Corrigendum: Methylmercury content in soil and litter from the Amazonian rainforest and its potential fate during forest fires
- 1Institute of Chemistry, University of Campinas (UNICAMP), Campinas, São Paulo, Brazil
- 2Université de Pau et des Pays de l’Adour, E2S UPPA, CNRS, Institut des Sciences Analytiques et de Physico-chimie pour l’Environnement et les Matériaux (IPREM), Pau, France
Methylmercury in soils and vegetation from the Amazonian rainforest is still poorly studied. Hence, the aim of this work was to determine the total mercury [THg] and methylmercury [MeHg] concentrations in litter and surface soil, MeHg deposition via litterfall, and the MeHg fate during a forest fire in the Amazonian rainforest. Sampling of litter, soil (0–1, 1–2, and 2–5 cm), and ash was carried out before (BB) and after (AB) a prescribed forest fire. The results showed a low [THg] (34 ± 8 ng g-1) and [MeHg] (0.16 ± 0.03 ng g-1) value in litter, with a correspondingly low estimate of the MeHg litterfall flux (0.13 ± 0.03 μgm-2 yr-1) which has been probably underestimated due to potential losses during the field experiment. In ashes, [THg] and [MeHg] values were 23 ± 8 ng g-1 and 0.11 ± 0.04 ng g-1, respectively. Although a significant part of Hg contained in the biomass was lost during the fire, the [MeHg]/[THg] ratio was not affected by it and was the same in litterfall and ash (∼0.5%). In soil, the average [THg] BB value was 149 ± 12 ng g-1 in the three layers and [MeHg] was between 0.8 and 1.0 ng g-1. The forest fire only affected the superficial soil, with a significant decrease of [THg] and [MeHg] in the 0-1 cm layer. Similarly, the decrease of the [MeHg]/[THg] ratio in the surface soil layer suggested that MeHg was more readily emitted or degraded than the other Hg species present.
1 Introduction
Forests have been recognized as key ecosystems in the global biogeochemical Hg cycle (Wang et al., 2016; Outridge et al., 2018; Bishop et al., 2020; Zhou et al., 2021). The forest canopy is highly efficient in trapping atmospheric Hg through the stomatal uptake of gaseous elemental mercury (Hg0) and by the adsorption of particulate-bounded mercury (PBM) and reactive gaseous mercury onto leaf surfaces (Jiskra et al., 2018; Zhou et al., 2021). Methylmercury (MeHg), one of the most toxic and bioaccumulating Hg species, is also expected in tree leaves, mainly originating from MeHg atmospheric depositions (Graydon et al., 2008; Tsui et al., 2012; Ma et al., 2015; Gerson et al., 2022). As the root absorption of Hg is very low, in noncontaminated sites, a significant contribution in this manner is not expected (Graydon et al., 2009; Tabatchnick et al., 2012). Mercury fixed in the foliage is then transferred to the forest floor via litterfall and throughfall (rain that washes plant surfaces off). Some studies showed the total mercury (THg) and MeHg fluxes in throughfall and litterfall are many times higher than those in direct wet deposition in open areas (Branfireun et al., 1998; Louis et al., 2001; Mowat et al., 2011; Gerson et al., 2022). Mercury is then sequestered in soil generally associated with organic matter (Bishop et al., 2020). However, in ferralitic soils (Oxisols), which are largely present in the Amazonian region, Hg mainly adsorbs onto Fe-oxy-hydroxides, while the MeHg concentration seems to be more dependent on the organic matter quality and quantity (Roulet and Lucotte, 1995; Roulet et al., 2001).
Global mercury deposition through litterfall has been estimated to be between 1,000 and 1,200 Mg yr-1 (Wang et al., 2016; Obrist et al., 2018) with 30% of the total deposition in temperate/boreal forests and 70% in tropical/subtropical forests. This accounts for approximately one-third of the total Hg deposition on land (Mason et al., 2012), also equivalent to 50%–60% of global anthropogenic emissions (Pirrone et al., 2010). A higher efficiency of tropical/subtropical forests in transferring atmospheric Hg via litterfall is mainly attributed to the higher litterfall biomass production (Wang et al., 2016; Schneider et al., 2023). Biological factors such as the presence of evergreen broadleaf species with long leaf lifespans and strong foliage assimilation also result in higher Hg concentrations in the foliage and litterfall (Fostier et al., 2015; Wang et al., 2016; Teixeira et al., 2017; Pleijel et al., 2021; Gerson et al., 2022). As for other Hg species, [MeHg] in tree leaves and litter also largely depend on the forest type (Witt et al., 2009). As tropical forests are commonly rich in perennial broadleaved species with high litter production, relatively high atmospheric MeHg inputs are expected in these environments (Zhou and Obrist, 2021). However, to our knowledge, [MeHg] has not yet been measured in tree leaves from tropical forests, and only one dataset of [MeHg] in litter samples from the Amazonian rainforest is available (Roulet et al., 2001).
Forests can also provide favorable environments for Hg methylation processes (Eklöf et al., 2018), enhancing the potential risk to the terrestrial biota (Rieder et al., 2011; Ackerman et al., 2016). Lowland forests are frequently pointed out as hotspots for Hg methylation due to soil saturation and anoxic conditions that favor the methylation process (Bishop et al., 2020). In the Amazonian region, some studies showed that seasonal flooding of the forest results in high [MeHg] in litter and organic horizons of forest soils (Guimaraes et al., 2000; Roulet et al., 2001; Guedron et al., 2011). Mercury methylation can also take place in organic surface soils in uplands (Bishop et al., 2020); however, this process was poorly studied, especially in forested tropical uplands (Shanley et al., 2020).
Deforestation and some forest management practices can enhance the mobilization of Hg stocked in soils through leaching and runoff processes, therefore increasing Hg transport toward the streams (Fostier et al., 2000; Roulet et al., 2001; Patry et al., 2013; Bishop et al., 2020), where the methylation process results in the production of MeHg (Bishop et al., 2020). By studying the long-term impact of forest fires on Hg concentrations at Acadia National Park (United States), Patel et al. (2019) suggested that fire enhanced MeHg production in superficial soil. However, the sources and fate of MeHg in forests are still poorly known, especially in tropical areas (Schneider et al., 2023). As far as we know, the effects of fire on MeHg volatilization and degradation have never been studied, albeit biomass burning was suggested as a possible source of atmospheric MeHg (Zhang et al., 2019).
The Amazonian rainforest represents over half of the planet’s remaining rainforest. The vegetation in the region is mainly semi-deciduous or evergreen tropical forests, but the species composition varies widely due to the differences in the soil type and altitude across the basin (Sombroek, 2000). From the different vegetation types, it was estimated that approximately 66% of soils are covered by the so-called old-growth terra firme forests (Saatchi et al., 2007; Chave et al., 2010), which are part of the upland (never flooded) forests. For this biome, the total Hg deposition via litterfall was estimated to be 268 ± 77 Mg y-1 (Fostier et al., 2015), whereas the total Hg input from the atmosphere was estimated to be 332 Mg y-1 (Feinberg et al., 2023). Conversely, in the Brazilian Amazon, which accounts for the main part of this tropical forest, approximately 8 × 103 km2 were deforested yearly over the 2008–2022 period, which was mainly performed by clearing and biomass burning (INPE, 2023). Although a growing body of evidence has shown the importance of tropical forests in the global Hg cycle, Hg in soils and vegetation from the Amazonian rainforest has still been poorly studied and very few data are available for MeHg in these regions. Therefore, the aim of this work was two-fold: 1) to determine THg and MeHg concentrations in litter and soil in an upland forest located in the Brazilian Amazon basin and 2) to calculate the fluxes of MeHg via litterfall, as well as the impact of prescribed forest burning on the MeHg fate at the interface soil/ash/atmosphere in the same region.
