- 1Division of Environmental Sciences, FoH, Sher-e-Kashmir University of Agricultural Sciences and Technology of Kashmir, Srinagar, India
- 2Dr. Ambedkar International Centre, Ministry of Social Justice and Empowerment Government of India, New Delhi, India
- 3Division of Basic Sciences and Humanities, FoH, Sher-e-Kashmir University of Agricultural Sciences and Technology of Kashmir, Srinagar, India
- 4Division of Agricultural Statistics, FoH, Sher-e-Kashmir University of Agricultural Sciences and Technology of Kashmir, Srinagar, India
- 5Division of Basic Science and Humanities, Faculty of Agriculture, Sher‐e‐Kashmir University of Agricultural Sciences and Technology, Sopore, India
- 6Division of Fruit Science, Faculty of Agriculture, Sher‐e‐Kashmir University of Agricultural Sciences and Technology, Sopore, India
Trace elements are a major pollutant in the river water and these pollutants are present in all components of the ecological system. Since time immemorial, the River Veshaw has been one of the important sources of water and has shaped the cultural and social values in the Kashmir Valley. This study was conducted in rural parts of South Kashmir in the Western Himalaya from February to January 2020–2022. The river Veshaw provides various ecosystem services to the local communities as well as in the upper and lower stream regions in the form of many direct and indirect ecosystem services. The river is polluted by human waste from both rural and urban communities, as well as by agricultural runoff and effluent discharges from a variety of industrial activities due to its proximity. Effluent that makes it to the river contains a variety of pollutants, some of which are trace elements that accumulate in the local ecosystem, killing off plants and animals and reducing biodiversity. Trace element levels in water and sediment were found to follow the trend as: Sangam > Khudwani > Kulgam > Nihama > Aharbal > Kingwattan. The dominance pattern of heavy metals in water was Pb > As > Cd. The overall trend showed a downward trend of heavy metals, indicating the effect of land area drainage and anthropogenic activities on the stream water. The dominance pattern of heavy metals in water was Pb > As > Cd. Heavy metals were not detected in the middle and upstream sites. The average levels of heavy metals were highest at Downstream (Sangam), with values of Cd, Pd ad As 0.0054, 0.038 and 0.038 mgL-1. This shows that land drainage and human activities have an effect on the water in the stream.
1 Introduction
The pollution of the aquatic ecological system with trace elements has become a worldwide ecological problem in recent years. These trace elements are indestructible and most of them have toxic effects on aquatic organisms (MacFarlane and Burchett, 2000; Storto et al., 2021). Among various environmental pollutants, heavy metals are of particular concern due to their potential toxic effects and ability to bioaccumulate in aquatic ecosystems (Fernandes et al., 2006; Khan N. A. et al., 2020; Bhat et al., 2022). Trace elements in general, and heavy metals in particular, are significant toxicants found in surface water (Buragohain et al., 2010). Trace elements are one of the most serious pollutants in the natural environment due to their toxicity, persistence, and bioaccumulation problems (Pekey, 2006; Nouri et al., 2006). Many scholars have studied the trace element contamination of surface water in different locations in India (Ram and Singh, 2007; Tiwari et al., 2016; Lohani et al., 2008). Trace element pollution has been assessed by the distribution of particle size and the organic content present in the water system (Saif and Khan, 2017; Bano et al., 2018; Prieto et al., 2018). The bioavailability of trace elements depends on the concentration of anions, chelating agents in the water, pH, and the presence of absorptive sediments. Ali and Khan, 2018 suggested in their study that the increase in trace elements in the surface water was due to the addition of effluents released from industrial and commercial areas.
Heavy metal (HM) contamination has piqued the world’s attention due to its toxicity to living organisms through the bioaccumulation process (Ekmekyapar et al., 2012). HMs are typically classified as metals or metalloids with a higher elemental density. These are subdivided on the basis of density, weight or atomic number (Teixeira de Souza et al., 2021; Bhat et al., 2022; Kumar et al., 2022). The new standard, stating that HM should be mineral mining (Ali et al., 2019; He et al., 2021) According to some researchers the main sources of heavy metal concentration (HMC) in China are agriculture and manufacturing, as a large amount of waste is produced in these activities, posing significant health risks to society on a large scale (Sun et al., 2010; Ohiagu et al., 2020; Karmakar et al., 2021; Bhat et al., 2022). In addition to the above, cosmetics and chemical fertilisers contribute to heavy metal pollution (Kanwar et al., 2020). Intriguingly, heavy metals released by vehicles have been deposited on plant leaves and the surface of soil (Harrison et al., 1981; Vardhan et al., 2019; Malunguja et al., 2022). Studies have demonstrated the occurrence of different hazardous HMs in the soil or farm areas (Turer and Maynard, 2003; Bhat et al., 2022). Recent studies conducted on untreated effluent water have been proven to be a substantial source of HM polluted water and other areas of the world (Tauqeer et al., 2022). Sewage water has been linked to the presence of potentially harmful elements like Cd, Cu, Ni, Cr, Pb and Zn in soil, plants, and foods (Natasha et al., 2022).
The unplanned and unregulated development of urbanisation during the last two decades has resulted in the degradation of environmental health, particularly in developing countries like India (Rauf et al., 2009; Madhav et al., 2020; Bano et al., 2022). A number of anthropogenic activities may alter the dynamic properties of lotic ecosystems by adding heavy metals to the water bodies (Cortecci et al., 2009; Bhat et al., 2011; Hasan et al., 2021; Khan et al., 2022). The presence of heavy metals in water is considered devastating and disturbs the aquatic system due to their toxicity, persistence, non-degradability, and bio-accumulation properties. All these factors are leading to serious anomalies in the aquatic ecosystem (Yang et al., 2009; Khan A. H. et al., 2020; Rather RA. et al., 2022). Trace metals may be naturally present in the biosphere and their fast release into the environment is augmented by human activities. The chemical cycling of trace metals is intricate due to the variety of factors (biotic and abiotic) involved in the manifestation of metal behavior, such as bio-chemical processes, hydrology, climatic factors, land use, and metal characteristics (Husain Khan et al., 2020; Mazhar et al., 2020; Khan et al., 2021). (Damian et al., 2019; Padder et al., 2021; Rather et al., 2022c; Padder et al., 2022) looked at the ecological value of trace metals in fresh water systems and the level of pollution in the water of the Kashmir Himalayas.
The present study is based on the analysis of seasonal changes in monitoring the quality of drinking water in the Veshaw River in the Indian Western Himalayas. The purpose of conducting this study was to improve the quality of drinking water in that area on the basis of the recommendation of the study. The Veshaw river is located adjacent to the agriculture and horticulture area and small workshop and mini-industrial units. The majority of these wastes and effluents are discharged into the environment as a result of these activities. These various forms of affluent discharged into the river are major concerns for changing the ecological system of the river. The Veshaw river is a major source of water for domestic, agricultural, and industrial uses in the south of Kashmir. Water from the Veshaw river is being used for various purposes that may expose the users to some health hazards, hence the need to determine the level of heavy metals it contains for the safety of the users. The main objective of this study is to investigate the presence and to examine the average concentration of heavy metals in the water of the Veshaw River; this involves investigating the possible natural sources and impacts of anthropogenic activities on the water quality.
