- China Southern Power Grid Digital Grid Research Institute Co., Ltd., Guangzhou, China
More than 40% of China’s emissions come from the power industry, and regional power grids are crucial to the country’s ability to transmit and distribute electricity. However, there is a lack of a complete framework on electricity-related carbon emissions, accounting for regional power grids across different stages of electricity production and distribution. In this study, we propose a framework for accounting for carbon emissions in electricity for regional power networks. Using the Southern Power Grid (SPG) as a case study, we estimate carbon intensity across various stages of electricity generation, supply, and consumption. We incorporate the temporal and regional variations to analyze carbon emissions across five provinces (Guangdong, Guangxi, Yunnan, Guizhou, and Hainan), identifying key trends and factors that influence carbon intensity. Our framework integrates data from multiple sources, including real-world emissions and renewable energy inputs, while validating results through method comparisons and real-time policy alignment. The results reveal significant disparities in carbon intensity reduction across provinces, with Yunnan achieving the most notable reduction and Guangdong showing the highest emissions on the consumption side. Our framework is versatile, reliable, and applicable to other regional grids in China, offering a critical tool for projecting future electricity-related carbon emissions and supporting the nation’s carbon neutrality goals.
1 Introduction
The accounting of carbon emissions in the power sector is crucial for tracking progress toward carbon reduction goals, especially in countries with large and complex power systems like China. The power sector is the largest contributor to carbon emissions in China, accounting for more than 40% of the nation’s total emissions (Huo et al., 2022; Liu et al., 2020; Liu et al., 2022; Liu et al., 2023; IEA, 2022). As China aims to achieve carbon neutrality by 2060, establishing accurate and comprehensive carbon accounting frameworks for the electricity sector is of paramount importance (Liu et al., 2015; Fu et al., 2022; Li et al., 2022; Liang et al., 2023; Cai et al., 2022). However, the complexity of regional power grids, which involves inter-regional electricity transfers and a mix of renewable and non-renewable energy sources, presents significant challenges for traditional carbon accounting methods (Wang et al., 2021; Ma, 2022; Yan et al., 2016; Zhang and Li, 2022; He et al., 2023).
Despite the recognized importance of carbon accounting in the power sector, current frameworks often lack the comprehensiveness required to capture the full scope of emissions from regional power grids. Most studies and existing frameworks focus on individual stages of electricity use (Davis and Caldeira, 2010; Shan et al., 2018; Shan et al., 2020), such as power generation or consumption, while neglecting the interconnected nature of the grid system, which involves electricity flows between regions, the use of both renewable and non-renewable energy sources, and the influence of supply-side factors. Although Scope 1 (direct emissions from owned or controlled sources) and Scope 2 (indirect emissions from purchased electricity) accounting methods are commonly applied in carbon tracking, these methods do not provide a holistic view of the emissions associated with regional grid operations. Although some literature studies have focused on carbon intensity estimates for regional grids, considering energy type-specific capacities (Chen R. et al., 2023) and green energy transmission (Chen X. et al., 2023), or constructing node-specific quasi-input–output models for precise grid-side emissions (He et al., 2023), most estimation of grid emissions is still directly using the average carbon intensity of the regional grid (Yang, 2023). Thus, few studies have integrated all three key stages—production, supply, and consumption—into a unified framework that accounts for regional variations and the unique energy dynamics of different provinces. This gap in the literature highlights the need for a comprehensive carbon accounting framework that harmonizes these perspectives, especially in regions like southern China, where electricity generation is highly diversified and inter-regional power transfers are common.
