- 1Division for Climate and Environmental Health, Norwegian Institute of Public Health, Oslo, Norway
- 2Institute of Epidemiology, Helmholtz Zentrum München - German Research Center for Environmental Health, Neuherberg, Germany
- 3Department of Environmental Health Sciences, Yale School of Public Health, New Haven, CT, United States
- 4Department of Biostatistics, University of Oslo, Oslo, Norway
- 5Division for Infection Control, Norwegian Institute of Public Health, Oslo, Norway
- 6Department of Mathematics, University of Oslo, Oslo, Norway
- 7Department of Environmental Science, Aarhus University, Roskilde, Denmark
- 8Department of Biostatistics, Institute of Basic Medical Sciences, University of Oslo, Oslo, Norway
Background: This time-stratified case-crossover study examined short-term associations of air temperature with cause-specific mortality (natural-cause, cardiovascular and respiratory) and potential effect modification by daily mean air pollution concentrations and individual characteristics in the Cohort of Norway (CONOR) cohort.
Methods: The CONOR cohort recruited ∼173,000 participants from 1994 to 2003. Participants’ vital status and the cause of death were collected from the Cause of Death Registry of Norway until 2018. Daily mean air temperatures and concentrations of fine particulate matter (PM2.5) and ozone (O3) estimated by spatial-temporal models were assigned to participants’ residences. We applied conditional logistic regression models with the distributed lag non-linear model approach to assess cold and heat effects on cause-specific mortality. The potential effect modification was analyzed by incorporating an interaction term between air temperature and the modifier in the regression model. The cold and heat effects were estimated for different subgroups of participants and at the low (5th percentile), medium (50th percentile), and high (95th percentile) levels of air pollution.
Results: We observed an increased risk of natural-cause mortality (OR: 1.26 95% CI: 1.09, 1.46) for a decrease in temperature from the minimum mortality temperature (MMT, 17.6°C) to the 1st percentile and an increased risk of cardiovascular mortality (OR: 1.32, 95% CI: 1.04, 1.67) for a decrease from MMT (16.1°C) to the 1st percentile. The cold effect on natural-cause mortality was more pronounced among women, former smokers, those aged below 75 years and people with a history of cardiovascular diseases. The cold effect on natural-cause mortality was stronger at higher levels of air pollution in winter, and the heat effect on cardiovascular mortality were stronger with elevated air pollution levels in summer.
Conclusion: We find adverse short-term cold effects on overall mortality in Norway. We further observe significant risk of cold related effects on natural and cardiovascular mortality in the wintertime and increased risk of respiratory mortality due to higher temperatures in the summer. We identified subpopulations who were likely to be more at risk for temperature-related mortality. We also see that increased air pollution impacts temperature related mortality in winter and summer time.
Introduction
Climate change and air pollution and their subsequent impacts on health remain an issue of concern (1). While there are numerous air-quality legislations in Europe, recent studies have shown that health impacts of air pollution are still a concern in Europe due to climate change (2). Changes in temperature levels in the future could imply an increase in chemical reaction rates that influence production and loss rates of gaseous pollutants and hence affect local and regional pollution levels (3).
Temperature and air pollution have been increasingly shown to be relevant environment-related factors in understanding mortality and morbidity, especially from cardiovascular and respiratory (CPD) causes. There have been numerous studies that examined the impact of temperatures on mortality in a historical context (4), including a few from Nordic countries (5). There have also been studies focusing on cardiovascular and respiratory mortality from both high and low temperatures (6, 7). In addition, air pollution has further been indicated to have significant impacts on mortality in the Nordic countries (8–10). Recent studies have increasingly started to examine the relationship between temperature and air pollution and their impacts on health, (11–14). These studies are generally designed to study exposure-health relationships in larger areas like cities or municipalities.
Short-term exposures are daily exposures of temperature and air pollution compared to long-term exposure which is calculated over the lifetime of the individual. Cohort-based data has extensively been used in longer-term air pollution analyses (15–17), including Nordic areas (18), but have only been recently applied in short-term temperature-related studies (19). There have been very few studies that have considered temperature and air pollution exposures together in cohort studies. The advantage of cohort-based studies is the information on geolocation of households of study participants which provides detailed exposure estimates for the participants and their individual characteristics that can be very relevant while understanding health impacts. Such studies require extensive data on address-based exposures and are usually difficult to construct. They also provide individual level socioeconomic data on a large number of participants, that can be useful to examine the vulnerability of these populations.
Norway has a wide range of climatic zones, thus highlighting a need for understanding the role of climatic variables on health outcomes. While air pollution levels in Norway are traditionally low, their interaction with temperature on health outcomes has not been explored earlier. In this paper, we investigate data from a nation-wide cohort study using daily exposures of temperature and air pollution to understand their impacts on natural (non-accidental) mortality as well as from cardiovascular and respiratory causes in Norway. To our knowledge, this is the first-time that a cohort based study has been applied to examine exposures related to ambient temperature and air pollution and the impacts on mortality in Norway.
Methods
Health and individual data
The Cohort of Norway (CONOR) is a collection of health data from ten Norwegian health surveys conducted between 1994 and 2003. The CONOR database contains data from around 173,000 individuals across Norway, which were gathered through questionnaires and physical examinations by experienced and trained personnel. The questionnaires cover the main topics of personal sociodemographic characteristics, lifestyle factors, general health status, comorbidities, and use of medications. In the health examinations, heart rate, blood pressure, height, weight, waist, and hip circumference are measured. All participants provided written informed consent for research and linkage to health registries. Further details about the CONOR cohort have been published elsewhere (20) and a summary is available in the Supplementary Table S1. We supplemented the CONOR data with additional individual level data on socio-economic conditions from the Statistical Bureau of Norway (SSB).
