- Department of Biochemistry and Microbiology, Faculty of Sciences, Engineering and Agriculture, University of Venda, Thohoyandou, South Africa
Developing countries facing population growth and increased energy demand present simultaneous challenges regarding wastewater treatment and electricity supply. Constructed wetland-microbial fuel cells (CW-MFCs) offer a dual solution to the challenges of insufficient electricity and wastewater treatment by integrating indigenous plant species. CW-MFCs provide sustainable power generation and wastewater treatment. This approach can enhance sustainability and foster a circular economy by utilising plant-derived byproducts as an added product. Current research indicates various novel designs for effective wastewater treatment and potential power generation. Future studies focusing on rural settings and upscaling operations can apply advanced techniques like mass spectrophotometry and metagenomics to refine the technology for grid use. Developing CW-MFC technology will catapult the idea of developing a robust strategy for addressing power supply and sanitation issues in developing regions. This will also aid in continuous research into system optimisation and microbial communities, aiding long-term viability.
1 Introduction
Water is fundamental to life and a constitutional right. Thus, recognising water as a vital resource and constitutional right emphasises the importance of developing solid policies that fully utilise sustainable wastewater treatment systems for potential reuse to improve water supply and sanitation (South African Human Rights commission, 2018). However, most developing countries require assistance in providing access to safe drinking water, adequate sanitation, and effective wastewater management. Rapid population growth, ageing infrastructure, pollution, and droughts have exacerbated the situation. Inadequate wastewater treatment, sanitation, and water scarcity endanger human health and the ecosystem (Adewumi et al., 2010; Nansubuga et al., 2016). To address these issues, sustainable and effective wastewater treatment will allow for potential reuse through a more closed and holistic system.
Despite the risks of faecal, parasitic, and bacterial diseases, agricultural communities in rural South Africa have turned to some form of wastewater reuse to alleviate poverty and produce income through irrigation or residential usage (Gumbo et al., 2010). Due to inadequate clean water, some rural communities rely on polluted water sources for home, recreational, agricultural, and industrial activities. Therefore, there is a need to underscore the importance of sanitary practice and efficient wastewater treatment before reuse or discharge into the environment due to their potential effects on human health (Gumbo et al., 2010; Traoré et al., 2016; Potgieter et al., 2020). According to Wilson and Pfaff (2008), in urban contexts, wastewater reuse is only considered if it is well treated because it was previously mixed with faeces, and individuals would not ordinarily ingest reclaimed wastewater. Drinking or using microbially impacted surface waters may result in future disease outbreaks such as diarrhoea and epatitis. Thus, effluents from wastewater treatment plants should ideally meet acceptable standards by removing high levels of pathogens and waste products (Traoré et al., 2016; Gcilitshana et al., 2017; Potgieter et al., 2020). Achieving such standards requires substantial resources. Therefore, it is critical to establish an efficient wastewater treatment system that uses as little energy as possible. Existing wastewater treatment plants in some countries, such as South Africa, are challenged with insufficient funding, maintenance, and noncompliance (DWS, 2023). As a result, in allocations with limited resources and inadequate infrastructure, a holistic approach to addressing wastewater treatment and power generation challenges is paramount. Constructed wetlands (CWs) are a low-cost natural wastewater treatment method that has been used successfully in both low- and upper-middle-income nations, including Egypt and South Africa, to remove pathogens and nutrients that cause eutrophication in open water sources (rivers, streams) (Hegazy et al., 2013; Lakay, 2013). However, the disadvantages of wetland systems include clogging issues and a need for standard design. This can be overcome for optimal use by suitable wastewater pretreatment and exploring diverse wetland designs to be useful for large-scale and decentralised home wastewater treatment, effectively removing faecal markers and enteric viruses (Hegazy et al., 2013; Lakay, 2013; Moreira and Dias, 2020). In addition, several studies have shown that constructed wetlands have the potential for integration with microbial fuel cells as constructed wetland microbial fuel cells (CW-MFCs) (Srivastava et al., 2015; Guadarrama-Pérez et al., 2019; Wang et al., 2020; Wang et al., 2022). This integration serves a dual purpose of wastewater treatment and energy generation and reduces essential water quality parameters, including chemical oxygen demand (COD) (Srivastava et al., 2015; Wang et al., 2020). It works on the principle that exo-electrogenic bacteria oxidise organic materials at the anoxic anode and then reduce oxygen (O2) via photosynthetic activity at the cathode to generate power (Jingyu et al., 2020; Shaikh et al., 2021; Sharma et al., 2022). This system can provide alternative energy supplies in resource-limited communities, such as cleaner energy powered by biofuels created as byproducts from plants and microbes (Rawat et al., 2011; Dawood et al., 2018; Ullah et al., 2021).
The CW-MFCs integrated system will also help governments accelerate the transition from high-carbon-footprint coal power generation to greener sources such as biofuels, which may address insufficient electricity supply (Dawood et al., 2018; Ullah et al., 2021). Frequent power outages cost developing economies such as South Africa up to R13.72 billion in losses from businesses (Goldberg, 2015). Therefore, this review evaluated the viability of CW-MFCs in developing countries, including their efficacy in wastewater treatment, effective power generation, and system design.
1.1 Methodology
1.1.1 Inclusion studies
To be eligible for inclusion in this assessment, studies had to (a) be original research articles conducted within the borders of developing countries and focusing on various types of wastewater treatment using CW-MFCs; (b) address the removal of pathogenic or indicator microbes and the mitigation of chemical parameters, such as nitrogen, phosphates, and others; (c) investigate CW-MFCs and their role in effective power generation; and lastly, (d) be published in the English language.
1.2 Data extraction
A literature search of Google Scholar, Web of Science, PubMed, and ScienceDirect was conducted in April 2024, using the search terms “Wastewater Treatment,” “Constructed Wetland-microbial Fuel Cell,” “Power Generation,” “Developing Countries,” and “Effectiveness” with no specific period. For the initial search, the abstract, title, and methodology were used to identify articles that fit the criteria. After that, full articles were screened according to the inclusion criteria. Information related to power generation, such as electric potential and power density, was extracted. Factors such as the removal of nutrients, microbial removal, COD and heavy metals, and additional advantages and disadvantages were examined for wastewater treatment. System design elements were extracted and subsequently discussed.
