- 1Department of Environmental Health, Institute of Health, Bule Hora University, Bule Hora, Ethiopia
- 2Department of Water and Public Health, Ethiopian Institute of Water Resources, Addis Ababa University, Addis Ababa, Ethiopia
- 3Department of Environmental Health Science and Technology, Institute of Health, Jimma University, Jimma, Ethiopia
Introduction: This study aimed to measure the concentration of toxic heavy metals in wastewater samples and Nile tilapia (Oreochromis niloticus) species inhabiting wastewater (waste stabilization ponds) and evaluate their safety as a food source in southwestern Ethiopia. For this purpose, toxic metals like lead (Pb), cadmium (Cd), arsenic (As), and mercury (Hg) in wastewater samples and fish tissues (muscle, gill, and liver) were independently examined.
Methods: A laboratory-based cross-sectional study was performed to ascertain the levels of Pb, Cd, As, and Hg in the fish tissues of O. niloticus and wastewater samples. Heavy metal levels were analyzed by microplasma atomic emission spectrometry (Agilent 4210 MP-AES) and hydrogen-generated atomic absorption spectrometry (HGAAS, novAA 400P, Germany).
Results: Heavy metal concentrations were measured in the following decreasing order (µg L−1): Cd > Pb > As > Hg in facultative and maturation ponds, with Cd (27.66 µg L−1) having the highest concentration and Hg (0.349 µg L−1) having the lowest concentration. Among the heavy metals detected in the wastewater samples, Hg showed a statistically significant difference between the sampling points (p = 0.023). The maximum metal concentration was measured for Pb (0.35 mg kg−1) and Cd (0.24 mg kg−1) in the muscle tissue of O. niloticus. The value of arsenic (0.02 mg kg−1) detected in fish edible muscles exceeded the FAO/WHO maximum permissible limit (MPL = 0.01) for human consumption. The carcinogenic and non-carcinogenic health risks of consuming fish due to trace metals were relatively low and posed fewer potential threats to human health. According to this finding, children were more susceptible to heavy metal exposure than adults.
Conclusion: Due to the high quantities of these harmful heavy metals, wastewater from oxidation ponds should not be used for fishing to avoid bioaccumulation. The target carcinogenic risk (TR) and target hazard quotient (THQ) indicated that all heavy metals were below the safe threshold. This research will provide a baseline for monitoring trace metals in various edible aquatic creatures and for future research in artificial habitats and regulatory considerations.
Introduction
Heavy metal pollution has been identified as a great concern for the aquatic environment and aquatic organisms including fish (1, 2). These metals accumulate harmful chemicals in the aquatic ecosystem and, consequently, in humans. Thus, it is critical to regulate the use of heavy metals (3). Moreover, some of the heavy metals are toxic, causing hazardous impacts on aquatic organisms and finally leading to serious human health concerns (4).
Several metals are considered important to organisms because they are constituents of several critical enzymes and play an important role in the metabolism of various essential nutrients (5). However, several studies have indicated that an excess of these metals creates significant abnormalities in an organism's cells and organs (6, 7). Heavy metal exposure causes a variety of complications, including liver damage, kidney dysfunction, cardiovascular irregularities, metabolic disruptions, and, in extreme cases, death (8, 9). Furthermore, heavy metals exert many neurotoxic and carcinogenic effects due to the buildup of metals in target human organs, such as the liver, kidney, and bones, upon intake of contaminated fish (10, 11). Cadmium (Cd) toxicity results in kidney dysfunction and proteinuria. Furthermore, severe lead (Pb) toxicity not only disrupts the cognitive development of children but is also accountable for various cardiac complications in adults (12).
Heavy metals build up in fish bodies through food consumption and the exchange of various ions in their gills (13). As a result, different studies and monitoring programs on metal accumulation in fish bodies are organized all over the world (14). Fish is a balanced, popular food item throughout the world that is considered an important source of protein, energy, and essential minerals. Several studies have revealed that heavy metals accumulate in the human body primarily when fish are taken as food (15, 16). For this reason, fish have been regarded as one of the primary indicators for estimating heavy metal contamination in freshwater ecosystems and associated human health risks.
A common global practice for water reuse involves utilizing treated municipal wastewater and its associated nutrients for aquaculture (17, 18). These concerns are significant since, in 2014, more fish for human consumption were obtained from artificial aquaculture than from global fisheries (19), and this needs to retain and satisfy future international food production needs. In Ethiopia, it is common practice for waste stabilization ponds (WSPs) to aid aquaculture operations. The misunderstanding of the links between wastewater reuse methods for fishing and the bioaccumulation of new pollutants, such as heavy metals, is exacerbated (20). Few studies have focused on integrated wastewater reuse with fish farming in developing countries. In Ethiopia, no published study has specifically monitored the quality of treated wastewater for fishing and the safety of fish harvested from artificial ponds. This study initially assessed the levels of toxic heavy metals in wastewater samples and fish tissues collected from a waste stabilization pond in southern Ethiopia. The main objective is to determine the levels of toxic heavy metals, including Pb, Cd, As, and Hg, in wastewater samples and fish tissues to establish their suitability for human consumption.
Materials and methods
Study area
The study was conducted at Jimma University, Jimma, Ethiopia. The wastewater samples and fish were taken from a waste stabilization pond located in the Institute of Technology campus of Jimma University in Jimma town, which is 352 km far from the capital of Addis Ababa in the southwest. The geographic coordinates are approximately 7°30′ latitude north and 36°15′ longitude east. The annual average temperature in the town is 19.3°C (11.5°C–27.1°C), with an annual rainfall of approximately 1,749.1 mm. The WSPs cover an area of 69,236.70 m2 (6.9 ha). The facility was designed to cater to a population of over 42,000 people. It consists of seven ponds with a total capacity of receiving more than 2,250 m3/day of raw wastewater. The WSP is designed to serve a population of 40,000 and contains seven ponds, as shown in Figure 1 (21). Initially, the wastewater generated from the student dorms is directed to septic tanks to remove solids, after which it is emptied into WSPs. The untreated domestic wastewater and runoff are combined and disposed of in the waste stabilization pond, resulting in elevated concentrations of various impurities, such as heavy metals, in the influent wastewater. To attain acceptable standard levels, the raw wastewater undergoes both physicochemical and biological treatments to minimize suspended solids and biochemical oxygen demand (BOD).
