- Department of Mechanical Engineering, Covenant University, Ota, Nigeria
Hydrogen is an abundant element and a flexible energy carrier, offering substantial potential as an environmentally friendly energy source to tackle global energy issues. When used as a fuel, hydrogen generates only water vapor upon combustion or in fuel cells, presenting a means to reduce carbon emissions in various sectors, including transportation, industry, and power generation. Nevertheless, conventional hydrogen production methods often depend on fossil fuels, leading to carbon emissions unless integrated with carbon capture and storage solutions. Conversely, green hydrogen is generated through electrolysis powered by renewable energy sources like solar and wind energy. This production method guarantees zero carbon emissions throughout the hydrogen’s lifecycle, positioning it as a critical component of global sustainable energy transitions. In Africa, where there are extensive renewable energy resources such as solar and wind power, green hydrogen is emerging as a viable solution to sustainably address the increasing energy demands. This research explores the influence of policy frameworks, technological innovations, and market forces in promoting green hydrogen adoption across Africa. Despite growing investments and favorable policies, challenges such as high production costs and inadequate infrastructure significantly hinder widespread adoption. To overcome these challenges and speed up the shift towards a sustainable hydrogen economy in Africa, strategic investments and collaborative efforts are essential. By harnessing its renewable energy potential and establishing strong policy frameworks, Africa can not only fulfill its energy requirements but also support global initiatives to mitigate climate change and achieve sustainable development objectives.
1 Introduction
Energy is vital for daily life, powering numerous aspects of our existence. Historically, Africa has primarily depended on fossil fuels for its energy needs. However, the prolonged use of fossil fuels has highlighted significant drawbacks, such as the substantial emission of greenhouse gases, as illustrated in Figure 1. These disadvantages have led to a growing imperative to seek alternative energy sources (Ajayi et al., 2016). The urgent need to combat climate change and cut greenhouse gas emissions has driven the global energy sector towards cleaner and more sustainable options. Within this framework, hydrogen, especially green hydrogen produced from renewable energy, has emerged as a viable solution for reducing carbon emissions in various sectors, including industry, transportation, and power generation (Ewing et al., 2020). With its abundant renewable energy resources, Africa is well-positioned to play a crucial role in the global hydrogen economy.
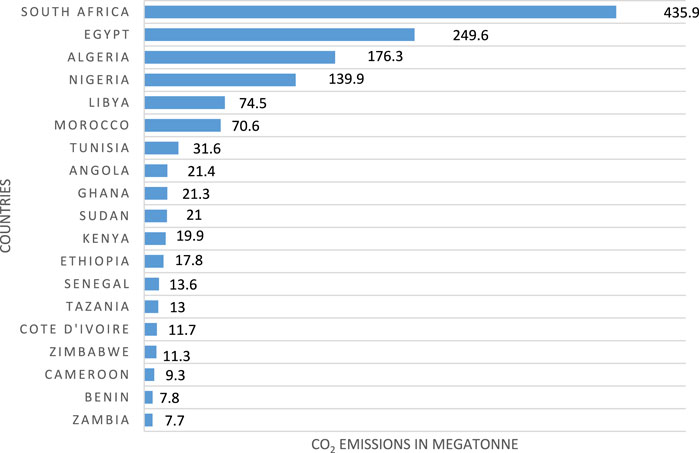
Figure 1. Production-based carbon dioxide (CO2) emissions in Africa in 2021 in mega metric tons (Global Carbon Budget, 2021). https://ourworldindata.org/co2-and-greenhouse-gas-emissions.
1.1 Renewable energy progress in Africa
Africa’s renewable energy landscape has seen remarkable advancements over the past decade. Countries across the continent have been harnessing their abundant natural resources which include solar, wind, and hydro in order to build a more sustainable and resilient energy system (Dirisu et al., 2024). According to the International Renewable Energy Agency (IRENA), the installed capacity for renewable energy in Africa grew by over 24% between 2010 and 2020, reaching approximately 56 GW (GW) by the end of 2020 and as at 2023, the total renewable energy capacity in Africa reached about 62 GW as depicted in Figure 2.
Solar Energy: Africa’s solar energy potential is immense, with several regions receiving over 2,000 kWh/m2 of solar irradiance annually (Ogunniyi and Pienaar, 2019). Countries such as Egypt, Morocco, and South Africa have made significant strides in solar power generation. South Africa had the largest solar energy capacity in Africa as of 2023, reaching over 6 GW (Pourasl et al., 2023). According to the International Energy Agency (IEA, 2023), Egypt recorded the second biggest capacity, at approximately 1.9 GW while Morocco followed with 934 MW of solar energy capacity. The Noor Ouarzazate Solar Complex in Morocco, one of the world’s largest concentrated solar power plants, exemplifies the continent’s capability in harnessing solar energy (Bakhti, 2024).
Wind Energy: Wind power is another critical component of Africa’s renewable energy mix. Coastal regions and highland areas, particularly in countries like Kenya and South Africa, offer excellent wind resources (Ajayi et al., 2016; Merem et al., 2022). The Lake Turkana Wind Power project in Kenya, the largest wind farm in Africa, has an installed capacity of 310 MW (MW) and is a testament to the continent’s wind energy potential (Ogeya et al., 2021).
Hydropower: Hydropower remains the most established renewable energy source in Africa, contributing significantly to the electricity supply in countries like Ethiopia, the Democratic Republic of Congo, Nigeria, and Zambia (Ohunakin et al., 2011; Tiruye et al., 2021). In 2023, 2 GW of hydropower capacity was installed which contributed in increasing the continent’s total energy capacity (Bamisile et al., 2023). The Grand Ethiopian Renaissance Dam (GERD), once fully operational, will be Africa’s largest hydroelectric power plant, significantly boosting the region’s renewable energy capacity (Tiruye et al., 2021).
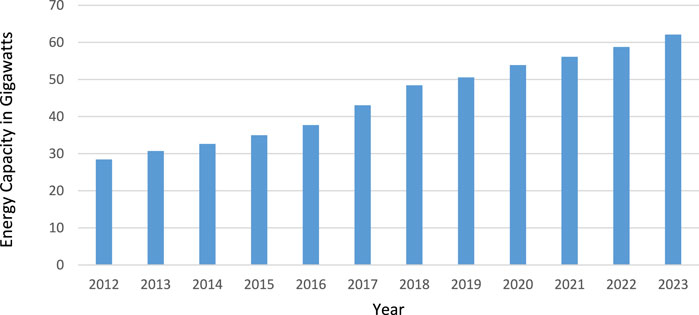
Figure 2. Renewable energy capacity in Africa (IRENA, 2024).
While the progress in renewable energy adoption in Africa is increasing, the emergence of hydrogen as a viable alternative to fossil fuels can also be adopted to aid the diversification of energy sources in Africa.
1.2 Hydrogen as a viable energy source
Hydrogen is gaining traction as a potential replacement for fossil fuels due to increasing concerns about their environmental and financial drawbacks (Mustafa et al., 2020; Pastore et al., 2022). Bhagwat and Olczak (2020) highlight hydrogen’s significant potential in various sectors, such as transportation, power generation, and industrial processes, with global production reaching around 120 million tonnes annually. In contrast, the continuous consumption of fossil fuels releases large amounts of CO2, exacerbating global warming, and depletes energy resources (Mustafa et al., 2020). Currently, China leads the world in hydrogen production and consumption, using approximately 23.9 million metric tons in 2020. The United States follows, with 11.3 million metric tons of global hydrogen consumption (Kumar et al., 2024).
Hydrogen’s appeal lies in its storage capabilities, efficiency, cleanliness, and compatibility with renewable energy sources (Qian et al., 2023). Consequently, hydrogen is crucial in decarbonizing various sectors and mitigating climate change, particularly in transportation and steel manufacturing (Banava, 2023). However, it is important to recognize that while burning hydrogen only emits water vapor, producing it from fossil fuels can still result in CO2 emissions. To achieve truly emission-free hydrogen production, dependence on renewable energy sources is essential (Stavroulakis et al., 2023). According to Liu et al. (2023), green hydrogen is expected to replace fossil fuels in the near future. Table 1 illustrates the colour classification of hydrogen, the energy sources, and the production methods used.
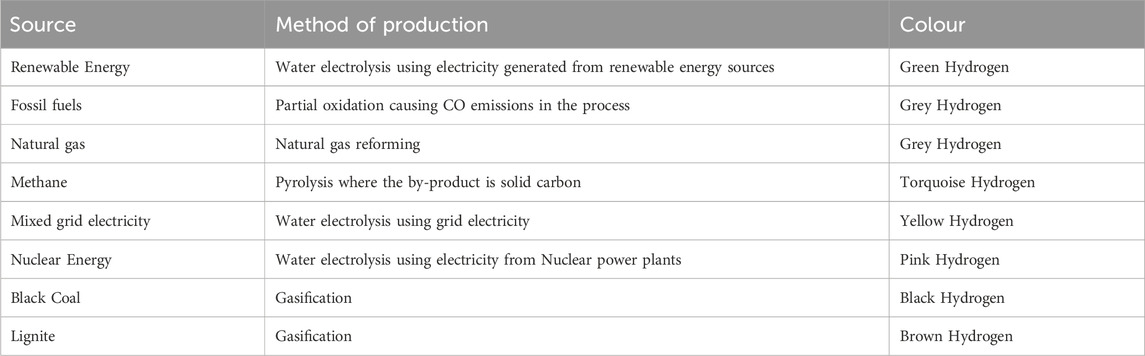
Table 1. Showing the colour scheme of hydrogen (Ajanovic et al., 2022; Khan and Al-Ghamdi, 2023).
Hydrogen, the simplest element, consistently yields a carbon-free molecule regardless of its production method (Van Hoecke et al., 2021). However, the methods used to produce hydrogen vary widely, influencing greenhouse gas emissions such as carbon dioxide (CO2) and methane (CH4) (Sánchez-Bastardo et al., 2021). Green hydrogen, produced from renewable sources like wind, solar, and hydropower, is viewed as a clean alternative to fossil-derived hydrogen (Kakoulaki et al., 2021). Its production represents a significant step toward achieving a carbon-neutral future, with applications spanning transportation, power generation, and industrial sectors (Kovač et al., 2021).
The process of electrolysis can be represented by the chemical equation:
Electrolytic hydrogen generation ensures purity levels exceeding 99.95%, free from hydrocarbon contamination (Burton et al., 2021; Newborough and Cooley, 2020). Electrolytic hydrogen production, unless powered by electricity from fossil fuel stations, is not associated with CO2 or methane emissions, thereby obviating the necessity for carbon capture and storage.
The growing demand for hydrogen across sectors such as transportation, power generation, and manufacturing underscores the urgency of producing hydrogen from renewable energy sources (Ahmed et al., 2023). The potential for green hydrogen to decarbonize hard to abate sectors like heavy industry such as cement production, steel manufacturing, and chemical processing, and shipping and aviation has made it a crucial component of the energy transition (Harichandan et al., 2023) however, several factors need to be considered when producing green hydrogen using renewable energy sources.
1.3 Factors considered when producing green hydrogen
Green hydrogen production hinges fundamentally on several key factors, each crucial for its economic viability, environmental sustainability, and widespread adoption as a clean energy solution.
1.3.1 Renewable energy availability
The production of green hydrogen is fundamentally anchored on the disposal and accessibility of renewable energy sources (Sarker et al., 2023). These sources, primarily solar, wind, and hydroelectric power, are pivotal in the electrolysis process that splits water into hydrogen and oxygen, producing green hydrogen. Regions endowed with a consistent and substantial supply of these renewable resources are naturally poised to become leaders in green hydrogen production, capitalizing on their sustainability and reduced environmental footprint compared to fossil fuel-based methods (Osman et al., 2023). As at 2018 however, Renewable energy sources was the least used in hydrogen production globally as depicted in Figure 3.
