- 1Université de Pau et des Pays de l’Adour, E2S UPPA, CNRS, IPREM, Institut des Sciences Analytiques et de Physico-chimie pour l’Environnement et les matériaux, Pau, France
- 2Institute of Biodiversity and Environment (BIOMA), University of Navarra, Pamplona, Navarra, Spain
- 3Université de Pau et des Pays de l’Adour, E2S UPPA, Univ. Bordeaux, Univ Bordeaux Montaigne, ENSAP Bordeaux, CNRS, Pau, France
- 4Department of Analytical Chemistry, University of the Basque Country, Bilbao, Spain
Mercury (Hg) and lead (Pb) isotopic compositions were investigated in mosses and lichens collected in a large mountainous beech forest (Iraty Forest) located on the French-Spanish Pyrenean border. Hg isotopic signature in topsoil samples were also analyzed in selected sampling sites. This is the first work that uses the complementary information of both isotopic systems in two distinct atmospheric bioaccumulators. Mosses and lichens present characteristic accumulation due to their integration times, displaying different information on metal pollution over the area. Hg and Pb concentrations in annual moss shoots represent recent atmospheric accumulation, while whole lichen thalli integrates a process of accumulation over a longer period. Lead isotope ratios in mosses are consistent with reported data corresponding to the actual European atmospheric background (206Pb/207Pb ∼ 1.158), while Hg isotopic composition reflects potential uptake of both dry and wet Hg depositions. For lichens, Pb isotopic composition exhibits the contribution of a longer integration period of both industrial Pb emissions and legacy of leaded gasoline pollution. Hg isotopes in lichens discriminate two main groups: a larger one representing the background atmospheric contribution and a second one corresponding to unexpected higher Hg content. The similarities in odd and even Mass-independent fractionation of Hg isotopes between topsoils and lichens from the larger group, support the idea that foliage uptake is the main input of Hg in soils. The second group of lichens exhibits more negative δ202Hg (down to –4.69‰) suggesting a new source of fractionation in this area, probably related to lichens aging and/or stubble and grass fires due to pastoral activities. This study demonstrates that using both Hg and Pb isotopic signature in lichens and mosses allows to trace atmospheric sources and environmental pathways of these metals in forested ecosystems. This original data set in a remote environment provides also new information on the fate of atmospheric Pb and Hg depositions.
1 Introduction
Lichens and mosses present excellent characteristics when monitoring air pollution by metals (Bargagli, 2016). The two biomonitors have neither cuticles nor roots, which means that the influence of substratum is negligible, and that the metal content of their tissues is supposed to originate exclusively from atmospheric deposition. Several recent studies have evaluated mosses and lichens as biomonitors of metal pollution in forest ecosystems and remote areas (Barre et al., 2013; Barre et al., 2015; Agnan et al., 2017; Fabri et al., 2018; Kłos et al., 2018; Ratier et al., 2018; Panichev et al., 2019; Shotyk and Cuss, 2019; Klapstein et al., 2020). These studies suggest that both bioaccumulators provide complementary information depending on their differences in trace elements uptake and integration times (Bargagli et al., 2002; Szczepaniak, 2003; Giordano et al., 2013). Since the development of analytical tools giving precise and accurate isotopic measurements, the analysis of mercury and lead stable isotopes provide information about pollution sources and environmental pathways of the elements (Lauretta et al., 2001; Hintelmann and Lu, 2003; Gratz et al., 2010; Perrot et al., 2010; Sherman et al., 2012; Barre et al., 2018; Wang et al., 2019).
Mercury (Hg) has seven stable isotopes (196, 198, 199, 200, 201, 202 and 204), which undergo mass dependent and mass independent fractionation (MDF and MIF) processes during their transformations or transfer between different reservoirs. Hg isotopic signature is commonly modified in the atmosphere and by the uptake by vegetation and deposition in soil. Several studies have employed Hg isotopes in lichens and mosses to discriminate between atmospheric pollution sources and environmental pathways (Carignan et al., 2009; Estrade et al., 2010; Estrade et al., 2011; Blum et al., 2012; Barre et al., 2013; Enrico et al., 2016; Barre et al., 2018). MDF is commonly reported with δ202/198Hg notation and can occur during biogeochemical transformations (Bergquist and Blum, 2009). Hg uptake in remote areas undergoes MDF generating lighter isotopic composition in plant leaves (Demers et al., 2013; Enrico et al., 2016; Yu et al., 2016). Odd MIF, reported as Δ199Hg and Δ201Hg, is a more complex mechanism and it is mainly associated with photochemical reduction of Hg(II) (Bergquist and Blum, 2009; Carignan et al., 2009; Wiederhold et al., 2010). In the atmosphere, both particulate bound mercury (PBM) and gaseous oxidized mercury (GOM) have more positive MIF compared to gaseous elemental mercury (GEM), which usually displays negative Δ199Hg (Gratz et al., 2010; Demers et al., 2013; Rolison et al., 2013; Fu et al., 2016a; Zheng et al., 2016).
Isotopic anomalies corresponding to MIF were also observed in several studies for even isotopes (Δ200Hg and Δ204Hg) in atmospheric samples (Gratz et al., 2010; Rolison et al., 2013; Sherman et al., 2013; Enrico et al., 2016; Sun et al., 2016). Although there is not a definitive explanation, the different studies suggest that processes involved in even MIF take place in the tropopause due to redox gas phase reactions (Gratz et al., 2010; Chen et al., 2012; Demers et al., 2013). These processes are supposed to be exclusive of the atmosphere and not related to transformations in the Earth’s surface or carried out by biota (Blum et al., 2014). Thus, even-MIF signature can trace the pathways of Hg atmospheric deposition to terrestrial ecosystems (Demers et al., 2013; Enrico et al., 2016; Wang et al., 2019; Fu et al., 2016b). In forested ecosystems, differences in MIF of Hg isotopes depend on the nature and pathway of Hg deposition, and are clear between atmospheric oxidized mercury forms (PBM/GOM) and GEM that may accumulate mainly in vegetation (plants and epiphytes) (Biswas et al., 2008; Demers et al., 2013; Enrico et al., 2016). Deposited oxidized Hg(II) shows positive values of even and odd isotopes, while Hg0 from dry deposition presents negligible and negative even and odd MIF, respectively. The common conclusion between several studies is that forest ecosystems are the largest sink of atmospheric Hg0 due to its accumulation in foliage and subsequent deposition in soils as litter (Demers et al., 2013; Jiskra et al., 2015; Enrico et al., 2016; Zheng et al., 2016; Obrist et al., 2017; Wang et al., 2017; Olson et al., 2019). On the other hand, mountainous ecosystems in the Pyrenees could be representative of the free troposphere, so atmospheric samples collected at high altitudes can be good indicators of Hg deposition pathways through even MIF evaluation (Fu et al., 2016a).
Lead is present in nature in four main isotopes: three radiogenic isotopes 208Pb (52%), 206Pb (24%), 207Pb (23%) (which are products of radioactive decay of 232Th, 235U and 238U, respectively), and 204Pb which is the only non-radiogenic isotope. Lead (Pb) isotopes are widely used to trace atmospheric pollution in the environment (Monna et al., 1997; Monna et al., 2011; Carignan et al., 2002; Carignan et al., 2005; Cloquet et al., 2006; Veschambre et al., 2008). The differences in the isotopic abundances allow to discriminate Pb between different sources (Stacey and Kramers, 1975; Spiro et al., 2004). Pb can be emitted into the atmosphere by high temperature anthropogenic processes and transported to remote areas (Gross et al., 2012). Lead isotopes are not significantly fractionated by physicochemical processes, and they reflect the composition of the ore of lead, however different reservoirs on Earth represent a mixture of lead from different sources. Thus, Pb isotopic composition is quite well known and allows to trace the source of Pb. In forest ecosystems, Pb results from the contribution of natural and anthropogenic sources, although atmospheric deposition is recognized as the main input of forest Pb (Zhou et al., 2019). Since the ban of leaded gasoline (major source of Pb in the 60–70 s), European atmospheric signature of Pb is representative of the metallurgical industrial emissions (Cloquet et al., 2006). In the particular case of using lichens as biomonitors, Pb isotopic analysis allows to identify several anthropogenic sources (Carignan and Gariépy, 1995; Cloquet et al., 2006; Dolgopolova et al., 2006; Monna et al., 2011; Barre et al., 2018).
This study reports for the first time the combination of Hg and Pb isotopic systems in mosses, lichens and soils collected at different altitudes in the French (North) and Spanish (South) slopes of the Iraty Forest (western Pyrenees), one of the largest beech forests in the south of Europe. This mountainous ecosystem is impacted by local anthropogenic sources (road traffic, local industrial emissions, agricultural and livestock activities), but also by the long-range atmospheric transport (Moldovan et al., 2007; Veschambre et al., 2008; Ezcurra et al., 2013; Agnan et al., 2015; Corella et al., 2017). The objective of the study is to compare the isotopic signature of Hg and Pb measured in both biomonitors and to differentiate the spatial information provided at different time scale. Hg and Pb isotopic signatures are complementary since Hg is able to trace the atmospheric processes, while Pb isotopic information is related to the elemental source. For the two atmospheric biomonitors, isotopic signatures demonstrate that the integration time is critical to evaluate main sources of Pb and deposition pathways of Hg. As it was previously demonstrated (Barre et al., 2018), the employment of two isotopic systems (stable and radiogenic isotopes) enhance the understanding of atmospheric and deposition pathways of the pollutants. Therefore, this work has an important implication for future research conducted on the use of such biomonitors to set up atmospheric monitoring programs at large scale.
Materials and Methods
Sampling Site
The study was performed for biomonitors and topsoils collected in one of the largest beech forests in the south of Europe: the Iraty forest. This forest is located on the border between France and Spain, one part belonging to the Spanish province of Navarra and the other to the French Basque Country, with a total surface area of 17,300 ha. The Iraty forest is located in a mountainous area far from urban and industrial regions, whose main activity is forestry and animal breeding in pastures (e.g., cows, sheep and horses). The Iraty forest receives major inputs from free tropospheric air mass circulation (Ezcurra et al., 2013), being considered a suitable monitoring site for long-range transport of air pollutants (i.e., monitoring of European background pollution). In the study, 51 sampling sites were selected in an area of 30 × 30 km2 in the center of the Iraty forest (Supplementary Figure S1). The sampling region was defined according to the extension of the Iraty forest and considering that each sampling site should have the following requirements: i) located within a beech wood, ii) at least 100 m away from any kind of road or building to avoid direct contamination effects, and iii) including a significant population of moss or lichen species. More details about the procedure of selection and design of the sampling area and sampling sites are described in Bustamante Alonso (2014). From these sampling sites, 30 were selected on the Spanish side of the border and 21 on the French side. On the Spanish side, the sampling area covers all the forest and altitudes range from 800 to more than 1,400 m. The difference in altitude in the areas of the French side is larger, ranging from 400 up to 1,400 m. In order to obtain the best representation of the study area, the location of the sampling points was determined according to the elevation and sorted in different ranges: below 400, 400–800, 800–1,000, 1,000–1,200 and above 1,200 m. In the Iraty area, highest sampling points (>1,200 m a.s.l.) were characterized as sites where free tropospheric air masses circulate, while downhill sampling sites were connected to local air masses circulation areas (Ezcurra et al., 2013).
Sample Collection and Preparation
The sampling campaign was performed during fall 2011 (three consecutive days in November). Seasonal effect on sampling for Pb and Hg was also tested in a previous study (Barre et al., 2018), and the conclusion was that it is negligible for remote locations and especially for Hg. The sampling strategy for lichens and mosses was similar to the one performed in other study for geographically close areas (Barre et al., 2013). Therefore, the collection consisted in samples of lichens (n = 30), mosses (n = 21), and topsoils only for several selected sites (n = 23). Samples were collected with gloves and stainless-steel tools and immediately packed in zip-lock PE bags and placed into a freezer (–20°C) to transport them to the laboratory.