2 Results
2.1 Characterisation of the studies
In developing countries, 615 studies were found in the initial search. After reviewing the titles and abstracts, 36 papers were chosen for full-text screening (Figure 1). Based on the inclusion criteria, only six studies were ultimately chosen (Table 1). Two studies were conducted in South Africa, three in India, and one in China. The few studies focusing on effective CW-MFC systems reflect the need for more literature in this sector in developing countries.
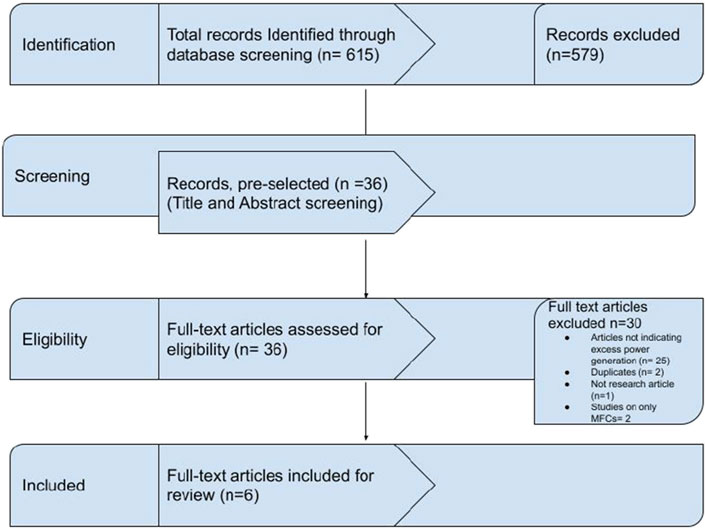
Figure 1. Flow diagram of the literature search strategy, search results, and inclusion and exclusion of articles.
It should be noted that each of these research papers was selected because they show potential for generating surplus power. All studies had a common goal: simultaneous wastewater treatment and power generation. The chosen studies used a variety of designs, including an open circuit, single-chamber planted CW-MFC, a biological sand filter (BSF), two-stage CW-MFCs, an ecological-microbial fuel cell, and a semi-pilot-scale hybrid eco-electrogenic engineering system (HEEES) (Table 1).
2.2 Power generation
The metrics assessed for power generation in these systems were surface power density (mWm−2), volumetric power density (mWm−3), and voltage (mV) (Table 1). The highest power density was recorded at 1029.2 mWm−2 in a study from India using a semi-pilot scale HEEES, with the lowest being from a study in China (15.9 mWm−2) using an E-MFC. Volumetric power densities were varied; the highest power density (229 ± 52 mWm−3) and the lowest power density (4.33 mWm−3) were recorded in studies from South Africa. Voltages were the highest (1011 mV) and lowest (26.33 mV) in systems from India using semi-pilot-scale HEEES and a single-chamber CW-MFC, respectively. For comparison, a benchmark was used to compare it to other studies (Table 2).
2.3 Wastewater treatment efficiency
On treatment efficiency, a single study from South Africa revealed 100% elimination of harmful microorganisms such as Escherichia coli, whereas the other studies reported zero removal (Table 1). In the case of nutrient removal (Figure 2), all investigations revealed >80% COD removal efficiency, >60% for nitrate removal efficiency, and finally, phosphate removal efficiency ranged from 65% to 99% (Table 1). The reduction of ammonia was 90.4%, which was reported by one of the studies, while others did not report any removal efficiency percentages (Table 1).
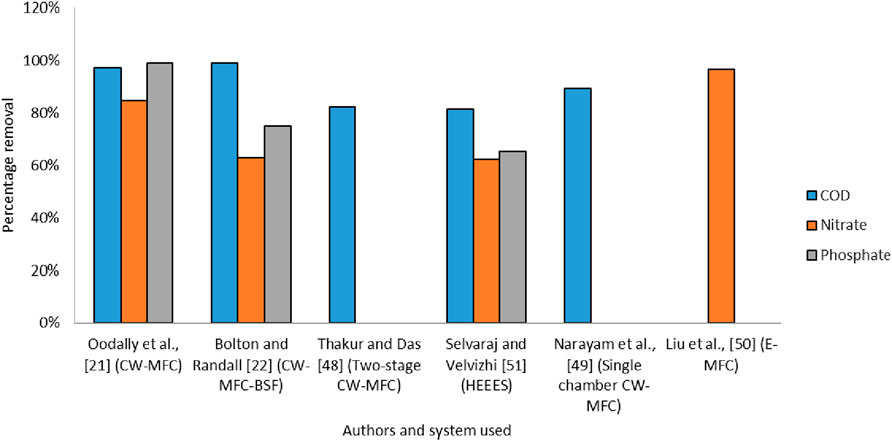
Figure 2. Comparison of nutrient removal by the various systems regarding chemical oxygen demand, phosphate, and nitrate removal.
2.4 Sustainability and system design
Studies were done on various systems (Table 1), and single-chamber CW-MFCs were found to be the simplest for laboratory testing and were carried out in South Africa and India. In another study from South Africa, there was the incorporation of a BSF into the system to enhance the wastewater treatment of handwashing water (Table 1). The primary differences between the single-chamber systems were the utilisation of macrophytes, circuitry, separator materials, MFC container materials, and operation volume. The biological sand filter, made of clear acrylic plastic containers, was outfitted with a peristaltic pump and a steel diffuser plate to disperse influent evenly throughout the CW-MFC-BSF. Glass wool was used as a separator, and nickel-coated copper wires were used for the electronics. The single-chamber CW-MFC from India used copper wiring and a polyacrylic plastic cylinder filled with four materials from the bottom, containing gravel, pebbles, sand, and silt. Only one study used a dual-chamber system comprising polyvinyl chloride pipes and glass wool separators. The electrodes were connected using insulated copper wires. Other unique systems, such as the E-MFC and the HEEES, were investigated in India and China. The E-MFC is a novel system that is like a single-chamber system. Still, sludge worms (Tubifex tubifex) were used with water lettuce (Ipomoea aquatica) plants to create a localised ecosystem. The column was filled with gravel, sandy soil as sediment, and a water layer, which comprised the filter and growth substrate for the plant, while the anode (granular activated carbon with stainless steel mesh) and cathode (stainless steel mesh) were connected using titanium wires. The HEEES system was the only pilot-scale system that tested three macrophytes for wastewater treatment and power generation efficacy. It was comprised of glass tanks with activated carbon bags as filter materials, and the circuitry was a hybrid parallel-series connection between graphite anodes and cathodes using copper wires.