2 Materials and methods
2.1 Experimental area and sampling
This study was performed in the western Brazilian Amazon region within a 150 m × 150 m native forest area (8°38’S 63°11′W) located approximately 20 km from Candeias do Jamari city (Rondônia state, Brazil) (Figure 1). The vegetation type present in the experimental area is old-growth terra firme forests (Chave et al., 2010) and the soil is classified as Oxisols (Almeida et al., 2005). The prescribed fire experiment was conducted according to the slash-and-burn practice commonly applied for deforestation in this region (Fire experiment authorization 204/2014 Secretaria de Estado do Desenvolvimento Ambiental). Details on the complete experiment can be found in the work of Carvalho et al. (2016). In brief, the first step was to perform a forest inventory of the trees with the diameter at breast height (DBH) being >10 cm in the central 1 ha (Supplementary Figure S1). The forest was then cleared at the beginning of the dry season (July), and burning was performed at the end of August when the vegetation was sufficiently dry. Although the total area (2.5 ha) was burned, the combustion completeness (CC) of the fine material (branches with diameter <10 cm, litter, and leaves) was estimated in 12 different 4 m2 subareas distributed in the 1 ha central area, as shown in Supplementary Figure S1. In each area, the biomass was weighted before (BB) and after the burning (AB) (both in August) and CC was calculated as the percentage of the burned biomass, as detailed in Supplementary Table S1. Details on fuel loading and ash mass in each subarea are presented in Supplementary Table S1. The consumption of logs (DBH >10 cm) and larger branches (D >10 cm) in the 1 ha central area was also estimated as detailed in the work of Carvalho et al. (2016). Litter, soil (0–1, 1–2, and 2–5 cm), and ash sampling was carried out in nine of these subareas (Supplementary Figure S1). In each area, each sample was composed of three subsamples that were mixed and homogenized. Soil was collected using an acid-washed plastic shovel and litter was collected with hands using gloves. The samples were stored in doubled plastic bags for transport to the laboratory, dried at room temperature in a laminar flow hood, milled in a mortar (with the aid of liquid N2 for litter), and stocked in decontaminated HDPE flasks.
2.2 Analyses and quality control
2.2.1 Total mercury
The determination of the total mercury concentration [THg] was carried out through thermodesorption atomic absorption spectrometry (DMA-80 Tri-Cell, Milestone), according to the work of Melendez-Perez and Fostier (2013). Accuracy, expressed as the average percentage of recovery (±1 standard deviation, s) of the THg certified concentration, was assessed by analyzing standard reference materials (SRMs). It was 113% ± 8% for NIST-2689 (coal fly ash), 113% ± 5% for NIST-1632d (trace elements in coal), 99% ± 5% for IAEA-433 (trace elements in marine sediments), and 93% ± 2% for IAEA-336 (trace and minor elements in lichens). Precision was calculated as the relative standard deviation (1 s) of three analytical replicates and was always <10%. The limit of detection (LOD) and of quantification (LOQ), calculated based on the angular coefficient of analytical curves, were 1.7 ± 0.1 and 5.6 ± 0.5 ng g-1, respectively, for litter analyses, 1.3 ± 0.1 ng g-1 and 4.5 ± 0.4 ng g-1 for ash, and 0.75 ± 0.06 and 2.5 ± 0.2 ng g-1 for soil.
2.2.2 Methylmercury
A precise and accurate method based on species-specific isotope dilution and gas chromatography coupled to inductively coupled plasma mass spectrometry (GC-ICPMS), which allows the simultaneous determination of the methylmercury concentration [MeHg] and inorganic Hg [iHg], was used for MeHg quantification in the different matrices studied in this work (Rodriguez Martin Doimeadios et al., 2003; Monperrus et al., 2008; Clemens et al., 2011). This analytical methodology has already been assessed for soil and vegetal samples (Feng et al., 2016) and more details on the protocol are briefly given in Supplementary Text S1 and in more detail in the previous works (Monperrus et al., 2008; Clemens et al., 2011). For MeHg, the analytical method recovery was assessed by analyzing the SRM IAEA-405 (trace elements and MeHg in marine sediments) and calculating the ratio (%) between the obtained and certified [MeHg]. Total Hg species (THgsp) recovery was also calculated for IAEA-405, IAEA-336, and litter, ash, and soil samples as the ratio between [MeHg] + [iHg] and [THg]. The precision was calculated as the relative standard deviation of three analytical replicates. The limit of detection and quantification were calculated based on the parameters of the analytical curves. For MeHg, LOD and LOQ were 0.006 and 0.020 ng g-1, respectively. Recovery for IAEA-405 was 100.4%, and the precision was <5%; detailed data are given in Supplementary Table S2.
For iHg, LOD was 0.07 ng g-1 and LOQ was 0.23 ng g-1. For THgsp, it was 95.4% and 89.1% for IAEA-405 and IAEA-336, respectively, and the precision was <5%. For litter and ash samples, THgsp recovery ([MeHg] + [iHg]) × 100/[THg]) was 73% ± 8% and 50% ± 12%, respectively (Supplementary Tables S3, S4). For soil, it ranged from 46% ± 7% to 52% ± 7% (Supplementary Table S5).
2.2.3 Determination of the organic matter content
The organic matter content was determined in litter, ash, and soil samples via gravimetric analysis. The previously dried samples were burned for 2 h at 360°C in a muffle furnace; then, the percentage lost on ignition was used to estimate the organic matter content (North Central Regional Research, 2012).
2.3 Statistical data treatment
Data processing was conducted using PAST (Paleontological Statistics, version 4.11) (Hammer et al., 2001), GraphPad Prism version 10.0.0 (Boston, MA, United States), and XLSTAT® software. The normality of the datasets was assessed using the Shapiro–Wilk test. To evaluate the statistical differences between normally distributed datasets, Student’s t-test was employed. Furthermore, one-way ANOVA was used to assess the differences among more than three sets of normally distributed data. All statistical analyses were carried out with a significance level of 95% (α = 0.05). Pearson’s correlation coefficient (r) was also analyzed to assess the degree of dependence between pairs of variables (i.e., combustion completeness (%), total biomass (kg m-2), OM lost from vegetation (%), THg lost from vegetation (%), MeHg lost from vegetation (%), OM lost from 0–1 cm soil (%), THg lost from 0–1 cm soil, and MeHg lost from 0–1 cm soil).
3 Results
3.1 Total [THg] and methylmercury concentrations
All the results presented a normal distribution, except the dataset of [MeHg] in the 0–1 cm soil layer AB, in which the CJ12 [MeHg] (1.18 ng g-1) appeared as an outlier (Grubbs’ test for outliers; p <0.05) (Figure 2). However, the rest of the data were normally distributed after removing this value. Paired Student’s t-test was then applied to compare the different datasets (i.e., litter and ash, soil BB or AB at different depths, and soil BB and AB at the same depth).
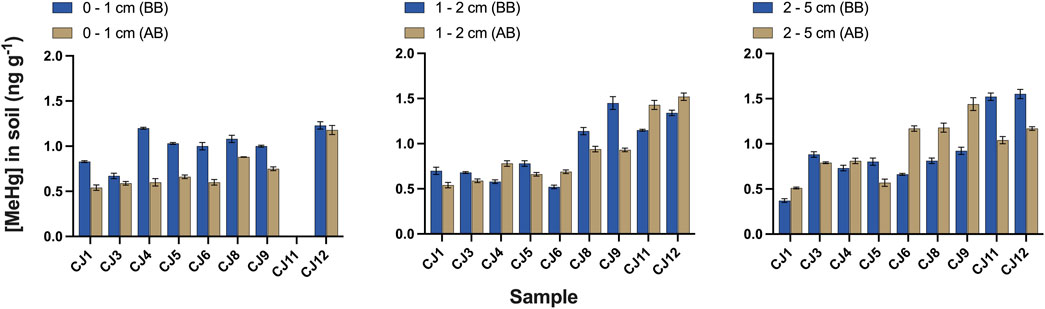
FIGURE 2. Methylmercury concentration (ng g-1) in soil samples from the experimental subareas before and after burning in the three sampled layers (0–1, 1–2, and 2–5 cm). The CJ11 0–1 cm layer was not analyzed.