The epiphytic lichens were collected at a height of 1–2 m on the tree bark, according to the French norm NF X903–43 (AFNOR, 2008). Three ubiquitous and very similar foliose lichens were sampled: Parmelia caperata (L.) Ach., Parmelia sulcata Taylor and Hypogymnia physodes (L.) Nyl. These lichens taken on the tree barks represent an integration period of up to a few years (Walther et al., 1990; Szczepaniak and Biziuk, 2003; Bačkor and Loppi, 2009). Lichens were separated by hand from their substratum and ground in a mixer mill (MM200, Retsch) in Teflon bowls, using to that end balls of the same material. The homogenized powder was transferred into glass vials and stored at −18°C before mineralization. About 0.5–1 g of sample was digested in a High Pressure Asher (HPA-S, Anton Paar) with 5 ml of sub-boiled nitric acid for 3 h at 300°C and 130 bar, according to the protocol published in Barre et al., 2018 and Estrade et al., 2009. For mosses, the pleurocarpous species Hypnum cupressiforme Hedw. was collected in soils and tree stumps. This species is fairly tolerant to air pollution and has been widely used in biomonitoring surveys (González-Miqueo et al., 2009; Harmens et al., 2010; Harmens et al., 2013; Schröder et al., 2013). Samples were taken following the guidelines of the UNECE ICP Vegetation Moss Manual (Vegetation Coordination Center, 2010) avoiding places close to roads, populated areas or sites under the influence of tree canopies when possible. Due to its structure forming dense mats of interwoven filaments, Hypnum cupressiforme can be easily confused with other moss species. For this reason all the samples collected were identified and separated at the laboratory of the University of Navarra (Spain) with a long experience in the use of this species for similar surveys (González-Miqueo et al., 2009; González-Miqueo et al., 2010; Izquieta-Rojano et al., 2018). Moss samples were oven-dried at 40°C for 72 h and the extreme apices (3 cm) of mosses were cut and ground. Sample digestion applied on mosses is the same as previously detailed for lichens. Surface organic forest soils samples (organo-mineral horizon) were collected at the sampling site after removing the top litter layer (sampling depth 1–5 cm) (Rodriguez Iruretagoiena, 2015). Surface soils (10 cm) were collected in the same area than mosses and lichens. Once in the laboratory, soils were lyophilized (150 mTorr, 52°C, 48 h) in a Cryodos 50 (Telstar, Spain) freeze-dryer. Then soils were sieved to <2 mm and different objects such as bones or stones were removed. After air dried in a fume hood during 24 h soils were ground in a planetary ball mill, and digested in a 4 ml of reverse aqua regia (HNO3:HCl, 3:1) in 50 ml polyethylene tubes at 85°C. To assess the quantitative recovery of the trace elements under this procedure, reference material IAEA 405 (sediments) were digested in the same way.
Hg and Pb Quantification Analyses
Hg concentrations in lichens, mosses and soils were measured by Atomic Absorption in an AMA-254 (LECO). About 30 mg of sample powder were added to a nickel boat for each analysis. The sample is dried for 60 s at 120°C and pyrolyzed at 750°C for 120 s under an oxygen flow. The resulting mercury in the gaseous form is amalgamated on a gold trap and subsequently released by thermal desorption toward the detector, where quantification takes place. The concentration of Pb in lichens was determined by Neb-ICP-MS (Thermo X2, ThermoFisher). Daily optimization of the ICP-MS parameters was performed by using tune solution of 1 ng g−1 of Li, Y, Tl and Ce standard. The quantification was performed by direct calibration, with internal standard (115In and 191Ir). The control of the methodological blanks allows to determine the detection limits that range from 1 to 10 ng L−1. More details on the analytical performance are presented in previous works (Barre et al., 2013; Barre et al., 2018). The QA/QC of the analytical procedures were evaluated by the analysis of two reference materials using the same procedures applied to lichens samples, BCR CRM 482 (Pseudevernia furfuracea) and IAEA 336 (E. prunastri).
Hg and Pb Isotopic Analyses
Mercury isotopes analyses were performed according to previous works (Lauretta et al., 2001; Bergquist and Blum, 2007; Gehrke et al., 2009), by using a cold vapor generation (CVG) with SnCl2 reduction, coupled to a Multicollector (MC) - ICP-MS (Nu Plasma, Nu Instrument). For samples with low concentrations of Hg, isotopic composition was determined using dual gold amalgamation technique (DGA) coupled to CVG by MC-ICP-MS (Bérail et al., 2017). This system works with two gold traps to pre-concentrate Hg and decrease the detection limits. In order to correct the instrumental mass-bias, internal standard of Tl (NIST 997, 205TL/203TL = 2.38714) and sample standard bracketing with NIST 3133 standard solution were used. All the samples and standards were measured at a concentration of 1 ng g−1 of Hg, to avoid any bias caused by differences in concentration. Mass-dependent fractionation (MDF) is reported as recommended by Bergquist and Blum (2007) relative to the NIST 3133 Hg solution as follows in Eq. 1:
where xxx is the studied isotope. Mass-independent fractionation (MIF) of Hg is reported as the difference between the theoretical value predicted by MDF of δ199Hg and δ201Hg and the measured values as Δ199Hg and Δ201Hg in ‰, according to the protocol suggested by Bergquist and Blum (2007):
where
For CVG-DGA/MC-ICP-MS, samples were analyzed at 50 pg ml−1 and NIST RM 8610 (n = 43) and BCR-482 (n = 45) were used as secondary standards with values of δ202Hg –0.57 ± 0.27‰ and –1.70 ± 0.33‰ and for Δ199Hg 0.00 ± 0.21‰ and –0.64 ± 0.15‰ and for Δ200Hg 0.02 ± 0.14‰ and 0.06 ± 0.14‰ respectively (see Supplementary Table S1). Analytical uncertainty for CVG/MC-ICP-MS was evaluated by multiple measurements of the secondary standard NIST RM 8610 (former UM-Almadén), and the certified reference material BCR-482 (lichen), with certified and consensus values for δ202Hg of –0.61 ± 0.26‰ and –1.69 ± 0.28‰, for Δ199Hg 0.00 ± 0.13‰ and –0.65 ± 0.1‰ and for Δ200Hg 0.01 ± 0.10‰ and 0.05 ± 0.09‰, respectively (uncertainties expressed as 2 SD). The comparison of long-term reproducibility for the measurement of NIST RM 8610 (former UM-Almadén) and BCR 482 (Lichen) for CVG-MC-ICP-MS and DGA/CVG-MC-ICP-MS, did not show any deviation (Table S1).
For Pb isotopic analysis, Pb was firstly pre-concentrated on ion exchange resin (Dowex 1 × 8, Acros organics 100–200 mesh) according to a previously published procedure (Manhes et al., 1980; Cloquet et al., 2006). Recoveries obtained after Pb isolation were comprised between 85 and 110% and fractionation of Pb isotopes during pre-concentration step was also controlled with NIST-981 and BCR-482 (Lichen). Pb isotopes were measured using MC-ICP-MS (Nu Instrument) coupled with a desolvator nebulizer unit (DSN-100, Nu Instrument). Analytical precision obtained for reference materials after column separation were as follows: NIST-981 (and BCR-482), 687 ppm (633 ppm) for 208Pb/204Pb, 498 ppm (307 ppm) for 207Pb/204Pb, and 380 ppm (865 ppm) for 206Pb/204Pb. In the same way as Hg isotopes, instrumental mass-bias was corrected with Tl internal standard added directly in the Pb solution (Pb/Tl = 10) and NIST-981 was analyzed every two samples for sample-standard bracketing correction.
Statistical Analysis
All the statistical calculations were performed using the software Origin (OriginLab corporation, Northampton, Massachusetts, USA). Since data set is not normally distributed for all the samples according to the results of Shapiro-Wilk test, non-parametric Kruskal-Wallis test was used at the p < 0.05 level of significance for all tests. Pearson’s correlation test was also performed to decide if the correlation between two variables was significantly different from zero or not.
Results
Pb Concentration in Bioaccumulators
The average concentrations of Pb in mosses and lichens are presented in Table 1 (the complementary raw data are shown in Supplementary Table S2). The concentrations range from 1.64 to 87.5 mg kg−1 in lichens and from 0.98 to 12.3 mg kg−1 in mosses. Generally, lichens have four times more Pb than mosses. The average value of Pb concentration for lichens in the Iraty forest (13.6 ± 12.5 mg kg−1) is consistent with the data previously published for samples of the Atlantic Pyrenees (Barre et al., 2018). Background Pb concentrations in lichens usually range from 4 to 6.4 mg kg−1 (Loppi and Pirintsos, 2003; Basile et al., 2008; Veschambre et al., 2008; Agnan et al., 2013; Barre et al., 2013). The comparison with these values shows that the concentration of Pb in lichens from the Iraty forest is two-fold higher than the previously reported background concentrations. The average Pb concentration in mosses is 3.9 ± 2.4 mg kg−1. Five of the mosses (4F, 5F, 19F, 13E and 17E), however, exhibit higher concentrations of Pb up to 12.31 mg kg−1. The average concentrations of Pb in lichens and mosses between north and south sides of the border are not statistically different, suggesting that the concentrations of Pb are representative of the whole sampling area. The Pb concentrations found in our study fall in the range of unpolluted sites reported in previous biomonitoring studies in France or Spain (Galsomiès et al., 1999; Fernández et al., 2002; Carballeira et al., 2008; González-Miqueo et al., 2010). Pb concentrations are represented in Supplementary Figure S2 (S.2.c and d) classified according to the altitudes of the sampling sites for mosses and lichens.
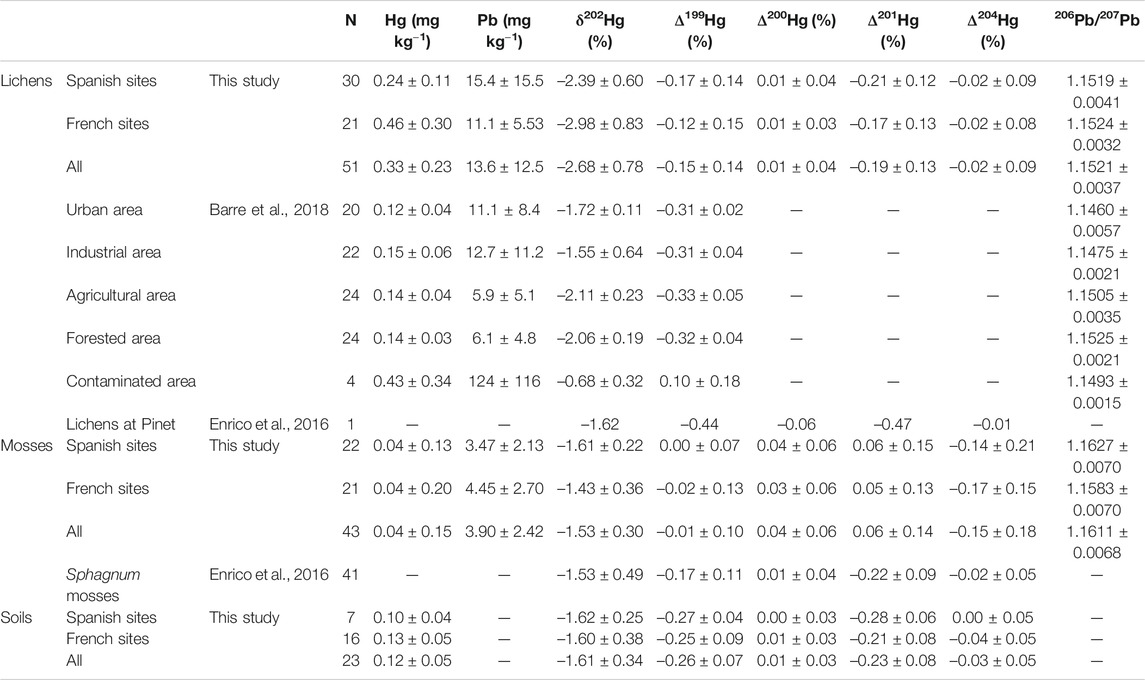
TABLE 1. Average values for concentrations, isotopic ratios and delta values of Hg and Pb for the samples of lichens, mosses and soils collected in the Iraty forest (Uncertainties are expressed as 1 SD). Results are compared with data from previous publications of samples collected in areas close to Iraty.