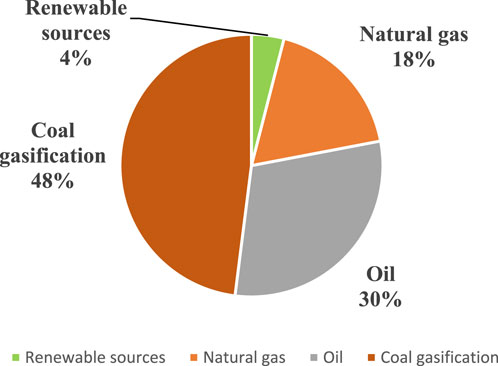
Figure 3. Global Hydrogen production by main source in 2018 (IRENA, 2018; Patonia and Poudineh, 2022).
The variability and intermittency inherent in renewable energy sources present unique challenges to green hydrogen production (Coban et al., 2023). Fluctuations in solar irradiation, wind speeds, or water flow rates can impact the efficiency and reliability of electrolysis processes (Kojima et al., 2023). As such, integrating advanced energy storage systems and smart grid technologies becomes imperative. These systems can store excess energy during peak production periods and release it during low or no production periods, ensuring a continuous and reliable energy supply for hydrogen production.
While some regions may have abundant solar resources, they may lack sufficient wind or hydroelectric power. This disparity necessitates a multifaceted approach to green hydrogen production, incorporating a mix of renewable energy sources to optimize production efficiency and reliability. Strategic planning and investment in interconnected energy grids and cross-regional energy trading can facilitate the seamless integration and distribution of diverse renewable energy sources, bolstering the feasibility and scalability of green hydrogen production initiatives (Okolie et al., 2021; Raman et al., 2022).
1.3.2 Electrolysis efficiency
According to Hassan et al. (2023a), Electrolysis efficiency stands as a pivotal factor influencing the economic viability and scalability of green hydrogen production. Electrolysis is the process through which water is split into hydrogen and oxygen using an electric current. The efficiency of this process determines the amount of electrical energy required to produce a given quantity of hydrogen, directly impacting production costs and overall competitiveness of green hydrogen against conventional hydrogen production methods (Orjuela-Abril. et al., 2023).
Advancements in electrolysis technology have led to improvements in efficiency and reduced energy consumption over recent years. Traditional alkaline electrolysis, although mature and well-established, typically exhibits lower efficiency rates compared to proton exchange membrane (PEM) and solid oxide electrolysis technologies (Li and Baek, 2021). PEM electrolysis according to Zhang et al. (2022) has garnered significant attention due to its higher efficiency, rapid response times, and scalability, making it particularly suitable for decentralized green hydrogen production applications. However, PEM is also associated with high costs and the need for rare and expensive materials (Wappler et al., 2022). Table 2 shows the characteristics of Alkaline, PEM and Solid Oxide (SOE) Electrolysers.
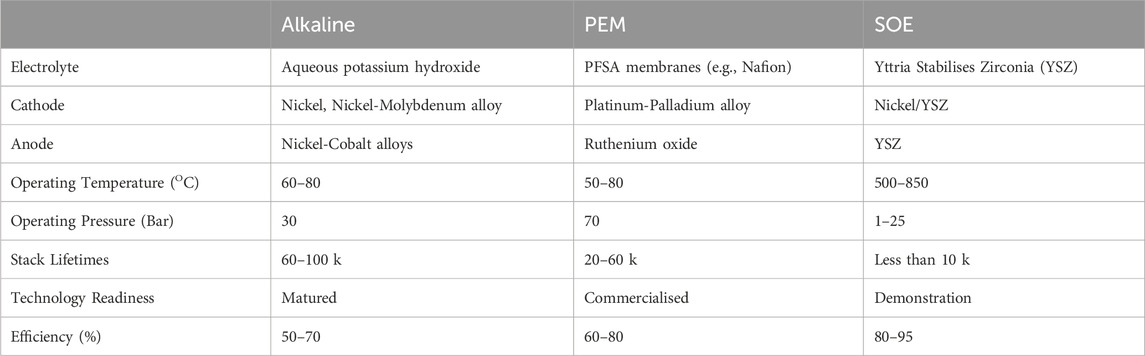
Table 2. Characteristics of Different types of electrolyser technologies (Conte et al., 2009).
Factors influencing electrolysis efficiency extend beyond technological advancements to include operational parameters and system design (Agyekum et al., 2022). Optimizing operating conditions, such as electrolyte concentration, temperature, and pressure, can significantly enhance electrolysis efficiency. Additionally, the integration of renewable energy sources with electrolysis systems, leveraging peak renewable energy production periods, can further improve overall system efficiency and reduce energy costs.
Moreover, according to Jiao et al. (2021), electrolysis efficiency is intrinsically linked to the quality and purity of the produced hydrogen. High-efficiency electrolysis processes typically yield hydrogen with lower impurity levels, meeting stringent quality requirements for various applications, from fuel cells to industrial processes. As such, continuous research and development efforts are focused on enhancing electrolysis efficiency, reducing energy consumption, and improving hydrogen purity to drive down production costs and enhance the competitiveness of green hydrogen.
1.3.3 Scale of production
The gauge of production is another significant factor to consider as it is crucial in the deployment and widespread adoption of green hydrogen technologies (Li et al., 2023). As with many industrial processes, economies of scale can significantly influence the cost-effectiveness and commercial viability of green hydrogen production. Large-scale production facilities can benefit from bulk purchasing, streamlined operations, and optimized supply chains, leading to reduced production costs per unit of hydrogen (Lagioia et al., 2023). The hydrogen production landscape has been dominated by large centralized production facilities, leveraging economies of scale to produce hydrogen from fossil fuel-based feedstocks (Jiao et al., 2021). However, the transition to green hydrogen necessitates a shift towards decentralized and distributed production models, leveraging renewable energy sources and modular electrolysis systems. These smaller-scale production facilities can be strategically located closer to renewable energy generation sites, reducing transmission losses and infrastructure costs while enhancing system efficiency (Osman et al., 2023).
The cost of hydrogen production varies significantly depending on the type of hydrogen, production technique, and the source of electricity (Ishaq et al., 2022). Grey hydrogen, produced from natural gas through steam methane reforming, is currently the cheapest option but is associated with high carbon emissions. Blue hydrogen, which incorporates carbon capture and storage (CCS) with steam methane reforming, has a higher cost due to the additional CCS processes but results in lower emissions (Oni et al., 2022). Green hydrogen, produced via electrolysis using renewable electricity, is the most environmentally friendly option but is also the most expensive. The cost of green hydrogen is heavily influenced by the price of renewable electricity and the efficiency of the electrolysis process. PEM electrolysis, for example, offers higher efficiency and rapid response times but comes with higher costs due to the need for rare and expensive materials (Zhang et al., 2022). Alkaline electrolysis is generally cheaper but less efficient and slower in response (Santos et al., 2021).
Ultimately, the choice of production technique and the type of hydrogen produced will depend on the specific economic and environmental priorities, as well as the availability of renewable energy sources. The push towards decentralized production models aims to optimize these factors by situating hydrogen production closer to renewable energy generation, thereby minimizing costs and maximizing system efficiency. Advancements in electrolysis technology and the decreasing costs of renewable energy sources, such as solar and wind power, are facilitating the development of larger-scale green hydrogen production facilities. The integration of renewable energy with electrolysis systems at scale can result in significant reductions in greenhouse gas emissions and enhance the overall sustainability credentials of green hydrogen (Zhang et al., 2022).
1.3.4 Infrastructure and logistics
The infrastructure and logistics required for producing and distributing green hydrogen are also important factors to consider according to Kumar et al. (2023). This includes the availability of water and renewable energy sources, as well as the transportation and storage of hydrogen. Various hydrogen storage techniques play a crucial role in this process, ensuring that hydrogen can be efficiently stored and readily available when needed (Liu et al., 2023). They include:
• Compressed Gas Storage: This is the most common method, where hydrogen is stored under high pressure in specially designed tanks. Compressed gas storage is relatively straightforward and widely used, especially in the transportation sector. However, it requires robust and heavy containers to withstand high pressures, which can impact efficiency and cost (Orlova et al., 2023).
• Liquid Hydrogen Storage: In this method, hydrogen is cooled to cryogenic temperatures (−253°C) and stored as a liquid. Liquid hydrogen has a higher energy density compared to compressed gas, allowing for more hydrogen to be stored in a given volume. However, the liquefaction process is energy-intensive and requires significant cooling infrastructure, making it costly (Aziz, 2021).
• Metal Hydride Storage: Hydrogen can be stored in metal hydrides, which are compounds formed by the reaction of hydrogen with metals or alloys. Metal hydride storage systems have high energy densities and can operate at lower pressures compared to compressed gas storage. However, they are often heavy and can be expensive due to the materials used (Klopčič et al., 2023).
• Chemical Storage: Hydrogen can be stored in chemical compounds such as ammonia, formic acid, or liquid organic hydrogen carriers (LOHCs). These chemicals can release hydrogen upon demand through chemical reactions. Chemical storage offers the advantage of high energy density and ease of transport but requires additional processes to release the stored hydrogen, which can impact efficiency (Carmo and Stolten, 2022).
• Underground Storage: Large-scale storage of hydrogen can be achieved in underground geological formations such as salt caverns, depleted oil and gas fields, or aquifers. Underground storage can handle large quantities of hydrogen and is suitable for long-term storage. However, it requires suitable geological conditions and significant infrastructure investments (Tarkowski and Uliasz-Misiak, 2022).
Developing an efficient and cost-effective infrastructure for the production and distribution of green hydrogen is critical to the success of the technology (Morlanés et al., 2021).
Countries that have launched National initiatives to promote the deployment of green hydrogen across different sectors include Germany, Japan, South Korea and others. In Europe, the European Union recently launched Hydrogen strategy aim to establish a hydrogen economy that is competitive, sustainable and able to drive long-term economic growth (Khan and Al-Ghamdi, 2023).
The estimation of the green hydrogen (H2) production potential represents the initial stage on the road to integrating the Hydrogen Economy into the energy systems of a country or region (Posso et al., 2023). Fuel cells and other hydrogen-based technologies are increasingly seen as a key pillar of global decarbonization efforts (Lindner, 2023).
Once hydrogen is generated and stored for future use, the focus shifts to methods of converting it into energy. The primary approach involves using hydrogen in fuel cells, which are electrochemical devices that convert chemical energy directly into electrical energy (Rai et al., 2022). Unlike batteries and other energy storage systems that rely on stored energy, fuel cells can continuously supply electricity as long as fuel is provided, offering uninterrupted power (Felseghi et al., 2019).
The cost-effectiveness of hydrogen production through electrolysis primarily hinges on the cost of electricity used in the process and the efficiency of electrolyzers (Martinez de Leon et al., 2023). This underscores the importance of optimizing electrolysis processes to enhance the economic viability of hydrogen as an energy carrier.
2 Applications of green hydrogen
One major advantage of green hydrogen is its environmental cleanliness. Unlike other types of hydrogen, green hydrogen is produced using renewable energy sources, resulting in zero carbon emissions. It can be used in a wide range of applications across various industries, including transportation, power generation, and industrial processes, offering a sustainable and carbon-free alternative.
2.1 Transportation
According to Pasini et al. (2023), green hydrogen can be used for transportation and is considered as one of the most promising options for decarbonizing the different modes of transportation, including cars, buses, trucks, trains, and airplanes as depicted in Figure 4.