In response to this gap, we propose a novel carbon accounting framework specifically designed to assess carbon emissions across all stages of electricity use in regional power grids by defining three carbon emission accounting scopes, i.e., generation-side, supply-side, and consumption side. Specifically, the Southern Power Grid (SPG) is selected as the case study for this framework as it represents a unique and diverse regional grid that spans five provinces: Guangdong, Guangxi, Yunnan, Guizhou, and Hainan. These provinces differ significantly in terms of their energy profiles, with Yunnan and Guizhou relying heavily on hydropower, while Guangdong, as one of China’s most economically developed provinces, imports substantial amounts of electricity to meet its industrial demand. The SPG also plays a critical role in China’s West–East Electricity Transfer Project, facilitating the transmission of cleaner energy from western provinces to the more developed coastal regions in the east. The complex energy mix and inter-regional electricity flows within the SPG make it an ideal representative for studying the challenges and opportunities in carbon accounting for regional grids (Jiang et al., 2016; Zhou B. et al., 2018; Zhou et al., 2016; Zhou L. et al., 2018). Moreover, the availability of detailed data on energy production, consumption, and inter-regional transfers in the SPG allows for a more accurate application of the proposed framework.
This paper is structured as follows: Section 2 outlines the methodology used to develop the carbon accounting framework, including the key parameters and data sources for emissions tracking. Section 3 presents the results from applying the framework to the Southern Power Grid, including a detailed analysis of carbon intensity and emissions across the stages of electricity generation, supply, and consumption. Section 4 discusses the key findings and implications for regional power grids and emphasizes the need for a standardized carbon accounting system for regional grids.
2 Materials and methods
2.1 Carbon accounting scopes for the three perspectives
In this study, we have developed an electricity carbon accounting framework by integrating carbon accounting methods, such as Scope 1 and Scope 2, with the key characteristics of energy flow within the grid. Specifically, we have estimated the emissions and carbon intensity for power generation, electricity supply, and electricity consumption, respectively. The delineation of the accounting scopes for these three stages is shown in Figure 1.
For power generation, we adopt the Scope 1 methodology, as described in Section 2.2. For electricity supply, we integrate scopes 1 and 2, considering both regional generation and purchased thermal power (detailed in Section 2.3). For electricity consumption, we calculate emissions from sold electricity to estimate the emissions associated with regional electricity consumption (Section 2.4). Due to data limitations, adjusted state grid carbon intensity is applied to account for the potential contribution of renewable energy in China. This framework thus incorporates renewable energy while harmonizing carbon intensity calculations for generation, supply, and consumption based on data from regional grids.
2.2 Generation-side emissions
We first estimate the carbon intensity of power generation. To calculate the carbon intensity for power generation, we utilize statistical data from the Southern Power Grid on the standard coal consumption per unit of electricity generation for each fuel and province. Based on these data, we convert the consumption values into carbon emissions per unit of electricity generated. Specifically, we apply emission factors for standard coal equivalent consumption, which are 2.66, 1.73, and 1.56 tCO2 per ton of standard coal equivalent (tce) for coal, oil, and natural gas, respectively. These factors are sourced from the “Provincial Carbon Peaking Action Plan Preparation Guide” issued by the Ministry of Ecology and Environment in February 2021. By multiplying the provincial standard coal consumption per unit of power generation with the corresponding emission factors, we obtain the carbon intensity of power generation for each fuel and province (
where
Generation-side carbon emissions (
where
2.3 Supply-side emissions
Supply-side emissions consider the carbon footprint from both locally generated power and purchased electricity. The total supplyside emissions for a province are (Equation 3):
For domestic generation, carbon intensity is the same as generation-side carbon intensity (Equation 4):
The domestic generation emissions, excluding exported electricity, are (Equation 5):
where
