is meant to indicate, as has been pointed out by a number of researchers (Spangenberg et al., 2014), that it is humans who determine what portion of soil functions ultimately transform into soil services and disservices. Soils, as one of their functions, provide nutrients to plants without discriminating between plants that are useful to humans and those that are not. That is a choice that we, humans, make. For example, a century ago, in parts of Europe, farmers cultivated up to 80 different crops every year. Over time, that number has decreased substantially, to such an extent that now, in some cases, only one or two crops are grown. Many of the other plants may still be present in nature but no longer have any commercial interest. They are now viewed as weeds and considered to be a nuisance when competing with crops for nutrients. In the next few decades, the situation may reverse as agriculture moves away from monoculture, and returns to what some now call “sustainable intensification,” which often calls for a diversification of crops and crop rotation. In this case, as with many other soil services, humans decide which soil functions are to be viewed as soil services or disservices.
To the left of Figure 6, one finds the soil “natural capital,” which one could view as the “stock,” producing, as “flows,” various functions/services. When trying to define or characterize this natural capital, many authors list various properties of soils. Dominati et al. (2010b), in their description of the soil natural capital, differentiate between “inherent” and “manageable” properties. The latter category corresponds to features, like pH or nutrient content, that can be readily modified by human populations, e.g., through agricultural practices, whereas the former category of properties, including stoniness, subsoil wetness, or high clay content in the surface horizon, presumably cannot.
In Figure 6, the soil natural capital is assumed to encompass both properties and processes. The key reason behind this perspective is that the richness of soils is not due solely to the physical constituents of soils, but also to the micro-, meso-, and macrofauna that finds a habitat in the soils, as well as to the myriad of (bio)chemical reactions that take place in soils. All of these dynamic features lead to an inextricable ensemble of interactions. Soil properties influence the nature and extent of the processes taking place in soils and, conversely, many of the processes occurring in soils eventually result in changes in soil properties, for example through more or less pronounced aggregation of solid particles to create various types of micro-scale architecture. Also, in Figure 6, properties are not separated into inherent and manageable classes, as suggested by Dominati et al. (2010b). Indeed, one might argue that this distinction is largely artificial (Robinson and Lebron, 2010). Stoniness, for example, can be viewed as an inherent property in many parts of the world, but it is easily managed by farmers in some regions (e.g., Puglia in Italy) by mechanically pulverizing stones. Similarly, high water table situations can be improved via the installation of drains, and the depth or clay content of soils is routinely modified, e.g., in brownfield reclamation strategies, via the replacement of the top layer of soils by fresh material.
The last component of the diagram of Figure 6, following Dominati et al. (2010b), consists of external drivers that affect the soil natural capital in various ways. These drivers can be natural as in the case of climate, natural hazards, geology, geomorphology, and biodiversity. Or they can be anthropogenic (Raymond et al., 2013), such as land use, farming practices, and the increasing “artificialization” of soils (for example during the construction of shopping malls or vast areas of parking), in which case they can be schematized by another arrow emanating from the human side of the diagram. As in the case of human influences on what constitutes soil services, this arrow indicates that human societies are deciding what types of pressure they are willing to exert on the soil natural capital, and how they are willing to make it evolve, positively or negatively (Vignola et al., 2010; Murray-Rust et al., 2011; Li et al., 2014).
The advantage of a graphical representation like that of Figure 6 is that is makes it easy to visualize where particular concepts fit in the broader picture. For example, soil biodiversity, soil fertility, or soil structure maintenance are commonly referred to as ecosystem services (e.g., van Eekeren et al., 2010; Atkinson et al., 2012; Thomsen et al., 2012). However, humans do not benefit directly from any of them. For biodiversity, neither does nature in general, so that biodiversity would not qualify even as a soil function, according to Figure 6. All of these cases illustrate properties that contribute to making a certain number of functions and services possible, like the production of food or, for biodiversity, the availability of new antibiotics to treat human diseases (Mace et al., 2012).
Descriptions of Soil Functions and Services can Foster Communication
Identification of the various services afforded by nature to human populations is merely the first step in the application of the “ESF” in its traditional form, and is typically followed by attempts to proceed with the monetary valuation of these services, so that they can be taken into account in financial and economic transactions (Figure 7). However, in the case of soils, without necessarily moving on to the next step, the work of carefully delineating the different soil services and documenting their relative significance in given regions, can in itself already be extremely useful. Indeed, the cataloging of soil services provides ammunition to educators and communication specialists to make the public at large and especially policy-makers better aware of the importance of soils for human well-being.
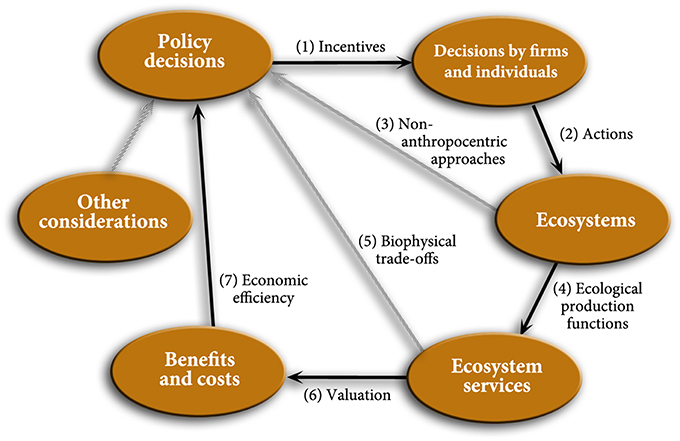
Figure 7. Framework for integrating economics and ecology in the study of ecosystem services. Solid lines indicate the links where the integration of the two disciplines can play an active role (Modified from Polasky and Segerson, 2009).
This communication is perhaps less crucial for other parts of nature, but experience has shown time and again that it is essential for soils. Yaalon and Arnold (2000) once pointed out judiciously: “The attitude of society to soils is no doubt affected greatly by how well the relevance of soil to all these functions and provision of services is understood and appreciated by the general public.” Seeing how soils tend to be ignored by society—soils are often referred to simply as “dirt” in the general public—, one is encouraged to conclude then that many if not most people are entirely unaware of several of the functions or services provided by soils. Relative to Figures 1, 2, it is likely that the majority of people (except perhaps in urban areas) have some notion of the fact that soils enable the production of the food they eat, or that soils support their dwellings. Some may know that soils contain groundwater that can be extracted for human use, although in many cases, their notion of how groundwater flows in the subsurface tends to be fuzzy or even erroneous. Also, there are frequent enough archeological digs in cities all around the world, or television programs on that topic, that educated people tend to know about the role of soils in the preservation of remnants of the past. However, when it comes to other soil functions/services in Figures 1, 2, the public at large tends to be entirely ignorant of what goes on in the subsurface.
It is no coincidence in this respect that quite a few countries that have water and air protection laws, have never been able to pass an equivalent one for soils. Certainly, part of the reason for this has to do with the fact that soils, unlike water bodies or air, can be owned by individuals. Yet, the fact that processes in soils are as a rule far less visible than in water or in the air, and in many cases involve organisms (bacteria, archaea, or fungi) that one cannot see with the naked eye also undoubtedly contribute to the absence of legislation. Also, numerous aspects of soils are still very poorly understood, even by specialists, and therefore are seldom communicated to the public. For example, very few people, beside soil scientists, are aware that only about one percent of all microorganisms living in soils have ever been isolated, let alone characterized, and that numerous fundamental questions concerning them, e.g., the reason behind their extreme biodiversity or their involvement in the fate of organic matter, have remained basically unanswered up to now.
This continuing, widespread public ignorance about soils has had a number of negative consequences in different respects. For example, it is likely to have been directly or indirectly the chief cause of the severe shrinkage of enrolments in soil science education programs in a number of countries over the last 2 decades (Baveye, 2006; Baveye et al., 2006; Hopmans, 2007). Several national and international soil science societies have actively tried to launch various initiatives to combat and reverse this trend. Some efforts have met with success, whereas others have failed to generate much public excitement. In that context, educational efforts emphasizing the range of services that soils provide to human populations would seem to afford the best chance to get people to relate to soils, and several authors have made concrete suggestions in that sense (Robinson et al., 2009; Cardona, 2012). However, experience has shown that translating lists of soil services into a picture that resonates with the public presents daunting challenges. Simply enumerating the many services provided directly or indirectly by soils to human populations, as some articles have done (Daily et al., 1997; Comerford et al., 2013), has a tendency to become rapidly dreary and indigestible even for specialists. Fortunately, there are alternatives. Computer-based virtual field trips (Jacobson et al., 2009; Strivelli et al., 2011), lively videotaped documentaries, and “webinars,” have been used with success in recent years to introduce selected aspects of soils or specific contexts in which soil issues arise, but these avenues have yet to be explored to enlighten the general public about the services of soils.
Direct Measurement of Soil Functions and Services
Once the various ecosystem services of soils have been clearly identified, the next step in the standard ESF consists of their “valuation.” From a scientific perspective, however, it is pertinent to first inquire if, and to what extent, some of the functions and services of soils can be quantified objectively, i.e., independently of subjective considerations one might have about their value.
A number of articles published by ecologists in the last decade (e.g., Hodder et al., 2014; Lamarque et al., 2014; Crouzat et al., 2015) give the impression that for general ecosystem services, including some directly related to soils, quantification does not present any particular difficulty. The recent article by Crouzat et al. (2015) is a case in point. In it, the authors map 18 ecological parameters—16 ecosystem services and 2 biodiversity metrics—in the French Alps, and explore “their co-occurrence patterns underpinning the supply of multiple ecosystem services.” They produce “self-organizing maps” encompassing five clusters of ecosystem services, represented graphically by wind-rose- or spider-like diagrams (Figure 8), which the authors argue could be very valuable tools in support of land use planning. However, close inspection shows that none of the normalized values displayed in the wind roses was actually measured. Some, like the hunting data, were estimated by disaggregation into 1 by 1 km grid cells of public statistics gathered at much larger spatial scales, whereas most other parameter values were obtained via modeling, in the absence of any local data suitable to ascertain how well, or how poorly, the models performed in the selected region. Under these conditions, it is unclear to what extent the map in Figure 8 is anywhere near the truth, and whether it is more than merely an educated, subjective, and therefore potentially misleading, guess.
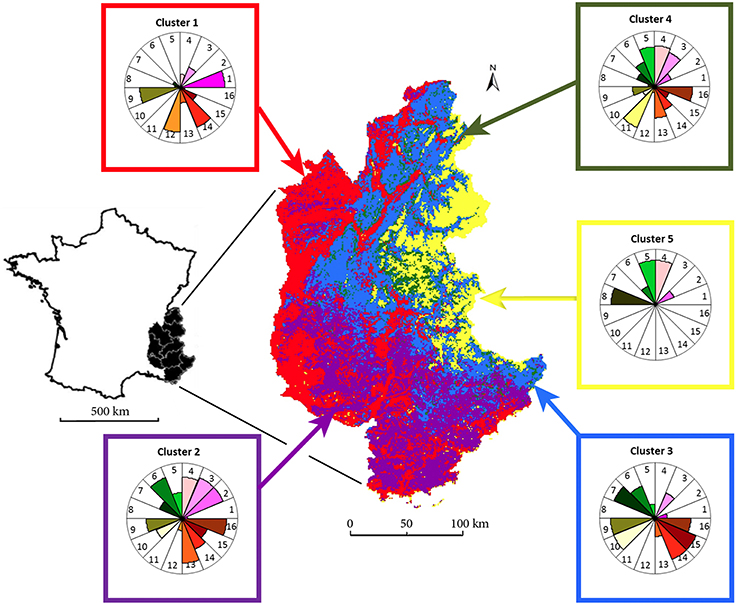
Figure 8. Self-organizing map of the French Alps, with five clusters and related ecological profiles (values normalized to 0–1) (Modified from Crouzat et al., 2015).
Clearly, application of the traditional scientific method to ecosystem services requires quantification that is far more objective and robust than that just alluded to. In some specific cases, it is possible to think of ways to measure services. Indeed, in the category of provisioning services, the supply of building materials, for example sand or clay extracted from soils, is measured routinely by companies selling them. Similarly, again for extractive activities, the amount of water that one pumps out of aquifers can be metered continuously. However, other services of soils are in principle significantly more challenging to quantify.
A service that has attracted considerable attention is the ability of soils to store carbon, thereby preventing or at least delaying its release in the atmosphere as CO2, CO, or CH4. But even the theoretically simple measurement of soil carbon can lead to operational difficulties, depending on the perspective from which it is approached. Traditionally, to determine the stock of carbon in soils at a given instant in time, a depth of 0.25–0.3 m at the surface is considered, associated with the plow zone. However, research carried out a decade ago, in particular by Baker et al. (2007) suggests that a much thicker layer of soil, of the order of a meter, needs to be considered to obtain meaningful data. More recently, various researchers have suggested that deeper soil horizons also contribute significantly to carbon storage and need to be paid more attention (Rumpel and Koegel-Knabner, 2011), which is not surprising since plant roots routinely extend to depths of 1–2 m, and often significantly more (Baveye and Laba, 2015). Therefore, if one factors in the need to take the spatial heterogeneity of soils into account, a simple accounting of the amount of organic carbon stored in a soil at any given instant of time can prove to be a time-consuming, not to mention extremely onerous, operation. To monitor changes in soil organic carbon content over time, one needs to be able to resample soils and to correctly interpret observed changes, which is fraught with uncertainties in soils exhibiting significant small-scale lateral heterogeneity or temporal changes in bulk density.
Another soil function that would seem relatively easy to quantify is related to the ability of soils to retain, via either sorption or filtration, a number of chemical compounds or biological pathogens that otherwise would transit through the vadose zone (the water-unsaturated portion of soils between the surface and the water table), and eventually contaminate groundwater (Keesstra et al., 2012). Potential contaminants are numerous, including, e.g., nitrate and uranium (added with fertilizers) under agricultural fields, human pathogens like Cryptosporidium parvum under pastures and wildlife reserves, and literally hundreds of organic or inorganic chemicals under industrial sites. With lysimeters, usually of one to a few m3 in volume, it is possible to monitor the transport of all these contaminants under more-or-less undisturbed conditions at any given location in the field (e.g., Song et al., 2009; Minamikawa et al., 2010; Robinson et al., 2013b). However, it is far more difficult and onerous to monitor contaminated transport at the scale of an agricultural field or a brownfield. This requires the installation of drains to capture groundwater before it leaves the site, which at best is feasible only in cases of shallow water tables. In general, the drains will collect only part of the groundwater exiting from underneath the site, and one needs to be able to relate that amount to the total, to get a more accurate estimate of the amount of leachate. For areas larger than a hectare or so, it is generally no longer manageable to try to directly measure the retardation services provided by soils.
This last example highlights the fact that the issue of scale can drastically complicate the quantification of soil services, as is also the case more generally for other ecosystem services (Konarska et al., 2002; Cohen et al., 2006; Hein et al., 2006; Glenk et al., 2012; Laterra et al., 2012; Setten et al., 2012; Kumar et al., 2013; Zhang et al., 2013; Grêt-Regamey et al., 2014; Andersson et al., 2015). Whenever one attempts to quantify a function or service of soils, a key decision to make is the scale at which this quantification is to be carried out. In the geography literature, this concept of scale is recognized to have two distinct components: extent and resolution. The first refers to the surface area one considers. For example, the amount of carbon stored in a soil can be quantified at a “point” (never really a point, but a very small area), over an area of a few m2 or of a ha, over an agricultural field, a watershed, or even beyond. The decision is arbitrary, and should be based on the objective pursued in the quantification. Within the specific area selected, data need to be presented at a certain resolution, which may be the pixel size if one uses aerial or satellite-based photographs (e.g., to describe land use patterns), or the cell size if one represents the landscape in a Geographical Information System (GIS). There again, the choice of a particular resolution may be subjective to some extent, within some constraints often set by the equipment or the availability of primary data. In principle, both extent and resolution could affect significantly the quantification of soil services.
One could consider many other examples of soil services that are not straightforward to measure directly. In fact, in the vast literature on the ecosystem services of soils, it is symptomatic that no publication to date has reported explicitly on the direct measurement of a single function or service. In other words, as others have already pointed out in the broader context of ecosystem services (Logsdon and Chaubey, 2013), there are currently no solid data at all on any function or service of soils. This has prompted Horrocks et al. (2014) to suggest that this knowledge gap be addressed as a matter of urgency. To palliate this lack of direct measurements, one option that has been explored is to use mathematical modeling.
Modeling Soil Functions and Services
The idea of using modeling is to estimate functions or services indirectly. Indeed, if the features of the soil that are key to the delivery of a particular service are identified over some expanse of land and can be assessed quantitatively, this information could in principle be used in one of a number of available mathematical models, like the very popular “Soil and Water Assessment Tool,” SWAT (Francesconi et al., 2016), to estimate the corresponding service. For example, the process of downward percolation of water and the concomitant filtration of pathogens, biocolloids, or particulate matter, leading eventually to aquifer recharge and to the supply of good-quality groundwater to human populations, can be approximated by measuring the hydraulic conductivity and filtration capacity of the soil at different locations and depths within the targeted area. Then after taking advantage of geostatistical or other spatial statistics methods to interpolate between measured locations and produce continuous maps of relevant properties, one could use the resulting maps as input in models of water and solute transport in the vadose zone, in order to predict the extent of aquifer recharge.
There are, however, several problems associated with this approach, which explain largely why it has not yet been used much to quantify soil functions or services. It may not be too difficult to model soil processes at selected locations in a field, even though recent attempts at predicting soil services under these conditions demonstrate that for some soil characteristics, predictions are not optimal (Aitkenhead et al., 2011). Still, it is significantly more difficulty to predict soil functions or services over specific land area and time spans longer than a few days. A key hurdle in the former case is that measurements are needed at a sufficient number of points to account for the spatial heterogeneity of the soil. Large numbers of measurements typically take an inordinate amount of time and therefore are onerous, especially if the area considered is large or the soil very heterogeneous spatially. Even if measurements could be carried out, the approach would still lead in most situations to relatively inaccurate predictions in the end. In the case of the prediction of aquifer recharge, uncertainty could result from inadequate handling of some key processes, like preferential transport, whose monitoring and modeling remain very challenging at this stage Beven and Germann (2013).
In addition to the tricky question of how big or small of an area needs to be considered, modeling efforts also run into the same resolution issue as does the direct quantification of soil functions and services. This is well illustrated in a landmark study by Kuo et al. (1999), who used different grid sizes to estimate inputs to a spatially explicit, variable-source-area hydrology model, with which they tried to predict soil water retention in a watershed in central New York. Data on topography, soil type, and land use were input at grid sizes from 10 to 600 m. Output data consisted of runoff and spatial pattern of soil moisture. Simulation results showed higher average soil water contents and higher evaporation rates for large grid sizes. During dry years, runoff was greatest for the smallest grid size.
In their review of the literature dealing with the estimation of ES with SWAT, Francesconi et al. (2016) present it as an advantage that “the simulation of watersheds in SWAT can produce outputs at different spatial and temporal scales. SWAT can provide data at the watershed, the sub-basin, and at the Hydrologic Response Unit (HRU) level, as well as for impounded areas (ponds, wetlands, etc.), reservoirs, and/or reach geographical features at the average annual, monthly, daily, and hourly time frames.” While this indeed shows the versatility of the modeling tool in question, it also raises a number of questions about the best way to approach the connection between ES and the various realities that are revealed at different spatial or temporal scales. Further research is definitely needed to provide guidance in this respect.
Using Soil Maps and Pedotransfer Functions
What a number of researchers have tried, instead of carrying out extensive measurements, is to take advantage of information that is already readily available, without requiring any extra effort. Wherever soil maps exist, they or the documentation that accompanies them typically, provide detailed spatial data on parameters like the particle size distribution in the different mapping units and horizons, as well as soil pH, CEC, organic matter content, and stoniness, among other soil parameters. They also provide information on land use pattern, at least at the time surveying took place. It would seem natural to think that this spatial information could serve as a foundation from which, in what is generally referred to as “bottom up” or “upscaling” approach, one could estimate soil functions or services over any surface area, small or large, and indeed that route has been explored by a number of researchers in the last few years (Lehmann et al., 2008; Lehmann and Stahr, 2010; Ungaro et al., 2014a; Calzolari et al., 2016).
Although simple in principle, this approach runs rapidly into a variety of difficulties. A first one is linked with the fact that soil surveyers have traditionally focused on a shallow portion of what other disciplines refer to as soil. When classifying soils, surveyers have concentrated on the part of subsurface materials whose morphological features are distinct from the underlying “parent” material. In some cases, this pedological soil may be as shallow as a few tens of centimeters, whereas to describe the functions and services at the same sites, the “soil” that needs to be taken into account may extend considerably below that depth (Robinson et al., 2014).
Another practical hurdle is associated with the essentially static character of the parameters about which information is available in soil maps. That they be static is not surprising, given the predominantly taxonomic context in which soil surveyors conceived and pursued their work; dynamic properties that change rapidly would have made their task very problematic. The same context explains why the parameters that were selected had to meet the additional criterion that they could be evaluated with relative ease, preferably in the field. A good example is granulometry, which soil surveyors can train to estimate by feel, a method that may be accurate enough for the purposes of classifying soils. However, detailed studies have shown that to estimate hydraulic properties and the travel time of chemical species to the groundwater, hand texturing in the field leads to significantly different estimates, compared to classical laboratory techniques like the hydrometer, pipette, and laser diffraction methods (e.g., Fenton et al., 2015).
In some cases, data about the parameters described in soil surveys can be used directly to predict the extent to which soils, locally, can fulfill various functions. For example, in the case of the provision of building materials (e.g., sand or clay), granulometry data given in the soil surveys would be informative in and of themselves. However, in general, the prediction of soil services requires knowledge of dynamic properties of soils, and one therefore has to try to estimate those on the basis of the static data that are available. For this purpose, various empirically-derived statistical correlations, known as “pedotransfer” functions, have been developed over the years, for example to jointly correlate the granulometry and organic matter content of a soil with its hydraulic conductivity, or to “guestimate” a soil erodibility factor, one of many empirical parameters required by the so-called Integrated Valuation of Ecosystem Services and Tradeoffs (INVEST) tool (Leh et al., 2013). Such functions can be applied systematically to predict how the hydraulic conductivity is likely to vary spatially in a certain area. The resulting data can in turn be used as input to a computer model in order to generate estimates of, e.g., groundwater recharge or nitrate leaching.
This approach has received enthusiastic endorsements. Palm et al. (2007), in particular, argue that “the natural capital of soils that underlies ecosystem services is primarily determined by three core soil properties: texture, mineralogy, and soil organic matter,” which are routinely provided in soil surveys. Supposedly all other properties of soils, like their aggregation, bulk density, nutrient concentration, and pH, can be deduced from combinations of the three core properties. In general, researchers are reluctant to go that far, do not presume they can predict everything with just three basic parameters, and tend to use as much as possible of the information contained in classical or digital soil survey maps, in conjunction with geostatistical or other spatial analysis methods, to quantify ecosystem services over regions of various extents (Jiang et al., 2007; Peukert et al., 2012; van Wijnen et al., 2012; Glendell et al., 2014; Menon et al., 2014; Tsonkova et al., 2015; Xiong et al., 2015).
Many of these articles focus on a single function or service. As a case in point, Ottoy et al. (2015), assess regional soil organic carbon stocks, related to climate mitigation. Their work reveals that even within this limited focus, some ambiguity is associated with the assessment, associated with a strong method-dependence in the spatial interpolation between map and profile data. Working in the same region and with the same original data set as two previous groups of researchers (Lettens et al., 2005; Meersmans et al., 2008) who used different upscaling techniques, Ottoy et al. (2015) predicted a value for the total carbon stock down to a reference depth of 1 m that was 12% lower than the estimate based on the individual profile approach of Lettens et al. (2005), and 10% lower than with the linear modeling approach of Meersmans et al. (2008).
Since, with few exceptions (e.g., Veronesi et al., 2014), comparisons between quantitative estimates of soil functions or services obtained with this general approach and actual, measured values are virtually non-existent, it is not easy to assess whether this work is heading in the right direction. For one thing, this approach is a viable option only wherever soil maps are available at a suitable resolution (Andrew et al., 2015). In some parts of the world, like the U.S., soil surveys have been completed, and soil maps, in hard or digital format, are available throughout the land. But in other countries, like France, that is not the case; for various reasons (including questions about the purpose of maps), soil surveying was stopped a number of years ago, and soil maps at a suitable scale are lacking in several regions. Furthermore, the empirical pedotransfer functions on which this approach relies heavily may provide reasonable approximations as long as one remains within the particular set of soils for which these functions have been painstakingly developed and thoroughly tested, but they offer no guarantee at all of being accurate outside that set (e.g., Wösten et al., 1990; Baveye and Laba, 2015). Therefore, a key practical incentive for using pedotransfer functions, namely that they reduce the time spent making time-consuming measurements, may be more apparent than real. Finally, for some parameters associated with dynamical processes occurring in soils, there is no pedotransfer function available at the moment linking them to the limited set of static parameters measured by soil surveyors, and there is no plausible reason to think that anyone would come up with a reliable function any time soon. For example, no empirical correlation has been found to date between the spatial frequency and temporal evolution of preferential pathways in soils (e.g., wormholes, decaying root holes) and any of the static features listed in soil surveys.
Various authors have advocated, implicitly or explicitly, that a way to redeem this indirect approach based on soil maps and pedotransfer functions, is to combine the information derived from soil maps with “environmental covariates,” which can include geological, land cover, or digital elevation maps. For example, the mapping method proposed by Veronesi et al. (2014) to assess the spatial variation of soil carbon content storage uses traditional soil maps as one among a number of what they call “environmental covariates,” which include geological, land cover and digital elevation maps. Cross-validation tests end up with low R2 values, even though, in independent validation, the majority of predicted topsoil C values are within 5% of measured data. Another type of “environmental covariate” that has been used relates to specific land use and management practices that are used locally (Turner et al., 2016). The assumption that is made when using this covariate is that knowledge about the type of agricultural practice that is applied to a given soil is useful to determine what the soil characteristics are under current conditions (Hewitt et al., 2015). For some parameters, this assumption may indeed be approximately satisfied, whenever sufficient data exist linking given land use practices to ecosystem services, but in general, this is likely to be the exception rather than the rule. In many situations of interest, solid data are entirely lacking and one has to fall back on model predictions (Caride et al., 2012) or speculations about “anticipated” changes (Bennett et al., 2010). In some cases, even these speculations are missing. For example, we currently have no clue what effect different conservation agriculture practices might have on the recharge of aquifers or the transformations of agrochemicals in the subsurface.
Uncertainties, Bayesian Belief Networks, and the Top-Down Approach
This rapid overview of attempts to model soil functions/services, either using direct, targeted measurements of soil properties or using pedotransfer functions to predict soil characteristics of interest on the basis of soil survey data, suggests that this modeling effort is accompanied by significant uncertainties (Barnaud et al., 2011; Grêt-Regamey et al., 2013; Tancoigne et al., 2013; Barnaud and Antona, 2014). A traditional way to insure that the uncertainties associated with initial measurements or “guestimates” based on survey data, as well as the inaccuracies resulting from spatial or temporal interpolations, do not vitiate model predictions entirely, is to carry out detailed uncertainty analyses. In general, such an analysis is carried out after a sensitivity analysis, which serves to identify how model outputs are sensitive to the value of specific parameters (Garrigues et al., 2013). Sanchez-Canales et al. (2012) have carried out an uncertainty analysis for the INVEST model, applied to a Mediterranean watershed, and have demonstrated that some parameters have a larger influence than others on estimates of ecosystem services, and would therefore need to be determined accurately in practice.
Another approach to this crucial question of uncertainties, which recently has attracted considerable attention, is to use Bayesian Belief Networks (BBNs). Since their introduction in the mid-80s, BBNs have been adopted in various scientific fields, and are becoming increasingly popular tools in environmental modeling (e.g., Sadoddin et al., 2009; Haines-Young, 2011; Farmani et al., 2012; Henriksen et al., 2012; Troldborg et al., 2013), in particular to quantify and map ecosystem services (Grêt-Regamey et al., 2013; Landuyt et al., 2013, 2015; Celio et al., 2014; Rositano and Ferraro, 2014; Taalab et al., 2015). Formally, a BBN is a probabilistic model that represents graphically a set of random variables and their conditional dependencies via a network, referred to technically as a directed acyclic graph (DAG). As shown in the illustrative example of Figure 9, this network or graph consists of nodes and arrows. The nodes visually represent system parameters, whereas the arrows correspond to (causal or non-causal) relationships between the nodes. Each node consists of multiple states that can be defined qualitatively (e.g., based on expert opinion) or quantitatively. The likelihood that a particular state of a node is realized is depicted by a probability, subjected to Bayes' theorem. The use of probabilities enables BBNs to deal with uncertain input variables and uncertain relations among system parameters. These uncertainties are propagated through the network and result in model predictions that explicitly account for uncertainties. At any given time, a BBN represents the state of our knowledge on a particular process. Parameters evolve over time to reflect the fact that our knowledge is progressively modified, as new information becomes available.