3 Discussion
3.1 Role of plant species: CW-MFC in energy production and wastewater treatment
Macrophyte photosynthetic activities are essential for the oxygen-rich conditions of the cathode. Investigations utilising plants (macrophytes) generated a wide range of power densities. Indigenous species, such as C. prolifer plants, were explicitly considered in one study and have the benefit of highly specialised root regions and biomass that aid in power production and nutrient removal (Table 1). Plant species like C. prolifer and C. nana are suitable for CW-MFCs because they can survive in the soggy conditions required for the anode of the CW-MFC system, and they can produce extra uses in textiles, fibre, and energy in the form of biofuels. According to the South African National Biodiversity Institute (SANBI), most of these plants are endangered in South Africa. Their cultivation should be carefully evaluated before deployment, so existing populations are not harmed (Notten, 2001; Mashau et al., 2015; Popay, 2022). Studies from India utilise several macrophytes. However, only one indigenous species, Hydrilla verticillata (water thymes), was tested in the HEEES. The plant species were not compared directly; instead, it was found that adding additional components such as aerators, activated carbon, and electrodes all improved the power generation and wastewater treatment efficiency of all three plants investigated. Other plants in the various systems, such as water hyacinths, are invasive and proliferate aggressively. However, this may be beneficial for biochar production and wastewater treatment. Biochar is obtained by thermochemically decomposing biomass into carbon, thus creating a circular system (Xiang et al., 2020).
Another unique and recently developed system in China utilised T. tubifex worms in tandem with macrophytes to improve both wastewater treatment efficacy and power generation. Excretions by T. tubifex served as extra nutrients for the plants and thus aided them in surviving harsh wastewater conditions. Several factors, including bacteria, wastewater type, plant species, accessible oxygen at the cathode, and exo-electrogens at the anode, may influence power generation disparities (Shaikh et al., 2021; Sharma et al., 2022). One of the primary goals of this study was to determine the efficacy of wastewater treatment, with a secondary focus on power generation using a biological sand filter (BSF) to improve waste treatment. Root biomass and oxygenation by macrophytes at the cathode and exo-electrogens at the anode influence power generation. Other significant changes in system design regarding overall volume include the employment of a variety of photosynthetic organisms with various users. Plants or algae, circuitry materials, bacteria introduced, and the type of wastewater used determine their power-generating efficacy in the various included studies.
In other planted systems with water hyacinth and Iris pseudacorus, the presence of macrophytes and the type of substrate have been found to promote heavy metal removal. This is important as heavy metals endanger human, crop, and soil health by modifying soil microbiota and impacting the decomposition of organic material (Gola et al., 2016). It is thus essential for future studies to consider this parameter. The presence of H2O2 and organics that act as electron donors and are created by electrochemically active microbes to oxidise or chelate metals is also significant. Additionally, some plants, like Arundo donax, can oxygenate water, aiding in the removal of some metals and nutrients as well as microorganisms, and have been shown to boost the treatment of other processes, such as anaerobic bioreactors, within the National Environmental Quality Standards (NEQS) in Pakistan. Microalgae used in the post-treatment of wastewater have also proven to be capable of effective nutrient and metal removal when combined with macrophytes. However, they are less effective compared to systems consisting of mixed macrophytes (Zeb et al., 2013; Gulzar et al., 2018; Wang et al., 2022).
Various indigenous species can be studied in developing countries for their ability to remove heavy metals and consortiums of bacteria, effectively treat wastewater, and remove heavy metals from anaerobic digesters while also successfully generating bioelectricity. Mixed systems should be considered to improve wastewater treatment efficiency and generate bioelectricity (Gola et al., 2016; Sharma et al., 2022). Biotic and abiotic variables also influence the removal of heavy metals by microalgae. This was also influenced by biotic parameters such as the volume and size of microalgae, microalgal biomass, and the presence of proteins and lipids capable of heavy metal sequestration. Abiotic parameters that are usually related to the conditions of the wastewater itself (such as pH, salinity, ionic strength, temperature, hardness, and metal diversity) are also included, which similarly affect macrophytes, depending on species (Pavithra et al., 2020).
3.2 Microbes involved in CW-MFCS in developing countries
Exo-electrogens are reported to be produced by microbes in activated sludge from various wastewater sources in multiple CW-MFC systems (Sharma et al., 2022). According to Sharma et al. (2022), genetic engineering and omics methods have been successfully used to boost electricity generation and wastewater treatment by various bacteria. Others reported that diverse metabolic pathways from bacterial species such as E. coli, A. butzleri, and S. oneidensis were altered to boost voltage output, current output, and power density (Sharma et al., 2022). Physical characteristics such as pH and COD may also play a role in driving the process at the cathode or temperature of the system. In this review, one study from China determined the microbial diversity of anodic biofilm microflora using the non-culture-based technique pyrosequencing, where they found a high abundance of exo-electrogenic bacteria such as Geobactericeae, Desulfobulbaceae, and Bacillaceae, which contributed to generating power. These bacteria are also responsible for various processes to remove nutrients, such as denitrification (Rhizobacter and Comamonadaceae_unclassified) and nitrite oxidation (Nitro-spira). Introducing T. tubifex led to higher microbial diversity, aiding in wastewater treatment and electricity generation. Adding benthic fauna such as T. tubifex thus helps generate power and treat wastewater (Liu et al., 2021).