For emissions purchased from grids outside the selected provinces, we first clarify two key terms commonly used in Chinese electricity grid enterprise: point-to-grid mode and grid-to-grid mode. The grid-to-grid mode refers to a transmission approach where the power source is connected directly to the sending-end power system. In contrast, the point-to-grid mode involves transmitting power via an independent power line from the power source to the electrical load center. The grid-to-grid mode enhances the utilization of transmission lines and facilitates electricity exchanges between grids to optimize peak demand. It is important to note that some purchased electricity (and associated emissions) may be transmitted through the grid-to-grid mode from other regional grids outside the Southern Power Grid’s coverage. To avoid confusion, we will describe this as “from the other regional grids in China to the Southern Power Grid.” Thus,
where
Thus, the provincial supply-side carbon intensity (
where
Additionally, emissions due to transmission losses or “grid-side carbon emissions” are calculated. Since these emissions arise from the electricity supply, they are calculated using the carbon intensity of electricity supply with the following equation (Equation 8):
2.4 Consumption-side emissions
According to Figure 1, consumption-side emissions for each province are calculated as Equation 9
The carbon intensity for electricity consumption is calculated using the following equation (Equation 10):
Based on this, we attribute carbon emissions to different end-use sectors. We assume that the total carbon emissions of all sectors, as estimated in this study, include both emissions caused by electricity consumption (
For emissions from electricity consumption, it can be calculated as Equation 12:
where
For emissions from other energy sources, the data are taken from the CEADs database. Sectoral carbon emissions from other sources are taken as the corresponding sectoral emissions values in the CEADs database in 2019. Therefore, the carbon emission ratio of the sectoral electricity consumption (
Local- and municipal-level sectoral purchased electricity is used, including 133 sectors according to the national standard and 48 sectors in the CEADs database. We aggregated the sectors into eight industries (i.e., building materials, chemical, civil aviation, electricity generation, ferrous, petrochemicals, pulp and paper, and steel) to present the consumption-side emissions of each province (detailed in Section 3.2.3).
3 Results
We calculated the carbon intensity of the Southern Power Grid, including the carbon intensity of power generation, electricity supply, and electricity consumption. In addition, the carbon emissions were calculated across the generation side, supply, grid, and consumption side of the Southern Power Grid. Section 3.1 presents carbon intensity results, while Section 3.2 discusses regional-level electricity carbon emissions.
3.1 Carbon intensity of the Southern Power Grid
Figure 2 highlights trends in the carbon intensity of electricity supply between 2005 and 2020. Across all provinces and the entire Southern Power Grid, carbon intensity exhibited a clear downward trend. The overall trend for the entire Southern Power Grid showed steady but moderate decreases, reflecting ongoing decarbonization efforts. These efforts are primarily driven by the increasing deployment of renewable energy, improvements in energy efficiency, and governmental policies aimed at reducing carbon emissions, although challenges such as regional disparities in resource availability and industrial demands have influenced the rate of progress.
Yunnan saw the most significant reduction of about 80%, which can be largely attributed to the province’s abundant hydropower resources and its increasing reliance on renewable energy sources. The expansion of hydropower infrastructure and reduced reliance on coal-based power generation played a key role in Yunnan’s steep decline in carbon intensity. In contrast, Guangxi showed an initial 40% reduction between 2005 and 2008, driven primarily by energy efficiency improvements and the adoption of cleaner energy sources in the early years. However, the subsequent fluctuations in Guangxi’s carbon intensity could be linked to varying levels of industrial activity and changes in energy demand, which periodically increased reliance on fossil fuels. Nonetheless, yet still ended with a 50% reduction by 2020. Guizhou displayed a more gradual decrease, reaching a decrease of approximately 40% by 2020. Although Hainan experienced minor fluctuations, it still achieved a 10% reduction by 2020. The smaller decrease in carbon intensity may be due to its geographical constraints and limited renewable energy resources. However, the island’s efforts to shift toward solar and wind energy in recent years, alongside energy-saving policies, helped stabilize and slightly reduce its carbon intensity.