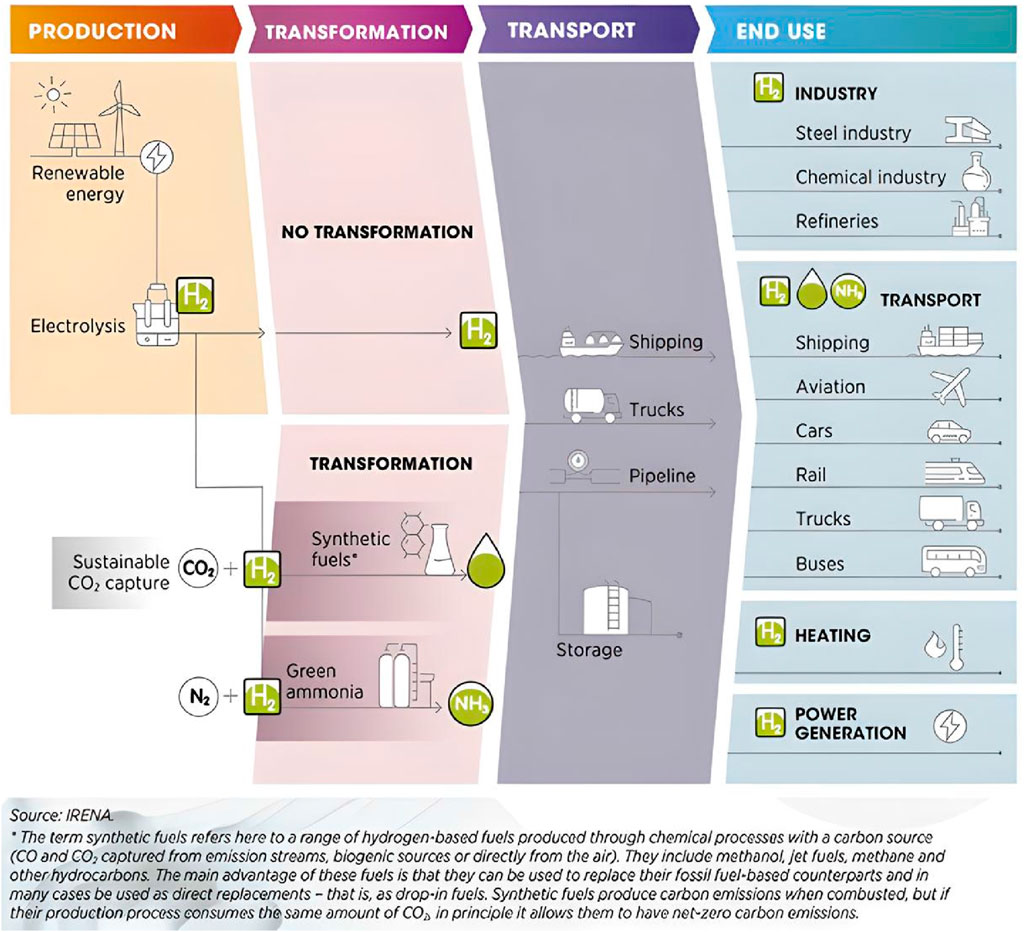
Figure 4. Process of Green hydrogen production to application (IRENA, 2020; Pachencko et al., 2023).
Green hydrogen can be used as a fuel for hydrogen fuel cell vehicles. These vehicles use a fuel cell to convert hydrogen into electricity, which then powers an electric motor (Inci et al., 2021). The only emission from a hydrogen fuel cell vehicle is water vapor, making it a zero-emission vehicle. The use of green hydrogen as a fuel for transportation can help to reduce greenhouse gas emissions and improve air quality (Vardhan et al., 2022).
Green hydrogen may also be used as a fuel for waterborne transport, such as ships and boats (Jie et al., 2023). Hydrogen fuel cell vessels are becoming increasingly popular, especially for ferries and small inland waterway ships (Chatelier, 2023).
2.2 Power generation
Green hydrogen can also be used to generate electricity in fuel cells or through combustion in a gas turbine (Hwang et al., 2023). Green hydrogen can be used as a fuel in fuel cells, which convert the chemical energy of hydrogen and oxygen into electrical energy, water and heat (Liu et al., 2023). Fuel cells are highly efficient and emit only water vapour, making them a sustainable alternative to traditional fossil fuel power plants (Azni et al., 2023).
This can be particularly useful in remote locations or off-grid settings, where traditional power sources may not be available. The use of green hydrogen for power generation can help to reduce greenhouse gas emissions and improve energy security. Green hydrogen can be used in fuel cells (an electrochemical device that converts the chemical energy of green hydrogen and oxygen into electricity) to produce electricity (Yu et al., 2023). Fuel cells have high efficiency and emit only water and heat as byproducts.
Green hydrogen can also be burned in combustion engines to generate electricity (Teoh et al., 2023), similar to burning natural gas, except that the only emission is water vapor. It can also be used in specially designed gas turbines to generate electricity (Hassan et al., 2023a). However, it is important to note that green hydrogen, like other forms of hydrogen, cannot be used in conventional gas turbines without modifications (Bothien et al., 2019). The development of specific turbines capable of efficiently burning hydrogen is an area of active research. Additionally, natural gas-hydrogen blends can be used for electricity generation via modified gas turbines, providing a transitional solution towards cleaner energy. Similarly, green ammonia produced from hydrogen can also be used for electricity generation in gas turbines, offering another pathway to leverage hydrogen for sustainable energy production (Ağbulut et al., 2023).
2.3 Industrial processes
Green hydrogen can also be used in a variety of industrial processes, such as refining, chemical production, and steel production (Genovese et al., 2023). The global transition to climate neutrality will necessitate not only renewable power but also climate-neutral energy carriers such as hydrogen and its derivatives (Runge et al., 2023). These processes typically require large amounts of energy and produce significant greenhouse gas emissions. The use of green hydrogen as a feedstock or fuel for these processes can help to reduce greenhouse gas emissions and improve the sustainability of these industries.
Considering the factors that need to be considered when producing green hydrogen from renewable energy sources, this paper will be able to determine the prospects for the development of green hydrogen in Africa as a continent and also identify the various challenges that may be able to further delay or even hinder the development of green hydrogen in Africa.
3 Prospects for the adoption of green hydrogen for africa
Africa has the potential to become a significant producer and exporter of green hydrogen due to its extensive renewable energy supplies (Panchenko et al., 2023). Green hydrogen adoption prospects in Africa are highly promising, and there are various elements that make this a potentially appealing alternative for the continent some of which are stated below.
3.1 Abundant renewable energy resources
As mentioned earlier, Africa has some of the world’s largest renewable energy resources. Africa has not fully tapped into its abundant renewable energy potential. Estimates indicate that the continent’s total renewable energy resources far exceed current and future energy requirements (Sohani et al., 2023). This underscores the immense opportunity for Africa to harness renewable sources to meet its energy demands sustainably and contribute significantly to global renewable energy goals. The continent has vast solar, wind, and hydropower potential, which make it an ideal location for the production of green hydrogen. With an abundance of renewable energy sources, African countries can produce green hydrogen in a sustainable and environmentally friendly way.
The continent is blessed with vast areas of land and water bodies that receive huge amounts of solar radiation, wind and hydrological resources. Solar energy is especially abundant in Africa, with large areas of the continent receiving high levels of sunlight throughout the year. Wind energy is also abundant in many parts of Africa, especially along the coasts and in high altitude areas. These resources could provide Africa with more energy than it could ever need and pave the way for the continent to become self-sufficient, environmentally sustainable, and economically prosperous.
3.2 Growing demand for energy
According to global demographics, Africa’s population was projected to grow by 2.37 percent in 2022 compared to the previous year. Since 2000, the continent has consistently experienced population growth rates exceeding 2.45 percent, reaching a peak of 2.59 percent between 2012 and 2013. Despite a slight slowdown in recent years, Africa’s population is expected to continue increasing significantly in the foreseeable future, as illustrated in Figure 5 (Saifaddin Galal, 2023).
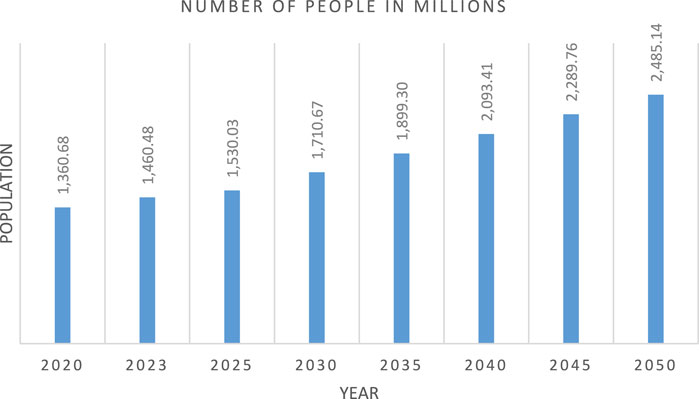
Figure 5. Forecast of the total population of Africa from 2020 to 2050 (Statista, 2024).
This demand is likely to rise more in the future years, and green hydrogen can assist supply it in a sustainable manner. Due to this demand, there is increasing international support and investment in green hydrogen development in Africa. The European Union, for instance, has identified Africa as a key partner for its green hydrogen strategy and has committed to supporting the adoption and development of green hydrogen in the continent (Sadik-Zada, 2021). Other countries, such as Japan and Australia, have also expressed interest in investing in green hydrogen projects in Africa (Kar et al., 2023).
3.3 Potential for export
Africa has the potential to become a significant producer and exporter of green hydrogen due to its extensive renewable energy supplies (Razi, Faran et al., 2022). With a growing emphasis on decarbonization and the need to transition towards clean and sustainable energy sources, green hydrogen presents a significant opportunity for African nations to drive economic growth while addressing environmental challenges.
According to Agyekum (2024), Africa’s strategic geographic location offers access to key global markets, positioning the continent as a crucial player in the emerging hydrogen economy. With proximity to Europe, Asia, and the Middle East, African nations have the opportunity to export green hydrogen to regions seeking to reduce their carbon footprint and meet their renewable energy targets. This not only drives economic growth and job creation but also strengthens Africa’s position as a reliable supplier of clean energy to the world.
Furthermore, Africa’s dedication to sustainable development and climate action strengthens its potential for exporting green hydrogen (Bouchene et al., 2021). Embracing clean energy technologies and reducing reliance on fossil fuels enables African nations to lead in mitigating climate change and promoting environmental stewardship. Exporting green hydrogen not only supports global efforts towards achieving net-zero emissions but also unlocks new economic opportunities and fosters inclusive growth throughout the continent (Nwokolo et al., 2023). This dual benefit of environmental leadership and economic advancement positions Africa strategically in the global energy transition.
4 Advantages of green hydrogen adoption in African countries
Green hydrogen development and adoption in Africa has its benefits to the people, the continent and even the world at large. The adoption of Green hydrogen in African countries can also aid in the transition from polluted energy sources to clean and renewable energy sources.
4.1 Energy security
The potential for solar and wind energy in Africa is very high, with the region having some of the best solar and wind resources globally. According to IRENA (2020), Africa receives about 325 days of bright sunshine annually in many regions, particularly in North Africa and the Sahel region. This high solar irradiance makes these areas ideal for solar energy development (Chun et al., 2022). Additionally, IRENA reports that Africa has an estimated wind energy potential of over 1800 GW, particularly along coastal regions and in high-altitude areas such as the Ethiopian Highlands. The production of green hydrogen using these renewable resources can contribute towards energy security for Africa, particularly in remote areas where grid infrastructure is not readily available (Bhandari, 2022). The development of green hydrogen can provide energy security to African countries in several ways.
By developing the capacity for green hydrogen production, these countries can diversify their energy sources and reduce their dependence on fossil fuels (Szemat-Vielma et al., 2023). This can make them less subject to price fluctuations in fossil fuels for their energy demands. Green hydrogen production, on the other hand, can diversify their energy mix by using renewable energy sources such as solar, wind, and hydro power. This can drastically reduce their reliance on fossil fuels, which are volatile in price and vulnerable to supply disruptions. With a more diversified energy mix, African countries can enhance their energy security and increase their resilience to external shocks (Chu, 2023).