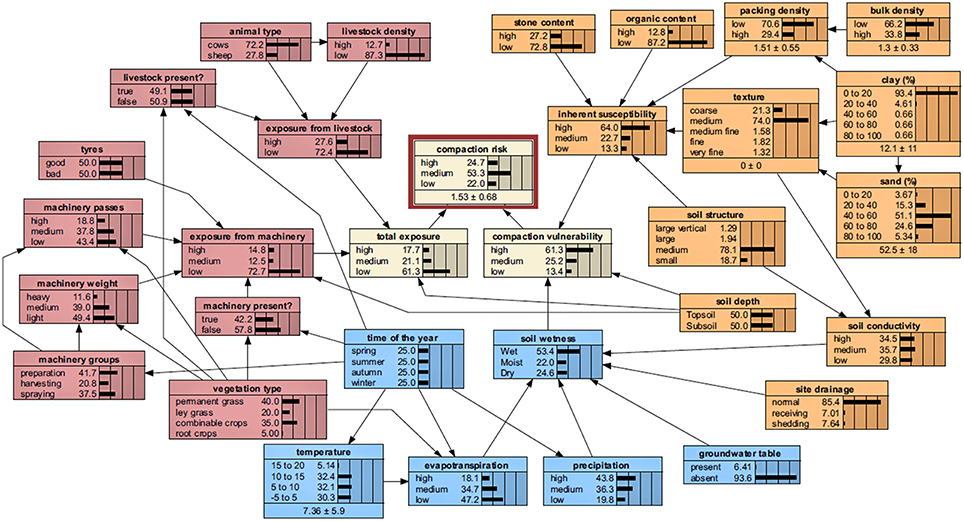
Figure 9. Schematic diagram of Bayesian Belief Network (BBN) corresponding to the assessment of the risk of soil compaction. The numbers in the boxes are the probabilities (%) of state values associated with each node, based on average conditions in Scotland (Modified from Troldborg et al., 2013).
This approach has been adopted by a number of authors, either to assess soil characteristics, like the extent of soil compaction (Figure 9), that are important in the delivery of specific services, or to quantify soil functions/services directly. An example of the latter is provided by the recent article of Landuyt et al. (2015), which deals with four services rendered by soils in an area in the northern part of Belgium: wood production, agricultural production, climate regulation through soil organic carbon sequestration, and clean water provision through infiltration. Basic data about these services were elicited from experts or extracted from the literature. Using a BBN approach, these authors propose a plug-in for a common GIS package, and with it are able to generate four different types of uncertainty maps: (1) conventional maps of expected values and (when feasible) standard deviations, (2) so-called “sampled maps” where the uncertainty in model output is visualized on the scale of land parcels, (3) “ignorance maps” in which areas where the probability of the model's predicted most probable state is < 60% are hidden, and (4) “cumulative probability maps” in which the value of a pixel is calculated as the sum of the probabilities of all the states above a certain threshold state. Depending on the situation and in particular on the question that needs to be answered, one or more of these different visualization options may be needed. Even though there is some subjectivity in the process of setting thresholds and in interpreting the results, the BBN approach may be extremely useful, not only because of its emphasis on uncertainties, but also, as Landuyt et al. (2015) suggest, because “it offers a structured and standardized approach to include expert knowledge into spatial analysis and decision support.”
In some cases, the uncertainties associated with estimates of soil functions/services may be overwhelming, for example when the number of parameters that need to be evaluated vastly exceeds our capacity to measure them, when soil survey data are scanty, and consequently when pedotransfer functions are of doubtful reliability. In situations like these, another way to handle the uncertainty problem, discussed in detail in Baveye and Laba (2015), is to look from the other side of the spyglass, i.e., to assess first which heterogeneity of soils, at what scale, needs to be included in the analysis of a specific issue. In some cases, for specific locations and soil functions/services, this can result in drastic simplifications. For example, Bouma (1989) argued, more than 25 years ago already, that soils that are pedologically different, in terms of a number of routinely measured static properties, are not necessarily so from a hydrological point of view, i.e., on the basis of their dynamic features related to the movement of water. Other researchers have reached similar conclusions (e.g., Santra et al., 2011). Basu et al. (2010) provide a vivid illustration in this respect. They investigated the hydrological response (hydrograph) of a mesoscale watershed (~700 km2) in northeastern Indiana. Survey maps indicate that soils in the watershed have been classified according to 80 soil series and ~14,000 soil mapping units, which suggests “the need for detailed, spatially explicit hydrologic models for description of water flow” (Basu et al., 2010). However, a detailed analysis using the Soil-Land Inference Model (SoLIM) showed that the available water storage (AWS), a key parameter for the prediction of hydrographs, was far more uniform within the watershed than the heterogeneity of soil survey maps would have led one to assume. Indeed, Basu et al. (2010) found out that more than 80% of the soils in the watershed had AWS values between 80 and 120 mm, with higher values restricted to soils located along the riparian zones. This relatively uniform distribution of AWS values made it possible to represent the entire watershed as a single, effectively homogeneous hydrologic unit. Therefore, in spite of significant physical heterogeneity, the watershed considered by Basu et al. (2010) exhibited “functional homogeneity.” Probably because of that, a very simple, parsimonious Threshold-Exceedance-Lagrangian Model (TELM) developed by the authors, using a top-down approach to predict the principal moments of event hydrographs, was able to perform as well, without parameter calibration, as did a complex physically-based hydrologic model (SWAT).
In this context, it is very reasonable to consider that, in the case of soil ecosystem services as well, the kind of top-down approach that is more and more advocated in hydrology (e.g., McDonnell et al., 2007; Basu et al., 2010) would be far preferable to a bottom-up perspective. A first step in a top-down approach would consist of first identifying and classifying soil functions or services at the spatial scale that one deems most relevant to the particular question that is being asked, then working backward to determine what soil parameters need to be measured or estimated in order to describe these functions and services adequately.
“Indicators” of Soil Functions and Services
Faced with the challenges that arise from direct measurements and the detailed, “bottom-up” modeling of the various functions that soils fulfill or of the services they provide, various authors have preferred to opt for yet another alternative. It consists of trying to identify biotic or abiotic characteristics that are correlated, or at least are thought to be correlated, with soil functions and services of interest. These characteristics are generally referred to via a number of terms, like “indicator variables,” “indicators,” “metrics,” “surrogates,” or “proxies” (Bockstaller et al., 1997; Egoh et al., 2008; Eigenbrod et al., 2010; Faber and van Wensem, 2012; Lautenbach et al., 2012; Pulleman et al., 2012; Thomsen et al., 2012; Ausseil et al., 2013; Williams and Hedlund, 2013; van Wensem, 2013; Dominati E. et al., 2014; Ungaro et al., 2014a; Wissen Hayek et al., 2016). In principle, they should satisfy a number of criteria, such as being easily quantified and responding to change in a predictable manner (Dale and Polasky, 2007). Indicators that meet these conditions are believed to afford reliable information with which one can assess how soil functions and services are likely to vary in space and time.
However, in the absence of any actual measurement of soil functions or services at this stage, one could argue that it is not possible to determine rigorously what constitutes a good indicator for them. Whenever, data are available to carry out some kind of assessment, the only statistical correlations that can be drawn are between indicators and processes that, based on expert knowledge, are considered likely to be involved in the delivery of services. Most often, in the literature, correlations are not even based on actual data, but emanate solely from expert opinion (e.g., Rutgers et al., 2012). A further criticism (e.g., Landuyt et al., 2015) is that indicator values generally are not accompanied with any estimate of related uncertainty, which prevents detailed analyses of the soundness of predictions eventually made on their basis.
Biodiversity affords a good example of indicator of soil services. The link between biodiversity and ecosystem services sometimes is believed to be so strong that, as pointed out by Mace et al. (2012), some authors consider implicitly in their writing that changes in biodiversity automatically correlate with changes in ecosystem services (e.g., Gaba et al., 2015). But this link is not at all certain (Glenk et al., 2012) and, to the extent that it exists, may be strongly scale-dependent (Beare et al., 1995). Close analysis suggests that “the quantitative relationship between biodiversity, ecosystem components and processes and services is still poorly understood” (de Groot et al., 2010). This view has been reiterated by others (Jaillard et al., 2014; Hellec et al., 2015; Jax and Heink, 2015), in particular by Mace et al. (2012), who write that “the relationship between biodiversity and the rapidly expanding research and policy field of ecosystem services is confused and is damaging efforts to create coherent policy.”
In the specific case of soils, various authors (e.g., Pimentel et al., 1997; Pulleman et al., 2012) have argued that there is a close link between the biodiversity of soils and their ability to deliver various functions or services. Schouten et al. (1997), for example, opine that most “life support functions” of soils “stem from activities of soil organisms,” and the literature is replete with rewordings of the same theme (e.g., Wall et al., 2004; Wall, 2012; Wall and Nielsen, 2012). From this perspective, maps of soil biodiversity are often argued to provide useful information on soil services. Not only does the lack of quantification of soil functions and services raise questions about these statements, but also difficulties with the assessment of biodiversity itself creates challenges. Reasons for this include: operational problems associated with the extraction of DNA from soil matrices; the fact that only a minute percentage, around 1.5% of soil bacteria, archaea, and fungi have ever been characterized and can be cultured (Baveye, 2009); limited understanding of why there seems to be so much functional redundancy among soil microorganisms, or of the respective roles of individual-level and community-level selection in shaping overall system properties (Loreau, 2010); uncertainty about whether to express biodiversity of soil organisms on a number or metabolic footprint basis (Ferris and Tuomisto, 2015); and doubts about whether the concept of species even makes sense for soil bacteria or archaea, given the extent of genetic material transfer among them.
In addition, the design of experiments to demonstrate a causal link between this soil biodiversity and ecosystem functions or services appears extremely problematic, because in most soils the effects of biodiversity are interconnected with those of the geometry and topology of the pore space, the biochemical composition of the solid phase, or the hydrologic regime (Kibblewhite et al., 2008). The effects due to these different characteristics of soils may be extremely difficult to tease apart. Wagg et al. (2014) admit as much in their description of microcosm experiments. These authors inoculated replicate samples of a sterile soil with communities of progressively decreasing diversity, obtained by passage through smaller, and smaller meshes (which successively eliminated the soil macrofauna and part of the microorganisms), and they evaluated a number of soil processes over the course of 14 or 24 weeks. The sieving procedure led to changes in both the abundance (overall number of live individuals) in the inocula, as well as in their diversity (assessed after the fact using molecular techniques), with no real way of determining which feature was more significant in the ensuing experiments. It is entirely possible that the most influential parameter in these experiments was not biodiversity but the fact that fewer organisms, more distant on average from food sources, had a lower overall metabolism. Even with this caveat, results are not straightforward to interpret. Some soil processes seem more sensitive to biodiversity/abundance changes when both microorganisms and mesofauna are present, whereas for others, higher sensitivity occurs when only microorganisms are left. Worse yet, the net productivity of plants grown in the microcosms, and two soil processes—N2O emission and nitrogen leaching—have non-monotonic relationships with biodiversity/abundance. A similar criticism can be levied against the work of Philippot et al. (2013), who also claim to relate biodiversity to nitrogen cycling, viewed as an ecosystem service. These authors carried out a 5-order of magnitude dilution of a soil extract in order to obtain different inocula with contrasted biodiversities. The more diluted inocula suffered a 75% reduction of estimated species richness. As a result of this dilution, the number of viable organisms inoculated in each soil sample was also decreased, so that it is entirely possible, as in the previous example, that biodiversity was not the only, let alone the key, reason of the observed four-fold to five-fold changes in denitrification in the experiments. Therefore, claims that biodiversity could serve as a reliable indicator of soil functions or services at this point appear uncertain.
A related question about the use of indicators or proxies to get an impression about the importance of soil functions or services is whether the results one gets are even remotely close to reality. A few researchers have tried to quantify the potential effects of using proxies on the results of studies mapping the spatial distribution and congruence of ecosystem services, and the results are not encouraging. A particularly detailed analysis is that of Eigenbrod et al. (2010), who investigated to what extent landcover-based proxies could be used to quantify services related to above-ground biodiversity, recreation activities, and combined above- and below-ground carbon storage. The latter is directly relevant to the ecosystem services of soils. It was not measured, but was estimated, at a spatial resolution of 1 × 1 km and down to a depth of 1 m, based on data about soil organic carbon concentration, bulk density, and stoniness, available for all 977 soil series in Britain. Eigenbrod et al. (2010) found that landcover proxy data resulted in ecosystem services maps that were poor fits to ones based on direct estimates calculated from soil data. Furthermore, correlations between ecosystem services changed depending on whether primary or proxy data were used in the analyses. Finally, the coincidence of hotspots of single ecosystem services was poor between maps, and consistency was extremely low when considering the locations that were coincident hotspots for multiple services.
Valuation of Ecosystem Services: Total Economic Value
Given this context of soil functions and services that no one has yet successfully measured, and whose estimation, or even characterization in terms of “indicator” or “proxy” variables, raises numerous questions, one may wonder whether it is worth continuing with the next step of the ESF, dealing with the valuation of functions/services. The answer is definitely affirmative because, as much as, from a scientist's perspective, it makes sense to advocate strongly that the assignment of values to soil functions/services should rely heavily on their quantification, in actuality these two ways of apprehending reality do not necessarily follow from each other. In particular, for a number of cultural, aesthetic, and spiritual services, quantification based on fundamental scientific principles would be entirely meaningless, and valuation is where the story starts.
According to the Oxford English Dictionary, “value” means “the regard that something is held to deserve; the importance, worth, or usefulness of something.” More specifically, Edwards-Jones et al. (2000) define value as “a framework for identifying positive or negative qualities in events, objects, or situations.” Implicit to the definition of value, for Robertson (2012), is the notion that it allows a comparison or ranking of different entities, like the functions or services of nature: “value is simply that quality of an object that permits measurability and therefore comparability.” Luck et al. (2012) define valuation as “scoring on the basis of importance.”
Beyond these straightforward definitions, the concept of value becomes rapidly multifaceted, subdivided into a complex array of different categories. A first level of distinction concerns whether or not an object or action serves a recognizable purpose and can thus valued by virtue of function. If that is the case, one talks about an “extrinsic” or “instrumental” value. Conversely, in the absence of any function, i.e., without being simply a means to an end, an object or action can have what is referred to as “intrinsic” or “inherent” value.
This notion of intrinsic value has raised considerable controversy (More et al., 1996; Attfield, 1998; McCauley, 2006; Vucetich et al., 2015). In particular, philosophers (e.g., Zimmerman, 2015) have extensively debated the issue, some of them even denying that intrinsic value exists. The customary division of intrinsic value into an “aesthetic” component, concerned with beauty, and a “moral” component, consisting of judgments of virtue, rightness of action, and justice, raises a number of tricky questions, especially when applied to nature. It is not clear, for example, how the aesthetic appeal of nature, as perceived by humans, is not a service, to which an instrumental value should be associated. Furthermore, many, perhaps most, animal species that, from a human perspective, “do not seem to have any conventional value at all, even hidden conventional value” (Ehrenfeld, 1988), e.g., very rare, poisonous frogs in inaccessible parts of the rain forest in Central America, may very well have a “moral right to exist” that is intrinsic, i.e., entirely independent of human experience. However, it is not obvious at all how human observers should go about assessing its value (Pearce et al., 2006).
Davidson (2013) has proposed that intrinsic value relates to functions that result in benefits to nature, not to humans and that it should be included, alongside the traditional instrumental value, in the total economic value (TEV) of objects or actions, as in Figure 10. This suggestion has been criticized on the grounds that the term “economic” is firmly bound to an anthropocentric perspective, which therefore excludes the value of nature in and of itself. Nevertheless, this proposal to include intrinsic value presents the advantage that all functions of nature are now accounted for, and not just the subset of functions (i.e., services) that benefit humans.
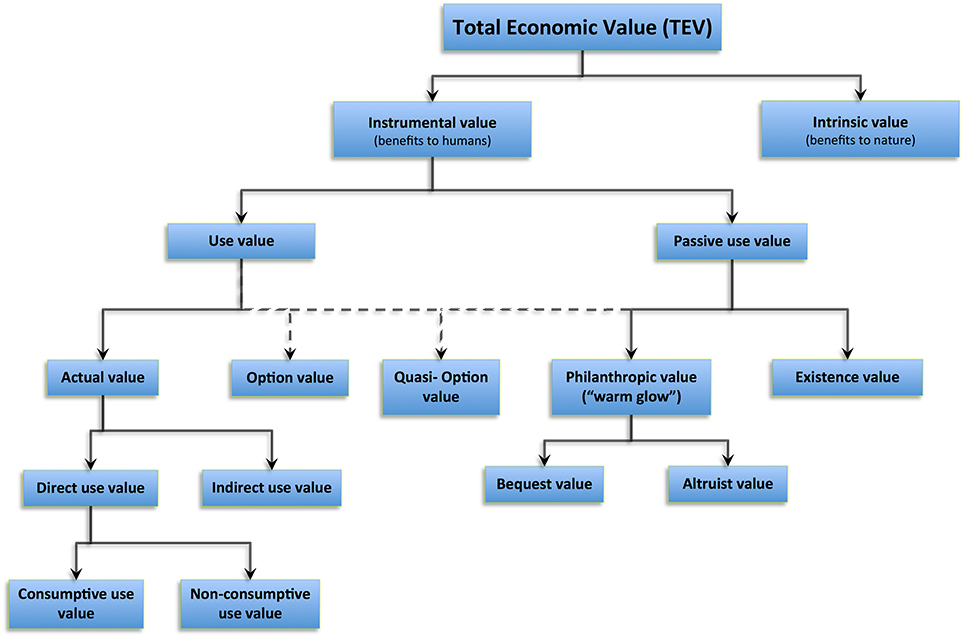
Figure 10. Schematic diagram of the subdivision of the Total Economic Value (TEV) into different types of values. The “intrinsic” or “inherent” value is included explicitly in this diagram, following Davidson (2013). There is no consensus in the literature on a single standard categorization or terminology of values. Therefore, the key purpose of this figure is to show how complex and multifaceted the overall value of an object or action can be.
Instrumental value is customarily divided into “use value,” and a component that traditionally has been referred to as “non-use” but now is increasingly termed “passive use value.” Use value is often divided into actual value, option value, and quasi-option value, although many classification schemes place the option and quasi-option values under passive use value.
Actual value is considered “direct” when goods and services are used or interacted with directly. Direct use value is often subdivided further into consumptive and non-consumptive categories, the difference between the two hinging on whether the value decreases as a result of use; it does in the former case (e.g., sod peat extraction), but not in the latter (e.g., enjoying a beautiful landscape). Direct use values of goods and services may be associated with an exchange value in some market place, but not necessarily. A builder fixing a house with adobe walls may get a few wheelbarrows of suitable soil from a plot of land nearby, without ever having recourse to commercial providers. “Indirect” use value refers to the life support services provided by the natural environment, which are enjoyed indirectly.
The option value (Weisbrod, 1964) of an object reflects the value people place on the ability to use the object in the future, and thus on the potential future benefits of the services the object may provide. The quasi-option value is sometimes defined as the value of the future information made available through the preservation of a given resource (Freeman, 1993). Another, far more abstract definition is that quasi-option value describes the welfare gain associated with delaying a decision when there is uncertainty about the payoffs of alternative choices, and when at least one of the choices involves an irreversible commitment of resources.
The classification of passive-use value can rapidly become very complex (e.g., Crowards, 1997). A simple classification distinguishes between existence value and philanthropic value. Existence value results from knowledge that goods and service exist and will continue to exist, independently of any actual or prospective use by the individual. Some authors consider that existence and intrinsic values are indistinguishable, whereas for others intrinsic value is entirely independent of any human perspective, unlike existence value. Philanthropic value represents inter- and intragenerational equity concerns, respectively. One may value a particular soil service in the hope that it will benefit one's descendants (bequest value) or other people, e.g., who live in its proximity (altruistic value). Even though they relate to other people, these passive-use values, like all the other values in Figure 10, are considered strictly from a perspective centered on an individual. This characteristic is particularly important for the altruistic value, otherwise there would be a risk of double-counting when aggregating values among different people.
Classifications of the components of the TEV, and in particular diagrams like that of Figure 10, are standard in most economics and environmental economics textbooks (e.g., Pearce and Turner, 1990; Freeman, 1993). However, in the overwhelming majority of cases, nothing is done with these different values directly, and the message that is given, implicitly but unambiguously, is that the only way to handle valuation is to try to associate a price to each component of the TEV. In other words, monetization is the only option; no attempt is made to quantify each value as such, independently of monetary considerations.
And yet, it is possible in principle to do so: “the economic metaphor does not necessarily need to be central to any communication regarding how ecosystems contribute to human well-being” (Luck et al., 2012). Individuals who have a stake in the management of soils within a given geographical area can be asked to quantify each entry in a value-vs.-soil function matrix. Methods need to be elaborated for this type of valuation, in particular to avoid elements of this matrix being overly affected by interconnections among functions/services, which at the moment are challenging to disentangle (Comerford et al., 2013). Nevertheless, Breure et al. (2012) and Rutgers et al. (2012) have carried out such a multi-stakeholder, multifunctional valuation of soil services at different spatial and temporal scales. They observed very different responses among different groups of stakeholders, with the natural degradation of organic compounds (natural attenuation) being valued very little by farmers but highly by regional or national policy officers, whereas exactly the reverse was true for soil architecture. These disparities in stakeholders' responses seem linked in part to the level of awareness about the importance of soil functions/services. In this respect, as suggested by Iniesta-Arandia et al. (2014), “linking values to other stakeholders perceptions might be a useful way to move forward in ecosystem services valuation.”
Valuation of Ecosystem Services: Collective Perspectives
To resolve these disparities, one could envisage aggregating in some manner the responses of all the people who are concerned directly or indirectly about the present and future use of a given piece of land, to obtain what would amount to a collective perspective. Unfortunately, economists and sociologists have shown long ago that aggregation methods are all very problematic in this context (Parks and Gowdy, 2013; Kenter et al., 2015). In particular, Arrow's (1950) impossibility theorem shows that, in situations where individuals are asked to rank different parameters (e.g., distinct values of a given object or service), it is not possible to convert the ranked preferences of individuals into a community-wide ranking while also meeting a pre-specified set of criteria, including consistency, non-dictatorship, universality, monotonicity, and independence. In other words, there is no logically infallible way to aggregate values across individuals (Feldman, 1987; Kenter et al., 2015).
Compounded to these issues with aggregation is the fact that each individual can hold both individual-centered values and community- or society-level values, which do not need to coincide (e.g., Sagoff, 2007). As an individual, one can for example associate a very high value to the sentimental bond people can have toward the land, but as a member of a community, e.g., as a citizen of a country, one can also feel that the interest of all should take precedence over individual interests under certain conditions, and therefore that a government should have the right (as it generally does, in most countries) to expropriate landowners, if need be.
Therefore, even if individual-centered values could be aggregated meaningfully, the aggregate might still not correspond at all to what is referred to as “shared, social, and shared-social values.” Kenter et al. (2015) consider that there are seven main types of these non-individual values, and that they can be organized along five principal dimensions (Figure 11), related to how values are elicited, who might provide values in a valuation setting, the type of value concept involved, value intention, and value scale. Of these different dimensions, the one related to the “elicitation process” is especially relevant to a growing body of the literature dealing with soil functions/services, where deliberative methods are used increasingly. Kenter et al. (2011) have shown in a practical situation in the Solomon Islands how the use of a group-based participatory approach instead of a conventional individual survey could overcome many of the impediments of decision-making based on traditional valuation, in particular the lack of information of some of the stakeholders, or the unwillingness of some stakeholders to trade-off key ecosystem services.
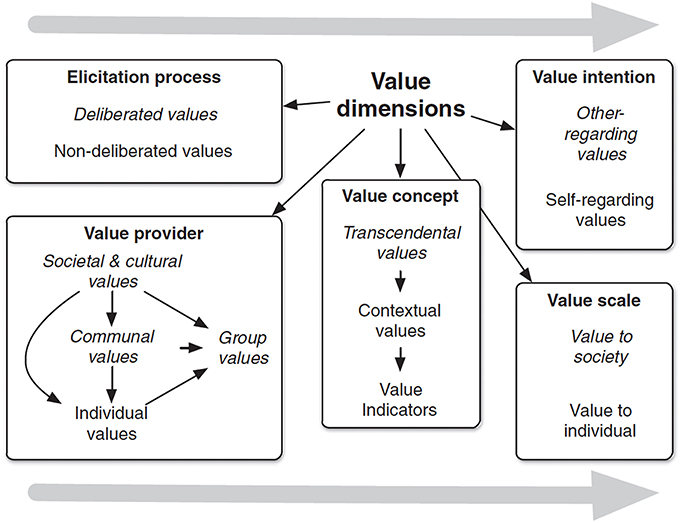
Figure 11. Shared and social values framework: the five dimensions and seven main types of shared and social values. Bold titles indicate non-mutually exclusive dimensions of value. Emerging from the dimensions, we can differentiate between types of values that might be termed shared, social, or shared social values (italicized) and other types of values. For example, provider is a dimension that indicates who might provide values in a valuation setting; societies, cultures, communities, and ad-hoc groups provide societal, cultural, communal, and group values, which are all types of shared or social values. Individuals also provide values, but these are not termed shared or social, unless they can be classified as such on a dimension other than that of value-provider. Arrows within boxes indicate directions of influence between different types of values. Gray arrows signify that the type of elicitation process and value provider strongly influence what value types are articulated along the concept, intention and scale dimensions (Modified from Kenter et al., 2015).
Group-based, participatory approaches can be adopted on their own, or they can be integrated in a very practical approach to decision making, known as Multi-Criteria Decision Analysis (MCDA), which has been the object of a lot of attention in the last 30 years. MCDA will be described succinctly later on, along with recent examples of its increasingly popular application to soils.
Market Prices for Ecosystem Services: Limited Scope
Values, individual or otherwise, and quantified in some manner, could have constituted the basis of economics, but for the last century and a half, economic theory has instead taken a path that encompasses prices, i.e., monetary values, exclusively. Reasoning in terms of prices has become so dominant, in particular in the mainstream literature on ecosystem services, that many articles dealing with “valuation” do not distinguish it at all from “monetary valuation” (e.g., Costanza et al., 2014) and, more insidiously, transfer the “unavoidable” character of valuation to monetary valuation. The rhetoric has become so dominant that in many diagrams like that of Figure 10, the category of “direct use value” is restricted to goods and services that have a price by virtue of being exchanged on a market. This may be the case for soils or soil constituents under some circumstances. When soils are used by, e.g., large landowners, to extract an income through rent, land market prices represent in a certain sense the direct use value of soils that is associated with this usage. Also, topsoil or peat, extracted from soils, are often sold in bags in gardening shops. There is also a market for topsoil in bulk form, by the truckload, often as a result of major construction projects. Various soil constituents, like silica sand, the clay fraction (< 2 μm, used for pottery), or clay minerals (used as additives, e.g., in toothpaste or paper), are extracted from soils and have their own dedicated market. In some countries, various soil organisms (e.g., ants, termites, earthworms) are used by local populations as sources of proteins, and in that context, occasionally (but not always) end up being sold on markets (Decaens et al., 2006; Walter et al., 2015). Some insects, whose larval stage occurs in soils, are similarly consumed as protein sources, or are eventually purchased on markets by collectors (e.g., butterflies or coleopteras).
As numerous as seem to be aspects of soils for which market prices exist, there are however many more that cannot be readily monetized. The assimilation of direct use values to market prices, and by association, a similar reduction of other values to prices as well, was not always the rule in economics (Sandelin et al., 2014). Until the mid-1860s, economists carefully distinguished between “value in use” and “value in exchange,” only the second of which was closely connected to prices (e.g., Kallis et al., 2013)1. Adam Smith, for example, highlighted the distinction between value and price by referring to a paradox that, much earlier, had already puzzled individuals like Plato, Copernicus, and Locke, among others: “The things which have the greatest value in use have frequently little or no value in exchange; on the contrary, those which have the greatest value in exchange have frequently little or no value in use. Nothing is more useful than water: but it will purchase scarcely anything; scarcely anything can be had in exchange for it. A diamond, on the contrary, has scarcely any use value; but a very great quantity of other goods may frequently be had in exchange for it” [Smith, 1776 (reprinted 1977)].