2 Materials and methods
2.1 Sampling procedure and analysis of heavy metals
Three different locations were used to collect water samples for testing the quality of the rice water, viz., Site-I and II (Kongwaton 33o62′80.3″N, 74o74′08″E and Aharbal 33°38′45.4560″N 74°47′50.4696″E, an upstream site); Site-III and IV (Nihama 33°38′8″N 74°53′34″E and Kulgam 33°38′24″ N 75°01′12″ E, a midstream site); and Site V and VI (Khudwani 33.7074° N, 75.1054° E and Sangam 33.828,217°N 75.070842°E, a completely downstream site) mentioned in Figures 1, 2. The locations were chosen depending on the impacts of anthropogenic activities or on the basis of the observation of the visual analysis of pollution sources like agriculture activities, horticulture activities, human habitation, commercial and other anthropogenic and livestock pressures along the banks of the Veshaw river. From these survey sites, the collected samples were analysed to identify seasonal fluctuations (Rather et al., 2022b; Rather et al., 2022e). The samples were collected at an interval of 2 months in the years 2020–2022. Five water samples were collected from each sampling site, and a total of 30 water samples were analysed in the laboratory. The seasonal sampling was carried out, and the samples were stored in sterilised bottles. In order to analyse the chemical features of the water, it was immediately refrigerated at -4°C and transferred to the lab. In addition to sorting data by season, the results were analysed to identify seasonal fluctuations.
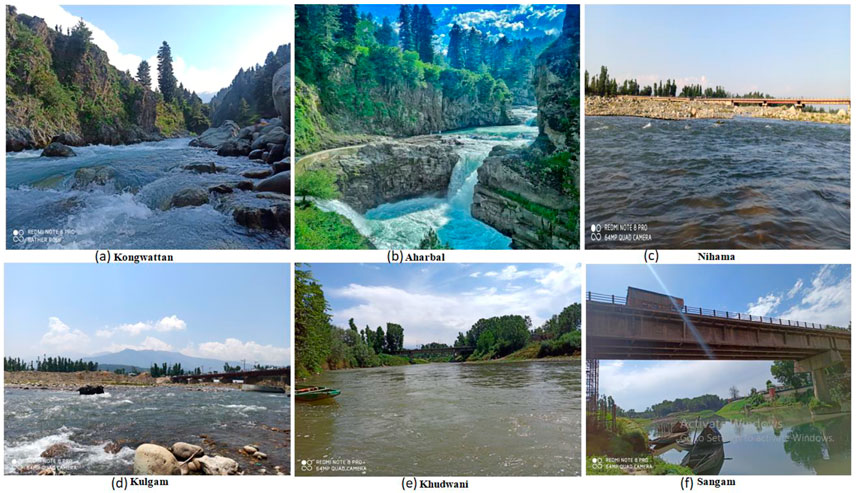
FIGURE 2. Sampling locations during collection of water samples from Veshaw river [(A):Kongwattan; (B)Aharbal; (C):Nihama; (D):Kulgam; (E):Khudwani; (F):Sangam].
2.2 Trace element estimation in water
A total of 500 ml of water was collected up to a depth of 30 cm and immediately transported to the laboratory where the samples were filtered and acidified with a few drops of concentrated HNO3 before being preserved in polythene bottles for subsequent trace element analysis using an Inductively Coupled Plasma Optical Emission Spectrophotometer (Varian Vista MPX, 720). Among the estimated trace elements are the trace elements (APHA. 2005; Rather et al., 2022a).
Principle: Equipment for ICP optical emission spectrometry consists of a light source unit, a spectrophotometer, a detector, and a data processing unit. An aqueous sample is converted to aerosols via a nebulizer. The aerosols are transported to the inductively coupled plasma, which is a high temperature zone (8,000–10,000°C). The analytes are excited to different (atomic and/or ionic) states and produce characteristic optical emissions. These emissions are separated based on their respective wavelengths and their intensities are measured. The intensities are proportional to the concentrations of analytes in the aqueous sample. Total elemental concentration is analysed by ICP-OES. The Merck-Certipur reference trace element standards were used to calibrate the ICP-OES and the detection limit for each trace element was also calculated before analysing the samples.
The achieved detection limits are: arsenic (As) 0.80; lead (Pb) 0.29; cadmium (Cd) 0.11 mg kg−1. The developed method is applied for the determination of hazardous metals in the water of the river Veshaw.
2.3 Statistical procedures
The mean values of parameters were presented and analysed using descriptive analysis. Using the four distinct seasons of the year, we were able to categories the information into 4 seasons: spring, summer, autumn, and winter. Correlation matrix through XL-stat after two-way ANOVA SPSS statistic version 23 was used to collect the data. ANOVA was used to examine the differences between all of the data points (ANOVA).
3 Result and discussion
Many harmful substances, including heavy metals and other trace elements, can be found in surface water. Many regions of India have had their surface water reported to be contaminated with trace elements (Lohani et al., 2008). Particle size distribution and the organic content in the water system are considered to be contributors to trace element pollution (Simokawa et al., 1984). All trace elements in the present investigation revealed substantial seasonal and spatial variability.
3.1 Cadmium
In river water, cadmium (Cd) is mostly present as CdCO3 species. In recent decades, there has been an increase in the production and use of Cd and its compounds. Cd enters river water from industrial discharges, mining wastes, and atmospheric deposition due to the combustion of fossil fuels (Syres et al., 1986; Patel et al., 2018). Another source is washout from agricultural land because some fertilisers contain Cd levels of even up to 40 mg/kg (Kumar et al., 2022). One of the major sources of diffuse Cd pollution is the production of inorganic fertilisers from phosphate ores (Paul et al., 1994; Rashmi et al., 2020). The major environmental Cd sources are Zn and Pb ores as it occurs naturally in their sulphide ores. Commercially, it is produced as a by-product of the Pb and Zn smelting processes (Stewart et al., 2022) Raw Zn, Zn alloys, and Zn compounds may have high concentrations of Cd, so that it may enter the river water from solid wastes as well (Emoyan et al., 2005). At sewage treatment plants, the presence of Cd in waste is a matter of great concern because sewage sludge contaminated with Cd becomes unfit for use in fertilising soils (Lokhande and Sathe, 2001; Penido et al., 2019). The sites of greatest Cd accumulation in the body are vital organs like the liver and kidney. After gastrointestinal absorption, Cd is concentrated in the kidney. Cd poisoning causes nephrotoxicity (kidney damage). Other toxic effects of Cd exposure include a decrease in haemoglobin concentration, erythrocyte destruction, an increase in blood pressure, liver damage, and male sterility (Aggarwal et al., 2000; Priyadarshanee et al., 2022).