[THg] in litter ranged from 25.0 to 50.0 ng g-1 (Table 1) and was significantly higher than that in ash (paired Student’s t-test, p <0.05). In soil, the overall average [THg] was 149 ± 12 ng g-1 (Table 2), and there was no difference among layers. After burning, a significant decrease was only observed in the 0–1 cm layer (p <0.05).
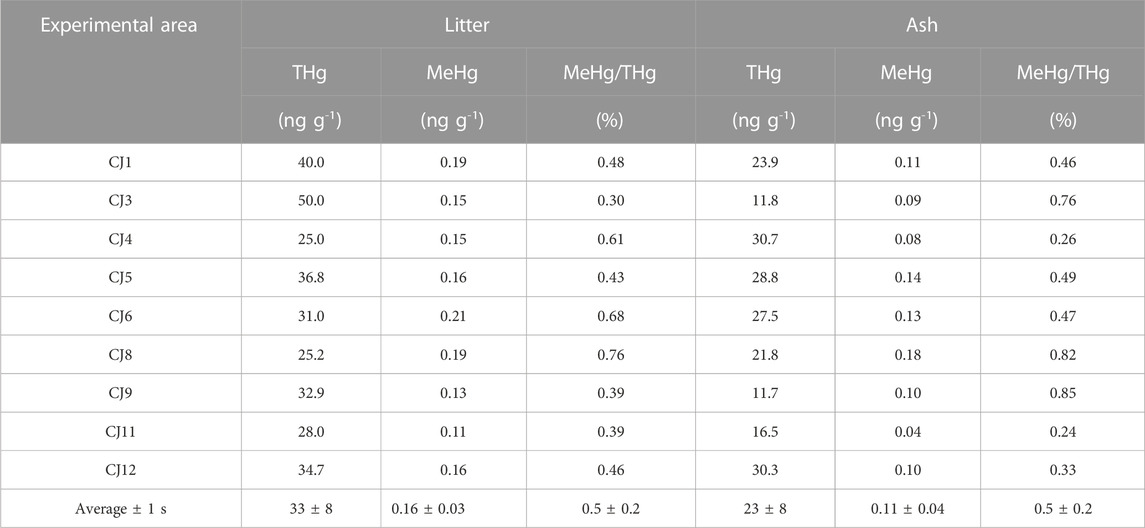
TABLE 1. Concentrations of total mercury [THg] and methylmercury [MeHg] (ng g-1) in litter and ash, with their respective [MeHg]/[THg] ratios expressed in %.
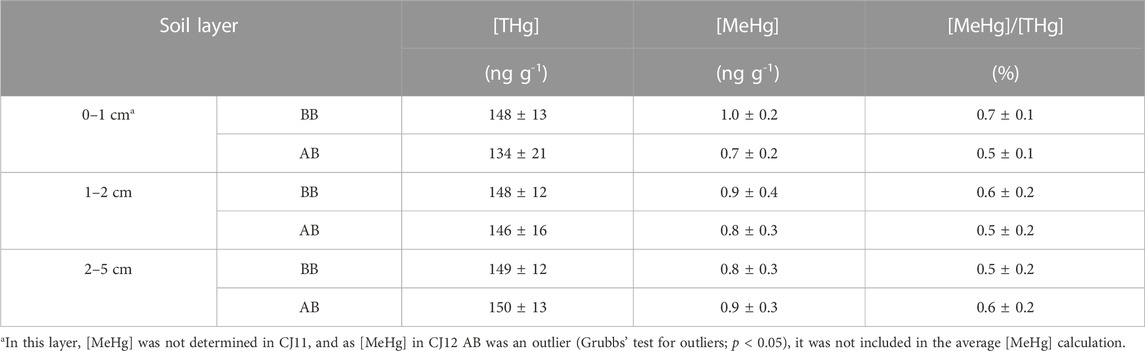
TABLE 2. Average concentrations of THg, MeHg (ng g-1), and MeHg/THg (%) in the 0–1, 1–2, and 2–5 cm soil layers before and after burning.
The average [MeHg] was 0.16 ± 0.03 ng g-1 in litter, ranging from 0.11 to 0.21 ng g-1 (Table 1). Following the same trend observed for [THg], it was significantly higher than the [MeHg] in ash (p <0.05), where the average was 0.11 ± 0.04 ng g-1, ranging from 0.04 to 0.18 ng g-1. Before burning, in the superficial soil layer (0–1 cm), the average [MeHg] was 1.0 ± 0.2 ng g-1 (Table 2), ranging from 0.67 to 1.23 ng g-1 (Figure 2), which was not significantly different from [MeHg] in the other two layers BB. After burning, a significant decrease was only observed in the 0–1 cm layer (paired Student’s t-test; p <0.05), in which the average [MeHg] value was 0.7 ± 0.1 ng g-1, ranging from 0.54 to 0.88 ng g-1, albeit it should be noted that in this layer, MeHg was not determined in the CJ11 sample. Furthermore, as CJ12 [MeHg] AB (1.18 ng g-1) appeared as an outlier, it was not included in the average calculation.
The average [MeHg]/[THg] ratio in litter (0.5 ± 0.2 ng g-1) (Table 1) was the same as in ash (0.5 ± 0.2 ng g-1). In the 0–1 cm soil layer, the average ratio was 0.7 ± 0.1 ng g-1 before (0.50–0.74 ng g-1) and 0.5 ± 0.1 ng g-1, ranging from 0.36 to 0.65 ng g-1 after burning. In the other soil layers, the average ratio varied between 0.5 ± 0.2 ng g-1 and 0.6 ± 0.2 ng g-1 BB and AB.
3.2 Organic matter content in litter, ash, and soil samples
The average OM content in litter was 91% ± 3% (w w−1), which significantly decreased to 29% ± 11% in ash (paired Student’s t-test, p <0.05) (Figure 3). In soil, before burning, it was 21% ± 5%, 10% ± 2%, and 7% ± 2% in the 0–1, 1–2, and 2–5 cm layers, respectively. After burning, it was 14% ± 4%, 8% ± 1%, and 7% ± 2% in the 0–1, 1–2, and 2–5 cm layers, respectively.
4 Discussion
4.1 Speciation method recovery
The extraction method used for Hg speciation presented high THgsp recovery for both CRM IAEA-405 (sediment) and IAEA-336 (lichens). However, THgsp recovery was lower in litter, soil, and ash samples (Supplementary Table S5). For Hg as for other heavy metals, the liquid/solid extraction efficiency depends on the reagent type and its ability to solubilize the different forms (i.e., more or less labile), in which the metal is present in the sample (Song et al., 2015; Ku et al., 2018; Zhou et al., 2018). Conversely, a complete release of Hg is expected with the thermodesorption method being used (Zhou et al., 2018).
A sequential extraction applied to fresh and dried leaves aiming at Zn, Cd, and Cu determination showed that for most extractants, the extraction efficiency was higher in fresh leaves than that in dried leaves, which could be related to the storage of the metals in vacuoles (Song et al., 2015). The authors also pointed out that the finer particle size of the dried samples may have increased the extraction efficiency. As our litter samples were ground manually, this latter point could explain the relatively low extraction efficiency (73% ± 8%) when compared to IAEA-336 (93 ± 2%). However, plant metal fractions have still been poorly studied (Zhou et al., 2018), and it is probable that many factors, such as plant species and the age of tissues, can have an impact on the metal lability. For soil and ash samples, recovery was approximately 50%, showing that only Hg species soluble in oxidizing and moderately acid media (HNO3 6 mol L-1) were extracted. This method is expected to solubilize Hg bound to the organic matter and Hg adsorbed by amorphous iron oxides and by clay in soil (Reis et al., 2016), but not the Hg present in the recalcitrant pools, which includes HgSe and HgS (Ku et al., 2018). Conversely, this method is not expected to digest charcoal or recalcitrant black carbon originating from biomass burning (Ku et al., 2018), and these authors reported that a recalcitrant Hg pool significantly increased in wildfire ash when compared with the unburned vegetation (litter and wood). In our study, a recalcitrant pool was calculated as 100 – [THsp] recovery (%) (Supplementary Table S5), which increased from 27% in litter to 50% in ash. So, our results suggest that a large part of Hg was likely present in the recalcitrant pool in the soil samples and included in charcoal and ash.