This diamond-water paradox caused significant debate until the end of the 1860s, from which emerged two main solutions. The first, known as the labor theory of value, consists of envisaging the amount of work required to obtain the object or service being priced. From that perspective, according to Smith, “the real price of everything, what everything really costs to the man who wants to acquire it, is the toil and trouble of acquiring it.” The second approach, which largely closed the debate, and whose development many historians of economics consider the birth of the now dominant “neoclassical” economics, is known as the theory of marginal utility. To explain the paradox, marginalists argue that it is not the total usefulness of water or diamonds that matters, but the usefulness of each unit of water or diamonds. Since water is plentiful, the marginal utility of water is low; any particular unit of water becomes worth less to people as the supply of water increases. By contrast, diamonds are scarce. They are found in such low supply that the (perceived) usefulness of one additional diamond is far greater than the usefulness of one additional glass of water.
Regardless of the modern theory of prices that one considers, labor- or marginalist, it is clear that “the price of a good does not reflect its importance in any overall social or philosophical sense” (Heal, 2000), to which one should probably add that price does not reflect importance in any overall ecological sense either. At a more detailed level, another observation is that the price of a good or service is set by a relatively minute portion of the population. Prices are acknowledged to reflect the distribution of income among potential buyers, and therefore the existing social order (Heal, 2000). Certainly, the wealthier a population is overall, the higher the price is likely to be within it for any given good or service. However, beyond that, the “market price of a good does not tell us […] how much some of the people buying it might be willing and able to pay rather than go without. It tells us what it is worth to the “swing buyer,” what economists call the marginal buyer. […] The marginal buyer is the buyer who is on the verge of not buying the good, […] who would drop out if the price were to rise only a small amount” (Heal, 2000). In terms of ecosystem services, Pearce (1998), along with others (e.g., Toman, 1998), has insisted on the need to keep the marginal character of prices in mind. He has argued on that basis that the absolute price estimates associated by Costanza et al. (1997) with the world's ecosystem services are not meaningful from the perspective of neoclassical economics theory.
The disconnect between price and value to society is a problem when one deals with the environment, and with soils in particular. To try to alleviate the issue, some economists (e.g., Goulding, 1945) have developed the concept of “consumer surplus,” which is supposed to better reflect the importance of goods and services, as long as a demand curve is known for them. Consumer surplus is defined as the monetary gain obtained by consumers who purchase a product for a price that is less than the highest price that they would be willing to pay. Clearly, this consumer surplus is relevant to a slightly larger segment of the population than just the marginal buyers. Nevertheless, it still falls short of providing information on the importance of goods or services for the population as a whole. The situation gets even more complicated when one envisages cases for which there is no market price.
Prices for Non-Directly-Marketable Ecosystem Services: Still Very Controversial
Aside from questions about what market prices represent and how they are established, a significant hurdle is that, in the case of soils, market prices are available only for a very limited number of provisioning (i.e., extractive) services. To associate prices with all the other functions or services of soils, a different approach toward monetary valuation needs to be adopted. Fortunately, this problem is not specific to soils. Indeed, in the 1940s, as soon as researchers set out to value nature monetarily, they realized that an entirely new set of methods was required. They were developed progressively in the 50s and 60s.
These methods are traditionally classified in three major categories (Figure 12). The first encompasses methods that still rely on actual markets, albeit not directly for the services themselves. In the second category, “make-believe” (i.e., surrogate or hypothetical) markets are invoked, corresponding either to revealed preferences of individuals, following Hotelling (1949), or to preferences people state when polled, as originally suggested by Ciriacy-Wantrup (1947). Finally, a last category of methods corresponds to transfers of existing service monetizations from studies already completed at other locations or in slightly different contexts.
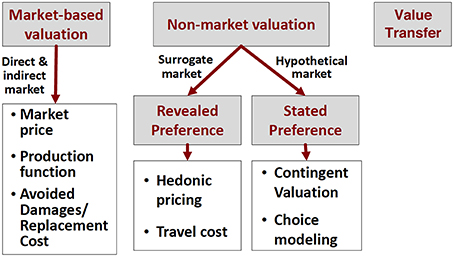
Figure 12. Schematic classification of the different methods that have been developed for the monetary valuation of ecosystem services.
Among market-based approaches, the productivity method is used to estimate the monetary value of ecosystem services that contribute to the production of commercially marketed goods (e.g., Barbier, 1994, 2003). In the second type of approach in this same category, monetary values are assessed on the basis of costs of avoided damages resulting from lost services, costs of replacing services, or costs of providing substitute services. For example, one can imagine estimating the price of water storage in a soil (which influences the hydrological regime) by the cost of a reservoir one would build to replace this storage function. But the price obtained in this manner would be a low estimate, because it would only account for one soil function (storage) and would not include, e.g., the fact that the stored water is available to plants. As Heal (2000) pointed out, “assessing the replacement cost is not a convincing way of valuing natural ecosystems and the services that they provide. Replacements rarely replace all of the services coming from the original system, so that this would capture only a part of the value.” The same author illustrates with examples another problem with replacement costs, namely the fact that in order for them to be accurately estimated, the costs have to be incurred, i.e., the replacements have to actually take place, and not just be hypothetical, as they are overwhelmingly.
There are two key revealed preference methods. In the Hedonic Pricing method (Ridker and Henning, 1967), the monetary value of ecosystem services is estimated via the direct effect they have on the market price of some other good. The Travel Cost method (Clawson, 1959), on the other hand, estimates prices of, e.g., a given landscape or ecosystem, on the basis of the amount of money people actually pay to travel to it. Unlike other methods, TCM is rarely applicable to soils, especially in areas of small extent. Indeed, until “pedotourism” develops and people routinely travel to admire soils, it genrally makes little sense to ask how much one would be willing to pay for such travel.
In terms of stated preference methods, the most commonly used one is the Contingent Valuation method (Davis, 1963; Mitchell and Carson, 1989), which asks people to directly state their willingness to pay (WTP) for specific ecosystem services, based on hypothetical scenarios. The Choice Modeling method is based on asking people to make tradeoffs among sets of ecosystem services or characteristics. It does not directly ask for WTP but rather infers it from tradeoffs that include cost as an attribute. In both of these methods, what is assessed is an aggregate of the private choices of each of the individuals surveyed. Research has shown that private choices may differ from social choices, made as citizens or members of a community (e.g., Sagoff, 1988; Gowdy, 1997), an important aspect of stated preferences methods, which will be addressed later.
Soon after the elaboration of these various monetary valuation methods, there was great enthusiasm among ecologists, in particular, to apply them to a variety of different environmental contexts (e.g., Leitch and Scott, 1977). In the wake of this surge of efforts, economists, and sociologists began to analyze closely the assumptions and implications of the different pricing methods. The sizeable literature devoted to this analysis contains a large number of very negative assessments. For example, Hanley (1992), in an in-depth review of the literature dealing with various methods for the valuation of non-marketed goods, including hedonic pricing and the travel cost method, found severe limitations with all of them.
Methodological criticisms have been frequently levied especially against stated preference methods. Diamond and Hausman (1994), at the close of a detailed analysis on the valuation of public goods, expressed their belief that “contingent valuation is a deeply flawed methodology for measuring nonuse values, one that does not estimate what its proponents claim to be estimating.” Research carried out since then has demonstrated that the willingness of individuals to pay for nature's services depends strongly not only on the socio-economic status of these individuals, but also, crucially, on the context in which questions are asked, on the level of information of respondents (Hanley et al., 1995), and on the way surveys are conducted. Unless extraordinary precautions are taken, a non-negligible portion of responses in WTP surveys often ends up being “$0” protest votes, for example by individuals who feel that they are paying too much income tax, or that their government is not using wisely the budget it already has (Luck et al., 2012). In terms of the way questions are asked, Bateman and Mawby (2004) observed that WTP for an environmental good was substantially higher if the interviewer wore formal clothing.
While it is possible that some of these shortcomings of monetary valuation methods will be eliminated in the future, it seems fair to say at this point that the research community still has not managed to resolve any of them satisfactorily over the last 60 years, as illustrated vividly by Hausman's (2012) recent downgrading of the status of the contingent valuation method from dubious to “hopeless”2.
A prerequisite of some of the valuation methods based on individuals' WTP, is that the people who are interviewed be well-informed and knowledgeable about the importance of the objects or services they are asked to monetarize. This is not a trivial requirement when it comes to soils, since, as alluded to earlier, the general public tends to be ill-informed about many of their characteristics and the myriad of processes they encompass. Even for specialists, numerous aspects of soils still remain nebulous and difficult to grasp. Therefore, to make sure that people asked to value soil services are aware of what is and is not known about them, “information must be conveyed in a way that reflects the substantial scientific or technological uncertainties surrounding ecosystem services. […] The information must also reflect a specified time frame so that people can distinguish, for example, an irreversible change from a long-term but reversible one” (Toman, 1998). Under these conditions, there is a possibility of significant bias in surveys, depending on the way interviewers formulate the questions, and on how much or which background information they provide prior to asking them. This possible bias led Toman (1998) to conclude that “economic valuation methods become more and more difficult to apply (to ecosystem services) as the services in question become more complex, large scale, interconnected, and subtle.”
Aside from technical aspects of available monetary valuation methods, their use is confronted to two additional, broader issues, which are very significant and remain largely unresolved to this day. The first concerns the fact that, as de Groot (1992) pointed out, “for some information functions such as the esthetic and spiritual value of nature, monetary evaluation is a difficult if not impossible procedure.” Over the last two decades, this same comment has been made numerous times (e.g., Rolston, 1985; Chee, 2004; Chan et al., 2012a,b; Kirchhoff, 2012; Setten et al., 2012; Parks and Gowdy, 2013; Winthrop, 2014; Boeraeve et al., 2015; Walter et al., 2015), with no clear solution in sight. There is definitely a risk in this respect that if monetary valuation becomes a standard step in the management of environmental resources, all the functions and services that cannot be monetarized will simply be ignored.
Another key criticism, particularly with valuation methods based on either revealed or stated preferences, is that only currently living individuals are observed or asked to voice their opinion. Certainly, a willingness by some individuals to pay may reflect to some extent a desire that the object or service being valuated continue to be readily available to future generations, but the questions asked typically do not emphasize this aspect.
Monetary Valuation of Soil Services: Waiting for Godot…
The various valuation methods presented in the preceding section were developed long ago, and were already used extensively in other contexts in the 60s and 70s, in particular to associate monetary values with wetlands and coastal areas (Barbier, 1994). In that context, one could have expected soil scientists to jump happily on the bandwagon. Some did, starting in the mid-80s. Nevertheless, progress has been very slow, so much so that, as Robinson et al. (2012b) pointed out recently, many articles being published these days still talk about assigning prices to soil services, but propose no number, nor suggest which method could be used to obtain one. There are notable exceptions to this general trend, but they are few and far between.
A portion of the articles that could potentially fit under this general umbrella of the monetary valuation of soil services actually do not deal with soils explicitly. They refer to “land,” which includes soils but also the vegetation (e.g., hedges, forested margins, crops) that they support. In what was apparently the first foray in this area, Halstead (1984) tried to assess the willingness of residents of three counties in Massachusetts to pay to preserve state agricultural land that provided services to local populations in terms of wildlife habitat, recreation, and scenic vistas. One third of the surveys had to be discarded because respondents refused to divulge their family income. Of the remaining responses, 25% were zero dollars bids, apparently in disagreement over the request to pay more taxes, even though indirect questioning showed that local residents “were prepared to pay substantial amounts to avoid residential development of agricultural land.” Bergstrom et al. (1985) proceeded to a mail survey to determine the willingness of inhabitants of a rural county in South Carolina to preserve 25, 50, 75, and 100% of the agricultural land in the county. Drake (1992) carried out a similar study in Sweden, using CVM, and found that WTP was affected by several factors, including income, age, level of education, general attitude toward land preservation, the type of landuse currently on the piece of land being valuated, and regional location. Drake concluded that survey results had low precision, in part because of the hypothetical character of the questions, and a lack of experience of the respondents with monetary valuation. A number of other researchers engaged in similar valuation efforts over the years (e.g., Kline and Wichelns, 1996; Bastian et al., 2002; Robbins et al., 2009; Wasson et al., 2013).
These different studies deal with land, not soil, and they address the TEV of the land, not the monetary value of individual soil services. Conceptually, one would think that it would be feasible to disaggregate land prices into the prices of the various below- and above ground components of land, and eventually to estimate the monetary value of soils. However, attempts along those lines by Ma and Swinton (2011) in southwestern Michigan, using a Hedonic Price method, show how complex such an analysis turns out to be in practice. Indeed, the price of a given piece of land is related in a non-negligible manner to recreational and aesthetic features of the landscape surrounding the land (Dale and Polasky, 2007), or to features within the land parcel, such as the presence of a stream or river, that may however not affect soil services per se. At the same time, some services of the soil within the targeted piece of land may fail to be capitalized in the land price, because of a lack of private incentives, a lack of awareness on the part of people surveyed, or a small perceived value of the services.
A sizeable body of literature concerns costs associated with the loss of topsoil (e.g., Cohen et al., 2006; Adhikari and Nadella, 2011; Telles et al., 2013). The physical processes involved are complex, scale-dependent, and modeled only imperfectly (Bilotta et al., 2012). Recognizing that some degree of erosion is unavoidably, if only at very small spatial scales (e.g., Bielders et al., 1996), researchers in the field still struggle with determining what “should be regarded as an acceptable level of erosion for a given environment and how this should be quantified and assessed” (Bilotta et al., 2012). Nevertheless, different methods have been developed to associate a cost with soil erosion. One of the simplest approaches, a direct application of the replacement method, consists of estimating the expense of replacing each cubic meter of soil lost through erosion (Almansa et al., 2012). As a recent example of this type of work, Robinson et al. (2014) carried out a web survey to find out what price was being asked in various countries for topsoil sold in quantities of one ton or more. Intrinsically, this price relates to an extractive (provisioning) service of soils. But indirectly, this price also corresponds to what would need to be paid, should one decide to buy soil at its market value to replace eroded soil material. Robinson et al. (2014) found prices to be very variable from location to location, with a median of $22 per ton in the US and Canada, and $47 in the UK. These authors also tried to figure out how much it would cost to purchase some of the typical constituents of soils (e.g., sand, silt, clay, organic matter), and to mix them in various proportions to artificially reconstitute different types of soil, which could be used to revert some of the damages caused by erosion. Aside from a possible connection with one type of provisioning service, it is not clear at all how the costs obtained with these different approaches relate to the array of services provided by soils.
A different way to estimate the economic cost of soil erosion, which has been adopted by many researchers (Adhikari and Nadella, 2011), is to assess the effect of losses of topsoil on agricultural production, in terms of decreased crop yields or diminished ability of pastures to feed animals. In principle, as noted by Pimentel et al. (1995), the total productivity loss should include off-site costs, such as those resulting from the progressive accumulation of sediments in streams, artificial lakes, and eventually in river effluents, all of which can cause significant problems for navigation, infrastructures, and the ecology of the associated ecosystems. However, rare are the articles that include these off-site costs (Adhikari and Nadella, 2011; Telles et al., 2013; Graves et al., 2015). Regardless, as with the replacement method, application of the production method to soil erosion costs reveals very little about the contribution of the different soil services to the total monetary value that is obtained.
A more comprehensive analysis, accounting specifically for individual services, has been adopted by a small number of researchers in the last few years. It consists of evaluating the total cost of erosion by summing individual costs associated with the replacement of a number of services that soils render. Along that line of work, but in a broader context than just soil erosion, Dominati E. et al. (2014); Dominati et al. (2016) have carried out what appears to be by far the most comprehensive monetary valuations available to date of multiple soil functions/services. In a volcanic soil under dairy in the Waikato region in New Zealand, Dominati E. et al. (2014) modeled various provisioning services, related respectively to “food quantity and quality,” and to “support for human infrastructures and animals,” as well as a number of regulating services, linked with “flood mitigation,” “filtering of nutrients and contaminants,” “detoxification and recycling of wastes,” “carbon storage and greenhouse gas regulation,” and “regulation of pest and disease populations.” A process-based dynamic model, the Soil Plant Atmosphere System Model (SPASMO) was used to explore the influence of a dairy farm operation on the soil properties and processes regulating the provision of ecosystem services. The dynamics of soil properties obtained with SPASMO were then linked to the provision of each soil service, by using integrative measures of soil properties as proxies for the quantification of ecosystem services.
The valuation of these services was carried out mostly through the replacement cost method. Flood mitigation was valued using the costs associated with building a water-retention dam on-farm, to substitute for the water retention capacity of the soil. The filtering of N or P was valued approximately by using the costs of existing mitigation techniques for limiting the loss of these two nutrients. The filtering of contaminants was valued using as proxy the cost of building an artificial wetland to decontaminate runoff water before it reaches water bodies. The valuation of waste recycling was estimated based on the development of an effluent treatment pond to degrade wastes and fertigation to return nutrients to pasture. Carbon market prices were used to value carbon storage and greenhouse gas regulation (after conversion to CO2 equivalent). Finally, the regulation of pest and disease populations in soils was only partially valued using a provision cost method, by limiting the analysis to the regulation of two major pasture pests.
Dominati E. et al. (2014) concluded from their calculations that the net present value (NPV) of the different services they considered is NZ$546,900/ha using a discount rate of 3%, and $164,070/ha using a discount rate of 10%. These numbers illustrate in a striking manner the significance of discounting rates in the monetary valuation of soil services. Interesting also in this study is the fact that, not only did the authors have to disregard all “cultural” services, but they also could not monetarize some regulating services because of the absence of man-made substitutes for them, for example in the case of the filtering of N and P. This analysis was extended recently by Dominati et al. (2016) to two different soils.
Prices of Soil Services: How Would they be Used Anyway?
One possible explanation for the fact that so few researchers have attempted to assign prices to soil functions or services so far, and for the flat out refusal of some soil scientists to even engage with the topic (Cardona, 2012), is that it is largely unclear how, pragmatically, these prices would be used down the line, and by whom. Should a price be associated with any key soil function or service, i.e., should this function or service become “billable,” Sullivan (2013) argues that then a key question is “who, via enforceable property or ownership rights, can either capture payments for this billable work right away, or profit by speculations on its future value.” It is clear in discussions among soil scientists on this topic that this question is crucial, especially after the severe economic crisis of 2008, where some of the major financial actors have shown what one might argue was a willingness to skirt both ethics and legality (e.g., Lalucq and Black, 2015). As Menzie et al. (2012) put it, “the crisis also revealed how some individuals and entities were able to game the system to extract profits at the expense of others.” From that perspective, letting some of the same actors decide on the fate of a resource that is essential for society at large may not be very wise. At the same time, the current absence of even the beginning of an answer to the question of how prices associated with soil functions/services would be used also weighs heavily on the mind of many.
Unfortunately, the general literature on ecosystem services offers no real clue with respect to how information on ecosystem services can be used for decision-making. In 2008, Daily and Matson (2008) wrote that “radical transformations will be required to move from conceptual frameworks and theory to practical integration of ecosystem services in decision making, in a way that is credible, replicable, scalable, and sustainable.” In spite of recent articles reiterating the connection between ecosystem services and decision-making (e.g., Guerry et al., 2015), it is now less clear than ever what the “radical transformations” alluded to Daily and Matson (2008) are, how one should account for the many sources of uncertainty associated with ES valuation (Knetsch, 2005; Johnson et al., 2012; Kumar et al., 2013), or how prices are going to be used in decision-making processes. Laurans et al. (2013) and Laurans and Mermet (2014) have carried out a comprehensive review of uses of ecosystem service valuation in the relevant literature, and they conclude to the existence of an overwhelming blind spot in this respect: “The common rule is to present an economic valuation, then suggest that it be used for decision-making, but without this use being either explicited or contextualized, and without concrete examples being provided nor analyzed” (Laurans et al., 2013).
Costanza et al. (2014) consider that “expressing the value of ecosystem services in monetary units does not mean that they should be treated as private commodities that can be traded in private markets.” In essence, these authors want readers to believe that their own previous reflection in terms of markets and prices was strictly a metaphor. This “eye-opening” metaphor, if indeed it ever was one, may have been “necessary to awaken a public deeply embedded in a global economy and distant from natural processes,” but as Norgaard (2010) writes, it “soon rose to become a central framework for scientifically assessing ecosystem change.” Under these conditions, what are the chances that prices are still going to be viewed as a metaphor, and will not be used simply as prices? It seems reasonable to consider that regardless of its original intent, the association of prices with ecosystem services paves the way toward their privatization and commodification (Vatn, 2000; Jax et al., 2013; Boeraeve et al., 2015; Walter et al., 2015).
For soils, this process might happen in a number of ways. First, landowners could in principle be paid for the amount of service provided by their land. A positive outcome of this, according to Bateman et al. (2013), “if farmers [were] rewarded for the delivery of a broad spectrum of ecosystem services,” would be to “provide policy-makers with a very powerful tool through which to secure beneficial land-use change.” However, right from the onset, this would eliminate the landless, people in the poorer social strata who have no or little land from which to provide ecosystem services (e.g., Kallis et al., 2013). In addition, unless prices were very high, it is unlikely that small landowners, whose property is just a fraction of a hectare, would find much financial reward in the operation, after factoring in the time needed to fill out the required paperwork. However, large landowners (like Ted Turner who owns over 800,000 ha in the US), could profit handsomely from payments for soil services, on top of what they already get paid in rent and farm subsidies (e.g., as incentives to leave land fallow). Undoubtedly, landowners would especially be in favor of any land-based Payment for Ecosystem Services (PES) scheme, and could lobby to have legislation enacted to stimulate it in countries where land tends to be concentrated in very few hands. The UK would be a good example in this respect, with a third of the registered land belonging to the aristocracy—among which the Duke of Buccleuch and the Queen, who own respectively about 96,000 ha and 54,000 ha. Having profited from payments for the services of the soil, these landowners would therefore likely be in a position to enlarge their properties still, which strictly from the viewpoint of the preservation of soils and of their use for the benefit of all, may not be a good thing. Indeed, as observed by Veblen (1923) already almost a century ago, “absentee” or “quasi-absentee” land owners tend to become “somewhat hasty and shiftless” in cultivating the soil, with potentially negative consequences for the soil resource itself in the long run (Lin, 2014).
The fact that financial institutions are reportedly eager for researchers to assign prices to ecosystem services (Sullivan, 2013; van Wensem, 2013) must mean that they expect to derive very large profits from various payment schemes for these services. One way to achieve this objective might be through some form of compensation, as in the case of carbon storage. In terms solely of the service of mitigating climate change, it does not matter where in the world carbon sequestration by soils is taking place. Therefore, one could envisage to compensate a relative loss of soil carbon in industrialized countries by a counterbalancing increase in soil organic carbon in Africa, for example. The same might be true if one looks at soil biodiversity solely as a potential source of genetic material, e.g., for the ultimate production of new medications. However, both for carbon sequestration or biodiversity, it is not true at all, in general, that compensation at another geographical location makes sense. The loss of overall soil services at a given location, say, in Europe, as a result of diminished carbon content and biodiversity, cannot be compensated simply by increasing these two parameters in a soil somewhere in Africa or Latin America. From a soil functioning standpoint, this type of compensation would make no sense at all.
Direct payments or compensations are two among many conceivable schemes for investors and financial institutions to profit from ecosystem services. The financial sector has demonstrated a lot of imagination in this respect in the last decade, coming up with “weather derivatives,” “catastrophe bonds,” “environmental mortgages,” and the notorious “species swaps” derivatives (Mandel et al., 2010), all of which seem motivated less and less by the idea of “selling nature to save it” (McAfee, 1999) but increasingly by that of “saving nature to trade it” (Sullivan, 2013). Some of these financial instruments, no doubt, could be adapted to exploit soils for financial gain. Even a catastrophic soil loss through erosion could become “a speculative opportunity like any other in a market hungry for critical events” (Cooper, 2010). No matter how much greed influences the motivation of the financial sector, the case of the New York city watershed in the Catskills shows that it is possible to modify any story to such an extent that facts become largely obscured to the general public (Sagoff, 2005; Laurans and Aoubid, 2011).
The various financial instruments that have been mentioned raise significant issues with regard to social justice, ethics, and the long-term preservation of nature (McAfee, 2012). Redford and Adams (2009) articulate no < 7 different categories of problems with payments for ecosystem services in these different respects. A common response to these concerns by the dominant (neoclassical) school of economics is that the inner working of financial markets guarantees that decisions about ecosystem services be optimal and efficient. However, that view is not widely shared universally. In a thought-provoking analysis, Karsenty and Ezzine de Blas (2014) have suggested recently that the metaphoric reference to the concept of market when considering PES essentially serves the purpose of making sure that governments do not step in to regulate payments that are made. Nevertheless, if markets have a less metaphoric role to play in the context of PES, the idea that their effect would be positive may be sound from an ideological standpoint, but not necessarily in a way that will do any good to either soils or human populations.
Pros and (Mostly) Cons of Cost-Benefit Analysis for Soil Services
To understand the fundamental problems associated with what economic and financial markets would do with prices of soil functions/services, it is useful to understand well how cost-benefit analysis (also sometimes referred to as cost-advantage or benefit-cost analysis) works in practice, as it is by far the favorite method to reach decisions in economics. In any given context where alternative decisions can be made, cost-benefit analysis compares their respective impacts (e.g., on ecosystem services) through a monetary metric. Positive changes are interpreted as benefits, and negative changes as costs (Wegner and Pascual, 2011). The resulting benefits and costs are then added to calculate the total net profit associated with each choice in terms of a NPV. The choices that lead to NPV > 0 are ranked in decreasing order, and typically the one with the highest NPV is selected, as it is assumed to bring about the largest increase in social welfare. Described in this manner, this approach seems particularly efficient and sound.
Popular in the 60s and early 70s, CBA appears to have fallen out of favor from the late 70s to the mid-90s, following intense criticisms (Pearce, 1976; Bromley, 1990; Gatto and De Leo, 2000; Chee, 2004; Wegner and Pascual, 2011; Baveye et al., 2013; Hockley, 2014). In particular, Ghiselin (1978) argued that “the usual technique of cost–benefit analysis is based on an inherently delusive method,” because it must require the “commensuration of the incommensurable.” Since CBA requires a common monetary yardstick to gauge ecosystem services, it must entirely ignore aspects that cannot be monetized, regardless of their importance (Anderson, 1993; Porthin et al., 2013; Söderqvist et al., 2015). Furthermore, not all of the values that could in principle be monetized can be included in CBA. Indeed, for CBA to be consistent with “rational choice theory,” one of the basic tenets of neoclassical economics, preferences expressed by individuals to monetize non-marketed goods or services are supposed to be “self-regarding,” i.e., entirely independent of other individuals. Therefore, the passive or non-use values in Figure 10, as well as intrinsic values, cannot be taken into account (Sunstein, 2005).
Leopold (1949) appears to have clearly understood these different limitations more than 60 years ago already (Lin, 2014), and considered that as a result, CBA cannot avoid being “hopelessly lopsided.” This lopsidedness may have consequences that are diametrically opposite to the purpose originally pursued. Indeed, a focus only on what can be monetized runs the risk of ultimately depreciating the object or service to which CBA is applied. This process is documented in detail by Sandel (2012), who illustrates in a variety of contexts the dramatic erosion of values that monetization can lead to.
Another extremely problematic aspect of CBA has to do with the temporal framework that is considered in the analyses. This issue, still entirely unresolved, has been the object of very contentious debates over the years (e.g., Bromley, 1990; Fromm and Bruggemann, 1999; Sunstein and Rowell, 2007; Gowdy et al., 2010; Adhikari and Nadella, 2011; Wegner and Pascual, 2011). In the case of soil functions/services, it is clear (or at least one would hope) that a given parcel of soil will continue to deliver them for many years, decades, or even centuries into the future. In principle, the benefits and costs that the soil will lead to in the years ahead should be taken into account in any cost-benefit analysis, along with present-day values, but this automatically would make such an analysis unmanageable unless it were possible, so to speak, to set an “end date” after which functions/services do not need to be considered any more. This is achieved by progressively decreasing the estimated, present-day value of future benefits and costs, using a so-called “discounting rate” (e.g., Fromm and Bruggemann, 1999; Anderson et al., 2015; O'sullivan et al., 2015). The higher the discounting rate, the shorter is the time during which we believe that a given function/service will be considered valuable by future generations. For example, with a discounting rate of 10% in applications of CBA to soil services Dominati E. et al. (2014), the assumption is that these services will cease to be considered valuable by local residents within the next 30–40 years, depending among other things on inflation. This figure of 10% is very high, but it is not considered excessive by economists, some of whom apparently thought the value of 3% used in the 2006 Stern report on the economic impacts of climate change was far too low. In their analysis of erosion control actions in Mediterranean basins, Almansa et al. (2012) considered discounting rates between 1 and 5%.
