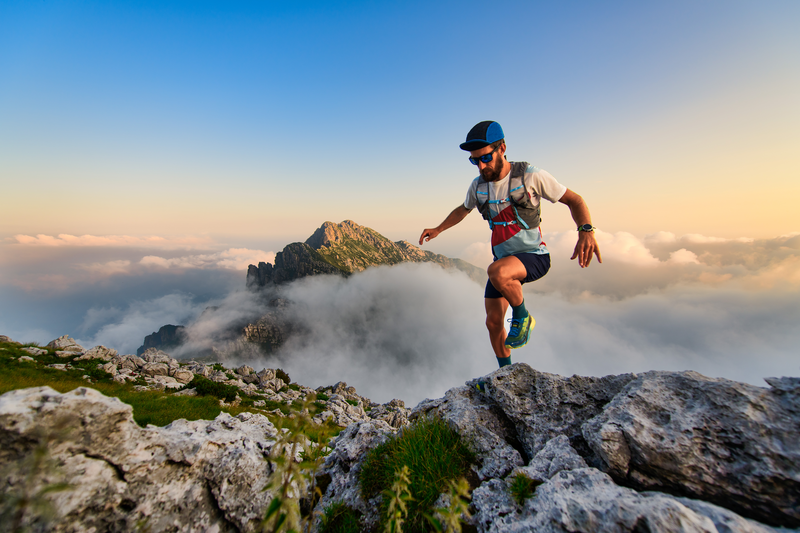
94% of researchers rate our articles as excellent or good
Learn more about the work of our research integrity team to safeguard the quality of each article we publish.
Find out more
ORIGINAL RESEARCH article
Front. Energy Res. , 05 December 2024
Sec. Sustainable Energy Systems
Volume 12 - 2024 | https://doi.org/10.3389/fenrg.2024.1457228
This article is part of the Research Topic Sustainable Energy Governance in the Context of Global Climate Change: Technologies, Mechanisms and Strategies View all 14 articles
This paper presents a comprehensive analysis of foldable solar panels used in agrivoltaics systems (AVS), focusing on the dual benefits of optimized land use for agriculture and solar power generation. Employing simulation techniques, the study investigates the impact of inter-panel shadow effects on power generation in systems using multiple foldable solar panels. Key findings indicate that foldable panels achieve optimal performance during periods with shorter daylight hours, demonstrating high seasonal variability in power generation. The study shows that foldable panels, which can adjust their angle relative to the sun’s position, are particularly effective at reducing the loss of solar irradiance due to shading, compared to fixed panels. Additionally, this study highlights the potential of foldable solar panel systems in AVS to adapt to varying solar conditions, thereby enhancing land use optimization for sustainable agricultural and power generation practices.
Agrivoltaics system (AVS) integrates crop production and photovoltaic (PV) power generation offering a promising solution to the dual challenge of producing enough food and renewable energy in limited land resources (Dupraz et al., 2011; Weselek et al., 2019; Toledo and Scognamiglio, 2021). Due to the potential to provide mutual benefits across the food-energy-water nexus, AVS has gained significant attention in recent days (Goetzberger and Zastrow, 1982) (Sarr et al., 2023) (Widmer et al., 2024). Economic analyses indicate that AVS can provide substantial financial returns, with profitability greatly influenced by factors such as feed-in tariffs and financing mechanisms (Chalgynbayeva et al., 2024). These systems contribute to sustainable development by improving land use efficiency, reducing water consumption, and enhancing the climate resilience of crops (Asa’a et al., 2024). Moreover, technological advancements, including wavelength-selective and spectral-shifting photovoltaics, have not only improved the feasibility and efficiency of AVS but also enhanced their compatibility with a diverse range of agricultural needs (Stallknecht et al., 2023). Recent developments in data-driven approaches (Mengi et al., 2023) and IoT-based systems (Zito et al., 2024) have further advanced AVS. The placement and configuration of solar panels in AVS play a crucial role in their efficiency and the benefits they can provide. Based on the arrangement of PV panels, AVS can be categorized into several types, each with its advantages and drawbacks (Trommsdorff et al., 2022). In the vertical mounting of PV-based configuration, solar panels are installed vertically, rather than the traditional horizontal layout. This setup can be particularly advantageous in narrow or limited spaces, allowing for agriculture to take place on either side of the panels. It can also reduce shading, thus minimizing the impact on crop growth, while producing solar energy efficiently. The plant-between PV setup involves arranging solar