The vital status of the CONOR participants was ascertained until December 31, 2018, by linkage to the Norwegian Cause of Death Registry using unique personal identification numbers of Norwegian citizens. The current study focused on death cases from natural causes (non-accidental) [International Classification of Diseases (ICD)-9: 001-799; ICD-10: A00-R99], cardiovascular diseases (ICD-9: 390-459; ICD-10: I00-I99), and respiratory diseases (ICD-9: 460-519; ICD-10: J00-J99). We excluded deaths occurring after 2018 or without information on the cause of death.
Temperature and air pollution data
Daily mean surface air temperatures on a 1 km × 1 km grid across Norway were obtained from the seNorge2 dataset developed by the Norwegian Meteorological Institute. This dataset has been created based on observations from Norway's climate database and the European Climate Assessment and Dataset since 1957 and is continuously updated daily. All observations have been quality-controlled by experienced staff. The spatial interpolation scheme builds upon classical methods, such as optimal interpolation and successive-correction schemes (21).
Daily mean concentrations of PM2.5 and O3 for 2000–2015 at a horizontal resolution of 1 km × 1 km were provided by the DEHM-UBM model setup, which combines the chemical-transport model DEHM [Danish Eulerian Hemispheric Model, (22)] with the Gaussian dispersion model UBM [Urban Background Model; (23, 24). The geographic domain of DEHM covers the Northern Hemisphere with higher resolution nests over Europe and Scandinavia. In contrast, UBM in the setup used here covers the continental Nordic countries (25) with a high horizontal resolution (1 km × 1 km). Full non-linear chemistry is included in DEHM, while a simple chemical model for the titration of ozone by nitrogen oxides is included in UBM. This combination makes it possible to describe the long-range atmospheric transport of pollutants into and within Europe while still having a high spatial resolution in the output over, e.g., populated areas. In the setup used for this study, a new high-resolution emission inventory for the Nordic region has been used as input to the DEHM and UBM models for the Nordic countries (25), while the emissions applied in DEHM on a European scale are based on the EMEP emission database. Daily mean surface air temperature and air pollutant concentrations from 1995-2018 were assigned to CONOR participants’ residences.
Statistical analysis
We used a time-stratified case-crossover study design. This approach controls for long-term and seasonal time trends as well as time-invariant confounders such as sociodemographic status, underlying health conditions, and long-term lifestyle factors. This method has been applied to study aggregated health outcomes and environmental exposures, such as air pollution, focusing on adjusting covariates and investigating effect modification (26). For each participant who died during the follow-up period, we compare the exposure profile on the day of death (case day) with exposures on days at the same day of the week within the same month (control days). By making within-participant comparisons between case and control days, the potential confounding effects of time-invariant participant characteristics, long-term trend, seasonality, and day of the week were controlled by design. While we did not specifically compare the design in this study with any other method of analysis, we use as a reference (27) who have reported that the case-crossover study design using conditional logistic regression is equivalent to time-series analysis in environmental epidemiology.
We applied conditional logistic regression with the distributed lag non-linear modeling (DLNM) approach to estimate the effects of temperature on mortality. The case day is used as the unit of analysis. The DLNM incorporates a cross-basis matrix that defines the relationship with mortality simultaneously in the conventional space of temperature and an additional dimension of the lags. The non-linear exposure-response association was described by a natural cubic spline with three internal knots placed at the 10th, 75th, and 90th percentiles of the temperature distribution, and the lag-response dimension was characterized by a natural cubic spline with an intercept and two internal knots placed at equally spaced values on the log scale for up to lag six days. The minimum mortality temperature (MMT) was defined as the temperature at which the mortality risk was the lowest. It was determined by the temperature-mortality function predicted by the DLNM. The effects of cold were estimated as the odds ratios (ORs) of mortality with 95% confidence intervals (95% CIs) for a decrease in daily mean air temperature from the MMT to the 1st percentile of the temperature distribution, and the effects of heat were estimated for an increase in temperature from the MMT to the 99th percentile. The temperature effects in our study can be interpreted as odds ratios, i.e., the odds of mortality associated with temperature on the case days, compared to the odds on control days. This is valid because the case-crossover design estimates the likelihood of death under different exposure conditions within the same individual, making the OR a direct measure of the change in risk related to the exposure.
We further evaluated seasonality in the cold and heat effects by restricting the analyses to summer (June-August) and winter (December-February) months. The statistical approach of the analysis stratified by season was similar to that of the main whole-year analysis, except that we used a natural spline with one internal knot at the 50th percentile of the season-specific temperature distribution to specify the exposure-response function. The cold and heat effects in the seasonal analysis are presented as ORs together with 95% CIs for a decrease in daily mean air temperature from the 25th to the 1st percentile and an increase from the 75th to the 99th percentile of the season-specific temperature distribution, respectively.
Additionally, we examine the temperature effect modification by incorporating an interaction term between the cross-basis of air temperature and individual-level factors, including age (< 75 years or ≥ 75 years), sex (females or males), education (< high school, high school, or university), occupation (employed, unemployed/homemaker, or retired), smoking status (current, former, or never smoker), obesity [no or yes, defined as body mass index (BMI)≥30 kg/m2], history of cardiovascular diseases (no or yes, defined as a history of myocardial infarction, angina pectoris, or stroke), and diabetes (no or yes). The statistical significance of the effect modification was evaluated by the two-sided Z-test (28).
