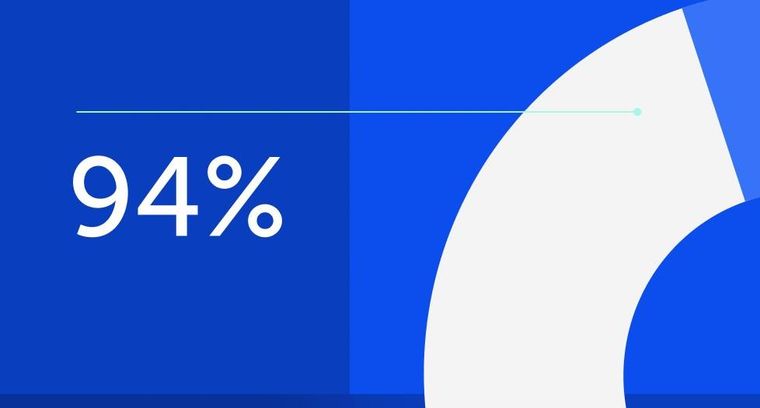
94% of researchers rate our articles as excellent or good
Learn more about the work of our research integrity team to safeguard the quality of each article we publish.
Find out more
ORIGINAL RESEARCH article
Front. Environ. Chem., 26 May 2023
Sec. Environmental Analytical Methods
Volume 4 - 2023 | https://doi.org/10.3389/fenvc.2023.1194664
This article is part of the Research TopicEmerging Trends in Advanced Oxidation Processes for Water TreatmentView all 3 articles
The chemical 6PPD-quinone is highly toxic to some fish species of the Oncorhynchus and Salvelinus genera and is the oxidation product of the common car tire additive 6PPD. We present a new sample preparation method that involves liquid-liquid extraction of water samples followed by silica-based solid phase extraction prior to LC–MS/MS analysis. The new sample preparation method showed good analyte recovery from spiked water samples (78%–91%) and a low ion suppression effect, surpassing previously published methods. This new method was successfully validated, achieving a limit of quantification of 5 ng/L and estimated expanded measurement uncertainty of 18.6%. In a proof-of-concept study, the method was applied to several water samples from various sources in Southern Norway. These were runoff samples from tunnel washing, from a tunnel runoff treatment plant and downstream of the plant drain. In addition, two water samples from puddles were included: one was run-off from an artificial soccer turf field and one from a puddle on a country road. The results of the analyses revealed that the concentration of 6PPD-quinone was above the LC50 reported for coho salmon (Oncorhynchus kisutch) in all samples except the samples from and downstream of the treatment plant. The highest measured concentration was 258 ng/L, which is the 2.7-fold of the reported LC50 in coho salmon (95 ng/L). Our initial data emphasize the need for more comprehensive environmental monitoring of 6PPD-quinone as well as toxicological studies in aquatic organisms.
The p-phenylenediamine rubber additive 6PPD (N-(1,3-dimethylbutyl)-N′-phenyl-p-phenylenediamine) (Figure 1) is one of the most popular antioxidants and antiozonants in rubber production (Datta et al., 2007). Ground level ozone (O3), also known as tropospheric ozone reacts with elastomers and induces so-called ozone cracking (Layer and Lattimer, 1990). Antiozonants such as 6PPD are used to reduce or inhibit thermal oxidation of polymers via conversion of hydroperoxide groups to non-radical products (Datta et al., 2007). The release of 6PPD and its decomposition and oxidation products into the environment occurs via leaching from tires (Johannessen et al., 2022), rubber manufacturing and recycling of used rubber products and reconstruction areas (Jarlskog et al., 2021).
FIGURE 1. Structure of 6PPD and possible pathway of formation of 6PPD-quinone (Tian et al., 2021).