4.2 Total and methylmercury concentrations in litter
The average total Hg concentration in litter (33 ± 8 ng g-1) was lower than that reviewed by Fostier et al. (2015) for the Amazonian upland forests, which ranged from 40 ± 5 to 119 ± 29 ng g-1 (average = 75 ± 30 ng g-1 and median = 61 ng g-1). As the tree species composition has a strong influence on Hg uptake by the foliage and because tree species are not homogeneously distributed in the forest, variations in the Hg concentration can result from the variations in litter composition. The relative high coefficient of variation (CV %) generally observed for any set of data on litter [THg] can largely be attributed to this factor. In our study, CV was 24%, which was lower than the data reported by Fostier et al. (2015) in the Amazonian forest. Conversely, previous results on [THg] in litter were obtained near the experimental site but under forested areas (Fostier et al., 2015), which showed that [THg] was higher in the forest area (61 ± 11 ng g-1) than that in our experimental conditions (33 ± 8 ng g-1). This difference could, therefore, be due to the exposure to solar radiation and rain washout for some months after the forest was cut, resulting in Hg loss. Richter et al. (2023) reached the same conclusion when comparing the isotopic composition of litter sampled in the same experimental site at CJ and in a forest site. Furthermore, Carpi et al. (2014) showed significant increases in Hg emissions when soils (covered or not by litter) were exposed to solar radiation after cutting the tree and suggested that solar UV radiation was the main driver responsible for these emissions.
As for [THg], the average [MeHg] in litter (0.16 ± 0.03 ng g-1) was also in the low range of those reviewed in other remote regions (Zhou et al., 2018), e.g., from 0.18 ng g-1 in the coniferous forest of Canada (Louis et al., 2001) to 0.84 ng g-1 in a subtropical altitude forest in China (Ma et al., 2015). In addition, Graydon et al. (2008) reported [MeHg] ranging from 0.22 ± 0.02 to 0.49 ± 0.14 ng g-1 in litter from jack pine and deciduous forests, respectively, in a remote boreal Canadian region. However, the average [MeHg]/[THg] ratio (%) in litter (0.5% ± 0.1%) was in the same range as for other remote regions, i.e., between 0.25% and 1.0% (Graydon et al., 2008; Zhou et al., 2018). This shows that Hg losses due to direct atmospheric exposure (solar radiation and rain washout) probably happen at the same rate for THg and MeHg. In Amazonian flooded forests (i.e., hydromorphic soils), a much higher [MeHg]/[THg] ratio in litter (2%–5%) has been reported by Roulet et al. (2001), suggesting that this environment is very favorable for Hg methylation and/or MeHg accumulation.
4.3 Total and methylmercury litterfall deposition
The total mercury annual deposition via litterfall (DTHg) can be estimated as DTHg = [THglit] × Mlit, where [THglit] is the average [THg] in litter and Mlit is the annual mass of litter deposited on the forest floor, which was estimated to be 8 ± 2 Mg ha-1 yr-1 by Fostier et al. (2015) for the Brazilian Amazonian rainforest. Then, the estimated DTHg value was 28 ± 14 μg m-2 yr-1. This value can be considered low compared to 49 ± 14 μg m-2 yr-1 estimated by Fostier et al. (2015) and to 84 ± 48 μg m-2 yr-1 by Wang et al. (2016) for different locations in the Amazonian rainforest. The MeHg litterfall deposition (DMeHg), calculated the same way, but using the average [MeHg] in litter, resulted in a net deposition of 0.13 ± 0.04 μg m-2 yr-1. This value is within the range of the previous estimations from 0.08 to 0.34 μg m-2 yr-1 reported by Zhou et al. (2018) for MeHg litterfall deposition in other remote regions. Nevertheless, as already pointed out, the litter exposed, for several months, to sun radiation and rain washout may have resulted in some loss of Hg and, consequently, to an underestimation of the initial net THg and MeHg depositions. Conversely, an estimate of Hg deposition based on a limited dataset obtained in the specific condition (after the vegetation was felled and dried) may be not representative of the Amazonian upland forest; however, it is paramount to highlight that this is the first dataset obtained on [MeHg] in litterfall in this ecosystem.
4.4 Total and methylmercury concentrations in soil before burning
The total Hg concentrations in soil before burning (149 ± 12 ng g-1) are within the values expected in Amazonian soils, which are highly variable, i.e., 61 ± 25 to 304 ± 62 ng g-1 for superficial (0–10 cm) pristine forest soils (Schneider et al., 2023). MeHg (∼1 ng g-1) and [MeHg]/[THg] (0.6%) concentrations obtained in the present study are in accordance with those reported for Oxisols from other locations of the Amazonian forest (Table 3), which are dominant in Candeias do Jamari (Roulet and Lucotte, 1995; Almeida et al., 2005). In contrast, higher [MeHg]/[THg] ratios were reported in hydromorphic soils, but the processes governing mercury methylation and/or accumulation in those soils are still unknown (Roulet et al., 2001; Guedron et al., 2011). The data presented in this work also agree with MeHg concentrations in soils from uncontaminated temperate and boreal forests, in which MeHg concentrations are generally below 1 ng g-1, although some values up to 10 ng g-1 have been reported under specific conditions, such as around fumarolic areas (Kodamatani et al., 2018), in a boreal coniferous clear-cut forest (Kronberg et al., 2016), and wetland forests (Bishop et al., 2020).
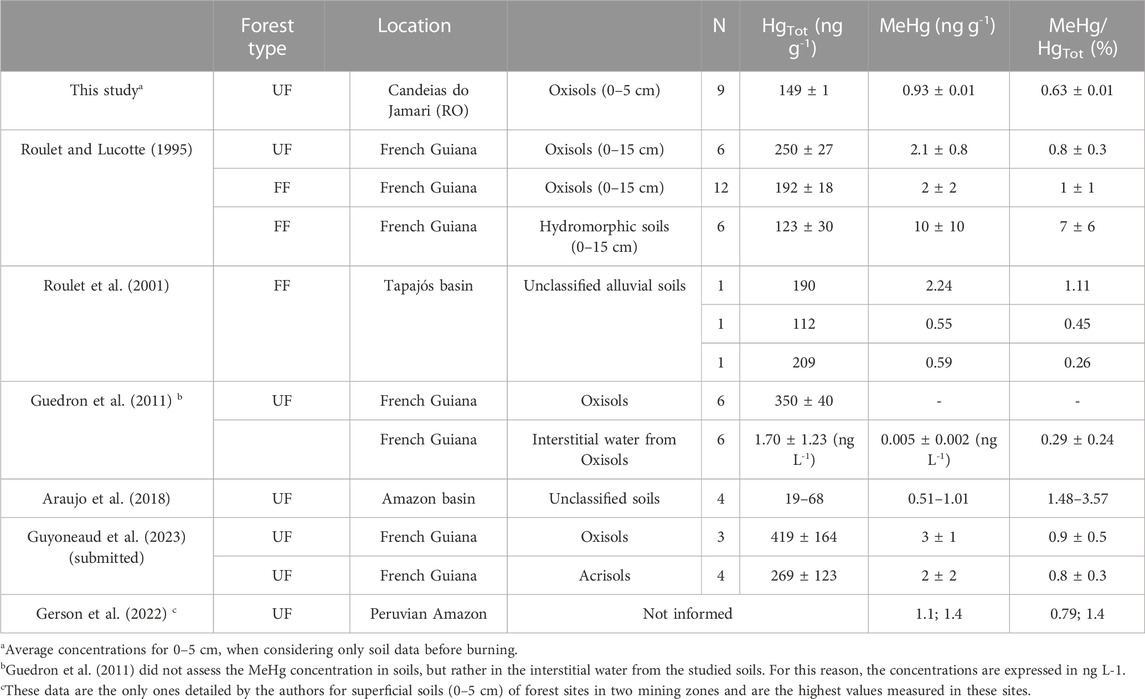
TABLE 3. Total (HgTot) and methylmercury (MeHg) concentrations (average ± 1 SD) and the percentage of MHg/HgTot in soil reported in the literature and from the present study for Amazonian flooding forests and upland forests.