Hg Concentration in Bioaccumulators and Soils
The average concentrations of Hg obtained for lichens, mosses and soils are presented in Table 1 (complete raw data is shown in Supplementary Table S2). Hg concentrations in epiphytic lichens of the Iraty forest range from 0.12–1.4 mg kg−1. These values are in agreement with the average concentrations of Hg found in other French forest areas located close to the Iraty forest (0.14 ± 0.03 mg kg−1) (Barre et al., 2018). In the same region Barre and coworkers, determined Hg concentrations up to 0.85 mg kg−1 in lichens contaminated by industrial metallurgical inputs (Barre et al., 2018). According to Garty et al. (2001), areas free of pollution can reveal concentrations ranging from 0.02 to 3 mg kg−1. Some lichens sampled in Patagonia showed Hg concentrations ranging from 0.07 to 0.57 mg kg1 (Ribeiro Guevara et al., 2004). In Europe, lichens sampled in a forested ecosystem from Italy showed concentrations ranging from 0.10 to 0.22 mg kg−1 (Loppi and Pirintsos, 2003). In our work, samples from the northern slope (French sites, 0.46 ± 0.30 mg kg−1) presented Hg concentrations significantly higher than samples from the southern slope (Spanish sites, 0.24 ± 0.11 mg kg−1). The average values of both groups are statistically different (Kruskal-Wallis, H = 10.3, p = 0.013)), suggesting differences in the inputs or uptake of atmospheric Hg between lichens in both slopes of the forest. Hg concentrations in mosses are significantly lower compared to lichens ranging from 0.02 to 0.07 mg kg−1. The concentrations in mosses are not statistically different between north and south sides of the border (Kruskal-Wallis, H = 0.0997, p = 0.752 thus they are representative of the whole sampling area. For forested areas in France, Hg concentrations in mosses are between 0.03 and 0.1 mg kg−1 (Galsomiès et al., 1999). The Hg concentrations found in mosses from the Iraty forest are the characteristic ones in rural to remote areas (Barre et al., 2018). Similar concentrations were also measured in mosses from Navarra, with mean concentrations of 0.12 ± 0.12 mg kg−1 (González-Miqueo et al., 2009).
Forest soil samples were analyzed in order to compare Hg concentrations and evaluate sources in lichens and mosses. The concentrations of Hg found in the 23 soil samples (see Supplementary Table S2) are quite homogeneous within the studied area (0.052–0.244 mg kg−1), with average values of 0.12 ± 0.05 mg kg−1 (Table 1). A negative correlation (Pearson, r = –0.31 at 0.05 significance level) was found between Hg concentrations in soil and mosses collected at the same sites. The correlation can be consistent with the fact that Hg in soils mainly originate from its atmospheric uptake by vegetation and the long-term decomposition of the litter (Zheng et al., 2016; Obrist et al., 2017; Jiskra et al., 2018; Huang et al., 2020). Hg concentrations as a function of altitude are presented in Supplementary Figure S3A,B, both for mosses and lichens. Hg concentrations are statistically correlated with altitude above sea level (Pearson, r = -0.47, at 0.05 level of significance), which is in agreement with the observation that the highest Hg concentrations measured in this study were found in some lichens collected at the lowest elevation (<800 m a.s.l.).
Pb Isotopes in Lichens and Mosses
The average isotope ratios of 206Pb/207Pb for mosses and lichens are summarized in Table 1. As previously shown, isotopic ratios using the non-radiogenic 204Pb did not show better discrimination of lichen samples collected in the same region (Barre et al., 2018). Moreover, 206Pb/207Pb and 208Pb/206Pb ratios were also preferred in this report in order to be compared with previously published studies that did not measure 204Pb. The complete raw data and 208Pb/206Pb and 206Pb/207Pb ratios for lichens and mosses are presented in Supplementary Table S3. 206Pb/207Pb isotopic signature range from 1.1465 to 1.1640 in lichens and from 1.1420 to 1.1801 in mosses. Box plots diagrams for Pb isotope ratios are presented in Supplementary Figure S4 in function of the altitude. In similar way as for Pb concentrations, Pb isotopic fingerprint seems to be rather homogeneous along the whole area (see Supplementary Figure S3). The results from mosses are consistent with values reported for Pb in the mountainous ecosystem of the Alps (1.150–1.170 for 206Pb/207Pb and 2.090–2.115 208Pb/206Pb) (Schnyder et al., 2018). Mosses on sites 19E, 22E and 25E (i.e., Spanish slope) present 206Pb/207Pb values of about 1.18, suggesting a more crustal signature. These samples, located in Spanish sites for altitudes higher than 1000 m, strongly suggest crustal absorbed inputs from soil particles on their surface. Many studies have reported increased values of 206Pb/207Pb since the leaded petrol ban, because atmospheric samples have decreased their contribution and therefore their origin is closer to that of lead in rock ores (Kunert et al., 1999; Komárek et al., 2008). The isotopic signature of Pb in lichens found here is similar to that reported in previous studies conducted in 2004 in the Aspe Valley (Western Pyrenees) for atmospheric particles (206Pb/207Pb 1,152 ± 0.009) (Veschambre et al., 2008), and in peat bogs from the Basque Country (Monna et al., 2004).
Hg Isotopic Composition in Biomonitors and Soils
Lichens and Mosses
The average values for the isotopic composition of Hg in lichens and mosses are presented in Table 1. Values reported in this study are in good agreement with those found for lichens, mosses or other atmospheric biomonitors in literature (Ghosh et al., 2008; Carignan et al., 2009; Estrade et al., 2010; Blum et al., 2012; Demers et al., 2013; Yin et al., 2013; Barre et al., 2018). The values of δ202Hg measured in lichens range from –1.71 to –4.69‰. A few sampling sites of lower altitude (under 800 m), mainly located on the French side, are characterized by significant negative δ202Hg signatures, with average values of -2.98 ± 0.83‰ (as 1 SD). The odd isotopes of Hg exhibit anomalies ranging from -0.43 to 0.08‰ for the Δ199Hg. On the contrary, Δ199Hg split in two groups, one of them indistinguishable from zero, and the other one with negative MIF values. Site 4F displayed the most negative values of δ202Hg (–4.69‰) with no odd-MIF (Δ199Hg = –0.03‰). This site is located at the lowest elevation considered in this study. The results for even-MIF (Δ200Hg) suggest that in the case of lichens it is indistinguishable from zero (average 0.01 ± 0.04‰ as 1 SD). δ202Hg values in mosses are different than those found in lichens, ranging from –1.99 and –0.86‰ (–1.53 ± 0.30‰ as 1 SD), and Δ199Hg from –0.38 to +0.20‰ (–0.01 ± 0.10‰ as 1 SD). For even-MIF (Δ200Hg) in mosses, we observed larger variability, with slightly positive shift in Δ200Hg values (average 0.04 ± 0.06‰ as 1 SD). In the case of Δ204Hg signature, the measurement of isotope 204Hg was not precise enough to establish a clear trend (see complete raw data in Supplementary Table S3). According to the box-plot diagrams in Supplementary Figure S5, a trend between δ202Hg and the sampling altitude can be observed (Pearson, r = 0.427, at a 0.05 significance level), while Hg isotopic composition obtained at higher altitudes is consistent with values previously reported in forested areas of the same region (Barre et al., 2018). δ202Hg between mosses and lichens are statistically different (Kruskal-Wallis, H = 69.4, p < 0.0001). A similar difference can be also observed for odd-MIF (Δ199Hg H = 20.8, p < 0.0001 and Δ201Hg H = 53.5, p < 0.0001). Therefore, Hg isotopic composition allows to discriminate the sources and uptake processes of Hg between both biomonitors. In addition, the results exhibit clear differences for δ202Hg in lichens between the Spanish and French slopes, due to an unknown source of fractionation leading to more negative values mainly in French samples (Supplementary Figure S6).
Soils
The soils analyzed displayed δ202Hg ranging from –2.06 to –0.36‰, quite similar to the MDF measured in mosses (Supplementary Figure S6). Soils exhibit a significant negative MIF (Δ199Hg between –0.40 and –0.06‰), close to values measured in lichens. The average values for even-MIF in soils (Δ200Hg = 0.01 ± 0.03‰ as 1 SD) show that they are consistent with the data obtained for lichens with values close to zero. Besides these general trends, the sampling site exhibiting the most negative MDF in lichens (4F, δ202Hg = –4.69‰) and in mosses (4F, δ202Hg = –1.99‰) have a soil with the less negative MDF and MIF (respectively, –0.36 and –0.06‰). The results are in good agreement with the data from vegetal biomass and soils obtained for other ecosystems. (Zheng et al., 2016; Obrist et al., 2017; Jiskra et al., 2018; Huang et al., 2020). Values reported in this study are comparable to those obtained for soils of North American forested ecosystems (Biswas et al., 2008; Demers et al., 2013) but having a slightly more negative shift in odd-MIF. The negative Hg isotopes odd-MIF observed in these soils is consistent with the MIF found in lichens. Unpolluted and less organic topsoils from Metz city display odd-MIF close to zero and MDF, ranging from –0.94 to –0.73‰ (Estrade et al., 2011). Demers et al. (2013), have shown that organic soils have more negative δ202Hg signatures than mineral soils, but similar MIF values. These results suggest that our topsoil samples are mostly representative of the organo-mineral horizon of temperate forest soils. Also, the soil located on site 4F, which has a δ202Hg comparable to mineral soils, could be representative of the mineral phase of the soils.
Discussion
Recent Versus “Older” Lead Isotopes Signature
Regarding to Figure 1, lichens and mosses are distributed along the same single line following a binary mixing model between the contribution of the crustal composition (Elbaz-Poulichet et al., 1984; Elbaz-Poulichet et al., 2011) and the so-called “French” industrial lead (Carignan et al., 2005). In a previous study, lichens from forested areas of the French South Aquitaine region, close to the Iraty Forest (Barre et al., 2018), presented a similar binary mixing line. The plot of 206Pb/207Pb vs. 1/[Pb] for lichens and mosses (Figure 2) allows to define three possible reservoirs of deposited Pb based on the isotopic signature: a local geogenic source, the “European” atmospheric background and a more regional anthropogenic and industrial source (“French” industrial lead). 206Pb/207Pb ratio in lichens for the highest concentrations of Pb, is representative of the “French” industrial Pb defined in previous works (Monna et al., 1997; Carignan et al., 2005; Geagea et al., 2008). Geogenic influence of soil in mosses is clearly represented with increasing Pb concentration and more radiogenic composition (Wedepohl, 1995; Weiss et al., 1999; Millot et al., 2004). Moreover, Al content in these mosses are particularly high, supporting this crustal origin of Pb (Rodriguez Iruretagoiena, 2015). The decrease in Pb concentration in mosses exhibits an end-member of the isotopic signature with a206Pb/207Pb ratio of about 1.158, which was set as the European atmospheric background (Monna et al., 1997; Geagea et al., 2008). This value is similar to Pb isotopic ratios measured in mosses from the arctic region (206Pb/207Pb = 1.157 ± 0.006) directly influenced by European pollution (Haack et al., 2004). The comparison of the isotopic fingerprint between the two biomonitors (see Figure 2) suggests that mosses have a more radiogenic composition than lichens, and closer to the geogenic isotopic signature of Pb. The isotopic measurements support that mosses mostly integrate recent atmospheric Pb during their last year of growth, due to their similarity to the current isotopic signature of the global atmospheric Pb pool in Europe. Lichens exhibit a less radiogenic signature (1.152 ± 0.004 for 206Pb/207Pb average ratio) and have an isotopic composition in agreement with atmospheric particles from the Aspe valley collected several years before (Veschambre et al., 2008). These observations can be consistent with data published in a previous work, suggesting that Pb in lichens in nearby territories can be influenced by resuspended particles of dust, still impacted by legacy contamination of leaded gasoline (Barre et al., 2018).