The release of rubbers and rubber particles into the air and wastewater is of great concern because they contain a range of chemicals that can affect the health of both humans and animals, and our natural ecosystems. Toxicological assessment of such contamination is entangled due to the complex chemistry of rubber leachates (Muller et al., 2022). In the northwest Pacific, the coho salmon (Oncorhynchus kisutch) population is suffering from unexplained acute mortality when the fish migrate to urban rivers (Peter et al., 2018; Chow et al., 2019). Recently, this “urban runoff mortality syndrome” has been linked to 6PPD, specifically to its previously unknown oxidation product—6PPD-quinone (Tian et al., 2021; Thorp, 2022), which was detected in urban waters receiving storm water runoff (Johannessen et al., 2021). 6PPD-quinone was found to be highly toxic to coho salmon, but not to chum salmon (Oncorhynchus keta) (McIntyre et al., 2021) and other freshwater fish species used for toxicity assessment, such as Danio reri and Oryzias latipes (Hiki et al., 2021). The chemical was also non-toxic to alevins of Atlantic salmon (Salmo salar) and brown trout (Salmo trutta) (Foldvik et al., 2022), nor to the water flee, Daphnia magna, and the amphipod Hyalella azteca (Hiki et al., 2021).
Only few quantitative techniques have been reported for the analysis of 6PPD-quinone since its discovery as an emerging pollutant. The initial approach for isolation and characterization of the compound employed a combination of different column chemistries (Tian et al., 2021). A quantitative method was developed and validated by Ji et al. (2022) to analyze 6PPD-quinone in honey and fish samples. Water samples were commonly analyzed directly without any sample preparation (Hiki et al., 2021). However, none of these techniques are appropriate for analyzing environmental water samples because of their high limits of quantification, typically in the lower µg/kg-range. In this study, we developed a new method for 6PPD-quinone quantification in environmental water samples. The applicability of this method for quantifying trace levels of 6PPD-quinone in real samples was validated with isotopically labeled internal standard 13C6-6PPD-quinone. We performed preliminary analyses of several environmental water samples from different sources in southern Norway and show the presence of the compound in most of the samples.
Methanol (gradient quality) was from Romil Ltd. (Cambridge, United Kingdom). Hexane and dichloromethane (DCM) used in extractions and sample preparation were all HPLC grade from Fisher Scientific (Hampton, NH, United States). Acetonitrile and water for instrumental analyses were Optima LC–MS grade (Thermo Fisher Scientific, Waltham, MA, United States), while water used for sample preparation was Type 1 grade (PURELAB Chorus, Elga LabWater, High Wycombe, United Kingdom). Formic acid (for LC-MS LiChropur™, 97.5%–98.5%) and sodium carbonate (ReagentPlus, ≥99.5%) were from Merck (Darmstadt, Germany). 13C6-6PPD-quinone and 6PPD-quinone calibrant solutions were acquired from Cambridge Isotope Laboratories (Tewksbury, MA, United States).
Nine water samples were collected and analyzed. Three samples were collected in connection with the washing of the Stavsjøfjell tunnel near the river Homla (Trøndelag county) and one was collected in connection with washing of the Bodøtunellen (Nordland county). In addition, two samples from the water treatment plant for runoff from the Soknedal tunnel were also included, as well as a sample downstream of the outlet of this treatment plant. This sample was most likely a mixture of water from the treatment plant as well as natural runoff that runs into the same culvert. Another sample was from a puddle with runoff from artificial turf at the Rosenborg soccer field (Trondheim). Finally, one sample was collected from a puddle on country road 152 (Kykkelsrudveien near Drøbak center, Viken county). Water samples were kept at 5°C prior to sample preparation and were analyzed within 2 weeks after collection.
Two extraction methods have been compared in this study. All samples were extracted and analyzed in triplicates. Method 1 was adopted from (Tian et al., 2021), and it was scaled down and modified to be used as analytical method. Water samples (ca. 11 mL) were transferred to 15 mL polypropylene tubes and centrifuged at 22°C and 4,700 × g for 20 min. Aliquots (10.0 mL) of clean supernatants were pipetted into new tubes. To each of these aliquots, 1 ng of isotopically labelled internal standard (ISTD), 13C6-6PPDQ (100 µL of a 10 ng/mL solution in acetonitrile) was added. Mixed-mode solid phase extraction (SPE) cartridges (30 mg/1 mL, Oasis HLB, Waters, MA, United States) were prewashed with 1 mL of methanol and equilibrated with 5 mL of water. Samples (10 mL) were applied to the SPE cartridges, then washed with 2 mL of water and vacuum dried for 15 min. 6PPD-quinone was eluted using MeOH (4 × 0.4 mL), and individual fractions were evaporated to dryness using a flow of nitrogen and a temperature of 60°C. The flow rate during SPE was kept around 0.4 mL/min for all steps. The dried residues were dissolved in 1 mL of acetonitrile and kept at −20°C until LC–MS/MS analysis.