The temperature effect on mortality modified by air pollution was investigated by incorporating a tensor smoother between temperature and the air pollutant (PM2.5 or O3) in the conditional logistic regression model (29). This analysis was performed for the winter (December-February) and summer (June-August) months separately, considering the longer delay of the cold effects and the more immediate effects of heat. Specifically, we used seven-day moving averages of air temperature and air pollutant concentrations on the same and previous six days (lag 0–6 days) in the tensor smoother for the winter, and two-day moving averages of the same and previous days (lag 0–1) for the summer. Temperature and air pollution were included in the smoother term as cubic splines with basis dimension 25. The combined relationship of air temperature and air pollution with mortality was depicted by a tridimensional surface, from which we extracted the temperature-mortality relationships at the 5th, 50th, and 95th percentiles of the season-specific air pollutant distribution, defined as the low, medium, and high levels of air pollution, respectively. ORs of mortality risks for a decrease in temperature from the 25th to the 1st percentile were estimated at these three levels of air pollution in winter to assess the cold effect modification by air pollution. For the heat effect, we estimated the ORs of mortality for an increase from the 75th to the 99th percentile in temperature at three air pollution levels during the summer. The analyses on temperature effect modification were restricted to the years 2000–2015 due to the availability of air pollution data.
In sensitivity analyses, we used alternative lags (0–3 and 0–10) to estimate cumulative temperature effects. For interactive effects of temperature and air pollution, we first extended the study months when investigating data from the cold (November to March) and warm (May to September) seasons. Second, we used the 25th, 50th, and 75th percentiles of air pollution distributions to define low, medium, and high air pollution levels, respectively.
The statistical analyses were performed with R software (version 4.2.1) using packages “mgcv” and “dlnm”. All tests were 2-sided with a 5% significance level. R codes will be made available on request.
Results
In the CONOR cohort, we identified 40,040 cases of natural-cause deaths through December 31, 2018, including 14,457 deaths from cardiovascular diseases and 3,699 deaths from respiratory diseases. Participants who died from natural causes had a mean age of 79.4 years at death and 55.4% of them were males (Table 1). Compared with the whole study population in the CONOR cohort, the deceased participants tended to be older and less educated and had a higher prevalence of cardiovascular diseases and diabetes at baseline (Table 1 and Supplementary Table S1).
The daily mean air temperature during the study period (1994–2018) ranged from −47.6°C to 27.7°C, with a mean of 5.0°C (standard deviation: 7.6°C). The averages of daily mean PM2.5 and O3 concentrations from 2000 to 2015 were 6.7 µg/m3 and 67.7 µg/m3, respectively (Table 2). The mean concentration of PM2.5 in summer (June-August) was lower than that in winter (December-February), whereas the mean O3 concentration was slightly higher in the summer months (Supplementary Tables S2, S3).

Table 2. Description of daily mean air temperature and air pollution concentrations during the study perioda.
Temperature effects on mortality
The exposure-response relationships between air temperature and different causes of mortality were all non-linear (Supplementary Figure S1). The MMTs were 17.6°C, 16.1°C, and −6.1°C, respectively, for natural-cause, cardiovascular, and respiratory mortality. We observed increased risks of natural-cause (OR: 1.26, 95% CI: 1.09, 1.46) and cardiovascular (OR: 1.32, 95% CI: 1.04, 1.67) mortality for a decrease in temperature from the MMT to the 1st percentile (Table 3). Our effect estimates in the main analyses did not indicate any association between cold and respiratory mortality or adverse heat effects on mortality (Table 3).
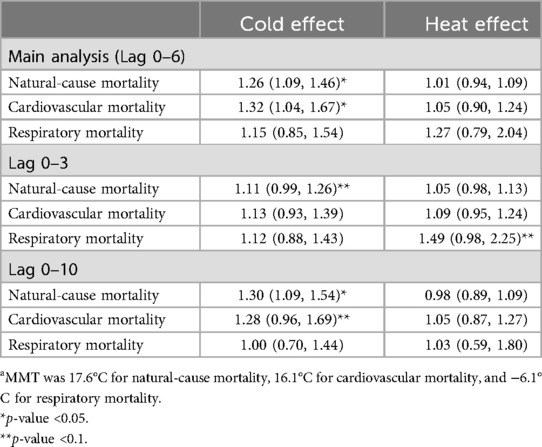
Table 3. Odds ratios (95% confidence intervals) of mortality for a decrease in daily mean air temperatures from the minimum mortality temperature (MMT)a to the 1st percentile (cold effects) and an increase in daily mean air temperatures from the MMTa to the 99th percentile (heat effects) in the main and sensitivity analyses.
The exposure-response functions in summer and winter are shown in Supplementary Figure S2. Analyses stratified by season suggest increased risks of natural-cause (OR: 1.18, 95% CI: 1.01, 1.37) and respiratory (OR: 2.12, 95% CI: 1.27, 3.56) mortality for a decrease in temperature from the 25th (10.8°C) to the 1st (5.6°C) percentile in summer (Table 4). Besides, we observed an increased risk of respiratory mortality (OR: 1.73, 95% CI: 1.03, 2.90) associated with an increase in temperature from the 75th (15.9°C) to the 99th (21.7°C) percentile in summer (Table 4).