panels with sufficient space between them, allowing crops to be planted in the gaps. This configuration optimizes land use by integrating solar energy production and agriculture in the same area. The spacing must be carefully planned to ensure that plants receive adequate sunlight while maximizing the solar panels’ exposure to light. In plants under PV systems, crops are grown directly beneath the solar panels. This arrangement can protect plants from excessive sunlight and reduce water usage due to lower evaporation rates. However, it requires careful selection of crops that can thrive under reduced light conditions and optimization of panel spacing and orientation to ensure adequate light reaches the plants. In a greenhouse under PV, solar panels are installed above the greenhouse in this configuration, providing power for greenhouse operations and potentially to the grid, while the greenhouse environment supports agricultural production. This setup can offer controlled environmental conditions for crops, extend growing seasons, and reduce the greenhouse’s energy consumption. Solar tracking systems adjust the orientation of solar panels throughout the day to follow the sun’s path, maximizing energy capture. Single-axis trackers rotate in one direction (e.g., east to west), while dual-axis trackers can also adjust tilt angles. When integrated into AVS, tracking can increase energy production but requires careful consideration of shadow movement to minimize adverse effects on crops.
A recent innovation, foldable panel-based systems, as shown in Figure 1 allows for the dynamic adjustment of solar panel coverage based on the needs of the crops beneath (Lama and Jeong, 2024). These systems can provide full sunlight when necessary and shade during excessively sunny conditions, offering a highly adaptable agrivoltaics solution. The concept of foldable panel-based agrivoltaics represents a significant advancement in integrating solar energy production with agriculture, offering a flexible solution to manage sunlight exposure for crops while optimizing energy generation. The adaptability of foldable solar panels enables dynamic adjustments to the panels’ orientation and coverage, responding to the varying needs of crops throughout their growth cycles. Despite its potential, the implementation of foldable panel-based agrivoltaics is still in the early stages, with a need for comprehensive theoretical models and empirical data to fully understand its effectiveness and guide its deployment across different scenarios. This approach claims a gain of electric energy generation of up to 16% as compared to a horizontally fixed panel-based system (Lama and Jeong, 2024). However, the study was performed only during a small timeframe of the year. The claim is based on an empirical study, with no theoretical base. Thus, we present a theoretical model of a foldable panel. This model provides the power generation pattern for the entire year and can be tested anywhere on Earth. Additionally, we investigate the impact of inter-panel shadow effects on energy capture in systems using multiple foldable solar panels.
Studying shadow formation is crucial because shadows directly affect the efficiency of solar panels. When panels are shadowed, their ability to generate electricity decreases, which can significantly impact the overall energy output of solar installations. By understanding how and when shadows occur, systems can be designed to minimize these effects, improving both the reliability and efficiency of solar power systems.