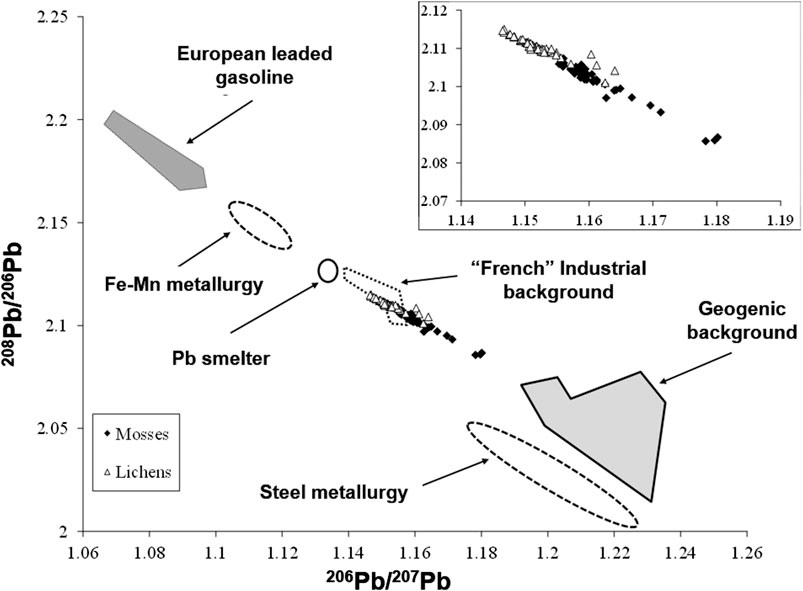
FIGURE 1. Tri-isotopic diagram (208Pb/206Pb vs. 206Pb/207Pb) of lichens and mosses compared with different European sources and geogenic background. (Elbaz-Poulichet et al., 1984; Grousset et al., 1994; Monna et al., 1997; Weiss et al., 1999; Millot et al., 2004; Monna et al., 2004; Carignan et al., 2005; Cloquet et al., 2006; Geagea et al., 2008; Elbaz-Poulichet et al., 2011).
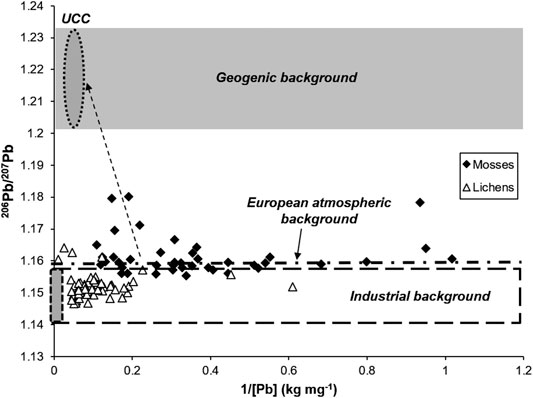
FIGURE 2. 206Pb/207Pb vs. 1/[Pb] in kg/mg diagram for lichens and mosses. Upper continental crust (UCC) data issued from Millot et al. (2004), Wedepohl (1995) et Weiss et al. (1999). Industrial data are from Geagea et al. (2008), Monna et al. (1997) and Cloquet et al. (2006).
In Figure 2, we observed that few lichens reflect more radiogenic composition (sites 5F, 6E, 9E and 23E with 206Pb/207Pb close to 1.16) also with increasing concentrations of Pb, which is consistent with a geogenic contribution. According to a previous work made in nearby areas of southern Aquitaine region (Barre et al., 2018), lichens collected in agricultural and forested sites present Pb isotopic compositions closer to pre-industrial values, which could be extended to several sites of the Iraty forest. In order to normalize the data and eliminate most of the variability induced by the different lichens samples, Ti can be used instead of Al, due to its terrigenous origin (Monna et al., 2011). In this way, it is also possible to reduce variability from the use of different lichen species, different ages of growth, different exposure rates or different morphology. Supplementary Figure S7, shows that isotopic composition measured in lichens seems to be a mixture between the pool of “French” industrial lead and “old” leaded gasoline, suggesting the reemission of particles containing legacy Pb pollution. Sites 6E and 23E do not follow the same trend than the rest of dataset and seem to be mainly influenced by industrial contamination, presenting lower Ti/(Pb*1,000) ratios.
Lichens collected can have a much longer life span (several years) than the annual shoots mosses collected. The Pb isotopes measured in mosses and lichens suggest that the actual atmospheric Pb (<1 year) accumulated in mosses have a composition close to the European background atmospheric lead. This observation is consistent with one hypothesis of Carignan et al. (2005), suggesting that Pb in mosses is representative of the European atmospheric pool. On the other hand, Pb accumulated in lichens may represent a mixture between actual Pb and “old” Pb due to longer exposure time (several years) within the entire thalli of lichen samples.
Mass Independent Fractionation of Even- and Odd-Hg Isotopes
It has been shown that Hg deposited from precipitation should be affected by even-MIF, which is reflected in the isotopic signature of Δ200Hg and Δ204Hg (Demers et al., 2007; Gratz et al., 2010; Chen et al., 2012; Enrico et al., 2016). Figure 3 shows the plot of Δ200Hg vs. MDF for mosses, lichens and soils in this study, and the comparison with other data from bibliography with similar values (Fu et al., 2016b; Yuan et al., 2019). Lichens, as well as soils, present a Δ200Hg close to zero, meaning that the main contribution is the uptake of gaseous elemental Hg (GEM) from dry deposition. The even-MIF signature of Hg in topsoils suggests a dominant influence of Hg inputs from foliage litterfall after leaves uptake of ambient gaseous Hg. Hg inputs to soils in deciduous forests are variable but are generally higher for litterfall than for precipitation (Lindberg, 1996; Grigal et al., 2000; Schwesig and Matzner, 2000; St. Louis et al., 2001; Demers et al., 2007; Demers et al., 2013; Zhou et al., 2013). In some cases they can represent about 93% of total deposition of Hg (Demers et al., 2013). For mosses, the set of samples are divided between Δ200Hg close to zero, consistent with samples of sphagnum mosses in other Pyrenean areas (Enrico et al., 2016), and some samples with significantly positive values. One hypothesis to explain such difference can be obtained by the respective isotopic composition of Hg in wet deposition and the Total Gaseous Mercury (TGM) in other Pyrenean regions (Fu et al., 2016a; Enrico et al., 2016). The moss samples with slightly positive even-MIF present a stronger contribution of wet deposition, according to the results obtained in previous studies (Gratz et al., 2010; Demers et al., 2013; Enrico et al., 2016).
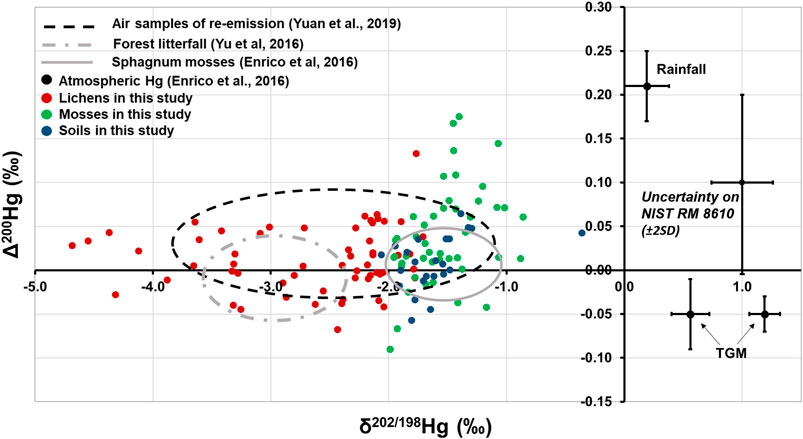
FIGURE 3. Δ200Hg vs. δ202/198Hg in lichens, mosses and soils. Uncertainty calculated for BCR 482 represent the reproducibility of the NIST RM 8610 (former UM-Almadén) (as 2 SD). The data were compared with other data from bibliography.
As shown in Figure 4, two classes of lichens can be discriminated: the ones with odd-MIF close to zero and the other group with negative odd-MIF. Lichens with negative odd-MIF have a Hg isotopic composition close to the TGM (mainly as GEM) samples also from the Pyrenean area (Enrico et al., 2016). The isotopic signature of lichens from background sites is representative of the Hg0 from the surrounding atmosphere, and beech leaves possibly have a similar isotopic fingerprint. Lichens which have a Δ199Hg close to zero could probably integrate both gaseous Hg0 (GEM) and Hg(II) (GOM) from dry deposition, or could be influenced by rainfall Hg(II) inputs (Enrico et al., 2016). The plot of Δ199Hg vs. Δ201Hg for lichens shows that all the samples follow a straight line with a slope of 1.017 ± 0.061 (r2 = 0.832). This slope suggests that both geogenic sources and photoreduction induce a magnetic isotopic effect (MIF), which makes atmospheric Hg0 the major source of contribution for lichens. The odd-MIF for soils is consistent with the values obtained for most lichens, confirming the values for even-MIF. Atmospheric gaseous Hg exhibits generally negative to close to zero Δ199Hg values (Gratz et al., 2010; Rolison et al., 2013; Fu et al., 2016a; Yu et al., 2016). Wet deposition samples exhibit positive odd-MIF (Gratz et al., 2010; Sherman et al., 2012). Therefore, odd-MIF distribution confirms the binary-mixing model exhibited by even-MIF of Hg inputs from wet deposition and from gaseous dry deposition in lichens, mosses and soils. Slightly odd-MIF positive values for mosses imply a more significant uptake of Hg (II) mainly from wet deposition, in opposition to lichens with an odd-MIF composition closer the atmospheric GEM.
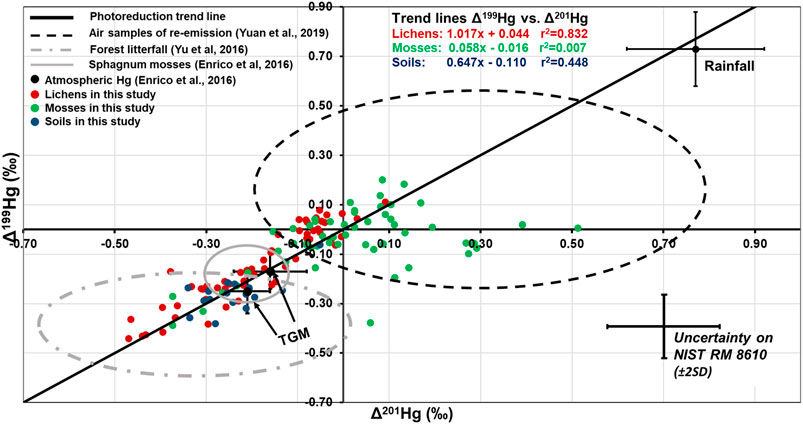
FIGURE 4. Δ199Hg vs. Δ201Hg in lichens, mosses and soils. Uncertainty calculated for NIST RM 8610 (former UM-Almadén) (as 2 SD) represents the long-term reproducibility of the method for CVG. Hg2+ photoreduction line is from Bergquist and Blum, 2009.