The concentration of cadmium in the river Veshaw is shown in Figure 3. The cadmium concentration was found below the detection limit in the upstream and in the middle stream during the entire study period of 2020–2022. The lowest amount of Cd was found in the middle stream (Kulgam) at 0.0013 mgL-1. In the summer, the maximum amount of Cd was found in the downstream (Sangam) area at 0.0091 mgL−1. In the Veshaw river, the cadmium concentration was found below the detection limit upstream at an undisturbed site (Kongwattan and Aharbal) and in the middle stream at Nihama during all the seasons (Figure 3). Cadmium was detected in the middle and downstream during all seasons. The overall trend showed a significant downstream increase (p 0.05) in the cadmium level of water. The increase in the cadmium concentration downstream may be attributed to increased anthropogenic sources of cadmium from the riparian zone in the form of increased domestic sewage, dumpsites, runoff from workshops, agricultural fields, and sanitary pipelines The results of the present study are in agreement with the results of Aggarwal et al. (2000), Lokhande and Sathe (2001), and Amuah et al. (2022). Seasonal variation of the collected samples revealed the highest concentration of this metal in the summer and autumn seasons, coinciding well with the low discharge during these seasons. A similar seasonal trend was observed by Mondol et al. (2011) around Tejgoan, Bangladesh. The study conducted by Yao et al. (2014) also reported the seasonal difference in the cadmium concentration in the rivers of China, with a high concentration during the dry (summer) season and related it to the background and long-term accumulation in the environment. Other related seasonal trends for Cd have been reported by Emoyan et al. (2005) in the river Ijana, Nigeria; Kar et al. (2008) in the river Ganga, West Bengal, India; and Buragohain et al. (2009) in the Dhemaji stream, Assam, India. In addition, Paul et al. (1994) also reported higher levels of Cd in the summer, while Kaushik et al. (2009) observed the lowest and highest Cd concentrations during the summer and rainy seasons in the river Periyar, Kerala and the river Yamuna, Haryana, Gwalior, respectively. The concentration of Cd at this point was found to be below the detection limit (BDL) in all the seasons. Sangam downstream showed high levels of Cd during the entire study period as this site receives heavy loads of sewage and effluents from a variety of small-scale industrial units, which dump their wastes directly into the river without any treatment. Cooper et al. (1978) and Aggarwal et al. (2000) have reported an increase in Cd concentration in water with an increase in the quantity of sewage, while high Cd content in water receiving commercial, domestic, and industrial effluents has been reported by Lokhande and Sathe (2001). Cd also finds its way into water from agricultural runoff as fertilisers are known to contain Cd impurities (Syres et al., 1986).
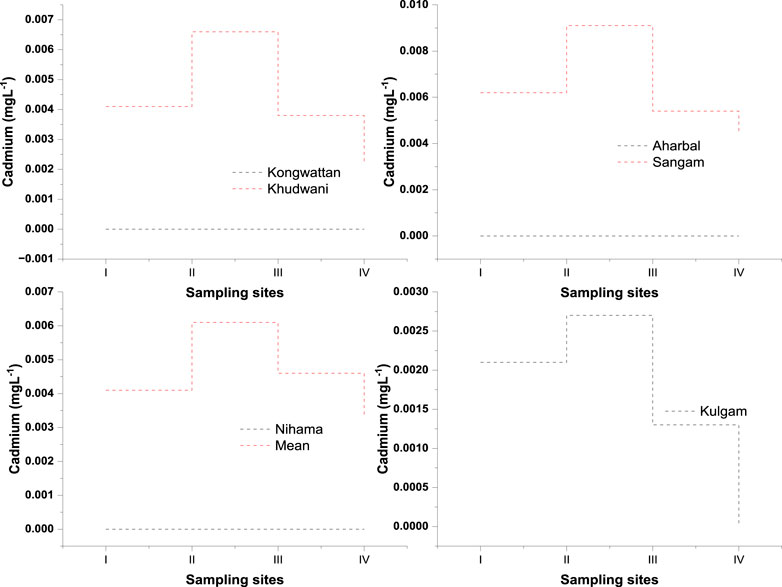
FIGURE 3. Figure depicting the comparative time-series seasonal variation analysis of Cadmium (Cd) in the water of the River Veshaw at six different sites collected during all the seasons from spring to winter (I:spring; II:summer; III:autumn; IV:winter; V) Each symbol represents the mean of triplicate values.
3.2 Lead (Pb)
Inorganic Pb occurs in water as carbonates and hydroxides. Major anthropogenic inputs of Pb in water include industries, mining, smelting plants, and vehicular inputs. It also enters into water bodies from sewage sludge. Pb is used in the production of lead acid batteries, solder, alloys, cable sheathing, pigments, rust inhibitors, ammunition, glazes, and plastic stabilizers. Tetraethyl and tetramethyl Pb are important because of their extensive use as antiknock compounds in petrol in many developing countries. Mild Pb poisoning can cause anaemia, headaches, sore muscles, fatigue, and irritability. Acute Pb poisoning causes severe kidney, liver, reproductive, and central nervous system dysfunction (Strehlow and Barltrop, 1987; Balali-Mood et al., 2021). It damages RBCs and delta-aminolevulinic acid dehydratase activity, interferes with heme synthesis, inhibits haematopoiesis and produces adverse effects on blood vessels (Shafiq-ur-Rehman, 2010; Rahimpoor et al., 2020). Pb can also cause osteoporosis by replacing calcium in bones (Ferner, 2001). The role of Pb in causing mental retardation, particularly in children, is also well documented by many researchers (Ferner, 2001). Pb is present in surface water as a result of its dissolution from natural sources, but primarily from household plumbing systems in which the pipes, solder, fittings, or service connections to homes contain Pb. Polyvinyl chloride (PVC) pipes also contain Pb compounds that can be leached from them and result in high Pb concentrations in surface water. The amount of lead dissolved from the plumbing system depends on several factors, including the presence of chloride and dissolved oxygen, pH, temperature, water softness, and standing time of the water Tam and Elefsiniotis, 2009). The concentration of the lead content in the Veshaw stream is shown in Figure 4. One striking difference found in the lead concentration was that the cadmium concentration was found below the detection limit at upstream (Kongwattan and Aharbal) during the entire study period in all the seasons. In the winter, the middle stream (Nihama) had the lowest average value (0.004 mgL−1), and in the summer, the downstream (Sangam) had the highest average value (0.046 mgL−1). In the present study, lead was found below the detection limit upstream at Kongwattan and Aharbal during all the seasons (Figure 4). Afterward, its concentration revealed a downward trend, with the maximum mean value observed in the lower reaches of a stream (Sangam). The highest concentration was observed in the summer season, followed by the autumn season, and the lowest was observed during the winter season. The highest concentration of lead in summer and autumn could be due to less dilution owing to the low discharge of the stream. A similar trend within the river was observed by Quan et al., 2022). Increased lead content downstream may be due to the inflow of waste from garbage dumps, domestic sewage, transportation, vehicle workshops, and industrial discharge from industrial units within the catchment zone of the stream. Our findings are in agreement with the previous studies carried out by Kaur. (2012); Baralkiewicz et al. (2014); and Appiah-Adjei et al., 2019). Koul et al. (1988) also observed the lowest Pb content in spring and the highest in summer and autumn in the Himalayan lakes of Kashmir. According to Kaur (2012), Pb was added to the river Veshaw from wastes, agricultural activities, transportation activities, and motor workshop effluents.
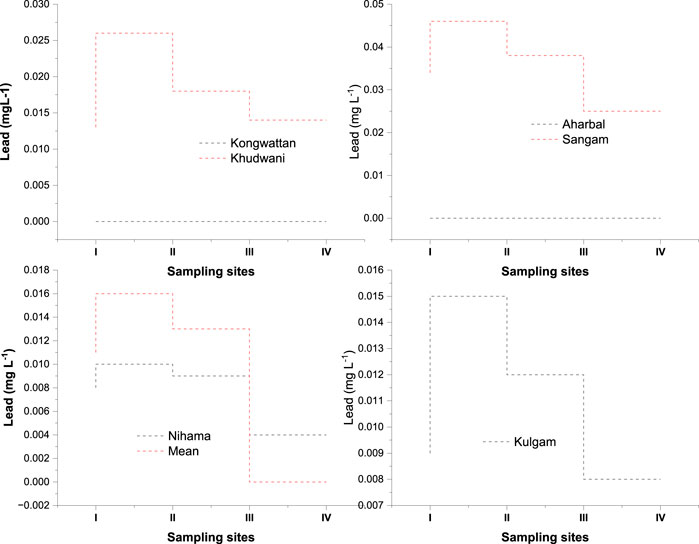
FIGURE 4. Figure depicting the comparative time-series seasonal variation analysis of Lead (Pb) in the water of the River Veshaw at six different sites collected during all the seasons from spring to winter (I:spring; II:summer; III:autumn; IV:winter; V) Each symbol represents the mean of triplicate values.