Numerous simulation and computational modeling methods (Mengi et al., 2023)- (Amaducci et al., 2018) have been developed for optimization of AVS. This study in (Mengi et al., 2023) introduces a framework combining digital simulation and genomic optimization to design AVS, which integrates solar panels with crop production for enhanced land use efficiency. Using a case study of SunnySD tomatoes in Davis, California, the research demonstrates a 28.9% improvement in combined crop yield and energy production efficiency through genomic optimization over 50 generations. The optimization process effectively balances the demands of agricultural production and solar energy generation, employing nearly vertical, highly reflective panels as an optimal solution. The findings challenge traditional agrivoltaics theories by prioritizing photovoltaic performance while maintaining crop yield within acceptable limits. The research underscores the need for advanced crop models and suggests further enhancements to the digital replica for more accurate AVS design. A study (Riaz et al., 2022) shows a simulation model of the light productivity factor (LPF) as a novel metric to optimize sunlight sharing in AVs, aiming to enhance the symbiotic relationship between solar energy generation and crop production. The LPF is used to determine the optimal design parameters for AVs, such as PV array density and orientation, especially for crops with varying shade tolerance. The finding demonstrates that while full PV array density and standard sun tracking maximize LPF for shade-tolerant crops, customized configurations are required for crops with moderate to high shade sensitivity to ensure adequate PAR for photosynthesis. East/West facing bifacial vertical PV arrays emerge as a preferred option due to their minimal seasonal yield variability for shade-sensitive crops and additional benefits like reduced soiling and ease of farm equipment movement. The study underscores the potential of LPF in guiding the design of AVs for improved food and energy production, highlighting the need for future research to incorporate socio-economic factors for region-specific agrivoltaics development. The study (Robledo et al., 2021) explores the integration of vertical bifacial photovoltaic (PV) modules with agriculture, using advanced 3D simulation tools to enhance energy yield assessment in AVs. Key challenges identified include accurately modeling albedo effects and light reflections. The study also introduces a novel approach for calculating performance ratios to evaluate bifacial PV modules effectively. Through a case study in southern France, the authors underscore the importance of ongoing research and collaboration to optimize and validate AV designs incorporating bifacial technology. The simulation-based study in (Gonocruz et al., 2022) investigates the integration of AVs within rural farming areas in Japan, focusing on optimizing the power grid with storage batteries and enhanced transmission capacities. Findings indicate that agrivoltaics are especially effective in rice paddy fields, contributing to significant reductions in CO₂ emissions and excess power output when combined with battery storage and improved transmission. The research highlights the importance of strategic spatial planning and additional experimental studies to maximize the economic and environmental advantages of agrivoltaics within Japan’s energy landscape. The study (Potenza et al., 2022) evaluates the impact of varying shade depths from AVS soybean crop morphology, physiology, and yield, using both field experiments and a simulation platform integrating the GECROS crop model. It was found that increased shading from AVs led to significant morphological adaptations in soybeans, such as heightened plant growth and larger leaf area, although it resulted in an average yield reduction of 8%. The research validates the simulation platform as a useful tool for exploring different AV scenarios and agronomic practices, highlighting its potential to inform optimal AV design and management strategies within regulatory yield reduction thresholds. Agrovoltaico® (Amaducci et al., 2018) combines soil-grown maize with overhead photovoltaic panels, leveraging a 40-year climate dataset from northern Italy to simulate outcomes. Results indicate that maize yields were both higher and more stable under AVS than in full sunlight, particularly in rainfed conditions and during drought stress. This suggests that agrivoltaics can enhance crop resilience to climate change. Additionally, the land equivalent ratio (LER) for Agrovoltaico® scenarios consistently exceeded 1, demonstrating greater energy production per unit area compared to conventional energy and monoculture systems.
The foldable panels are designed by adapting to the sun’s position throughout the day to optimize both energy production and agricultural productivity.
The foldable solar panel system comprises bifacial panels connected via hinges, allowing them to fold. The system is mounted on a sliding base, enabling adjustment of the panels’ tilt angle as shown in Figure 2. This design ensures that one side of the bifacial panel captures sunlight from sunrise to noon, while the other side captures sunlight from noon until sunset. The adjustability in tilt angle is pivotal for balancing energy collection with the required sunlight exposure for crops beneath.
The operation of foldable solar panels is governed by a dynamic adjustment strategy, encapsulated in Algorithm 1. This strategy involves adjusting the tilt angle of the panels from 90° at sunrise to 0° at solar noon, and then back to 90° at sunset. This adjustment ensures maximum solar irradiation capture by optimally aligning the panels with the sun’s path across the sky.
Algorithm 1.Time-based Solar Tracking.
To estimate the power generation of both the east-facing and west-facing panels, we first need to determine the angle of incidence on the plane. This angle represents the angular difference between the panel’s normal and the sun’s elevation angle and is expressed as in Equation 1.