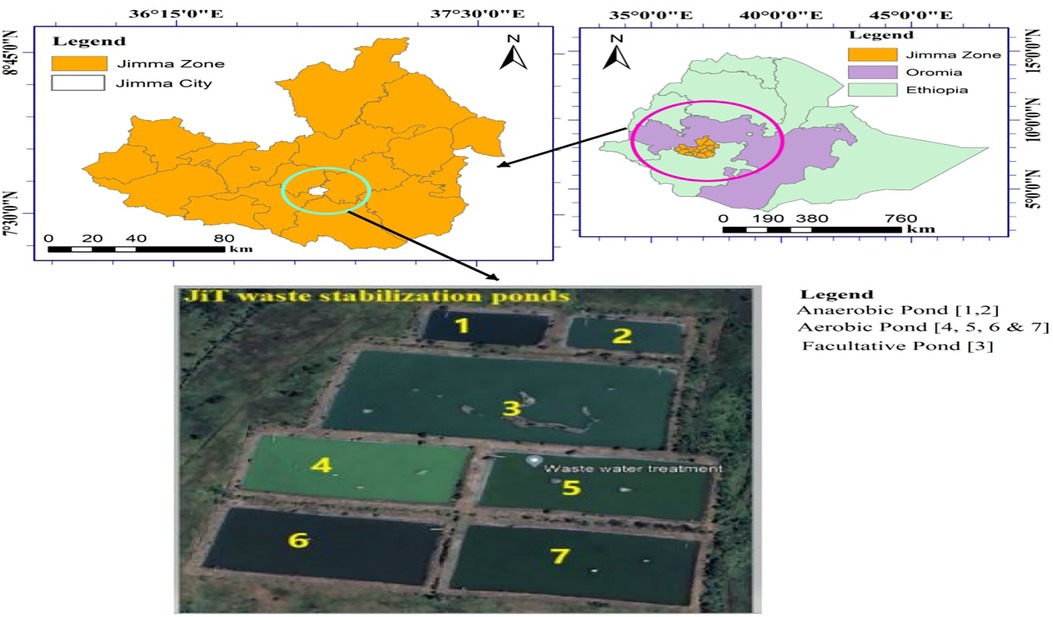
Figure 1 Location Map of waste stabilization ponds in southwestern Ethiopia. Source: Google Earth, 2022.
The waste stabilization pond consists of three components. Each has a distinct size and depth, although the facultative and maturation ponds share the same depth. There are two dimensions to the ponds: anaerobic ponds are deeper and smaller than other ponds. The size and depth are shown as follows:
A = anaerobic pond (2 in number) = 77.94 (L) × 46.49 (W) × 4.75 (D) m
F = facultative pond (1 in number) = 193.83 (L) × 101.53 (W) × 2.10 (D) m
M = maturation pond (4 in number) = 122.83 (L) × 65.61 (W) × 2.10 (D) m
Study design
A laboratory-based cross-sectional study design was employed to assess the levels of heavy metals in wastewater and fish tissues (muscle, gill, and liver) collected from the WSPs. Health risk estimation models were employed to assess the human health risks from fish consumption.
Sample collection
The wastewater samples were collected from the surface (0–50 cm), middle (1–1.5 m), and bottom (2.10 m) of the facultative and maturation ponds using a heart valve water sampler, as described in a previous study (22). To ensure the accuracy of the analyses, the samples were then homogenized (23). The wastewater samples were collected in triplicate from each point using 300 ml polyethylene bottles, which were rinsed twice using distilled water, followed by the wastewater samples before the sample collection. The samples were then stored by adding 2.5 ml of concentrated HNO3 to prevent heavy metal precipitation and algal growth, preserved in the cold chain, and analyzed further in the laboratory of the Department of Environmental Health Science & Technology of Jimma University.
A total of 32 Oreochromis niloticus species were collected from the facultative and maturation ponds. The fish samples were collected by local fishermen using gill nets and wrapped in plastic biosafety bags and then were transported using a cold chain storage to the laboratory for the identification, dissection, and collection of fish tissues (muscle, gill, and liver) for the heavy metal analysis (24, 25).
Sample preparation, digestion, and analysis
Wastewater: 100 ml of the filtered wastewater sample was pipetted into a digestion flask with 10 ml of aqua regia (a mixture of HNO3 and HCl) in a 3:1 ratio. When the sample stopped reacting with HNO3 and HCl, the flask was placed on a hot plate and heated at 60°C for 30 min. The sample was then allowed to cool before adding 5 ml of H2O2 until a clear solution was observed. The content of the flask was transferred into a 50 ml volumetric flask and diluted to the mark of 0.01 N HNO3 according to a previous study (26). All the procedures were performed in the fume hood (27).
Fish: In the laboratory, the samples were cleaned by washing with distilled water. Subsequently, fish tissues, such as muscle, gill, and liver, were separated and sliced into small pieces using a stainless steel knife. Each tissue was then washed with distilled water and dried in an oven at 103°C for 24 h. A 3 g portion was taken into a Teflon beaker and mixed with 5 ml of 70% HNO3 (Spectrosol). The beaker was placed on a hot plate and heated at 60°C for 30 min. After cooling the beaker, 15 ml of HNO3 was added and gradually heated to 120°C before removing it from the hot plate once the temperature reached 150°C, turning the contents black. The beaker was allowed to cool before adding 5 ml of hydrogen peroxide (ACS reagent, Aldrich, UK). The sample was then filtered into a 100 ml volumetric flask. The sample blank was prepared in the same manner as the sample preparation. Finally, the treated samples were cooled and filtered using the Whatman No. 42 filter paper. The contents of the beaker were diluted up to 50 ml with 0.01 N HNO3 for analysis (28–30).
The analysis of the physicochemical parameters in the wastewater samples involved the use of a multiparameter probe (HQ40d) for the field monitoring of the pH, temperature, dissolved oxygen (DO), and electrical conductivity. Turbidity was measured using a turbidity meter (EUTECH TN-100, Singapore). Chloride, total suspended solids (TSS), BOD, nitrate, and phosphate were analyzed in the Department of Environmental Health Science & Technology of Jimma University. The nitrate and phosphate concentrations were determined using a spectrophotometer (DR 5000) at a wavelength of 410 nm and 690 nm, respectively (31).
Heavy metal analysis: Heavy metals (Pb, Cd, As, and Hg) were detected in the pre-treated samples of wastewater and fish tissues through micro plasma atomic emission spectrometry (Agilent 4210 MP-AES) for Pb and Cd and hydride-generated atomic absorption spectrometry (HGAAS, novAA 400P, Germany) for As and Hg. The prepared sample was sent to the Ethiopian Food and Drug Authority and the Ethiopian Construction Design and Supervision Work Authority in Addis Ababa for laboratory analysis. The wavelength and detection limits of each heavy metal were as follows: 405.781 nm and 0.005 mg L−1 for Pb; 193.7 nm, 228.80 nm, and 0.005 mg L−1 for Cd; 253.7 nm, 253.65 nm, and 0.0001 mg L−1 for Hg; and 193.7 nm and 0.0001 mg L−1 for As.
Bioaccumulation factors: The accumulation of toxic metals in fish was calculated with a bioaccumulation factor (BAF) as the ratio of the concentration of an individual heavy metal in the fish tissues to the concentration of the metals in the water (32). The BAF was estimated based on a study done by Kumar (16).
Health risk assessment
The possible public health risks of toxic metals through fish consumption from the study area were evaluated. The data and reference values for the input parameters and the heavy metal contents detected in the fish muscles used for the human health risk assessment are summarized in Table 1.