3.2 Provincial characteristics of carbon emissions
3.2.1 Provincial emissions under different accounting scopes
Our comparative analysis for 2021 examines carbon emissions across the Southern Power Grid from three perspectives (Figure 3). Guangdong’s consumption-side emissions were the highest, exceeding supply-side emissions by 6% and generation-side emissions by 11%. These discrepancies reflect the interprovincial electricity imports, as outlined in the West–East Electricity Transmission plan in the Southern Power Grid Tenth Five-Year Plan (Dong Da Electricity, 2020). Guangdong, as an industrial and economic hub, faces high electricity demand but lacks sufficient local generation capacity, especially from low-emission sources. Consequently, it imports substantial amounts of electricity, including both renewable energy and high-emission intensity electricity from other provinces, leading to higher consumption-side emissions.
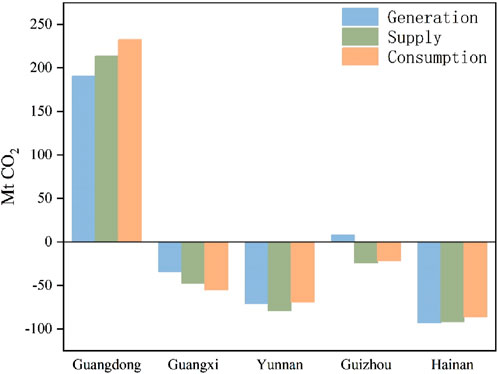
Figure 3. Carbon emission variation across five provinces relative to the Southern Power Grid average from the three perspectives, i.e., generation-side (blue bars), supply-side (green bars), and consumption-side (red bars).
Conversely, in the other four provinces, consumption-side emissions were the lowest, followed by supply-side emissions second and generation-side emissions—the highest. This suggests that high-emission intensity electricity is exported to Guangdong, while renewable energy is consumed locally. Provinces like Yunnan, which have abundant hydropower resources, generate cleaner energy locally and export fossil-fuel-based electricity, resulting in lower consumption-side emissions but higher generation-side emissions.
The total emissions of the five provinces follow a similar trend, where consumption-side emissions are lower than production-side emissions, indicating that some production-side emissions are displaced to meet electricity demands in other regions. This finding aligns with China’s electricity transfer strategies and underscores the importance of interregional electricity flows in shaping emissions dynamics.
3.2.2 Provincial shares of carbon emissions
We present the provincial shares of the generation-side, supply-side, grid-side, and consumption-side carbon emissions for the Southern Power Grid in 2021 (Figure 4). In 2021, Guangdong contributed the largest share of the generation-side carbon emissions at 55.5%, followed by Guizhou (21.5%), Guangxi (13.6%), Yunnan (6.8%), and Hainan (2.7%). For supply-side emissions, Guangdong again led with 64.9%, followed by Guizhou (16.2%), Guangxi (11.5%), Yunnan (5%), and Hainan (2.5%). The same pattern is observed for the grid-side emissions, with Guangdong at 62.8%, followed by Guizhou (23%), Guangxi (9.6%), Yunnan (4.2%), and Hainan (0.4%). On the consumption side, Guangdong maintained the largest share at 67%, followed by Guizhou (15.6%), Guangxi (8.8%), Yunnan (6%), and Hainan (2.5%). For all perspectives, Guangdong consistently held the largest share.
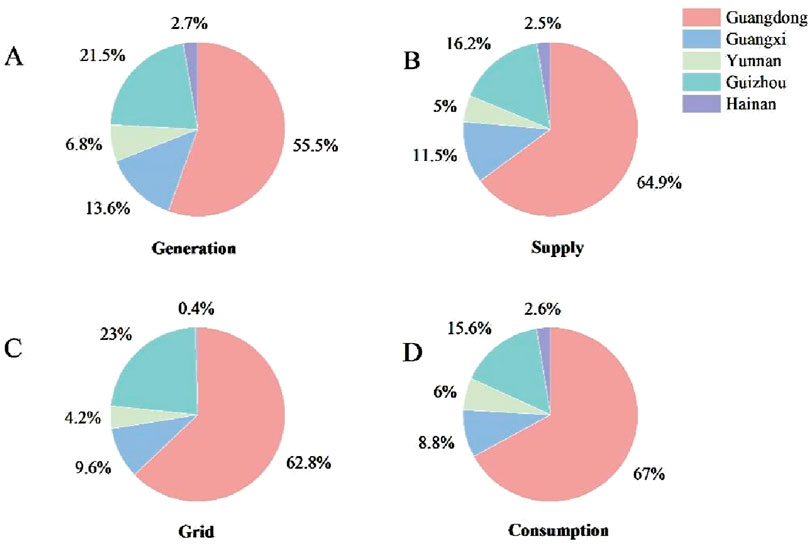
Figure 4. Shares of carbon emission from (A) generation, (B) supply, (C) grid, and (D) consumption perspectives for five provinces in 2021.