Where
Similarly, the normal vector of the panel is defined by the panel’s azimuth, denoted as
Given the zenith angle
In the given equation,
where
The value for solar constant
Based on Equation 4, we can estimate the total irradiation of east-facing and west-facing panels as
and
The system employs multiple foldable solar panels for large-scale power and agriculture generation. Algorithm 1 adjusts the panels at nearly 90° during the early morning and late afternoon. We analyze the impact of shadow in an AVS using foldable solar panels.
During the morning, the east-facing first panel generates shadow on the second, the second panel generates shadow on third and so on as shown in Figure 3. Similarly, at late noon, the west-facing panel generates a shadow on the second panel, and the second panel generates a shadow on the third panel. The shadow cast by one solar panel onto another can significantly affect the overall efficiency of the solar array. When panels are shadowed, their ability to generate electricity is reduced, directly impacting power generation efficiency.
Given the panel of length PL and width PB, the shadow of the first panel casts a shadow on the second panel placed at the distance of DP. Figure 4 shows the detailed three-dimensional view of the shadow of the length SL and width of the shadow SB cast on the second panel.
Mathematically, the length and the width of the shadow can be estimated as
As the area of the shadow increases, the shadow will be cast on the adjacent panel reducing the power generated by the panel.
Before conducting the simulation tests, we established the geographical coordinates for Suncheon, South Korea, where the experiments were implemented. The latitude and longitude of this location are 34.95 and 127.48, respectively, and the parameters detailed in Table 1 were set for the testing environment. Our experimental setup included two foldable modules, each measuring 1 m in width and 2.2 m in length, spaced 2.4 m apart. We employed the technique proposed by reference (alraven, 1978) to calculate the solar azimuth and the sun’s elevation. Using this method, we can estimate the position of the sun for any given year, month, day, and time. We can predict the sun’s position for an entire year, including future dates beyond the simulation period. Thus, the tests were performed on four significant days of the year: the summer and winter solstices, as well as the spring and autumn equinoxes of 2024 assuming the clear sky condition. Simulated power output data were generated for the entire year, including future dates beyond the day the simulation was conducted. Additionally, critical solar times, such as sunrise, sunset, and solar noon, were determined to adjust the panel angles according to Algorithm 1. The configurations tested included both single- and multi-panel models. The gain in power generation was calculated using the following equation:
where,
Here,
Figure 5 illustrates the daily power generation patterns of three different panel setups throughout four distinct days of the year: the summer solstice, the winter solstice, and the spring and autumn equinoxes of the entire year. For these tests, the azimuth angle of the panels was set at 90° north. The gains and losses observed in these setups are detailed quantitatively in Table 3. Figure 6 displays the power generation patterns on the same 4 days of the year as Figure 5 however with the panel azimuth angle adjusted to +15° from the east. This adjustment simulates the solar panel’s response to a slight eastward deviation from due north, providing insights into the energy capture efficiency under different solar exposures. Similarly, Figure 7 shows the power generation patterns for the panels set at an azimuth angle of −15° from the east, representing a westward orientation deviation.
Figure 5. Solar power generation pattern of single panel system. (A) Day of the year n = 80 (B) Day of the year n = 173 (C) Day of the year n = 266 (D) Day of the year n = 356.
Figure 7. Solar power generation pattern of single panel system (−15°). (A) Day of the year n = 80 (B) Day of the year n = 173 (C) Day of the year n = 266 (D) Day of the year n = 356.