Mass Dependent Fractionation of Hg Isotopes
Figure 5 shows the plot of Δ199Hg vs. δ202Hg for lichens, mosses and soil samples collected in this study. The results obtained were compared with other data of vegetation and samples from the same region in previous studies (Enrico et al., 2016; Barre et al., 2018). Regarding the major part of the samples, the results are consistent with other data obtained for lichens in forested areas of the Atlantic Pyrenees on the French side (Barre et al., 2018). The lichens analyzed in this previous work represented a regional background Hg isotopic signature of the gaseous atmospheric Hg uptake by lichens. In the case of mosses, MDF is similar to the values obtained for soil samples, while MIF remains close to zero. Mosses isotopic signatures are also close to the Sphagnum sp. samples analyzed by Enrico et al. (2016). The differences between the signatures of the two biomonitors can be explained by their different Hg absorption fraction because of the relative physical configuration (height, substrate, specific surface … ) of the collected organisms. As was mentioned before, epiphytic lichens represent an integration period of up to few years, while moss shoots samples correspond to their last year of growth. Recent studies have demonstrated that Hg concentrations in mosses are not well correlated with atmospheric Hg0 uptake by dry deposition, but are strongly influenced by precipitation (Harmens et al., 2010; Schröder et al., 2010). Since the moss samples representative of the last year of growth did not display any significant contamination, this suggests that the isotopic signature obtained in lichens is a past phenomenon or related to lichens aging. Most importantly, we can conclude that Hg isotopic composition in mosses and lichens is significantly different combining both MDF and odd-MIF as it is shown in Figure 5. Lichens uptake more atmospheric or oxidized Hg from dry deposition while mosses present evidence of both types of deposition (dry and wet). The values representing MIF and MDF in mosses are between values of rainfall (wet deposition) and TGM (dry deposition) reported by Enrico et al. (2016), leading to more positive MDF values (red arrow). However the Hg uptake by vegetation produces more negative MDF values (green arrow).
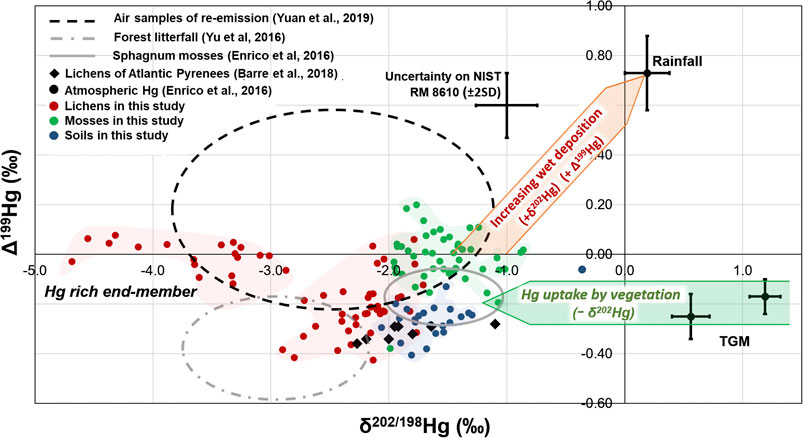
FIGURE 5. Tri-isotopic plot of Δ199Hg vs. δ202/198Hg (‰) for lichens, mosses and soils from Iraty forest. Uncertainties are expressed for the long-term reproducibility of NIST RM 8610 (former UM-Almadén) (as 2 SD) by CVG.
The plot of δ202Hg vs. 1/[Hg] for lichens in Figure 6 shows a correlation between MDF and Hg concentrations typical of a binary mixing model involving a contamination source and the atmospheric background. Through this plot (Figure 6), we can clearly differentiate the two groups of lichens, as we mentioned in the previous section. The majority of samples (n = 39) corresponds to the background isotopic signature of Hg in the Iraty forest, which is consistent with results from other studies carried out in Pyrenean regions located close to the Iraty forest (Enrico et al., 2016; Barre et al., 2018). On the other hand, there is a group of samples (n = 19) exhibiting more negative δ202Hg values with increasing Hg concentrations. An extrapolated, so-called, Hg rich end-member would exhibit a δ202Hg of –4.86 ± 0.21‰ (y-axis intercept) and follows a linear trend correlated with 1/[Hg] (Pearson, r = 0.88 at 0.05 level of significance). The source of such significant fractionation is difficult to assess, and it is supposed that several fractionation processes could be involved. Very negative values of δ202Hg (–4.37 ± 0.13‰ as a confidence 1 SD) for atmospheric measurement are not common but were already observed near a coal-fired power plant in Florida (Sherman et al., 2012). Supplementary Figure S8 represents Pb isotope ratios vs. δ202Hg for lichens and mosses in this study and the comparison with lichens of a previous study (Barre et al., 2018). The results suggest that the isotopic composition of the Hg rich end-member does not match the expected values for contaminated samples of lichens geographically equivalents, obtained for anthropogenic or industrial areas. This is consistent with the results of concentrations for Pb and Hg, and other analyzed elements (see more details about other trace elements concentrations in section S1 in the Supplementary material), that do not exhibit any local metallic pollution source influencing any of the sampling sites.
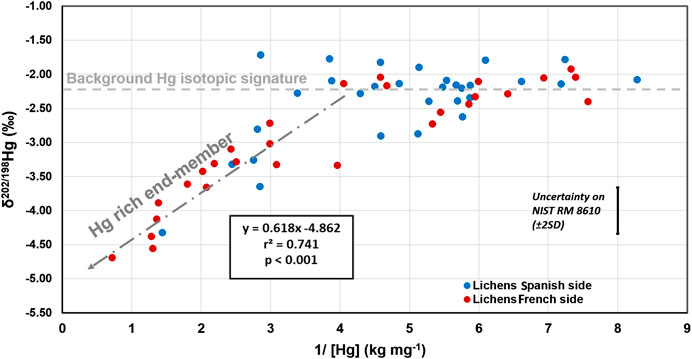
FIGURE 6. δ202/198Hg vs. 1/[Hg] for lichens from Iraty Forest. Blue dots correspond to lichens of the Spanish sampling sites while red dots correspond to lichens of the French sampling sites. The trend suggests a contamination end member of about –4.86 ± 0.21‰ (p < 0.001) for 1/[Hg] = 0.
An unknown anthropogenic emission of Hg or an unidentified source of fractionation must be the origin of the negative δ202Hg values. Natural processes such as re-emission of mercury from soils causing the fractionation of Hg isotopes during its diffusion in the surrounding atmosphere could be involved (Demers et al., 2013). In addition, it has been demonstrated that biologically mediated reduction, which is likely to occur in soils, produces Hg0 with a lighter signature but without significant MIF (Kritee et al., 2007). Recently, Yuan et al. (2019) have observed that after continuous processes of Hg0 uptake and re-emission, the mature foliage becomes progressively enriched in lighter Hg isotopes (more negative δ202Hg values) and depleted in odd-mass isotopes (i.e. negative shift of odd-MIF). Lichens from the Hg rich end-member present also a very negative MDF but, on the contrary, a positive shift in odd-MIF. Thus, the balance between accumulation and re-emission of Hg over time in a forest ecosystem leads to vegetal biomass enriched in lighter isotopes. This is consistent with the observation that the samples of lichens with the most negative MDF are also the samples with the highest Hg concentrations (i.e. larger accumulation with time). The isotopic shift to more positive odd-MIF values in those lichens could then be explained if larger re-emitted Hg0 from lichen surface Hg photoreduction have preferentially preserved odd Hg isotopes (Demers et al., 2013; Yuan et al., 2019). In addition, such lichens may also have accumulated re-emitted Hg0 enriched with odd Hg isotopes (i.e. from foliage and soil) as a consequence of thiol induced Hg photoreduction (Yuan et al., 2019).
In the area of the Iraty forest, a major anthropogenic activity is related to animal breeding in pastures (e.g. cows, sheep and horses). The burning of natural grasses is largely employed annually to increase pasture renewal and supply. Regarding to our sampling area, uncontrolled grass burning between November and April of 2002 lead to large forest fires just after deciduous trees had lost their foliage (Ministère de l’Agriculture et de la Pêche, 2008). Supplementary Figure S9 presents a geographical distribution of the Hg isotopic composition of each sampling site compared with the grasslands burned regularly and during the fire event in 2002 along the Iraty forest. We clearly observe on the map that the most negative values of δ202Hg correspond mainly to samples on the French slope, and that grass burning exhibits rather significant spatial overlap with such specific negative isotopic signature of Hg. During the fire, Hg in ashes and Hg0 are released from soils to the atmosphere (Caldwell et al., 2000; Dicosty et al., 2006; Engle et al., 2006; Biswas et al., 2008). Reactive Hg in ashes may display a lighter isotopic composition (consistent with negative values) as suggested by a binary mixing model set for coal power plant (Sun et al., 2013). Thus, the combination of the effect of re-emission from soil litterfall together with the past effect of grassland and forest fires, could provide an explanation for the isotopic signature of the lichens in the so-called Hg rich end-member.
Conclusion
This work demonstrates for the first time that the employment of Pb and Hg isotopic systems in mosses and lichens improves the understanding of deposition processes with complementary information between both bioaccumulators. Pb isotopes track well major anthropogenic inputs and background contribution, while Hg isotopes are much more informative for Hg deposition pathways. Since lichens have integrated an isotopic signature of Pb exhibiting a mixture between legacy leaded gasoline and the modern European and Industrial Pb fingerprints, they do not equilibrate with the present surrounding atmosphere and integrate variations among several years. Hg isotopic composition is more directly influenced by the type of Hg depositions and uptake pathways by biomonitors than by interannual changes. Interestingly, the isotopic fingerprint of Hg in mosses represents a mixture of Hg uptake from the gaseous phase (Hg0) and from wet deposition (Hg (II)). In this sense, mosses shoots are better indicator for recent depositions, as expected, but can be altered by local soil dust events for Pb and by mixed wet and dry deposition for Hg. In the case of lichens, the isotopic fingerprint is closer to the isotopic composition of top organic soils influenced by tree foliage deposition (e.g., litterfall). In addition, the differences between elemental integration times of both bioaccumulators represent significant differences. Mosses (annual “shoots”) represent a shorter integration period than entire lichens thalli for which annual growth cannot be determined. For lichens, integration time is much uncertain and altered but Hg isotopic signature only reflect dry gaseous Hg contribution in this remote location. This confirms that gaseous elemental Hg is the main source of Hg in epiphytic lichens in such temperate forest. This work also exhibits that the spatial distribution of the Hg isotopes composition over a small scale in a forest ecosystem could be altered by natural or anthropogenic events such as climatic conditions, land use and forest fires.
Data Availability Statement
The raw data supporting the conclusions of this article will be made available by the authors, without undue reservation.
Author Contributions
JB, DV, JS, AD, DA, and GD conceived the scientific questions and objectives, and decided the sampling strategy. JB, SB, ET, DV, DA, JS, AD, and GD performed the sampling strategy and sample conditioning. JB, SQ-A, CS-L, SB, and ET have performed the sample preparation, sample analysis and data treatment. JB, SQ-A, and DA interpreted the data and wrote the first draft, which was also edited and commented on by the other authors.
Funding
This project was supported by the Aquitaine Region and the Pyrenean Working Community (EU Interreg program) and Conseil Départemental des Pyrénées Atlantiques, in the framework of the PYNATEO and Lichen-64 projects. Julien Barre doctoral fellowship (ED211) was funded by CNRS-INEE and UPPA. This work is a contribution to MercOx European project (EMPIR EURAMET, Grant number 16ENV01).
Conflict of Interest
The authors declare that the research was conducted in the absence of any commercial or financial relationships that could be construed as a potential conflict of interest.
Acknowledgments
The authors would like to thank Hervé Pinaly, Jean Martin (IPREM); Silvia Fernández-Ortiz de Vallejuelo, Azibar Rodríguez-Iruretagoiena, Julen Bustamente (UPV/EHU); Raúl Bermejo-Orduna (UNAV) for their help during the sampling campaign. Julien Barre is grateful to CNRS-INEE and UPPA for his doctoral fellowship (ED211).