3.3 Arsenic
Arsenic (As) is the 20th most common element in the earth’s crust and is associated with igneous and sedimentary rocks. Although elemental arsenic is not soluble in water, its salts exhibit a wide range of solubilities depending on pH and the ionic environment. As can exist in both inorganic and organic forms. Arsenic (As+3) and arsenate (As+5) are inorganic forms of arsenic, whereas monomethylarsenic acid, dimethylarsenic acid, arsenobetaine, arsenocholine, arsenolipids, and arsenosugars are organic (Elci et al., 2008; Kalia & Khambholja 2015). Arsenicals are used commercially as alloying agents in the manufacture of transistors, lasers, and semiconductors, as well as in the processing of glass, pigments, textiles, paper, metal adhesives, wood preservatives, and ammunition. As concentrations in natural waters range between 1 and 2 g/L (Hindmarsh and McCurdy, 1986; Rohanifar et al., 2018). As concentrations rise in areas with volcanic rock and sulphide mineral deposits, as well as in areas with human activity (Hood and Bishop, 1972; Gibson and Gage, 1982; Hindmarsh and McCurdy, 1986; Wang et al., 2019).
Arsenic was found below the detection limit in the upper reaches of the upstream (Kongwattan and Aharbal) in all seasons. Figure 5. During the summer season, the middle stream (Nihama) had the lowest As concentration (0.005 mgL−1) and the highest (0.042 mgL−1) downstream (Sangam), followed by Khudwani (0.038 mgL−1). In the downstream, the middle stream (Nihama) had the lowest As level (0.009 mgL−1) and the downstream (Sangam) had the highest As level (0.038 mgL−1). The overall increasing downward trend was statistically significant during all the seasons. summer recorded the highest arsenic value, followed by autumn and spring, respectively. As levels in natural waters are typically between 1 and 2 mgL−1 (Hindmarsh and McCurdy, 1986), Human habitation and the presence of sulphide mineral deposits (found in volcanic rock) lead to increased concentrations of As (Hood and Bishop, 1972; Gibson and Gage, 1982; Hindmarsh and McCurdy, 1986). As has been detected at all locations except those further upstream. The amount of As found upstream (in Kongwattan and Aharbal) was higher than in other areas, while being much below the detectable limit. Data suggests that the Veshaw’s middle stream and downstream get a disproportionate share of the river’s toxic waste when compared to the river’s other catchment areas. As such, concentration has also been shown to be higher in the summer and fall than in the winter and spring. It suggests that middle and down stream area of the Jhelum river are receiving more toxic wastes as compared to the catchment area of the Veshaw river. Moreover, As concentration has been recorded more in summer and autumn compared to winter and spring seasons.
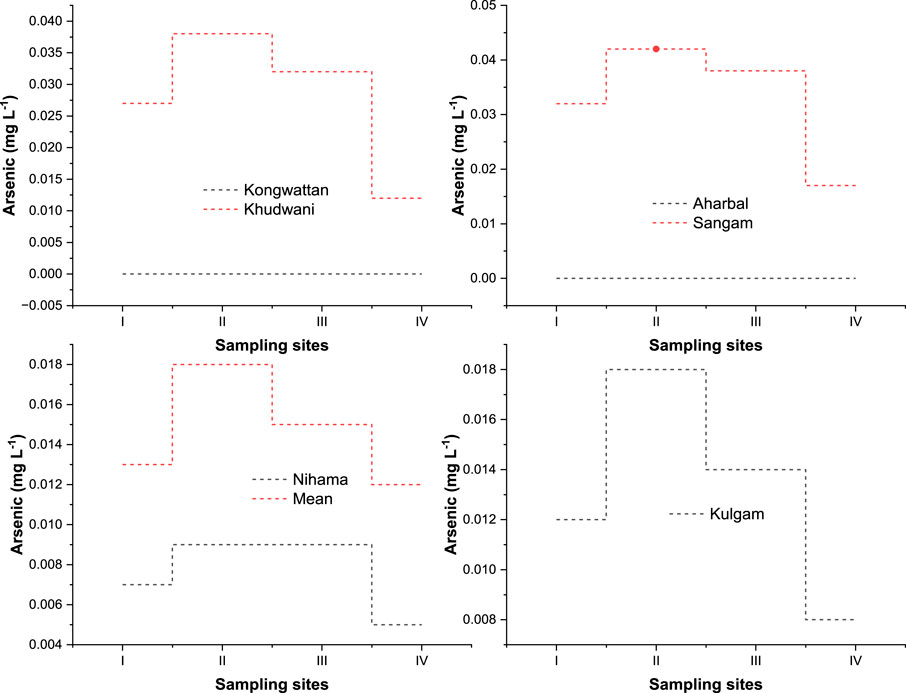
FIGURE 5. Figure depicting the comparative time-series seasonal variation analysis of Arsenic (As) in the water of the River Veshaw at six different sites collected during all the seasons from spring to winter (I:spring; II:summer; III:autumn; IV:winter; V) Each symbol represents the mean of triplicate values.
3.4 Correlation between the heavy metal parameters
To observe the relationship between heavy metal parameters during the different seasons, a correlation matrix based on the Pearson correlation coefficient (2-tailed) was employed between important parameters of water samples Figures 5A,B.
3.4.1 Pearson correlation between the heavy metal parameters
Figure 6 depicts the results of an investigation into the relationship between heavy metal water quality and cadmium, lead, and arsenic, which have all been found to have a significant positive correlation with one another. This is the degree of association among the following water quality factors. Figure 6A also shows that arsenic has the highest correlation (0.989**) with cadmium, while lead has the lowest correlation (0.872*) with cadmium. The correlation among pH, EC,WT, Turbidity, N, P, K, TH, Ca, Mg, Na, Fe, Cu, Zn, Pb, Cd and As contents in water Coliform were found statistically highly significant at (p ≤ 0.05) confidence.
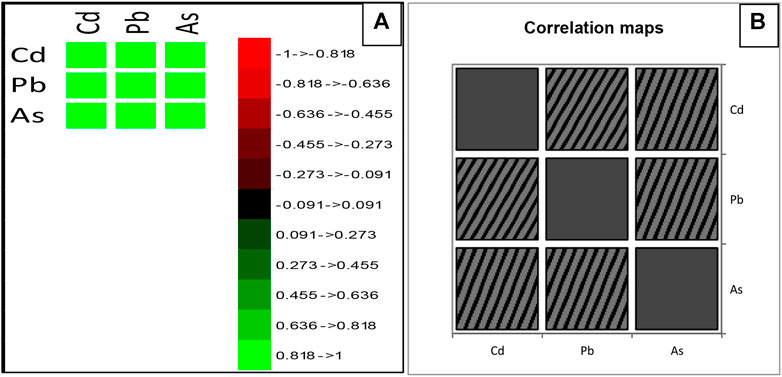
FIGURE 6. Pearson Correlation Coefficient (r) matrix of heavy metal parameters studied from Veshaw River of Kashmir Himalaya.