When it comes to soils, one could consider, that any value higher than 0% would not be particularly meaningful. Actually, it would even make sense to envisage negative discounting rates (Gowdy et al., 2010; Wegner and Pascual, 2011). Indeed, with a fast increasing human population on earth, and shrinking agricultural land resources (because of degradation and “artificialization”), we could consider that future generations might depend even more on soils for agricultural production than is the case now, leading to far higher monetary values for associated functions/services. Even if the soils that are left in a 100 or 200 years are no longer used for food production (which may be entirely based on hydroponics or seafarming by then), their services may still have a very large value for human populations in other ways.
Yet another fundamental criticism of CBA emanates from ecological research itself. It is widely acknowledged that the extreme complexity of ecosystems confers them a strong resilience, i.e., an ability to weather perturbations, but also, at the same time, a great vulnerability. Ecosystems can resist all kinds of aggressions and find ways to adapt in order to maintain their stability, but up to a certain point only. Beyond a critical threshold, one often witnesses sudden changes, which may have highly undesirable consequences for humans (Salles, 2012; Tardieu et al., 2015). The instant at which the threshold is crossed is often unpredictable, and warning signals generally occur too late. “As long as one is far from thresholds, one can perturb ecosystems in all impunity. A cost-benefit analysis at that point is useless, or a foregone conclusion, since when benefits and costs are weighed, there is apparently nothing to put on the costs side. It is because of this that humanity has been able for centuries not to care at all about the impact of its development pattern on the environment. However, as one gets near a critical threshold, cost-benefit analysis becomes irrelevant. The only thing that matters then is not to cross the threshold. Useless or irrelevant, we see that because of reasons that are not related to the temporarily insufficient nature of our knowledge, but are linked to objectives and structural properties of ecosystems, economic calculus affords very little helpful assistance. To that, one needs to add that we do not even know where the thresholds are” (Dupuy, 2002).
None of these conceptual issues with CBA has been resolved so far, even though some of them were raised more than 40 years ago. At this point, it is not clear at all that they can be resolved. Perhaps not surprisingly, therefore, CBA has not had a very good track record so far relative to environmental issues. Historically, there have been many situations where an approach based on CBA has failed. An example recounted in detail by Baveye et al. (2013) involves one of the first applications of CBA to nature's services, carried out in the late fifties and meant to identify the most profitable allocation of “unappropriated” water in the San Juan and Rio Grande basins, in New Mexico (USA). A 426-page technical report by a committee headed by Wollman (1962) detailed an extremely extensive CBA analysis of the various facets of this allocation. The conclusion, strictly on economic grounds, was that the water should be entirely devoted to industry and mining, which guaranteed a higher return than agriculture and consumption by humans. The committee ended the report by recognizing that its recommendation was not socially acceptable. Several years later, Krutilla argued similarly that “private market allocations are likely to preserve less than the socially optimal amount of natural environments” (Fisher et al., 1972).
Another key example is Clark's (1973) landmark article, in which he developed a simple mathematical model for the CBA analysis of the commercial exploitation of an animal population in the wild. His key conclusion was that, depending on certain easily stated (and quantifiable) biological and economic conditions, in particular a preference of harvesters for present over future profit, extermination of the entire population may appear to be the most attractive policy, more profitable in the short run than conservation.
Recent modeling efforts, related to soils, entirely support Clarks' conclusions. Bulte et al. (2000), for example, described a model that can be used to analyze economically-optimal nutrient (nitrogen) stocks in agricultural lands, and they applied it to study cattle ranching in humid Costa Rica. Simulation results indicate that, “for current meat prices and discount rate, it is privately optimal to “mine” soil nitrogen. In the long run, efficiency is consistent with degraded and abandoned pastures, as observed in the study region. Sustainable pasture management is economically efficient only for a discount rate close to zero or for meat prices at about twice the highest recorded value.”
In a different part of the world, in the semi-arid Sahel, Weikard and Hein (2011) have obtained similar results. These authors developed a model for livestock management based on a detailed analysis of ecosystem dynamics, and they applied it to calculate optimal and sustainable livestock stocking rates in a Sahelian rangeland. Their general model accounts for stochastic rainfall, and for the long-term impact of grazing on rangeland productivity. For the selected case study area, the model shows that the optimal stocking rate is higher than the sustainable stocking rate. Hence, with current prices, it is economically “optimal” for the pastoralist society to deplete their resource base and effectively mine the soils, which is indeed what pastors do, since the current, unsustainable stocking rate they apply exceeds the optimal stocking rate. One could argue that this is also what is currently happening with the nutrient stripping (Jones et al., 2013) that appears to often accompany the large-scale “land grab” in a number of African and South American countries (Baveye et al., 2011; Fairhead et al., 2012; Rulli et al., 2013).
Another well-publicized example of the failure of cost-benefit analysis, and generally of market economy principles, to preserve nature is related to wildlife trade. In the last decade, poaching and illegal trade have reached devastating levels that are causing a global decline not only in African elephants (Wittemyer et al., 2014), but also in rhinoceros and other wild animals. One could argue that this is not a consequence of market transactions, but of illegal trading. However, the difference between the two appears hard to pinpoint sometimes, given that large corporations routinely circumvent the law by buying politicians outright so that political decisions go their way, by lobbying elected representatives to change laws, or by trying to “rig markets for their own benefit” (Spash, 2010). According to Silvertown (2015), “since 2008 it has become clear that the financial markets are not immune to illegal and risky behavior on a scale that has threatened the stability of the entire global economy. Is it wise to stake the survival of 30,000 species on a bet that they can be saved by the market, legal or otherwise?” In this respect, Kroeger and Casey (2007) consider that in general, the risk is too large for market failure, and they reach the conclusion that “in many cases, some form of well-designed government involvement will be required to seek outcomes that protect the public interest.”
These different examples indicate clearly that applications of CBA can potentially lead to dramatic losses of sustainability. This point has been made forcefully by various authors, especially in recent years (Glenk et al., 2012). Anderson et al. (2015), for example, state unambiguously that “as currently formulated and practiced, benefit-cost analysis is incompatible with the ideology of sustainability science and should not be used to evaluate proposed solutions to sustainability problems.”
In spite of these various shortcomings of the approach, some researchers in recent years have applied cost-benefit analysis to soils (van Wezel et al., 2008; Balana et al., 2012; Bizoza and De Graaff, 2012; Sun et al., 2013; Dominati E. et al., 2014; Söderqvist et al., 2015). The number of soil functions/services considered in these articles is generally small and oriented toward agricultural production. For example, Bateman et al's (2013) analysis of ecosystem services associated with land use ignores carbon storage, hydrological services, the filtering of chemicals, aesthetic or cultural services, and envisages biodiversity only with respect to bird populations. Even Dominati E. et al. (2014), who consider a significantly larger number of functions/services and demonstrate that their inclusion markedly changes the decision-making process with CBA, ignore the potentially significant cultural services. Nevertheless, what several of these studies share, however, is a concern about the sensitivity of CBA results toward input data. As Mishra and Rai (2014) pointed out, “any CBA is based on less than perfect information regarding past and current costs and benefits, and this is even more true regarding the future.” In the case of the different soil and water conservation (SWC) measures that they considered in India, Mishra and Rai (2014) found that parameter uncertainty did not affect conclusions about the appropriateness of specific measures. By contrast, other authors found that a change in discounting rate from 4 to 12% is enough to reverse the financial attractiveness of specific SWC measures (Balana et al., 2012). Dominati E. J. et al. (2014) consider the NPV of recovered services after the planting of trees following a dramatic erosion event in New Zealand. Their conclusion is that in some cases, the NPV increases with a hike of the discounting rate from 0 to 10%, and under other scenarios, it goes from positive to negative over the same range of discounting rates. Depending on how one is disposed toward CBA, one may see in these results a clear indication that it is important to state precisely what value of the discounting rate is used in calculations. Other people may instead view this extreme sensitivity as a further nail in the coffin of CBA, since the outcome of this process depends very subjectively on how the person carrying out the calculations perceives the future.
Limitations of the Neoclassical Framework
Advocates of a monetary perspective toward soil functions/services might contend that the drawbacks of CBA may not be affecting at all the more general concepts or approaches of neoclassical economics, which they view as robust and convincing. The claim is often made, for example by Atkinson et al. (2012), that the market economy affords “powerful means of communicating the importance of conservation to a wider (and previously unreceptive) audience.” These same authors refer to the “persuasiveness of economic language” as a valuable asset when trying to convince the public to preserve nature. But, as with CBA, one may wonder whether this apparent “persuasiveness” of neoclassical economics is not actually misleading.
Part of the reason the economic discourse may appear so convincing is undoubtedly related to some of the terms that it encompasses. Since the marginalist revolution, economics has been defined as the body of knowledge relevant to the optimal allocation of scarce resources among alternative ends. The first fundamental theorem of welfare economics states that if market prices correctly reflect individual preferences, any competitive or Walrasian equilibrium leads to an allocation of resources that is efficient in the sense of Pareto, i.e., such that no further trading can increase the well-being of one person without decreasing the well-being of another (Gowdy, 2009). Not all possible Pareto-efficient allocations are necessarily equally desirable by society. Fortunately, the second fundamental theorem of welfare economics guarantees that different types of intervention, e.g., by governments, can stir the economy toward a Pareto-efficient outcome that has desired features, such as distributional equity, and market forces can take over after that.
All these references to optimality and efficiency appear really persuasive, and the high level of mathematical formalization of economic thinking ads a significant layer of scientific veneer to the edifice, but closer scrutiny reveals that many of the basic hypotheses that are needed to support most of this edifice do not have much connection with reality. In more ways than one, from a scientific perspective, the emperor has no clothes.
For example, the first fundamental theorem of welfare economics requires that all participants in economic transactions be perfectly informed about the nature of these transactions and about the conditions under which they take place. Stiglitz and Greenwald (1986) show however that since this prerequisite is met only under exceptional circumstances, markets are generally not Pareto efficient. A lack of information can sometimes be overcome by improved communication and education, but in many cases, e.g., with soil services, the lack of information relates to a fundamentally deficient understanding of numerous processes and of their practical significance. This uncertainty cannot simply be alleviated by improved communication.
A key assumption embodied in neoclassical economics is that most goods and services are fungible or substitutable. In particular, this means that every natural resource/process has an adequate substitute and is freely interchangeable with another of like nature or kind (Chee, 2004). This perspective in turn engenders technological optimism, i.e., the belief that human ingenuity and technological progress will manage to find suitable alternatives, thereby solving the problems that dwindling natural resources pose for economic growth. In reality, things are a little more complicated than that (Salles, 2012; Setten et al., 2012). For example, in the case of multifunctional systems like soils, it may be possible to find a substitute for one specific function/service that a soil renders, but experience shows that it is impossible to come up with substitutes for all functions/services at once.
Another fundamental principle of neoclassical economics is referred to as “consumer sovereignty” and corresponds to the “view that a configuration of economic activity and resource allocation should be chosen so as to satisfy to the maximum extent possible the wants of individuals” (Chee, 2004). According to the canonical model —known popularly as Homo economicus—which is central to consumer choice theory, each of these individuals behaves as a “rational actor.” This means, among other things, that he/she is self-interested and purposeful, has a single, stable, invariant set of preferences that are internally consistent, is omniscient, in particular about the likelihood of different outcomes of his/her decisions (Chee, 2004), maximizes utility, and makes decisions independently of any social or environmental context (Gowdy and Erickson, 2005). “In the most radically economistic, but still widely accepted, interpretation of this model, Homo economicus' self-interest is assumed to be primarily, if not entirely, material, concerned chiefly with increasing one's ability to consume, by amassing wealth” (Levine et al., 2015). A large body of psychological research carried out by behavioral economists over the last two decades has demonstrated that virtually every single aspect of this “canonical” model fails to represent reality adequately. For example, human wants are socially contingent, not atomistic. People make different decisions as members of a social group than they do as isolated individuals. Preferences are mutable, particularly over the long timescales that need to be considered when dealing with sustainability issues (Chee, 2004). In game theoretic simulations of consumer behavior, Homo economicus tends to be often out-competed by other species of economic actors (Gowdy and Erickson, 2005).
These criticisms of neoclassical economics, and in particular of the “rational actor” model, have been around for at least a century. To be fair, many neoclassical economists, in the research they conduct, have recognized the limitations of the traditional theory, and have by and large abandoned the concepts of economic man and perfect competition. The new neoclassical economics, consecrated by a number of Nobel prizes, is far more evidence-based and “positive” (i.e., descriptive) than its predecessor, as are also a number of alternative, heterodox approaches to economic theory, the most prominent of which is ecological economics.
Nevertheless, when it comes to policy recommendations, neoclassical economics dominates debates overwhelmingly, and the viewpoint that is adopted is entirely “normative,” i.e., focusing on how things should be, in a make-belief world based on outdated representations of human behavior and commodity production, and which does not bear much resemblance to the world we live in. As a result, “neoclassical economics continues to offer bad advice in dealing with some of the most pressing environmental and social issues faced in the twenty-first century, including income disparity, global climate change, and biodiversity loss” (Gowdy and Erickson, 2005).
Given the fact that the premises of neoclassical economics have been so “resoundingly debunked by a wide array of contemporary findings” (Levine et al., 2015), two questions seem to be in order. The first is why this school of thought continues to have such a stronghold on policy-making. The second question, which is perhaps the most pressing one in the context of this article, concerns the extent to which one should have confidence that market dynamics can sustainably manage natural resources, and soils more specifically.
Part of the response to the first question is related to the fact that, around the world, policies motivated by neoclassical economics have enabled major corporations, banks, and a select number of speculators to amass inordinate amounts of wealth over the years. This wealth in turn gives them tremendous weight in politics at all levels, especially in countries like the US where large direct financial donations by private citizens or corporations to support politicians' campaign are considered legal. This has led to a severe lack of pluralism in the discipline of economics (Norgaard, 1989), which is in turn accompanied by biases in education and news reporting, both of which keep the public in the dark. In the US and France, at least, researchers have found that the teaching of economics in many institutions focuses exclusively on neoclassical theories, and that this dominance steadily increases, still. Not only are current heterodox theories not taught, but also the history of economic thought tends to be ignored. In 2014, these two trends encouraged students from 20 different countries to create an “International Student Initiative for Pluralism in Economics” (http://www.isipe.net/open-letter/). In terms of reporting, the same spin techniques that are so successful with politicians seem to be equally effective with the economy. Mirowski (2013), for example, shows in detail how the role of neoclassical ideas (combined with incredible greed) was spun very craftily in a couple years from being considered one of the major causes of the 2008 financial debacle, to being viewed as the main reason the crisis did not end up getting much worse than it already was.
The answer to the second question, dealing with the confidence one should have in markets to manage the environment, parallels in many ways the conclusion reached earlier for CBA. Even if a market-driven outcome is efficient or optimal from the perspective of economic theory, absolutely nothing guarantees that this outcome will pass muster from other perspectives, for example in terms of ecological stability (e.g., Gowdy et al., 2013). As Pearce and Turner (1990) puts it, “Modern economics lacks what we call an existence theorem: a guarantee that any economic optimum is associated with a stable ecologic equilibrium.” In other words, it takes a leap of faith, or being blinded by ideology, to assert, as various authors have done since 1997, that nature conservation can best be achieved by assigning prices to ecosystem services and letting economic or financial market forces manage them.
Viewing Soils as Natural “Capital,” “Infrastructure,” “Matrimony,” or “Heritage”
In the last few years, attention seems to have shifted to some extent away from hard-to-valuate functions/services and toward soil structures and processes, i.e., to the soil “natural capital” that eventually produces the functions/services. Indeed, this concept, or a variant like the soil “infrastructure” (Bristow et al., 2010), has been mentioned with increasing frequency in the soil science literature over the last few years (e.g., Palm et al., 2007; Clothier et al., 2011; Robinson et al., 2012a,b, 2013a,b; Dominati E. J. et al., 2014; Hewitt et al., 2015).
In terms of raising the profile of soils in economic and financial markets, it is not entirely clear how this switch from services to the natural capital itself is going to resolve issues, since it does not overcome some of the problems mentioned earlier, in particular in terms of discounting the future. Also, almost 20 years ago already, Daily et al. (1997) cautioned that “the total value of soil is incalculable, as it includes the value of human society and of millions of other species.” Neoclassical economics is not particularly equipped to deal with infinite capital.
Yet, in another way, this focus on natural capital, and the recognition that in the case of soils, this capital is unfathomably large, may be very helpful. Indeed, this may encourage soils to be managed in the same way that has traditionally been adopted to deal with objects whose value is equally infinite. All around us, monuments are considered to be priceless treasures of humanity, and as a result escape entirely from the grasp of economic or financial markets. The Taj Mahal or the pyramids in Egypt are not, and will never be, for sale to the highest bidder. Governments at all levels have found non-economic ways to manage such “cultural capital.” One example, among many others, is the Notre Dame cathedral in Paris, acknowledged for over eight centuries as one of the most beautiful gothic cathedrals in the world. The cathedral provides a number of services, including organ music concerts and religious celebrations, to Parisians and to countless tourists visiting it every year (for free), but preservation of the building has never been tied to any monetary valuation of these services. The perspective of the French government, shared by pretty much all governments in the same situation, is that it is responsible for keeping the cathedral in good shape, so that future generations can enjoy it as much as the current one does. In other words, the cathedral is managed as a “national heritage,” a “patrimony” (or “matrimony”), to be handed down to future citizens of France.
There are clearly many aspects that distinguish soils from a historical monument, and one should not push the analogy too far. Yet, the idea that soils, globally, should be dealt with as a patrimony or societal “heritage” (Keller et al., 2012; Perrings, 2014) is appealing at several different levels. A key one, of course, is that, unlike with CBA, this perspective mandates that soils be managed sustainably. Another advantage is that, as with buildings that are on the list of national monuments in countries like France or Italy, the label of societal heritage associated with soils would give public authorities some measure of control over what private landowners do with their land. The laws in this context vary from one country to another (Bergel, 2005). Nevertheless, under current laws in many countries, landowners have the right to do as they please with the soil they own (provided they respect local zoning guidelines and the government does not need the land for specific uses). Landowners can compact, degrade, or mine their soil of all nutrients in complete impunity, regardless of the potential of soils to fulfill specific functions/services. A switch to heritage status, as for example with the recent “Pachamama” laws enacted in Bolivia and Ecuador and giving legal rights to Mother Earth, would enable governments to either take legal action when soils are not managed sustainably, or to have a say, democratically, in any major proposed change in land use.
A concept that is closely related to that of heritage or matrimony, is that of stewardship (McCallister and Nowak, 1998; Wander and Drinkwater, 2000; Raymond et al., 2013; Keith et al., 2016). Once soils are viewed as a heritage, the role of land managers becomes that of “stewards,” whose moral duty or responsibility, to use Jonas's (1984) terminology, is to ensure that soils are transmitted to the next generations in good condition.
Multi-criteria Decision Analysis and Soil Services
Regardless of whether one focuses on soil functions/services or on the heritage aspect of soil resources, decisions have to be made about the most appropriate management to be adopted. Among the non-monetary (or, at least, not exclusively monetary) approaches that are available to facilitate this process, a class of methods is experiencing a resurgence of interest in the context of soil uses. MCDA emerged as a decision-making methodology over four decades ago in the fields of operations research and mathematics. When CBA fell temporarily out of favor after the 70s as a methodology to deal with environmental issues, MCDA began to attract attention as a very attractive alternative in that context as well (Gatto and De Leo, 2000). The pendulum swung back to CBA for a while after 1997, but since none of the earlier criticisms of CBA were addressed or resolved, some of the focus has returned eventually to MCDA in the last few years. At this point, the literature dealing with the use of MCDA in environmental management is expanding rapidly (Linkov et al., 2006; Munda, 2008; Huang et al., 2011; Schwenk et al., 2012; Davies et al., 2013; Knights et al., 2013; Liu et al., 2013).
The basic idea of MCDA is to “evaluate the performance of alternative courses of action (e.g., management or policy options) with respect to criteria that capture the key dimensions of the decision-making problem (e.g., ecological, economic, and social sustainability), involving human judgment and preferences” (Saarikoski et al., 2015). The different steps in a typical MCDA consist typically of the definition of goals and objectives, identification of a set of decision options, selection of criteria for assessing performance relative to objectives, determination of weights for the various criteria, and application of procedures and mathematical algorithms for ranking options. A slightly more complete list of steps, in a participative context, is presented in Figure 3, along with different challenges that may or may not materialize at each of the steps.
Performance data accommodate a plurality of dimensions of values involved in environmental decision-making. These data may be in the form of objective metrics such as physical quantities, observed measures such as market prices, or, finally, of informed opinion of experts or stakeholders. Criteria are scored on interval or ratio scales, and are then normalized to ensure commensurability, before algorithms based on value- or utility functions, goal programming, outranking, or descriptive/multivariate statistical methods are applied to rank the options (Chee, 2004). A key feature of MCDA with respect to these criteria and ranking processes is the complete transparency with which they are handled, including some that otherwise would likely remain ignored or at least unmentioned (Rosen et al., 2015). Each of the steps in Figure 13 is carried out in the open, with all of the participants being fully aware of each others' criteria and of the assumptions that are made collectively, all of which can be revisited at later stages of the discussions, if necessary.
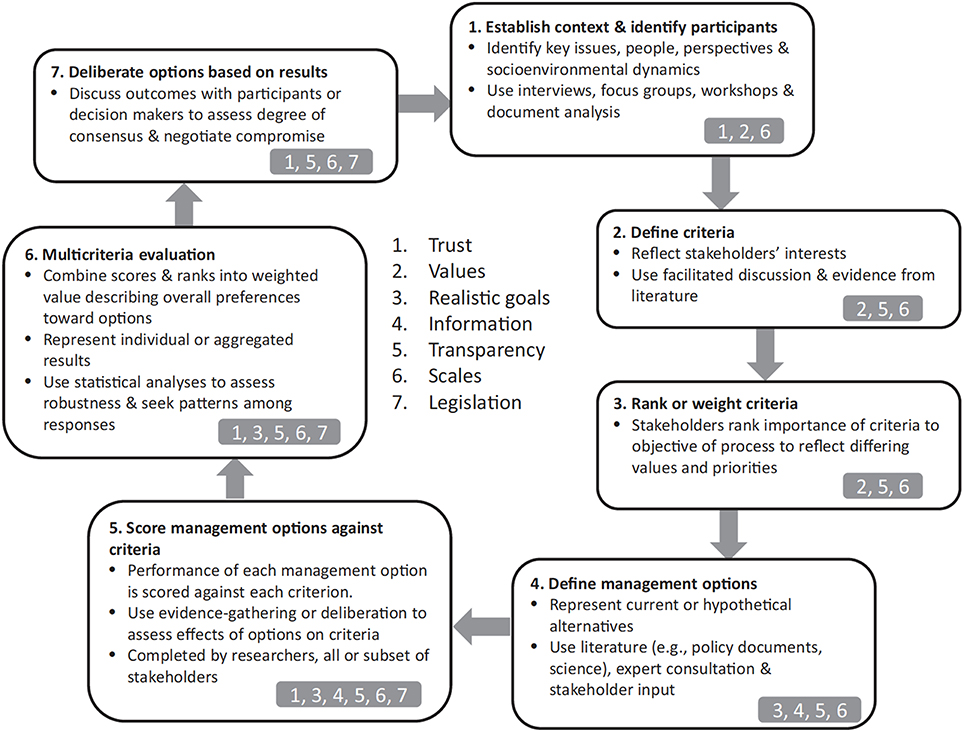
Figure 13. Schematic outline of the seven main stages of participatory multicriteria decision analysis. Key conflict challenges (numbers 1–7 at the center of the chart) can be addressed at multiple stages, and many of these conflicts are dealt with throughout the process, notably issues of scale, and the need for legislative flexibility (Modified from Davies et al., 2013).
In some cases, trade-offs between different criteria can be implemented, where a poor performance on one criterion may be compensated by a good performance on another. However, non-compensatory methods exist to deal with cases when trade-offs between different criteria should not be allowed on ethical grounds, for example, or are refused by some participants for spiritual reasons (Chan et al., 2012b). Outranking methods, like NAIADE (Munda, 2008) allow adjustments for the level of compensability of certain criteria. For example, a threshold could be set to guarantee that aquifer recharge at a particular location remains above an agreed-upon minimum, which is then subsequently excluded from trade-off with other soil functions/services.
Traditional MCDA methods used to assume that there was a single decision-maker, confronted with a number of clear, unambiguous, non-conflicting objectives. In addition, criteria were assumed to be well-defined, independent of each other, and measurable with certainty. In the last 2 decades, however, MCDA methods have been developed that alleviate some of these restrictions, and in particular can handle group decision and deliberative situations where there might be conflicting objectives (Chee, 2004). Similarly, MCDA methods have been combined with BBNs (e.g., Fenton and Neil, 2001) or fuzzy sets concepts (Bojorquez-Tapia et al., 2013) in order to take into account the uncertainty unavoidably associated with the criteria used.
In the last few years, MCDA methods have been increasingly applied to a wide range of situations directly or indirectly involving soil functions/services. Researchers have used MCDA, e.g., to choose among alternative land uses (Fontana et al., 2013; Restrepo Estrada and Alvia Ramirez, 2013; Borgogno-Mondino et al., 2015), to select among a number of flood protection options in watersheds (Porthin et al., 2013), to locate sites suitable for new landfills (Sener et al., 2006; El Baba et al., 2015), to establish sustainable industrial areas (Ruiz et al., 2012), to develop tourism, including ecotourism (Erfani et al., 2015), to assess the sustainability of alternative cropping systems (Sadok et al., 2008, 2009), to optimize the reclamation and redevelopment of brownfields and open pit mines (Bascetin, 2007; Morio et al., 2013), or to assess and map land degradation (Bojorquez-Tapia et al., 2013).
Among this expanding literature, several articles have demonstrated in great detail how MCDA allows soil functions/services to be taken into account explicitly in decision-making. In particular, Volchko et al. (2013, 2014) have shown how soil functions, as defined in the context of the EU Soil Directive, could be used as key criteria, along with various socio-economic considerations, when trying to reach a decision about the best reclamation option for contaminated brownfields. The Excel-based computer program SCORE used in the research has since been developed further and is made available (Rosen et al., 2015; Söderqvist et al., 2015).
Another example of the application of MCDA to a situation involving soil functions is the participatory effort of Rodenburg et al. (2014) to develop a methodology to take advantage of the regional agricultural potential of inland valleys in sub-Saharan Africa. Specifically, the goal is to benefit local rural livelihoods and meet regional objectives of reducing poverty and increasing food safety, while simultaneously safeguarding other inland-valley ecosystem services of local and regional importance, like biodiversity conservation, water storage, local flood and erosion control, nutrient retention, and stabilization of the micro-climate. Yet another example is the work of Teshome et al. (2014), where MCDA is used to evaluate different SWC practices used by farmers in the north-western Ethiopian highlands. Whereas, international and aid agencies tend to evaluate the benefits of SWC measures solely in financial terms (i.e., following CBA), the detailed analysis of Teshome et al. (2014), based on surveys and group discussions with local farmers, suggests that these farmers employ a wider set of criteria, including non-monetary ones and some of the least quantifiable (social and ecological) impacts of SWC practices, in their decision to adopt or not various SWC measures.
It is clear from this quick overview of the fast-growing body of literature on the use of MCDA methods to deal with soil issues, that MCDA seems to fulfill a clear need among practitioners. Their features that are most often put forth as key advantages of MCDA are that it allows the active participation of stakeholders in decision-making, makes it possible to take technical information about soil functions/services explicitly into account, can use cost-benefit types of arguments if/when suitable, but otherwise is flexible enough not to require all criteria to be expressed in monetary terms.