Similar to the investigation in Figures 5–7 for single panels, Figures 8–10 display the power generation patterns for multiple modules set at different azimuth orientations: 0°, +15°, and −15° from the east, respectively. These figures show how shifting orientations influence shadow casting and energy efficiency, particularly during times of day when shadows are most impactful. Figure 8 shows a baseline orientation directly east, while Figures 9, 10 assess the effects of slight deviations to the east and west. These orientations provide insights into optimizing panel arrangements to mitigate shadow effects and maximize energy production in a multi-panel setup. Additionally, Table 2 quantifies the gains and losses in cumulative power generation, comparing foldable panels to fixed panels, thereby highlighting the benefits of dynamic adaptability in panel configurations to enhance solar yield under varying shadow conditions and orientations. For the comparison, we considered the single-axis method, taking into account whether the design is intended for implementation in AVS or power generation. Additionally, we examined whether power generation is affected by the shadows cast by adjacent panels. We selected three different methods of solar tracking for comparison, as shown in Table 2. The date of data collection was chosen to be close to the autumn equinox, except for the study in (Lama and Jeong, 2024). The tilted rotating axis and dual-axis method proposed in the study (Zhu et al., 2020) is superior in terms of power generation. However, if we analyze the installation method, it is not ideally suitable for AVS. Additionally, the study (Huang et al., 2023) includes an analysis of the shadow impact on adjacent panels. However, the feasibility of implementing this on an AVS system is still unclear.
Figure 8. Solar power generation pattern of a two-panel system. (A) Day of the year n = 80 (B) Day of the year n = 173 (C) Day of the year n = 266 (D) Day of the year n = 356.
Figure 9. Solar power generation pattern of single panel system (+15°). (A) Day of the year n = 80 (B) Day of the year n = 173 (C) Day of the year n = 266 (D) Day of the year n = 356.
Figure 10. Solar power generation pattern of single panel system (−15°). (A) Day of the year n = 80 (B) Day of the year n = 173 (C) Day of the year n = 266 (D) Day of the year n = 356.
Table 3 provides a detailed quantitative analysis of the cumulative power generation gains and losses associated with foldable versus fixed panel configurations under various azimuth angles throughout the year. It shows the performance of the solar panels on selected key days, representing different seasonal conditions: the 80th, 173rd, 265th, and 356th days of the year, which correspond to near the spring equinox, summer solstice, autumn equinox, and winter solstice, respectively.
This table shows that the foldable solar panels demonstrate significant seasonal variability in power generation, achieving their best performance during days with shorter daylight hours. This adaptability highlights their potential to optimize energy efficiency in AVS by effectively adjusting to varying solar conditions throughout the year.
The analysis of solar radiation gains from various panel configurations presented in Table 2 reveals significant insights into the performance dynamics of foldable solar panels across different times of the year. These results highlight the noticeable seasonal variability in power generation, with foldable panels demonstrating enhanced performance, particularly during days with shorter daylight hours. The data for days numbered n = 80, 173, 265, and 356 represent the ordinal dates in the year. Day 80 typically falls on March 21st, which is around the time of the spring equinox. Day 173 generally falls on June 22nd, near the summer solstice. Day 265 commonly occurs around September 22nd, close to the autumn equinox. Day 356 falls on December 22nd, near the winter solstice. These days are chosen because they are close to days of the astronomical events that mark the beginnings of the four seasons spring, summer, autumn, and winter. The performance metrics show that foldable panels configured at 90° (SPS and DPSSC) tend to exhibit increased gains during the winter solstice (n = 356) with 6.40% and 5.73% gains respectively, compared to significant losses during the summer solstice (n = 173). This pattern indicates the panels’ capacity to exploit the low solar elevation angles during winter, effectively reducing shadow impacts and optimizing direct solar interception.
Conversely, during the summer, when daylight hours are longer and the sun is at a higher elevation, the panels exhibit negative gains (e.g., −2.58% for SPS at 0° from the east), suggesting an inefficiency in energy capture. This could be attributed to prolonged exposure of the sun to fixed panels compared to dynamic panels and suboptimal angle of incidence to the sun’s rays, which might not be fully mitigated even with adjustable panel systems.
Moreover, the variability observed in the panel performances at different azimuth adjustments (15° and −15° from the east) further highlights the critical role of panel orientation in maximizing energy capture. Panels adjusted to 15° and −15° do not show improved performance near the equinoxes and face challenges during the summer and winter as well, emphasizing the need for dynamic adjustment capabilities in foldable panel systems to adapt to the changing position of the sun throughout the year.