The regression statistics of the physicochemical data set of the Veshaw stream are summarized in Figure 7. The connection with heavy metal water quality variables was significantly equal to each other. Cadmium exhibited a similar proportionate association with Pb and As. The relative influence of the components was evaluated as follows: Cd > Pb > As. The regression findings using all station data indicated the significant water quality characteristics at each location. At each site, the water quality parameters are affected by the level of heavy metals in the Veshaw river water. Related works were performed by authors (Hong et al., 2015; Jung et al., 2016; Seo et al., 2019) who similarly spatially categorised the Nakdong River as the up-and-midstream and mid-and downstream portions at a non-weir station between Dasa (Gangjeong-Goryeong weir) and Nongong (Dalseong weir) locations using cluster analysis.
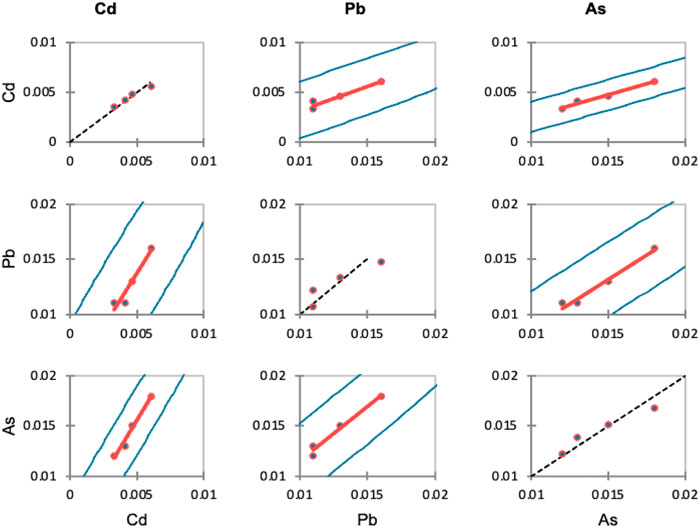
FIGURE 7. Scatter plots: linear regression model indicating the relationship among the various physicochemical parameters of the Veshaw stream.
4 Conclusion
The Veshaw River shows an increasing trend of pollution from its upper to lower reaches. The upstream water quality analysis reveals an oligsaprobic (low pollution load) nature, whereas the -mesosaprobicc nature (critical pollution load) of water in the middle and downstream reaches The results of the present study have indicated that trace elements have made their entry into the aquatic biological ecosystem and are a matter of great concern. Trace element levels in water were found to follow the trend: Sangam > Khudwani > Kulgam > Nihama > Aharbal > Kingwattan. Comparing the water quality parameters to the Indian drinking water quality standards shows that the concentrations in Khudwani and Sangam, which are near the end of the river Veshaw, are too high.
• Concerned authorities at national, state and district levels should take immediate measures (such as a complete ban on the discharge of untreated industrial effluents and municipal sewage into the river) for the restoration of the river Veshaw.
• In the area where the Veshaw river starts, there should be rules about how effluents from human activities can be made and where they can be dumped.
• Stream management activities should be prioritised in urban, rural, industrial, and agricultural areas.
Data availability statement
The original contributions presented in the study are included in the article/supplementary material, further inquiries can be directed to the corresponding authors.
Author contributions
RR, SA provided the conceptualization and Methodology; Visualization and Investigation by SA, SS; Software SP, TB; Validation and framed the manuscript by RR, SP, SM, FL, ZB performed the analysis and formatting; RR, SA, TB, and SP helped data collection data analysis; SS helped in the initial draft of the manuscript text and revised and commented on the manuscript critically.
Acknowledgments
First author is highly greatful to the Dr. Ambedkar International centre, Ministry of Social Justice and Empowerment, Govt. of India for providing financial assistance and is highly acknowledged.
Conflict of interest
The authors declare that the research was conducted in the absence of any commercial or financial relationships that could be construed as a potential conflict of interest.
Publisher’s note
All claims expressed in this article are solely those of the authors and do not necessarily represent those of their affiliated organizations, or those of the publisher, the editors and the reviewers. Any product that may be evaluated in this article, or claim that may be made by its manufacturer, is not guaranteed or endorsed by the publisher.
References
Aggarwal, T. R., Singh, K. N., and Gupta, A. K. (2000). Impact of sewage containing domestic wastes and heavy metals on the chemistry of Varuna river water. Pollut. Res. 19 (3), 491–494.
Amuah, E. E. Y., Boadu, J. A., and Nandomah, S. (2022). Emerging issues and approaches to protecting and sustaining surface and groundwater resources: Emphasis on Ghana. Groundw. Sustain. Dev. 16, 100705. doi:10.1016/j.gsd.2021.100705
APHA (2005). Standard methods for the examination of water and wastewater. Washington DC: American Public Health Association.
Appiah-Adjei, E. K., Baidu, E. E., Adjei, K. A., and Nkansah, M. A. (2019). Potential heavy metal pollution of soils from artisanal automobile workshops: The case of suame magazine, Ghana. Environ. Earth Sci. 78, 62. doi:10.1007/s12665-019-8069-7
Balali-Mood, M., Naseri, K., Tahergorabi, Z., Khazdair, M. R., and Sadeghi, M. (2021). Toxic mechanisms of five heavy metals: Mercury, lead, chromium, cadmium, and arsenic. Front. Pharmacol. 12, 643972. doi:10.3389/fphar.2021.643972
Bano, H., Lone, F. A., Bhat, J. I., Rather, R. A., Malik, S., and Bhat, M. A. (2018). Hokersar wet land of Kashmir: Its utility and factors responsible for its degradation. Plant Arch. 18, 1905–1910.
Bano, H., Malik, S., Rather, R. A., Bhat, J. I., Islam, S., Bhat, T. A., et al. (2022). Impact of anthropogenic activities on physico-chemical properties of sediment of hokersar wetland: A protected wildlife reserve (ramsar site No. 1570) of Kashmir Himalaya. Bangladesh J. Bot. 51 (1), 83–92. doi:10.3329/bjb.v51i1.58824
Baralkiewicz, D., Chudzinska, M., Szpakowska, B., Swierk, D., Goldyn, R., and Dondajewska, R. (2014). Storm water contamination and its effect on the quality of urban surface waters. Environ. Monit. Assess. 186, 6789–6803. doi:10.1007/s10661-014-3889-0
Bhat, S. A., Bashir, O., Haq, S. A. U., Amin, T., Rafiq, A., Ali, M., et al. (2022). Phytoremediation of heavy metals in soil and water: An eco-friendly, sustainable and multidisciplinary approach. Chemosphere 303, 134788. doi:10.1016/j.chemosphere.2022.134788
Bhat, S. A., Singh, P., Jogi, K. S., Rather, R. A., and Thenua, O. V. S. (2011). Influence of biofertilizers on yield and phosphorus uptake at various levels of phosphorus in mungbean(vigna radiate L. Wilczek.). Environ. Ecol. 29, 1.
Buragohain, M., Bhuyan, B., and Sarma, H. P. (2009). Seasonal variations of lead, arsenic, cadmium and aluminium contamination of groundwater in Dhemaji district, Assam, India. Environ. Monit. Assess. 170, 345–351. doi:10.1007/s10661-009-1237-6
Buragohain, M., Bhuyan, B., and Sarma, H. P. (2010). Seasonal variations of lead, arsenic, cadmium and aluminium contamination of groundwater in Dhemaji district, Assam, India. Environ. Monit. Assess. 170, 345–351.