Supplementary Material
The Supplementary Material for this article can be found online at: https://www.frontiersin.org/articles/10.3389/fenvc.2020.582001/full#supplementary-material
Glossary
A.s.l. Above Sea Level
CVG Cold Vapor Generation
DGA Dual Gold Amalgamation
GEM Gaseous Elemental Mercury
GOM Gaseous Oxidized Mercury
ICP-MS Inductively coupled Plasma Mass Spectrometry
MC Multicollector
MDF Mass Dependent Fractionation
MIF Mass Independent Fractionation
PE Polyethylene
References
Agnan, Y., Probst, A., and Séjalon-Delmas, N. (2017). Evaluation of lichen species resistance to atmospheric metal pollution by coupling diversity and bioaccumulation approaches: a new bioindication scale for French forested areas. Ecol. Indicat. 72, 99–110. doi:10.1016/j.ecolind.2016.08.006
Agnan, Y., Séjalon-Delmas, N., Claustres, A., and Probst, A. (2015). Investigation of spatial and temporal metal atmospheric deposition in France through lichen and moss bioaccumulation over one century. Sci. Total Environ. 529, 285–296. doi:10.1016/j.scitotenv.2015.05.083
Agnan, Y., Séjalon-Delmas, N., and Probst, A. (2013). Comparing early twentieth century and present-day atmospheric pollution in SW France: a story of lichens. Environ. Pollut. 172, 139–148. doi:10.1016/j.envpol.2012.09.008
Bérail, S., Cavalheiro, J., Tessier, E., Barre, J. P. G., Pedrero, Z., Donard, O. F. X., et al. (2017). Determination of total Hg isotopic composition at ultra-trace levels by on line cold vapor generation and dual gold-amalgamation coupled to MC-ICP-MS. J. Anal. At. Spectrom. 32, 373–384. doi:10.1039/c6ja00375c
Bačkor, M., and Loppi, S. (2009). Interactions of lichens with heavy metals. Biol. Plant. 53, 214–222. doi:10.1007/s10535-009-0042-y
Bargagli, R., Monaci, F., Borghini, F., Bravi, F., and Agnorelli, C. (2002). Mosses and lichens as biomonitors of trace metals . A comparison study on Hypnum cupressiforme and Parmelia caperata in a former mining district in Italy. Environ. Pollut. 116, 279–287. doi:10.1016/S0269-7491(01)00125-7
Bargagli, R. (2016). Moss and lichen biomonitoring of atmospheric mercury: a review. Sci. Total Environ. 572, 216–231. doi:10.1016/j.scitotenv.2016.07.202
Barre, J. P. G., Amouroux, D., Deletraz, G., Bérail, S., Pinaly, H., Donard, O. F. X., et al. (2013). Investigating the isotopic composition of mercury and lead in epiphytic lichens from South-western France (Pyrénées-Atlantiques) to better constrain the spatial variability of their atmospheric transport and deposition. E3S Web Conf. 1, 1–4. doi:10.1051/e3sconf/20130129002.
Barre, J. P. G., Deletraz, G., Frayret, J., Pinaly, H., Donard, O. F. X., and Amouroux, D. (2015). Approach to spatialize local to long-range atmospheric metal input (Cd, Cu, Hg, Pb) in epiphytic lichens over a meso-scale area (Pyrénées-Atlantiques, southwestern France). Environ. Sci. Pollut. Res. 22, 8536–8548. doi:10.1007/s11356-014-3990-5
Barre, J. P. G., Deletraz, G., Sola-Larranaga, C., Santamaría, J. M., Bérail, S., Donard, O. F. X., et al. (2018). Multi-element isotopic signature (C, N, Pb, Hg) in epiphytic lichens to discriminate atmospheric 2 contamination as a function of land-use characteristics (Pyrénées-Atlantiques, SW France). Environ. Pollut. 243, 961–971. doi:10.1017/CBO9781107415324.004
Basile, A., Sorbo, S., Aprile, G., Conte, B., and Castaldo Cobianchi, R. (2008). Comparison of the heavy metal bioaccumulation capacity of an epiphytic moss and an epiphytic lichen. Environ. Pollut. 151, 401–407. doi:10.1016/j.envpol.2007.07.004
Bergquist, B. A., and Blum, J. D. (2007). Mass-dependent and -independent fractionation of Hg isotopes by photoreduction in aquatic systems. Science 318, 417–420. doi:10.1126/science.1148050.
Bergquist, B. A., and Blum, J. D. (2009). The odds and evens of mercury isotopes: applications of mass-dependent and mass-independent isotope fractionation. Elements 5, 353–357. doi:10.2113/gselements.5.6.353
Biswas, A., Blum, J. D., Bergquist, B. A., Keeler, G. J., and Xie, Z. (2008). Natural mercury isotope variation in coal deposits and organic soils. Environ. Sci. Technol. 42, 8303–8309. doi:10.1021/es801444b
Blum, J. D., and Bergquist, B. A. (2007). Reporting of variations in the natural isotopic composition of mercury. Anal. Bioanal. Chem. 388, 353–359. doi:10.1007/s00216-007-1236-9
Blum, J. D., Johnson, M. W., Gleason, J. D., Demers, J. D., Landis, M. S., and Krupa, S. (2012). “Mercury concentration and isotopic composition of epiphytic tree lichens in the athabasca oil sands region,” in Alberta oil sands: energy, industry, and the environment. Editor K. E. Percy (Amsterdam, Netherlands: Elsevier), 373–390. doi:10.1016/B978-0-08-097760-7.00016-0
Blum, J. D., Sherman, L. S., and Johnson, M. W. (2014). Mercury isotopes in earth and environmental sciences. Annu. Rev. Earth Planet Sci. 42, 249–269. doi:10.1146/annurev-earth-050212-124107
Bustamante Alonso, J. (2014). Monitoring of organic contamination in atmospheric, aquatic and terrestrial ecosystems. Available at: https://www.ehu.eus/en/web/estudiosdeposgrado-graduondokoikasketak/-/kutsadura-organikoaren-jarraipena-ur-ekosistematan-eta-atmosferan
Caldwell, C. A., Canavan, C. M., and Bloom, N. S. (2000). Potential effects of forest fire and storm flow on total mercury and methylmercury in sediments of an arid-lands reservoir. Sci. Total Environ. 260, 125–133. doi:10.1016/s0048-9697(00)00554-4
Carballeira, C. B., Aboal, J. R., Fernández, J. A., and Carballeira, A. (2008). Comparison of the accumulation of elements in two terrestrial moss species. Atmos. Environ. 42, 4904–4917. doi:10.1016/j.atmosenv.2008.02.028
Carignan, J., Estrade, N., Sonke, J. E., and Donard, O. F. X. (2009). Odd isotope deficits in atmospheric Hg measured in lichens. Environ. Sci. Technol. 43, 5660–5664. doi:10.1021/es900578v
Carignan, J., and Gariépy, C. (1995). Isotopic composition of epiphytic lichens as a tracer of the sources of atmospheric emissions in southern Québec, Canada. Geochim. Cosmochim. Acta 59, 4427–4433. doi:10.1016/0016-7037(95)00302-G
Carignan, J., Libourel, G., Cloquet, C., and Le Forestier, L. (2005). Lead isotopic composition of fly ash and flue gas residues from municipal solid waste combustors in France: implications for atmospheric lead source tracing. Environ. Sci. Technol. 39, 2018–2024. doi:10.1021/es048693x
Carignan, J., Simonetti, A., and Gariépy, C. (2002). Dispersal of atmospheric lead in northeastern North America as recorded by epiphytic lichens. Atmos. Environ. 36, 3759–3766. doi:10.1016/S1352-2310(02)00294-7
Chen, J. B., Hintelmann, H., Feng, X. B., and Dimock, B. (2012). Unusual fractionation of both odd and even mercury isotopes in precipitation from Peterborough, ON, Canada. Geochim. Cosmochim. Acta 90, 33–46. doi:10.1016/j.gca.2012.05.005
Cloquet, C., Carignan, J., and Libourel, G. (2006). Atmospheric pollutant dispersion around an urban area using trace metal concentrations and Pb isotopic compositions in epiphytic lichens. Atmos. Environ. 40, 574–587. doi:10.1016/j.atmosenv.2005.09.073
Corella, J. P., Valero-Garcés, B. L., Wang, F., Martínez-Cortizas, A., Cuevas, C. A., and Saiz-Lopez, A. (2017). 700 years reconstruction of mercury and lead atmospheric deposition in the Pyrenees (NE Spain). Atmos. Environ. 155, 97–107. doi:10.1016/j.atmosenv.2017.02.018
Demers, J. D., Blum, J. D., and Zak, D. R. (2013). Mercury isotopes in a forested ecosystem: implications for air-surface exchange dynamics and the global mercury cycle. Global Biogeochem. Cycles 27, 222–238. doi:10.1002/gbc.20021
Demers, J. D., Driscoll, C. T., Fahey, T. J., and Yavitt, J. B. (2007). Mercury cycling in litter and soil in different forest types in the Adirondack Region, New York, USA. Ecol. Appl. 17, 1341–1351. doi:10.1890/06-1697.1
Dicosty, R. J., Callaham, M. a., and Stanturf, J. a. (2006). Atmospheric deposition and Re-emission of mercury estimated in a prescribed forest-fire experiment in Florida, USA. Water. Air. Soil Pollut. 176, 77–91. doi:10.1007/s11270-006-9149-3
Dolgopolova, A., Weiss, D. J., Seltmann, R., Kober, B., Mason, T. F. D., Coles, B., et al. (2006). Use of isotope ratios to assess sources of Pb and Zn dispersed in the environment during mining and ore processing within the Orlovka–Spokoinoe mining site (Russia). Appl. Geochem. 21, 563–579. doi:10.1016/j.apgeochem.2005.12.014
Elbaz-Poulichet, F., Dezileau, L., Freydier, R., Cossa, D., and Sabatier, P. (2011). A 3500-year record of Hg and Pb contamination in a mediterranean sedimentary archive (the Pierre Blanche Lagoon, France). Environ. Sci. Technol. 45, 8642–8647. doi:10.1021/es2004599
Elbaz-Poulichet, F., Holliger, P., Huang, W. W., and Martin, J.-M. (1984). Lead cycling in estuaries, illustrated by the Gironde estuary, France. Nature 308, 409–414. doi:10.1038/308409a0
Engle, M. A., Sexauer Gustin, M., Johnson, D. W., Murphy, J. F., Miller, W. W., Walker, R. F., et al. (2006). Mercury distribution in two Sierran forest and one desert sagebrush steppe ecosystems and the effects of fire. Sci. Total Environ. 367, 222–233. doi:10.1016/j.scitotenv.2005.11.025
Enrico, M., Le Roux, G., Marusczak, N., Heimbürger, L. E., Claustres, A., Fu, X., et al. (2016). Atmospheric mercury transfer to peat bogs dominated by gaseous elemental mercury dry deposition. Environ. Sci. Technol. 50, 2405–2412. doi:10.1021/acs.est.5b06058
Estrade, N., Carignan, J., and Donard, O. F. X. (2009). Measuring Hg isotopes in bio-geo-environmental reference materials. Geostand. Geoanal. Res. 34, 79–93. doi:10.1111/j.1751-908X.2009.00040.x
Estrade, N., Carignan, J., and Donard, O. F. X. (2010). Isotope tracing of atmospheric mercury sources in an urban area of northeastern France. Environ. Sci. Technol. 44, 6062–6067. doi:10.1021/es100674a
Estrade, N., Carignan, J., and Donard, O. F. X. (2011). Tracing and quantifying anthropogenic mercury sources in soils of northern France using isotopic signatures. Environ. Sci. Technol. 45, 1235–1242. doi:10.1021/es1026823
Ezcurra, A., Benech, B., Echelecou, A., Santamaría, J. M., Herrero, I., and Zulueta, E. (2013). Influence of local air flow regimes on the ozone content of two Pyrenean valleys. Atmos. Environ. 74, 367–377. doi:10.1016/j.atmosenv.2013.03.051
Fabri, R., Krause, M., Dalfior, B. M., Salles, R. C., de Freitas, A. C., da Silva, H. E., et al. (2018). Trace elements in soil, lichens, and mosses from fildes Peninsula, Antarctica: spatial distribution and possible origins. Environ. Earth Sci. 77, 124. doi:10.1007/s12665-018-7298-5
Fernández, J. A., Ederra, A., Núñez, E., Martínez-Abaigar, J., Infante, M., Heras, P., et al. (2002). Biomonitoring of metal deposition in northern Spain by moss analysis. Sci. Total Environ. 300, 115–127. doi:10.1016/S0048-9697(02)00230-9
Fu, X., Marusczak, N., Wang, X., Gheusi, F., and Sonke, J. E. (2016a). Isotopic composition of gaseous elemental mercury in the free troposphere of the Pic du Midi observatory, France. Environ. Sci. Technol. 50, 5641–5650. doi:10.1021/acs.est.6b00033