This pattern can be attributed to Guangdong’s role as the economic powerhouse of the Southern Power Grid region, with its energy-intensive industries and large population-driving substantial electricity demand. Despite its efforts to transition toward cleaner energy sources, Guangdong still relies heavily on fossil fuel-based power generation to meet its vast energy needs, resulting in its high share of generation-side emissions. Additionally, Guangdong imports significant amounts of electricity from other provinces under the West–East Electricity Transmission plan, which further contributes to its supply-side and grid-side emissions. The province’s prominent industrial sector and economic activities ensure that it also holds the largest share of consumption-side emissions, reflecting its high electricity usage.
Guizhou’s relatively high share of generation-side and supply-side emissions (21.5% and 16.2%, respectively) is due to its heavy reliance on coal-fired power plants as it is a major coal-producing region. Although Guizhou exports much of its electricity, its lower consumption-side emissions reflect its smaller population and industrial base compared to Guangdong. In contrast, Guangxi’s moderate share of emissions (13.6% generation-side and 11.5% supply-side) reflects its role as both a producer and consumer of electricity, with a mix of fossil fuels and renewable energy. Although Guangxi exports energy to Guangdong, its overall lower demand keeps consumption-side emissions relatively low. Yunnan, with its abundant hydropower resources, shows a smaller share of generation-side (6.8%) and grid-side (4.2%) emissions as it heavily relies on renewable energy and exports clean electricity. Hainan, with the smallest shares across all perspectives, reflects its lower population, limited industrialization, and constrained energy generation capacity, resulting in minimal emissions. Together, these provinces illustrate the diverse energy profiles within the Southern Power Grid, driven by local resource availability and regional electricity trade.
3.2.3 Sectoral contribution to the provincial consumption-side emissions
We examined emissions from eight sectors: building materials, chemical, civil aviation, electricity generation, ferrous, petrochemicals, pulp and paper, and steel (Figure 5). The chemical sector is the dominant contributor to carbon emissions in each province due to the high electricity demand for processes like heating, distillation, and refrigeration. These processes require large amounts of continuous energy, making the sector particularly carbon-intensive, especially in provinces like Guangdong, which has a large industrial base driven by the chemical industry. The prominence of the chemical sector in Guangdong, where it accounts for around 40% of total emissions, is a direct reflection of the province’s industrial composition and its reliance on electricity to support production.
The pulp and paper sector also contributes significantly, particularly in Guangdong and Guizhou, where it accounts for 50% and 27.3% of total sector emission, respectively. The substantial share in Guangdong can be attributed to the high demand for paper products due to the region’s large consumer market and industrial base. In Guizhou, the pulp and paper industry is similarly energy-intensive, relying heavily on electricity for operations such as mechanical pulping and paper drying, which drive up emissions.
In Yunnan, Guangxi, and Guizhou, the ferrous sector’s emissions are substantial, largely because of the power-intensive nature of ferrous metal production, including iron and steel manufacturing, which requires continuous high-energy processes such as smelting and rolling. These provinces rank 3rd, 7th, and 13th in China for ferrous production in 2022 (China Securities Data, 2023), making the sector a key contributor to their overall emissions. The reliance on coal-based electricity to power these operations exacerbates the sector’s carbon footprint, especially in regions where cleaner energy sources are less prevalent.