Take-Home Messages
This article started with a mention of the extreme enthusiasm, and very high level of research activity, centered at the moment on the “ecosystem” services of soils. The flurry of articles that have been published on this topic in the last few years, and continue to come out at a frantic pace, might suggest at a casual glance that the idea of considering and especially valuing soil services emerged only recently, as a result of a broader interest in ecosystem services that started in 1997. However, such a perspective would be misleading. The review of the literature presented above demonstrates that this is not the case at all. Soil functions and services have been the object of significant interest and in-depth reflection for the last five decades, before ecosystem services ever became fashionable. Valuation of soil services also started quite a few years ago, in the mid-80s, using methods that were developed in the 40s and 50s to associate monetary values with parks or wetlands.
Given this half-century of awareness of the multifunctionality of soils, and the even longer existence of methods to associate monetary values with services rendered by nature, it is very revealing that very little progress has been made, on a number of fronts. In particular, Eigenbrod et al. (2010) remarked that “Perhaps the greatest obstacle to substantial progress in assessing ecosystem services is a lack of data—there is simply none available for most services in most of the world.” Indeed, over the last 50 years that researchers have been discussing about the various functions of soils, precious little progress has been achieved in this area. To date, there are no direct quantitative measurements of the functions/services of soils. The very few estimates that are available in this respect result from numerous simplifying assumptions and approximations, whose soundness is difficult to assess in the absence of actual measurements, and without a detailed uncertainty analysis. Among these estimates, those based on soil service “indicators” seem particularly uncertain at this stage. In order for the use of soil functions/services in decision making to progress beyond mere rhetoric, this issue of quantitative measurements will need to be addressed.
Similarly, very few researchers have attempted so far to put any kind of price tag on non-marketed soil functions or services. The manifest reluctance of the soil science community to do so could be due to a number of different reasons (Baveye, 2015), among which are conceptual shortcomings with all the methods available in principle to evaluate soil services monetarily, the fact that soil functions or services are interdependent and (for some, at least) still poorly understood, or the current impossibility to assign prices meaningfully to a whole range of cultural, spiritual, or emotional services of soils. In addition, an analysis of cost-benefit analysis, a pillar of neoclassical economics, which would control what is done with soils services if they were assigned prices, suggests that the management of soils that would ensue from CBA may not be in the best interest of human populations at large, not to mention that it could lead to disaster for soils themselves. In addition, the idea that economic thinking, and the dynamics of financial markets, automatically results in an optimal and efficient allocation of natural resources, does not hold very long to scrutiny, in view of the very shaky foundations of the now-dominant neoclassical theory.
At this juncture, it seems logical to state, as Kelman (1981) did already many years ago, that “There are good reasons to oppose efforts to put dollar values on non-marketed benefits and costs.” Some soil scientists, however, feel uncomfortable with this viewpoint, and consider that since governmental and international agencies are asking us to provide them with prices for the different functions and services of soils, we have no real choice and it is our responsibility to deliver the requested numbers, regardless of what we may think. In the eighteenth century, Rousseau (1754) alluded to a similar dilemma, in the closely related context of private property: “The first man who, having fenced in a piece of land, said “This is mine,” and found people naïve enough to believe him, that man was the true founder of civil society. From how many crimes, wars, and murders, from how many horrors and misfortunes might not any one have saved mankind, by pulling up the stakes, or filling up the ditch, and crying to his fellows: Beware of listening to this impostor; you are undone if you once forget that the fruits of the soil belong to us all, and the soil itself to nobody.”
In this day and age, we may find that, except in some parts of the world where communal lands still subsist, the battleground now is no longer about the concept of property rights. Even though some may still view it as “perhaps the weirdest of all the undertakings of our ancestors” (Polanyi, 1944), property rights no longer are an object of discussion. However, who will own, and benefit financially, from the many services soils provide to human populations may very well be where some of the next battles are waged.
In this case as well, as in Rousseau's time, the responsible thing to do for scientists may be to cry to our fellows: “Beware of listening to the impostors who try to sell you their cost-benefit approach and have little understanding of soils.” Until now, no one has made that call explicitly, but instead, soil scientists have clearly voted with their feet. Rather than investing time and effort into monetizing soil functions or services, they have actively explored alternative, non-monetary approaches. A significant body of work has been devoted in the past decade to what Chee (2004) called for: “more comprehensive approaches for facilitating genuine, substantive stakeholder participation with opportunities for social learning, value formation, problem exploration, risk assessment, analysis of uncertainty, broad-based debate, and reconciliation of interests.” Research carried out to date demonstrates that approaches based, e.g., on MCDA or BBNs, afford many advantages over strictly Cost-Benefit Analysis, and are very effective at leading to decisions that are optimal for society as a whole.
Regardless of the approach that decision makers end up adopting to deal with the allocation of soil resources among competing demands, it seems clear that an important component of their action will have to be devoted to educating members of the public about the huge significance of soils in their lives. Only an educated citizenry, keenly aware of the complex array of functions, and services that soils fulfill, will be able to make appropriate decisions collaboratively, or to support them through democratic channels. We are arguably very far from that now. Ehrenfeld (1988) points out that the reason we need to write articles about the value that nature has for human societies shows the extent to which nature is in trouble. A century ago, “that value would have been taken for granted.” In this respect, the concept of soil function/service can serve a very useful purpose, in that it can constitute an easy and attractive entry point for didactic programs aimed at explaining how soils are structured, how we think they work, and how much uncertainty remains in our understanding of both properties and processes. Perhaps if these messages got across to a broader portion of the population, soils would be less threatened than they are now, and we would not have to constantly advocate their value.
Author Contributions
This article resulted from frequent discussions among the three authors over several years. PB did most of the writing. JB and JG made editorial comments on the whole text, and contributed particularly to the sections dealing with the economic aspects of ecosystem services.
Funding
The writing of this article was supported financially by the Kodak Endowment, made available to one of us (PB) at the Rensselaer Polytechnic Institute, and through a grant of the Métaprogramme ECOSERV of the Institut National de la Recherche Agronomique (INRA, France, grant 2015 PERSES_P10652), which allowed the organization of a research workshop on the ecosystem services of soils near Paris, in December 2015, at which many of the topics addressed in this article were discussed in depth.
Conflict of Interest Statement
The authors declare that the research was conducted in the absence of any commercial or financial relationships that could be construed as a potential conflict of interest.
Footnotes
1. ^The general public was also clearly conscious of the distinction, as illustrated by the response Oscar Wilde makes one of his characters give to the question of what a cynic is: “A man who knows the price of everything and the value of nothing.”
2. ^This comment has to be placed in context. In the early 1990s, courts were attempting to determine what local residents should receive as a monetary compensation in relation with the Exxon Valdez oil spill of 1989 near Anchorage (Alaska). The use of CVM resulted in fines considered to be abusive by Exxon Corp. and by the consultants it had hired, among them Hausman.
References
Adamowicz, W. L. (1991). Valuation of environmental amenities. Can. J. Agric. Econ. 39, 609–618. doi: 10.1111/j.1744-7976.1991.tb03612.x
Adhikari, B., and Nadella, K. (2011). Ecological economics of soil erosion: a review of the current state of knowledge. Ecol. Econ. Rev. 1219, 134–152. doi: 10.1111/j.1749-6632.2010.05910.x
Aitkenhead, M. J., Albanito, F., Jones, M. B., and Black, H. I. J. (2011). Development and testing of a process-based model (MOSES) for simulating soil processes, functions and ecosystem services. Ecol. Model. 222, 3795–3810. doi: 10.1016/j.ecolmodel.2011.09.014
Almansa, C., Calatrava, J., and Martinez-Paz, J. M. (2012). Extending the framework of the economic evaluation of erosion control actions in Mediterranean basins. Land Use Policy 29, 294–308. doi: 10.1016/j.landusepol.2011.06.013
Anderson, M., Teisl, M., Noblet, C., and Klein, S. (2015). The incompatibility of benefit-cost analysis with sustainability science. Sustain. Sci. 10, 33–41. doi: 10.1007/s11625-014-0266-4
Andersson, E., McPhearson, T., Kremer, P., Gomez-Baggethun, E., Haase, D., Tuvendal, M., et al. (2015). Scale and context dependence of ecosystem service providing units. Ecosys. Serv. 12, 157–164. doi: 10.1016/j.ecoser.2014.08.001
Andrén, O., and Balandreau, J. (1999). Biodiversity and soil functioning - from black box to can of worms? Appl. Soil Ecol. 13, 105–108.
Andrew, M. E., Wulder, M. A., Nelson, T. A., and Coops, N. C. (2015). Spatial data, analysis approaches, and information needs for spatial ecosystem service assessments: a review. GiScience Remote Sens. 52, 344–373. doi: 10.1080/15481603.2015.1033809
Ango, T. G., Borjeson, L., Senbeta, F., and Hylander, K. (2014). Balancing ecosystem services and disservices: smallholder farmers' use and management of forest and trees in an agricultural landscape in Southwestern Ethiopia. Ecol. Soc. 19:30. doi: 10.5751/ES-06279-190130
Arrow, K. J. (1950). A difficulty in the concept of social welfare. J. Polit. Econ. 58, 328–346. doi: 10.1086/256963
Atkinson, G., Bateman, I., and Mourato, S. (2012). Recent advances in the valuation of ecosystem services and biodiversity. Oxford Rev. Econ. Policy 28, 22–47. doi: 10.1093/oxrep/grs007
Attfield, R. (1998). Existence value and intrinsic value. Ecol. Econ. 24, 163–168. doi: 10.1016/S0921-8009(97)00140-7
Ausseil, A. G. E., Dymond, J. R., Kirschbaum, M. U. F., Andrew, R. M., and Parfitt, R. L. (2013). Assessment of multiple ecosystem services in New Zealand at the catchment scale. Environ. Model. Softw. 43, 37–48. doi: 10.1016/j.envsoft.2013.01.006
Baker, J. M., Ochsner, T. E., Venterea, R. T., and Griffis, T. J. (2007). Tillage and soil carbon sequestration - What do we really know? Agric. Ecosys. Environ. 118, 1–5. doi: 10.1016/j.agee.2006.05.014
Balana, B. B., Muys, B., Haregeweyn, N., Descheemaeker, K., Deckers, J., Poesen, J., et al. (2012). Cost-benefit analysis of soil and water conservation measure: the case of exclosures in northern Ethiopia. For. Policy Econ. 15, 27–36. doi: 10.1016/j.forpol.2011.09.008
Barancikova, G., and Madaras, M. (2003). Attempt to assessment of non-production soil functions - Filtration of organic contaminants. Ekologia Bratislava 22, 323–336.
Barbier, E. B. (1994). Valuing environmental functions: tropical wetlands. Land Econ. 70, 155–173. doi: 10.2307/3146319
Barbier, E. B. (2003). Habitat-fishery linkages and mangrove loss in Thailand. Contemp. Econ. Policy 21, 59–77. doi: 10.1093/cep/21.1.59
Bardgett, R. D., and van der Putten, W. H. (2014). Belowground biodiversity and ecosystem functioning. Nature 515, 505–511. doi: 10.1038/nature13855
Barnaud, C., and Antona, M. (2014). Deconstructing ecosystem services: uncertainties and controversies around a socially constructed concept. Geoforum 56, 113–123. doi: 10.1016/j.geoforum.2014.07.003
Barnaud, C., Antona, M., and Marzin, J. (2011). Vers une mise en débat des incertitudes associées à la notion de service écosystémique. Vertigo 11. doi: 10.4000/vertigo.10905. Available online at: http://vertigo.revues.org/10905
Barrett, J. E., Virginia, R. A., Wall, D. H., and Adams, B. J. (2008). Decline in a dominant invertebrate species contributes to altered carbon cycling in a low-diversity soil ecosystem. Glob. Chang. Biol. 14, 1734–1744. doi: 10.1111/j.1365-2486.2008.01611.x
Barrios, E. (2007). Soil biota, ecosystem services and land productivity. Ecol. Econ. 64, 269–285. doi: 10.1016/j.ecolecon.2007.03.004
Bascetin, A. (2007). A decision support system using analytical hierarchy process (AHP) for the optimal environmental reclamation of an open-pit mine. Environ. Geol. 52, 663–672. doi: 10.1007/s00254-006-0495-7
Bastian, C. T., McLeod, D. M., Germino, M. J., Reiners, W. A., and Blasko, B. J. (2002). Environmental amenities and agricultural land values: a hedonic model using geographic information systems data. Ecol. Econ. 40, 337–349. doi: 10.1016/S0921-8009(01)00278-6
Bastian, O., Syrbe, R. U., Rosenberg, M., Rahe, D., and Grunewald, K. (2013). The five pillar EPPS framework for quantifying, mapping and managing ecosystem services. Ecosys. Serv. 4, 15–24. doi: 10.1016/j.ecoser.2013.04.003
Basu, N. B., Rao, P. S. C., Winzeler, H. E., Kumar, S., Owens, P., and Merwade, V. (2010). Parsimonious modeling of hydrologic responses in engineered watersheds: structural heterogeneity versus functional homogeneity. Water Resour. Res. 46:W04501. doi: 10.1029/2009WR007803
Bateman, I. J., Harwood, A. R., Mace, G. M., Watson, R. T., Abson, D. J., Andrews, B., et al. (2013). Bringing ecosystem services into economic decision-making: land use in the United Kingdom. Science 341, 45–50. doi: 10.1126/science.1234379
Bateman, I. J., and Mawby, J. (2004). First impressions count: interviewer appearance and information effects in stated preference studies. Ecol. Econ. 49, 47–55. doi: 10.1016/j.ecolecon.2003.12.006
Baveye, P. (2014). Ecological economic perspective in environmental practice: much-needed common sense amid overwhelming market rhetoric. Environ. Pract. 16, 246–248. doi: 10.1017/S1466046614000179
Baveye, P., Jacobson, A. R., Allaire, S. E., Tandarich, J. P., and Bryant, R. B. (2006). Whither goes soil science in the United States and Canada? Soil Sci. 171, 501–518. doi: 10.1097/01.ss.0000228032.26905.a9
Baveye, P. C. (2009). To sequence or not to sequence the whole-soil metagenome? Nat. Rev. Microbiol. 7:756. doi: 10.1038/nrmicro2119-c2
Baveye, P. C. (2015). Grand challenges in the research on soil processes. Front. Environ. Sci. 3:10. doi: 10.3389/fenvs.2015.00010
Baveye, P. C., Baveye, J., and Gowdy, J. (2013). Monetary valuation of ecosystem services: it matters to get the timeline right. Ecol. Econ. 95, 231–235. doi: 10.1016/j.ecolecon.2013.09.009
Baveye, P. C., and Laba, M. (2015). Moving away from the geostatistical lamppost: why, where, and how does the spatial heterogeneity of soils matter? Ecol. Model. 298, 24–38. doi: 10.1016/j.ecolmodel.2014.03.018
Baveye, P. C., Rangel, D., Jacobson, A. R., Laba, M., Darnault, C., Otten, W., et al. (2011). From dust bowl to dust bowl: soils are still very much a frontier of science. Soil Sci. Soc. Am. J. 75, 2037–2048. doi: 10.2136/sssaj2011.0145
Beare, M. H., Coleman, D. C., Crossley, D. A., Hendrix, P. F., and Odum, E. P. (1995). A hierarchical approach to evaluating the significance of soil biodiversity to biogeochemical cycling. Plant Soil 170, 5–22. doi: 10.1007/BF02183051
Bennett, L. T., Mele, P. M., Annett, S., and Kasel, S. (2010). Examining links between soil management, soil health, and public benefits in agricultural landscapes: an Australian perspective. Agric. Ecosys. Environ. 139, 1–12. doi: 10.1016/j.agee.2010.06.017
Bergel, P. (2005). Appropriation de l'espace et propriété du sol. Novois 195, 17–27. doi: 10.4000/norois.479
Bergstrom, J. C., Dillman, B. L., and Stoll, J. R. (1985). Public environmental amenity benefits of private land: the case of prime agricultural land. South. J. Agric. Econ. 17, 139–149.
Beven, K., and Germann, P. (2013). Macropores and water flow in soils revisited. Water Resour. Res. 49, 3071–3092. doi: 10.1002/wrcr.20156
Bielders, C. L., and Baveye, P. (1995). Processes of structural crust formation on coarse-textured soils. Eur. J. Soil Sci. 46, 221–232. doi: 10.1111/j.1365-2389.1995.tb01830.x
Bielders, C. L., Baveye, P., Wilding, L. P., Drees, L. R., and Valentin, C. (1996). Tillage-induced spatial distribution of surface crusts on a sandy Paleustult from Togo. Soil Sci. Soc. Am. J. 60, 843–855. doi: 10.2136/sssaj1996.03615995006000030024x
Bilotta, G. S., Grove, M., and Mudd, S. M. (2012). Assessing the significance of soil erosion. Trans. Inst. Br. Geogr. 37, 342–345. doi: 10.1111/j.1475-5661.2011.00497.x
Binkley, D. (2006). “Soils in ecology and ecology in soils,” in Footprints in the Soil: People and Ideas in Soil History, ed B. Warkentin (Amsterdam: Elsevier), 259–278.
Bizoza, A. R., and De Graaff, J. (2012). Financial cost-benefit analysis of bench terraces in Rwanda. Land Degrad. Dev. 23, 103–115. doi: 10.1002/ldr.1051
Blouin, M., Sery, N., Cluzeau, D., Brun, J.-J., and Bedecarrats, A. (2013). Balkanized research in ecological engineering revealed by a bibliometric analysis of earthworms and ecosystem services. Environ. Manage. 52, 309–320. doi: 10.1007/s00267-013-0079-8
Blum, W. E. H. (1988). “Problems of soil conservation,” in Nature and Environment Series, Vol. 39. Strasbourg: Council of Europe, Steering Committee for the Conservation and Management of the Environment and Natural Habitats (CDPE).
Blum, W. E. H. (1990). The challenge of soil protection in Europe. Environ. Conserv. 17, 72–74. doi: 10.1017/S037689290001732X
Blum, W. E. H. (1993). “Soil protection concept of the Council of Europe and integrated soil research,” in Soil and Environment, Integrated Soil and Sediment Research: A Basis for Proper Protection, Vol. 1, eds H. J. P. Eijsackers and T. Hamers (Dordrecht: Kluwer Academic Publisher), 37–47.
Blum, W. E. H. (2005). Functions of soil for society and the environment. Rev. Environ. Sci. Biotechnol. 4, 75–79. doi: 10.1007/s11157-005-2236-x
Blum, W. E. H., Büsing, J., and Montanarella, L. (2004). Research needs in support of the European thematic strategy for soil protection. Trends Anal. Chem. 23, 680–685. doi: 10.1016/j.trac.2004.07.007
Blume, H.-P., Brümmer, G. W., Fleige, H., Horn, R., Kandeler, E., Kögel-Knabner, I., et al. (2016). Scheffer/Schachtschabel Soil Science. Berlin: Springer Verlag.
Blume, H.-P., Horn, R., and Thiele-Bruhn, S. (2010). Handbuch des Bodenschutzes: Bodenökologie und -belastung Vorbeugende und abwehrende Schutzmaßnahmen. Weinheim: Wiley-VCH.
Blum, W. E. H., Warkentin, B. P., and Frossard, E. (2006). “Soil, human society and the environment,” in Functions of Soils for Human Societies and the Environment, Vol. 266, eds E. Frossard, W. E. H. Blum, and B. P. Warkentin (London: The Geological Society of London), 1–8.
Bockstaller, C., Girardin, P., and vanderWerf, H. M. G. (1997). Use of agro-ecological indicators for the evaluation of farming systems. Eur. J. Agron. 7, 261–270. doi: 10.1016/S1161-0301(97)00041-5
Boeraeve, F., Dendoncker, N., Jacobs, S., Gomez-Baggethun, E., and Dufrene, M. (2015). How (not) to perform ecosystem service valuations: pricing gorillas in the mist. Biodivers. Conserv. 24, 187–197. doi: 10.1007/s10531-014-0829-9
Boivin, N., and Owoc, M. A. (2004). Soils Stones and Symbols: Cultural Perceptions of the Mineral World. London: Routledge.
Bojorquez-Tapia, L. A., Cruz-Bello, G. M., and Luna-Gonzalez, L. (2013). Connotative land degradation mapping: a knowledge-based approach to land degradation assessment. Environ. Model. Softw. 40, 51–64. doi: 10.1016/j.envsoft.2012.07.009
Borgogno-Mondino, E., Fabietti, G., and Ajmone-Marsan, F. (2015). Soil quality and landscape metrics as driving factors in a multi-criteria GIS procedure for peri-urban land use planning. Urban For. Urban Green. 14, 743–750. doi: 10.1016/j.ufug.2015.07.004
Bouma, J. (1989). “Using soil survey data for quantitative land evaluation,” in Advances in Soil Science, Vol. 9, ed B. A. Stewart (New York, NY: Springer Verlag), 177–213. doi: 10.1007/978-1-4612-3532-3_4
Bouma, J. (2002). Land quality indicators of sustainable land management across scales. Agric. Ecosys. Environ. 88, 129–136. doi: 10.1016/S0167-8809(01)00248-1
Bouma, J. (2010). Implications of the knowledge paradox for soil science. Adv. Agron. 106, 143–171. doi: 10.1016/s0065-2113(10)06004-9
Bouma, J. (2014). Soil science contributions towards sustainable development goals and their implementation: linking soil functions with ecosystem services. J. Plant Nutr. Soil Sci. 177, 111–120. doi: 10.1002/jpln.201300646
Boyd, J., and Banzhaf, S. (2007). What are ecosystem services? The need for standardized environmental accounting units. Ecol. Econ. 63, 616–626. doi: 10.1016/j.ecolecon.2007.01.002
Breure, A. M., De Deyn, G. B., Dominati, E., Eglin, T., Hedlund, K., Van Orshoven, J., et al. (2012). Ecosystem services: a useful concept for soil policy making! Curr. Opin. Environ. Sustain. 4, 578–585. doi: 10.1016/j.cosust.2012.10.010
Brevik, E. C., and Arnold, R. W. (2015). Is the traditional pedologic definition of soil meaningful in the modern context? Soil Horizons 56. doi: 10.2136/sh15-01-0002
Briones, M. J. I. (2014). Soil fauna and soil functions: a jigsaw puzzle. Front. Environ. Sci. 2:7. doi: 10.3389/fenvs.2014.00007
Bristow, K. L., Marchant, S. M., Deurer, M., and Clothier, B. E. (2010). “Enhancing the ecological infrastructure of soils,” in Proceedings of the 19th World Congress of Soil Science: Soil Solutions for a Changing World, 1-6 August 2010. Symposium 4.1.1 Valuing the Soil's Natural Capital (Brisbane, QLD),13–16.
Bromley, D. W. (1990). The ideology of efficiency - Searching for a theory of policy analysis. J. Environ. Econ. Manage. 19, 86–107. doi: 10.1016/0095-0696(90)90062-4
Brown, J. R., and MacLeod, N. D. (1996). Integrating ecology into natural resource management policy. Environ. Manage. 20, 289–296. doi: 10.1007/BF01203838
Brümmer, G. (1978). Funktionen des Bodens im Stoffhaushalt der Ökosphäre. Deutscher Rat für Landespflege: Zum ökologischen Landbau, 31, 13–20.
Brussaard, L. (2012). “Ecosystem services provided by the soil biota,” in Soil Ecology and Ecosystem Services, ed D. H. Wall (Oxford: Oxford University Press), 45–58.
Brussaard, L., Aanen, D. K., Briones, M. J. I., Decaëns, T., De Beyn, G. B., Fayle, T. M., et al. (2012). “Biogeography and phylogenetic community structure of soil invertebrate ecosystem engineers: Global to local patterns, implications for ecosystem functioning and services and global environmental change impacts,” in Soil Ecology and Ecosystem Services, ed D. H. Wall (Oxford: Oxford University Press), 201–232.
Brussaard, L., Behan-Pelletier, V. M., Bignell, D. E., Brown, V. K., Didden, W., Folgarait, P., et al. (1997). Biodiversity and ecosystem functioning in soil. Ambio 26, 563–570.
Bujnovsky, R., and Vilcek, J. (2012). Soil degradation and soil value in Slovakia - Two problems with common denominator. Agriculturae Conspectus Scientificus 76, 9–14.
Bulte, E. H., Bouman, B. A. M., Plant, R. A. J., Nieuwenhuyse, A., and Jansen, H. G. P. (2000). The economics of soil nutrient stocks and cattle ranching in the tropics: optimal pasture degradation in humid Costa Rica. Eur. Rev. Agric. Econ. 27, 207–226. doi: 10.1093/erae/27.2.207
Burgin, A., Lazar, J. G., Groffman, P. M., Gold, A. J., and Kellogg, D. Q. (2013). Balancing nitrogen retention ecosystem services and greenhouse gas disservices at the landscape scale. Ecol. Eng. 56, 26–35. doi: 10.1016/j.ecoleng.2012.05.003
Burkhard, B., Kroll, F., Mûller, F., and Windhorst, W. (2009). Landscapes capacities to provide ecosystem services – A concept for land-cover based assessments. Landscape Online 15, 1–22. doi: 10.3097/LO.200915
Cairns, J., and Niederlehner, B. R. (1994). Estimating the effects of toxicants on ecosystem services. Environ. Health Perspect. 102, 936–939. doi: 10.1289/ehp.94102936
Calvet, R. (2003). Le sol: Propriétés et Fonctions. Tome 1: Constitution et Structure, Phénomènes aux Interfaces. Paris: Editions France Agricole, Dunod.
Calzolari, C., Ungaro, F., Filippi, N., Guermandi, M., Malucelli, F., Marchi, N., et al. (2016). A methodological framework to assess the multiple contributions of soils to ecosystem services delivery at regional scale. Geoderma 261, 190–203. doi: 10.1016/j.geoderma.2015.07.013
Cammas, C. (2003). “L'architecture en terre crue à l' Âge du Fer et à l'époque romaine: apports de la discrimination micromorphologique des modes de mise en œuvre,” in Échanges Transdisciplinaires sur les Constructions en terre Crue, 1, Table Ronde de Montpellier, eds H. Guillaud, C.-A. de Chazelles, and A. Klein (Montpellier: Éditions de l'espérou), 33–53.
Cardona, A. (2012). “L'introduction de la notion de “services écosystémiques”: Pour un nouveau regard sur le sol?,” in 6ème Journées de Recherches en Sciences Sociales. Toulouse. Available online at: www.sfer.asso.fr/content/download/4204/35256/version/2/file/b1_cardona
Caride, C., Pineiro, G., and Maria Paruelo, J. (2012). How does agricultural management modify ecosystem services in the argentine Pampas? The effects on soil C dynamics. Agric. Ecosys. Environ. 154, 23–33. doi: 10.1016/j.agee.2011.05.031
Celio, E., Koellner, T., and Gret-Regamey, A. (2014). Modeling land use decisions with Bayesian networks: spatially explicit analysis of driving forces on land use change. Environ. Model. Softw. 52, 222–233. doi: 10.1016/j.envsoft.2013.10.014
Cellauro, L., and Richaud, G. (2005). Thomas Jefferson and Francois Cointereaux, professor of rural architecture in revolutionary Paris. Archit. Hist. 48, 173–206.
Chan, K. M. A., Guerry, A. D., Balvanera, P., Klain, S., Satterfield, T., Basurto, X., et al. (2012a). Where are cultural and social in ecosystem services? A framework for constructive engagement. Bioscience 62, 744–756. doi: 10.1525/bio.2012.62.8.7
Chan, K. M. A., Satterfield, T., and Goldstein, J. (2012b). Rethinking ecosystem services to better address and navigate cultural values. Ecol. Econ. 74, 8–18. doi: 10.1016/j.ecolecon.2011.11.011
Charlet, L., Chapron, Y., Faller, P., Kirsch, R., Stone, A. T., and Baveye, P. C. (2012). Neurodegenerative diseases and exposure to the environmental metals Mn, Pb, and Hg. Coord. Chem. Rev. 256, 2147–2163. doi: 10.1016/j.ccr.2012.05.012
Chee, Y. E. (2004). An ecological perspective on the valuation of ecosystem services. Biol. Conserv. 120, 549–565. doi: 10.1016/j.biocon.2004.03.028
Ciriacy-Wantrup, S. V. (1947). Capital returns from soil conservation practices. J. Farm Econ. 29, 1181–1202. doi: 10.2307/1232747
Clark, C. W. (1973). Profit maximization and extinction of species. J. Polit. Econ. 81, 950–961. doi: 10.1086/260090
Clawson, M. (1959). Methods of Measuring the Demand for and Value of Outdoor Recreation. Washington, DC: Resources for the Future.