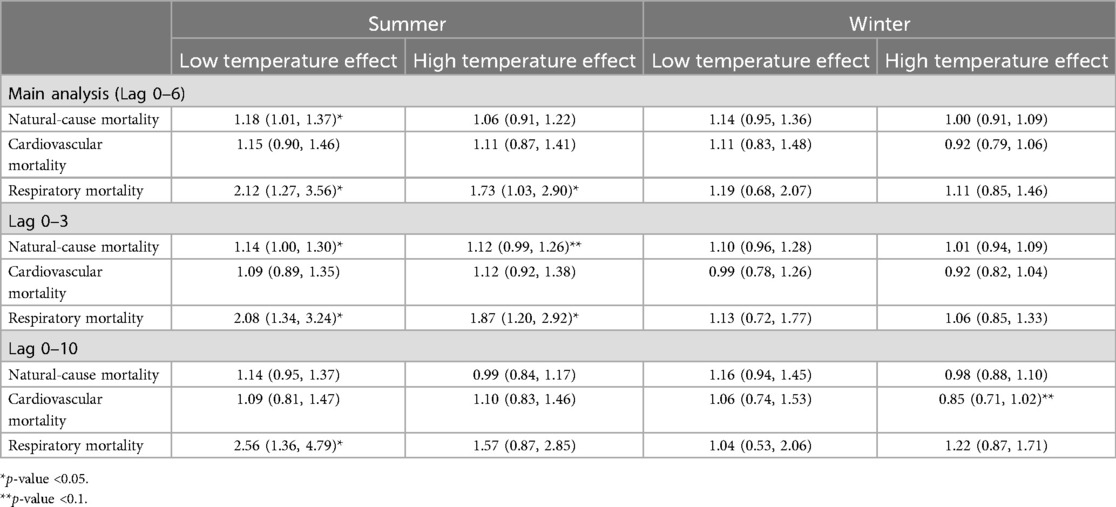
Table 4. Odds ratios (95% confidence intervals) of mortality for a decrease in daily mean air temperatures from the 25th to the 1st percentile (low temperature effects) and an increase in daily mean air temperatures from the 75th to the 99th percentile (high temperature effects) in the main and sensitivity analyses for summer (June–August) and winter (December-February).
The cold effects on natural-cause and cardiovascular mortality remained stable in the sensitivity analysis of using lag 0–10 days whereas were reduced when shortening the lag to 0–3 days (Table 3). In addition, using a lag of 0–3 days strengthened the adverse heat effects on respiratory mortality during the whole year and the high temperature effect on natural-cause mortality in summer (Tables 3, 4). When comparing the temperature-mortality relationships during the whole study period (shown in Supplementary Figure S1) to those in summer and winter (Supplementary Figure S2), the patterns for the whole-year relationships were in general consistent with a combination of the patterns for the relationship in summer and winter. The “contradictory” effect estimates in Tables 3, 4 could be mainly due to the different calculation of the ORs in the whole-year and seasonal analyses.
Effect modification by air pollution
We observed stronger cold effects on natural-cause mortality at higher levels of air pollution in winter, though the between-level differences were not significant (Figure 1). For a decrease in air temperature from the 25th to the 1st percentile in winter, the ORs of natural-cause mortality were 1.04 (95% CI: 0.95, 1.13), and 1.16 (0.99, 1.33) respectively, at low, medium, and high levels of PM2.5 (Supplementary Table S4). Similarly, heat effects on cardiovascular mortality were stronger with elevated air pollution levels in summer (Figure 2). The heat-associated percent changes in cardiovascular mortality risks were 1.24 (95% CI: 0.88, 1.60), 1.30 (1.03, 1.57), and 1.39 (1.04, 1.73) at low, medium, and high levels of O3, respectively (Supplementary Table S7).
Effect modification on the associations between temperature and mortality by air pollution was less evident when analyzing extended data of the cold (November-March) and warm (May-September) seasons (Supplementary Tables S4–S7). Using the 25th and 75th percentiles to define low and high air pollution reduced the differences in temperature effect estimates across air pollution levels (Supplementary Tables S4–S7). See also Supplementary Figures 5, 6 for an example of the tridimensional figures used in the tensor analysis.
Effect modification by individual characteristics
The cold effect on natural-cause mortality was slightly stronger for people aged below 75 years and women (Figure 3). Besides, the cold effect on cardiovascular mortality was slightly stronger among former smokers and people with a history of cardiovascular diseases (Figure 3). However, the between-group differences in effect estimates were not statistically significant (Supplementary Table S8). Moreover, we observed increased natural-cause and cardiovascular mortality risks associated with heat exposure in unemployed people or homemakers (Supplementary Figure S3, Table S9). No significant effect modification by participant characteristics was found for respiratory mortality (Supplementary Figure S4).