Estimated daily intake
The estimated daily intake (EDI) was used to assess the susceptibility to heavy metals in children and adults from direct consumption exposure pathways. The EDI of heavy metals from muscle consumption was assessed by using the concentration of heavy metals in fish samples, daily ingestion of fish by the community, and average Body weight (BW) by using the method followed in the previous studies (39, 41):
FIR is the daily fish consumption rate. Although people around fish sources are usually meat consumers, consumption lifestyles have currently been changing to fish in regions and societies where fixed and adequate fish stock is available. In such societies, the annual fish ingestion can surpass 10 kg/capita (19). Accordingly, the daily mean fish consumption rates (CR) projected for adults and children were considered to be 0.03 and 0.016 kg/person/day, respectively (33). These data were taken from previous studies because of the absence of national per capita fish consumption databases in the country.
MC is the concentration of heavy metals (by milligrams per kilogram) detected in fish meat.
BWav denotes the mean body weights set by the WHO as 15 kg and 60 kg for Ethiopian children and adults, respectively (34).
Daily consumption limit
In terms of carcinogenic risk, the following model predicted the maximum permitted daily ingestion rate or limit (CRlim) of metals in fish (kg day−1) (40):
where CRlim is the maximum allowable daily consumption rate or limit of contaminated fish (kg day−1); BW is the mean BW of the consumer population (kg); RfD stands for the oral reference dose (mg kg−1day−1); and MC is the metal concentration in the edible part of fish (mg kg−1) (39).
In terms of non-carcinogenic risk, the maximum allowed daily intake of fish was estimated using the following equation (40):
where CRlim is the most permissible daily consumption rate or restriction of contaminated fish tissue (kg day−1); BW is the mean BW of the consumer population (kg); RfD is the oral reference dose (mg kg−1 day−1); and MC is the concentration of heavy metals determined in fish tissue (mg kg−1).
Non-carcinogenic risk assessment
The non-carcinogenic risk was explored using the target hazard quotient (THQ), which is a measure of the risk level (non-carcinogenic) associated with pollutant exposure. The hazard quotient was computed using the following equation (40):
The oral reference dose (RfD) for Cd = 0.001, Pb = 0.004, As = 0.0003, and Hg = 0.0001 (37).
While the oral RfD = oral reference dose of a substance (mg/kg/day) based on the oral intake for every metal for a grown-up client with an average BW of 60 kg, THQ < 1.0 points out that negative fitness chances are not likely to occur. If the THQ is greater than or equal to at least one, it is most probable that an adverse health hazard could happen (42).
Exposure to two or more metal contaminants may have additive health consequences. Therefore, the cumulative health risk was calculated by summing the THQ [also known as the hazard index (HI)] (43).
A value of HI greater than 1 shows a greater health risk.
Carcinogenic risk assessment
The target cancer risk (TR) was computed to assess the carcinogenic effects. The method for estimating the TR is also available in the USEPA Region III Risk-Based Concentration Table (37). The model for estimating the TR is as follows (29):
where CSF is the cancer slope factor (mg kg−1 day−1), which was 1.5 mg kg−1 day−1 for As, 0.0085 mg kg−1 day−1 for Pb, and 0.38 for Cd, while the other parameters have been defined previously. The TCR was estimated for As, Cd, and Pb since these elements may promote both non-carcinogenic and carcinogenic effects depending on the exposure dose. As and Cd are known as Group A carcinogens, and Pb is known as a Group B carcinogen.
Data management, analysis, and quality assurance
The collected data were coded and entered into Epi Data version 3.1 before being exported to SPSS (version 26) for analysis. Descriptive analysis was conducted for each variable, and correlation analysis was performed to analyze the intermetallic association and the significance level with physicochemical parameters. A paired t-test was conducted to determine the statistical significance between two sampling points. To evaluate whether heavy metal concentrations in fish tissues varied based on the site, a one-way analysis of variance was employed at a significance level of α = 0.05. The concentrations of all metals were reported in micrograms per liter for wastewater and in milligrams per kilogram on a dry weight basis for fish tissues.
To maintain the quality of the data, laboratory instruments were calibrated, bank measurements were performed, and triplicate analyses were made for each sample. Stock standard solutions (1,000 mg L−1) containing 2% HNO3 of Pb, Cd, Hg, and As (Buck Scientific Puro-Graphic) were used. The calibration curve was determined using serial dilutions. All the reagents used in the experiments were analytically pure. The samples were then digested without delay and analyzed by HGAAS and MP-AES following documented procedures.
Ethical considerations
The study was carried out following acceptance of an ethical clearance letter from Jimma University's Institute of Health Science. To get the necessary cooperation for the study, the Institute of Health, Jimma University, issued a letter of support to the Institute of Technology, Jimma University and laboratory facilities.
Results
Physicochemical characteristics of the wastewater
The assessments of various physicochemical parameters, namely, pH, temperature, turbidity, BOD, DO, conductivity, TSS, chloride, nitrate, and phosphate, were conducted using standard methods as described in the American Public Health Association (44). The average pH and DO values increased from the inlet to the outlet of the pond, while the other parameters showed a decreasing order along the wastewater treatment process (Table 2).
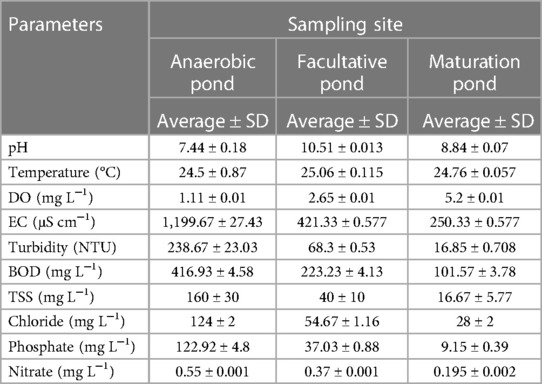
Table 2 Level of physicochemical characteristics in wastewater samples from a waste stabilization pond in southwestern Ethiopia.
Heavy metal concentration in the wastewater samples
The calibration curves for Cd, Pb, As, and Hg were obtained by using standard solutions prepared from their respective stock solutions. The correlation coefficients of heavy metals ranged from 0.996 to 0.999, indicating a strong relationship. The correlation coefficients of the heavy metals were determined using prepared standards vs. their corresponding absorbance. Finally, quality results were obtained from the sample analysis for each heavy metal using HGAAS and MP-AES.
The heavy metals were found to be in decreasing order of concentration (µg L−1), that is, Cd > Pb > As > Hg, in both the facultative and maturation ponds. Cd showed the highest concentration among the heavy metals throughout, while Hg had the lowest concentration. The maximum levels of all heavy metals were obtained from the facultative pond. Furthermore, the Cd and Pb concentrations exceeded the acceptable levels established by the WHO (32) and the USEPA (36), which may pose a huge threat to public health and the natural environment (Table 3).

Table 3 Mean and standard deviation of the heavy metal concentration (μg L−1) in wastewater from a waste stabilization pond in southwestern Ethiopia compared to the international standard.