Clothier, B. E., Hall, A. J., Deurer, M., Green, S. R., and Mackay, A. D. (2011). “Soil ecosystem services: Sustaining returns on investment into natural capital,” in Sustaining Soil Productivity in Response to Global Climate Change: Science, Policy, and Ethics, eds T. J. Sauer, J. Norman, and M. Sivakumar (New York, NY: John Wiley and Sons), 304–356.
Cohen, M. J., Brown, M. T., and Shepherd, K. D. (2006). Estimating the environmental costs of soil erosion at multiple scales in Kenya using emergy synthesis. Agric. Ecosys. Environ. 114, 249–269. doi: 10.1016/j.agee.2005.10.021
Collard, S. J., and Zammit, C. (2006). Effects of land-use intensification on soil carbon and ecosystem services in Brigalow (Acacia harpophylla) landscapes of southeast Queensland, Australia. Agric. Ecosys. Environ. 117, 185–194. doi: 10.1016/j.agee.2006.04.004
Comerford, N. B., Franzluebbers, A. J., Stromberger, M. E., Morris, L., Markewitz, D., and Moore, R. (2013). Assessment and evaluation of soil ecosystem services. Soil Horizons 54, 1–14. doi: 10.2136/sh12-10-0028
Commission of the European Communities (2006). Proposal for a Directive of the European Parliament and of the Council Establishing a Framework for the Protection of Soil and Amending Directive 2004/35/EC. Brussels.
Coomber, N. H., and Biswas, A. K. (1973). Evaluation of Environmental Intangibles. Bronxville, NY: Genera Press.
Cooper, M. (2010). Turbulent worlds: financial markets and environmental crisis. Theory Cult. Soc. 27, 167–190. doi: 10.1177/0263276409358727
Costanza, R. (2008). Ecosystem services: multiple classification systems are needed. Biol. Conserv. 141, 350–352. doi: 10.1016/j.biocon.2007.12.020
Costanza, R., de Groot, R., Sutton, P., van der Ploeg, S., Anderson, S. J., Kubiszewski, I., et al. (2014). Changes in the global value of ecosystem services. Glob. Environ. Change Hum. Policy Dimens. 26, 152–158. doi: 10.1016/j.gloenvcha.2014.04.002
Costanza, R., d'Arge, R., de Groot, R., Farber, S., Grasso, M., Hannon, B., et al. (1997). The value of the world's ecosystem services and natural capital. Nature 387, 253–260.
Crouzat, E., Mouchet, M., Turkelboom, F., Byczek, C., Meersmans, J., Berger, F., et al. (2015). Assessing bundles of ecosystem services from regional to landscape scale: insights from the French Alps. J. Appl. Ecol. 52, 1145–1155. doi: 10.1111/1365-2664.12502
Crowards, T. M. (1997). Nonuse values and the environment: economic and ethical motivations. Environ. Values 6, 143–167. doi: 10.3197/096327197776679167
Daily, G. C. (1997). “Introduction: what are ecosystem services?,” in Nature's Services: Societal Dependence on Natural Ecosystems, ed G. C. Daily (Washington, DC: Island Press), 1–10.
Daily, G. C., and Matson, P. A. (2008). Ecosystem services: from theory to implementation. Proc. Natl. Acad. Sci. U.S.A. 105, 9455–9456. doi: 10.1073/pnas.0804960105
Daily, G. C., Matson, P. A., and Vitousek, P. M. (1997). “Ecosystem services supplied by soil,” in Nature's Services: Societal Dependence on Natural Ecosystems, ed G. C. Daily (Washington, DC: Island Press), 113–132.
Dale, V. H., and Polasky, S. (2007). Measures of the effects of agricultural practices on ecosystem services. Ecol. Econ. 64, 286–296. doi: 10.1016/j.ecolecon.2007.05.009
Davidson, M. D. (2013). On the relation between ecosystem services, intrinsic value, existence value and economic valuation. Ecol. Econ. 95, 171–177. doi: 10.1016/j.ecolecon.2013.09.002
Davies, A. L., Bryce, R., and Redpath, S. M. (2013). Use of multicriteria decision analysis to address conservation conflicts. Conserv. Biol. 27, 936–944. doi: 10.1111/cobi.12090
Decaens, T., Jimenez, J. J., Gioia, C., Measey, G. J., and Lavelle, P. (2006). The values of soil animals for conservation biology. Eur. J. Soil Biol. 42, S23–S38. doi: 10.1016/j.ejsobi.2006.07.001
de Groot, R. S. (1992). Functions of Nature: Evaluation of Nature in Environmental Planning, Management and Decision Making. Groningen: Wolters-Noordhoff.
de Groot, R. S., Alkemade, R., Braat, L., Hein, L., and Willemen, L. (2010). Challenges in integrating the concept of ecosystem services and values in landscape planning, management and decision making. Ecol. Complexity 7, 260–272. doi: 10.1016/j.ecocom.2009.10.006
de Groot, R. S., Wilson, M. A., and Boumans, R. M. J. (2002). A typology for the classification, description and valuation of ecosystem functions, goods and services. Ecol. Econ. 41, 393–408. doi: 10.1016/S0921-8009(02)00089-7
deLaplante, K., and Picasso, V. (2011). “The biodiversity-ecosystem function debate in ecology,” in Philosophy of Ecology, Vol. 11, eds K. DeLaplante, B. Brown, and K. A. Peacock (Oxford: North Holland), 169–200.
Deloire, A., Vaudour, E., Carey, V., Bonnardot, V., and Van Leeuwen, C. (2005). Grapevine responses to terroir: A global approach. J. Int. Sci. Vigne Vin. 39, 149–162.
de Silva, N. R., Brooker, S., Hotez, P. J., Montresor, A., Engels, D., and Savioli, L. (2003). Soil-transmitted helminth infections: updating the global picture. Trends Parasitol. 19, 547–551. doi: 10.1016/j.pt.2003.10.002
Diamond, P. A., and Hausman, J. A. (1994). Contingent valuation - Is some number better than no number. J. Econ. Perspect. 8, 45–64. doi: 10.1257/jep.8.4.45
di Castri, F. (1970). “Major problems facing ecologists in the study of soil ecosystems (in French),” in Methods of Study in Soil Ecology, Proceedings of a Symposium Organized in Paris by Unesco and the International Biological Programme, ed J. Phillipson (Paris: Unesco), 15–31.
Diderot, D., and D'Alembert, J. L. R. (1777–1780). Encyclopédie ou Dictionnaire Raisonné des Sciences, des Arts et des Métiers par une Société de gens de Lettres (Nouvelle édition). Genève: Pellet.
Dominati, E., Mackay, A., Green, S., and Patterson, M. (2014). A soil change-based methodology for the quantification and valuation of ecosystem services from agro-ecosystems: a case study of pastoral agriculture in New Zealand. Ecol. Econ. 100, 119–129. doi: 10.1016/j.ecolecon.2014.02.008
Dominati, E., Mackay, A., and Patterson, M. (2010a). “Modelling the provision of ecosystem services from soil natural capital,” in Proceedings of the 19th World Congress of Soil Science: Soil Solutions for a Changing World, 1-6 August 2010. Congress Symposium 2: Soil Ecosystem Services (Brisbane, QLD), 32–35.
Dominati, E., Patterson, M., and Mackay, A. (2010b). A framework for classifying and quantifying the natural capital and ecosystem services of soils. Ecol. Econ. 69, 1858–1868. doi: 10.1016/j.ecolecon.2010.05.002
Dominati, E. J., MacKay, A. D., Bouna, J., and Green, S. (2016). An ecosystems approach to quantify soil performance for multiple outcomes: The future of land evaluation? Soil Sci. Soc. Am. J. 80, 438–449. doi: 10.2136/sssaj2015.07.0266
Dominati, E. J., Mackay, A., Lynch, B., Heath, N., and Millner, I. (2014). An ecosystem services approach to the quantification of shallow mass movement erosion and the value of soil conservation practices. Ecosys. Serv. 9, 204–215. doi: 10.1016/j.ecoser.2014.06.006
Douguet, J. M., and O'Connor, M. (2003). Maintaining the integrity of the French terroir: a study of critical natural capital in its cultural context. Ecol. Econ. 44, 233–254. doi: 10.1016/S0921-8009(02)00276-8
Drake, L. (1992). The non-market value of the Swedish agricultural landscape. Eur. Rev. Agric. Econ. 19, 351–364. doi: 10.1093/erae/19.3.351
Dunn, R. R. (2010). Global mapping of ecosystem disservices: the unspoken reality that nature sometimes kills us. Biotropica 42, 555–557. doi: 10.1111/j.1744-7429.2010.00698.x
Dupuy, J.-P. (2002). Pour un Catastrophisme Éclairé: Quand L'impossible est Certain. Paris: Éditions du Seuil.
Edwards-Jones, G., Davies, B., and Hussain, S. (2000). Ecological Economics: an Introduction. Oxford; Malden, MA: Blackwell Science.
Egoh, B., Reyers, B., Rouget, M., Richardson, D. M., Le Maitre, D. C., and van Jaarsveld, A. S. (2008). Mapping ecosystem services for planning and management. Agric. Ecosys. Environ. 127, 135–140. doi: 10.1016/j.agee.2008.03.013
Ehrenfeld, D. W. (1988). “Why put a value on biodiversity?,” in Biodiversity, eds E. O. Wilson and F. M. Peter (Washington, DC: National Academy Press), 212–216.
Ehrlich, P. R., and Ehrlich, A. H. (1981). Extinction: The Causes and Consequences of the Disappearance of Species. New York, NY: Random House.
Eigenbrod, F., Armsworth, P. R., Anderson, B. J., Heinemeyer, A., Gillings, S., Roy, D. B., et al. (2010). The impact of proxy-based methods on mapping the distribution of ecosystem services. J. Appl. Ecol. 47, 377–385. doi: 10.1111/j.1365-2664.2010.01777.x
El Baba, M., Kayastha, P., and De Smedt, F. (2015). Landfill site selection using multi-criteria evaluation in the GIS interface: a case study from the Gaza Strip, Palestine. Arab. J. Geosci. 8, 7499–7513. doi: 10.1007/s12517-014-1736-9
Erfani, M., Afrougheh, S., Ardakani, T., and Sadeghi, A. (2015). Tourism positioning using decision support system (case study: Chahnime-Zabol, Iran). Environ. Earth Sci. 74, 3135–3144. doi: 10.1007/s12665-015-4365-z
Faber, J. H., and van Wensem, J. (2012). Elaborations on the use of the ecosystem services concept for application in ecological risk assessment for soils. Sci. Total Environ. 415, 3–8. doi: 10.1016/j.scitotenv.2011.05.059
Fairhead, J., Leach, M., and Scoones, I. (2012). Green Grabbing: a new appropriation of nature? J. Peasant Stud. 39, 237–261. doi: 10.1080/03066150.2012.671770
Farmani, R., Henriksen, H. J., Savic, D., and Butler, D. (2012). An evolutionary Bayesian belief network methodology for participatory decision making under uncertainty: an application to groundwater management. Integr. Environ. Assess. Manag. 8, 456–461. doi: 10.1002/ieam.192
Feldman, A. (1987). “Welfare economics,” in The New Palgrave: A dictionary of Economics, eds J. Eatwell, M. Milgate, and P. K. Newman (London: MacMillan), 889–895.
Fenton, G. R. (1947). The soil fauna, with special reference to the ecosystem of forest soil. J. Anim. Ecol. 16, 76–93. doi: 10.2307/1508
Fenton, N., and Neil, M. (2001). Making decisions: using Bayesian nets and MCDA. Knowl. Based Syst. 14, 307–325. doi: 10.1016/S0950-7051(00)00071-X
Fenton, O., Vero, S., Ibrahim, T. G., Murphy, P. N. C., Sherriff, S. C., and Huallachain, D. O. (2015). Consequences of using different soil texture determination methodologies for soil physical quality and unsaturated zone time lag estimates. J. Contam. Hydrol. 182, 16–24. doi: 10.1016/j.jconhyd.2015.07.004
Ferris, H., and Tuomisto, H. (2015). Unearthing the role of biological diversity in soil health. Soil Biol. Biochem. 85, 101–109. doi: 10.1016/j.soilbio.2015.02.037
Fisher, A. C., Krutilla, J. V., and Cicchett, C. J. (1972). Economics of environmental preservation - Theoretical and empirical analysis. Am. Econ. Rev. 62, 605–619.
Fisher, B., Turner, R. K., and Morling, P. (2009). Defining and classifying ecosystem services for decision making. Ecol. Econ. 68, 643–653. doi: 10.1016/j.ecolecon.2008.09.014
Fontana, V., Radtke, A., Fedrigotti, V. B., Tappeiner, U., Tasser, E., Zerbe, S., et al. (2013). Comparing land-use alternatives: using the ecosystem services concept to define a multi-criteria decision analysis. Ecol. Econ. 93, 128–136. doi: 10.1016/j.ecolecon.2013.05.007
Foudi, S. (2012). The role of farmers' property rights in soil ecosystem services conservation. Ecol. Econ. 83, 90–96. doi: 10.1016/j.ecolecon.2012.08.015
Francesconi, W., Srinivasan, R., Perez-Minana, E., Willcock, S. P., and Quintero, M. (2016). Using the Soil and Water Assessment Tool (SWAT) to model ecosystem services: a systematic review. J. Hydrol. 535, 625–636. doi: 10.1016/j.jhydrol.2016.01.034
Freckman, W. D., Blackburn, T. H., Brussaard, L., Hutchings, P., Palmer, M. A., and Snelgrove, P. V. R. (1997). Linking biodiversity and ecosystem functioning of soils and sediments. Ambio 26, 556–562.
Frede, H. G., Bach, M., Fohrer, N., and Breuer, L. (2002). Interdisciplinary modeling and the significance of soil functions. J. Plant Nutr. Soil Sci. 165, 460–467. doi: 10.1002/1522-2624(200208)165:4<460::AID-JPLN460>3.0.CO;2-B
Freeman, M. (1993). The Measurement of Environmental and Resource Values: Theory and Methods. Washington, DC: Resources for the future.
Fromm, O., and Bruggemann, R. (1999). Economic methods of ecosystem valuation - The soil example. Part III: Economic valuation for the future: the discounting problem (in German). Umweltwissenschaften Schadstoff Forschung 11, 235–240. doi: 10.1007/BF03038039
Gaba, S., Lescourret, F., Boudsocq, S., Enjalbert, J., Hinisger, P., Journet, E.-P., et al. (2015). Multiple cropping systems as drivers for providing multiple ecosystem services: from concepts to design. Agron. Sustain. Dev. 35, 607–623. doi: 10.1007/s13593-014-0272-z
Garcia-Llorente, M., Martin-Lopez, B., Diaz, S., and Montes, C. (2012). Can ecosystem properties be fully translated into service values? An economic valuation of aquatic plant services. Ecol. Appl. 21, 3083–3103. doi: 10.1890/10-1744.1
Garrigues, E., Corson, M. S., Angers, D. A., van der Werf, H. M. G., and Walter, C. (2013). Development of a soil compaction indicator in life cycle assessment. Int. J. Life Cycle Assess. 18, 1316–1324. doi: 10.1007/s11367-013-0586-0
Gatto, M., and De Leo, G. A. (2000). Pricing biodiversity and ecosystem services: the never-ending story. Bioscience 50, 347–355. doi: 10.1641/0006-3568(2000)050[0347:PBAEST]2.3.CO;2
Ghiselin, J. (1978). Perils of the orderly mind: cost-benefit analysis and other logical pitfalls. Environ. Manage. 2, 295–300.
Giger, M. (2006). “Soil and land in the millenium ecosystem assessment and the Rio conventions,” in Soils on the Global Agenda. Developing International Mechanisms for Sustainable Land Management, eds H. Hurni, M. Giger, and K. Meyer (Bern: Geographica Bernensis), 37–39.
Gignoux, J., Davies, I. D., Flint, S. R., and Zucker, J.-D. (2012). The ecosystem in practice: interest and problems of an old definition for constructing ecological models. Ecosystems 14, 1039–1054. doi: 10.1007/s10021-011-9466-2
Glaesner, N., Helming, K., and de Vries, W. (2014). Do current European policies prevent soil threats and support soil functions? Sustainability 6, 9538–9563. doi: 10.3390/su6129538
Glendell, M., Granger, S. J., Bol, R., and Brazier, R. E. (2014). Quantifying the spatial variability of soil physical and chemical properties in relation to mitigation of diffuse water pollution. Geoderma 214, 25–41. doi: 10.1016/j.geoderma.2013.10.008
Glenk, K., McVittie, A., and Moran, D. (2012). Soil and Soil Organic Carbon within an Ecosystem Services Approach Linking Biophysical and Economic Data. Cupar: Scottish Agricultural College.
Gowdy, J. (2009). Microeconomic Theory Old and New: A Student's Guide. Stanford, CA: Stanford Economics and Finance.
Gowdy, J., and Erickson, J. D. (2005). The approach of ecological economics. Cam. J. Econ. 29, 207–222. doi: 10.1093/cje/bei033
Gowdy, J., Krall, L., and Chen, Y. (2013). The parable of the bees: beyond proximate causes in ecosystem service valuation. Environ. Ethics 35, 41–55. doi: 10.5840/enviroethics20133515
Gowdy, J. M. (1997). The value of biodiversity: markets, society, and ecosystems. Land Econ. 73, 25–41. doi: 10.2307/3147075
Gowdy, J. M., Howarth, R. B., and Tisdell, C. (2010). “Discounting, ethics, and options for maintaining biodiversity and ecosystem integrity,” in The Economics of Ecosystems and Biodiversity: Ecological and Economic Foundations, ed P. Kumar (London: Earthscan), 257–283.
Graves, A. R., Morris, J., Deeks, L. K., Rickson, R. J., Kibblewhite, M. G., Harris, J. A., et al. (2015). The total costs of soil degradation in England and Wales. Ecol. Econ. 119, 399–413. doi: 10.1016/j.ecolecon.2015.07.026
Grêt-Regamey, A., Brunner, S. H., Altwegg, J., and Bebi, P. (2013). Facing uncertainty in ecosystem services-based resource management. J. Environ. Manage. 127, S145–S154. doi: 10.1016/j.jenvman.2012.07.028
Grêt-Regamey, A., Weibel, B., Bagstad, K. J., Ferrari, M., Geneletti, D., Klug, H., et al. (2014). On the effects of scale for ecosystem services mapping. PLoS ONE 9:e112601. doi: 10.1371/journal.pone.0112601
Guerry, A. D., Polasky, S., Lubchenco, J., Chaplin-Kramer, R., Daily, G. C., Griffin, R., et al. (2015). Natural capital and ecosystem services informing decisions: from promise to practice. Proc. Natl. Acad. Sci. U.S.A. 112, 7348–7355. doi: 10.1073/pnas.1503751112
Guillaud, H. (1997). Une Grande Figure du Patrimoine Régional Rhône-Alpes: François Cointereaux (1740–1830) - Pionnier de la Construction Moderne en pisé. Les Carnets de l'Architecture de Terre, Monographie Numéro 3. Villefontaine: CRATerre-EAG; École Nationale Supérieure d'Architecture de Grenoble.
Haines-Young, R. (2011). Exploring ecosystem service issues across diverse knowledge domains using Bayesian Belief Networks. Prog. Phys. Geogr. 35, 681–699. doi: 10.1177/0309133311422977
Halstead, J. M. (1984). Measuring the nonmarket value of Massachusetts agricultural land: a case study. J. Northeast. Agric. Econ. Counc. 13, 12–19.
Hanley, N. (1992). Are there environmental limits to cost benefit analysis? Environ. Resour. Econ. 2, 33–59.
Hanley, N., Spash, C., and Walker, L. (1995). Problems in valuing the benefits of biodiversity protection. Envir. Res. Econ. 5, 249–272. doi: 10.1007/BF00691519
Hausman, J. (2012). Contingent valuation: from dubious to hopeless. J. Econ. Perspect. 26, 43–56. doi: 10.1257/jep.26.4.43
Haygarth, P. M., and Ritz, K. (2009). The future of soils and land use in the UK: soil systems for the provision of land-based ecosystem services. Land Use Policy 26, S187–S197. doi: 10.1016/j.landusepol.2009.09.016
Hedlund, K., and Harris, J. (2012). “Delivery of ecosystem services: From Gaia to genes,” in Soil Ecology and Ecosystem Services, ed D. H. Wall (Oxford: Oxford University Press), 98–109.
Hein, L., van Koppen, K., de Groot, R. S., and van Ierland, E. C. (2006). Spatial scales, stakeholders and the valuation of ecosystem services. Ecol. Econ. 57, 209–228. doi: 10.1016/j.ecolecon.2005.04.005
Hellec, F., Brives, H., Blanchart, E., Deverre, C., Garnier, P., Payet, V., et al. (2015). L'évolution des sciences du sol face à l'émergence de la notion de service écosystémique. Étude Gestion Sols 22, 101–115.
Henriksen, H. J., Zorrilla-Miras, P., de la Hera, A., and Brugnach, M. (2012). Use of Bayesian belief networks for dealing with ambiguity in integrated groundwater management. Integr. Environ. Assess. Manag. 8, 430–444. doi: 10.1002/ieam.195
Herrmann, B. (2006). Man is made of mud: “Soil,” bio-logical facts and fiction, and environmental history. Die Bodenkultur 57, 215–230.
Hewitt, A., Dominati, E., Webb, T., and Cuthill, T. (2015). Soil natural capital quantification by the stock adequacy method. Geoderma 241, 107–114. doi: 10.1016/j.geoderma.2014.11.014
Hines, R. (1991). On valuing nature. Account. Audit. Account. J. 4, 27–29. doi: 10.1108/09513579110144802
Hockley, N. (2014). Cost-benefit analysis: a decision-support tool or a venue for contesting ecosystem knowledge? Environ. Plan. C Gov. Policy 32, 283–300. doi: 10.1068/c1384j
Hodder, K. H., Newton, A. C., Cantarello, E., and Perrella, L. (2014). Does landscape-scale conservation management enhance the provision of ecosystem services? Int. J. Biodivers. Sci. Ecosys. Serv. Manage. 10, 71–83. doi: 10.1080/21513732.2014.883430
Hopmans, J. W. (2007). A plea to reform soil science education. Soil Sci. Soc. of Am. J. 71, 639–640. doi: 10.2136/sssaj2007.0083l
Horrocks, C. A., Dungait, J. A. J., Cardenas, L. M., and Heal, K. V. (2014). Does extensification lead to enhanced provision of ecosystems services from soils in UK agriculture? Land Use Policy 38, 123–128. doi: 10.1016/j.landusepol.2013.10.023
Hotelling, H. (1949). “Letter to the director of national park service,” in The Economics of Public Recreation: The Prewitt Report, ed R. A. Prewitt (Washington, DC: Department of Interior).
Huang, I. B., Keisler, J., and Linkov, I. (2011). Multi-criteria decision analysis in environmental sciences: ten years of applications and trends. Sci. Total Environ. 409, 3578–3594. doi: 10.1016/j.scitotenv.2011.06.022
Hueting, R. (1970). Wat is de Natuur ons Waard? Een Econoom Over Milieuverslechtering. Amsterdam: Het wereldvenster/baarn.
Humphries, D., Sara, N., Boakye, D., Wilson, M., and Cappello, M. (2012). The promise and pitfalls of mass drug administration to control intestinal helminth infections. Curr. Opin. Infect. Dis. 25, 584–589. doi: 10.1097/QCO.0b013e328357e4cf
Iniesta-Arandia, I., Garcia-Llorente, M., Aguilera, P. A., Montes, C., and Martin-Lopez, B. (2014). Socio-cultural valuation of ecosystem services: uncovering the links between values, drivers of change, and human well-being. Ecol. Econ. 108, 36–48. doi: 10.1016/j.ecolecon.2014.09.028
Jacobson, A. R., Militello, R., and Baveye, P. C. (2009). Development of computer-assisted virtual field trips to support multidisciplinary learning. Comput. Educ. 52, 571–580. doi: 10.1016/j.compedu.2008.11.007
Jaillard, B., Rapaport, A., Harmand, J., Brauman, A., and Nunan, N. (2014). Community assembly effects shape the biodiversity-ecosystem functioning relationships. Funct. Ecol. 28, 1523–1533. doi: 10.1111/1365-2435.12267
Jax, K. (2005). Function and “functioning” in ecology: what does it mean? Oikos 111, 641–648. doi: 10.1111/j.1600-0706.2005.13851.x
Jax, K., Barton, D. N., Chan, K. M. A., de Groot, R., Doyle, U., Eser, U., et al. (2013). Ecosystem services and ethics. Ecol. Econ. 93, 260–268. doi: 10.1016/j.ecolecon.2013.06.008
Jax, K., and Heink, U. (2015). Searching for the place of biodiversity in the ecosystem services discourse. Biol. Conserv. 191, 198–205. doi: 10.1016/j.biocon.2015.06.032
Jiang, M., Lu, X. G., Xu, L. S., Chu, L. J., and Tong, S. Z. (2007). Flood mitigation benefit of wetland soil - A case study in momoge national nature reserve in China. Ecol. Econ. 61, 217–223. doi: 10.1016/j.ecolecon.2006.10.019
Johnson, K. A., Polasky, S., Nelson, E., and Pennington, D. (2012). Uncertainty in ecosystem services valuation and implications for assessing land use tradeoffs: an agriculural case study in the Minnesota River Basin. Ecol. Econ. 79, 71–79. doi: 10.1016/j.ecolecon.2012.04.020
Jonas, H. (1984). The Imperative of Responsibility: In Search of Ethics for the Technological Age. Chicago: University of Chicago Press.
Jones, D. L., Cross, P., Withers, P. J. A., DeLuca, T. H., Robinson, D. A., Quilliam, R. S., et al. (2013). Nutrient stripping: the global disparity between food security and soil nutrient stocks. J. Appl. Ecol. 50, 851–862. doi: 10.1111/1365-2664.12089
Kallis, G., Gomez-Baggethun, E., and Zografos, C. (2013). To value or not to value? That is not the question. Ecol. Econ. 94, 97–105. doi: 10.1016/j.ecolecon.2013.07.002
Karl, J. (1997). Soil evaluation in landscape planning. Method of assessing impact on the soil and the soil water balance. Naturschutz Landschaftsplanung 29, 5–17.
Karsenty, A., and Ezzine de Blas, D. (2014). “Du mésusage des métaphores: Les paiements pour services environnementaux sont-ils des instruments de marchandisation de la nature?,” in L'instrumentation de l'Action Publique: Controverses, Résistances, Effets, eds C. Halpern, P. Lascoumes, and P. Le Galès (Paris: Presse des Sciences Po), 161–189.
Keesstra, S. D., Geissen, V., Mosse, K., Piirainen, S., Scudiero, E., Leistra, M., et al. (2012). Soil as a filter for groundwater quality. Curr. Opin. Environ. Sustain. 4, 507–516. doi: 10.1016/j.cosust.2012.10.007
Keith, A. M., Schmidt, O., and McMahon, B. J. (2016). Soil stewardship as a nexus between ecosystem services and one health. Ecosys. Serv. 17, 40–42. doi: 10.1016/j.ecoser.2015.11.008
Keller, C., Lambert-Habib, M.-L., Robert, S., Ambrosi, J.-P., and Rabot, É. (2012). Méthodologie pour la prise en compte des sols dans les documents d'urbanisme: application à deux communes du bassin minier de Provence. Sud Ouest Eur. 33, 11–24. doi: 10.4000/soe.173
Kenter, J. O., Hyde, T., Christie, M., and Fazey, I. (2011). The importance of deliberation in valuing ecosystem services in developing countries-evidence from the Solomon Islands. Glob. Environ. Change Hum. Policy Dimens. 21, 505–521. doi: 10.1016/j.gloenvcha.2011.01.001
Kenter, J. O., O'Brien, L., Hockley, N., Ravenscroft, N., Fazey, I., Irvine, K. N., et al. (2015). What are shared and social values of ecosystems? Ecol. Econ. 111, 86–99. doi: 10.1016/j.ecolecon.2015.01.006
Kibblewhite, M. G., Ritz, K., and Swift, M. J. (2008). Soil health in agricultural systems. Philos. Trans. R. Soc. B Biol. Sci. 363, 685–701. doi: 10.1098/rstb.2007.2178
Kirchhoff, T. (2012). Pivotal cultural values of nature cannot be integrated into the ecosystem services framework. Proc. Natl. Acad. Sci. U.S.A. 109, E3146–E3146. doi: 10.1073/pnas.1212409109
Kline, J., and Wichelns, D. (1996). Measuring public preferences for the environmental amenities provided by farmland. Eur. Rev. Agric. Econ. 23, 421–436. doi: 10.1093/erae/23.4.421
Knetsch, J. L. (2005). Gains, losses, and the US-EPA Economic analyses guidelines: a hazardous product? Environ. Resour. Econ. 32, 91–112. doi: 10.1007/s10640-005-6029-z
Knights, P., Admiraal, J., Wossink, A. N., Banerjee, P., O'Neill, J., and Scott, M. (2013). Economic Environmental Valuation: An Analysis of Limitations and Alternatives - Biomot Project Deliverable 1.1. Brussels: European Commission.