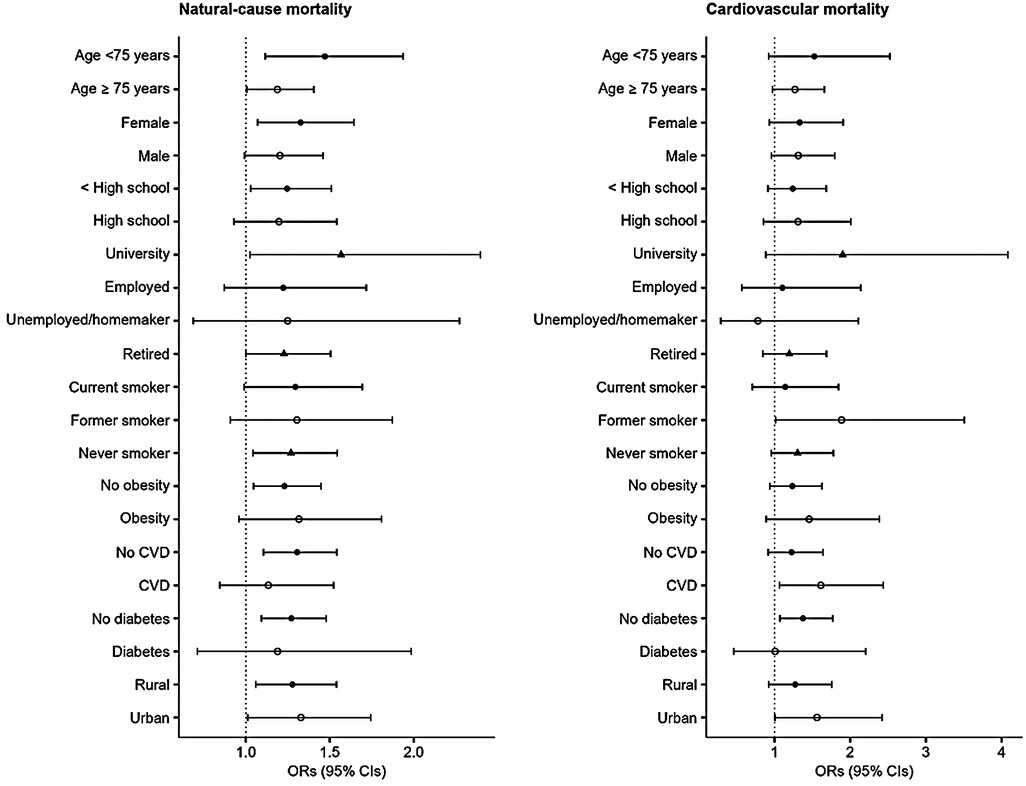
Figure 3. Cold effects on natural-cause and cardiovascular mortality modified by participant characteristics.
Discussion
In this population-based cohort, our findings provide evidence for adverse short-term cold effects on mortality. We did not find any associations between heat and mortality in the general cohort population. The cold effects were more pronounced in participants below 75 years, women, former smokers, and people with a history of cardiovascular diseases. We observe increased natural-cause and cardiovascular mortality risks associated with heat exposure in unemployed people or homemakers. Our findings also indicate a potential seasonal effect of short-term temperature, with increased risks of natural-cause and respiratory mortality due to low temperatures and increase in respiratory mortality due to high temperature in summer. For combined exposure with air pollution, we observed stronger cold effects on natural-cause mortality at higher levels of air pollution in winter and stronger heat effects on cardiovascular mortality with elevated air pollution levels in summer.
Our findings of an association between cold weather and natural-cause, cardiovascular and respiratory mortality is in line with several previous studies conducted in Northern Europe (4, 30, 31). Cold temperatures may for example reduce natural defensive mechanisms of the elderly, socially isolated individuals, and those people with less adaptive and coping strategies.
Our results also suggest that an unexpected change or a greater variability in temperatures could be an important driver for increased mortality burdens in Norway. These results are consistent with other recently published articles that indicate for example that moderate cold temperatures are responsible for a higher proportion of the mortality burden especially in colder countries (4, 31). Our results on increased respiratory mortality in summer periods are in line with recent studies like (14) who finds that exposure to heat mainly affects patients with chronic respiratory diseases. While we did not find significant findings on increased respiratory mortality in winter, this can probably be attributed to heating, adaptation, behavioral changes, and freely available health care that are found in Norway. Understanding these differing burdens of disease due to heat and cold is important in Norway. Our results could also be extremely relevant as global climate models predict changes to the frequency, intensity and duration of climate extremes and a larger impact of seasonal variation affecting mortality outcomes in the future (32).
Our results on stronger cold effects on natural-cause mortality at higher levels of air pollution in winter and stronger heat effects on cardiovascular mortality with elevated air pollution levels in summer is supported by a number of studies (33, 34). Given potential increases in air pollution due to changes in temperatures in the future, we see a need for integrating air pollution policies with climate change policies.
Our findings on the modification of socio-economic factors are in line with existing studies (14, 30), although the results should be interpreted carefully. For example, the exact mechanism through which women are more affected by changes in temperatures is unclear, but it is hypothesized to be related to the effect of female reproductive hormones and menopause on thermoregulation. Alternatively, social vulnerability factors may play a role in the differential outcomes for men and women. Similar to our findings, others have observed that higher unemployment was associated with a greater susceptibility to the impacts of heat (35) and can significantly increase the rates of all-cause mortality (36, 37). Unemployment could for example lead to a drop in socio-economic status resulting in poor living conditions and thus reduced capacity to cope with increased cold.
This study is strengthened by its large population-based design, and detailed exposures and disease and socio-economic information at the individual level available from a range of high-quality health and social registries in Norway. Additionally, we were able to obtain detailed information on temperature and various air pollutants and link them to study participants at the address level based on respective geocodes to evaluate their impact on mortality. This yields much better estimates of exposure compared to using weighted averages from monitoring stations over larger areas. Given that, monitoring networks are spatially sparse and fail to adequately capture the spatial variability, we limit issues of bias and exposure misclassification. This allows for looking closer at the combined effects of multiple environmental exposures.
When evaluating our results in the greater context, it is important to note that the association between cold weather and mortality could be confounded by influenza which is related to several cardiovascular and respiratory conditions and which peaks in winter (38, 39). However, due to a lack of data on influenza outbreaks in Norway over the different years, we have not been able to include this as a potentially confounding factor in our models. In Norway, influenza vaccines are recommended for the elderly and those with known vulnerability factors, with at least 65% of those over 65 receiving an influenza vaccine in the 2021/2022 flu season (40), reducing the risk of influenza-related mortality. We also need to include morbidity outcomes in future studies on health outcomes as the effects of temperature and air pollution on hospital admissions may be significant in this regard.