Additionally, The DPSSC configurations show a consistent decrease in power generation across almost all measured days compared to the SPS configurations. For example, on the 173rd day of the year, corresponding to a high sun elevation near the summer solstice, the SPS (0°) configuration showed a decrease in power output of −2.58%, whereas the DPSSC (0°) exhibited a more substantial decrease of −5.43%. This pattern suggests that double panels, due to their setup, experience more noticeable shadowing effects that adversely affect their performance. The proximity of panels in DPSSC likely contributes to this phenomenon, where one panel casts a shadow on another, thereby reducing the irradiance received and, consequently, the power output. Since figures a and c from Figures 5–10 represent the power generation patterns during the spring and autumn equinoxes, and the Sun’s position is the same on both days, the power generation patterns appear similar.
In this study, we have discussed the simulation model of foldable solar panels and the impact of shadows solely in terms of power generation. This study has laid the foundation for exploring other crucial aspects of AVS based on foldable solar panels. These areas include the study of the distribution of light and shade on the crops beneath AVS (Valle et al., 2017), and comprehensive AVS simulation models (Torrente et al., 2024) with greenhouse farming underneath the panel. These studies offer valuable data on the collaborations between solar energy production and enhanced agricultural output in a variety of climate conditions.
The primary aim of this study is to develop an AVS that is integrated into agricultural systems. This will optimize land usage while simultaneously enhancing both energy efficiency and agricultural productivity. This initiative is aligned with the United Nations sustainable development goals (SDGs) (Huang et al., 2023), including affordable and clean energy (SDG 7), life on land (SDG 15), and industry, innovation, and infrastructure (SDG 9) By innovatively combining solar energy production with crop cultivation, this AVS is designed to not only provide a sustainable energy source that reduces reliance on fossil fuels but also to improve the microclimates of crops. This integration leads to more resilient agricultural practices and increased biodiversity.
The study’s findings reveal that the gains from foldable configurations are significantly influenced by the time of year, underscoring the critical role of seasonal factors in solar energy production. The positive gains noted during particular seasons highlight the potential for strategic deployment of solar technologies that adapt to seasonal variations in solar exposure. Future research should focus on developing adaptive solar tracking systems and dynamic panel adjustments that can optimize energy capture year-round. Additionally, further studies should explore the complex dynamics of light distribution, Photosynthetically Active Radiation (PAR), and comprehensive simulation models that could pave the way for innovative agricultural practices synergizing with renewable energy technologies. This would not only refine current models but also significantly contribute to the sustainable integration of energy and agricultural systems globally.
The original contributions presented in the study are included in the article/Supplementary Material, further inquiries can be directed to the corresponding author.
RL: Conceptualization, Data curation, Formal Analysis, Funding acquisition, Investigation, Methodology, Project administration, Resources, Software, Supervision, Validation, Visualization, Writing–original draft, Writing–review and editing. HJ: Conceptualization, Funding acquisition, Methodology, Project administration, Supervision, Validation, Writing–review and editing, Writing–original draft.
The author(s) declare that financial support was received for the research, authorship, and/or publication of this article. This work was supported by the ‘Regional Innovation Strategy (RIS)’ through the National Research Foundation of Korea (NRF) and funded by the Ministry of Education (MOE) (No. 2021RIS-002) and Institute of Information and Communications Technology Planning and Evaluation (IITP) grant funded by the Korea Government (MIST) (No. 2022-0-00530).
Author RL was employed by U Energy Co., Ltd.
The remaining author declares that the research was conducted in the absence of any commercial or financial relationships that could be construed as a potential conflict of interest.
All claims expressed in this article are solely those of the authors and do not necessarily represent those of their affiliated organizations, or those of the publisher, the editors and the reviewers. Any product that may be evaluated in this article, or claim that may be made by its manufacturer, is not guaranteed or endorsed by the publisher.