Konarska, K. M., Sutton, P. C., and Castellon, M. (2002). Evaluating scale dependence of ecosystem service valuation: a comparison of NOAA-AVHRR and Landsat TM datasets. Ecol. Econ. 41, 491–507. doi: 10.1016/S0921-8009(02)00096-4
Kovda, V. A., Bugrovskii, V. V., Kerzhentsev, A. S., and Zelenskaia, N. N. (1990). A model of organic matter transformation in soil for quantitative study of soil functions in ecosystems. Dokl. Akad. Nauk SSSR 312, 759–762.
Kroeger, T., and Casey, F. (2007). An assessment of market-based approaches to providing ecosystem services on agricultural lands. Ecol. Econ. 64, 321–332. doi: 10.1016/j.ecolecon.2007.07.021
Kumar, P. (2010). The Economics of Ecosystems and Biodiversity: Ecological and Economic Foundations. London: Earthscan.
Kumar, P., Brondizio, E., Gatzweiler, F., Gowdy, J., de Groot, R., Pascual, U., et al. (2013). The economics of ecosystem services: from local analaysis to national policies. Curr. Opin. Environ. Sustain. 5, 78–86. doi: 10.1016/j.cosust.2013.02.001
Kuo, W. L., Steenhuis, T. S., McCulloch, C. E., Mohler, C. L., Weinstein, D. A., and Swaney, D. P. (1999). Effect of grid size on runoff and soil moisture for a variable-source-area hydrology model. Water Resour. Res. 35, 3419–3428. doi: 10.1029/1999WR900183
Lal, R., Lorenz, K., Hüttl, R. F., Schneider, B. U., and von Braun, J. (2013). Ecosystem Services and Carbon Sequestration in the Biosphere. Dordrecht: Springer.
Lalucq, A., and Black, W. K. (2015). Les Banquiers Contre les Banques: Le Rôle de la Criminalité en col Blanc Dans les Crises Financières. Paris: Les Petits Matins/Veblen Institute.
Lamarque, P., Lavorel, S., Mouchet, M., and Quétier, F. (2014). Plant trait-based models identify direct and indirect effects of climate change on bundles of grassland ecosystem services. Proc. Natl. Acad. Sci. U.S.A. 111, 13751–13756. doi: 10.1073/pnas.1216051111
Landuyt, D., Broekx, S., D'Hondt, R., Engelen, G., Aertsens, J., and Goethals, P. L. M. (2013). A review of Bayesian belief networks in ecosystem service modelling. Environ. Model. Softw. 46, 1–11. doi: 10.1016/j.envsoft.2013.03.011
Landuyt, D., Van der Biest, K., Broekx, S., Staes, J., Meire, P., and Goethals, P. L. M. (2015). A GIS plug-in for Bayesian belief networks: towards a transparent software framework to assess and visualise uncertainties in ecosystem service mapping. Environ. Model. Softw. 71, 30–38. doi: 10.1016/j.envsoft.2015.05.002
Laterra, P., Orue, M. E., and Booman, G. C. (2012). Spatial complexity and ecosystem services in rural landscapes. Agric. Ecosyst. Environ. 154, 56–67. doi: 10.1016/j.agee.2011.05.013
Laurans, Y., and Aoubid, S. (2011). “L'économie au secours de la biodiversité? La légende des Catskills revisitée,” in Working Paper, Vol. 14. Paris: Institut du Développement Durable et des Relations Internationales (IDDRI), Sciences Po.
Laurans, Y., and Mermet, L. (2014). Ecosystem services economic valuation, decision-support system or advocacy? Ecosys. Serv. 7, 98–105. doi: 10.1016/j.ecoser.2013.10.002
Laurans, Y., Rankovic, A., Bille, R., Pirard, R., and Mermet, L. (2013). Use of ecosystem services economic valuation for decision making: Questioning a literature blindspot. J. Environ. Manage. 119, 208–219. doi: 10.1016/j.jenvman.2013.01.008
Lautenbach, S., Maes, J., Kattwinkel, M., Seppelt, R., Strauch, M., Scholz, M., et al. (2012). Mapping water quality-related ecosystem services: concepts and applications for nitrogen retention and pesticide risk reduction. Int. J. Biodivers. Sci. Ecosys. Serv. Manage. 8, 35–49. doi: 10.1080/21513732.2011.631940
Lavelle, P. (2000). Ecological challenges for soil science. Soil Sci. 165, 73–86. doi: 10.1097/00010694-200001000-00009
Lavelle, P., Decaens, T., Aubert, M., Barot, S., Blouin, M., Bureau, F., et al. (2006). Soil invertebrates and ecosystem services. Eur. J. Soil Biol. 42, S3–S15. doi: 10.1016/j.ejsobi.2006.10.002
Lavelle, P., Lattaud, C., Trigo, D., and Barois, I. (1995). Mutualism and biodiversity in soils. Plant Soil 170, 23–33. doi: 10.1007/BF02183052
Lehmann, A., David, S., and Stahr, K. (2008). Technique for Soil Evaluation and Categorization for Natural and Anthropogenic Soils. Series Hohenheimer Bodenkundliche Hefte, Vol. 86. Hohenheim: Universität Hohenheim; Institut für Bodenkunde und Standortstlehre.
Lehmann, A., and Stahr, K. (2010). The potential of soil functions and planner-oriented soil evaluation to achieve sustainable land use. J. Soils Sediments 10, 1092–1102. doi: 10.1007/s11368-010-0207-5
Leh, M. D. K., Matlock, M. D., Cummings, E. C., and Nalley, L. L. (2013). Quantifying and mapping multiple ecosystem services change in West Africa. Agric. Ecosys. Environ. 165, 6–18. doi: 10.1016/j.agee.2012.12.001
Leitch, J. A., and Scott, D. F. (1977). A Selected Annotated Bibliography of Economic Values of Fish and Wildlife and their Habitats. Agricultural Economics Miscellaneous Report No. 27, Department of Agricultural Economics, North Dakota State University, Fargo, North Dakota.
Lele, S., Springate-Baginski, O., Lakerveld, R., Deb, D., and Dashe, P. (2013). Ecosystem services: origins, contributions, pitfalls, and alternatives. Conserv. Soc. 11, 343–358. doi: 10.4103/0972-4923.125752
Letey, J., Sojka, R. E., Upchurch, D. R., Cassel, D. K., Olson, K. R., Payne, W. A., et al. (2003). Deficiencies in the soil quality concept and its application. J. Soil Water Conserv. 58, 180–187.
Lettens, S., Van Orshoven, J., van Wesemael, B., De Vos, B., and Muys, B. (2005). Stocks and fluxes of soil organic carbon for landscape units in Belgium derived from heterogeneous data sets for 1990 and 2000. Geoderma 127, 11–23. doi: 10.1016/j.geoderma.2004.11.001
Levine, J., Chan, K. M. A., and Satterfield, T. (2015). From rational actor to efficient complexity manager: exorcising the ghost of Homo economicus with a unified synthesis of cognition research. Ecol. Econ. 114, 22–32. doi: 10.1016/j.ecolecon.2015.03.010
Liang, S.-Y., Lehmann, A., Wu, K.-N., and Stahr, K. (2014). Perspectives of function-based soil evaluation in land-use planning in China. J. Soils Sediments 14, 10–22. doi: 10.1007/s11368-013-0787-y
Li, F., Wang, R., Hu, D., Ye, Y., Yang, W., and Liu, H. (2014). Measurement methods and applications for beneficial and detrimental effects of ecological services. Ecol. Indic. 47, 102–111. doi: 10.1016/j.ecolind.2014.06.032
Linkov, I., Satterstrom, F. K., Kiker, G., Seager, T. P., Bridges, T., Gardner, K. H., et al. (2006). Multicriteria decision analysis: a comprehensive decision approach for management of contaminated sediments. Risk Anal. 26, 61–78. doi: 10.1111/j.1539-6924.2006.00713.x
Lin, Q. F. (2014). Aldo Leopold's unrealized proposals to rethink economics. Ecol. Econ. 108, 104–114. doi: 10.1016/j.ecolecon.2014.10.018
Liu, S., Crossman, N. D., Nolan, M., and Ghirmay, H. (2013). Bringing ecosystem services into integrated water resources management. J. Environ. Manage. 129, 92–102. doi: 10.1016/j.jenvman.2013.06.047
Ljung, K., Maley, F., Cook, A., and Weinstein, P. (2009). Acid sulfate soils and human health-a millennium ecosystem assessment. Environ. Int. 35, 1234–1242. doi: 10.1016/j.envint.2009.07.002
Logsdon, R. A., and Chaubey, I. (2013). A quantitative approach to evaluating ecosystem services. Ecol. Model. 257, 57–65. doi: 10.1016/j.ecolmodel.2013.02.009
Loreau, M. (2010). Linking biodiversity and ecosystems: towards a unifying ecological theory. Philos. Trans. R. Soc. B Biol. Sci. 365, 49–60. doi: 10.1098/rstb.2009.0155
Luck, G. W., Chan, K. M. A., Eser, U., Gomez-Baggethun, E., Matzdorf, B., Norton, B., et al. (2012). Ethical considerations in on-ground applications of the ecosystem services concept. Bioscience 62, 1020–1029. doi: 10.1525/bio.2012.62.12.4
Lyytimaki, J. (2015). Ecosystem disservices: embrace the catchword. Ecosys. Serv. 12, 136–136. doi: 10.1016/j.ecoser.2014.11.008
Lyytimäki, J., Petersen, L. K., Normander, B., and Bezák, P. (2008). Nature as nuisance? Ecosystem services and disservices to urban lifestyle. Environ. Sci. 5, 161–172. doi: 10.1080/15693430802055524
Mace, G. M., Norris, K., and Fitter, A. H. (2012). Biodiversity and ecosystem services: a multilayered relationship. Trends Ecol. Evol. 27, 19–26. doi: 10.1016/j.tree.2011.08.006
Madena, K., Bormann, H., and Giani, L. (2012). Soil functions - Today's situation and further development under climate change. Arch. Sci. Geogr. 66, 221–237. doi: 10.3112/erdkunde.2012.03.03
Mandel, J. T., Donlan, C. J., and Armstrong, J. (2010). A derivative approach to endangered species conservation. Front. Ecol. Environ. 8, 44–49. doi: 10.1890/070170
Martins, M. D. R., and Angers, D. A. (2015). Different plant types for different soil ecosystem services. Geoderma 237, 266–269. doi: 10.1016/j.geoderma.2014.09.013
Maslow, A. H. (1943). A theory of human motivation. Psychol. Rev. 50, 370–396. doi: 10.1037/h0054346
Ma, S., and Swinton, S. M. (2011). Valuation of ecosystem services from rural landscapes using agricultural land prices. Ecol Econ. 70, 1649–1659. doi: 10.1016/j.ecolecon.2011.04.004
McAfee, K. (1999). Selling nature to save it? Biodiversity and green developmentalism. Environ. Plan. D Soc. Space 17, 133–154. doi: 10.1068/d170133
McAfee, K. (2012). The contradictory logic of global ecosystem services markets. Dev. Change 43, 105–131. doi: 10.1111/j.1467-7660.2011.01745.x
McBratney, A., Field, D. J., and Koch, A. (2014). The dimensions of soil security. Geoderma 213, 203–213. doi: 10.1016/j.geoderma.2013.08.013
McCallister, R., and Nowak, P. (1998). “Whole-soil knowledge and management: a foundation of soil quality,” in Soil Quality and Soil Erosion, ed R. Lal (Ankeny, IA: CRC Press), 173–193.
McDonnell, J. J., Sivapalan, M., Vaché, K., Dunn, S., Grant, G., Haggerty, R., et al. (2007). Moving beyond heterogeneity and process complexity: a new vision for watershed hydrology. Water Resour. Res. 43:W07301. doi: 10.1029/2006WR005467
McNeill, J. R., and Winiwarter, A. (2004). Breaking the sod: humankind, history, and soil. Science 304, 1627–1629. doi: 10.1126/science.1099893
Meersmans, J., De Ridder, F., Canters, F., De Baets, S., and Van Molle, M. (2008). A multiple regression approach to assess the spatial distribution of Soil Organic Carbon (SOC) at the regional scale (Flanders, Belgium). Geoderma 143, 1–13. doi: 10.1016/j.geoderma.2007.08.025
Menon, M., Rousseva, S., Nikolaidis, N. P., van Gaans, P., Panagos, P., Souza, D. M., et al. (2014). SoilTrEC: a global initiative on critical zone research and integration. Environ. Sci. Pollut. Res. 21, 3191–3195. doi: 10.1007/s11356-013-2346-x
Menzie, C. A., Deardorff, T., Booth, P., and Wickwire, T. (2012). Refocusing on nature: holistic assessment of ecosystem services. Integr. Environ. Assess. Manag. 8, 401–411. doi: 10.1002/ieam.1279
Milcu, A. I., Hanspach, J., and Fischer, J. (2013). Cultural ecosystem services: a literature review and prospects for future research. Ecol. Soc. 18, 44. doi: 10.5751/ES-05790-180344
Millennium Ecosystem Assessment (2005). Ecosystems and Human Well-Being. Washington, DC: Island Press.
Millikan, R. G. (1989). An ambiguity in the notion of function. Biol. Philos. 4, 172–176. doi: 10.1007/BF00127747
Minamikawa, K., Nishimura, S., Sawamoto, T., Nakajima, Y., and Yagi, K. (2010). Annual emissions of dissolved CO2, CH4, and N2O in the subsurface drainage from three cropping systems. Glob. Chang. Biol. 16, 796–809. doi: 10.1111/j.1365-2486.2009.01931.x
Mirowski, P. (2013). Never Let a Serious Crisis go to Waste: How Neoliberalism Survived the Financial Meltdown. London: Verso.
Mishra, P. K., and Rai, S. C. (2014). A cost-benefit analysis of indigenous soil and water conservation measures in Sikkim Himalaya, India. Mt. Res. Dev. 34, 27–35. doi: 10.1659/MRD-JOURNAL-D-12-00013.1
Mitchell, R. C., and Carson, R. T. (1989). Using Surveys to Value Public Goods: The Contingent Valuation Method. Washington, DC: Resources for the Future.
Morel, J. L., Chenu, C., and Lorenz, K. (2014). Ecosystem services provided by soils of urban, industrial, traffic, mining, and military areas (SUITMAs). J. Soils Sediments 15, 1659–1666. doi: 10.1007/s11368-014-0926-0
More, T. A., Averill, J. R., and Stevens, T. H. (1996). Values and economics in environmental management: a perspective and critique. J. Environ. Manage. 48, 397–409. doi: 10.1006/jema.1996.0086
Morio, M., Schadler, S., and Finkel, M. (2013). Applying a multi-criteria genetic algorithm framework for brownfield reuse optimization: improving redevelopment options based on stakeholder preferences. J. Environ. Manage. 130, 331–346. doi: 10.1016/j.jenvman.2013.09.002
Mulder, C., Boit, A., Bonkowski, M., De Ruiter, P. C., Mancinelli, G., Van der Heijden, M. G. A., et al. (2011). “A belowground perspective on Dutch agroecosystems: how soil organisms interact to support ecosystem services,” Adv. Ecol. Res. 44, 277–357.
Munda, G. (2008). Social Multi-Criteria Evaluation for a Sustainable Economy. Berlin: Springer-Verlag.
Murray-Rust, D., Dendoncker, N., Dawson, T. P., Acosta-Michlik, L., Karali, E., Guillem, E., et al. (2011). Conceptualising the analysis of socio-ecological suystems through ecosystem services and agent-based modelling. J. Land Use Sci. 6, 83–99. doi: 10.1080/1747423X.2011.558600
Nagel, E. (1961). The Structure of Science: Problems in the Logic of Scientific Explanation. New York, NY: Harcourt, Brace and World.
Nahmani, J., van Oort, F., Lapied, E., Rouland, C., and Lavelle, P. (2003). The effects of metals on the soil ecosystems. Biofutur, 40–42.
Norgaard, R. B. (1989). The case for methodological pluralism. Ecol. Econ. 1, 37–57. doi: 10.1016/0921-8009(89)90023-2
Norgaard, R. B. (2010). Ecosystem services: from eye-opening metaphor to complexity blinder. Ecol. Econ. 69, 1219–1227. doi: 10.1016/j.ecolecon.2009.11.009
Nortcliff, S. (2002). Standardisation of soil quality attributes. Agric. Ecosyst. Environ. 88, 161–168. doi: 10.1016/S0167-8809(01)00253-5
O'Neill, R. V. (2001). Is it time to bury the ecosystem concept? (With full military honors of course!). Ecology 82, 3275–3284. doi: 10.1890/0012-9658(2001)082[3275:IITTBT]2.0.CO;2
O'sullivan, L., Creamer, R. E., Fealy, R., Lanigan, G., Simo, I., Fenton, O., et al. (2015). Functional land management for managing soil functions: a case-study of the trade-off between primary productivity and carbon storage in response to the intervention of drainage systems in Ireland. Land Use Policy 47, 42–54. doi: 10.1016/j.landusepol.2015.03.007
Oliver, M. A. (1997). Soil and human health: a review. Eur. J. Soil Sci. 48, 573–592. doi: 10.1046/j.1365-2389.1997.00124.x
Oosterbroek, B., de Kraker, J., Huynen, M. M. T. E., and PimMartens, P. (2016). Assessing ecosystem impacts on health: a tool review. Ecosyst. Serv. 17, 237–254. doi: 10.1016/j.ecoser.2015.12.008
Orenstein, D. (2013). More than language is needed in valuing ecosystem services. Bioscience 63, 913–913. doi: 10.1525/bio.2013.63.12.17
Ottoy, S., Beckers, V., Jacxsens, P., Hermy, M., and Van Orshoven, J. (2015). Multi-level statistical soil profiles for assessing regional soil organic carbon stocks. Geoderma 253, 12–20. doi: 10.1016/j.geoderma.2015.04.001
Palm, C., Sanchez, P., Ahamed, S., and Awiti, A. (2007). Soils: a contemporary perspective. Ann. Rev. Environ. Res. 32, 99–129. doi: 10.1146/annurev.energy.31.020105.100307
Parks, S., and Gowdy, J. M. (2013). What have economists learned about valuing nature? A review essay. Ecosyst. Serv. 3, e1–e10. doi: 10.1016/j.ecoser.2012.12.002
Pascual, U., Termansen, M., Hedlund, K., Brussaard, L., Faber, J. H., Foudi, S., et al. (2015). On the value of soil biodiversity and ecosystem services. Ecosyst. Serv. 15, 11–18. doi: 10.1016/j.ecoser.2015.06.002
Pearce, D. (1976). Limits of cost-benefit analysis as a guide to environmental policy. Kyklos 29, 97–112. doi: 10.1111/j.1467-6435.1976.tb01962.x
Pearce, D., Atkinson, G., and Mourato, S. (2006). Cost-Benefit Analysis and the Environment: Recent Developments. Paris: OECD Publishing.
Pearce, D. W., and Turner, R. K. (1990). Economics of Natural Resources and the Environment. Baltimore, MA: The Johns Hopkins University Press.
Pepper, I. L., Gerba, C. P., Newby, D. T., and Rice, C. W. (2009). Soil: a public health threat or savior? Crit. Rev. Environ. Sci. Technol. 39, 416–432. doi: 10.1080/10643380701664748
Perrings, C. (2014). Our Uncommon Heritage: Biodiversity Change, Ecosystem Services, and Human Wellbeing. Cambridge, UK: Cambridge University Press.
Pesche, D. (2013). Le millennium ecosystem assessment: anatomie d'une evaluation environnementale globale. Nat. Sci. Soc. 21, 363–372. doi: 10.1051/nss/2014001
Peukert, S., Bol, R., Roberts, W., Macleod, C. J. A., Murray, P. J., Dixon, E. R., et al. (2012). Understanding spatial variability of soil properties: a key step in establishing field- to farm-scale agro-ecosystem experiments. Rapid Commun. Mass Spectrom. 26, 2413–2421. doi: 10.1002/rcm.6336
Philippot, L., Spor, A., Henault, C., Bru, D., Bizouard, F., Jones, C. M., et al. (2013). Loss in microbial diversity affects nitrogen cycling in soil. ISME J. 7, 1609–1619. doi: 10.1038/ismej.2013.34
Pimentel, D., Harvey, C., Resosudarmo, P., Sinclair, K., Kurz, D., McNair, M., et al. (1995). Environmental and economic costs of soil erosion and conservation benefits. Science 267, 1117–1123. doi: 10.1126/science.267.5201.1117
Pimentel, D., Wilson, C., McCullum, C., Huang, R., Dwen, P., Flack, J., et al. (1997). Economic and environmental benefits of biodiversity. Bioscience 47, 747–757. doi: 10.2307/1313097
Polanyi, K. (1944). The Great Transformation: The Political and Economic Origins of our Time. Boston, MA: Beacon Press.
Polasky, S., and Segerson, K. (2009). Integrating ecology and economics in the study of ecosystem services: some lessons learned. Ann. Rev. Resour. Econ. 1, 409–434. doi: 10.1146/annurev.resource.050708.144110
Porthin, M., Rosqvist, T., Perrels, A., and Molarius, R. (2013). Multi-criteria decision analysis in adaptation decision-making: a flood case study in Finland. Reg. Environ. Change 13, 1171–1180. doi: 10.1007/s10113-013-0423-9
Power, A. G. (2012). Ecosystem services and agriculture: tradeoffs and synergies. Philos. Trans. R. Soc. B. Biol. Sci. 365, 2959–2971. doi: 10.1098/rstb.2010.0143
President's Council for Sustainable Development (1996). A New Consensus for the Prosperity, Opportunity and a Healthy Environment for the Future. Washington, DC. Available online at: http://clinton2.nara.gov/PCSD/Publications/TF_Reports/amer-top.html (Accessed May 29, 2016).
Pulleman, M., Creamer, R., Hamer, U., Helder, J., Pelosi, C., Peres, G., et al. (2012). Soil biodiversity, biological indicators and soil ecosystem services-an overview of European approaches. Curr. Opin. Environ. Sustainability 4, 529–538. doi: 10.1016/j.cosust.2012.10.009
Rampazzo, N., Blum, W. E. H., and Wimmer, B. (1998). Assessment of soil structure parameters and functions in agricultural soils. Bodenkultur 49, 69–84.
Raymond, C. M., Singh, G. G., Benessaiah, K., Bernhardt, J. R., Levine, J., Nelson, H., et al. (2013). Ecosystem services and beyond: using multiple metaphors to understand human-environment relationships. Bioscience 63, 536–546. doi: 10.1525/bio.2013.63.7.7
Redford, K. H., and Adams, W. M. (2009). Payment for ecosystem services and the challenge of saving nature. Conserv. Biol. 23, 785–787. doi: 10.1111/j.1523-1739.2009.01271.x
Reed, M. S., Hubacek, K., Bonn, A., Burt, T. P., Holden, J., Stringer, L. C., et al. (2013). Anticipating and managing future trade-offs and complementarities between ecosystem services. Ecol. Soc. 18:5. doi: 10.5751/ES-04924-180105
Renison, D., Hensen, I., Suarez, R., Cingolani, A. M., Marcora, P., and Giorgis, M. A. (2010). Soil conservation in Polylepis mountain forests of Central Argentia: is livesotck reducing our natural capital? Aust. Ecol. 35, 435–443. doi: 10.1111/j.1442-9993.2009.02055.x
Restrepo Estrada, C., and Alvia Ramirez, M. (2013). Optimal land use under environmental and economical approaches (in Spanish). Rev. Fac. Ing. Univ. Antioquia 69, 229–243.
Ridker, R. G., and Henning, J. A. (1967). The determinants of residential property values with special reference to air pollution. Rev. Econ. Stat. 49, 246–257. doi: 10.2307/1928231
Ritz, K., Black, H. I. J., Campbell, C. D., Harris, J. A., and Wood, C. (2009). Selecting biological indicators for monitoring soils: a framework for balancing scientific and technical opinion to assist policy development. Ecol. Indic. 9, 1212–1221. doi: 10.1016/j.ecolind.2009.02.009
Robbins, M., Olewiler, N., and Robinson, M. (2009). An Estimate of the Public Amenity Benefits and Ecological Goods Provided by Farmland in Metro Vancouver. Vancouver, BC: Fraser Basin Council.
Robertson, M. (2012). Functions, Services and Values. Wetlandia. Available online at: http://wetlandia.blogspot.co.uk/2012/07/functions-services-and-values.html (Accessed 29 May 2016).
Robert, M. (2005). “La Resource en Sols: Menaces, Nouveaux Enjeux et Mesures de Protection,” in Sols et Environnement, eds M. C. Girard, C. Walter, J. C. Rémy, J. Berthelin and J. L. Morel (Paris, Dunod), 833–858.
Robinson, D. A., Emmett, B. A., Reynolds, B., Rowe, E. C., Spurgeon, D., Keith, A. M., et al. (2012a). “Soil natural capital and ecosystem service delivery in a world of global soil change,” in Soils and Food Security, eds R. E. Hester and R. M. Harrison (London: Royal Society of Chemistry), 41–68.
Robinson, D. A., Fraser, I., Dominati, E. J., Davidsdottir, B., Jonsson, J. O. G., Jones, L., et al. (2014). On the value of soil resources in the context of natural capital and ecosystem service delivery. Soil Sci. Soc. Am. J. 78, 685–700. doi: 10.2136/sssaj2014.01.0017
Robinson, D. A., Hockley, N., Cooper, D. M., Emmett, B. A., Keith, A. M., Lebron, I., et al. (2013a). Natural capital and ecosystem services, developing an appropriate soils framework as a basis for valuation. Soil Biol. Biochem. 57, 1023–1033. doi: 10.1016/j.soilbio.2012.09.008
Robinson, D. A., Hockley, N., Dominati, E., Lebron, I., Scow, K. M., Reynolds, B., et al. (2012b). Natural capital, ecosystem services, and soil change: why soil science must embrace an ecosystems approach. Vadose Zone J. 11. doi: 10.2136/vzj2011.0051
Robinson, D. A., Jackson, B. M., Clothier, B. E., Dominati, E. J., Marchant, S. C., Cooper, D. M., et al. (2013b). Advances in soil ecosystem services: concepts, models, and applications for earth system life support. Vadose Zone J. 12. doi: 10.2136/vzj2013.01.0027
Robinson, D. A., and Lebron, I. (2010). On the natural capital and ecosystem services of soils. Ecol. Econ. 70, 137–138. doi: 10.1016/j.ecolecon.2010.08.012
Robinson, D. A., Lebron, I., and Vereecken, H. (2009). On the definition of the natural capital of soils: a framework for description, evaluation, and monitoring. Soil Sci. Soc. Am. J. 73, 1904–1911. doi: 10.2136/sssaj2008.0332
Rodenburg, J., Zwart, S. J., Kiepe, P., Narteh, L. T., Dogbe, W., and Wopereis, M. C. S. (2014). Sustainable rice production in African inland valleys: seizing regional potentials through local approaches. Agric. Syst. 123, 1–11. doi: 10.1016/j.agsy.2013.09.004
Rodrigues, S. M., Pereira, M. E., da Silva, E. F., Hursthouse, A. S., and Duarte, A. C. (2009). A review of regulatory decisions for environmental protection: part I - challenges in the implementation of national soil policies. Environ. Int. 35, 202–213. doi: 10.1016/j.envint.2008.08.007
Rosen, L., Back, P. E., Soderqvist, T., Norrman, J., Brinkhoff, P., Norberg, T., et al. (2015). SCORE: a novel multi-criteria decision analysis approach to assessing the sustainability of contaminated land remediation. Sci. Total Environ. 511, 621–638. doi: 10.1016/j.scitotenv.2014.12.058
Rositano, F., and Ferraro, D. O. (2014). Ecosystem services provided by agroecosystems: a qualitative and quantitative assessment of this relatiionship in the Pampa region, Argentina. Environ. Manage. 53, 606–619. doi: 10.1007/s00267-013-0211-9
Rousseau, J.-J. (1754) (reprinted in 1992). Discours sur l'Origine et les Fondements de l'inégalité parmi les hommes. Paris: Garnier-Flammarion.