Also, most African countries are net energy importers, which makes them vulnerable to fluctuations in global oil and gas prices (Galimova et al., 2023). Developing their own green hydrogen production capabilities can help them become more energy independent and reduce their dependence on imported fuel (Müller et al., 2023). This can ensure a consistent and predictable energy supply, improve their balance of payments, and improve their overall energy security.
4.2 Economic growth and employment opportunities
The adoption of green hydrogen in Africa can accelerate economic growth and create employment opportunities (Bhagwat and Olczak, 2020). The establishment of the green hydrogen industry can create job opportunities from manufacturing, installation, and maintenance of green hydrogen facilities to the production of green hydrogen fuel cells. The sector can also provide an opportunity for African countries to diversify their economies and reduce their reliance on fossil fuels.
The development of green hydrogen technology can generate economic growth in African countries by creating new job opportunities, developing local industries, and attracting foreign investments. Countries such as Morocco, Egypt, and South Africa have already started investing in this technology (Agyekum et al., 2023), and this could significantly reduce their dependence on fossil fuels and strengthen their energy security.
Green hydrogen production can support sustainable development in African countries by providing a clean and renewable source of energy. The development of a green hydrogen economy can also lead to the creation of new job possibilities, the expansion of local economies, and the development of new industries.
4.3 Reduction of greenhouse gas emissions and dependence on fossil fuels
Green hydrogen offers substantial benefits for Africa, including the potential to reduce global and local greenhouse gas emissions (Li et al., 2023). In Africa, where fossil fuels dominate the energy landscape and contribute significantly to greenhouse gas emissions, green hydrogen can serve as a cleaner alternative in sectors like transportation, power generation, and industry, fostering a more sustainable future. By generating green hydrogen from renewable sources, African countries can advance sustainable development goals, improving air quality and promoting environmental sustainability.
Adopting green hydrogen also holds promise for reducing Africa’s reliance on imported fossil fuels, which exposes the region to volatility in global oil prices and supply disruptions (Odoom et al., 2023). Instead, green hydrogen production offers a stable and locally sourced energy option, enhancing energy security across the continent. Furthermore, embracing green hydrogen technology presents Africa with an opportunity to lead the global transition to renewable energy, a role that has often been overlooked in past energy revolutions (Panchencko et al., 2023).
The adoption of green hydrogen stands to benefit Africa through enhanced energy security, job creation, reduced dependence on imported fossil fuels, and leadership in renewable energy innovation. It is crucial for African governments and stakeholders to prioritize the development of green hydrogen infrastructure, supported by robust policies and regulations that facilitate its widespread adoption across the region.
5 Challenges in the development and adoption of green hydrogen
While the prospects for green hydrogen development in Africa are quite promising, there are several challenges that will need to be addressed in order to make it a viable option for the continent. Lack of infrastructure, high cost of production and limited technical expertise are challenges in the development of green hydrogen.
5.1 Lack of infrastructure
Developing green hydrogen in Africa faces significant challenges, primarily due to inadequate infrastructure (Sadik-Zada, 2021). The production, storage, and transportation of green hydrogen necessitate substantial investments in facilities such as production plants, pipelines, storage tanks, and refueling stations (Schaffert, 2022; Hassan et al., 2023b). Without proper infrastructure, the cost and feasibility of generating and distributing hydrogen could become prohibitively high (Azadnia et al., 2023).
Moreover, the lack of infrastructure poses a barrier to the adoption of hydrogen fuel cell vehicles, as the availability of refueling stations is crucial for their practical use (Geçici et al., 2023; Latapi et al., 2023). This limited accessibility may deter potential consumers from embracing hydrogen technology. Furthermore, insufficient infrastructure could restrict access to the renewable energy sources required for green hydrogen production (Nemmour et al., 2023). In regions lacking adequate renewable energy capacity, the potential for producing green hydrogen may be constrained, hindering the development of a hydrogen-based economy (Khan and Al-Ghamdi, 2023).
Overall, the expansion and widespread adoption of green hydrogen as a sustainable energy solution in Africa hinge significantly on substantial investments in infrastructure. Addressing these infrastructure gaps is crucial to unlocking the full potential of green hydrogen in the region.
5.2 High costs of production
Developing green hydrogen in Africa faces a significant hurdle due to its high production costs (Sontakke, Ujwal, and Santosh Jaju, 2021). While the costs of renewable energy technologies have decreased recently, producing green hydrogen remains more expensive than traditional fossil fuels as shown in Figure 6 (Dong et al., 2022). Green hydrogen production involves electrolyzing water using renewable energy sources like wind and solar power, which adds to its cost compared to hydrogen derived from fossil fuels (Schnuelle et al., 2022).
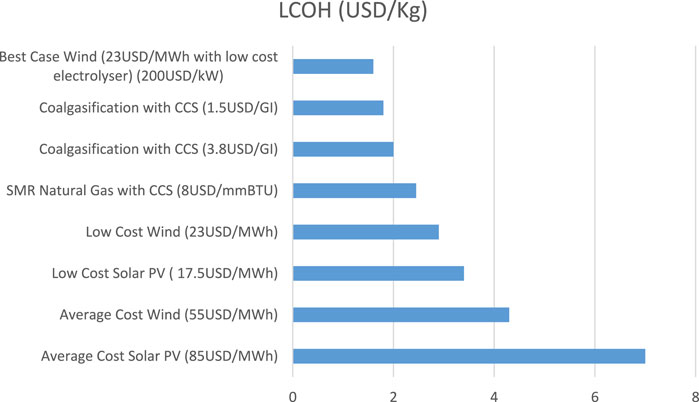
Figure 6. Hydrogen production cost by renewable and non-renewable energies in 2019 (IRENA, 2020).
This higher production cost poses several challenges for green hydrogen development. Firstly, it limits the competitiveness of green hydrogen against cheaper energy sources such as natural gas or gasoline (Kendall Kelvin, 2022). Without substantial government subsidies or incentives, green hydrogen may struggle to penetrate the market (Kar et al., 2023). Despite efforts to reduce electrolyzer technology costs since 2020 (Newborough and Cooley, 2020), the costs remain relatively high. For African countries, which often have limited financial resources, the high cost of green hydrogen production further complicates investment in its development. Addressing these cost barriers is crucial to making green hydrogen a viable and widespread energy solution in the region.
Secondly, the high cost of production could limit the availability of green hydrogen (Terlouw, et al., 2022). If the cost of creating green hydrogen is too high, it may be difficult to scale up production to meet demand, limiting the technology’s potential for widespread adoption. Even with the current subsidy removal which has rapidly increased the price of diesel, gasoline, kerosene and other fuels in Nigeria, the most populated country in Africa, the cost of the conventional fuels will still not match up to that of the production and development of green hydrogen.
The high cost of production could also slow down innovation and research in green hydrogen development (Jilani et al., 2023). If the cost of manufacturing green hydrogen is too expensive, it may be more difficult to invest in more research and development to enhance efficiency and lower the cost of the technology. The average price of producing hydrogen by solar PV and wind energy is much higher than that of fossil fuel, while the decrease in the price of solar PV and wind energy is considered competitive. The International Renewable Energy Agency (IRENA) is expecting that the cost of hydrogen production via renewable energies will fall and become even cheaper than fossil fuel in 2050 (International Renewable Energy Agency, 2020)
5.3 Limited technical/qualified expertise
Developing green hydrogen requires technical expertise in areas such as renewable energy, chemistry, and engineering. Africa as a continent has a lot of academic potential but many African countries have limited qualified expertise to develop and maintain green hydrogen infrastructure (Mneimneh et al., 2023), while some of the personnel that may have the skills and are qualified to develop and maintain green hydrogen infrastructure end up migrating to other countries due to the high rate of unemployment (Rufus et al., 2022). The select few that remain may end up becoming entrepreneurs and having their own personal businesses which may be different from their area of specialization because of the need to generate funds for personal use. This can make it difficult to design and operate green hydrogen production facilities, as well as maintain and repair them over time (Mali et al., 2021).
It will be challenging to develop new procedures and technologies for manufacturing green hydrogen without technical competence. This may limit the ability of the required technical expertise, and the development of green hydrogen technology may be slowed or hampered by concerns about safety, efficiency, and cost-effectiveness.
Green hydrogen production from renewable sources such as solar and wind, for example, necessitates extensive knowledge of electrolysis technology and the materials used in electrolysis cells. There is a danger of errors in the design and execution of the electrolysis process if technical expertise is absent or lacking, which could result in decreased efficiency or even safety issues.
Furthermore, technical skill is required for the transportation and storage of hydrogen (Sun et al., 2023). Hydrogen is a highly combustible gas that must be handled with care to ensure safe transit and storage (Tang Dan et al., 2023). Also, the storage of hydrogen necessitates sophisticated and advanced knowledge of materials science in order to design efficient and cost-effective storage solutions (Singh et al., 2023).
5.4 Policy and regulatory framework
The lack of clear policy and regulatory frameworks is another challenge facing green hydrogen development in Africa (Ballo et al., 2022). In many cases, there is a lack of clarity around the legal and regulatory frameworks that govern the production, distribution, and use of green hydrogen (Chege, 2023; Gordon et al., 2023). This can generate uncertainty for investors and make it difficult to obtain the necessary funds to promote green hydrogen development in developing countries. (Hassan et al., 2023b).
5.5 Competing priorities
Although electrolyser technology is currently being upscaled and cost-reduced in preparation for volume production (Kampouta, 2022), some African countries would still be unable to make it a top priority because it is still very expensive. For example, according to Gigastack phase 1 report 1, 2020, the price of a 100 MW PEM electrolyser system from ITM Power is expected to fall to $530 per kW by 2024, but many African countries may not still be able to concentrate on this as they have other competing priorities which would also involve a large amount of investment and financial assistance (Gigastack, 2020). These African countries face competing priorities and basic needs, such as addressing poverty and improving access to healthcare and education (Ayoo, 2022). These priorities can make it even more difficult to prioritize investment in green hydrogen development, which may be viewed as a longer-term priority in comparison to other more pressing demands.
Also, some governments and businesses may prioritize renewable energy sources like wind and solar power over green hydrogen (Munim et al., 2023), while others may prioritize energy storage technologies like batteries. Competing priorities might be a big obstacle for green hydrogen development. It is possible however to find areas of common ground and work toward a shared vision for the development of a sustainable hydrogen economy through good communication and collaboration among stakeholders.
5.6 Research development and innovation
Research, development, and innovation (RDI) are pivotal in advancing green hydrogen technologies, addressing technical challenges, and enhancing competitiveness in the energy sector. Efforts in RDI focus on several critical areas to accelerate the adoption and scale-up of green hydrogen (Heilala et al., 2022).
In electrolysis efficiency, ongoing research aims to improve the performance of electrolyzers, which convert water into hydrogen and oxygen using electricity. Innovations in materials and catalysts are crucial for enhancing electrolyzer efficiency and durability, reducing energy consumption, and lowering production costs (Burton et al., 2021). Additionally, advancements in storage and transportation technologies are essential. Researchers are exploring novel storage materials and infrastructure solutions to safely and efficiently store and transport hydrogen, ensuring its availability as a reliable energy carrier (Ahmad et al., 2021). Integrating green hydrogen production with renewable energy sources, such as solar and wind power, is another key area. Innovations in grid integration technologies help optimise the use of fluctuating renewable energy outputs, ensuring continuous and efficient hydrogen production (Zachary et al., 2022).
Scaling up production processes for electrolyzers and other hydrogen technologies is critical to achieve economies of scale and reduce costs. Strategic investments in RDI foster collaboration between academia, industry, and government, driving breakthroughs that accelerate the commercialisation of green hydrogen technologies globally.