As expected, [THg] and [MeHg] concentrations were significantly higher in soil than those in litter (paired Student’s t-test; p <0.05) because the litter mass decreases during its decomposition and is then incorporated into the superficial soil (Zhou et al., 2021). However, the dynamics of Hg in soil strongly depend on the soil composition. The decomposition of the litter results in the formation of organic horizons (Oh), and Hg originating from this litter may bind to the organic matter, accumulating in superficial soil. Nevertheless, in Oxisols, Hg can mainly be adsorbed onto Fe-oxy-hydroxides (Roulet and Lucotte, 1995).
Methylmercury in litterfall can originate from MeHg atmospheric depositions on tree leaves (Graydon et al., 2008; Tsui et al., 2012; Ma et al., 2015), but this process has never been evaluated in the Amazonian forest. In addition to the atmospheric input, MeHg in soil can also increase due to the methylation process, mainly driven by anaerobic bacteria (Kronberg et al., 2016; Gerson et al., 2017; Eklöf et al., 2018). High [MeHg] and [THg] were observed in dried superficial soils in an Amazonian forest area, where a high atmospheric Hg input was attributed to the proximity to artisanal small gold mining (Gerson et al., 2022). However, as [MeHg]% (0.79%) was in the same order as in remote areas around the globe, the authors concluded that the methylation rate did not increase in the study area and that high [MeHg] and [THg] can mainly be attributed to a high Hg input, litter decomposition, and high storage capacity of Hg in soil. However, the examination of the sources of MeHg in forest soils via either atmospheric depositions or in situ methylation pathways and the importance of each source still remain to be clearly assessed to better understand the Hg cycle in terrestrial environments (Tsui et al., 2012; Tao et al., 2018; Zhou et al., 2018). This aspect is a critical gap in the knowledge of tropical soils and forests, for which the available studies and data are very scarce (Gerson et al., 2022).
4.5 Combustion completeness and fire severity
In forest fires, the combustion process depends on several factors, such as initial mass, nature, and moisture of fuel; air temperature and humidity; wind speed; and the topography of the site (Certini, 2005). The impact of fire on the physical, chemical, and biological properties of the soil (the fire severity) depends on the heat release from the combusting biomass (the fire intensity) and on the duration of combustion (Neary et al., 2005). A low-to-moderate severity is expected in the prescribed fire experiments (Certini, 2005). In our experimental site, the fine material (D <10 cm) accounted for 13.3% of the total fresh biomass estimated to 701.5 t ha-1, which was in the upper range of the average forest biomass in western Amazonia (612.4 ± 142.5 t ha-1) (Carvalho et al., 2016). In the nine 4-m2 areas considered in the present study, the CC of the fine material ranged from 66.6% to 100%, showing the heterogeneity of the combustion process. Consumption completeness showed no correlation (p >0.05) with any of the other variables (Supplementary Table S6). In two other prescribed fire experiments conducted in the same way in western Amazonia, Carvalho et al. (2016) reported the CC of small size materials of 85.5% and 89.2%. Conversely, when also considering logs (DBH >10 cm) and larger branches (D >10 cm), the overall CC was only 16.0% (Carvalho et al. (2016). The typical superficial soil temperature in forest fires rises 200–300°C (Rundel, 1983), although the temperature up to 500–700°C can be reached in case of heavy fuels (Certini, 2005). One of the first effects of forest fires on soil properties is the removal of OM, whose substantial consumption begins at 250–300°C and completes at approximately 460°C (Giovannini et al., 1988). The organic matter release can, therefore, be used as an indicator of fire severity. In our experiment, the OM content significantly decreased only in the 0–1 cm layer (paired Student’s t-test; p <0.05). However, the average decrease of the OM content in this layer was only 33% ± 25%, indicating a moderate fire in the sampled subareas. It also suggests that, at these places, relatively low temperatures were reached, as complete combustion of soil only occurs at temperatures >450–500°C (Gonzalez-Perez et al., 2004; Mataix-Solera et al., 2011).
4.6 Total and methylmercury release due to forest burning
In soil, a significant decrease of [THg] and [MeHg] was only observed in the 0-1 cm layer, indicating that the fire intensity was only able to promote Hg release from the very superficial soil, which is in accordance with the observed OM decrease in the same layer. However, no correlation was observed between the OM lost and [THg] and [MeHg] lost in this soil layer (Supplementary Table S6). The impacts of the prescribed forest fires on the very superficial soil in the Amazonian rainforest were also reported by Melendez-Perez et al. (2014) and Michelazzo et al. (2010). A significant decrease (paired Student’s t-test; p <0.05) of the [MeHg]/[THg] ratio was observed in the 0–1 cm layer after burning (Table 2), suggesting that with the increase in temperature, MeHg was more easily degraded and/or emitted than other Hg species (i.e., iHg compounds) present in such soils. The impact of fire on soil OM (and others soil components) is a complex issue, as it depends on many parameters, such as soil properties and composition, the temperature reached during the fire, and the burning duration. It was reported that the distillation of volatiles and the loss of organic carbon in soils start at temperatures between 100 and 200°C and that the charring process starts above 190–200°C (Gonzalez-Perez et al., 2004). So, the effects of forest fire on MeHg release and degradation can also vary with fire severity. Mercury speciation by thermodesorption shows that different species are released at different temperature ranges (Reis et al., 2016). Applying this technique to Amazonian soil spiked with Hg2+, Do Valle et al. (2005) showed that Hg bound to the mineral phases was released at a lower temperature than Hg bound to humic acids. Therefore, our results suggest that MeHg was mainly bound to weak organic matter ligands and/or mineral phases. It is important to notice that after burning, the 0-1 cm layer visually corresponded to the partially burned O-horizon, while newly formed ashes were mainly produced from the burned overlaying biomass. Both [THg] and [MeHg] were significantly lower in ash than in litter (30% and 31% decrease for [THg] and [MeHg], respectively), clearly showing that the fire conditions in our experimental site were able to remobilize a significant part of Hg sequestered in the vegetation, with likely a larger fraction of it emitted into the atmosphere (Outridge et al., 2018). Furthermore, as [MeHg]/[THg] ratios were not different in litter and ash, one can conclude that MeHg was released with a similar efficiency as the main fraction of the inorganic Hg species. Biomass burning was pointed out as a potential source of MeHg in atmospheric fine particles (Zhang et al., 2019). However, the release of MeHg from soil and litter does not imply that it was directly emitted into the atmosphere. First, MeHg can be readsorbed into ash particles that have a high adsorption capacity for organic and inorganic Hg compounds (Ku et al., 2018). Furthermore, MeHg under heating conditions can be transformed into gaseous and volatile Hg forms (i.e., mainly Hg0). However, to our knowledge, the effects of the high temperature generated by biomass burning on MeHg present in the vegetation have not yet been studied in detail. Recently, a study performed on the same litter, ash, and soil samples (Richter et al., 2023) using the Hg stable isotopic composition suggested that Hg emission and reallocation during forest fire is quite complex and demonstrated that the Hg present in newly produced ash was mainly originating from Hg emitted from the superficial soil layer (0-1 cm).