Cooper, V. A., Solbe, J. F., and De, L. G. (1978). Report on fish populations and water quality of the river tean staffs. London: Water Research Centre.
Cortecci, G., Boschetti, T., Dinellim, E., Cidu, R., Podda, F., and Doveri, M. (2009). Geochemistry of trace elements in surface waters of the Arno River Basin, northern Tuscany, Italy. Appl. Geochem. 24, 1005–1022. doi:10.1016/j.apgeochem.2009.03.002
Damian, E. C., Obinwanne, O. C., and Obinna, E. C. (2019). Ecological assessment for trace metal pollution in a freshwater ecosystem. Int. J. Ecotoxicol. Ecobiol. 4 (3), 73–80.
Elci, L., Divrikli, U., and Soylak, M. (2008). Inorganic arsenic speciation in various water samples with GF-AAS using co-precipitation. Int. J. Environ. Anal. Chem. 88, 711–723. doi:10.1080/03067310802094984
Ekmekyapar, F., Sabudak, T., and Seren, G. (2012). Assessment of heavy metal contamination in soil and wheat (Triticum aestivum L.) plant around the Çorlu–Çerkezkoy highway in Thrace region. Glob. Nest J. 14 (4), 496–504.
Emoyan, O. O., Ogban, F. E., and Akarah, E. (2005). Evaluation of heavy metalsloading of river Ijana in Ekpan-Warri, Nigeria. J. Appl. Sci. Environ. Manag. 10 (2), 121–127. doi:10.4314/jasem.v10i2.43690
Gibson, R. S., and Gage, L. A. (1982). Changes in hair arsenic levels in breast and bottle fed infants during the first year of infancy. Sci. Total Environ. 26, 33–40. doi:10.1016/0048-9697(82)90094-8
Harrison, R. M., and Laxen, D. P. H. (1981). Lead pollution Causes and Control, (Berlin/Heidelberg, Germany: Springer).
Hasan, M. N., Altaf, M. M., Khan, N. A., Khan, A. H., Khan, A. A., Ahmed, S., et al. (2021). Recent technologies for nutrient removal and recovery from wastewaters: A review. Chemosphere 277, 130328. doi:10.1016/j.chemosphere.2021.130328
He, B., Wang, W., Geng, R., Ding, Z., Luo, D., Qiu, J., et al. (2021). Exploring the fate of heavy metals from mining and smelting activities in soil-crop system in Baiyin, NW China. Ecotoxicol. Environ. Saf. 207, 111234. doi:10.1016/j.ecoenv.2020.111234
Hindmarsh, J. T., McCurdy, R. F., and Savory, J. (1986). Clinical and environmental aspects of arsenic toxicity. CRC Crit. Rev. Clin. Lab. Sci. 23, 315–347. doi:10.3109/10408368609167122
Hong, S., Do, Y., Kim, J. Y., Kim, D. K., and Joo, G. J. (2015). Distribution, spread and habitat preferences of nutria (Myocastor coypus) invading the lower Nakdong River, South Korea. Biol. Invasions 17, 1485–1496. doi:10.1007/s10530-014-0809-8
Hood, R. D., and Bishop, S. L. (1972). Teratogenic effects of sodium arsenate in mice. Archives Environ. Health Int. J. 24, 62–65. doi:10.1080/00039896.1972.10666051
Husain Khan, A., Abdul Aziz, H., Khan, N. A., Ahmed, S., Mehtab, M. S., Vambol, S., et al. (2020). Pharmaceuticals of emerging concern in hospital wastewater: Removal of ibuprofen and ofloxacin drugs using MBBR method. Int. J. Environ. Anal. Chem. 2020, 1–15. doi:10.1080/03067319.2020.1855333
Jung, K. Y., Ahn, J. M., Kim, K., Lee, I. J., and Yang, D. S. (2016). Evaluation of water quality characteristics and water quality improvement grade classification of Geumho River tributaries. J. Environ. Sci. Int. 25, 767–787. doi:10.5322/jesi.2016.25.6.767
Kalia, K., and Khambholja, D. B. (2015). “Arsenic contents and its biotransformation in the marine environment,” in Handbook of arsenic toxicology (Academic Press), 675–700.
Kanwar, V. S., Sharma, A., Srivastav, A. L., and Rani, L. (2020). ;Phytoremediation of toxic metals present in soil and water environment: A critical review. Environ. Sci. Pollut. Res. 27 (36, 44835–44860.
Kar, D., Surr, P., Mandal, S. K., Saha, T., and Kole, R. K. (2008). Assessment ofheavy metal pollution in surface water. Int. J. Environ. Sci. Technol. (Tehran). 5 (1), 119–124. doi:10.1007/bf03326004
Karmakar, B., Singh, M. K., Choudhary, B. K., Singh, S. K., Egbueri, J. C., Gautam, S. K., et al. (2021). Investigation of the hydrogeochemistry, groundwater quality, and associated health risks in industrialized regions of Tripura, northeast India. Environ. Forensics 2021, 1–22. doi:10.1080/15275922.2021.2006363
Kaur, S. (2012). Assessment of heavy metals in summer and winter seasons in river Yamuna segment flowing through Delhi, India. J. Environ. Ecol. 3 (1), 149–165.
Kaushik, A., Kansal, A., Santosh, M., Kumari, S., and Kaushik, C. P. (2009). Heavy metal contamination of river Yamuna, Haryana, India: Assessment by metal enrichment factor of the sediments. J. Hazard. Mater. 164 (1), 265–270. doi:10.1016/j.jhazmat.2008.08.031
Khan, A. H., Aziz, H. A., Khan, N. A., Dhingra, A., Ahmed, S., and Naushad, M. (2021). Effect of seasonal variation on the occurrences of high-risk pharmaceutical in drain-laden surface water: A risk analysis of Yamuna river. Sci. Total Environ. 794, 148484. doi:10.1016/j.scitotenv.2021.148484
Khan, A. H., Khan, N. A., Ahmed, S., Dhingra, A., Singh, C. P., Khan, S. U., et al. (2020b). Application of advanced oxidation processes followed by different treatment technologies for hospital wastewater treatment. J. Clean. Prod. 269, 122411. doi:10.1016/j.jclepro.2020.122411
Khan, A. H., Khan, N. A., Zubair, M., Shaida, M. A., Manzar, M. S., Abutaleb, A., et al. (2022). Sustainable green nanoadsorbents for remediation of pharmaceuticals from water and wastewater: A critical review. Environ. Res. 204, 112243. doi:10.1016/j.envres.2021.112243
Khan, N. A., Ahmed, S., Farooqi, I. H., Ali, I., Vambol, V., Changani, F., et al. (2020a). Occurrence, sources and conventional treatment techniques for various antibiotics present in hospital wastewaters: A critical review. TrAC Trends Anal. Chem. 129, 115921. doi:10.1016/j.trac.2020.115921
Koul, V. K., Zutshi, D. P., and Dubey, K. P. (1988). Trace metal concentrations in some Kashmir Himalayan lakes. Water Air Soil Pollut. 39, 237–245. doi:10.1007/bf00279471
Kumar, S., Rahman, M. A., Islam, M. R., Hashem, M. A., and Rahman, M. M. (2022). Lead and other elements-based pollution in soil, crops and water near a lead-acid battery recycling factory in Bangladesh. Chemosphere 290, 133288. doi:10.1016/j.chemosphere.2021.133288
Lohani, M., Singh, A., Rupainwar, D. C., and Dhar, D. N. (2008). Seasonal variations of heavy metal contamination in river Gomti of Lucknow city region. Environ. Monit. Assess. 147, 253–263. doi:10.1007/s10661-007-0117-1
Lokhande, R. S., and Sathe, C. N. (2001). Monitoring and assessment of heavy metal contents in the industrial effluents from Ambarnath area (Maharashtra). Pollut. Res. 20 (2), 239–243.