5.7 Hydrogen standardisation and safety
Hydrogen standardisation and safety are paramount to ensure the reliability, interoperability, and public acceptance of hydrogen technologies. Establishing international standards is crucial for harmonising technical specifications across hydrogen production, storage, transportation, and utilisation. Standardisation efforts aim to facilitate global trade in hydrogen and hydrogen-based products while ensuring compatibility and reliability of equipment and infrastructure (Kumar et al., 2024). Safety regulations play a vital role in mitigating risks associated with hydrogen handling, storage, and utilisation (Li et al., 2022). Developing robust safety standards and certification processes ensures the protection of workers, communities, and the environment. Certification and testing protocols verify compliance with safety standards, promoting confidence in hydrogen technologies among stakeholders.
Public awareness and education initiatives are essential to increase understanding of hydrogen safety principles and practices. Educating stakeholders including industry professionals, policymakers, emergency responders, and the public on safe hydrogen handling and usage fosters a supportive regulatory environment and encourages responsible deployment of hydrogen technologies (Tchouvelev et al., 2019).
By prioritising hydrogen standardisation and safety alongside RDI, stakeholders can pave the way for the widespread adoption of green hydrogen as a clean and sustainable energy solution. These efforts contribute to achieving global energy transition goals and addressing climate challenges effectively.
6 Efforts of African countries in adopting green hydrogen
Green hydrogen is rapidly gaining popularity amongst African countries and the efforts of some of the countries in the adoption of green hydrogen cannot be overlooked. Both South Africa and Morocco are making significant efforts in order to position themselves as leaders in the adoption of green hydrogen in Africa (Pinto and Chege, 2024).
6.1 South Africa’s green hydrogen initiatives
South Africa, with its significant renewable energy resources and industrial capabilities, has emerged as a key player in the development and adoption of green hydrogen (Pinto and Chege, 2024). Recognizing the potential of green hydrogen to transform its energy landscape, reduce carbon emissions, and create economic opportunities, South Africa has launched several initiatives aimed at positioning itself as a leader in the global hydrogen economy. South Africa’s vast renewable energy resources are central to its green hydrogen strategy. The country enjoys abundant solar and wind resources, particularly in the Northern Cape region, which is one of the sunniest places on earth. This makes it an ideal location for large-scale solar photovoltaic (PV) and concentrated solar power (CSP) projects. Additionally, the coastal regions are well-suited for wind energy projects, further enhancing the country’s renewable energy potential (Gaeatlholwe, 2021). Some of South Africa’s initiatives regarding green hydrogen include:
• Hydrogen South Africa (HySA) Programme Launched in 2008 by the Department of Science and Innovation (DSI) which aims to develop and guide innovation along the hydrogen and fuel cell technology value chain (Roos and Wright, 2020). The programme focuses on developing efficient and cost-effective methods for hydrogen production, primarily through water electrolysis using renewable energy, and establishing infrastructure for storage and distribution (Roos, 2021), advancing fuel cell technologies for various applications, including transportation, stationary power generation, and portable power and demonstrating integrated hydrogen systems in real-world applications to showcase their feasibility and benefits.
• Hydrogen Valley Initiative The Hydrogen Valley Initiative, a collaborative effort between the DSI, Anglo American, Bambili Energy, and Engie, aims to create a hydrogen corridor linking the country’s industrial hubs (Grobbelaar and Ngubevana, 2022). Similar to the HySA programme, it seeks to develop a hydrogen production and distribution network along the corridor, promote the use of hydrogen in various sectors, including mining, transportation, and manufacturing and attract investment and create jobs in the emerging hydrogen economy.
There are also renewable hydrogen production projects that South Africa has initiated aimed at producing green hydrogen using renewable energy. They include projects like the Boegoebbai hydrogen project and the Prieska Power reserve project (Kneebone, 2022; Kritzinger and Snyman, 2022).
To support the development of the hydrogen economy, the South African government has introduced the National Hydrogen strategy. The strategy outlines the country’s vision for becoming a leading producer and exporter of green hydrogen and its derivatives. It emphasizes the development of domestic hydrogen markets, international partnerships, and the establishment of a conducive regulatory environment. Incentives and Funding: The government has introduced incentives and funding mechanisms to encourage investment in hydrogen technologies and infrastructure. These include tax breaks, grants, and low-interest loans for hydrogen projects (Bessarabov et al., 2012; Bessarabov and Pollet, 2022).
6.1.1 International collaboration
South Africa actively seeks international partnerships to advance its hydrogen agenda. Key collaborations include the agreements with Germany to develop green hydrogen projects, focusing on technology transfer, capacity building, and market development (Brauner et al., 2023). South Africa also partnered with Japanese companies and research institutions aim to advance hydrogen production technologies and explore potential markets for South African green hydrogen (Patel, 2020).
6.1.2 Challenges and opportunities
While South Africa’s green hydrogen initiatives hold great promise, several challenges need to be addressed:
• Significant investment is required to develop the necessary infrastructure for hydrogen production, storage, and distribution (Ayodele and Munda, 2019).
• Reducing the cost of green hydrogen production to make it competitive with fossil fuels remains a key challenge.
• Establishing a clear and supportive regulatory framework is essential to attract investment and ensure the safe and efficient development of the hydrogen economy.
Despite these challenges, the opportunities for South Africa are substantial. By leveraging its renewable energy resources, industrial capabilities, and strategic location, South Africa can become a major player in the global green hydrogen market. The country’s initiatives not only aim to reduce carbon emissions and enhance energy security but also to create economic growth and job opportunities in the emerging hydrogen sector.
6.2 Morocco’s green hydrogen strategy
Morocco has emerged as a leader in green hydrogen within Africa, establishing the National Hydrogen Commission in 2019 to formulate a comprehensive strategy for green hydrogen production and use. Leveraging its renewable energy capacity, Morocco aims to play a significant role in the global green hydrogen market (van Wijk et al., 2019). The country’s robust renewable energy infrastructure, including projects like the Noor Ouarzazate Solar Complex and Tarfaya Wind Farm, provides a reliable and scalable energy source for green hydrogen production (Bibih et al., 2024; Gawusu and Ahmed, 2024). These initiatives underscore Morocco’s strong commitment to advancing green hydrogen technologies. Additionally, through projects like Green H2A, in collaboration with international partners, Morocco plans to produce green hydrogen for domestic consumption and export, supported by agreements with European nations such as Germany to develop production facilities and export routes (Weko et al., 2024).
6.3 Key projects in African green hydrogen development
In the framework of green hydrogen adoption in Africa, ongoing projects are pivotal in shaping the region’s energy landscape. One notable initiative is the Sahara Green Hydrogen Project, spearheaded by a consortium of international and local partners. This project aims to harness the vast solar energy potential of North Africa, particularly in Morocco and Algeria, to produce green hydrogen for domestic use and export (Tiar et al., 2024). Another significant project is the Hydrogen Initiative in Egypt, which focuses on utilizing renewable energy sources along the Nile Delta region. This initiative aims to establish a sustainable hydrogen economy by leveraging both solar and wind resources abundant in the area and also make the country a potential supplier of low-carbon hydrogen for Europe owing to its proximity and its large renewable energy potential (Ruseckas, 2022). The initiative not only aims at domestic energy security but also positions Egypt as a key player in the international green hydrogen market, catering to Europe’s increasing demand for clean energy.
These ongoing projects underscore the growing momentum towards green hydrogen adoption in Africa, highlighting strategic investments and collaborative efforts aimed at achieving energy security and sustainability goals.
7 Conclusion
The adoption of green hydrogen in Africa offers promising prospects for a sustainable and low-carbon future especially due to the rapid increase in population which automatically increases the energy demand in the continent. Green hydrogen would be advantageous to African countries in several sectors and would be a better fuel compared to conventional fuels. Despite the abundance of renewable energy resources, the continent still faces challenges in harnessing them. Lack of infrastructure, financing, and political issues will have all hindered the growth of renewable energy in Africa. Additionally, many African countries still rely heavily on fossil fuels for their energy needs. However, recent years have seen a growing focus on renewable energy in Africa, with increased investments and policy support aimed at unlocking the vast potential of renewable energy resources on the continent. Addressing these challenges will require collaboration and support from international partners, as well as a commitment from African governments to prioritize and invest in green hydrogen development.
South Africa and Morocco’s efforts in adopting green hydrogen demonstrate their commitment to sustainable energy and climate action. Through strategic policies, investments in renewable energy, and international collaborations, these countries are paving the way for a green hydrogen economy. Their initiatives serve as a model for other African nations and highlight the continent’s potential to contribute significantly to the global green hydrogen landscape. Although it may take some time due to the basic amenities and immediate requirements that African countries must address first, tackling each of these difficulties will be advantageous to the continent and its residents in the long term. African countries may use these chances to establish a sustainable and low-carbon economy with the correct policies, investments, and technical knowledge.
In so doing, green hydrogen may be developed in Africa and could also be used as a substitute for fossil fuels thus reducing the emission of greenhouse gases which causes the depletion of the ozone layer and which may in turn reduce global warming in general.
Author contributions
EO: Conceptualization, Writing–original draft, Writing–review and editing. JD: Methodology, Supervision, Writing–original draft, Writing–review and editing. OK: Methodology, Supervision, Writing–review and editing. ES: Investigation, Methodology, Supervision, Writing–review and editing. OA: Conceptualization, Investigation, Methodology, Project administration, Supervision, Visualization, Writing–review and editing.
Funding
The author(s) declare that no financial support was received for the research, authorship, and/or publication of this article.
Acknowledgments
The Authors want to express their gratitude to Covenant University’s administration and CUCRID Directorate for their support.
Conflict of interest
The authors declare that the research was conducted in the absence of any commercial or financial relationships that could be construed as a potential conflict of interest.
Publisher’s note
All claims expressed in this article are solely those of the authors and do not necessarily represent those of their affiliated organizations, or those of the publisher, the editors and the reviewers. Any product that may be evaluated in this article, or claim that may be made by its manufacturer, is not guaranteed or endorsed by the publisher.
References
Ağbulut, Ü., Yıldız, G., Bakır, H., Polat, F., Biçen, Y., Ergün, A., et al. (2023). Current practices, potentials, challenges, future opportunities, environmental and economic assumptions for Türkiye’s clean and sustainable energy policy: a comprehensive assessment. Sustain. Energy Technol. Assessments 56, 103019. doi:10.1016/j.seta.2023.103019
Agyekum, E. B. (2024). Is Africa ready for green hydrogen energy takeoff? A multi-criteria analysis approach to the opportunities and barriers of hydrogen production on the continent. Int. J. Hydrogen Energy 49, 219–233. doi:10.1016/j.ijhydene.2023.07.229
Agyekum, E. B., Ampah, J. D., Uhunamure, S. E., Shale, K., Onyenegecha, I. P., and Velkin, V. I. (2023). Can africa serve Europe with hydrogen energy from its renewables? assessing the economics of shipping hydrogen and hydrogen carriers to Europe from different parts of the continent. Sustainability 15 (8), 6509. doi:10.3390/su15086509
Agyekum, E. B., Nutakor, C., Agwa, A. M., and Kamel, S. (2022). A critical review of renewable hydrogen production methods: factors affecting their scale-up and its role in future energy generation. Membranes 12 (2), 173. doi:10.3390/membranes12020173
Ahmad, F., Khalid, M., and Panigrahi, B. K. (2021). Development in energy storage system for electric transportation: a comprehensive review. J. Energy Storage 43, 103153. doi:10.1016/j.est.2021.103153
Ahmed, M. R., Barua, T., and Das, B. K. (2023). A comprehensive review on techno-environmental analysis of state-of-the-art production and storage of hydrogen energy: challenges and way forward. Energy Sources, Part A Recovery, Util. Environ. Eff. 45 (2), 5905–5937. doi:10.1080/15567036.2023.2211029
Ajanovic, A., Sayer, M., and Haas, R. (2022). The economics and the environmental benignity of different colors of hydrogen. Int. J. Hydrogen Energy 47 (Issue 57), 24136–24154. doi:10.1016/j.ijhydene.2022.02.094
Ajayi, O. O., Ohijeagbon, O. D., Mercy, O., and Ameh, A. (2016). Potential and econometrics analysis of standalone RE facility for rural community utilization and embedded generation in North-East, Nigeria. Sustain. Cities Soc. 21, 66–77. doi:10.1016/j.scs.2016.01.003
Ayodele, T. R., and Munda, J. L. (2019). The potential role of green hydrogen production in the South Africa energy mix. J. Renew. Sustain. Energy 11 (4), 044301. doi:10.1063/1.5089958
Ayoo, C. (2022). Poverty reduction strategies in developing countries. Rural. Development-Education, Sustain. Multifunct.