3.3 Power generation capabilities in developing countries
In this study, the planted CW-MFC systems performed well in power density (15.59 mWm−2 to 1029.2 mWm−2) and volumetric power density (4.33 mWm−3 to 229 ± 52 mWm−3) (Table 1) compared to other planted systems (Table 2). Power density (mWm−2) in CW-MFCs refers to the amount of power produced per unit surface area of the electrode, which measures how efficiently the MFC can convert organic matter into electrical energy. A higher power density means the MFC can generate more power from the same electrode surface area. Power density is vital for limited-space applications, such as portable or implantable devices, where maximising power output from a small surface area is crucial. On the other hand, volumetric power density is the amount of power produced per unit volume of the entire system. This is paramount for applications where the physical size of the CW-MFC system is a restricting factor, such as in remote monitoring systems or wastewater treatment plants, where space for installation may be sparse. Plants in this study, such as C. indica and P. australis, are also used in the benchmark and show effective volumetric and surface power densities at 40 mWm−3 and 229 ± 52 mWm−3/1029.2 mWm-2. Studies such as those by Oodally et al. (2019) help in elucidating the power generation capabilities of different plant species. Additionally, the overall system design is an important aspect, as shown by Selvaraj and Velvizhi (2023). Adding components such as aerators assists in improving power generation and removal efficacies. Despite these, the additional costs must be considered for implementation in more rural settings where funds are scarce and low-maintenance systems are crucial, as well as power demand from pumps. Determining either power density or volumetric power density depends on a system’s space constraints.
3.4 Electric potential of CW-MFCs in developing countries
Voltage, which is the pressure at which electrons are pushed from a power source through a circuit, enabling them to power devices, varied in each of the selected studies, which could be linked to system design, plants used, microbes involved, and type of wastewater used in the system. The highest voltage was recorded by Selvaraj and Velvizhi (2023) in their novel HEEES system, which was developed in India. This is possibly due to their system using hybrid circuitry, as they stated, “The parallel connection generally implies high cumulative current and less voltage, whereas the series circuit shows high cumulative voltage without any voltage reversal and less current. Hence, the series and parallel connections individually show less power”; thus, they used a hybrid system to maximise power generation and electric potential. Another factor to consider is the volume of the HEEES, which was much higher than that of other systems. The effects of volume on power generation could be considered to determine if the system is viable in highly populated areas and crowded spaces.
3.5 Improvements to power generation in CW-MFCs
In South Africa and other developing countries like India and China, indigenous species of macrophytes have demonstrated the ability to create acceptable amounts of electricity. Still, more is needed to generate a net gain in electricity for large devices and alleviate constraints in the power grid. To mitigate these obstacles, such as the low power output of these devices, effective energy harvesting systems can be designed or purchased to increase electric potentials from 0.2–0.5V to 17–99 V (Koffi and Okabe, 2020). Although implementing such systems may incur additional costs, it may enable the powering of simple electronics, such as a single light-emitting diode (LED) that requires 2 V to operate and is used as a light source on a large scale for photosynthetic plants and algae. Another advantage of booster systems like the one examined by Koffi and Okabe (2020) is that a continuation provides power even while detaching from the MFC. This could thus allow for continuous power supply for lights and small devices during power interruptions due to maintenance or outages. A wide range of energy harvesting technologies can be employed for CW-MFCs, and commercial energy harvesting systems are available to help with overall system sustainability. Capacitor-based systems, charge pump systems, booster-converter systems, and customisable maximum power point/active energy harvesting systems are among the energy harvesting technologies (Wang et al., 2015; Koffi and Okabe, 2020). These technologies also aid in stabilising observed current and voltage variations, which could be helpful if added to a system using hybrid circuitry to improve efficiency further. Novel conductive materials have been produced using chemical engineering principles to increase the mobility of electrons and reduce CO2 and dyes for improved photocatalytic production of important fuels, such as hydrogen and methane, using light. This is typically done by incorporating various elements, such as sulphur and phosphorous, and altering the chemical structures of the conductive materials. Analysing those structures using methods such as transmission electron microscopy has given key insights on their electrogenic capabilities (Khan et al., 2019; Khan et al., 2020; Kakar et al., 2021; Khan et al., 2021; Raziq et al., 2022). The gases (H2 and CO2) are useful and a renewable energy source; thus, CW-MFC electrodes enhanced for energy production in this way could potentially increase the total energy harvested from the sun by both the plants and the system itself.
3.6 Wastewater treatment capabilities of CW-MFCs in developing countries
The treatment of wastewater is frequently the focus of CW-MFC systems. The addition of a biological sand filter, comprised of crushed rock aggregate and builders’ sand, as well as a polyester layer, aids in the removal of COD, microbes, and the treatment of larger volumes of wastewater, which may explain why the addition of a filter resulted in high levels of COD reduction as reported in one study conducted in South Africa compared to the other systems (Table 1). Nutrient removal in wastewater systems is critical for mitigating the impacts of eutrophication on receiving water bodies, which is harmful to aquatic ecosystems. COD, total suspended particles, and nitrogen are removed as nutrients (Akpor and Muchie, 2011). COD removal is critical for reducing organic compounds that may deplete oxygen in wastewater. The planted wetland systems outperformed the microalgal system regarding nutrient removal efficiency, effectively lowering COD. The Cyperus species have an extensive root surface area for nutrient adsorption, as do the microorganisms that live in those roots (Ahiahonu et al., 2022). Oxygen released by macrophytes is pivotal in removing various nutrients, metals, and cathodic conditions for electricity generation. Oxygen content is affected by plant growth and environmental conditions, including temperature and light, which are used for the growing conditions of macrophytes. This is important when considering upscaling and system design. Plants such as P. australis, Saurus cernuus (Lizard’s Tail), Pistia stratiotes (Water Lettuce), and Typha latifolia have been shown to increase oxygen levels in CW systems (Rehman et al., 2017; Rehman et al., 2023). Ammonia removal was determined in planted CW-MFC systems, where C. prolifer plants eliminated them with the help of a large root surface area that aids in sequestration. In another study, 90.4% of the of the removal was probably due to the activities of plants and the presence of ammonia-oxidising bacteria found using 16S rRNA sequencing. Nitrate removal was successful in the system, and according to this study, anoxic conditions, root biomass, and plant type affect nitrate elimination in plant systems. Phosphate removal was also effective in CW-MFCs and was primarily controlled by factors such as plant species employed, filtration media selection, and the presence of phosphate-accumulating organisms.