Method 2 was specifically developed for dirty water samples. Samples (ca. 11 mL) were transferred to 15 mL polypropylene tubes and centrifuged at 22°C and 4,700 × g for 20 min. Aliquots (10.0 mL) of supernatants were pipetted into new tubes. To each of the aliquots, 1 ng of ISTD (i.e., 100 µL of a 10 ng/mL solution in acetonitrile) and 100 µL of 10% (m/v) sodium carbonate in water were added and solutions were vigorously mixed for 5 s. Lipophilic constituents of the samples were extracted by addition of 1 mL of DCM and shaking at 22°C on an orbital shaker at 1,100 × rpm for 15 min. Phase separation was facilitated by centrifugation at 22°C and 4,700 × g for 3 min. The organic phase was collected and diluted with 2 mL of hexane. Phenomenex Strata® Si-1 silica (55 μm, 70 Å, 100 mg/1 mL) SPE columns were washed with 2 mL of DCM and equilibrated with 3 mL of a 40:60 (v/v) mixture of DCM/hexane followed by applying the DCM/hexane extracts. The columns were washed with 1 mL of a 40:60 mixture of DCM/hexane, and 6PPD-quinone was eluted with 3 mL of DCM. The flow rate during SPE using the silica gel columns was kept at approximately 2 mL/min. The solutions were dried using a nitrogen flow and a temperature of 60°C. The residues were dissolved in 200 µL of acetonitrile and kept at −20°C until LC–MS/MS analysis.
Samples were analyzed on an Agilent 6470A triple stage quadrupole instrument interfaced to an Agilent 1290 Infinity II LC System (Agilent Technologies, Santa Clara, CA, United States). Three microliters of each sample was injected onto an Agilent Eclipse Plus C18 RRHD LC column (1.8 µm, 3.0 × 50 mm, Agilent Technologies). Mobile phase solvent A was 0.1% formic acid in water and mobile phase B was 0.1% formic acid in acetonitrile. The column was kept at 30°C and eluted using a linear gradient starting with 20% B and rise to 90% B over 4 min, followed by isocratic elution with 90% B for 1 min and then equilibration with 20% B for 1 min. The flow rate was set to 0.6 mL/min across the entire LC run. Two multiple reaction monitoring transitions (MRM) were acquired for each of 6PPD-quinone and its isotopically labelled ISTD in positive ionization mode: 6PPD-quinone, m/z 299.1→187.3 (collision energy, CE = 31 V) and m/z 299.1→77.3 (CE = 77 V), 13C6-6PPD-quinone, m/z 305.1→193.3 (CE = 31 V) and m/z 305.1→83.3 (CE = 77 V) for quantifier and qualifier ion transitions, respectively. Other instrument parameters were: gas temperature, 250°C; gas flow, 5 L/min; nebulizer, 45 psi; sheath gas temperature, 350°C; sheath gas flow, 10 L/min; capillary voltage, 3500 V and nozzle voltage, 500 V. The instrument was calibrated in the range 0.250–100 ng/mL using a set of ten calibration standards in acetonitrile. The concentration of 13C6-6PPD-quinone in the calibrants was 5 ng/mL. The raw data were processed using Agilent MassHunter Qualitative Analysis Navigator and Quantitative Analysis software, both version B.08.00.