The correlation analyses performed on the data enabled the identification of the possible common characteristics of heavy metals in wastewater. Cd exhibited a negative correlation with As (r2 = −0.314, p < 0.05) and a positive correlation with Hg (r = 0.239, p < 0.05). Pb showed a strong positive correlation with Hg (r2 = 0.779) and a moderately positive correlation with As and cadmium (r2 = 0.229, 0.415). The significant positive correlations obtained among some heavy metals proved that they may have similar accumulation behaviors or originate from the same environmental sources of pollution (45).
The association between heavy metals and other physicochemical parameters has also been assessed using the Pearson correlation coefficient (r), and the association was statistically significant at two-tailed (p < 0.01). Pb showed a strong positive correlation with the pH, EC, turbidity, BOD, Cl, PO4−3, and NO3, and the association was statistically significant at two-tailed (p < 0.05). Moreover, Hg and Pb showed a strong negative correlation with DO. The significant positive correlations of Hg and Pb with other physicochemical parameters may confirm that a considerable share of wastewater parameters is associated with the adsorption and oxidation of trace metals. The lack of a significant correlation between heavy metals and other wastewater parameters might be caused by the compositional variety controlling heavy metals. A paired sample t-test analysis revealed no significant variation for the heavy metals being studied, except for Hg, which demonstrated a substantial statistical difference between facultative and maturation ponds (t = 6.450, p = 0.023).
Heavy metal concentration in the fish tissues
Biometric data, such as the length and weight of O. niloticus fish collected from the WSPs, were measured. The average length and weight of O. niloticus (n = 32) collected from the facultative and maturation ponds were 24.76 ± 0.64 cm and 108.33 ± 7.89 g, 16.54 ± 1.68 cm, and 82.33 ± 4.67 g, respectively. It can be used to provide information concerning the aquatic health and environment.
The heavy metal concentrations expressed as mg kg−1 dry weight were detected in the muscle, liver, and gill of O. niloticus through HGAAS and MP-AES. In the liver, O. niloticus accumulated the highest concentration of Pb, which ranged from 0.34 mg kg−1 to 0.37 mg kg−1, and the gill exhibited the highest value of Cd, which ranged from 0.345 mg kg−1 to 0.406 mg kg− in the maturation and facultative ponds, respectively. However, the muscle showed the lowest concentration of all heavy metals. It can be noticed that different organs exhibited different patterns of accumulation, that is, gill, Cd > Pb > Hg > As; liver, Pb > Cd > Hg > As; and muscle, Pb > Cd > Hg > As from both ponds (Figures 2 and 3).
The results of the analysis of variance revealed that there are significant differences in the metal concentrations in the different fish tissues (p < 0.05). Mercury was selectively accumulated in all types of fish tissues, with a statistically significant difference at p < 0.05. The Cd concentration showed significant differences between the liver and the muscle (p < 0.05), whereas As and Pb did not show significant differences between the fish tissues. It indicates the accumulation efficiency of any particular metal in fish tissues.
The highest concentrations of lead and cadmium were recorded in the muscle of O. niloticus. The arsenic concentration in the muscles was above the MPL of 0.01 mg kg−1 as the FAO/WHO recommended standard (46) (Figure 4).
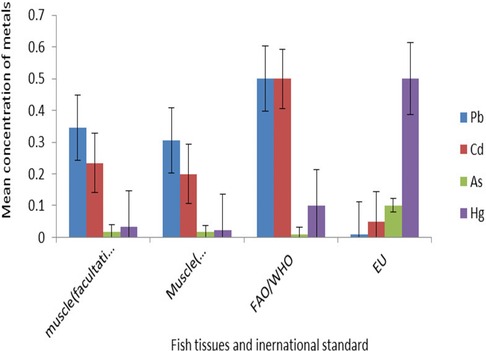
Figure 4 Comparison of the mean concentration of heavy metals in muscle with international standards.
The bioaccumulation of toxic metals in the fish tissues collected from the WSPs was determined. The maximum BAF of Hg in the liver of O. niloticus was calculated using Equation 1, which was 1,302.5 and 764.73 at the maturation and facultative ponds, respectively. It was observed that the bioaccumulation factors were increased in the order of Cd < Pb < As << Hg for all tissues (Table 4). This is a clear indication that the bioaccumulation factors of Hg in all O. niloticus tissues were higher compared with other metals.
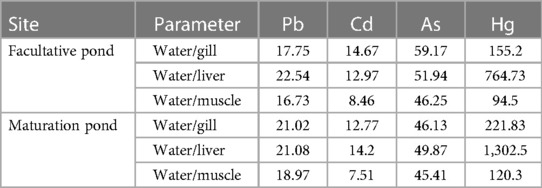
Table 4 Bioaccumulation factor of heavy metals in the tissues of O. niloticus from a waste stabilization pond in southwestern Ethiopia.
Human health risk assessment
EDI
The EDI of heavy metals was evaluated according to the average concentrations of each metal in fish muscle and the respective daily consumption rate. The daily intake of As, Cd, Hg, and Pb through the consumption of fish muscle from WSPs by adults and children was estimated (Equation 2). Carcinogenic and non-carcinogenic effects of the contaminants, the maximum allowable daily consumption of fish was determined using Equation 3, 4 respectively. The results are expressed as per unit BW per day (mg kg−1 day−1) (Table 5). The highest toxic metal consumption by fish ingestion was linked to Pb, which was estimated from 3.18 × 10−5 mg kg−1 day−1 to 3.5 × 10−5 mg kg−1 day−1 for adult consumers and 6.78 × 10−5 mg kg−1 day−1 to 7.19 × 10−5 mg kg−1 day−1 for children.
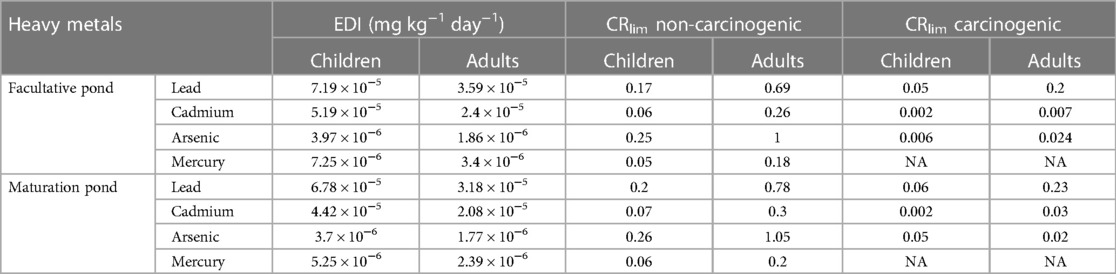
Table 5 Estimated daily intake (EDI) of heavy metals in the muscle of O. niloticus from waste stabilization ponds in southwestern Ethiopia in 2022.