Microbiological quality is a key aspect of monitoring the entire wastewater treatment process. Wastewater and other human activities, such as car washing and laundry, impact rivers and dams in rural South Africa (Traoré et al., 2016). Enteric pathogens such as E. coli and other enteric viruses (Norovirus, Human Adenovirus, and Rotavirus) have been found in conventional wastewater treatment effluents and sewage-impacted rivers in South Africa. They are typically transmitted through the faecal-oral route (Gcilitshana et al., 2017; Osuolale and Okoh, 2017; Potgieter et al., 2020). When a biological sand filter was added to a planted CW-MFC, it effectively removed E. coli from synthetic greywater (Table 1). Some studies did not emphasise the removal of E. coli in some systems, such as microalgal CW-MFCs, but rather explained how the bacteria are engineered as exo-electrogens in the anodic chamber due to their facultative anaerobic nature (Sharma et al., 2022). The study by Bolton and Randall (2019) used culture-based techniques on MacConkey agar to investigate the removal of the indicator organism, E. coli. They found that a simple CW-MFC system with the addition of a biological sand filter allowed the full removal of viable E. coli cells. A significant drawback of this assessment is the need for a detailed comparison of microbiological parameter removal between different systems and plant species using easy and cost-effective culture procedures in conjunction with synthetic wastewater. It is critical to evaluate a wide range of indicators, apart from E. coli, which may not accurately represent the presence of other human pathogens, such as parasites and viruses, in wastewater quality monitoring (Ashbolt et al., 2001).
3.7 CW-MFCs: design and sustainability in developing countries
Cost and purpose influence the design sustainability and scalability of different CW-MFC systems. The created wetland component can be integrated with the microbial fuel cell and other elements, such as a BSF (Table 1). The cathodes (Platinum-coated carbon paper) and anodes (Granular activated carbon around a steel mesh electron collector) were made of comparable materials in both planted CW-MFC systems. Materials used for vessels, electrodes, wires, pipes, and other equipment, such as pumps, can be cost-effectively optimised to fit economically tricky environments. CW-MFCs can also be built using recycled materials such as aluminium cans, thus adding to their value as a sustainable system. Capable of utilising various available materials to generate power density, voltage, and COD removal of 304.46 mWm−2, 0.71 V, and 80%, respectively (Thakur and Das, 2021a). The choice of materials is also important in determining the adhesion and biofilm formation on electrodes by exoelectrogens and the electrical conductivity of the system (Guadarrama-Pérez et al., 2019; Shaikh et al., 2021). Synthetic materials have been applied to increase the effectiveness of other wastewater treatment technologies, such as activated granular sludge (Hussain et al., 2024). This could be considered in CW-MFC as well, in a hybrid technology whereby aerobic granules are added to cathodes to increase aeration and wastewater treatment efficiency at a lower cost.
In systems like CW-MFCs, electrode materials may impact economic feasibility. Titanium and platinum are good conductors but are pricey materials, making it difficult to scale up CW-MFCs or use them in low-income regions. Hydraulic retention time, affected by system volume, is also important in power generation and wastewater treatment (Jingyu et al., 2020; Sharma et al., 2022). Photosynthetic activity is a powerful reducing component in the cathodic section of the fuel cell in planted CW-MFC systems, and the additional biomass generated from CO2 reduction into carbohydrates gives biomass to the microorganisms associated with the plants and anode (Shaikh et al., 2021). The existence of a peristaltic pump may impose an additional energy sink; nonetheless, it has been claimed that in pilot-scale systems, they were able to power themselves using MFCs as an energy source (Stoll et al., 2018). Separators are used in planted CW-MFCs to separate the anode and cathode environments, like how Nafion Proton Exchange Membrane (PEM) works. The difference in power generation without a separator may be insignificant. Hence, its presence affects the design cost of planted systems (Guadarrama-Pérez et al., 2019). Similarly, integration with other low-cost water treatment systems for homes, such as silver-impregnated filters and tablets, has been used domestically in rural South African settings. This has effectively reduced total coliform bacteria (from 3 log and 1.2 log reductions, respectively) in water treatment systems (Hill et al., 2020). Because of its low cost, this technology could be examined alongside CW-MFC systems in decentralised wastewater treatment and rural energy generation.
4 Limitations and future studies
One significant drawback of this analysis is that few papers are available in developing countries examining effective electricity generation while treating wastewater using CW-MFCs. In addition, there are no universal measurements of some factors, and valuable quantitative tools such as statistical tests are still missing or are in their infancy stages. Cost is another important limitation of the study; as the context is in developing countries, policymakers and funders should consider these technologies while multiple issues should be resolved, such as cost-effectiveness, on a larger scale. Similar factors, such as indicator microorganisms, power density, heavy metal diversity, and several nutrients, were absent in the included studies or the standard benchmark.
System design differs; however, this system variety highlighted in this review is advantageous in identifying whether a system is practical for future exploration. In addition, these technologies are available to effectively increase the performance of CW-MFC systems. Another strength is that pilot-scale operations are being explored, showing potential for up-scaling.
Future studies should concentrate on optimising system design for upscaling, incorporating energy harvesting technologies such as boosters, and genetic engineering of plants, algae, and exo-electrogenic bacteria involved in wastewater treatment and power generation processes. A wide range of pathogenic organisms and indicators, including viruses, protozoa, and fungi, might be explored to establish their removal and impacts on CW-MFC systems. The viral element was not investigated in this research, although it may influence microbe activity in electricity generation, which is presently being studied in CW-MFC systems. Regarding bacteriophages, phages that infect enteric bacteria (e.g., E. coli), such as somatic coliphages and F-specific RNA bacteriophages, have been observed in normal-built wetland systems. This is significant because the CW-MFC-BSF study used E. coli as an indicator organism. Even though E. coli has been genetically altered to aid in improving power generation, it may be affected by phages.