Azadnia, A. H., McDaid, C., Amin, M. A., and Seyed, E. H. (2023). Green hydrogen supply chain risk analysis: a european hard-to-abate sectors perspective. Renew. Sustain. Energy Rev. 182, 113371. doi:10.1016/j.rser.2023.113371
Aziz, M. (2021). Liquid hydrogen: a review on liquefaction, storage, transportation, and safety. Energies 14 (18), 5917. doi:10.3390/en14185917
Azni, M. A., Khalid, R.Md, Hasran, U. A., and Kamarudin, S. K. (2023). Review of the effects of fossil fuels and the need for a hydrogen fuel cell policy in Malaysia. Sustainability 15 (5), 4033. doi:10.3390/su15054033
Bakhti, H. (2024). Model-based design of solar thermal power plants. Hamburg, Germany: Universitätsbibliothek Hamburg.
Ballo, A., Valentin, K. K., Korgo, B., Ogunjobi, K. O., Agbo, S. N., Kone, D., et al. (2022). Law and policy review on green hydrogen potential in ECOWAS countries. Energies 15 (7), 2304. doi:10.3390/en15072304
Bamisile, O., Dongsheng, C., Li, J., Adun, H., Olukoya, R., Bamisile, O., et al. (2023). Renewable energy and electricity incapacitation in sub-Sahara Africa: analysis of a 100% renewable electrification in Chad. Energy Rep. 9, 1–12. doi:10.1016/j.egyr.2023.05.049
Banava, A. (2023). The EU Green Deal for climate neutrality by 2050-The European energy and environmental policy for climate change. Greece: European Parliament.
Bessarabov, D., and Pollet, B. G. (2022). “Hydrogen (H2) technologies in the republic of South Africa,” in Hydrogen in an international context (New York, NY: River Publishers), 203–228.
Bessarabov, D., van Niekerk, F., van der Merwe, F., Vosloo, M., North, B., and Mathe, M. (2012). Hydrogen infrastructure within HySA national program in South Africa: road map and specific needs. Energy Procedia 29, 42–52. doi:10.1016/j.egypro.2012.09.007
Bhagwat, S., and Olczak, M. (2020). Green hydrogen: bridging the energy transition in Africa and Europe. Italy: European University Institute.
Bhandari, R. (2022). Green hydrogen production potential in west africa–case of Niger. Renew. Energy 196, 800–811. doi:10.1016/j.renene.2022.07.052
Bibih, M., Choukri, K., El Khaili, M., Babkrani, Y., and Chakir, H. (2024). “Renewable energy integration and green hydrogen prospects in Morocco: a comprehensive analysis of energy transition dynamics,” in 2024 4th International Conference on Innovative Research in Applied Science, Engineering and Technology (IRASET), FEZ, Morocco, 16-17 May, 2024 (IEEE), 1–7.
Bothien, M. R., Ciani, A., Wood, J. P., and Fruechtel, G. (2019). Toward decarbonized power generation with gas turbines by using sequential combustion for burning hydrogen. J. Eng. Gas Turbines Power 141 (12), 121013. doi:10.1115/1.4045256
Bouchene, L., Cassim, Z., Engel, H., Jayaram, K., and Kendall, A. (2021). Green Africa: a growth and resilience agenda for the continent. McKinsey Sustain. 28, 28.
Brauner, S., Lahnaoui, A., Agbo, S., Böschen, S., and Kuckshinrichs, W. (2023). Towards green hydrogen? A comparison of German and African visions and expectations in the context of the H2Atlas-Africa project. Energy Strategy Rev. 50, 101204. doi:10.1016/j.esr.2023.101204
Burton, N. A., Padilla, R. V., Rose, A., and Habibullah, H. (2021). Increasing the efficiency of hydrogen production from solar powered water electrolysis. Renew. Sustain. Energy Rev. 135, 110255. doi:10.1016/j.rser.2020.110255
Carmo, M., and Stolten, D. (2019). “Energy storage using hydrogen produced from excess renewable electricity: power to hydrogen,” in Science and engineering of hydrogen-based energy technologies (Academic Press), 165–199.
Chatelier, J. M. (2023). Entering a new era for electrical vessel on inland waterways. Ship Science and Technology. 16 (32), 21–32.
Chege, K. (2023) “Legal/policy tools and strategies for hydrogen in the low-carbon transition,” in Handbook of energy law in the low-carbon transition, 217.
Chu, L. K. (2023). The role of energy security and economic complexity in renewable energy development: evidence from G7 countries. Environ. Sci. Pollut. Res. 30 (19), 56073–56093. doi:10.1007/s11356-023-26208-w
Chun, G., Days, J., Hwangbo, J., Ismatullayeva, Y., Kamb, E., Kobayashi, C., et al. (2022). Global trends in energy and emissions: key points for policy decision-making.
Coban, H. H., Lewicki, W., Miśkiewicz, R., and Drożdż, W. (2023). The economic dimension of using the integration of highway sound screens with solar panels in the process of generating green energy. Energies 16 (1), 178. doi:10.3390/en16010178
Conte, M., Di Mario, F., Iacobazzi, A., Mattucci, A., Moreno, A., and Ronchetti, M. (2009). Hydrogen as future energy carrier: the ENEA point of view on technology and application prospects. Energies 2 (1), 150–179. doi:10.3390/en20100150
Dirisu, J. O., Salawu, E. Y., Ekpe, I. C., Udoye, N. E., Falodun, O. E., Oyedepo, S. O., et al. (2024). Promoting the use of bioenergy in developing nations: a CDM route to sustainable development. Front. Energy Res. 11, 1184348. doi:10.3389/fenrg.2023.1184348
Dong, Z. Y., Yang, J., Yu, Li, Daiyan, R., and Rose, A. (2022). A green hydrogen credit framework for international green hydrogen trading towards a carbon neutral future. Int. J. Hydrogen Energy 47 (2), 728–734. doi:10.1016/j.ijhydene.2021.10.084
Ewing, M., Israel, B., Jutt, T., Talebian, H., and Stepanik, L. (2020). Hydrogen on the path to net-zero emissions. Calgary, AB, Canada: PEMBINA Institute.
Felseghi, R. A., Carcadea, E., Raboaca, M. S., Trufin, C. N., and Filote, C. (2019). Hydrogen fuel cell technology for the sustainable future of stationary applications. Energies 12 (23), 4593. doi:10.3390/en12234593
Gaeatlholwe, V. T. (2021). A GIS-based approach for the evaluation of the land-use impact of future energy scenarios in South Africa for 2050. Johannesburg (South Africa): University of.
Galimova, T., Ram, M., Bogdanov, D., Fasihi, M., Gulagi, A., Khalili, S., et al. (2023). Global trading of renewable electricity-based fuels and chemicals to enhance the energy transition across all sectors towards sustainability. Renew. Sustain. Energy Rev. 183, 113420. doi:10.1016/j.rser.2023.113420
Gawusu, S., and Ahmed, A. (2024). “Africa's transition to cleaner energy: regulatory imperatives and governance dynamics,” in Energy regulation in Africa: dynamics, challenges, and opportunities (Cham: Springer Nature Switzerland), 25–51.
Geçici, E., Güler, M. G., and Bilgiç, T. (2022). Multi-period planning of hydrogen refuelling stations using flow data: a case study for Istanbul. Int. J. Hydrogen Energy 47 (95), 40138–40155. doi:10.1016/j.ijhydene.2022.08.068
Genovese, M., Schlüter, A., Scionti, E., Piraino, F., Corigliano, O., and Fragiacomo, P. (2023). Power-to-hydrogen and hydrogen-to-X energy systems for the industry of the future in Europe. Int. J. Hydrogen Energy 48, 16545–16568. doi:10.1016/j.ijhydene.2023.01.194
Global Carbon Budget (2021). Global carbon Budget. Available at: https://ourworldindata.org/co2-and-greenhouse-gas-emissions.
Gordon, J. A., Balta-Ozkan, N., and Ali Nabavi, S. (2023). Socio-technical barriers to domestic hydrogen futures: repurposing pipelines, policies, and public perceptions. Appl. Energy 336, 120850. doi:10.1016/j.apenergy.2023.120850
Grobbelaar, N., and Ngubevana, L. (2022). Ensuring a just energy transition through hydrogen: how the G20 can support Africa. South Afr. Inst. Int. Aff., 1–19.
Harichandan, S., Kar, S. K., and Rai, P. K. (2023). A systematic and critical review of green hydrogen economy in India. Int. J. Hydrogen Energy 48, 31425–31442. doi:10.1016/j.ijhydene.2023.04.316
Hassan, Q., Abdulateef, A. M., Abdul Hafedh, S., Al-samari, A., Abdulateef, J., Sameen, A. Z., et al. (2023a). Renewable energy-to-green hydrogen: a review of main resources routes, processes and evaluation. Int. J. Hydrogen Energy 48, 17383–17408. doi:10.1016/j.ijhydene.2023.01.175
Hassan, Q., Sameen, A. Z., Salman, H. M., and Jaszczur, M. (2023b). A roadmap with strategic policy toward green hydrogen production: the case of Iraq. Sustainability 15 (6), 5258. doi:10.3390/su15065258
Heilala, J., Paasi, J., Lanz, M., Aho, M., Salminen, K., and Kutvonen, A. (2022). Sustainable industry X research ecosystem (SIRE): strategic research and innovation agenda SRIA 2022: project deliverable december 2022.
Hwang, J., Maharjan, K., and Cho, H. (2023). A review of hydrogen utilization in power generation and transportation sectors: achievements and future challenges. Int. J. hydrogen energy 48 (74), 28629–28648. doi:10.1016/j.ijhydene.2023.04.024
Inci, M., Büyük, M., Demir, M. H., and İlbey, G. (2021). A review and research on fuel cell electric vehicles: topologies, power electronic converters, energy management methods, technical challenges, marketing and future aspects. Renew. Sustain. Energy Rev. 137, 110648. doi:10.1016/j.rser.2020.110648
International Renewable Energy Agency (2020). Green Hydrogen: a guide to policy making, 52. Abu Dhabi: International Renewable Energy Agency. Available at: https://www.irena.org/-/media/Files/IRENA/Agency/Publication/2020/Nov/IRENA_Green_hydrogen_policy_2020.pdf (Accessed November 20, 2023).
IRENA (2018). Hydrogen from renewable power. Technology outlook for the energy transition. Available at: https://www.irena.org//media/Files/IRENA/Agency/Publication/2018/Sep/IRENA_Hydrogen_from_renewable_power_2018.pdf (Accessed May 20, 2024).
IRENA (2020). Green hydrogen cost reduction. Scaling up electrolysers to meet the 1.5oC climate goal. Available at: https://www.irena.org/-/media/Files/IRENA/Agency/Publication/2020/Dec/IRENA_Green_hydrogen_cost_2020.pdf (Accessed June 19, 2024).
