- New Smart City High-Quality Power Supply Joint Laboratory of China Southern Power Grid, Shenzhen Power Supply Co., Ltd., Shenzhen, China
1 Introduction
With the widespread utilization of distributed renewable energy and flexible power electronics devices in power distribution networks, the paradigm and characteristics of active distribution networks (ADNs) have undergone profound changes as the traditional radial electricity network will be transformed into multi-layer, multi-ring, AC-DC hybrid networks (Wang et al., 2019). Meanwhile, the unpredictable and intermittent nature of distributed wind and photovoltaic generation poses formidable challenges, hindering the efficient utilization of distributed energy resources (DERs). Furthermore, the renewable-dominated ADNs are usually vulnerable to extreme climate events, such as rainstorm, floods, blizzards and forest fires (Shi et al., 2022; Hua et al., 2023; Cao et al., 2024). Such events generally cause sustained faults of distribution system and renewable energy components, and various renewable energy units with power electronic converters will increase fault currents of distribution networks, resulting in voltage drop, network isolation, or even electricity outages (Khodayar et al., 2014; Ma et al., 2018; Yang et al., 2022). With the increasing penetration level of distributed energy storage (Fang et al., 2023) and electric vehicles (Khatami et al., 2020), the support capability of these flexibility resources can be used for improving the power supply quality and system resilience of active distribution networks. Hence, this investigation aims to offer insightful perspectives and discussions on the resilient operations of ADNs with diversified flexibility resources under extreme events.
The main contributions of this work can be twofold as listed: (1) A cellular architecture of ADNs is presented for renewable energy accommodation and interactive emergency support to enhance multi-microgrid resilience with diversified flexibility resources, and a two-stage resilient model including pre-event preparation and post-disaster restoration is formulated to decrease the load curtailment cost; (2) A multi-level distributed control strategy based on alternating direction method of multipliers (ADMM) is presented to solve the proposed two-stage model of cellular ADNs, thereby autonomous resilient operations and privacy preservation of multi-microgrids can be achieved.
2 Cellular architecture of active distribution networks with high renewables
With the growing grid-integration of distributed renewable energies and plug-and-play loads in traditional distribution networks, a series of operational issued are emerged, such as unbalanced three-phase power, voltage sag, transformer overload (Awad et al., 2021; Vijay et al., 2021). The morphology of “cellular architecture” would be an efficient way to solve these electrical issues of future distribution networks. Cellular architecture of active distribution networks is a set of adjustable hexagonal feeder grids for fine-grained point-to-point power transport control of the ADN system with soft open points (SOPs), and then the economy and reliability of ADN operation can be improved. The unit in the cellular architecture of ADNs is actually an autonomous microgrid system, with high reliability of power supply and integration of flexible renewable energy (Wang et al., 2022).
In the cellular architecture of ADNs, heterogeneous microgrids are interconnected with each other with SOPs and smart information exchange stations (SIESs) (Zhou B. et al., 2021a) to improve the operational flexibility and efficient accommodation of DERs, as shown in Figure 1A. In each microgrid, DERs can be converted and regulated by various converters (Xiao et al., 2023) and storages to mitigate fluctuating renewable generations. The microgrid with surplus renewable energy can directly share with other microgrids, while the microgrid can be injected with energy support from others. Besides, communication techniques among microgrids can be performed by SIESs through the advanced measuring instruments and optical fiber deployed at the engaged sites (Cao et al., 2021). SIESs are designed as interfaces to coordinate the operation of multiple autonomous microgrids with DERs in cellular ADNs, and only informs the dispatch center on the total amount of surplus/deficit energy (Zhou B. et al., 2021a). Though the decentralized structure and coordinated operation, the intermittency and volatility of DERs within cellular ADNs can then be accommodated by diversified flexibility resources such as distributed energy storages and electric vehicles, and then power transmission losses and renewable energy curtailments can be alleviated.