MacFarlane, G. R., and Burchett, M. D. (2000). Cellular distribution of copper, lead and zinc in the grey mangrove, Avicennia marina (Forsk.) Vierh, SAquat. Bot. 68 (1)), 45–59.
Madhav, S., Ahamad, A., Singh, A. K., Kushawaha, J., Chauhan, J. S., Sharma, S., et al. (2020). “Water pollutants: Sources and impact on the environment and human health,” in Sensors in water pollutants monitoring: Role of material (Spinger), 43–62.
Malunguja, G. K., Thakur, B., and Devi, A. (2022). Heavy metal contamination of forest soils by vehicular emissions: Ecological risks and effects on tree productivity, Environmental Processes 9 (1), 1–33.
Mazhar, M. A., Khan, N. A., Ahmed, S., Khan, A. H., Hussain, A., Changani, F., et al. (2020). Chlorination disinfection by-products in municipal drinking water–a review. J. Clean. Prod. 273, 123159. doi:10.1016/j.jclepro.2020.123159
Mondol, M. N., Chamon, A. S., Faiz, B., and Elahi, S. F. (2011). Seasonal variation of heavy metal concentrations in water and plant samples around tejgaon industrial area of Bangladesh. J. Bangladesh Acad. Sci. 35 (1), 19–41. doi:10.3329/jbas.v35i1.7968
Natasha, N., Shahid, M., Khalid, S., Bibi, I., Naeem, M. A., Niazi, N. K., et al. (2022). Influence of biochar on trace element uptake, toxicity and detoxification in plants and associated health risks: A critical review. Crit. Rev. Environ. Sci. Technol. 52 (16), 2803–2843. doi:10.1080/10643389.2021.1894064
Nouri, J., Mahvi, A. H., Babaei, A. A., Jahed, G. R., and Ahmadpour, E. (2006). Investigation of heavy metals in groundwater. Pak. J. Biol. Sci. 9 (3), 377–384.
Ohiagu, F. O., Lele, K. C., Chikezie, P. C., Verla, A. W., and Enyoh, C. E. (2020). Bioaccumulation and health risk assessment of heavy metals in Musa paradisiaca, Zea mays, Cucumeropsis manii and Manihot esculenta cultivated in Onne, Rivers State, Nigeria. Environ. Anal. Health Toxicol. 35 (2), e2020011. doi:10.5620/eaht.e2020011
Padder, S. A., Mansoor, S., Bhat, S. A., Baba, T. A., Rather, R. A., Wani, S. M., et al. (2021). Bacterial endophyte community dynamics in apple (malus domestica borkh.) germplasm and their evaluation for scab management strategies. J. Fungi (Basel). 7 (11), 923. doi:10.3390/jof7110923
Padder, S. A., Rather, R. A., Bhat, S. A., Shah, M. D., Baba, T. R., and Mubarak, N. M. (2022). Dynamics, phylogeny and phyto-stimulating potential of chitinase synthesizing bacterial root endosymbiosiome of North Western Himalayan Brassica rapa L. Sci. Rep. 12 (1), 6742–6819. doi:10.1038/s41598-022-11030-0
Patel, P., Raju, N. J., Reddy, B. C., Suresh, U., Sankar, D. B., and Reddy, T. V. K. (2018). Heavy metal contamination in river water and sediments of the swarnamukhi river basin, India: Risk assessment and environmental implications. Environ. Geochem. Health 40 (2), 609–623. doi:10.1007/s10653-017-0006-7
Paul, A. C., Parameswaran, M., and Pillai, K. C. (1994). Trace metals and lanthanides in a tropical river environment. Water Air Soil Pollut. 74, 141–153. doi:10.1007/bf01257152
Pekey, H. (2006). The distribution and sources of heavy metals in Izmit Bay surface sediments affected by a polluted stream. Mar. Pollut. Bull. 52 (10), 1197–1208.
Penido, E. S., Martins, G. C., Mendes, T. B. M., Melo, L. C. A., do Rosário Guimarães, I., and Guilherme, L. R. G. (2019). Combining biochar and sewage sludge for immobilization of heavy metals in mining soils. Ecotoxicol. Environ. Saf. 172, 326–333. doi:10.1016/j.ecoenv.2019.01.110
Prieto, M. J., Acevedo, S. O. A., Prieto, G. F., and González, N. T. (2018). Phytoremediation of soils contaminated with heavy metals. Biodivers. Int. J. 2, 362–376. doi:10.15406/bij.2018.02.00088
Priyadarshanee, M., Mahto, U., and Das, S. (2022). “Mechanism of toxicity and adverse health effects of environmental pollutants,” in Microbial biodegradation and bioremediation (Elsevier), 33–53.
Quan, J., Xu, Y., Ma, T., Wilson, J. P., Zhao, N., and Ni, Y. (2022). Improving surface water quality of the Yellow River Basin due to anthropogenic changes. Sci. Total Environ. 836, 155607. doi:10.1016/j.scitotenv.2022.155607
Rahimpoor, R., Rostami, M., Assari, M. J., Mirzaei, A., and Zare, M. R. (2020). Evaluation of blood lead levels and their effects on hematological parameters and renal function in iranian lead mine workers. Health Scope 9 (4), 95917. doi:10.5812/jhealthscope.95917
Ram, P., and Singh, A. K. (2007). Studies on distribution of heavy metals in Ganga water and its bed sediments along the Patna stretch. J. Environ. Health Sci. Eng. 49 (3), 211–214.
Rashmi, I., Roy, T., Kartika, K. S., Pal, R., Coumar, V., Kala, S., et al. (2020). “Organic and inorganic fertilizer contaminants in agriculture: Impact on soil and water resources,” in Contaminants in agriculture (Cham: Springer), 3–41.
Rather, R. A., Wani, A. W., Mumtaz, S., Padder, S. A., Khan, A. H., Almohana, A. I., et al. (2022d). Bioenergy: A foundation to environmental sustainability in a changing global climate scenario. J. King Saud Univ. - Sci. 34 (1), 101734. doi:10.1016/j.jksus.2021.101734
Rather, R. A., Bano, H., Firoz, A., Mohammed, A. H., Bhat, M. A., Padder, S. A., et al. (2022c). The assessment of morphological diversity of Colchicum luteum L., an economically important threatened medicinal plant of Kashmir Himalaya. Sustainability 14 (3), 1327. doi:10.3390/su14031327
Rather, R. A., Bano, H., Padder, S. A., Baba, T. R., Ara, S., Lone, F. A., et al. (2022a). Impact of anthropogenic pressure on physico-chemical characteristics of forest soils of Kashmir Himalaya. Bull. Environ. Contam. Toxicol. 108, 1088–1097. doi:10.1007/s00128-022-03458-x
Rather, R. A., Bano, H., Padder, S. A., Perveen, K., Masoudi, L. Al., Alam, S. S., et al. (2022b). Anthropogenic impacts on phytosociological features and soil microbial health of colchicum luteum L. An endangered medicinal plant of north western Himalaya. Saudi J. Biol. Sci. S1319-562X (22), 00011–20. doi:10.1016/j.sjbs.2022.01.011
Rather, R. A., Bano, H., Perveen, K., Bukhari, N. A., Padder, S. A., Baba, T. R., et al. (2022e). Antifungal potential of Colchicum luteum and determination of colchicine content using HPLC for application as a fungicide. J. King Saud Univ. - Sci. 34 (3), 101876. doi:10.1016/j.jksus.2022.101876
Rauf, A., Javed, M., Ubaidullah, M., and Abdullah, S. (2009). Assessment of heavy metals in sediments of the river Ravi, Pakistan. Int. J. Agric. Biol. 11, 197–200.