Rouvellac, E. (2008). Le concept de Terroir, existence, définition et adéquation avec la viticulture. Historiens et Géographes 404, 79–90.
Ruiz, M. C., Romero, E., Perez, M. A., and Fernandez, I. (2012). Development and application of a multi-criteria spatial decision support system for planning sustainable industrial areas in Northern Spain. Autom. Constr. 22, 320–333. doi: 10.1016/j.autcon.2011.09.009
Rulli, M. C., Saviori, A., and D'Odorico, P. (2013). Global land and water grabbing. Proc. Natl. Acad. Sci. U.S.A. 110, 892–897. doi: 10.1073/pnas.1213163110
Rumpel, C., and Koegel-Knabner, I. (2011). Deep soil organic matter-a key but poorly understood component of terrestrial C cycle. Plant Soil 338, 143–158. doi: 10.1007/s11104-010-0391-5
Rutgers, M., van Wijnen, H. J., Schouten, A. J., Mulder, C., Kuiten, A. M. P., Brussaard, L., et al. (2012). A method to assess ecosystem services developed from soil attributes with stakeholders and data of four arable farms. Sci. Total Environ. 415, 39–48. doi: 10.1016/j.scitotenv.2011.04.041
Saarikoski, H., Barton, D. N., Mustajoki, J. H K, Gomez-Baggethun, E., and Langemeyer, J. (2015). “Multi-criteria decision analysis (MCDA) in ecosystem service valuation,” in OpenNESS Ecosystem Service Reference Book, Vol. EC FP7, eds M. Potschin and K. Jax Brussels (Belgium: European Commission) Grant Agreement no. 308428. Available online at: http://www.openness-project.eu/sites/default/files/SP_MCDA.pdf (Accessed 29 May 2016).
Sadoddin, A., Letcher, R. A., Jakeman, A. J., Croke, B., and Newham, L. T. H. (2009). “Bayesian Network Modelling for assessing the biophysical and socio-economic impacts of dryland salinity management,” in 18th World Imacs Congress and Modsim09 International Congress on Modelling and Simulation: Interfacing Modelling and Simulation with Mathematical and Computational Sciences (Cairns, QLD), 3273–3279.
Sadok, W., Angevin, F., Bergez, J. E., Bockstaller, C., Colomb, B., Guichard, L., et al. (2008). Ex ante assessment of the sustainability of alternative cropping systems: implications for using multi-criteria decision-aid methods. A review. Agron. Sustainable Dev. 28, 163–174. doi: 10.1051/agro:2007043
Sadok, W., Angevin, F., Bergez, J. E., Bockstaller, C., Colomb, B., Guichard, L., et al. (2009). MASC, a qualitative multi-attribute decision model for ex ante assessment of the sustainability of cropping systems. Agron. Sustainable Dev. 29, 447–461. doi: 10.1051/agro/2009006
Sagoff, M. (2005). The Catskills parable: a billion dollar misunderstanding. PERC Reports 23. Available online at: http://www.perc.org/articles/catskills-parable (Accessed 29 May 2016).
Sagoff, M. (2007). The Economy of the Earth: Philosophy, Law and the Environment, 2nd Edn. Cambridge, UK: Cambridge University Press.
Salisbury, R. B. (2012). Engaging with soil, past and present. J. Mater. Cult. 17, 23–41. doi: 10.1177/1359183511432990
Salles, J.-M. (2012). Valuing biodiversity and ecosystem services: why put economic values on Nature? C. R. Biol. 334, 469–482. doi: 10.1016/j.crvi.2011.03.008
Sanchez-Canales, M., Lopez Benito, A., Passuello, A., Terrado, M., Ziv, G., Acuna, V., et al. (2012). Sensitivity analysis of ecosystem service valuation in a Mediterranean watershed. Sci. Total Environ. 440, 140–153. doi: 10.1016/j.scitotenv.2012.07.071
Sandbrook, C. G., and Burgess, N. D. (2015). Biodiversity and ecosystem services: not all positive. Ecosyst. Serv. 12, 29–29. doi: 10.1016/j.ecoser.2014.12.006
Sandelin, B., Trautwein, H.-M., and Wundrak, R. (2014). A Short History of Economic Thought. Milton Park, UK: Routledge.
Sandel, M. (2012). What Money Can't Buy: The Moral Limits of markets. New York, NY: Farrar, Straus and Giroux.
Santra, P., Das, B. S., and Chakravarty, D. (2011). Delineation of hydrologically similar units in a watershed based on fuzzy classification of soil hydraulic properties. Hydrol. Process. 25, 64–79. doi: 10.1002/hyp.7820
SCEP (1970). Man's Impact on the Global Environment: Assessment and Recommendations for Action. Report of the Study of Critical Environmental Problems. The Massachusetts Institute of Technology, Cambridge, MA.
Schenker, M. (2000). Exposures and health effects from inorganic agricultural dusts. Environ. Health Perspect. 108, 661–664. doi: 10.1289/ehp.00108s4661
Schlichting, E. (1972). Böden puffern Umwelteinflüsse ab (Soil buffering from environmental influences). Umsch. Wiss. Tech. 72, 50–52.
Schouten, A. J., Brussaard, L., de Ruiter, P. C., Siepel, H., and van Stralen, N. M. (1997). An Indicator System for Life Support Functions of the Soil in Relation to Biodiversity. Bilthoven: Rijskinstituut voor volksgezonheid en milieu.
Schroeder, D., and Lamp, J. (1976). Prinzipein der Aufstellung von Boden klassifikationssystemen (Principles for construction of soil classification systems) Z. Pflanzenernaehrung und Bodenkunde 5, 617–630.
Schulte, R. P. O., Creamer, R. E., Donnellan, T., Farrelly, N., Fealy, R., O'Donoghue, C., et al. (2014). Functional land management: a framework for managing soil-based ecosystem services for the sustainable intensification of agriculture. Environ. Sci. Policy 38, 45–58. doi: 10.1016/j.envsci.2013.10.002
Schwenk, W. S., Donovan, T. M., Keeton, W. S., and Nunery, J. S. (2012). Carbon storage, timber production, and biodiversity: comparing ecosystem services with multi-criteria decision analysis. Ecol. Appl. 22, 1612–1627. doi: 10.1890/11-0864.1
Semenov, A. V., Pereira e Silva, M. C., Salles, J. F., Schmitt, H., and van Elsas, J. D. (2014). Quantitative assessment of soil functioning across a representative range of Dutch soils. Ecol. Indic. 39, 88–93. doi: 10.1016/j.ecolind.2013.11.009
Sener, B., Suzen, M. L., and Doyuran, V. (2006). Landfill site selection by using geographic information systems. Environ. Geol. 49, 376–388. doi: 10.1007/s00254-005-0075-2
Sepúlveda, M., Rousseliere, H., Val Elslande, E., Arriaza, B., Standen, V., Santoro, C. M., et al. (2014). Study of color pigments associated to archaic chinchorro mummies and grave goods in Northern Chile (7000–3500 B. P.). Herit. Sci. 2, 7. doi: 10.1186/2050-7445-2-7
Setala, H., Bardgett, R. D., Birkhofer, K., Brady, M., Byrne, L., de Ruiter, P. C., et al. (2014). Urban and agricultural soils: conflicts and trade-offs in the optimization of ecosystem services. Urban Ecos. 17, 239–253. doi: 10.1007/s11252-013-0311-6
Setten, G., Stenseke, M., and Moen, J. (2012). Ecosystem services and landscape management: three challenges and one plea. Int. J. Biodivers. Sci. Ecos. Serv. Manage. 8, 305–312. doi: 10.1080/21513732.2012.722127
Shackleton, C. M., Ruwanza, S., Sinasson Sanni, G. K., Bennett, S., De Lacy, P., Modipa, R., et al. (2016). Unpacking pandora's box: understanding and categorising ecosystem disservices for environmental management and human wellbeing. Ecosystems. 19, 587–600. doi: 10.1007/s10021-015-9952-z
Shapiro, J., and Báldi, A. (2014). Accurate accounting: how to balance ecosystem services and disservices. Ecosyst. Serv. 7, 201–202. doi: 10.1016/j.ecoser.2014.01.002
Silvertown, J. (2015). Have ecosystem services been oversold? Trends Ecol. Evol. 30, 641–648. doi: 10.1016/j.tree.2015.08.007
Simonson, R. W. (1966). Shifts in the usefulness of soil resources in the USA. Agriculture 23, 11–15.
Simpson, R. D. (2011). The “Ecosystem Service Framework”: A critical Assessment. Nairobi: The United Nations Environment Programme.
Simpson, R. D., and Christensen, N. L. (1997). Ecosystem Function and Human Activities. New York, NY: Chapman and Hall, International Thomson Publishing.
Smith, A. (1776) (reprinted 1977). An Inquiry into the Nature Causes of the Wealth of Nations. Chicago, IL: University of Chicago Press.
Söderqvist, T., Brinkhoff, P., Norberg, T., Rosén, L., Back, P.-E., and Norrman, J. (2015). Cost-benefit analysis as a part of sustainability assessment of remediation alternatives for contaminated land. J. Environ. Manage. 157, 267–278. doi: 10.1016/j.jenvman.2015.04.024
Sojka, R. E., and Upchurch, D. R. (1999). Reservations regarding the soil quality concept. Soil Sci. Soc. Am. J. 63, 1039–1054. doi: 10.2136/sssaj1999.6351039x
Song, X.-Z., Zhao, C.-X., Wang, X.-L., and Li, J. (2009). Study of nitrate leaching and nitrogen fate under intensive vegetable production pattern in northern China. C. R. Biol. 332, 385–392. doi: 10.1016/j.crvi.2008.11.005
Spangenberg, J. H., Görg, C., Truong, D. T., Tekken, V., Bustamante, J. V., and Settele, J. (2014). Provision of ecosystem services is determined by human agency, not ecosystem functions. Four case studies. Int. J. Biodivers. Sci. Ecosyst. Serv. Manage. 10, 40–53. doi: 10.1080/21513732.2014.884166
Spash, C. L. (2010). The Brave New World of carbon trading. New Pol. Econ. 15, 169–195. doi: 10.1080/13563460903556049
Staubach, S. (2005). Clay: The History and Evolution of Humankind's Relationship with Earth's Most Primal Element. New York, NY: Berkley Books.
Steinnes, E. (2009). Soils and geomedicine. Environ. Geochem. Health 31, 523–535. doi: 10.1007/s10653-009-9257-2
Stiglat, K. (2012). Aus der Frühzeit des Betonbaus. Bautechnik 89, 484–491. doi: 10.1002/bate.201201549
Stiglitz, J. E., and Greenwald, B. C. (1986). Externalities in economies with imperfect information and incomplete markets. Q. J. Econ. 101, 229–264. doi: 10.2307/1891114
Strivelli, R. A., Krzic, M., Crowley, C., Dyanatkar, S., Bomke, A. A., Simard, S. W., et al. (2011). Integration of problem-based learning and web-based multimedia to enhance a soil management course. J. Nat. Res. Life Sci. Educ. 40, 215–223. doi: 10.4195/jnrlse.2010.0032n
Sullivan, S. (2013). Banking nature? The spectacular financialisation of environmental conservation. Antipode 45, 198–217. doi: 10.1111/j.1467-8330.2012.00989.x
Sun, L., Lu, W., Yang, Q., Delgado Martin, J., and Li, D. (2013). Ecological compensation estimation of soil and water conservation based on cost-benefit analysis. Water Res. Manage. 27, 2709–2727. doi: 10.1007/s11269-013-0268-5
Sunstein, C. R. (2005). Cost-benefit analysis and the environment. Ethics 115, 351–385. doi: 10.1086/426308
Sunstein, C. R., and Rowell, A. (2007). On discounting regulatory benefits: Risk, money, and Intergenerational equity. Univ. Chic. Law Rev. 74, 171–208.
Taalab, K., Corstanje, R., Zawadzka, J., Mayr, T., Whelan, M. J., Hannam, J. A., et al. (2015). On the application of bayesian networks in digital soil mapping. Geoderma 259/260, 134–148. doi: 10.1016/j.geoderma.2015.05.014
Tancoigne, E., Barbier, M., Cointet, J.-P., and Richard, G. (2013). Les Services Écosystémiques Dans la Littérature Scientifique: Démarche D'exploration et Résultats D'analyse. Rapport D'étude Pour la Phase D'exploration du Métaprogramme EcoServ. Paris: INRA.
Tardieu, L., Roussel, S., Thompson, J. D., Labarraque, D., and Salles, J.-M. (2015). Combining direct and indirect impacts to assess ecosystem service loss due to infrastructure construction. J. Environ. Manage. 152, 145–157. doi: 10.1016/j.jenvman.2015.01.034
Telles, T. S., Falci Dechen, S. C., Antonio de Souza, L. G., and Guimaraes, M. D. F. (2013). Valuation and assessment of soil erosion costs. Sci. Agric. 70, 209–216. doi: 10.1590/S0103-90162013000300010
Tengberg, A., Fredholm, S., Eliasson, I., Knez, I., Saltzman, K., and Wetterberg, O. (2012). Cultural ecosystem services provided by landscapes: assessment of heritage values and identity. Ecosyst. Serv. 2, 14–26. doi: 10.1016/j.ecoser.2012.07.006
Teshome, A., de Graaff, J., and Stroosnijder, L. (2014). Evaluation of soil and water conservation practices in the north-western Ethiopian highlands using multi-criteria analysis. Front. Environ. Sci. 2:60. doi: 10.3389/fenvs.2014.00060
Thomsen, M., Faber, J. H., and Sorensen, P. B. (2012). Soil ecosystem health and services - Evaluation of ecological indicators susceptible to chemical stressors. Ecol. Indic. 16, 67–75. doi: 10.1016/j.ecolind.2011.05.012
Toman, M. (1998). Why not to calculate the value of the world's ecosystem services and natural capital. Ecol. Econ. 25, 57–60. doi: 10.1016/S0921-8009(98)00017-2
Troldborg, M., Aalders, I., Towers, W., Hallett, P. D., McKenzie, B. M., Bengough, A. G., et al. (2013). Application of bayesian belief networks to quantify and map areas at risk to soil threats: using soil compaction as an example. Soil Tillage Res. 132, 56–68. doi: 10.1016/j.still.2013.05.005
Tsonkova, P., Böhm, C., Quinkenstein, A., and Freese, D. (2015). Application of partial order ranking to identify enhancement potentials for the provision of selected ecosystem services by different land use strategies. Agric. Syst. 135, 112–121. doi: 10.1016/j.agsy.2015.01.002
Turner, K. G., Anderson, S., Gonzales-Chang, M., Costanza, R., Courville, S., Dalgaard, T., et al. (2016). A review of methods, data, and models to assess changes in the value of ecosystem services from land degradation and restoration. Ecol. Modell. 319, 190–207. doi: 10.1016/j.ecolmodel.2015.07.017
Turner, R. K., and Daily, G. C. (2008). The ecosystem services framework and natural capital conservation. Environ. Res. Econ. 39, 25–35. doi: 10.1007/s10640-007-9176-6
Tzilivakis, J., Lewis, K. A., and Williamson, A. R. (2005). A prototype framework for assessing risks to soil functions. Environ. Impact Assess. Rev. 25, 181–195. doi: 10.1016/j.eiar.2004.02.003
Ungaro, F., Calzolari, C., Pistocchi, A., and Malucelli, F. (2014a). Modelling the impact of increasing soil sealing on runoff coefficients at regional scale: a hydropedological approach. J. Hydrol. Hydromech. 62, 33–42. doi: 10.2478/johh-2014
Ungaro, F., Zasada, I., and Piorr, A. (2014b). Mapping landscape services, spatial synergies and trade-offs. A case study using variogram models and geostatistical simulations in an agrarian landscape in North-East Germany. Ecol. Indic. 46, 367–378. doi: 10.1016/j.ecolind.2014.06.039
Valentin, C., and Bresson, L. M. (1992). Morphology, genesis and classification of surface crusts in loamy and sandy soils. Geoderma 55, 225–245. doi: 10.1016/0016-7061(92)90085-L
van Eekeren, N., de Boer, H., Hanegraaf, M., Bokhorst, J., Nierop, D., Bloem, J., et al. (2010). Ecosystem services in grassland associated with biotic and abiotic soil parameters. Soil Biol. Biochem. 42, 1491–1504. doi: 10.1016/j.soilbio.2010.05.016
van Wensem, J. (2013). Use of the ecosystem services concept in landscape management in the Netherlands. Integr. Environ. Assess. Manag. 9, 237–242. doi: 10.1002/ieam.1391
van Wezel, A. P., Franken, R. O. G., Drissen, E., Versluijs, K. C. W., and van den Berg, R. (2008). Societal cost-benefit analysis for soil remediation in The Netherlands. Integr. Environ. Assess. Manag. 4, 61–74. doi: 10.1897/IEAM_2007-034.1
van Wilgen, B. W., Cowling, R. M., and Burgers, C. J. (1996). Valuation of ecosystem services. Bioscience 46, 184–189. doi: 10.2307/1312739
van Wijnen, H. J., Rutgers, M., Schouten, A. J., Mulder, C., de Zwart, D., and Breure, A. M. (2012). How to calculate the spatial distribution of ecosystem services - Natural attenuation as example from The Netherlands. Sci. Total Environ. 415, 49–55. doi: 10.1016/j.scitotenv.2011.05.058
Várallyay, G. (1987). A Talaj Vízgazdálkodása (Soil Water Management). Budapest: MTA Doktori Értekezés.
Várallyay, G. (1989). “Land evaluation in Hungary - Scientific problems, practical applications,” in Land Qualities in Space and Time, Symposium Organized by the International Society of Soil Science, eds J. Bouma and A. K. Bregt (Wageningen: Pudoc), 241–252.
Vatn, A. (2000). The environment as a commodity. Environ. Values 9, 493–509. doi: 10.3197/096327100129342173
Vatn, A., and Bromley, D. W. (1994). Choices without prices without apologies. J. Environ. Econ. Manage. 26, 129–148. doi: 10.1006/jeem.1994.1008
Veblen, T. (1923). Absentee Ownership and Buesiness Enterprise in Recent Times” The Case of America. New York, NY: B. W. Huebsch.
Vejre, H., Vesterager, J. P., Andersen, P. S., Olafsson, A. S., Brandt, J., and Dalgaard, T. (2015). Does cadastral division of area-based ecosystem services obstruct comprehensive management? Ecol. Model. 295, 176–187. doi: 10.1016/j.ecolmodel.2014.09.027
Veronesi, F., Corstanje, R., and Mayr, T. (2014). Landscape scale estimation of soil carbon stock using 3D modelling. Sci. Total Environ. 487, 578–586. doi: 10.1016/j.scitotenv.2014.02.061
Vignola, R., Koellner, T., Scholz, R. W., and McDaniels, T. L. (2010). Decision-making by farmers regarding ecosystem services: factors affecting soil conservation efforts in Costa Rica. Land Use Policy 27, 1132–1142. doi: 10.1016/j.landusepol.2010.03.003
Villa, F., Bagstad, K. J., Voigt, B., Johnson, G. W., Athanasiadis, I. N., and Balbi, S. (2014). The misconception of ecosystem disservices: how a catchy term may yield the wrong messages for science and society. Ecosyst. Serv. 10, 52–53. doi: 10.1016/j.ecoser.2014.09.003
Vittoria, L. M., and Goldberg, F. (1975). Le funzioni del suolo nella biosfera (The functions of the soil in the biosphere). Rilancio Agricolo Veterinario Zootecnico 7, 22–23.
Volchko, Y., Norrman, J., Bergknut, M., Rosen, L., and Soderqvist, T. (2013). Incorporating the soil function concept into sustainability appraisal of remediation alternatives. J. Environ. Manage. 129, 367–376. doi: 10.1016/j.jenvman.2013.07.025
Volchko, Y., Norrman, J., Rosen, L., Bergknut, M., Josefsson, S., Soderqvist, T., et al. (2014). Using soil function evaluation in multi-criteria decision analysis for sustainability appraisal of remediation alternatives. Sci. Total Environ. 485, 785–791. doi: 10.1016/j.scitotenv.2014.01.087
Vucetich, J. A., Bruskotter, J. T., and Nelson, M. P. (2015). Evaluating whether nature's intrinsic value is an axiom of or anathema to conservation. Conserv. Biol. 29, 321–332. doi: 10.1111/cobi.12464
Wagg, C., Bender, S. F., Widmer, F., and van der Heijden, M. G. A. (2014). Soil biodiversity and soil community composition determine ecosystem multifunctionality. Proc. Natl. Acad. Sci. U.S.A. 111, 5266–5270. doi: 10.1073/pnas.1320054111
Wallace, K. J. (2007). Classification of ecosystem services: problems and solutions. Biol. Conserv. 139, 235–246. doi: 10.1016/j.biocon.2007.07.015
Wall, D. H., Bardgett, R. D., Covich, A. P., and Snelgrove, P. V. R. (2004). “The need for understanding how biodiversity and ecosystem functioning affect ecosystem services in soils and sediments,” in Sustaining Biodiversity and Ecosystem Services in Soils and Sediments, ed D. H. Wall (Washington, DC: Island Press), 1–12.
Wall, D. H., and Nielsen, U. N. (2012). Biodiversity and ecosystem services: Is it the same below ground? Nat. Educ. Knowl. 3, 8. doi: 10.1016/j.ecolmodel.2014.09.027
Wall, D. H., and Virginia, R. A. (2000). “The world beneath our feet: Soil biodiversity and ecosystem functioning,” in Nature and Human Society: The Ques for a Sustainable World, eds P. R. Raven and T. Williams (Washington, DC: National Academy of Sciences and National Research Council), 225–241.
Walter, C., Bispo, A., Chenu, C., Langlais-Hesse, A., and Schwartz, C. (2015). “Les services écosystémiques des sols: Du concept à sa valorisation,” in Agriculture et Foncier - Concurrences entre Usages des sols et entre Usagers des sols Agricoles: La Question Foncière Renouvelée, ed C. Demeter (Paris: Cahier Demeter), 51–68.
Wander, M. M., and Drinkwater, L. E. (2000). Fostering soil stewardship through soil quality assessment. Appl. Soil Ecol. 15, 61–73. doi: 10.1016/S0929-1393(00)00072-X
Warkentin, B. P., and Fletcher, H. F. (1977). “Soil quality for intensive agriculture,” in International Seminar on Soil Environment and Fertility Management in Intensive Agriculture: Society for Science of Soil and Manure (Tokyo: National Institute of Agricultural Science), 594–598.
Wasson, J. R., McLeod, D. M., Bastian, C. T., and Rashford, B. S. (2013). The effects of environmental amenities on agricultural land values. Land Econ. 89, 466–478. doi: 10.3368/le.89.3.466
Wegner, G., and Pascual, U. (2011). Cost-benefit analysis in the context of ecosystem services for human well-being: a multidisciplinary critique. Global Environ. Change Hum. Policy Dimens. 21, 492–504. doi: 10.1016/j.gloenvcha.2010.12.008
Weikard, H.-P., and Hein, L. (2011). Efficient versus sustainable livestock grazing in the Sahel. J. Agric. Eco. 62, 153–171. doi: 10.1111/j.1477-9552.2010.00275.x
Weisbrod, B. (1964). Collective-consumption services of individual-consumption goods. Q. J. Eco. 78, 471–477. doi: 10.2307/1879478
Wellington, E. M. H., Boxall, A. B. A., Cross, P., Feil, E. J., Gaze, W. H., Hawkey, P. M., et al. (2013). The role of the natural environment in the emergence of antibiotic resistance in Gram-negative bacteria. Lancet Infect. Dis. 13, 155–165. doi: 10.1016/S1473-3099(12)70317-1
Wells, E. C. (2006). “Cultural soilscapes,” in Functions of Soils for Human Societies and The Environment, eds E. Frossard, W. E. H. Blum and B. P. Warkentin (London: The Geological Society of London), 125–132.
Westman, W. E. (1977). How much are nature's services worth. Science 197, 960–964. doi: 10.1126/science.197.4307.960
Willemen, L., Veldkamp, A., Verburg, P. H., Hein, L., and Leemans, R. (2012). A multi-scale modelling approach for analysing landscape service dynamics. J. Environ. Manage. 100, 86–95. doi: 10.1016/j.jenvman.2012.01.022
Williams, A., and Hedlund, K. (2013). Indicators of soil ecosystem services in conventional and organic arable fields along a gradient of landscape heterogeneity in southern Sweden. Appl. Soil Ecol. 65, 1–7. doi: 10.1016/j.apsoil.2012.12.019
Winthrop, R. H. (2014). The strange case of cultural services: limits of the ecosystem services paradigm. Ecol. Econ. 108, 208–214. doi: 10.1016/j.ecolecon.2014.10.005
Wissen Hayek, U., Teich, M., Klein, T. M., and Grêt-Regamey, A. (2016). Bringing ecosystem services indicators into spatial planning practice: lessons from collaborative development of a web-based visualization platform. Ecol. Indic. 61, 90–99. doi: 10.1016/j.ecolind.2015.03.035
Wittemyer, G., Northrup, J. M., Blanc, J., Douglas-Hamilton, I., Omondi, P., and Burnham, K. P. (2014). Illegal killing for ivory drives global decline in African elephants. Proc. Natl. Acad. Sci. U.S.A. 111, 13117–13121. doi: 10.1073/pnas.1403984111
Wollman, N. (ed.) (1962). The Value of Water in Alternative Uses, with Special Application to Water Use in the San Juan and Rio Grande Basins of New Mexico. Albuquerque, NM: The University of New Mexico Press.
Wösten, J. H. M., Schuren, C. H. J. E., Bouma, J., and Stein, A. (1990). Comparing four methods to generate soil hydraulic functions in terms of their effect on simulated soil water budgets. Soil Sci. Soc. Am. J. 54, 827–832.
Xiong, X., Grunwald, S., Myers, D. B., Kim, J., Harris, W. G., and Bliznyuk, N. (2015). Assessing uncertainty in soil organic carbon modeling across a highly heterogeneous landscape. Geoderma 251, 105–116. doi: 10.1016/j.geoderma.2015.03.028
Yaalon, D. H., and Arnold, R. W. (2000). Attitudes toward soils and their societal relevance: then and now. Soil Sci. 165, 5–12. doi: 10.1097/00010694-200001000-00003
Zhang, W., Ricketts, T. H., Kremen, C., Carney, K., and Swinton, S. M. (2007). Ecosystem services and disservices to agriculture. Ecol. Econ. 64, 253–260. doi: 10.1016/j.ecolecon.2007.02.024
Zhang, Y., Holzapfel, C., and Yuan, X. (2013). “Scale dependent ecosystem service,” in Ecosystem Services in Agricultural and Urban Landscapes, eds S. Wratten, H. Sandhu, R. Cullen, and R. Costanza (Oxford: Wiley-Blackwell), 107–121.
Zimmerman, M. J. (2015). “Intrinsic vs. extrinsic value,” in The Stanford Encyclopedia of Philosophy, ed E. N. Zalta (New York, NY: Wiley-Blackwell). Available online at: http://plato.stanford.edu/archives/spr2015/entries/value-intrinsic-extrinsic/ (Accessed 29 May 2016).
Keywords: ecosystem services, valuation, sustainability, commodification, soil functions, decision making, land use and management
Citation: Baveye PC, Baveye J and Gowdy J (2016) Soil “Ecosystem” Services and Natural Capital: Critical Appraisal of Research on Uncertain Ground. Front. Environ. Sci. 4:41. doi: 10.3389/fenvs.2016.00041
Received: 25 January 2016; Accepted: 24 May 2016;
Published: 07 June 2016.
Edited by:
Denis Angers, Agriculture and Agri-Food Canada, CanadaReviewed by:
Ingo Schöning, Max-Planck-Institute for Biogeochemistry, GermanyChristian Walter, Agrocampus Ouest, France
Estelle Dominati, Agresearch, New Zealand
Copyright © 2016 Baveye, Baveye and Gowdy. This is an open-access article distributed under the terms of the Creative Commons Attribution License (CC BY). The use, distribution or reproduction in other forums is permitted, provided the original author(s) or licensor are credited and that the original publication in this journal is cited, in accordance with accepted academic practice. No use, distribution or reproduction is permitted which does not comply with these terms.
*Correspondence: Philippe C. Baveye, cGhpbGlwcGUuYmF2ZXllQGFncm9wYXJpc3RlY2guZnI=