The CONOR cohort that we used in this analysis also has some disadvantages (20) the overall participation rate is 58% and is lowest in the surveys in Oslo and other urban areas and became lower throughout the study period. However, the overall participation rate is influenced by low participation rate in those aged ≤30 years. The study population is somewhat heterogeneous as it includes sampling from 10 geographical areas with various age groups included over a 10-year period. The number of core variables is limited, and in some cases the wording of questions is slightly changed over the years. Additionally, as the cohort contained participants from all over the country, we were limited in the extent to which we could study sociodemographic factors as effect modifiers as their effects tend to be most pronounced in Oslo and other major cities and may have been muted by participants from other parts of the country. Another methodological issue is that the CONOR study consists mainly of older adults with an average death age of 79.4 years. This would be an important issue in investigating causal mechanisms related to temperature and air pollution exposures.
Our results highlight the need to examine both heat and cold related health burdens in Norway. Given recent evidence on changes to the frequency, intensity and duration of climate extremes and a larger impact of seasonal variation affecting mortality outcomes in the future, our results are important for examining adaptative strategies that can adequately reduce such burdens. Secondly, the results underscore the need for devising interactive climate change and air pollution strategies in Norway. Focusing on cities; investing in cross-sector energy efficiency and low-carbon technologies; and promoting inclusive, collaborative governance have been highlighted as important strategies in this regard. We suggest the need for further research investigating associated health risks of temperature and air pollution in wintertime and explaining related biological mechanisms in this regard. Additionally, the findings motivate further research into how socio-economic status modifies environment related health risks and the impact of incorporating these in devising health-equitable long-term adaptation strategies.
We conclude by stating that research and policy efforts should continue on the role that environmental exposures play in understanding the extent and distribution of current and future health burdens in Norway.
Data availability statement
The data analyzed in this study is subject to the following licenses/restrictions: Dataset used is from individual participants and cannot be shared in any form. Requests to access these datasets should be directed to https://www.fhi.no/hs/cohort-of-norway/.
Ethics statement
The studies involving humans were approved by Regional Committee for Medical Research Ethics South East Norway. The studies were conducted in accordance with the local legislation and institutional requirements. Written informed consent for participation was not required from the participants or the participants’ legal guardians/next of kin in accordance with the national legislation and institutional requirements.
Author contributions
SR: Conceptualization, Investigation, Supervision, Writing – original draft, Writing – review & editing, Funding acquisition, Validation. SZ: Formal Analysis, Investigation, Software, Visualization, Writing – review & editing, Writing – original draft. AA: Validation, Writing – review & editing, Writing – original draft. TB: Data curation, Project administration, Validation, Writing – review & editing, Writing – original draft. FD: Software, Validation, Visualization, Writing – review & editing, Writing – original draft. AD-LP: Data curation, Validation, Writing – review & editing, Writing – original draft. LF: Data curation, Writing – review & editing. CG: Data curation, Writing – review & editing. LV: Software, Validation, Visualization, Writing – review & editing. AS: Supervision, Writing – review & editing, Methodology.
Funding
The author(s) declare financial support was received for the research, authorship, and/or publication of this article. All authors acknowledge European Union’s Horizon Project EXHAUSTION (Grant ID: 820655) for data and collaboration.
Conflict of interest
The authors declare that the research was conducted in the absence of any commercial or financial relationships that could be construed as a potential conflict of interest.
Publisher's note
All claims expressed in this article are solely those of the authors and do not necessarily represent those of their affiliated organizations, or those of the publisher, the editors and the reviewers. Any product that may be evaluated in this article, or claim that may be made by its manufacturer, is not guaranteed or endorsed by the publisher.
Supplementary material
The Supplementary Material for this article can be found online at: https://www.frontiersin.org/articles/10.3389/fenvh.2024.1419261/full#supplementary-material
References
1. Orru H, Ebi KL, Forsberg B. The interplay of climate change and air pollution on health. Curr Environ Health Rep. (2017) 4(4):504–13. doi: 10.1007/s40572-017-0168-6
2. Rodopoulou S, Stafoggia M, Chen J, de Hoogh K, Bauwelinck M, Mehta AJ, et al. Long-term exposure to fine particle elemental components and mortality in Europe: results from six European administrative cohorts within the ELAPSE project. Sci Total Environ. (2022) 809:152205. doi: 10.1016/j.scitotenv.2021.152205