5 Conclusion
The review aimed to investigate CW-MFC systems’ effectiveness, sustainability, and efficiency in wastewater treatment and power generation. Furthermore, these systems were reported to produce equivalent electricity to other similar systems and efficiently clean water in laboratory and pilot-scale settings, regardless of microorganisms and nutrients. Plants added to the systems also contributed to adequate wastewater treatment by removing at least 63% of nutrients from various wastewaters. One study from South Africa also achieved 100% E. coli eradication using a CW-MFC-BSF system, whereas the other trials did not. Power generation was completed by all types of systems, with some systems based on ecological principles demonstrating higher generation values. Because CW-MFC systems are holistic solutions with multiple components, holistic research approaches may be required to make this a feasible solution to developing countries’ expanding wastewater treatment and energy supply concerns. Future research should investigate system elements such as upscale design, enhanced electricity generation, and effective wastewater treatment employing indigenous plant and microbial species. This will help to reveal their diversity and further influence with constructed-wetland-microbial fuel cells implemented in developing countries.
Author contributions
DJ: Conceptualization, Data curation, Formal Analysis, Investigation, Methodology, Project administration, Validation, Writing–original draft, Writing–review and editing, Visualization. LK: Data curation, Formal Analysis, Investigation, Methodology, Project administration, Supervision, Validation, Writing–original draft, Writing–review and editing, Conceptualization, Visualization. MR: Conceptualization, Data curation, Formal Analysis, Investigation, Methodology, Project administration, Validation, Writing–original draft, Writing–review and editing, Supervision, Visualization. AA: Data curation, Formal Analysis, Methodology, Project administration, Supervision, Validation, Writing–review and editing, Visualization. AT: Data curation, Formal Analysis, Project administration, Supervision, Validation, Writing–review and editing, Visualization. NP: Data curation, Formal Analysis, Funding acquisition, Methodology, Project administration, Resources, Supervision, Validation, Writing–review and editing.
Funding
The author(s) declare that no financial support was received for the research, authorship, and/or publication of this article.
Conflict of interest
The authors declare that the research was conducted in the absence of any commercial or financial relationships that could be construed as a potential conflict of interest.
Publisher’s note
All claims expressed in this article are solely those of the authors and do not necessarily represent those of their affiliated organizations, or those of the publisher, the editors and the reviewers. Any product that may be evaluated in this article, or claim that may be made by its manufacturer, is not guaranteed or endorsed by the publisher.
References
Adewumi, J. R., Ilemobade, A. A., and Van Zyl, J. E. (2010). Treated wastewater reuse in South Africa: Overview, potential and challenges. Resour. Conservation Recycl. 55 (2), 221–231. doi:10.1016/j.resconrec.2010.09.012
Ahiahonu, E. K., Anku, W. W., Roopnarain, A., Green, E., Govender, P. P., and Serepa-Dlamini, M. H. (2022). Bioresource potential of Tetradesmus obliquus UJEA_AD: critical evaluation of bio-sequestration rate, biochemical and fatty acid composition in BG11 media. J. Chem. Technol. & Biotechnol. 97 (3), 689–697. doi:10.1002/jctb.6951
Akpor, O. B., and Muchie, B. (2011). Environmental and public health implications of wastewater quality. Afr. J. Biotechnol. 10 (13), 2379–2387. doi:10.5897/AJB10.1797
Ashbolt, N. J., Grabow, W. O. K., Snozzi, M., Fewtrell, L., and Bartram, J. (2001). “Water quality: guidelines, standards and health,” in Indicators of microbial water quality. Editors L. Fewtrell, and J. Bartram (London: IWA Publishing).
Bolton, C. R., and Randall, D. G. (2019). Development of an integrated wetland microbial fuel cell and sand filtration system for greywater treatment. J. Environ. Chem. Eng. 7 (4), 103249. doi:10.1016/j.jece.2019.103249
Dawood, S., Ahmad, M., Ullah, K., Zafar, M., and Khan, K. (2018). Synthesis and characterization of methyl esters from non-edible plant species yellow oleander oil, using magnesium oxide (MgO) nano-catalyst. Mater. Res. Bull. 101, 371–379. doi:10.1016/j.materresbull.2018.01.047
Department of Water and Sanitation (2023). Green drop watch report. DWS. Available at: https://ws.dws.gov.za/IRIS/releases/GDWR.pdf (Accessed April 01, 2024).
Gcilitshana, O., Sibanda, T., Zhou, L., and Okoh, A. I. (2017). Assessment of the prevalence of enteric viruses in the final effluents of two peri-urban wastewater treatment plants. Asian Pac. J. Trop. Dis. 7, 121–126. doi:10.12980/apjtd.7.2017D6-380
Gola, D., Malik, A., Shaikh, Z. A., and Sreekrishnan, T. R. (2016). Impact of heavy metal containing wastewater on agricultural soil and produce: relevance of biological treatment. Environ. Process. 3, 1063–1080. doi:10.1007/s40710-016-0176-9
Goldberg, A. (2015). The economic impact of load shedding: the case of South African retailers. Doctoral Thesis. South Africa: University of Pretoria.