Ishaq, H., Dincer, I., and Crawford, C. (2022). A review on hydrogen production and utilization: challenges and opportunities. Int. J. Hydrogen Energy 47 (62), 26238–26264. doi:10.1016/j.ijhydene.2021.11.149
Jiao, S., Fu, X., Wang, S., and Zhao, Y. (2021). Perfecting electrocatalysts via imperfections: towards the large-scale deployment of water electrolysis technology. Energy and Environ. Sci. 14 (4), 1722–1770. doi:10.1039/d0ee03635h
Jie, S., Zhu, Y., Feng, Y., Yang, J., and Xia, C. (2023). A prompt decarbonization pathway for shipping: green hydrogen, ammonia, and methanol production and utilization in marine engines. Atmosphere 14 (3), 584. doi:10.3390/atmos14030584
Jilani, A., Hussain, S. Z., Melaibari, A. A., and Abu-Hamdeh, N. H. (2022). Development and mechanistic studies of ternary nanocomposites for hydrogen production from water splitting to yield sustainable/green energy and environmental remediation. Polymers 14 (7), 1290. doi:10.3390/polym14071290
Kakoulaki, G., Kougias, I., Taylor, N., Dolci, F., Moya, J., and Jäger-Waldau, A. (2021). Green hydrogen in Europe – a regional assessment: substituting existing production with electrolysis powered by renewables. Energy Convers. Manag. 228, 113649. doi:10.1016/j.enconman.2020.113649
Kampouta, D. (2022). Hydrogen economy-legislative framework and current status. PhD diss., Univ. Piraeus (Greece).
Kar, S. K., Kumar Sinha, A. S., Bansal, R., Shabani, B., and Harichandan, S. (2023). Overview of hydrogen economy in Australia. Wiley Interdiscip. Rev. Energy Environ. 12 (1), e457. doi:10.1002/wene.457
Kendall, K. (2022). Green hydrogen in the UK: progress and prospects. Clean. Technol. 4 (2), 345–355. doi:10.3390/cleantechnol4020020
Khan, M. I., and Al-Ghamdi, S. G. (2023). Hydrogen economy for sustainable development in GCC countries: a SWOT analysis considering current situation, challenges, and prospects. Int. J. Hydrogen Energy 48 (28), 10315–10344. doi:10.1016/j.ijhydene.2022.12.033
Klopčič, N., Grimmer, I., Winkler, F., Sartory, M., and Trattner, A. (2023). A review on metal hydride materials for hydrogen storage. J. Energy Storage 72, 108456. doi:10.1016/j.est.2023.108456
Kneebone, J. (2022). Redrawing the EU’s energy relations: getting it right with African renewable hydrogen.
Kojima, H., Nagasawa, K., Todoroki, N., Ito, Y., Matsui, T., and Nakajima, R. (2023). Influence of renewable energy power fluctuations on water electrolysis for green hydrogen production. Int. J. hydrogen energy 48 (12), 4572–4593. doi:10.1016/j.ijhydene.2022.11.018
Kovač, A., Paranos, M., and Marciuš, D. (2021). Hydrogen in energy transition: a review. Int. J. Hydrogen Energy 46 (16), 10016–10035. doi:10.1016/j.ijhydene.2020.11.256
Kritzinger, A., and Snyman, I. (2022). Socio-economic impact assessment report for the Prieska power reserve solar PV plant and wind energy facility phase 1. Prieska, North. Cape.
Kumar, S., Arzaghi, E., Baalisampang, T., Garaniya, V., and Abbassi, R. (2023). Insights into decision-making for offshore green hydrogen infrastructure developments. Process Saf. Environ. Prot. 174, 805–817. doi:10.1016/j.psep.2023.04.042
Kumar, S., Nanan-Surujbally, A., Sharma, D. P., and Pathak, D. (2024). “Hydrogen safety/standards (national and international document standards on hydrogen energy and fuel cell),” in Towards hydrogen infrastructure (Elsevier), 315–346.
Lagioia, G., Spinelli, M. P., and Vera, A. (2023). Blue and green hydrogen energy to meet European Union decarbonisation objectives. An overview of perspectives and the current state of affairs. Int. J. Hydrogen Energy 48 (4), 1304–1322. doi:10.1016/j.ijhydene.2022.10.044
Latapí, M., Davíðsdóttir, B., and Jóhannsdóttir, L. (2023). Drivers and barriers for the large-scale adoption of hydrogen fuel cells by Nordic shipping companies. Int. J. Hydrogen Energy 48 (15), 6099–6119. doi:10.1016/j.ijhydene.2022.11.108
Li, C., and Baek, J. B. (2021). The promise of hydrogen production from alkaline anion exchange membrane electrolyzers. Nano Energy 87, 106162. doi:10.1016/j.nanoen.2021.106162
Li, H., Cao, X., Liu, Y., Shao, Y., Nan, Z., Teng, L., et al. (2022). Safety of hydrogen storage and transportation: an overview on mechanisms, techniques, and challenges. Energy Rep. 8, 6258–6269. doi:10.1016/j.egyr.2022.04.067
Li, X., Raorane, C. J., Xia, C., Wu, Y., Tran, T. K. N., and Khademi, T. (2023). Latest approaches on green hydrogen as a potential source of renewable energy towards sustainable energy: spotlighting of recent innovations, challenges, and future insights. Fuel 334, 126684. doi:10.1016/j.fuel.2022.126684
Lindner, R. (2023). Green hydrogen partnerships with the Global South. Advancing an energy justice perspective on “tomorrow's oil”. Sustain. Dev. 31 (2), 1038–1053. doi:10.1002/sd.2439
Liu, H., Zhang, J., Sun, P., Zhou, C., Liu, Y., and Fang, Z. Z. (2023a). An overview of TiFe alloys for hydrogen storage: structure, processes, properties, and applications. J. Energy Storage 68, 107772. doi:10.1016/j.est.2023.107772
Liu, L., Zhai, R., and Hu, Y. (2023b). Performance evaluation of wind-solar-hydrogen system for renewable energy generation and green hydrogen generation and storage: energy, exergy, economic, and enviroeconomic. Energy 276, 127386. doi:10.1016/j.energy.2023.127386
Mali, B., Niraula, D., Kafle, R., and Bhusal, A. (2021) “Green hydrogen: production methodology, applications and challenges in Nepal,” in In2021 7th International Conference on Engineering, Applied Sciences and Technology (ICEAST). IEEE, 68–76.
Martinez de Leon, C., Ríos, C., and Brey, J. J. (2023). Cost of green hydrogen: limitations of production from a stand-alone photovoltaic system. Int. J. Hydrogen Energy 48 (32), 11885–11898. doi:10.1016/j.ijhydene.2022.05.090
Merem, E. C., Twumasi, Y., Wesley, J., Olagbegi, D., Crisler, M., Romorno, C., et al. (2022). The evaluation of wind energy potentials in South Africa. Energy Power 12 (1), 9–25. doi:10.5923/j.ep.20221201.02
Mneimneh, F., Ghazzawi, H., Abu Hejjeh, M., Manganelli, M., and Ramakrishna, S. (2023). Roadmap to achieving sustainable development via green hydrogen. Energies 16 (3), 1368. doi:10.3390/en16031368
Morlanés, N., Katikaneni, S. P., Paglieri, S. N., Harale, A., Solami, B., Mani Sarathy, S., et al. (2021). A technological roadmap to the ammonia energy economy: current state and missing technologies. Chem. Eng. J. 408, 127310. doi:10.1016/j.cej.2020.127310
Müller, L. A., Leonard, A., Trotter, P. A., and Stephanie, H. (2023). Green hydrogen production and use in low-and middle-income countries: a least-cost geospatial modelling approach applied to Kenya. Appl. Energy 343, 121219. doi:10.1016/j.apenergy.2023.121219
Munim, Z. H., Chowdhury, M. M. H., Tusher, H. M., and Notteboom, T. (2023). Towards a prioritization of alternative energy sources for sustainable shipping. Mar. Policy 152, 105579. doi:10.1016/j.marpol.2023.105579
Mustafa, A., Lougou, B. G., Shuai, Y., Wang, Z., and Tan, H. (2020). Current technology development for CO2 utilization into solar fuels and chemicals: a review. J. Energy Chem. 49, 96–123. doi:10.1016/j.jechem.2020.01.023
Nemmour, A., Inayat, A., Janajreh, I., and Ghenai, C. (2023). Green hydrogen-based E-fuels (E-methane, E-methanol, E-ammonia) to support clean energy transition: a literature review. Int. J. Hydrogen Energy 48 (75), 29011–29033. doi:10.1016/j.ijhydene.2023.03.240
Newborough, M., and Cooley, G. (2020). Developments in the global hydrogen market: the spectrum of hydrogen colours. Fuel Cells Bull. 2020 (11), 16–22. doi:10.1016/s1464-2859(20)30546-0
Nwokolo, S. C., Singh, R., Khan, S., Kumar, A., and Luthra, S. (2023). “Technological pathways to net-zero goals in africa,” in Africa's path to net-zero: exploring scenarios for a sustainable energy transition (Cham: Springer Nature Switzerland), 93–210.
Odoom, R., Brännlund, R., Amin, K., and Nanzoninge, J. (2023). Oil and gas energy security. Econ. Oil Gas Industry Emerg. Mark. Dev. Econ., 5.
Ogeya, M. C., Osano, P., Kingiri, A., and Okemwa, J. M. (2021). Challenges and opportunities for the expansion of renewable electrification in Kenya. Youba Sokona, Vice-Chair Intergov. Panel Clim. Change (IPCC) 46, 46–70. doi:10.4324/9781003054665-3
Ogunniyi, E., and Pienaar, C. (2019). “Paradox of Africa’s renewable energy potentials and quest towards powering africa,” in 2019 IEEE PES/IAS PowerAfrica (IEEE), 556–562.
Ohunakin, O. S., Ojolo, S. J., and Ajayi, O. O. (2011). Small hydropower (SHP) development in Nigeria: an assessment. Renew. Sustain. Energy Rev. 15 (4), 2006–2013. doi:10.1016/j.rser.2011.01.003
Okolie, J. A., Patra, B. R., Mukherjee, A., Nanda, S., Dalai, A. K., and Kozinski, J. A. (2021). Futuristic applications of hydrogen in energy, biorefining, aerospace, pharmaceuticals and metallurgy. Int. J. hydrogen energy 46 (13), 8885–8905. doi:10.1016/j.ijhydene.2021.01.014
Oni, A. O., Anaya, K., Giwa, T., Di Lullo, G., and Kumar, A. (2022). Comparative assessment of blue hydrogen from steam methane reforming, autothermal reforming, and natural gas decomposition technologies for natural gas-producing regions. Energy Convers. Manag. 254, 115245. doi:10.1016/j.enconman.2022.115245
Orjuela-Abril, S., Torregroza-Espinosa, A., and Duarte-Forero, J. (2023). Innovative technology strategies for the sustainable development of self-produced energy in the Colombian industry. Sustainability 15 (7), 5720. doi:10.3390/su15075720
Orlova, S., Mezeckis, N., and Vasudev, V. P. K. (2023). Compression of hydrogen gas for energy storage: a review. Latv. J. Phys. Tech. Sci. 60 (2), 4–16. doi:10.2478/lpts-2023-0007
Osman, A. I., Chen, L., Yang, M., Msigwa, G., Farghali, M., Fawzy, S., et al. (2023). Cost, environmental impact, and resilience of renewable energy under a changing climate: a review. Environ. Chem. Lett. 21 (2), 741–764. doi:10.1007/s10311-022-01532-8
Panchenko, V. A., Daus, Y. V., Kovalev, A. A., Yudaev, I. V., and Litti, Y. V. (2023). Prospects for the production of green hydrogen: review of countries with high potential. Int. J. Hydrogen Energy 48 (12), 4551–4571. doi:10.1016/j.ijhydene.2022.10.084
Pasini, G., Lutzemberger, G., and Ferrari, L. (2023). Renewable electricity for decarbonisation of road transport: batteries or E-fuels? Batteries 9 (2), 135. doi:10.3390/batteries9020135
Pastore, L. M., Lo Basso, G., Sforzini, M., Santoli, L., and de Santoli, L. (2022). Technical, economic and environmental issues related to electrolysers capacity targets according to the Italian Hydrogen Strategy: a critical analysis. Renew. Sustain. Energy Rev. 166, 112685. doi:10.1016/j.rser.2022.112685
Patel, M. (2020). Green hydrogen: a potential export commodity in a new global marketplace. Trade and Industrial Policy Strategies (TIPS) Pretoria, S. Afr.