Sample S3 (Table 1) was selected for the determination of matrix effects. This sample was tunnel-wash runoff water, and it was visually the most contaminated sample with road dust and tire wear. Two sets of triplicates, alongside with blanks (pure water) were treated following both Method 1 and Method 2, but with addition of ISTD before or after extraction. Matrix effects were calculated as 100%−(100% × PAsample/PAstandard), where PAsample and PAstandard are peak areas of the ISTD quantifier ion measured in sample extract or pure solvent, respectively. Absolute recovery (or recovery factor) was calculated by comparing the peak area ratios between 6PPD-quinone and the isotopically labeled internal standard (ISTD, 13C6-6PPD-quinone) for two Quality Control samples (QC samples) with concentrations of 23 ng/L and 500 ng/L for QC1 and QC2, respectively, with ISTD added before or after extraction.
TABLE 1. Sample type and location including geographical coordinates, date of collection and measured concentration of 6PPD-quinone (relative standard deviation from measurement of triplicates in parentheses).
Method validation was conducted according to Supersedes Document No. SANTE/2019/1268, 2022. Negative sample S5 (Table 1) was selected for determination of the limit of quantification (LOQ) and expanded measurement uncertainty (U′) (Supplementary Material S1). For LOQ determination, six replicates of Sample S5 were spiked at a final concentration of 5.0 ng/L, which had been estimated as a provisional LOQ by serial dilution of pure 6-PPD-quinone. Measurement of method uncertainty was based on six replicates spiked at a concentration 43 ng/L (Supplementary Material S1). Spiked samples were extracted according to Method 2 and analyzed on five consecutive days. Ion ratios of quantifier/qualifier ion transitions from spiked samples and calibration standards as well as the signal/noise ratios were obtained from MassHunter Quantitative software. All calculations were conducted in Microsoft Excel 2018. Bias was defined as difference between mean measured concentration and expected concentration, precision was expressed as relative standard deviation of measured concentration, within-day and inter-day (U′) was calculated according to Supersedes Document No. SANTE/2019/1268, 2022.
In recent reports, 6PPD-quinone analysis was based on a method that utilizes reverse-phase SPE for sample clean-up and up-concentration (Hou et al., 2019; Tian et al., 2021). This method (herein referred to as Method 1) was originally developed for non-target screening allowing analysis of a wide range of lipophilic compounds in complex water samples (Peter et al., 2019). We have employed and tested this method and found that it is not suitable for trace analysis of 6PPD-quinone in tunnel-wash runoff samples due to strong ion suppression of co-eluting matrix contaminants during electrospray ionization. Method 1 provided clear MRM chromatograms in less complex samples, such as the runoff from an artificial turf pitch and the sample from a road puddle. Analysis of 6PPD-quinone in complex samples, e.g., tunnel-wash runoff, processed by Method 1 was hampered by a noisy background (Figures 2). Most likely, the instrumental noise was caused by the presence of a relatively high concentration of detergents present in the water for cleaning of the tunnels. We addressed this issue and developed a targeted method employing liquid-liquid extraction (LLE) and normal phase SPE. LLE was found to be highly effective for removing water-soluble molecules. Additionally, normal phase SPE provided better clean-up than reverse-phase SPE due to its orthogonality to the reverse-phase UHPLC column used during instrumental analysis. All these modifications lead to a significant improvement in the detection of 6PPD-quinone (Figure 2). We observed that the signal/noise was in average 13 times higher when sample S3 was processed with Method 2 compared to Method 1. This difference was due to ion suppression effects, which were 95% for Method 1, compared to 3.2% for Method 2. Another disadvantage of Method 1 is that it is rather time consuming since it requires passing large volumes of water through SPE columns at low flow rate, as opposed to Method 2 where up-concentration is done by a single LLE step. Furthermore, normal phase SPE could be performed at higher flow rates (ca. 2 mL/min vs. 0.4 mL/min) without substantial analyte losses. As a consequence, Method 2 was faster to perform, although it formally includes more steps (LLE and SPE) compared to Method 1 (SPE only).
FIGURE 2. Chromatograms from LC–MS/MS analyses of sample S3 from tunnel-wash runoff, containing 143 ng/L 6PPD-quinone, using two different sample preparation protocols. (A,B), MRM chromatograms based on the quantifier ion of 6PPD-quinone following sample preparation with Method 1 and Method 2, respectively; (C,D), MRM chromatograms of 13C6-6PPD-quinone following sample preparation with Method 1 and Method 2, respectively. To ensure realistic comparison between the two methods, the final volume for Method 2 was adjusted to 1 mL in accord with Method 1.