Guadarrama-Pérez, O., Gutiérrez-Macías, T., García-Sánchez, L., Guadarrama-Pérez, V. H., and Estrada-Arriaga, E. B. (2019). Recent advances in constructed wetland-microbial fuel cells for simultaneous bioelectricity production and wastewater treatment: a review. Int. J. Energy Res. 43 (10), 5106–5127. doi:10.1002/er.4496
Gulzar, F., Mahmood, Q., Bhatti, Z. A., Zeb, B. S., Shaheen, S., Hayat, T., et al. (2018). Industrial wastewater treatment in internal circulation bioreactor followed by wetlands containing emergent plants and algae. World J. Microbiol. Biotechnol. 34, 119–128. doi:10.1007/s11274-018-2496-6
Gumbo, J. R., Malaka, E. M., Odiyo, J. O., and Nare, L. (2010). The health implications of wastewater reuse in vegetable irrigation: a case study from Malamulele, South Africa. Int. J. Environ. Health Res. 20 (3), 201–211. doi:10.1080/09603120903511093
Hegazy, A. M., El-Salakawy, A. H., Shaban, M. M., Yehia, M. M., and AbuSalama, M. S. (2013). Eco-friendly management of enteroviruses in wastewater. Water Sci. 27 (54), 19–29. doi:10.1016/j.wsj.2013.12.002
Hill, C. L., McCain, K., Nyathi, M. E., Edokpayi, J. N., Kahler, D. M., Operario, D. J., et al. (2020). Impact of low-cost point-of-use water treatment technologies on enteric infections and growth among children in Limpopo, South Africa. Am. J. Trop. Med. Hyg. 103 (4), 1405–1415. doi:10.4269/ajtmh.20-0228
Hussain, S., Ferrentino, R., Khan, K., Ali, Z., Yousif, M., and Andreottola, G. (2024). Rapid startup of aerobic granular sludge: recent advances and future challenges. Results Eng. 22, 102035. doi:10.1016/j.rineng.2024.102035
Jingyu, H., Miwornunyuie, N., Ewusi-Mensah, D., and Koomson, D. A. (2020). Assessing the factors influencing the performance of constructed wetland–microbial fuel cell integration. Water Sci. Technol. 81 (4), 631–643. doi:10.2166/wst.2020.135
Kakar, M. U., Khan, K., Akram, M., Sami, R., Khojah, E., Iqbal, I., et al. (2021). Synthesis of bimetallic nanoparticles loaded on to PNIPAM hybrid microgel and their catalytic activity. Sci. Rep. 11 (1), 14759. doi:10.1038/s41598-021-94177-6
Khan, K., Tao, X., Shi, M., Zeng, B., Feng, Z., Li, C., et al. (2020). Visible-light-driven photocatalytic hydrogen production on Cd0.5Zn0.5S nanorods with an apparent quantum efficiency exceeding 80%. Adv. Funct. Mater. 30 (42), 2003731. doi:10.1002/adfm.202003731
Khan, K., Tao, X., Zhao, Y., Zeng, B., Shi, M., Ta, N., et al. (2019). Spatial separation of dual-cocatalysts on one-dimensional semiconductors for photocatalytic hydrogen production. J. Mater. Chem. A 7 (26), 15607–15614. doi:10.1039/C9TA03090E
Khan, K., Xu, L., Shi, M., Qu, J., Tao, X., Feng, Z., et al. (2021). Surface assembly of cobalt species for simultaneous acceleration of interfacial charge separation and catalytic reactions on Cd0. 9Zn0. 1S photocatalyst. Chin. J. Catal. 42 (6), 1004–1012. doi:10.1016/S1872-2067(20)63717-2
Koffi, N. D. J., and Okabe, S. (2020). High voltage generation from wastewater by microbial fuel cells equipped with a newly designed low voltage booster multiplier (LVBM). Sci. Rep. 10 (1), 18985. doi:10.1038/s41598-020-75916-7
Lakay, V. M. (2013). An analysis of the performance of constructed wetlands in the treatment of domestic wastewater in the Western Cape, South Africa.
Liu, S., Feng, X., Xue, H., Qiu, D., Huang, Z., and Wang, N. (2021). Bioenergy generation and nitrogen removal in a novel ecological-microbial fuel cell. Chemosphere 278, 130450. doi:10.1016/j.chemosphere.2021.130450
Mashau, A., Ephraim, M., and Reynolds, Y. (2015). Phragmites australis, phragmites australis PlantZ Africa. Available at: https://pza.sanbi.org/phragmites-australis (Accessed April 15, 2024).
Moreira, F. D., and Dias, E. H. O. (2020). Constructed wetlands applied in rural sanitation: a review. Environ. Res. 190, 110016. doi:10.1016/j.envres.2020.110016
Nansubuga, I., Banadda, N., Verstraete, W., and Rabaey, K. (2016). A review of sustainable sanitation systems in Africa. Rev. Environ. Sci. bio/technology 15, 465–478. doi:10.1007/s11157-016-9400-3
Narayan, M., Solanki, P., Rabha, A. K., and Srivastava, R. K. (2018). Treatment of pulp and paper industry effluent and electricity generation by constructed wetland coupled with microbial fuel cell (CW-MFC). J. Pharmacogn. Phytochemistry 7 (6), 493–498.
Notten, A. (2001). Wachendorfia thyrsiflora, wachendorfia thyrsiflora PlantZ Africa. Available at: https://pza.sanbi.org/wachendorfia-thyrsiflora (Accessed August 15, 2023).
Oodally, A., Gulamhussein, M., and Randall, D. G. (2019). Investigating the performance of constructed wetland microbial fuel cells using three indigenous South African wetland plants. J. Water Process Eng. 32, 100930. doi:10.1016/j.jwpe.2019.100930
Osuolale, O., and Okoh, A. (2017). Human enteric bacteria and viruses in five wastewater treatment plants in the Eastern Cape, South Africa. J. Infect. public health 10 (5), 541–547. doi:10.1016/j.jiph.2016.11.012
Pavithra, K. G., Kumar, P. S., Jaikumar, V., Vardhan, K. H., and Sundar, R. P. (2020). Microalgae for biofuel production and removal of heavy metals: a review. Environ. Chem. Lett. 18, 1905–1923. doi:10.1007/s10311-020-01046-1
Potgieter, N., Karambwe, S., Mudau, L. S., Barnard, T., and Traore, A. (2020). Human enteric pathogens in eight rivers used as rural household drinking water sources in the northern region of South Africa. Int. J. Environ. Res. Public Health 17 (6), 2079. doi:10.3390/ijerph17062079
Rawat, I., Kumar, R. R., Mutanda, T., and Bux, F. (2011). Dual role of microalgae: phycoremediation of domestic wastewater and biomass production for sustainable biofuels production. Appl. energy 88 (10), 3411–3424. doi:10.1016/j.apenergy.2010.11.025
Raziq, F., Khan, K., Ali, S., Ali, S., Xu, H., Ali, I., et al. (2022). Accelerating CO2 reduction on novel double perovskite oxide with sulfur, carbon incorporation: synergistic electronic and chemical engineering. Chem. Eng. J. 446, 137161. doi:10.1016/j.cej.2022.137161
Rehman, F., Pervez, A., Mahmood, Q., and Nawab, B. (2017). Wastewater remediation by optimum dissolve oxygen enhanced by macrophytes in constructed wetlands. Ecol. Eng. 102, 112–126. doi:10.1016/j.ecoleng.2017.01.030
Rehman, F., Usman, A., Ditta, A., Khan, F. S., Mahmood, Q., Alataway, A., et al. (2023). Optimal root oxygen release from two macrophytes Saururus cernuus L. and Pistia stratiotes L. varies with light and temperature in simulated constructed wetlands microcosms. Rhizosphere 26, 100697. doi:10.1016/j.rhisph.2023.100697
Selvaraj, D., and Velvizhi, G. (2023). Self-sustained semi-pilot scale Hybrid Eco-Electrogenic Engineered System for the wastewater treatment and bioenergy generation. J. Water Process Eng. 51, 103474. doi:10.1016/j.jwpe.2022.103474
Shaikh, R., Rizvi, A., Quraishi, M., Pandit, S., Mathuriya, A. S., Gupta, P. K., et al. (2021). Bioelectricity production using plant-microbial fuel cell: present state of art. South Afr. J. Bot. 140, 393–408. doi:10.1016/j.sajb.2020.09.025
Sharma, M., Salama, E. S., Zhang, P., Zhang, L., Xing, X., Yue, J., et al. (2022). Microalgae-assisted microbial fuel cells for electricity generation coupled with wastewater treatment: biotechnological perspective. J. Water Process Eng. 49, 102966. doi:10.1016/j.jwpe.2022.102966
South African Human Rights commission (2018) The right to water and sanitation. Johannesburg: SAHRC, 1–10. Available at: https://www.sahrc.org.za/home/21/files/SAHRC%20Water%20and%20Sanitation%20revised%20pamphlet%2020%20March%202018.pdf (Accessed: April 11, 2024).