In Guangxi, the ferrous (36.6%) and steel (30.4%) industries contribute the most, due to the province’s position as a major player in steel production, which is energy-intensive and highly dependent on electricity. In Yunnan and Guizhou, the ferrous sector is responsible for 54.7% and 46.1% of total electricity-related emissions, respectively. These trends reflect the sectoral reliance on electricity and highlight the significant role of specific industries, such as pulp and paper and ferrous metals, in overall provincial emissions.
4 Conclusion and discussion
4.1 Key findings and implications
In this study, we developed a comprehensive electricity carbon accounting framework, specifically applying it to the Southern Power Grid as a case study, to assess carbon emissions of regional power grids across different stages of electricity production, transmission, and consumption. The framework integrates four critical perspectives, i.e., generation, supply, grid, and consumption. Our analysis of five provinces (Guangdong, Guangxi, Yunnan, Guizhou, and Hainan) reveals notable differences in carbon intensity reduction and emission patterns across regions.
Our findings show that Yunnan achieved the most significant reduction in carbon intensity, with an approximate 80% decrease between 2005 and 2020, largely due to its reliance on renewable energy, particularly hydropower. In contrast, Guangdong, as the largest electricity consumer, had much higher emissions on the consumption side, with a reduction of only around 50% over the same period, primarily due to its dependence on imported electricity and industrial demand. This reliance on external power, much of which was generated from fossil fuels, resulted in a mismatch between Guangdong’s local generation emissions and consumption emissions, emphasizing the importance of accounting for inter-regional electricity transfers when assessing carbon emissions.
The findings underscore the critical role that renewable energy integration plays in reducing carbon intensity. Provinces with higher shares of renewable energy, such as Yunnan, consistently showed lower carbon emissions, demonstrating the need for more aggressive renewable energy strategies in regions like Guangdong. The analysis also highlights the complex role of inter-regional electricity transfers as provinces like Guangdong benefit from electricity imports but shift their emissions burden to the exporting regions, such as Yunnan and Guizhou. This emphasizes the importance of a comprehensive approach to carbon accounting that fully captures electricity flows between regions.
The framework provides a reliable tool for understanding the emission landscape across regional power grids, enabling more targeted decarbonization strategies. Provinces like Guangdong must prioritize increasing local renewable energy generation and improving energy efficiency in high-demand sectors to achieve significant reductions in emissions. The framework supports China’s broader carbon neutrality goals by offering a structured method for tracking and reducing emissions at the regional level. Moreover, this method is adaptable and can be applied to the other regional grids both within and outside China, making it a valuable tool for global efforts in decarbonizing the power sector.
4.2 Limitations and future work
However, while the framework was designed based on the Southern Power Grid, its application to other regional grids may require modifications, particularly regarding data sources and specific methodological adjustments. Nevertheless, our validation efforts—such as comparing different methods and aligning results with real-world data and policies—demonstrate the framework’s reliability and feasibility for other grids, provided the necessary data and methodological adaptations are made.
Looking ahead, there is a pressing need for a standard carbon accounting framework that encourages grid-operating enterprises to publish key data, facilitating cross-comparisons and validation of emissions data. We hope this framework serves as a foundational step toward such a framework, offering insights for future electricity carbon accounting. Our framework can act as a reference point for future research in this area, helping drive open-source data initiatives and refinements in carbon accounting methodologies.
Data availability statement
The raw data supporting the conclusions of this article will be made available by the authors, without undue reservation.
Author contributions
YH: conceptualization, writing–original draft, and writing–review and editing. XH: data curation, methodology, and writing–original draft. FZ: data curation, methodology, and writing–original draft. SP: writing–original draft. JT: writing–original draft.
Funding
The author(s) declare that no financial support was received for the research, authorship, and/or publication of this article.