Non-carcinogenic risk assessment
The non-carcinogenic THQ and HI of the four heavy metals due to muscle consumption from the WSP in Jimma were estimated by Equation 5. The THQ of the contaminants declined in the following order: Hg > Cd > Pb > As. The THQ is a ratio between potential exposure to a particular toxic heavy metal and the respective oral reference dose. It is employed to calculate the potential health effects related to chronic exposure to nutritional metals. The risk index (HI) risk values were 0.069 and 0.156 at the facultative pond and 0.059 and 0.126 at the maturation pond for adults and children, respectively, for the entire study duration (Table 6). HI is the mathematical sum of the calculated THQ values. The contribution of individual THQ values to the HI was evaluated (Equation 6), and the results showed that Hg contributed more than 49.27% to the combined THQ through this ingestion exposure pathway of edible muscles. Consequently, for the non-carcinogenic effects, further consideration should be given to Hg pollution in the study area.

Table 6 Target hazard quotient (THQ) and hazard index (HI) of heavy metals from consumption of O. niloticus from a waste stabilization pond in southwestern Ethiopia in 2022.
Carcinogenic risk assessment
The carcinogenic risks were estimated by calculating the incremental probability of an individual developing cancer over a lifetime as a result of exposure to potential carcinogen metals using Equation 7. According to the findings, Pb, As, and Cd were below the safe limit of 1 × 10−4. Pb, As, and Cd exposure had no carcinogenic risk on adults or children. When the risk levels were compared, the concentration of Cd was higher than that of As and Pb, demonstrating that Cd is the primary pollutant responsible for carcinogenesis. Comparing the health risks between adults and children, children have exhibited more carcinogenic risks than adults, indicating that children are vulnerable to toxic metals in fish muscle (Table 7).
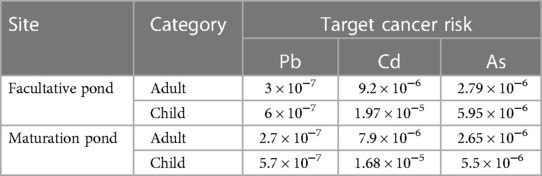
Table 7 Target cancer risk of heavy metals due to consumption of O. niloticus from a waste stabilization pond in southwestern Ethiopia in 2022.
Discussion
The highest concentration of Cd was found in the facultative pond, exceeding the WHO limit of 3 μg L−1. For several decades, this area has been associated with intense cropping and substantial inputs of agrochemicals, such as phosphate fertilizer. Other sources of Cd include welding, fertilizer, surface runoff, and solid waste disposal, which all contribute to the nickel–cadmium battery's leakage into neighboring water bodies (47, 48). The Cd released from these sources affects aquatic environments, which can easily affect humans through the food chain, drinking water, and breathing. The USEPA (2004) recognized Cd as a probable human carcinogen, with both acute and chronic exposures resulting in detrimental health effects for people and animals (49).
The highest concentration of Pb was observed in the facultative pond, which was above the legal limits set by the USEPA in world river water (WRW) (3 μg L−1). In developing countries, leaded gasoline is still widely used, which considerably increases the amount of Pb in urban soils due to its non-degradability (50) and is finally disposed of in surrounding water bodies. Pesticides, car washing at the side of the streams, and lead pipe from the city's old and corroded wastewater distribution line were the other sources of elevated lead levels (51). According to several studies, lead predominates in the majority of regularly used metallic products, such as paints, cables, pipelines, and insecticides, and this may account for the higher levels seen in wastewater (52, 53).
The mean concentrations of As were 0.38 ± 0.12 μg L−1 and 0.39 ± 0.10 μg L−1 in the maturation and facultative ponds, respectively. Agricultural activities and the use of arsenic trioxide insecticides are responsible for the highest concentration of As (54, 55). WSPs are located in the city, where pesticides, insecticides, herbicides, pigments, and the use of wood preservatives containing arsenic have contributed to environmental contamination (54). More significantly, the persistence of As in water bodies causes pollution, kills aquatic and terrestrial creatures, and eventually has an impact on human health (56).
A higher Hg (0.35 ± 0.01 μg L−1) concentration was detected in the facultative pond, which is much lower than the legal limits set by the WHO and the USEPA (57). This is not unusual because 80% and 90% of heavy metals in influent wastewater are known to accumulate in sewage sludge. This value is lower than the finding by Astatkie et al. (58), who reported that the Hg value was 12.1 ± 1.7 μg L−1 in Awetu River. Thus, it suggests the elemental mercury contained in dental amalgam, emission of fossil fuels and batteries, combustion of medical waste generated by laboratories and dental clinics, and inorganic mercury from the aquatic environment (59). Anthropogenic activities, such as agriculture, municipal wastewater releases, mining, incineration, and emissions of commercial wastewater, are the most significant sources of mercury contamination (60).
The concentration of As detected in the muscle tissue exceeded the MPL, which is recommended by FAO/WHO (0.01 mg kg−1). Similar As values were found in fish tissue in Ghanaian seawaters, which were 1.52 ± 0.70 mg kg−1 (61). This might be due to the chemical properties and bioavailability of the metal. Arsenic poses a significant risk to humans due to its effects on plasma, liver, kidneys, and cell systems, potentially leading to bone marrow disruption, central necrosis, and cirrhosis (62).
Pb and Cd were bioaccumulated mainly in the liver and gills. The bioaccumulation patterns of pollutants in aquatic organisms are mainly determined by their absorption and elimination rates (63). The anatomical position of the gill tissues allows for direct and constant interaction with external contaminants, resulting in significant metal accumulation patterns. Gills are the main route for the metal ion exchange from water due to their large surface area and ability to rapidly diffuse hazardous metals (64). Lead and cadmium are non-essential and, therefore, have toxic effects on living organisms by accumulating in the tissues and bodies of aquatic organisms and being biomagnified in the food chain, causing physiological damage to human consumers. The health effects of lead and cadmium are cancer and damage to the nervous, urinary, reproductive, circulatory, and respiratory systems (65, 66).
In this study, the THQ and the HI for all heavy metals were lower than 1, indicating that the fish muscle was safe for consumption. This showed that adverse health effects are still unlikely to result from eating 30 g and 16 g of fish per day for adults and children, respectively, in the study area. Accordingly, Hg exhibited a higher non-carcinogenic risk than other metals due to its low RfD value. Another study also estimated the maximum THQ for mercury from the intake of fish tissues from Lake Hawasa and the Boacha River, which ranged from 0.29 to 2.02 (29).
The highest TCR value was obtained due to Cd exposure; however, it was within the acceptable range. A cancer risk lower than 10–6 represents the metals' insignificant exposure, ranging from 10–6 to 10–4 means an acceptable range, and higher than 10–4 indicates a terrible exposure (67). Furthermore, Cd causes endocrine disruption, which can result in the failure of key organs, such as the kidney and the brain. Long-term Cd exposure can cause problems with the blood circulation system, bone weakening, and the prostate. Cadmium has been shown to cause cancer in both people and animals (68). As claimed, a prolonged Cd exposure could promote breast cancer. Girls and boys aged 5 years old with low levels of Cd exposure are linked to lower IQ, and boys aged 10 years old with low levels of Cd exposure are linked to reduced effectiveness (69).