5 Conclusion
Total and MeHg concentrations in litter from the study area were relatively low compared to other Amazonian rainforest areas. Consequently, the estimated MeHg litterfall flux was also low, possibly due to the extended exposure time of litter to solar radiation and rain washout after forest cutting. Conversely, the [MeHg]-to-[THg] ratio in soil and litter was of the same order as that in many remote forests from upland temperate and boreal forests. For the first time, it was shown that the release of MeHg occurs with a similar efficiency as the primary fraction of inorganic Hg species from litter. Although the forest fire promoted a significant decrease in [THg] and [MeHg] in the very superficial soil layer (0-1 cm), in such organic topsoil MeHg was more readily emitted or degraded compared to the other Hg species. The complexity of understanding the MeHg dynamics and fate in fire-impacted soils was highlighted, especially considering the strong modifications that fire can induce in soil OM, as MeHg in soils is primarily linked to OM. This study sheds new light on the behavior of MeHg in the fire-affected areas of the Amazonian rainforest and emphasizes the need for further research to fully comprehend the intricate interplay between fire, soil, and Hg dynamics.
Data availability statement
The original contributions presented in the study are included in the article/Supplementary Material; further inquiries can be directed to the corresponding author.
Author contributions
AF: conceptualization, project administration, writing—original draft, writing—review and editing, and supervision. DA: conceptualization, project administration, writing—original draft, writing—review and editing, and supervision. LR: methodology, investigation, and formal analysis. ET: methodology and investigation. JV: statistical analysis, writing—review and editing. All authors contributed to the article and approved the submitted version.
Funding
This work was funded by the São Paulo Research Foundation (FAPESP) (projects 2008/04490-4, 2010/19040-4) and also partially supported by The University of Campinas (UNICAMP/FAEPEX 2932/23), the CNRS, French ANR, and New Aquitaine Region council. LR received a fellowship from the Brazilian Coordination for the Improvement of Higher Education Personnel, CAPES (financing code 001). JV received a fellowship from FAPESP (2019/18124-4). The burning in Candeias do Jamari was conducted under permission 204/2014 from the Secretaria de Estado do Desenvolvimento Ambiental de Rondônia (SEDAM) and under permission from CNPq.
Acknowledgments
The authors also thank their coworkers from the Brazilian Institute of Environment and Renewable Natural Resources (IBAMA) and the Brazilian National Institute of Spatial Research (INPE). Jim Hesson edited the manuscript (https://www.academicenglishsolutions.com/editing-service).
Conflict of interest
The authors declare that the research was conducted in the absence of any commercial or financial relationships that could be construed as a potential conflict of interest.
Publisher’s note
All claims expressed in this article are solely those of the authors and do not necessarily represent those of their affiliated organizations, or those of the publisher, the editors, and the reviewers. Any product that may be evaluated in this article, or claim that may be made by its manufacturer, is not guaranteed or endorsed by the publisher.
Supplementary material
The Supplementary Material for this article can be found online at: https://www.frontiersin.org/articles/10.3389/fenvc.2023.1242915/full#supplementary-material
References
Ackerman, J. T., Eagles-Smith, C. A., Herzog, M. P., Hartman, C. A., Peterson, S. H., Evers, D. C., et al. (2016). Avian mercury exposure and toxicological risk across western north America: A synthesis. Sci. Total Environ. 568, 749–769. doi:10.1016/j.scitotenv.2016.03.071
Almeida, M. D., Lacerda, L. D., Bastos, W. R., and Herrmann, J. C. (2005). Mercury loss from soils following conversion from forest to pasture in Rondônia, Western Amazon, Brazil. Environ. Pollut. 137 (2), 179–186. doi:10.1016/j.envpol.2005.02.026
Araujo, B. F., Hintelmann, H., Dimock, B., de Lima Sobrinho, R., Bernardes, M. C., de Almeida, M. G., et al. (2018). Mercury speciation and Hg stable isotope ratios in sediments from Amazon floodplain lakes—Brazil. Limnol. Oceanogr. 63 (3), 1134–1145. doi:10.1002/lno.10758
Bishop, K., Shanley, J. B., Riscassi, A., de Wit, H. A., Eklöf, K., Meng, B., et al. (2020). Recent advances in understanding and measurement of mercury in the environment: terrestrial Hg cycling. Sci. Total Environ. 721, 137647. doi:10.1016/j.scitotenv.2020.137647
Branfireun, B. A., Hilbert, D., and Roulet, N. T. (1998). Sinks and sources of methylmercury in a boreal catchment. Biogeochemistry 41, 277–291. doi:10.1023/a:1005964603828
Carpi, A., Fostier, A. H., Orta, O. R., dos Santos, J. C., and Gittings, M. (2014). Gaseous mercury emissions from soil following forest loss and land use changes: field experiments in the and Brazil. Atmos. Environ. 96, 423–429. doi:10.1016/j.atmosenv.2014.08.004
Carvalho, J. A., Amaral, S. S., Costa, M. A. M., Soares Neto, T. G., Veras, C. A. G., Costa, F. S., et al. (2016). CO2 and CO emission rates from three forest fire-controlled experiments in Western Amazonia. Atmos. Environ. 135, 73–83. doi:10.1016/j.atmosenv.2016.03.043
Certini, G. (2005). Effects of fire on properties of forest soils: a review. Oecologia 143, 1–10. doi:10.1007/s00442-004-1788-8
Chave, J., Navarrete, D., Almeida, S., Alvarez, E. ´, Aragão, L. E. O. C., Bonal, D., et al. (2010). Regional and seasonal patterns of litterfall in tropical South America. Biogeosciences 7, 43–55. doi:10.5194/bg-7-43-2010
Clémens, S., Monperrus, M., Donard, O. F. X., Amouroux, D., and Guérin, T. (2011). Mercury speciation analysis in seafood by species-specific isotope dilution: method validation and occurrence data. Anal. Bioanal. Chem. 401 (9), 2699–2711. doi:10.1007/s00216-011-5040-1
Do Valle, C. M., Santana, G. P., Augusti, R., Egreja Filho, F. B., and Windmöller, C. C. (2005). Speciation and quantification of mercury in oxisol, ultisol, and spodosol from Amazon (manaus, Brazil). Chemosphere 58 (6), 779–792. doi:10.1016/j.chemosphere.2004.09.005
Eklöf, K., Bishop, K., Bertilsson, S., Björn, E., Buck, M., Skyllberg, U., et al. (2018). Formation of mercury methylation hotspots as a consequence of forestry operations. Sci. Total Environ. 613, 1069–1078. doi:10.1016/j.scitotenv.2017.09.151
Feinberg, A., Jiskra, M., Borrelli, P., and Biswakarma, J. (2023). Land use change as an anthropogenic driver of mercury pollution. arXiv. doi:10.31223/X5TQ03
Feng, C., Pedrero, Z., Li, P., Du, B., Feng, X., Monperrus, M., et al. (2016). Investigation of Hg uptake and transport between paddy soil and rice seeds combining Hg isotopic composition and speciation. Elementa 2016. doi:10.12952/journal.elementa.000087
Fostier, A.-H., Forti, M. C., Guimaraes, J. R. D., Melfi, A. J., Boulet, R., Santo, C. M. E., et al. (2000). Mercury fluxes in a natural forested amazonian catchment serra do navio, amapa state, Brazil. Sci. Total Environ. 260, 201–211. doi:10.1016/s0048-9697(00)00564-7
Fostier, A. H., Melendez-Perez, J. J., and Richter, L. (2015). Litter mercury deposition in the Amazonian rainforest. Environ. Pollut. 206, 605–610. doi:10.1016/j.envpol.2015.08.010
Gerson, J. R., Driscoll, C. T., Demers, J. D., Sauer, A. K., Blackwell, B. D., Montesdeoca, M. R., et al. (2017). Deposition of mercury in forests across a montane elevation gradient: elevational and seasonal patterns in methylmercury inputs and production. J. Geophys. Res. Biogeosciences 122 (8), 1922–1939. doi:10.1002/2016JG003721
Gerson, J. R., Szponar, N., Zambrano, A. A., Bergquist, B., Broadbent, E., Driscoll, C. T., et al. (2022). Amazon forests capture high levels of atmospheric mercury pollution from artisanal gold mining. Nat. Commun. 13 (1), 559. doi:10.1038/s41467-022-27997-3
Giovannini, G., Lucchesi, S., and Giachetti, M. (1988). Effects of heating on some physical and chemical parameters related to soil aggregation and erodibility. Soil Sci. 146, 255–261. doi:10.1097/00010694-198810000-00006
Gonzalez-Perez, J. A., Gonzalez-Vila, F. J., Almendros, G., and Knicker, H. (2004). The effect of fire on soil organic matter—A review. Environ. Int. 30, 855–870. doi:10.1016/j.envint.2004.02.003
Graydon, J. A., Louis, V. L., Hintelmann, H., Lindberg, S. E., Sandilands, K. A., Rudd, J. W. M., et al. (2008). Long-term wet and dry deposition of total and methyl mercury in the remote boreal ecoregion of Canada. Environ. Sci. Technol. 42 (22), 8345–8351. doi:10.1021/es801056j
Graydon, J. A., Louis, V. L., Hintelmann, H., Lindberg, S. E., Sandilands, K. A., Rudd, J. W. M., et al. (2009). Investigation of uptake and retention of atmospheric Hg(II) by boreal forest plants using stable Hg isotopes. Environ. Sci. Technol. 43 (13), 4960–4966. doi:10.1021/es900357s
Guedron, S., Grimaldi, M., Grimaldi, C., Cossa, D., Tisserand, D., and Charlet, L. (2011). Amazonian former gold mined soils as a source of methylmercury: evidence from a small scale watershed in French guiana. Water Res. 45 (8), 2659–2669. doi:10.1016/j.watres.2011.02.022
Guimaraes, J. R. D., Roulet, M., Lucotte, M., and Mergler, D. (2000). Mercury methylation along a lakeforest transect in the tapajos river floodplain, Brazilian Amazon: seasonal and vertical variations. Sci. Total Environ. 261, 91–98. doi:10.1016/s0048-9697(00)00627-6
Guyoneaud, R., Tessier, E., Colin, Y., Gassie, C., Guédron, S., Monperrus, M., et al. (2023). Microbial communities and environmental factors controlling methylmercury fate in an Amazonian watershed (Crique Combat, French Guyana) in wet and dry season. Soil syst. (in preparation 2023).