The retention time of 6PPD-quinone was 3.6 min, which allowed good separation of the compound from other lipophilic matrix constituents as well as parent 6PPD (retention time 1.9 min, data not shown). The total run time for one LC–MS/MS analysis was 6 min. The selectivity and sensitivity have been evaluated based on solvent blanks and negative samples, and no interfering peaks were observed. The instrument response was linear over a wide range of concentrations (250 pg/mL to 100 ng/mL) with regression coefficients R2 > 0.99 for linear, ten-point calibration curves based on internal calibration. No carryover was observed after injection of the highest calibration standard. The absolute recovery of 6PPD-quinone was 91% for QC sample 1% and 78% for QC sample 2 (average for n = 3, RSD = 2.3% and 3.7%, respectively). Since quantification was based on an isotope-dilution procedure, the internal standard (13C6-6PPD-quinone) was added before extraction and thus efficiently compensated for remaining matrix effects and variations in absolute recovery. The LOQ for 6PPD-quinone was determined to 5.0 ng/L. At this concentration, the qualifier-to-quantifier ion ratio from spiked samples was in the range 45%–65%, which was within 30% (relative) of the average ratio for calibration standards acquired on the same day (51%). The signal/noise ratio was ≥3 for the qualifier ion. Bias, defined as difference between mean measured value and true value was in range 2.61%–14.8%. Precision, defined as relative standard deviation of measured results at the LOQ was in the range 2.13%–6.21%. Expanded measurement uncertainty (U′) was estimated using six replicate samples spiked at a concentration of 43.0 ng/mL. Within-day U′ was 7.46%–23.8%, while inter-day U′ was measured by analysis of the same sample replicate on consecutive 5 days and found to be 18.6%.
Nine water samples were analyzed in this pilot study (Figure 3), and 6PPD-quinone could be detected in most samples (Table 1). The three tunnel-wash water samples (S1–S3) from Stavsjøfjell tunnel contained average concentrations of 110–143 ng/L, which decreased to 7–23 ng/L in the water from the treatment facility (S4 and S6) (Table 1). Concentrations of 6PPD-quinone in a sample from the culvert following the treatment plant were found to be below the LOQ (<5 ng/L). This indicated that the tunnel-wash water treatment facility was effective in reducing 6PPD-quinone. Rubber granules produced from discarded car tires are often used for artificial turf pitches. Another sample was thus runoff from an artificial turf pitch (S7), and it was contaminated with 6PPD-quinone at a level of 159 ng/L. The highest concentration of 6PPD-quinone (258 ng/L) was found in a country road puddle (S8), indicating the spread of this pollutant in urban areas. Finally, a filtered sample from tunnel-wash runoff from Bodøtunnelen was found to be moderately contaminated (49.5 ng/mL).
FIGURE 3. Map over southern and central Norway showing the collection sites for the water samples that were included in this pilot study.
The new method for preparation of water samples for the targeted analysis of 6PPD-quinone utilizes an isotopically labeled internal standard, 13C6-6PPD-quinone, and it possesses significant advantages over previously published methods such as excellent apparent recovery, low ion suppression effects and a low limit of quantification. Application of this method for analysis of environmental samples showed that 6PPD-quinone is likely widespread in the Norwegian environment at concentrations that exceed the LC50 for some fish species. Our pilot data warrant the necessity of a larger survey on the presence of 6PPD-quinone in the environment, including toxicity assessments for key species. Due to seasonal variation in precipitation, concentrations of 6PPD-quinone might vary significantly. It is also possible that during the snowmelt period there is an increased influx of accumulated 6PPD-quinone into the environment. Further studies should thus investigate seasonal and regional variation of the chemical. Finally, the decomposition pathways of 6PPD in the environment have so far only been studied to some extent.
The datasets presented in this study can be found in online repositories at https://doi.org/10.6084/m9.figshare.22618999.v2.