Conclusion
This study presented data on levels of heavy metals in wastewater and tissues of O. niloticus from the WSPs in southwestern Ethiopia. The results obtained show high concentrations of Cd, Pb, As, and Hg in the wastewater, indicating that it is unsuitable for fishing to prevent human exposure and health risks. It can be concluded that toxic metals bioaccumulate in fish when arsenic exceeds the permissible limit set for heavy metals by the WHO and the USEPA. Therefore, these fish are not fit for consumption. These results call for significant concerns and highlight the need for constant monitoring of the WSPs to ensure the safety and well-being of people associated with them.
Data availability statement
The original contributions presented in the study are included in the article/Supplementary Material, further inquiries can be directed to the corresponding author.
Ethics statement
The manuscript presents research on animals that do not require ethical approval for their study.
Author contributions
GB: Conceptualization, Data curation, Formal Analysis, Funding acquisition, Investigation, Methodology, Project administration, Resources, Software, Supervision, Validation, Visualization, Writing – original draft, Writing – review & editing. HL: Writing – original draft, Writing – review & editing. SM: Methodology, Supervision, Writing – original draft, Writing – review & editing. DD: Methodology, Supervision, Writing – original draft, Writing – review & editing.
Funding
The authors declare that no financial support was received for the research, authorship, and/or publication of this article.
Acknowledgments
The authors thank Jimma University and Bule Hora University for their valuable support and the Ethiopian Food and Drug Control Authority (EFDA) and Ethiopian Construction Design and Supervision Work Authority (ECDSWA) for their help in the laboratory analyses of the samples.
Conflict of interest
The authors declare that the research was conducted in the absence of any commercial or financial relationships that could be construed as a potential conflict of interest.
Publisher's note
All claims expressed in this article are solely those of the authors and do not necessarily represent those of their affiliated organizations, or those of the publisher, the editors and the reviewers. Any product that may be evaluated in this article, or claim that may be made by its manufacturer, is not guaranteed or endorsed by the publisher.
References
1. Islam SM, Rohani MF, Zabed SA, Islam MT, Jannat R, Akter Y, et al. Acute effects of chromium on hemato-biochemical parameters and morphology of erythrocytes in striped catfish Pangasianodon hypophthalmus. Toxicol Rep. (2020) 7:664–70. doi: 10.1016/j.toxrep.2020.04.016
2. Biswas C, Soma SS, Rohani MF, Rahman MH, Bashar A, Hossain MS. Assessment of heavy metals in farmed shrimp, Penaeus monodon sampled from Khulna, Bangladesh: an inimical to food safety aspects. Heliyon. (2021) 7(3):147777. doi: 10.1016/j.heliyon.2021.e06587
3. Nargis A, Habib A, Harun HB, Sarker MSI, Jin R, Liu G, et al. Status of multielement in water of the river Buriganga, Bangladesh: aquatic chemistry of metal ions in polluted river water. Emerg Contam. (2021) 7:99–115. doi: 10.1016/j.emcon.2021.03.001
4. Budi HS, Catalan Opulencia MJ, Afra A, Abdelbasset WK, Abdullaev D, Majdi A, et al. Source, toxicity and carcinogenic health risk assessment of heavy metals. Rev Environ Health. (2024) 39(1):77–90. doi: 10.1515/reveh-2022-0096
5. Akter S, Jahan N, Rohani MF, Akter Y, Shahjahan M. Chromium supplementation in diet enhances growth and feed utilization of striped catfish (Pangasianodon hypophthalmus). Biol Trace Elem Res. (2021) 199(12):1–9. doi: 10.1007/s12011-021-02608-2
6. Edo GI, Samuel PO, Oloni GO, Ezekiel GO, Ikpekoro VO, Obasohan P, et al. Environmental persistence, bioaccumulation, and ecotoxicology of heavy metals. Chem Ecol. (2024) 40(3):1–28. doi: 10.1080/02757540.2024.2306839
7. Alam M, Rohani MF, Hossain MS. Heavy metals accumulation in some important fish species cultured in commercial fish farm of Natore, Bangladesh and possible health risk evaluation. Emerg Contam. (2023) 9(4):100254. doi: 10.1016/j.emcon.2023.100254
8. Al-Busaidi M, Yesudhason P, Al-Mughairi S, Al-Rahbi W, Al-Harthy K, Al-Mazrooei N, et al. Toxic metals in commercial marine fish in Oman with reference to national and international standards. Chemosphere. (2011) 85(1):67–73. doi: 10.1016/j.chemosphere.2011.05.057
9. Hina B, Rizwani GH, Naseem S. Determination of toxic metals in some herbal drugs through atomic absorption spectroscopy. Pak J Pharm Sci. (2011) 24(3):353–8. http://www.pakmedinet.com/PJPS21715269
10. Duruibe JO, Ogwuegbu M, Egwurugwu J. Heavy metal pollution and human biotoxic effects. Int J Phys Sci. (2007) 2(5):112–8. http://www.academicjournals.org/IJPS
11. Sapkota A, Sapkota AR, Kucharski M, Burke J, McKenzie S, Walker P, et al. Aquaculture practices and potential human health risks: current knowledge and future priorities. Environ Int. (2008) 34(8):1215–26. doi: 10.1016/j.envint.2008.04.009
12. Al-Hossainy AF, Mohamed AE, Hassan FS, Abd Allah M. Determination of cadmium and lead in perch fish samples by differential pulse anodic stripping voltammetry and furnace atomic absorption spectrometry. Arab J Chem. (2017) 10:S347–S54. doi: 10.1016/j.arabjc.2012.09.005
13. Mansour S, Sidky M. Ecotoxicological studies. 3. Heavy metals contaminating water and fish from Fayoum Governorate, Egypt. Food Chem. (2002) 78(1):15–22. doi: 10.1016/S0308-8146(01)00197-2
14. Erdoğrul Ö, Ateş DA. Determination of cadmium and copper in fish samples from Sir and Menzelet Dam Lake Kahramanmaraş, Turkey. Environ Monit Assess. (2006) 117:281–90. doi: 10.1007/s10661-006-0806-1
15. Ali AS, US SA AR. Effect of different heavy metal pollution on fish. Res J Chem Environ Sci. (2014) 2(1):74–9. http://www.aelsindia.com/
16. Maurya PK, Malik D, Yadav KK, Kumar A, Kumar S, Kamyab H. Bioaccumulation and potential sources of heavy metal contamination in fish species in River Ganga basin: possible human health risks evaluation. Toxicol Rep. (2019) 6:472–81. doi: 10.1016/j.toxrep.2019.05.012
17. Alderson MP, dos Santos AB, Mota Filho CR. Reliability analysis of low-cost, full-scale domestic wastewater treatment plants for reuse in aquaculture and agriculture. Ecol Eng. 2015;82:6–14. doi: 10.1016/j.ecoleng.2015.04.081