Fu, X., Zhu, W., Zhang, H., Sommar, J., Yu, B., Yang, X., et al. (2016b). Depletion of atmospheric gaseous elemental mercury by plant uptake at Mt. Changbai, Northeast China. Atmos. Chem. Phys. 16, 12861–12873. doi:10.5194/acp-16-12861-2016
Galsomiès, L., Letrouit, M. A., Deschamps, C., Savanne, D., and Avnaim, M. (1999). Atmospheric metal deposition in France: initial results on moss calibration from the 1996 biomonitoring. Sci. Total Environ. 232, 39–47. doi:10.1016/S0048-9697(99)00108-4
Garty, J., Weissman, L., Cohen, Y., Karnieli, a., and Orlovsky, L. (2001). Transplanted lichens in and around the Mount Carmel National Park and the Haifa Bay industrial region in Israel: physiological and chemical responses. Environ. Res. 85, 159–176. doi:10.1006/enrs.2000.4222
Geagea, M. L., Stille, P., Gauthier-Lafaye, F., and Millet, M. (2008). Tracing of industrial aerosol sources in an urban environment using Pb, Sr, and Nd isotopes. Environ. Sci. Technol. 42, 692–698. doi:10.1021/es071704c
Gehrke, G. E., Blum, J. D., and Meyers, P. A. (2009). The geochemical behavior and isotopic composition of Hg in a mid-Pleistocene western Mediterranean sapropel. Geochim. Cosmochim. Acta 73, 1651–1665. doi:10.1016/j.gca.2008.12.012
Ghosh, S., Xu, Y., Humayun, M., and Odom, L. (2008). Mass-independent fractionation of mercury isotopes in the environment. Geochem. Geophys. Geosyst. 9, Q03004. doi:10.1029/2007GC001827
Giordano, S., Adamo, P., Spagnuolo, V., Tretiach, M., and Bargagli, R. (2013). Accumulation of airborne trace elements in mosses, lichens and synthetic materials exposed at urban monitoring stations: towards a harmonisation of the moss-bag technique. Chemosphere 90, 292–299. doi:10.1016/j.chemosphere.2012.07.006
González-Miqueo, L., Elustondo, D., Lasheras, E., Bermejo, R., and Santamaría, J. M. (2009). Spatial trends in heavy metals and nitrogen deposition in Navarra (Northern Spain) based on moss analysis. J. Atmos. Chem. 62, 59–72. doi:10.1007/s10874-009-9139-0
González-Miqueo, L., Elustondo, D., Lasheras, E., and Santamaría, J. M. (2010). Use of native mosses as biomonitors of heavy metals and nitrogen deposition in the surroundings of two steel works. Chemosphere 78, 965–971. doi:10.1016/j.chemosphere.2009.12.028
Gratz, L. E., Keeler, G. J., Blum, J. D., and Sherman, L. S. (2010). Isotopic composition and fractionation of mercury in Great Lakes precipitation and ambient air. Environ. Sci. Technol. 44, 7764–7770. doi:10.1021/es100383w
Grigal, D. F., Kolka, R. K., Fleck, J. A., and Nater, E. A. (2000). Mercury budget of an upland-peatland watershed. Biogeochemistry 50, 95–109. doi:10.1023/A:1006322705566
Gross, B. H., Kreutz, K. J., Osterberg, E. C., McConnell, J. R., Handley, M., Wake, C. P., and Yalcin, K. (2012). Constraining recent lead pollution sources in the North Pacific using ice core stable lead isotopes. J. Geophys. Res., 117, D16307. doi:10.1029/2011JD017270
Grousset, F. E., Quétel, C. R., Thomas, B., Buat-Ménard, P., Donard, O. F. X., and Bucher, A. (1994). Transient Pb isotopic signatures in the western European atmosphere. Environ. Sci. Technol. 28, 1605–1608. doi:10.1021/es00058a011
Haack, U., Kienholz, B., Reimann, C., Schneider, J., and Stumpfl, E. (2004). Isotopic composition of lead in moss and soil of the European Arctic. Geochim. Cosmochim. Acta 68, 2613–2622. doi:10.1016/j.gca.2003.12.019
Harmens, H., Norris, D. A., Steinnes, E., Kubin, E., Piispanen, J., Alber, R., et al. (2010). Mosses as biomonitors of atmospheric heavy metal deposition: spatial patterns and temporal trends in Europe. Environ. Pollut. 158, 3144–3156. doi:10.1016/j.envpol.2010.06.039.
Harmens, H., Norris, D., and Mills, G. (2013). Heavy metals and nitrogen in mosses: spatial patterns in 2010/2011 and long-term temporal trends in Europe. Bangor, UK: NERC/Centre for Ecology & Hydrology. 63.
Hintelmann, H., and Lu, S. (2003). High precision isotope ratio measurements of mercury isotopes in cinnabar ores using multi-collector inductively coupled plasma mass spectrometry. Analyst 128, 635. doi:10.1039/b300451a
Huang, S., Jiang, R., Song, Q., Zhang, Y., Huang, Q., Su, B., et al. (2020). Study of mercury transport and transformation in mangrove forests using stable mercury isotopes. Sci. Total Environ. 704, 135928. doi:10.1016/j.scitotenv.2019.135928
Izquieta-Rojano, S., López-Aizpún, M., Irigoyen, J. J., Santamaría, J. M., Santamaría, C., Lasheras, E., et al. (2018). Eco-physiological response of Hypnum cupressiforme Hedw. to increased atmospheric ammonia concentrations in a forest agrosystem. Sci. Total Environ. 619–620, 883–895. doi:10.1016/j.scitotenv.2017.11.139
Jiskra, M., Sonke, J. E., Obrist, D., Bieser, J., Ebinghaus, R., Myhre, C. L., et al. (2018). A vegetation control on seasonal variations in global atmospheric mercury. Nat. Geosci. 11, 244–250. doi:10.1038/s41561-018-0078-8
Jiskra, M., Wiederhold, J. G., Skyllberg, U., Kronberg, R. M., Hajdas, I., and Kretzschmar, R. (2015). Mercury deposition and Re-emission pathways in boreal forest soils investigated with Hg isotope signatures. Environ. Sci. Technol. 49, 7188–7196. doi:10.1021/acs.est.5b00742
Kłos, A., Ziembik, Z., Rajfur, M., Dołhańczuk-Śródka, A., Bochenek, Z., Bjerke, J. W., et al. (2018). Using moss and lichens in biomonitoring of heavy-metal contamination of forest areas in southern and north-eastern Poland. Sci. Total Environ. 627, 438–449. doi:10.1016/j.scitotenv.2018.01.211
Klapstein, S. J., Walker, A. K., Saunders, C. H., Cameron, R. P., Murimboh, J. D., and O’Driscoll, N. J. (2020). Spatial distribution of mercury and other potentially toxic elements using epiphytic lichens in Nova Scotia. Chemosphere 241, 125064. doi:10.1016/j.chemosphere.2019.125064
Komárek, M., Ettler, V., Chrastný, V., and Mihaljevič, M. (2008). Lead isotopes in environmental sciences: a review. Environ. Int. 34, 562–577. doi:10.1016/j.envint.2007.10.005
Kritee, K., Blum, J. D., Johnson, M. W., Bergquist, B. A., and Barkay, T. (2007). Mercury stable isotope fractionation during reduction of Hg(II) to Hg(0) by mercury resistant microorganisms. Environ. Sci. Technol. 41, 1889–1895. doi:10.1021/es062019t
Kunert, M., Friese, K., Weckert, V., and Markert, B. (1999). Lead isotope systematics in Polytrichum formosum: an example from a biomonitoring field study with mosses. Environ. Sci. Technol. 33, 3502–3505. doi:10.1021/es981352x
Lauretta, D. S., Klaue, B., Blum, J. D., and Buseck, P. R. (2001). Mercury abundances and isotopic compositions in the murchison (CM) and allende (CV)carbonaceous chondrites. Geochim. Cosmochim. Acta 65, 2807–2818. doi:10.1016/S0016-7037(01)00630-5
Lindberg, S. E. (1996). “Forests and the global biogeochemical cycle of mercury: the importance of understanding air/vegetation exchange processes,” in Global and regional mercury cycles: sources, fluxes and mass balances. Editors W. Baeyens, R. Ebinghaus, and O. Vasiliev (Dordrecht, Netherlands: Springer), 359–380. doi:10.1007/978-94-009-1780-4_18
Loppi, S., and Pirintsos, S. A. (2003). Epiphytic lichens as sentinels for heavy metal pollution at forest ecosystems (central Italy). Environ. Pollut. 121, 327–332. doi:10.1016/S0269-7491(02)00269-5
St. Louis, V. L., Rudd, J. W. M., Kelly, C. A., Hall, B. D., Rolfhus, K. R., Scott, K. J., et al. (2001). Importance of the forest canopy to fluxes of methyl mercury and total mercury to boreal ecosystems. Environ. Sci. Technol. 35, 3089–3098. doi:10.1021/es001924p
Manhes, G., Allègre, B. D., and Hamelin, B. (1980). Lead isotope study of basic-ultrabasic layered complexes: speculations about the age of earth and primitive mantle characteristics. Earth Planet Sci. Lett. 47, 370–382. doi:10.1016/0012-821X(80)90024-2
Millot, R., Allègre, C.-J., Gaillardet, J., and Roy, S. (2004). Lead isotopic systematics of major river sediments: a new estimate of the Pb isotopic composition of the Upper Continental Crust. Chem. Geol. 203, 75–90. doi:10.1016/j.chemgeo.2003.09.002
Ministère de l’Agriculture et de la Pêche (2008). Plan de Protection des Forêts. Available at: https://www.dfci-aquitaine.fr/wp-content/uploads/2017/12/PPFCI_light.pdf
Moldovan, M., Veschambre, S., Amouroux, D., Bénech, B., and Donard, O. F. X. (2007). Platinum, palladium, and rhodium in fresh snow from the Aspe valley (Pyrenees Mountains, France). Environ. Sci. Technol. 41, 66–73. doi:10.1021/es061483v
Monna, F., Bouchaou, L., Rambeau, C., and Losno, R. (2011). Lichens used as monitors of atmospheric pollution around agadir (Southwestern Morocco )— a case study predating lead-free gasoline. Water Air Soil Pollut. 223, 1263–1274. doi:10.1007/s11270-011-0942-2
Monna, F., Galop, D., Carozza, L., Tual, M., Beyrie, a., Marembert, F., et al. (2004). Environmental impact of early Basque mining and smelting recorded in a high ash minerogenic peat deposit. Sci. Total Environ. 327, 197–214. doi:10.1016/j.scitotenv.2004.01.010
Monna, F., Lancelot, J., Croudace, I. W., Cundy, A. B., and Lewis, J. T. (1997). Pb isotopic composition of airborne particulate material from France and the southern United Kingdom: implications for Pb pollution sources in urban areas. Environ. Sci. Technol. 31, 2277–2286. doi:10.1021/es960870
Obrist, D., Agnan, Y., Jiskra, M., Olson, C. L., Colegrove, D. P., Hueber, J., et al. (2017). Tundra uptake of atmospheric elemental mercury drives Arctic mercury pollution. Nature 547, 201–204. doi:10.1038/nature22997
Olson, C. L., Jiskra, M., Sonke, J. E., and Obrist, D. (2019). Mercury in tundra vegetation of Alaska: spatial and temporal dynamics and stable isotope patterns. Sci. Total Environ. 660, 1502–1512. doi:10.1016/j.scitotenv.2019.01.058
Panichev, N., Mokgalaka, N., and Panicheva, S. (2019). Assessment of air pollution by mercury in South African provinces using lichens Parmelia caperata as bioindicators. Environ. Geochem. Health 41, 2239–2250. doi:10.1007/s10653-019-00283-w
Perrot, V., Epov, V. N., Pastukhov, M. V., Grebenshchikova, V. I., Zouiten, C., Sonke, J. E., et al. (2010). Tracing sources and bioaccumulation of mercury in fish of Lake Baikal-Angara River using Hg isotopic composition. Environ. Sci. Technol. 44, 8030–8037. doi:10.1021/es101898e
Ratier, A., Dron, J., Revenko, G., Austruy, A., Dauphin, C. E., Chaspoul, F., et al. (2018). Characterization of atmospheric emission sources in lichen from metal and organic contaminant patterns. Environ. Sci. Pollut. Res. 25, 8364–8376. doi:10.1007/s11356-017-1173-x
Ribeiro Guevara, S., Bubach, D., and Arribére, M. (2004). Mercury in lichens of Nahuel Huapi national park, Patagonia, Argentina. J. Radioanal. Nucl. Chem. 261, 679–687. doi:10.1023/B:JRNC.0000037113.24485.47
Rodriguez Iruretagoiena, A. (2015). Metal pollution in estuarines and high altitude mountains: geographical distribution, evolution in time and toxicological implications. Available from: https://addi.ehu.es/handle/10810/21702.