FK: methodology, validation, formal analysis, writing—original draft. AF: supervision, formal analysis, sampling, funding acquisition, project administration, writing—review and editing. RS: supervision, formal analysis, funding acquisition, project administration, writing—review and editing. SU: methodology, validation, formal analysis, writing—original draft, writing—review and editing. All authors contributed to the article and approved the submitted version.
This study was supported from The Norwegian Environment Agency (Miljødirektoratet).
We thank Pål Asgeir Olsvik and Shubham Varshney (Nord University, Bodø, Norway) for providing one of the samples for analysis and method validation. Øystein Nordeide Kielland is acknowledged for preparing the map with sampling information.
The authors declare that the research was conducted in the absence of any commercial or financial relationships that could be construed as a potential conflict of interest.
All claims expressed in this article are solely those of the authors and do not necessarily represent those of their affiliated organizations, or those of the publisher, the editors and the reviewers. Any product that may be evaluated in this article, or claim that may be made by its manufacturer, is not guaranteed or endorsed by the publisher.
The Supplementary Material for this article can be found online at: https://www.frontiersin.org/articles/10.3389/fenvc.2023.1194664/full#supplementary-material
Chow, M. I., Lundin, J. I., Mitchell, C. J., DavisYoungScholz, J. W. G. N. L., and McIntyre, J. K. (2019). An urban stormwater runoff mortality syndrome in juvenile coho salmon. Aquat. Toxicol. 214, 105231. doi:10.1016/j.aquatox.2019.105231
Datta, R. N., HuntinkDatta, N. M. S., and Talma, A. G. (2007). Rubber vulcanizates degradation and stabilization. Rubber Chem. Technol. 80 (3), 436–480. doi:10.5254/1.3548174
Foldvik, A., Kryuchkov, F., Sandodden, R., and Uhlig, S. (2022). Acute toxicity testing of the tire rubber–derived chemical 6PPD-quinone on Atlantic salmon (Salmo salar) and Brown trout (Salmo trutta). Environ. Toxicol. Chem. 41 (12), 3041–3045. doi:10.1002/etc.5487
Hiki, K., Asahina, K., Kato, K., Yamagishi, T., Omagari, R., Iwasaki, Y., et al. (2021). Acute toxicity of a tire rubber-derived chemical, 6PPD quinone, to freshwater fish and Crustacean species. Environ. Sci. Technol. Lett. 8 (9), 779–784. doi:10.1021/acs.estlett.1c00453
Hou, F., Tian, Z. Y., Peter, K. T., Wu, C., Gipe, A. D., Zhao, H. Q., et al. (2019). Quantification of organic contaminants in urban stormwater by isotope dilution and liquid chromatography-tandem mass spectrometry. Anal. Bioanal. Chem. 411 (29), 7791–7806. doi:10.1007/s00216-019-02177-3
Jarlskog, I., Stromvall, A. M., Magnusson, K., Galfi, H., Bjorklund, K., Polukarova, M., et al. (2021). Traffic-related microplastic particles, metals, and organic pollutants in an urban area under reconstruction. Sci.Total Environ. 774, 145503. doi:10.1016/j.scitotenv.2021.145503
Ji, J., Li, C., Zhang, B., Wu, W., Wang, J., Zhu, J., et al. (2022). Exploration of emerging environmental pollutants 6PPD and 6PPDQ in honey and fish samples. Food Chem. 396, 133640. doi:10.1016/j.foodchem.2022.133640
Johannessen, C., Helm, P., Lashuk, B., Yargeau, V., and Metcalfe, C. D. (2022). The tire wear compounds 6PPD-quinone and 1,3-diphenylguanidine in an urban watershed. Arch. Environ. Contam. Toxicol. 82 (2), 171–179. doi:10.1007/s00244-021-00878-4
Johannessen, C., Helm, P., and Metcalfe, C. D. (2021). Detection of selected tire wear compounds in urban receiving waters. Environ. Pollut. 287, 117659. doi:10.1016/j.envpol.2021.117659