Patonia, A., and Poudineh, R. (2022). Cost-competitive green hydrogen: how to lower the cost of electrolysers? (No. 47). OIES Paper: EL.
Pinto, J., and Chege, K. (2024). Regulating green and low-carbon hydrogen in africa: a case study of South Africa. Adv. Sci. Technol. 142, 15–24.
Posso, F., Pulido, A., and Acevedo-Páez, J. C. (2023). Towards the hydrogen economy: estimation of green hydrogen production potential and the impact of its uses in Ecuador as a case study. Int. J. Hydrogen Energy 48 (32), 11922–11942. doi:10.1016/j.ijhydene.2022.05.128
Pourasl, H. H., Barenji, R. V., and Khojastehnezhad, V. M. (2023). Solar energy status in the world: a comprehensive review. Energy Rep. 10, 3474–3493. doi:10.1016/j.egyr.2023.10.022
Qian, Y., Yu, J., Lyu, Z., Zhang, Q., Lee, T. H., Pang, H., et al. (2023). Durable hierarchical phosphorus-doped biphase MoS2 electrocatalysts with enhanced H* adsorption. Carbon Energy 6, e376. doi:10.1002/cey2.376
Rai, A., and Pramanik, S. (2022). Fuel cell utilization for energy storage. Renew. Energy Sustain. Growth Assess., 389–407. doi:10.1002/9781119785460.ch14
Raman, R., Kumar Nair, V., Prakash, V., Patwardhan, A., and Nedungadi, P. (2022). Green-hydrogen research: what have we achieved, and where are we going? Bibliometrics analysis. Energy Rep. 8, 9242–9260. doi:10.1016/j.egyr.2022.07.058
Razi, F., and Ibrahim, D. (2022). Renewable energy development and hydrogen economy in MENA region: a review. Renew. Sustain. Energy Rev. 168, 112763. doi:10.1016/j.rser.2022.112763
Roos, T., and Wright, J. (2020). Powerfuels and green hydrogen (public version). The Council for Scientific and Industrial Research CSIR.
Roos, T. H. (2021). The cost of production and storage of renewable hydrogen in South Africa and transport to Japan and EU up to 2050 under different scenarios. Int. J. hydrogen energy 46 (72), 35814–35830. doi:10.1016/j.ijhydene.2021.08.193
Rufus, O. S., Olusola Edward, O., and Bamidele Abel, I. (2022). Unemployment among technical students: implication for managers of higher education. Education 4 (1), 1–7.
Runge, P., Sölch, C., Albert, J., Wasserscheid, P., Zöttl, G., and Grimm, V. (2023). Economic comparison of electric fuels for heavy duty mobility produced at excellent global sites-a 2035 scenario. Appl. Energy 347, 121379. doi:10.1016/j.apenergy.2023.121379
Ruseckas, L. (2022). Europe and the Eastern Mediterranean: the potential for hydrogen partnership. Germany: SWP Comment. No. 50/2022.
Sadik-Zada, E. R. (2021). Political economy of green hydrogen rollout: a global perspective. Sustainability 13 (23), 13464. doi:10.3390/su132313464
Sánchez-Bastardo, N., Schlogl, R., and Ruland, H. (2021). Methane pyrolysis for zero-emission hydrogen production: a potential bridge technology from fossil fuels to a renewable and sustainable hydrogen economy. Industrial and Eng. Chem. Res. 60 (32), 11855–11881. doi:10.1021/acs.iecr.1c01679
Santos, A. L., Cebola, M. J., and Santos, D. M. (2021). Towards the hydrogen economy—a review of the parameters that influence the efficiency of alkaline water electrolyzers. Energies 14 (11), 3193. doi:10.3390/en14113193
Sarker, A. K., Kalam Azad, A., Rasul, M. G., and Doppalapudi, A. T. (2023). Prospect of green hydrogen generation from hybrid renewable energy sources: a review. Energies 16 (3), 1556. doi:10.3390/en16031556
Schaffert, J. (2022). Progress in power-to-gas energy systems. Energies 16 (1), 135. doi:10.3390/en16010135
Schnuelle, C., Wassermann, T., and Stuehrmann, T. (2022). Mind the gap—a socio-economic analysis on price developments of green hydrogen, synthetic fuels, and conventional energy carriers in Germany. Energies 15 (10), 3541. doi:10.3390/en15103541
Singh, G., Ramadass, K., Dbc DasiReddy, V., Yuan, X., Ok, Y. S., Bolan, N., et al. (2023). Material-based generation, storage, and utilisation of hydrogen. Prog. Mater. Sci. 135, 101104. doi:10.1016/j.pmatsci.2023.101104
Sohani, A., Cornaro, C., Hassan Shahverdian, M., Pierro, M., Moser, D., Nižetić, S., et al. (2023). Building integrated photovoltaic/thermal technologies in Middle Eastern and North African countries: current trends and future perspectives. Renew. Sustain. Energy Rev. 182, 113370. doi:10.1016/j.rser.2023.113370
Sontakke, U., and Jaju, S. (2021) “Green hydrogen economy and opportunities for India,” in IOP Conference Series: Materials Science and Engineering.
Statista (2024). Forecast of the total population of Africa 2020-2050. Available at: https://www.statista.com/statistics/1224205/forecast-of-the-total-population-of-africa/(Accessed July 08, 2024).
Stavroulakis, P., Koutsouradi, M., Kyriakopoulou-Roussou, M.-C., Manologlou, E.-A., Tsioumas, V., and Papadimitriou, S. (2023). Decarbonization and sustainable shipping in a post COVID-19 world. Sci. Afr. 21, e01758. doi:10.1016/j.sciaf.2023.e01758
Sun, D., Guo, Di, and Xie, D. (2023). Using multicriteria decision making to evaluate the risk of hydrogen energy storage and transportation in cities. Sustainability 15 (2), 1088. doi:10.3390/su15021088
Szemat-Vielma, W., Scheibz, J., Kasraoui, N., and Al-Omar, F. (2023). “Sun powered green hydrogen-A comparative analysis from the kingdoms of Morocco and Saudi arabia,” in SPE EuropEC-Europe Energy Conference featured at the 84th EAGE Annual Conference and Exhibition, Dhahran, Saudi Arabia, February 12, 2024.
Tang, D., Tan, G.-L., Li, G.-W., Liang, J.-G., Ahmad, S. M., Bahadur, A., et al. (2023). State-of-the-art hydrogen generation techniques and storage methods: a critical review. J. Energy Storage 64, 107196. doi:10.1016/j.est.2023.107196
Tarkowski, R., and Uliasz-Misiak, B. (2022). Towards underground hydrogen storage: a review of barriers. Renew. Sustain. Energy Rev. 162, 112451. doi:10.1016/j.rser.2022.112451
Tchouvelev, A. V., de Oliveira, S. P., and Neves, N. P. (2019). “Regulatory framework, safety aspects, and social acceptance of hydrogen energy technologies,” in Science and engineering of hydrogen-based energy technologies (Academic Press), 303–356.
Teoh, Y. H., Geok How, H., Danh Le, T., Nguyen, H. T., Lin Loo, D., Rashid, T., et al. (2023). A review on production and implementation of hydrogen as a green fuel in internal combustion engines. Fuel 333, 126525. doi:10.1016/j.fuel.2022.126525
Terlouw, T., Bauer, C., McKenna, R., and Mazzotti, M. (2022). Large-scale hydrogen production via water electrolysis: a techno-economic and environmental assessment. Energy Environ. Sci. 15 (9), 3583–3602.
Tiar, B., Fadlallah, S. O., Serradj, D. E. B., Graham, P., and Aagela, H. (2024). Navigating Algeria towards a sustainable green hydrogen future to empower North Africa and Europe's clean hydrogen transition. Int. J. Hydrogen Energy 61, 783–802. doi:10.1016/j.ijhydene.2024.02.328
Tiruye, G. A., Besha, A. T., Mekonnen, Y. S., Benti, N. E., Gebreslase, G. A., and Tufa, R. A. (2021). Opportunities and challenges of renewable energy production in Ethiopia. Sustainability 13 (18), 10381. doi:10.3390/su131810381
Van Hoecke, L., Laffineur, L., Campe, R., Perreault, P., Verbruggen, S. W., and Lenaerts, S. (2021). Challenges in the use of hydrogen for maritime applications. Energy and Environ. Sci. 14 (2), 815–843. doi:10.1039/d0ee01545h
van Wijk, A., Wouters, F., Rachidi, S., and Ikken, B. (2019). A North africa—europe hydrogen manifesto. Dii Desert Energy.
Vardhan, R. V., Mahalakshmi, R., Anand, R., and Mohanty, A. (2022). “A review on green hydrogen: future of green hydrogen in India,” in 2022 6th International Conference on Devices, Circuits and Systems (ICDCS), Coimbatore, India, 21-22 April 2022 (IEEE), 303–309.
Wappler, M., Unguder, D., Lu, X., Ohlmeyer, H., Teschke, H., and Lueke, W. (2022). Building the green hydrogen market–Current state and outlook on green hydrogen demand and electrolyzer manufacturing. Int. J. Hydrogen Energy 47 (79), 33551–33570. doi:10.1016/j.ijhydene.2022.07.253
Weko, S., Farrand, A., Fakoussa, D., and Quitzow, R. (2024). The politics of green hydrogen cooperation.
Yu, D., Duan, C., and Gu, B. (2023). Design and evaluation of a novel plan for thermochemical cycles and PEM fuel cells to produce hydrogen and power: application of environmental perspective. Chemosphere 334, 138935. doi:10.1016/j.chemosphere.2023.138935
Zachary, J. B., Diaz, L. L., Tatyana, K., and Zhou, Q. A. (2022). Materials research directions toward a green hydrogen economy: a review. ACS Omega 7 (37), 32908–32935. doi:10.1021/acsomega.2c03996
Keywords: green hydrogen, renewable energy, energy transition, energy availability, Africa
Citation: Obanor EI, Dirisu JO, Kilanko OO, Salawu EY and Ajayi OO (2024) Progress in green hydrogen adoption in the African context. Front. Energy Res. 12:1429118. doi: 10.3389/fenrg.2024.1429118
Received: 07 May 2024; Accepted: 06 August 2024;
Published: 23 August 2024.
Edited by:
Sunday Olayinka Oyedepo, Bells University of Technology, NigeriaReviewed by:
Solomon Giwa, Olabisi Onabanjo University, NigeriaLindley Andres Maxwell, Universidad de Antofagasta, Chile
Copyright © 2024 Obanor, Dirisu, Kilanko, Salawu and Ajayi. This is an open-access article distributed under the terms of the Creative Commons Attribution License (CC BY). The use, distribution or reproduction in other forums is permitted, provided the original author(s) and the copyright owner(s) are credited and that the original publication in this journal is cited, in accordance with accepted academic practice. No use, distribution or reproduction is permitted which does not comply with these terms.
*Correspondence: Enoch I. Obanor, ZW5vY2gub2Jhbm9ycGdzQHN0dS5jdS5lZHUubmc=; Enesi Y. Salawu, ZW5lc2kuc2FsYXd1QGNvdmVuYW50dW5pdmVyc2l0eS5lZHUubmc=; Oluseyi O. Ajayi, b2x1c2V5aS5hamF5aUBjb3ZlbmFudHVuaXZlcnNpdC5lZHUubmc=