Rohanifar, A., Rodriguez, L. B., Devasurendra, A. M., Alipourasiabi, N., Anderson, J. L., and Kirchhoff, J. R. (2018). Solid-phase microextraction of heavy metals in natural water with a polypyrrole/carbon nanotube/1, 10–phenanthroline composite sorbent material. Talanta 188, 570–577. doi:10.1016/j.talanta.2018.05.100
Saif, S., and Khan, M. S. (2017). Assessment of heavy metals toxicity on plant growth promoting rhizobacteria and seedling characteristics of Pseudomonas putida SFB3 inoculated greengram. Acta Sci. Agric. 1, 47–56.
Seo, M., Cho, C., Im, T., Kim, S., Yoon, H., Kim, Y., et al. (2019). Statistical analysis of the spatio-temporal water quality characteristics of the Nakdong River. J. Environ. Sci. Int. 28, 303–320. doi:10.5322/jesi.2019.28.3.303
Shafiq-ur-Rehman, (2010). Use of domestic sewage effluent for sustainable production of vegetable in Kashmir valley. Int. J. Environ. Waste Manag. 6, 149–161. doi:10.1504/ijewm.2010.033990
Simokawa, K., Takada, H., Kato, K., Takashashi, H., Mori, H., Watanabe, N., et al. (1984). A study on pathway and trend of mercury pollution of bottom sediment in the Suimon river. Jpn. J. Water Pollut. Res. 7, 164–171.
Stewart, D. J., Scrimshire, A., Thomson, D., Bingham, P. A., and Barron, A. R. (2022). The chemical suitability for recycling of zinc contaminated steelmaking by-product dusts: The case of the UK steel plant. Resour. Conservation Recycl. Adv. 14, 200073. doi:10.1016/j.rcradv.2022.200073
Storto, D., Nara, L. B. C., Kozusny-Andreani, D. I., Vanzela, L. S., Mansano, C. F. M., Bilal, M., et al. (2021). Seasonal dynamics of microbial contamination and antibiotic resistance in the water at the Tietê Ecological Park, Brazil. Water Air Soil Pollut. 232 (7), 257–318. doi:10.1007/s11270-021-05207-y
Strehlow, C. D., and Barltrop, D. (1987). Temporal trends in urban and rural blood lead concentrations. Environ. Geochem. Health 9, 74–79. doi:10.1007/bf02057279
Syres, J. K., Mackay, A. D., Brown, M. W., and Curie, L. D. (1986). Chemical andphysical characteristics of phosphate rock material of varying reactivity. J. Sci. Food Agric. 37, 1057–1064.
Tam, Y. S., and Elefsiniotis, P. (2009). Corrosion control in water supply systems: Effect of pH, alkalinity, and orthophosphate on lead and copper leaching from brass plumbing. J. Environ. Sci. Health Part A 44 (12), 1251–1260. doi:10.1080/10934520903140009
Tauqeer, H. M., Turan, V., and Iqbal, M. (2022). “Production of safer vegetables from heavy metals contaminated soils: The current situation, concerns associated with human health and novel management strategies,” in Advances in bioremediation and phytoremediation for sustainable soil management (Cham: Springer), 301–312.
Teixeira de Souza, A., Carneiro, L. A. T., da Silva Junior, O. P., de Carvalho, S. L., and Américo-Pinheiro, J. H. P. (2021). Assessment of water quality using principal component analysis: A case study of the marrecas stream basin in Brazil. Environ. Technol. 42 (27), 4286–4295. doi:10.1080/09593330.2020.1754922
Tiwari, A. K., Singh, P. K., Singh, A. K., and De Maio, M. (2016). Estimation of heavy metal contamination in groundwater and development of a heavy metal pollution index by using GIS technique. Bull Environ. Contam. Toxicol. 96 (4), 508–515.
Turer, D. G., and Maynard, B. J. (2003). Heavy metal contamination in highway soils. Comparison of Corpus Christi, Texas and Cincinnati, Ohio shows organic matter is key to mobility. Clean Technol. Environ. Policy 4 (4), 235–245.
Vardhan, K. H., Kumar, P. S., and Panda, R. C. (2019). A review on heavy metal pollution, toxicity and remedial measures: Current trends and future perspectives. J. Mol. Liq. 290, 111197. doi:10.1016/j.molliq.2019.111197
Wang, Y. Y., Chai, L. Y., and Yang, W. C. (2019). “Arsenic distribution and pollution characteristics,” in Arsenic pollution control in nonferrous metallurgy (Singapore: Springer), 1–15.
Yang, Z., Wang, Y., Shen, Z. H., Niu, J., and Tang, Z. H. (2009). Distribution and speciation of heavy metals in sediments from the mainstream, tributaries and lakes of the Yangtze River catchment of Wuhan, China. J. Hazard. Mater. 166, 1186–1194. doi:10.1016/j.jhazmat.2008.12.034
Keywords: seasonal changes, heavy metal, cadmium, lead, arsenic, himalaya
Citation: Rather RA, Ara S, Sharma S, Padder SA, Lone FA, Mir SA, Baba ZA, Ayoub IB, Mir IA, Bhat TA and Baba TR (2022) Seasonal changes and determination of heavy metal concentrations in Veshaw river of the Indian western Himalaya. Front. Environ. Chem. 3:1018576. doi: 10.3389/fenvc.2022.1018576
Received: 13 August 2022; Accepted: 15 September 2022;
Published: 10 October 2022.
Edited by:
Afzal Husain Khan, Jazan University, Saudi ArabiaReviewed by:
Juliana Heloisa Pinê Américo-Pinheiro, Brazil University, BrazilNahid Pervez, University of Salerno, Italy
Copyright © 2022 Rather, Ara, Sharma, Padder, Lone, Mir, Baba, Ayoub, Mir, Bhat and Baba. This is an open-access article distributed under the terms of the Creative Commons Attribution License (CC BY). The use, distribution or reproduction in other forums is permitted, provided the original author(s) and the copyright owner(s) are credited and that the original publication in this journal is cited, in accordance with accepted academic practice. No use, distribution or reproduction is permitted which does not comply with these terms.
*Correspondence: Rauoof Ahmad Rather, cm91Zi5oYXFAZ21haWwuY29t; Shahid Ahmad Padder, c2hhaGlkcGFkZGVyQHNrdWFzdGthc2htaXIuYWMuaW4=; Tawseef Rehman Baba, dGF3c2VlZnJlaG1hbjI3QGdtYWlsLmNvbQ==