3. Im U, Geels C, Hanninen R, Kukkonen J, Rao S, Ruuhela R, et al. Reviewing the links and feedbacks between climate change and air pollution in Europe. Front Environ Sci. (2022) 10:954045. doi: 10.3389/fenvs.2022.954045
4. Gasparrini A, Guo Y, Hashizume M, Lavigne E, Zanobetti A, Schwartz J, et al. Mortality risk attributable to high and low ambient temperature: a multicountry observational study. Lancet. (2015) 386(9991):369–75. doi: 10.1016/S0140-6736(14)62114-0
5. Oudin Åström D, Forsberg B, Ebi KL, Rocklöv J. Attributing mortality from extreme temperatures to climate change in Stockholm, Sweden. Nat Clim Change. (2013) 3(12):1050–4. doi: 10.1038/nclimate2022
6. Liu J, Varghese BM, Hansen A, Zhang Y, Driscoll T, Morgan G, et al. Heat exposure and cardiovascular health outcomes: a systematic review and meta-analysis. Lancet Planet Health. (2022) 6(6):e484–95. doi: 10.1016/S2542-5196(22)00117-6
7. Moghadamnia MT, Ardalan A, Mesdaghinia A, Keshtkar A, Naddafi K, Yekaninejad MS. Ambient temperature and cardiovascular mortality: a systematic review and meta-analysis. PeerJ. (2017) 5:e3574. doi: 10.7717/peerj.3574
8. Beelen R, Raaschou-Nielsen O, Stafoggia M, Andersen ZJ, Weinmayr G, Hoffmann B, et al. Effects of long-term exposure to air pollution on natural-cause mortality: an analysis of 22 European cohorts within the multicentre ESCAPE project. Lancet. (2014) 383(9919):785–95. doi: 10.1016/S0140-6736(13)62158-3
9. Konduracka E, Rostoff P. Links between chronic exposure to outdoor air pollution and cardiovascular diseases: a review. Environ Chem Lett. (2022) 20(5):2971–88. doi: 10.1007/s10311-022-01450-9
10. Lee BJ, Kim B, Lee K. Air pollution exposure and cardiovascular disease. Toxicol Res. (2014) 30(2):71–5. doi: 10.5487/TR.2014.30.2.071
11. Anenberg SC, Haines S, Wang E, Nassikas N, Kinney PL. Synergistic health effects of air pollution, temperature, and pollen exposure: a systematic review of epidemiological evidence. Environ Health. (2020) 19(1):130. doi: 10.1186/s12940-020-00681-z
12. Mokoena KK, Ethan CJ, Yu Y, Quachie AT. Interaction effects of air pollution and climatic factors on circulatory and respiratory mortality in Xi'an, China between 2014 and 2016. Int J Environ Res Public Health. (2020) 17(23):9027. doi: 10.3390/ijerph17239027
13. Sillmann J, Aunan K, Emberson L, Büker P, Van Oort B, O’Neill C, et al. Combined impacts of climate and air pollution on human health and agricultural productivity. Environ Res Lett. (2021) 16(9):093004. doi: 10.1088/1748-9326/ac1df8
14. Zafeiratou S, Samoli E, Analitis A, Dimakopoulou K, Giannakopoulos C, Varotsos KV, et al. Modification of heat-related effects on mortality by air pollution concentration, at small-area level, in the Attica prefecture, Greece. Environ Health. (2024) 23(1):10. doi: 10.1186/s12940-024-01053-7
15. Cao J, Yang C, Li J, Chen R, Chen B, Gu D, et al. Association between long-term exposure to outdoor air pollution and mortality in China: a cohort study. J Hazard Mater. (2011) 186(2-3):1594–600. doi: 10.1016/j.jhazmat.2010.12.036
16. Hales S, Atkinson J, Metcalfe J, Kuschel G, Woodward A. Long term exposure to air pollution, mortality and morbidity in New Zealand: cohort study. Sci Total Environ. (2021) 801:149660. doi: 10.1016/j.scitotenv.2021.149660
17. Hoek G, Brunekreef B, Goldbohm S, Fischer P, van den Brandt PA. Association between mortality and indicators of traffic-related air pollution in The Netherlands: a cohort study. Lancet. (2002) 360(9341):1203–9. doi: 10.1016/S0140-6736(02)11280-3
18. Raaschou-Nielsen O, Thorsteinson E, Antonsen S, Holst GJ, Sigsgaard T, Geels C, et al. Long-term exposure to air pollution and mortality in the Danish population a nationwide study. EClinicalMedicine. (2020) 28:100605. doi: 10.1016/j.eclinm.2020.100605
19. Zheng S, Zhu W, Shi Q, Wang M, Nie Y, Zhang D, et al. Effects of cold and hot temperature on metabolic indicators in adults from a prospective cohort study. Sci Total Environ. (2021) 772:145046. doi: 10.1016/j.scitotenv.2021.145046
20. Naess O, Søgaard AJ, Arnesen E, Beckstrøm AC, Bjertness E, Engeland A, et al. Cohort profile: cohort of Norway (CONOR). Int J Epidemiol. (2008) 37(3):481–5. doi: 10.1093/ije/dym217
21. Lussana C, Tveito OE, Dobler A, Tunheim K. Senorge_2018, daily precipitation, and temperature datasets over Norway. Earth Syst Sci Data. (2019) 11(4):1531–51. doi: 10.5194/essd-11-1531-2019
22. Christensen JH. The Danish eulerian hemispheric model — a three-dimensional air pollution model used for the Arctic. Atmos Environ. (1997) 31(24):4169–91. doi: 10.1016/S1352-2310(97)00264-1
23. Brandt J, Christensen JH, Frohn LM, Berkowicz R. Air pollution forecasting from regional to urban street scale––implementation and validation for two cities in Denmark. Phys Chem Earth (Pt A B,C). (2003) 28(8):335–44. doi: 10.1016/S1474-7065(03)00054-8
24. Frohn LM, Geels C, Andersen C, Andersson C, Bennet C, Christensen JH, et al. Evaluation of multidecadal high-resolution atmospheric chemistry-transport modelling for exposure assessments in the continental Nordic countries. Atmos Environ. (2022) 290:119334. doi: 10.1016/j.atmosenv.2022.119334
25. Paunu V-V, Karvosenoja N, Segersson D, Lopez-Aparicio S, Nielsen O-K, Plejdrup MS, et al. New nordic emission inventory – spatial distribution of machinery and residential combustion emissions. In: Moussiopoulos N, Sokhi RS, Tsegas G, Fragkou E, Chourdakis E, Pipilis I, editors. In Proceedings of 12th International Conference on Air Quality, Science and Application. Hatfield, U.K.: University of Hertfordshire (2020). p. 17.