Srivastava, P., Yadav, A. K., and Mishra, B. K. (2015). The effects of microbial fuel cell integration into constructed wetland on the performance of constructed wetland. Bioresour. Technol. 195, 223–230. doi:10.1016/j.biortech.2015.05.072
Stoll, Z. A., Dolfing, J., and Xu, P. (2018). Minimum performance requirements for microbial fuel cells to achieve energy-neutral wastewater treatment. Water 10 (3), 243. doi:10.3390/w10030243
Thakur, S., and Das, B. (2021a). Bio-electrochemical evaluation of two-stage constructed wetland microbial fuel cells with high strength raw domestic wastewater and simultaneous energy recovery. Water Environ. J. 35 (4), 1239–1248. doi:10.1111/wej.12714
Thakur, S., and Das, B. (2021b). Investigation on microbial fuel cells fabricated from recyclable materials for energy generation and wastewater treatment. Nat. Environ. Pollut. Technol. 20 (4), 1555–1563. doi:10.46488/NEPT.2021.v20i04.017
Traoré, A. N., Mulaudzi, K., Chari, G. J., Foord, S. H., Mudau, L. S., Barnard, T. G., et al. (2016). The impact of human activities on microbial quality of rivers in the Vhembe District, South Africa. Int. J. Environ. Res. Public Health 13 (8), 817. doi:10.3390/ijerph13080817
Ullah, K., Khan, K., and Ullah, A. (2021). Synthesis and structural characterization of energy crop peelu methyl esters, using hybrid metallic nano-particles. A step forward to bioenergy industry. Fuel 300, 119241. doi:10.1016/j.fuel.2020.119241
Wang, H., Park, J. D., and Ren, Z. J. (2015). Practical energy harvesting for microbial fuel cells: a review. Environ. Sci. Technol. 49 (6), 3267–3277. doi:10.1021/es5047765
Wang, L., Xu, D., Zhang, Q., Liu, T., and Tao, Z. (2022). Simultaneous removal of heavy metals and bioelectricity generation in microbial fuel cell coupled with constructed wetland: an optimization study on substrate and plant types. Environ. Sci. Pollut. Res. 29, 768–778. doi:10.1007/s11356-021-15688-3
Wang, W., Zhang, Y., Li, M., Wei, X., Wang, Y., Liu, L., et al. (2020). Operation mechanism of constructed wetland-microbial fuel cells for wastewater treatment and electricity generation: a review. Bioresour. Technol. 314, 123808. doi:10.1016/j.biortech.2020.123808
Wilson, Z., and Pfaff, B. (2008). Religious, philosophical and environmentalist perspectives on potable wastewater reuse in Durban, South Africa. Desalination 228 (1-3), 1–9. doi:10.1016/j.desal.2007.07.022
Xiang, W., Zhang, X., Chen, J., Zou, W., He, F., Hu, X., et al. (2020). Biochar technology in wastewater treatment: a critical review. Chemosphere 252, 126539. doi:10.1016/j.chemosphere.2020.126539
Zeb, B. S., Mahmood, Q., Jadoon, S., Pervez, A., Irshad, M., Bilal, M., et al. (2013). Combined industrial wastewater treatment in anaerobic bioreactor posttreated in constructed wetland. BioMed Res. Int. 2013 (1), 1–8. doi:10.1155/2013/957853
Nomenclature
Keywords: wastewater treatment, constructed wetland-microbial fuel cell, power generation, developing countries, effectiveness, macrophytes, biological sand filter, power density
Citation: Jacobs DG, Kachienga LO, Rikhotso MC, Abia ALK, Traoré AN and Potgieter N (2024) Assessing the current situation of constructed wetland-microbial fuel cells as an alternative power generation and wastewater treatment in developing countries. Front. Energy Res. 12:1448730. doi: 10.3389/fenrg.2024.1448730
Received: 13 June 2024; Accepted: 31 July 2024;
Published: 09 September 2024.
Edited by:
Anwar Ullah, COMSATS Institute of Information Technology, PakistanReviewed by:
Hemalatha Manupati, Greeneple Inc., Republic of KoreaCopyright © 2024 Jacobs, Kachienga, Rikhotso, Abia, Traoré and Potgieter. This is an open-access article distributed under the terms of the Creative Commons Attribution License (CC BY). The use, distribution or reproduction in other forums is permitted, provided the original author(s) and the copyright owner(s) are credited and that the original publication in this journal is cited, in accordance with accepted academic practice. No use, distribution or reproduction is permitted which does not comply with these terms.
*Correspondence: Leonard Owino Kachienga, bGVva2FjaGllbmdhQGdtYWlsLmNvbQ==