Hammer, O., Harper, D. A., and Ryan, P. D. (2001). Past: paleontological statistics software pack-age for education and data analysis. Palaeontol. Electron. 4, 9. doi:10.1016/j.bcp.2008.05.025
INPE (Instituto Nacional de Pesquisas Espaciais) (2023). TerraBrasilis. PRODES (desmatamento). Available at http://terrabrasilis.dpi.inpe.br/app/dashboard/deforestation/biomes/legal_amazon/rates (Accessed May 15, 2023).
Jiskra, M., Sonke, J. E., Obrist, D., Bieser, J., Ebinghaus, R., Myhre, C. L., et al. (2018). A vegetation control on seasonal variations in global atmospheric mercury concentrations. Nat. Geosci. 11 (4), 244–250. doi:10.1038/s41561-018-0078-8
Kodamatani, H., Katsuma, S., Shigetomi, A., Hokazono, T., Imura, R., Kanzaki, R., et al. (2018). Behavior of mercury from the fumarolic activity of Mt. Myoko, Japan: production of methylmercury and ethylmercury in forest soil. Environ. Earth Sci. 77 (13), 478. doi:10.1007/s12665-018-7616-y
Kronberg, R. M., Jiskra, M., Wiederhold, J. G., Björn, E., and Skyllberg, U. (2016). Methyl mercury formation in hillslope soils of boreal forests: the role of forest harvest and anaerobic microbes. Environ. Sci. Technol. 50 (17), 9177–9186. doi:10.1021/acs.est.6b00762
Ku, P., Tsui, M. T. K., Nie, X., Chen, H., Hoang, T. C., Blum, J. D., et al. (2018). Origin, reactivity, and bioavailability of mercury in wildfire ash. Environ. Sci. Technol. 52 (24), 14149–14157. doi:10.1021/acs.est.8b03729
Louis, V. L. S. T., Rudd, J. W. M., Kelly, C. A., Hall, B. D., Rolfhus, K. R., Scott, K. J., et al. (2001). Importance of the forest canopy to fluxes of methyl mercury and total mercury to boreal ecosystems. Environ. Sci. Technol. 35 (15), 3089–3098. doi:10.1021/es001924p
Ma, M., Wang, D., Du, H., Sun, T., Zhao, Z., and Wei, S. (2015). Atmospheric mercury deposition and its contribution of the regional atmospheric transport to mercury pollution at a national forest nature reserve, southwest China. Environ. Sci. Pollut. Res. 22 (24), 20007–20018. doi:10.1007/s11356-015-5152-9
Mason, R. P., Choi, A. L., Fitzgerald, W. F., Hammerschmidt, C. R., Lamborg, C. H., Soerensen, A. L., et al. (2012). Mercury biogeochemical cycling in the ocean and policy implications. Environ. Res. 119, 101–117. doi:10.1016/j.envres.2012.03.013
Mataix-Solera, J., Cerd_a, A., Arcenegui, V., Jord_an, A., and Zavala, L. M. (2011). Fire effects on soil aggregation: a review. Earth-Science Rev. 109, 44–60. doi:10.1016/j.earscirev.2011.08.002
Melendez-Perez, J. J., and Fostier, A. H. (2013). Assessment of direct Mercury Analyzer® to quantify mercury in soils and leaf samples. J. Braz. Chem. Soc. 24 (11), 1880–1886. doi:10.5935/0103-5053.20130235
Melendez-Perez, J. J., Fostier, A. H., Carvalho, J. A., Windmöller, C. C., Santos, J. C., and Carpi, A. (2014). Soil and biomass mercury emissions during a prescribed fire in the Amazonian rain forest. Atmos. Environ. 96, 415–422. doi:10.1016/j.atmosenv.2014.06.032
Michelazzo, P. A. M., Fostier, A. H., Magarelli, G., Santos, J. C., and De Carvalho, J. A. (2010). Mercury emissions from forest burning in southern Amazon. Geophys. Res. Lett. 37 (9). doi:10.1029/2009GL042220
Monperrus, M., Rodriguez Gonzalez, P., Amouroux, D., Garcia Alonso, J. I., and Donard, O. F. X. (2008). Evaluating the potential and limitations of double-spiking species-specific isotope dilution analysis for the accurate quantification of mercury species in different environmental matrices. Anal. Bioanal. Chem. 390 (2), 655–666. doi:10.1007/s00216-007-1598-z
Mowat, L. D., Louis, V. L., Graydon, J. A., and Lehnherr, I. (2011). Influence of forest canopies on the deposition of methylmercury to boreal ecosystem watersheds. Environ. Sci. Technol. 45 (12), 5178–5185. doi:10.1021/es104377y
Neary, D. G., Ryan, K. C., and Debano, L. F. Editors (2005). Wildland fire in ecosystems: effects of fire on soils and water. Gen. Tech. Rep. RMRS-GTR-42-vol.4. Ogden, UT: U.S. Department of Agriculture, Forest Service, Rocky Mountain Research Station, 250.
North Central Regional Research (2012). Recommended chemical soil test procedures for the north central region. United States of America: North Central Regional Research.