26. Tobias A, Kim Y, Madaniyazi L. Time-stratified case-crossover studies for aggregated data in environmental epidemiology: a tutorial. Int J Epidemiol. (2024) 53(2):dyae020. doi: 10.1093/ije/dyae020
27. Lu Y, Zeger SL. On the equivalence of case-crossover and time series methods in environmental epidemiology. Biostatistics. (2007) 8(2):337–44. doi: 10.1093/biostatistics/kxl013
28. Altman DG, Bland JM. Interaction revisited: the difference between two estimates. Br Med J. (2003) 326(7382):219. doi: 10.1136/bmj.326.7382.219
29. Zhang S, Breitner S, Stafoggia M, Donato FD, Samoli E, Zafeiratou S, et al. Effect modification of air pollution on the association between heat and mortality in five European countries. Environ Res. (2024) 263(Pt 1):120023. doi: 10.1016/j.envres.2024.120023
30. Ikäheimo TM, Jokelainen J, Näyhä S, Laatikainen T, Jousilahti P, Laukkanen J, et al. Cold weather-related cardiorespiratory symptoms predict higher morbidity and mortality. Environ Res. (2020) 191:110108. doi: 10.1016/j.envres.2020.110108
31. Vázquez Fernández L, Diz-Lois Palomares A, Vicedo Cabrera AM, Freiesleben De Blasio B, Di Ruscio F, Wisløff T, et al. Short-term association between air temperature and mortality in seven cities in Norway: a time series analysis. Scand J Public Health. (2024):14034948241233359. doi: 10.1177/14034948241233359
32. Madaniyazi L, Armstrong B, Tobias A, Mistry MN, Bell ML, Urban A, et al. Seasonality of mortality under climate change: a multicountry projection study. Lancet Planetary Health. (2024) 8(2):e86–94. doi: 10.1016/S2542-5196(23)00269-3
33. Rai M, Stafoggia M, de'Donato F, Scortichini M, Zafeiratou S, Vazquez Fernandez L, et al. Heat-related cardiorespiratory mortality: effect modification by air pollution across 482 cities from 24 countries. Environ Int. (2023) 174:107825. doi: 10.1016/j.envint.2023.107825
34. Wine O, Osornio Vargas A, Campbell SM, Hosseini V, Koch CR, Shahbakhti M. Cold climate impact on air-pollution-related health outcomes: a scoping review. Int J Environ Res Public Health. (2022) 19(3):1473. doi: 10.3390/ijerph19031473
35. Leone M, D’Ippoliti D, De Sario M, Analitis A, Menne B, Katsouyanni K, et al. A time series study on the effects of heat on mortality and evaluation of heterogeneity into European and eastern-southern Mediterranean cities: results of EU CIRCE project. Environ Health. (2013) 12(1):55. doi: 10.1186/1476-069X-12-55
36. Gerdtham U-G, Johannesson M. A note on the effect of unemployment on mortality. J Health Econ. (2003) 22(3):505–18. doi: 10.1016/S0167-6296(03)00004-3
37. Roelfs DJ, Shor E, Davidson KW, Schwartz JE. Losing life and livelihood: a systematic review and meta-analysis of unemployment and all-cause mortality. Soc Sci Med. (2011) 72(6):840–54. doi: 10.1016/j.socscimed.2011.01.005
38. Barnes M, Heywood AE, Mahimbo A, Rahman B, Newall AT, Macintyre CR. Acute myocardial infarction and influenza: a meta-analysis of case-control studies. Heart. (2015) 101(21):1738–47. doi: 10.1136/heartjnl-2015-307691
39. Fuhrmann C. The effects of weather and climate on the seasonality of influenza: what we know and what we need to know. Geography Compass. (2010) 4(7):718–30. doi: 10.1111/j.1749-8198.2010.00343.x
40. Karoline Bragstad THP, Seppälä EM, Tønnessen R, Klüwer B, Rydland K, Aune T, et al. Influenza Virological and Epidemiological Season Report Prepared for the WHO Consultation on the Composition of Influenza Virus Vaccines for the Northern Hemisphere 2022/2023. Norway: Norwegian Institute of Public Health (2022).
Keywords: temperature, air pollution, cohort, mortality, Norway
Citation: Rao S, Zhang S, Ahimbisibwe A, Bekkevold T, Di Ruscio F, Diz-Lois Palomares A, Frohn LM, Geels C, Vázquez Fernández L and Schneider A (2024) Short-term effects of temperature and air pollution on mortality in Norway: a nationwide cohort-based study. Front. Environ. Health 3:1419261. doi: 10.3389/fenvh.2024.1419261
Received: 18 April 2024; Accepted: 8 October 2024;
Published: 10 December 2024.
Edited by:
Sawaid Abbas, University of the Punjab, PakistanReviewed by:
Syed Muhammad Muslim Raza, Virtual University of Pakistan, PakistanMuhammad Riaz, Cardiff University, United Kingdom
Copyright: © 2024 Rao, Zhang, Ahimbisibwe, Bekkevold, Di Ruscio, Diz-Lois Palomares, Frohn, Geels, Vázquez Fernández and Schneider. This is an open-access article distributed under the terms of the Creative Commons Attribution License (CC BY). The use, distribution or reproduction in other forums is permitted, provided the original author(s) and the copyright owner(s) are credited and that the original publication in this journal is cited, in accordance with accepted academic practice. No use, distribution or reproduction is permitted which does not comply with these terms.
*Correspondence: Shilpa Rao, c2hpbHBhLnJhb0BmaGkubm8=