Conflict of interest
Authors YH, XH, FZ, SP, and JT were employed by China Southern Power Grid Digital Grid Research Institute Co., Ltd.
Publisher’s note
All claims expressed in this article are solely those of the authors and do not necessarily represent those of their affiliated organizations, or those of the publisher, the editors, and the reviewers. Any product that may be evaluated in this article, or claim that may be made by its manufacturer, is not guaranteed or endorsed by the publisher.
References
Cai, B., Liu, H., Zhang, X., Pan, H., Zhao, M., Zheng, T., et al. (2022). High-resolution accounting of urban emissions in China. Appl. Energy 325, 119896. doi:10.1016/j.apenergy.2022.119896
Chen, R., Xu, P., and Yao, H. (2023). Decarbonization of China's regional power grid by 2050 in the government development planning scenario. Environ. Impact Assess. Rev. 101, 107129. doi:10.1016/j.eiar.2023.107129
Chen X., X., Ge, J., Huang, J., Lai, Y., and Xue, F. (2023). “A method for calculating the average carbon emission factor of interconnected power grids considering green electricity trading,” in Second International Conference on Energy, Power, and Electrical Technology (ICEPET 2023) (SPIE), 1172–1179.
CHINA SECURITIES DATA (2023). Ranking of ten kinds of ferrous metal production in each province of China (in Chinese). Available at: https://www.phb123.com/keji/ziran/65821.html (Accessed October 12, 2023).
Davis, S. J., and Caldeira, K. (2010). Consumption-based accounting of CO2 emissions. Proc. Natl. Acad. Sci. 107, 5687–5692. doi:10.1073/pnas.0906974107
DONG DA ELECTRICITY (2020). West-East Electricity transmission by my side: West-East Electricity transmission south line Project (in Chinese). Available at: https://www.sohu.com/a/373350069_99941458 (Accessed October 12, 2023).
Fu, J., Ding, R., Zhang, Y., Zhou, T., Du, Y., Zhu, Y., et al. (2022). The spatial-temporal transition and influencing factors of green and low-carbon utilization efficiency of urban land in China under the goal of carbon neutralization. Int. J. Environ. Res. Public Health 19, 16149. doi:10.3390/ijerph192316149
He, H., Zhou, S., Zhang, L., Zhao, W., and Xiao, X. (2023). Dynamic accounting model and method for carbon emissions on the power grid side. Energies 16, 5016. doi:10.3390/en16135016
Huo, D., Liu, K., Liu, J., Huang, Y., Sun, T., Sun, Y., et al. (2022). Near-real-time daily estimates of fossil fuel CO(2) emissions from major high-emission cities in China. Sci. Data 9, 684. doi:10.1038/s41597-022-01796-3
Jiang, T., Mu, Y., Jia, H., Lu, N., Yuan, H., Yan, J., et al. (2016). A novel dominant mode estimation method for analyzing inter-area oscillation in China southern power grid. IEEE Trans. Smart Grid 7, 2549–2560. doi:10.1109/tsg.2016.2533621
Liang, X., Xu, Z., Wang, Z., and Wei, Z. (2023). Low-carbon economic growth in Chinese cities: a case study in Shenzhen city. Environ. Sci. Pollut. Res. 30, 25740–25754. doi:10.1007/s11356-022-24001-9
Li, J., Wei, Y.-M., Liu, L., Li, X., and Yan, R. (2022). The carbon footprint and cost of coal-based hydrogen production with and without carbon capture and storage technology in China. J. Clean. Prod. 362, 132514. doi:10.1016/j.jclepro.2022.132514
Liu, Z., Ciais, P., Deng, Z., Lei, R., Davis, S. J., Feng, S., et al. (2020). Near-real-time monitoring of global CO 2 emissions reveals the effects of the COVID-19 pandemic. Nat. Commun. 11, 5172–5212. doi:10.1038/s41467-020-18922-7