Obrist, D., Kirk, J. L., Zhang, L., Sunderland, E. M., Jiskra, M., and Selin, N. E. (2018). A review of global environmental mercury processes in response to human and natural perturbations: changes of emissions, climate, and land use. Ambio 47 (2), 116–140. doi:10.1007/s13280-017-1004-9
Outridge, P. M., Mason, R. P., Wang, F., Guerrero, S., and Heimbürger-Boavida, L. E. (2018). Updated global and oceanic mercury budgets for the united nations global mercury assessment 2018. Environ. Sci. Technol. 52 (20), 11466–11477. doi:10.1021/acs.est.8b01246
Patel, K. F., Jakubowski, M. D., Fernandez, I. J., Nelson, S. J., and Gawley, W. (2019). Soil nitrogen and mercury dynamics seven decades after a fire disturbance: a case study at Acadia national Park. Water, Air, Soil Pollut. 230 (2), 29. doi:10.1007/s11270-019-4085-1
Patry, C., Davidson, R., Lucotte, M., and Béliveau, A. (2013). Impact of forested fallows on fertility and mercury content in soils of the Tapajós River region, Brazilian Amazon. Sci. Total Environ. 458 (460), 228–237. doi:10.1016/j.scitotenv.2013.04.037
Pirrone, N., Cinnirella, S., Feng, X., Finkelman, R. B., Friedli, H. R., Leaner, J., et al. (2010). Global mercury emissions to the atmosphere from anthropogenic and natural sources. Atmos. Chem. Phys. 10 (13), 5951–5964. doi:10.5194/acp-10-5951-2010
Pleijel, H., Klingberg, J., Nerentorp, M., Broberg, M. C., Nyirambangutse, B., Munthe, J., et al. (2021). Mercury accumulation in leaves of different plant types-the significance of tissue age and specific leaf area. Biogeosciences 18 (23), 6313–6328. doi:10.5194/bg-18-6313-2021
Reis, A. T., Davidson, C. M., Vale, C., and Pereira, E. (2016). Overview and challenges of mercury fractionation and speciation in soils. TrAC - Trends Anal. Chem. 82, 109–117. doi:10.1016/j.trac.2016.05.008
Richter, L., Amouroux, D., Tessier, E., and Fostier, A. H. (2023). Impact of forest fire on the mercury stable isotope composition in litter and soil in the Amazon. Chemosphere 339, 139779. doi:10.1016/j.chemosphere.2023.139779
Rieder, S. R., Brunner, I., Horvat, M., Jacobs, A., and Frey, B. (2011). Accumulation of mercury and methylmercury by mushrooms and earthworms from forest soils. Environ. Pollut. 159 (10), 2861–2869. doi:10.1016/j.envpol.2011.04.040
Rodríguez Martin-Doimeadios, R. C., Monperrus, M., Krupp, E., Amouroux, D., and Donard, O. F. X. (2003). Using speciated isotope dilution with GC-inductively coupled plasma MS to determine and unravel the artificial formation of monomethylmercury in certified reference sediments. Anal. Chem. 75 (13), 3202–3211. doi:10.1021/ac026411a
Roulet, M., Guimarães, J.-R. D., and Lucotte, M. (2001). Methylmercury production and accumulation in sediments and soils of an amazonian floodplain-effect of seasonal inundation. Water, Air Soil Pollut. 128, 41–60. doi:10.1023/a:1010379103335
Roulet, M., and Lucotte, M. (1995). Geochemistry of mercury in pristine and flooded ferralitic soils of a tropical rain forest in French Guiana, South America. Air Soil Pollut. 80, 1079–1088. doi:10.1007/bf01189768
Rundel, P. W. (1983). “Impact of fire on nutrient cycles in mediterranean-type ecosystems with reference to chaparral,” in Mediterranean-type ecosystems: The role of nutrients. Editors F. J. Kruger, D. T. Mitchell, and J. U. M. Jarvis (New York, USA: Springer-Verlag), 192–2207.
Saatchi, S., Houghton, R. A., Dos Santos Alvalá, R. C., Soares, J. V., and Yu, Y. (2007). Distribution of aboveground live biomass in the Amazon basin. Glob. Change Biol. 13 (4), 816–837. doi:10.1111/j.1365-2486.2007.01323.x
Schneider, L., Fisher, J. A., Diéguez, M. C., Fostier, A.-H., Guimaraes, J. R. D., Leaner, J. J., et al. (2023). A synthesis of mercury research in the southern hemisphere, part 1: natural processes. Ambio 52, 897–917. doi:10.1007/s13280-023-01832-5
Shanley, J. B., Marvin-DiPasquale, M., Lane, O., Arendt, W., Hall, S., and McDowell, W. H. (2020). Resolving a paradox—High mercury deposition, but low bioaccumulation in northeastern Puerto Rico. Ecotoxicology 29 (8), 1207–1220. doi:10.1007/s10646-019-02108-z
Sombroek, W. (2000). Amazon landforms and soils in relation to biological diversity. Acta Amaz. 30 (1), 81–100. doi:10.1590/1809-43922000301100
Song, Z. W., Zhong, Z. P., ZhongXu, D. W., Hua, L., and Luo, Y. (2015). Comparison between sequential and single extraction procedures for metal speciation in fresh and dried Sedum Plumbizincicola. J. Central South Univ. 22 (2), 487–494. doi:10.1007/s11771-015-2547-1
Tabatchnick, M. D., Nogaro, G., and Hammerschmidt, C. R. (2012). Potential sources of methylmercury in tree foliage. Environ. Pollut. 160 (1), 82–87. doi:10.1016/j.envpol.2011.09.013
Tao, Z., Bian, R., and Chai, X. (2018). Methylmercury levels in cover soils from two landfills in Xi’an and Shanghai, China: implications for mercury methylation potentials. Waste Manag. 78, 331–336. doi:10.1016/j.wasman.2018.06.008
Teixeira, D. C., Lacerda, L. D., and Silva-Filho, E. V. (2017). Mercury sequestration by rainforests: the influence of microclimate and different successional stages. Chemosphere 168, 1186–1193. doi:10.1016/j.chemosphere.2016.10.081
Tsui, M. T. K., Blum, J. D., Kwon, S. Y., Finlay, J. C., Balogh, S. J., and Nollet, Y. H. (2012). Sources and transfers of methylmercury in adjacent river and forest food webs. Environ. Sci. Technol. 46 (20), 10957–10964. doi:10.1021/es3019836
Wang, X., Bao, Z., Lin, C. J., Yuan, W., and Feng, X. (2016). Assessment of global mercury deposition through litterfall. Environ. Sci. Technol. 50 (16), 8548–8557. doi:10.1021/acs.est.5b06351
Witt, E. L., Kolka, R. K., Nater, E. A., and Wickman, T. R. (2009). Influence of the forest canopy on total and methyl mercury deposition in the boreal forest. Water, Air, Soil Pollut. 199 (1–4), 3–11. doi:10.1007/s11270-008-9854-1
Zhang, H., Wang, Z., and Zhang, X. (2019a). Methylmercury concentrations and potential sources in atmospheric fine particles in Beijing, China. Sci. Total Environ. 681, 183–190. doi:10.1016/j.scitotenv.2019.05.128
Zhou, J., Obrist, D., Dastoor, A., Jiskra, M., and Ryjkov, A. (2021). Vegetation uptake of mercury and impacts on global cycling. Nat. Rev. Earth Environ. 2 (4), 269–284. doi:10.1038/s43017-021-00146-y
Zhou, J., and Obrist, D. (2021). Global mercury assimilation by vegetation. Environ. Sci. Technol. 55 (20), 14245–14257. doi:10.1021/acs.est.1c03530
Keywords: mercury, methylmercury, litterfall, soil, ash, forest fire, tropical forest
Citation: Fostier AH, Amouroux D, Tessier E, Viana JLM and Richter L (2023) Methylmercury content in soil and litter from the Amazonian rainforest and its potential fate during forest fires. Front. Environ. Chem. 4:1242915. doi: 10.3389/fenvc.2023.1242915
Received: 19 June 2023; Accepted: 29 August 2023;
Published: 14 September 2023.
Edited by:
Claude Fortin, Université du Québec, CanadaReviewed by:
Marc Amyot, Montreal University, CanadaAndrew Mitchell Graham, Grinnell College, United States
Copyright © 2023 Fostier, Amouroux, Tessier, Viana and Richter. This is an open-access article distributed under the terms of the Creative Commons Attribution License (CC BY). The use, distribution or reproduction in other forums is permitted, provided the original author(s) and the copyright owner(s) are credited and that the original publication in this journal is cited, in accordance with accepted academic practice. No use, distribution or reproduction is permitted which does not comply with these terms.
*Correspondence: Anne Helene Fostier, annehfostier@gmail.com
†These authors have contributed equally to this work and share senior authorship