18. Kumar D, Asolekar SR. Significance of natural treatment systems to enhance reuse of treated effluent: a critical assessment. Ecol Eng. (2016) 94:225–37. doi: 10.1016/j.ecoleng.2016.05.067
19. FAO I. The State of World Fisheries and Aquaculture 2016. Contributing to Food Security and Nutrition for All. Rome: FAO (2016). p. 175–90. Available online at: http://www.fao.org/publications
20. Koba O, Grabicova K, Cerveny D, Turek J, Kolarova J, Randak T, et al. Transport of pharmaceuticals and their metabolites between water and sediments as a further potential exposure for aquatic organisms. J Hazard Mater. (2018) 342:401–7. doi: 10.1016/j.jhazmat.2017.08.039
21. Desye B, Belete B, Amare Alemseged E, Angaw Y, Asfaw Gebrezgi Z. Evaluation of waste stabilization pond efficiency and its effluent water quality: a case study of Kito Furdisa Campus, Jimma University, Southwest Ethiopia. The Scientific World Journal. (2022) 2022:2800034. doi: 10.1155/2022/2800034
22. Asgedom AG, Desta MB, Gebremedh Y. Bioaccumulation of heavy metals in fishes of Hashenge Lake, Tigray, Northern Highlands of Ethiopia. Am J Chem. (2012) 2(6):326–34. doi: 10.5923/j.chemistry.20120206.06
23. Duan B, Zhang W, Zheng H, Wu C, Zhang Q, Bu Y. Comparison of health risk assessments of heavy metals and as in sewage sludge from wastewater treatment plants (WWTPs) for adults and children in the urban district of Taiyuan, China. Int J Environ Res Public Health. (2017) 14(10):1194. doi: 10.3390/ijerph14101194
24. Gebremedhin K, Berhanu T. Determination of some selected heavy metals in fish and water samples from Hawassa and Ziway lakes. Sci J Anal Chem. (2015) 3(1):10–6. doi: 10.11648/j.sjac.20150301.13
25. Mensoor M, Said A. Determination of heavy metals in freshwater fishes of the Tigris River in Baghdad. Fishes. (2018) 3(2):23. doi: 10.3390/fishes3020023
26. Douglas R, Albert G, Reuben O, Paul O, Hellen N, Boniface G, et al. Assessment of heavy metal concentrations (Cu, Cd, Pb, and Zn) in wastewater from Gusii treatment plant in Kisii county, Kenya. Pan Afr Sci J. (2022) 1(02):122–38. doi: 10.47787/pasj.v1i02.12
27. Perkin E, Elmer C. Analytical Methods for Atomic Absorption Spectroscopy. USA: The Perkin Elmer Corporation (1996). p. 5–6.
28. Hossain MB, Bhuiyan NZ, Kasem A, Hossain MK, Sultana S, Nur A-AU, et al. Heavy metals in four marine fish and shrimp species from a subtropical coastal area: accumulation and consumer health risk assessment. Biology (Basel). (2022) 11(12):1780. doi: 10.3390/biology11121780
29. Samuel B, Sorsa S, Daniel F, Riise G, Zinabu G. Heavy metals in fish muscle from an Ethiopian Rift-Valley Lake (Hawassa) and a neighboring stream (Boicha): assessment of human health risks. J Appl Sci Environ Manag. (2020) 24(8):1409–18. doi: 10.4314/jasem.v24i8.16
30. Olowu R, Ayejuyo O, Adewuyi G, Adejoro I, Denloye A, Babatunde A, et al. Determination of heavy metals in fish tissues, water and sediment from Epe and Badagry lagoons, Lagos, Nigeria. J Chem. (2010) 7:215–21.
31. Oliveira S, Souza R, Nunes É, Carvalho C, Menezes G, Marcon JL, et al. Tolerance to temperature, pH, ammonia and nitrite in cardinal tetra, Paracheirodon axelrodi, an Amazonian ornamental fish. Acta Amazonica. (2008) 38:773–9. doi: 10.1590/S0044-59672008000400023
33. Yohannes YB, Ikenaka Y, Saengtienchai A, Watanabe KP, Nakayama SM, Ishizuka M. Concentrations and human health risk assessment of organochlorine pesticides in edible fish species from a rift valley lake—Lake Ziway, Ethiopia. Ecotoxicol Environ Saf. (2014) 106:95–101. doi: 10.1016/j.ecoenv.2014.04.014
35. Walpole SC, Prieto-Merino D, Edwards P, Cleland J, Stevens G, Roberts I. The weight of nations: an estimation of adult human biomass. BMC Public Health. (2012) 12(1):1–6. doi: 10.1186/1471-2458-12-439
36. Tong R, Cheng M, Zhang L, Liu M, Yang X, Li X, et al. The construction dust-induced occupational health risk using Monte Carlo simulation. J Cleaner Prod. (2018) 184:598–608. doi: 10.1016/j.jclepro.2018.02.286
37. Epa U. Regional Screening Level (RSL) Summary Table (TR= 1E-06, HQ= 1). Washington DC: US Environmental Protection Agency (2020).
38. Endale YT, Ambelu A, Mees B, Du Laing G. Exposure and health risk assessment from consumption of Pb contaminated water in Addis Ababa, Ethiopia. Heliyon. (2021) 7(9):e07946. doi: 10.1016/j.heliyon.2021.e07946
39. Yu Y, Wang X, Yang D, Lei B, Zhang X, Zhang X. Evaluation of human health risks posed by carcinogenic and non-carcinogenic multiple contaminants associated with consumption of fish from Taihu Lake, China. Food Chem Toxicol. (2014) 69:86–93. doi: 10.1016/j.fct.2014.04.001
40. Miri M, Akbari E, Amrane A, Jafari SJ, Eslami H, Hoseinzadeh E, et al. Health risk assessment of heavy metal intake due to fish consumption in the Sistan region, Iran. Environ Monit Assess. (2017) 189(11):1–10. doi: 10.1007/s10661-017-6286-7
41. Shakeri A, Shakeri R, Mehrabi B. Potentially toxic elements and persistent organic pollutants in water and fish at Shahid Rajaei Dam, north of Iran. Int J Environ Sci Technol (Tehran). (2015) 12(7):2201–12. doi: 10.1007/s13762-015-0754-9
42. Abdel-Khalek AA, Elhaddad E, Mamdouh S, Marie M-AS. Assessment of metal pollution around sabal drainage in river Nile and its impacts on bioaccumulation level, metals correlation and human risk hazard using Oreochromis niloticus as a bioindicator. Turk J Fish Aquat Sci. (2016) 16(2):227–39. doi: 10.4194/1303-2712-v16_2_02
43. Giri S, Singh AK. Assessment of human health risk for heavy metals in fish and shrimp collected from Subarnarekha River, India. Int J Environ Health Res. (2014) 24(5):429–49. doi: 10.1080/09603123.2013.857391
44. Association APH. Standard Methods for the Examination of Water and Wastewater. Washington, DC: American Public Health Association (1926).
45. Tytła M. Assessment of heavy metal pollution and potential ecological risk in sewage sludge from municipal wastewater treatment plant located in the most industrialized region in Poland—case study. Int J Environ Res Public Health. (2019) 16(13):2430. doi: 10.3390/ijerph16132430
46. Joint F, Organization WH, Additives WECoF. Evaluation of Certain Food Additives and Contaminants: Seventy-seventh Report of the Joint FAO/WHO Expert Committee on Food Additives. Geneva: World Health Organization (2013).