Liu, Z., Deng, Z., Davis, S. J., Giron, C., and Ciais, P. (2022). Monitoring global carbon emissions in 2021. Nat. Rev. Earth Environ. 3, 217–219. doi:10.1038/s43017-022-00285-w
Liu, Z., Deng, Z., Davis, S., and Ciais, P. (2023). Monitoring global carbon emissions in 2022. Nat. Rev. Earth and Environ. 4, 205–206. doi:10.1038/s43017-023-00406-z
Liu, Z., Guan, D., Moore, S., Lee, H., Su, J., and Zhang, Q. (2015). Climate policy: steps to China's carbon peak. Nature 522, 279–281. doi:10.1038/522279a
Ma, J.-J. (2022). Who shapes the embodied carbon dioxide emissions of interconnected power grids in China? A seasonal perspective. J. Environ. Manag. 324, 116422. doi:10.1016/j.jenvman.2022.116422
Shan, Y., Guan, D., Zheng, H., Ou, J., Li, Y., Meng, J., et al. (2018). China CO 2 emission accounts 1997–2015. Sci. Data 5, 170201–170214. doi:10.1038/sdata.2017.201
Shan, Y., Huang, Q., Guan, D., and Hubacek, K. (2020). China CO 2 emission accounts 2016–2017. Sci. Data 7, 54–59. doi:10.1038/s41597-020-0393-y
Wang, P., Lin, C.-K., Wang, Y., Liu, D., Song, D., and Wu, T. (2021). Location-specific co-benefits of carbon emissions reduction from coal-fired power plants in China. Nat. Commun. 12, 6948. doi:10.1038/s41467-021-27252-1
Yang, F. (2023). “Research on power carbon emission accounting method for a new type of power system construction,” in 2023 6th Asia Conference on Energy and Electrical Engineering (ACEEE) (IEEE), 551–556.
Yan, Q., Zhang, Q., and Zou, X. (2016). Decomposition analysis of carbon dioxide emissions in China's regional thermal electricity generation, 2000–2020. Energy 112, 788–794. doi:10.1016/j.energy.2016.06.136
Zhang, H., and Li, S. (2022). Carbon emissions’ spatial-temporal heterogeneity and identification from rural energy consumption in China. J. Environ. Manag. 304, 114286. doi:10.1016/j.jenvman.2021.114286
Zhou, B., Rao, H., Wu, W., Wang, T., Hong, C., Huang, D., et al. (2018). Principle and application of asynchronous operation of China southern power grid. IEEE J. Emerg. Sel. Top. Power Electron. 6, 1032–1040. doi:10.1109/jestpe.2018.2830409
Zhou, H., Su, Y., Chen, Y., Ma, Q., and Mo, W. (2016). The China southern power grid: solutions to operation risks and planning challenges. IEEE Power Energy Mag. 14, 72–78. doi:10.1109/mpe.2016.2547283
Keywords: CO2 emissions, power grid, China, Southern China Grid, accounting scopes
Citation: Huang Y, Hu X, Zhang F, Pan S and Tan J (2024) Electricity carbon accounting framework for China’s regional power grids: a case study of the Southern Power Grid. Front. Energy Res. 12:1479316. doi: 10.3389/fenrg.2024.1479316
Received: 13 August 2024; Accepted: 30 October 2024;
Published: 27 November 2024.
Edited by:
Feng Zhang, Shandong University, ChinaReviewed by:
Ruiling Han, Hebei Normal University, ChinaMuren Bai, North China Electric Power University, China
Copyright © 2024 Huang, Hu, Zhang, Pan and Tan. This is an open-access article distributed under the terms of the Creative Commons Attribution License (CC BY). The use, distribution or reproduction in other forums is permitted, provided the original author(s) and the copyright owner(s) are credited and that the original publication in this journal is cited, in accordance with accepted academic practice. No use, distribution or reproduction is permitted which does not comply with these terms.
*Correspondence: Yanlu Huang, aHVhbmd5bEBjc2cuY24=