Rolison, J. M., Landing, W. M., Luke, W., Cohen, M., and Salters, V. J. M. (2013). Isotopic composition of species-specific atmospheric Hg in a coastal environment. Chem. Geol. 336, 37–49. doi:10.1016/j.chemgeo.2012.10.007
Schnyder, E., Štrok, M., Kosonen, Z., Skudnik, M., Mazej, D., Jeran, Z., et al. (2018). Lead concentrations and stable lead isotope ratios in moss in Slovenia and Switzerland. Ecol. Indicat. 95, 250–259. doi:10.1016/j.ecolind.2018.06.072
Schröder, W., Holy, M., Pesch, R., Harmens, H., Ilyin, I., Steinnes, E., et al. (2010). Are cadmium, lead and mercury concentrations in mosses across Europe primarily determined by atmospheric deposition of these metals? J. Soils Sediments 10, 1572–1584. doi:10.1007/s11368-010-0254-y
Schröder, W., Pesch, R., Hertel, A., Schonrock, S., Harmens, H., Mills, G., et al. (2013). Correlation between atmospheric deposition of Cd, Hg and Pb and their concentrations in mosses specified for ecological land classes covering Europe. Atmos. Pollut. Res. 4, 267–274. doi:10.5094/APR.2013.029
Schwesig, D., and Matzner, E. (2000). Pools and fluxes of mercury and methylmercury in two forested catchments in Germany. Sci. Total Environ. 260, 213–223. doi:10.1016/s0048-9697(00)00565-9
Sherman, L. S., Blum, J. D., Franzblau, A., and Basu, N. (2013). New insight into biomarkers of human mercury exposure using naturally occurring mercury stable isotopes. Environ. Sci. Technol. 47, 3403–3409. doi:10.1021/es305250z
Sherman, L. S., Blum, J. D., Keeler, G. J., Demers, J. D., and Dvonch, J. T. (2012). Investigation of local mercury deposition from a coal-fired power plant using mercury isotopes. Environ. Sci. Technol. 46, 382–390. doi:10.1021/es202793c
Shotyk, W., and Cuss, C. W. (2019). Atmospheric Hg accumulation rates determined using Sphagnum moss from ombrotrophic (rain-fed) bogs in the Athabasca Bituminous Sands region of northern Alberta, Canada. Ecol. Indicat. 107, 105626. doi:10.1016/j.ecolind.2019.105626
Spiro, B., Weiss, D. J., Purvis, O. W., Mikhailova, I., Williamson, B. J., Coles, B. J., et al. (2004). Lead isotopes in lichen transplants around a Cu smelter in Russia determined by MC-ICP-MS reveal transient records of multiple sources. Environ. Sci. Technol. 38, 6522–6528. doi:10.1021/es049277f
Stacey, J. S., and Kramers, J. D. (1975). Approximation of terrestrial lead isotope evolution by a two-stage model. Earth Planet Sci. Lett. 26, 207–221. doi:10.1016/0012-821X(75)90088-6
Sun, R., Heimbürger, L.-E., Sonke, J. E., Liu, G., Amouroux, D., and Berail, S. (2013). Mercury stable isotope fractionation in six utility boilers of two large coal-fired power plants. Chem. Geol. 336, 103–111. doi:10.1016/j.chemgeo.2012.10.055
Sun, R., Streets, D. G., Horowitz, H. M., Amos, H. M., Liu, G., Perrot, V., et al. (2016). Historical (1850-2010) mercury stable isotope inventory from anthropogenic sources to the atmosphere. Elem. Anthr. 4, 1–15. doi:10.12952/journal.elementa.000091
Szczepaniak, K. Ã., and Biziuk, M. (2003). Aspects of the biomonitoring studies using mosses and lichens as indicators of metal pollution. Environ. Res. 93, 221–230. doi:10.1016/S0013-9351(03)00141-5
Szczepaniak, K. (2003). Aspects of the biomonitoring studies using mosses and lichens as indicators of metal pollution. Environ. Res. 93, 221–230. doi:10.1016/S0013-9351(03)00141-5
AFNOR (2008). Norme NF X43-903. Biosurveillance de l’environnement. Détermination d’un indice biologique de lichens épiphytes (IBLE). Paris, France: AFNOR - Association Française de Normalisation, 19
Vegetation Coordination Center, (2010). Heavy metals in European mosses: 2010 survey. Available from: https://core.ac.uk/download/pdf/57335.pdf.
Veschambre, S., Moldovan, M., Amouroux, D., Santamaría Ulecia, J., Benech, B., Etchelecou, A., et al. (2008). Apports atmosphériques des éléments traces métalliques dans la vallée dʼAspe et le tunnel niveau de contamination et évaluation des sources dʼémissions Import of atmospheric trace metal elements in the Aspe valley and Somport tunnel ( Pyrénées Atlantiques. Pollut. Atmos. 198–199, 215–234. doi:10.4267/pollution-atmospherique.1342
Walther, D. A., Ramelow, G. J., and Beck, J. N. (1990). Temporal changes in metal levels of the lichens parmotrema praesorediosum and ramalina stenospora, southwest Louisiana. Water Air Soil Pollut. 53, 189–200. doi:10.1017/CBO9781107415324.004.
Wang, X., Luo, J., Yin, R., Yuan, W., Lin, C. J., Sommar, J., et al. (2017). Using mercury isotopes to understand mercury accumulation in the montane forest floor of the Eastern Tibetan Plateau. Environ. Sci. Technol. 51, 801–809. doi:10.1021/acs.est.6b03806.
Wang, X., Yuan, W., Feng, X., Wang, D., and Luo, J. (2019). Moss facilitating mercury, lead and cadmium enhanced accumulation in organic soils over glacial erratic at Mt. Gongga, China. Environ. Pollut. 254, 112974. doi:10.1016/j.envpol.2019.112974.
Wedepohl, K. H. (1995). The composition of the continental crust. Geochim. Cosmochim. Acta 59, 1217–1232. doi:10.1016/0016-7037(95)00038-2.
Weiss, D., Shotyk, W., Appleby, P. G., Kramers, J. D., and Cheburkin, A. K. (1999). Atmospheric Pb deposition since the industrial revolution recorded by five Swiss peat profiles: enrichment factors, fluxes, isotopic composition, and sources. Environ. Sci. Technol. 33, 1340–1352. doi:10.1021/es980882q.
Wiederhold, J. G., Cramer, C. J., Daniel, K., Infante, I., Bourdon, B., and Kretzschmar, R. (2010). Equilibrium mercury isotope fractionation between dissolved Hg(II) species and thiol-bound Hg. Environ. Sci. Technol. 44, 4191–4197. doi:10.1021/es100205t.
Yin, R., Feng, X., and Meng, B. (2013). Stable mercury isotope variation in rice plants (Oryza sativa L.) from the Wanshan mercury mining district, SW China. Environ. Sci. Technol. 47, 2238–2245. doi:10.1021/es304302a.
Yu, B., Fu, X., Yin, R., Zhang, H., Wang, X., Lin, C. J., et al. (2016). Isotopic composition of atmospheric mercury in China: new evidence for sources and transformation processes in air and in vegetation. Environ. Sci. Technol. 50, 9362–9369. doi:10.1021/acs.est.6b01782.
Yuan, W., Sommar, J., Lin, C. J., Wang, X., Li, K., Liu, Y., et al. (2019). Stable isotope evidence shows Re-emission of elemental mercury vapor occurring after reductive loss from foliage. Environ. Sci. Technol. 53, 651–660. doi:10.1021/acs.est.8b04865.
Zheng, W., Obrist, D., Weis, D., and Bergquist, B. A. (2016). Mercury isotope compositions across North American forests. Global Biogeochem. Cycles 30, 1475–1492. doi:10.1111/1462-2920.13280.
Zhou, J., Du, B., Wang, Z., Zhang, W., Xu, L., Fan, X., et al. (2019). Distributions and pools of lead (Pb) in a terrestrial forest ecosystem with highly elevated atmospheric Pb deposition and ecological risks to insects. Sci. Total Environ. 647, 932–941. doi:10.1016/j.scitotenv.2018.08.091.
Keywords: bioaccumulator, lichens, mosses, soils, mercury, lead, isotopes, forest ecosystem
Citation: Barre JPG, Queipo-Abad S, Sola-Larrañaga C, Deletraz G, Bérail S, Tessier E, Elustondo Valencia D, Santamaría JM, de Diego A and Amouroux D (2020) Comparison of the Isotopic Composition of Hg and Pb in Two Atmospheric Bioaccumulators in a Pyrenean Beech Forest (Iraty Forest, Western Pyrenees, France/Spain). Front. Environ. Chem. 1:582001. doi: 10.3389/fenvc.2020.582001
Received: 10 July 2020; Accepted: 30 October 2020;
Published: 23 November 2020.
Edited by:
Marc Amyot, Université de Montréal, CanadaReviewed by:
Jun Zhou, University of Massachusetts Lowell, United StatesJoão Canário, University of Lisbon, Portugal
Copyright © 2020 Barre, Queipo-Abad, Sola-Larrañaga, Deletraz, Bérail, Tessier, Elustondo Valencia, Santamaría, De Diego and Amouroux. This is an open-access article distributed under the terms of the Creative Commons Attribution License (CC BY). The use, distribution or reproduction in other forums is permitted, provided the original author(s) and the copyright owner(s) are credited and that the original publication in this journal is cited, in accordance with accepted academic practice. No use, distribution or reproduction is permitted which does not comply with these terms.
*Correspondence: David Amouroux, ZGF2aWQuYW1vdXJvdXhAdW5pdi1wYXUuZnI=, Silvia Queipo-Abad, c2lsdmlhLnF1ZWlwby1hYmFkQHVuaXYtcGF1LmZy