The Supplementary Material for this article can be found online at: https://www.frontiersin.org/articles/10.3389/fenrg.2024.1457228/full#supplementary-material
alraven, R. (1978). Calculating the position of the sun. Sol. energy 20 (5), 393–397. doi:10.1016/0038-092x(78)90155-x
Amaducci, S., Yin, X., and Colauzzi, M. (2018). Agrivoltaic systems to optimise land use for electric energy production. Appl. energy 220, 545–561. doi:10.1016/j.apenergy.2018.03.081
Asa'a, S., Reher, T., Rongé, J., Diels, J., Poortmans, J., Radhakrishnan, H. S., et al. (2024). A multidisciplinary view on agrivoltaics: future of energy and agriculture. Renew. Sustain. Energy Rev. 200, 114515. doi:10.1016/j.rser.2024.114515
Chalgynbayeva, A., Balogh, P., Szőllősi, L., Gabnai, Z., Apáti, F., Sipos, M., et al. (2024). The economic potential of agrivoltaic systems in apple cultiv.
Dupraz, C., Marrou, H., Talbot, G., Dufour, L., Nogier, A., and Ferard, Y. (2011). Combining solar photovoltaic panels and food crops for optimising land use: towards new agrivoltaic schemes. Renew. energy 36 (10), 2725–2732. doi:10.1016/j.renene.2011.03.005
Goetzberger, A., and Zastrow, A. (1982). On the coexistence of solar-energy conversion and plant cultivation. Int. J. Sol. Energy 1 (1), 55–69. doi:10.1080/01425918208909875
Gonocruz, R. A., Uchiyama, S., and Yoshida, Y. (2022). Modeling of large-scale integration of agrivoltaic systems: impact on the Japanese power grid. J. Clean. Prod. 363, 132545. doi:10.1016/j.jclepro.2022.132545
Hottel, H. C. (1976). A simple model for estimating the transmittance of direct solar radiation through clear atmospheres. Sol. Energy 18 (2), 129–134. doi:10.1016/0038-092x(76)90045-1
Huang, B., Huang, J., Xing, K., Liao, L., Xie, P., Xiao, M., et al. (2023). Development of a solar-tracking system for horizontal single-axis PV arrays using spatial projection analysis. Energies 16 (10), 4008. doi:10.3390/en16104008
Kelly, N. A., and Gibson, T. L. (2011). Increasing the solar photovoltaic energy capture on sunny and cloudy days. Sol. Energy 85 (1), 111–125. doi:10.1016/j.solener.2010.10.015
Lama, R. K., and Jeong, H. (2024). Design and performance analysis of foldable solar panel for agrivoltaics system. Sensors 24 (4), 1167. doi:10.3390/s24041167
Mengi, E., Samara, O. A., and Zohdi, T. I. (2023). Crop-driven optimization of agrivoltaics using a digital-replica framework. Smart Agric. Technol. 4, 100168. doi:10.1016/j.atech.2022.100168
Potenza, E., Croci, M., Colauzzi, M., and Amaducci, S. (2022). Agrivoltaic system and modelling simulation: a case study of soybean (Glycine max L.) in Italy. Horticulturae 8 (12), 1160. doi:10.3390/horticulturae8121160
Riaz, M. H., Imran, H., Alam, H., Alam, M. A., and Butt, N. Z. (2022). Crop-specific optimization of bifacial PV arrays for agrivoltaic food-energy production: the light-productivity-factor approach. IEEE J. Photovoltaics 12 (2), 572–580. doi:10.1109/jphotov.2021.3136158
Robledo, J., Leloux, J., Sarr, B., Gueymard, C. A., Driesse, A., Drouin, P. F., et al. (2021). “Lessons learned from simulating the energy yield of an agrivoltaic project with vertical bifacial photovoltaic modules in France,” in 38th European photovoltaic solar energy conference and exhibition.