47. Sharma H, Rawal N, Mathew BB. The characteristics, toxicity and effects of cadmium. Int J Nanotechnol Nanosci. (2015) 3(10):1–9. https://www.researchgate.net/publication/305778858
48. Ayangbenro AS, Babalola OO. A new strategy for heavy metal polluted environments: a review of microbial biosorbents. Int J Environ Res Public Health. (2017) 14(1):94. doi: 10.3390/ijerph14010094
49. Dökmeci AH, Öngen A, Dağdeviren S. Environmental toxicity of cadmium and health effect. J Environ Protect Ecol. (2009) 10(1):84-93. https://hdl.handle.net/20.500.11776/6231
50. Hashmi MZ, Yu C, Shen H, Duan D, Shen C, Lou L, et al. Risk assessment of heavy metals pollution in agricultural soils of Siling reservoir watershed in Zhejiang province, China. BioMed Res Int. (2013) 2013. doi: 10.1155/2013/590306
51. Getaneh Z, Mekonen S, Ambelu A. Exposure and health risk assessment of lead in communities of Jimma town, southwestern Ethiopia. Bull Environ Contam Toxicol. (2014) 93:245–50. doi: 10.1007/s00128-014-1293-7
52. Tyagi R. Assessment of the uptake of toxic heavy metals on cultivation of vegetables of family Solanaceae in contaminated soil (Phd graduate thesis). University of Kota, Kota, Rajasthan (2014).
53. Zeng S-Y, Xin D, Chen J-N. Toxicity assessment of metals in sediment from the lower reaches of the Haihe River Basin in China. Int J Sediment Res. (2013) 28(2):172–81. doi: 10.1016/S1001-6279(13)60029-3
54. Bencko V, Yan Li Foong F. The history of arsenical pesticides and health risks related to the use of Agent Blue. Ann Agric Environ Med. (2017) 24(2):312–6. doi: 10.26444/aaem/74715
55. Wang C, Li W, Guo M, Ji J. Ecological risk assessment on heavy metals in soils: use of soil diffuse reflectance mid-infrared Fourier-transform spectroscopy. Sci Rep. (2017) 7(1):40709. doi: 10.1038/srep40709
56. Gupta DK, Chatterjee S. Arsenic Contamination in the Environment. the Issues and Solutions: Springer (2017).
57. Kendir E, Kentel E, Sanin FD. Evaluation of heavy metals and associated health risks in a metropolitan wastewater treatment plant’s sludge for its land application. Hum Ecol Risk Assess Int J. (2015) 21(6):1631–43. doi: 10.1080/10807039.2014.966590
58. Astatkie H, Ambelu A, Beyene EM. Sources and level of heavy metal contamination in the water of Awetu watershed streams, southwestern Ethiopia. Heliyon. (2021) 7(3):e06385. doi: 10.1016/j.heliyon.2021.e06385
59. Paraquetti HHM, Ayres GA, De Almeida MD, Molisani MM, De Lacerda LD. Mercury distribution, speciation and flux in the Sepetiba Bay tributaries, SE Brazil. Water Res. (2004) 38(6):1439–48. doi: 10.1016/j.watres.2003.11.039
60. Chen C-W, Chen C-F, Dong C-D. Distribution and accumulation of mercury in sediments of Kaohsiung River Mouth, Taiwan. Apcbee Procedia. (2012) 1:153–8. doi: 10.1016/j.apcbee.2012.03.025
61. Nyantakyi A, Wiafe S, Akoto O, Fei-Baffoe B. Heavy metal concentrations in fish from river Tano in Ghana and the health risks posed to consumers. J Environ Public Health. (2021) 2021:5834720. doi: 10.1155/2021/5834720
62. Medeiros RJ, dos Santos LMG, Freire AS, Santelli RE, Braga AMC, Krauss TM, et al. Determination of inorganic trace elements in edible marine fish from Rio de Janeiro State, Brazil. Food Control. (2012) 23(2):535–41. doi: 10.1016/j.foodcont.2011.08.027
63. Streit B. Bioaccumulation of contaminants in fish. Fish Ecotoxicol. (1998) 10:353–87. doi: 10.1007/978-3-0348-8853-0_12
64. Maetz J. Fish gills: mechanisms of salt transfer in fresh water and sea water. Phil Trans R Soc Lond B Biol Sci. (1971) 262(842) 86:209–49. doi: 10.1098/rstb.1971.0090
65. Rahimzadeh MR, Rahimzadeh MR, Kazemi S, Moghadamnia A-A. Cadmium toxicity and treatment: an update. Caspian J Intern Med. (2017) 8(3):135. doi: 10.22088/cjim.8.3.135
66. León OLL, Pacheco JMS. Effects of Lead on Reproductive Health. Lead Chemistry. London, UK: IntechOpen (2020). doi: 10.5772/intechopen.91992
67. Baki MA, Hossain MM, Akter J, Quraishi SB, Shojib MFH, Ullah AA, et al. Concentration of heavy metals in seafood (fishes, shrimp, lobster and crabs) and human health assessment in Saint Martin Island, Bangladesh. Ecotoxicol Environ Saf. (2018) 159:153–63. doi: 10.1016/j.ecoenv.2018.04.035
68. Buha A, Matovic V, Antonijevic B, Bulat Z, Curcic M, Renieri EA, et al. Overview of cadmium thyroid disrupting effects and mechanisms. Int J Mol Sci. (2018) 19(5):1501. doi: 10.3390/ijms19051501
Keywords: fish, human health risk, heavy metals, waste stabilization pond, southwestern Ethiopia
Citation: Berhanu G, Lemma H, Mekonnen S and Dadi D (2024) Heavy metals in wastewater and fish collected from waste stabilization pond and human health risks in southwestern Ethiopia. Front. Environ. Health 3:1386827. doi: 10.3389/fenvh.2024.1386827
Received: 16 February 2024; Accepted: 28 May 2024;
Published: 25 June 2024.
Edited by:
Rakesh Kumar, Auburn University, United StatesReviewed by:
Pawan Kumar Rose, Chaudhary Devi Lal University, IndiaKavita Verma, Indian Institute of Science (IISc), India
Melvin Samuel, University of Wisconsin–Milwaukee, United States
© 2024 Berhanu, Lemma, Mekonnen and Dadi. This is an open-access article distributed under the terms of the Creative Commons Attribution License (CC BY). The use, distribution or reproduction in other forums is permitted, provided the original author(s) and the copyright owner(s) are credited and that the original publication in this journal is cited, in accordance with accepted academic practice. No use, distribution or reproduction is permitted which does not comply with these terms.
*Correspondence: Girma Berhanu, Z2JlcmhhbnUyMzRAZ21haWwuY29t
†ORCID:
Girma Berhanu
orcid.org/0009-0007-5710-4176