Layer, R. W., and Lattimer, R. P. (1990). Protection of rubber against ozone. Rubber Chem. Technol. 63 (3), 426–450. doi:10.5254/1.3538264
McIntyre, J. K., Prat, J., Cameron, J., Wetzel, J., Mudrock, E., Peter, K. T., et al. (2021). Treading water: Tire wear particle leachate recreates an urban runoff mortality syndrome in coho but not chum salmon. Environ. Sci. Technol. 55 (17), 11767–11774. doi:10.1021/acs.est.1c03569
Muller, K., Hubner, D., Huppertsberg, S., Knepper, T. P., and Zahn, D. (2022). Probing the chemical complexity of tires: Identification of potential tire-borne water contaminants with high-resolution mass spectrometry. Sci. Total Environ. 802, 149799. doi:10.1016/j.scitotenv.2021.149799
Peter, K. T., Herzog, S., Tian, Z. Y., Wu, C., McCray, J. E., Lynch, K., et al. (2019). Evaluating emerging organic contaminant removal in an engineered hyporheic zone using high resolution mass spectrometry. Water Res. 150, 140–152. doi:10.1016/j.watres.2018.11.050
Peter, K. T., Tian, Z. Y., Wu, C., Lin, P., White, S., Du, B. W., et al. (2018). Using high-resolution mass spectrometry to identify organic contaminants linked to urban stormwater mortality syndrome in coho salmon. Environ. Sci. Technol. 52 (18), 10317–10327. doi:10.1021/acs.est.8b03287
Supersedes Document No. SANTE/2019/1268 (2022). Analytical quality control and method validation procedures for pesticide residues analysis in food and feed. Available at: https://www.eurl-pesticides.eu/userfiles/file/EurlALL/SANTE_11312_2021.pdf (Accessed March 23, 2023).
Thorp, H. H. (2022). Erratum for the Report “A ubiquitous tire rubber–derived chemical induces acute mortality in coho salmon,” by Z. Tian, H. Zhao, K T. Peter, M. Gonzalez, J. Wetzel, C. Wu, X. Hu, J. Prat, E. Mudrock, R. Hettinger, A. E. Cortina, R. G. Biswas, F. V. C. Kock, R. Soong, A. Jenne, B. Du, F. Hou, H. He, R. Lundeen, A. Gilbreath, R. Sutton, N. L. Scholz, J. W. Davis, M. C. Dodd, A. Simpson, J. K. Mcintyre, E. P. Kolodziej. Science 375 (6582), eabo5785. doi:10.1126/science.abo5785
Keywords: car tire, liquid chromatography, mass spectrometry, runoff water, artificial turf pitch, 6PPDQ, 6PPD-quinone
Citation: Kryuchkov F, Foldvik A, Sandodden R and Uhlig S (2023) Presence of 6PPD-quinone in runoff water samples from Norway using a new LC–MS/MS method. Front. Environ. Chem. 4:1194664. doi: 10.3389/fenvc.2023.1194664
Received: 27 March 2023; Accepted: 08 May 2023;
Published: 26 May 2023.
Edited by:
Massoud Kaykhaii, Gdansk University of Technology, PolandReviewed by:
Joanna Zembrzuska, Poznań University of Technology, PolandCopyright © 2023 Kryuchkov, Foldvik, Sandodden and Uhlig. This is an open-access article distributed under the terms of the Creative Commons Attribution License (CC BY). The use, distribution or reproduction in other forums is permitted, provided the original author(s) and the copyright owner(s) are credited and that the original publication in this journal is cited, in accordance with accepted academic practice. No use, distribution or reproduction is permitted which does not comply with these terms.
*Correspondence: Silvio Uhlig, c2lsdmlvdUB1aW8ubm8=
Disclaimer: All claims expressed in this article are solely those of the authors and do not necessarily represent those of their affiliated organizations, or those of the publisher, the editors and the reviewers. Any product that may be evaluated in this article or claim that may be made by its manufacturer is not guaranteed or endorsed by the publisher.
Research integrity at Frontiers
Learn more about the work of our research integrity team to safeguard the quality of each article we publish.