Sarr, A., Soro, Y. M., Tossa, A. K., and Diop, L. (2023). Agrivoltaic, a synergistic co-location of agricultural and energy production in perpetual mutation: a comprehensive review. Processes 11 (3), 948. doi:10.3390/pr11030948
Stallknecht, E. J., Herrera, C. K., Yang, C., King, I., Sharkey, T. D., Lunt, R. R., et al. (2023). Designing plant–transparent agrivoltaics. Sci. Rep. 13 (1), 1903. doi:10.1038/s41598-023-28484-5
Toledo, C., and Scognamiglio, A. (2021). Agrivoltaic systems design and assessment: a critical review, and a descriptive model towards a sustainable landscape vision (three-dimensional agrivoltaic patterns). Sustainability 13 (12), 6871. doi:10.3390/su13126871
Torrente, C. J., Reca, J., López-Luque, R., Martínez, J., and Casares, F. J. (2024). Simulation model to analyze the spatial distribution of solar radiation in agrivoltaic Mediterranean greenhouses and its effect on crop water needs. Appl. Energy 353, 122050. doi:10.1016/j.apenergy.2023.122050
Trommsdorff, M., Gruber, S., Keinath, T., Hopf, M., Hermann, C., Schönberger, F., et al. (2022). “Agrivoltaics: Opportunities for Agriculture and the Energy Transition,” in Fraunhofer Institute for solar energy systems ISE. 2nd ed. Freiburg, Germany: Fraunhofer Institute for Solar Energy Systems ISE Heidenhofstrasse.
United Nations Sustainable Development Logo (2024). Available at: https://www.un.org/sustainabledevelopment/sustainable-development-goals/.
Valle, B., Simonneau, T., Sourd, F., Pechier, P., Hamard, P., Frisson, T., et al. (2017). Increasing the total productivity of a land by combining mobile photovoltaic panels and food crops. Appl. energy 206, 1495–1507. doi:10.1016/j.apenergy.2017.09.113
Weselek, A., Ehmann, A., Zikeli, S., Lewandowski, I., Schindele, S., and Högy, P. (2019). Agrophotovoltaic systems: applications, challenges, and opportunities. A Rev. Agron. Sustain. Dev. 39, 1–20. doi:10.1007/s13593-019-0581-3
Widmer, J., Christ, B., Grenz, J., and Norgrove, L. (2024). Agrivoltaics, a promising new tool for electricity and food production: a systematic review. Renew. Sustain. Energy Rev. 192, 114277. doi:10.1016/j.rser.2023.114277
Zhu, Y., Liu, J., and Yang, X. (2020). Design and performance analysis of a solar tracking system with a novel single-axis tracking structure to maximize energy collection. Appl. Energy 264, 114647. doi:10.1016/j.apenergy.2020.114647
Keywords: agrivolatics systems, foldable panels, solar power, shadow effect, sustainable agriculture
Citation: Lama RK and Jeong H (2024) Simulation model of power generation and the shadow effect of foldable solar panels used in agrivoltaics system. Front. Energy Res. 12:1457228. doi: 10.3389/fenrg.2024.1457228
Received: 30 June 2024; Accepted: 21 October 2024;
Published: 05 December 2024.
Edited by:
Gang Liu, Tianjin University, ChinaReviewed by:
Mobi Mathew, Rajiv Gandhi Institute of Petroleum Technology, IndiaCopyright © 2024 Lama and Jeong. This is an open-access article distributed under the terms of the Creative Commons Attribution License (CC BY). The use, distribution or reproduction in other forums is permitted, provided the original author(s) and the copyright owner(s) are credited and that the original publication in this journal is cited, in accordance with accepted academic practice. No use, distribution or reproduction is permitted which does not comply with these terms.
*Correspondence: Heon Jeong, aGplb25nQGNkdS5hYy5rcg==
Disclaimer: All claims expressed in this article are solely those of the authors and do not necessarily represent those of their affiliated organizations, or those of the publisher, the editors and the reviewers. Any product that may be evaluated in this article or claim that may be made by its manufacturer is not guaranteed or endorsed by the publisher.
Research integrity at Frontiers
Learn more about the work of our research integrity team to safeguard the quality of each article we publish.