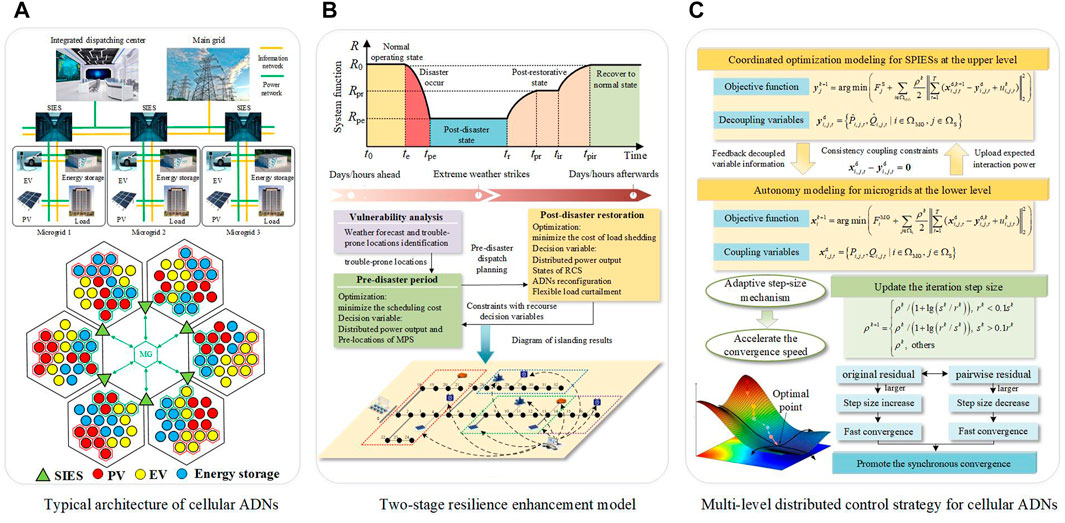
FIGURE 1. The proposed architecture, model and distributed control strategy for cellular ADNs. (A) Typical architecture of cellular ADNs (B) Two-stage resilience enhancement model (C) Multi-level distributed control strategy for cellular ADNs.
The resilience of ADNs is generally used to assess the system capability for withstanding and recovering from significant energy outages under extreme weather events (Chen et al., 2017a). The resilient operation of cellular ADNs can rapidly disconnect microgrids from the main grid so as to protect power components from upstream disturbances, or to shield voltage sensitive loads from sudden voltage drops (Shi et al., 2022). Under the coordinated control of SIES, SOPs have the potential to island the microgrid from interferences such as failures or power quality incidents. After islanding, the reconnection of microgrids can be performed autonomously during fault recovery phase (Llaria et al., 2011). If the microgrid is partly damaged after a major outage, the SIES will restore energy supply services to emergency and nonemergency loads sequentially using dispatchable DERs such as mobile power sources (MPS) (Li C. et al., 2022a) and energy storages. Cellular ADNs can also be resynchronized with the main grid and shift from island mode to grid-connected mode after extreme events. Therefore, the cellular ADN structure can offer an effective and efficient way to utilize diversified flexibility resources to accommodate renewable energy and enhance system resilience.
3 Autonomous resilient operations of distribution networks under extreme climates
Generally, cellular ADNs are vulnerable to extreme climates and then lead to serious power outages and huge economic losses. A conceptual resilience curve related to an extreme weather is shown in Figure 1B to represent pre-disturbance, post-disaster degraded and post-disaster restorative stages for distribution networks (Shi et al., 2022). In this paper, a two-stage resilience dispatch model is proposed to facilitate the pre-disaster prevention and post-disaster recovery of cellular ADNs. In the first stage, MPSs, SIES and SOPs are optimally scheduled to decrease the risk of damage to fault-prone power devices. In the second stage, optimal dispatch of network reconfiguration and flexibility resources is implemented to maintain sufficient and reliable power supplies to critical loads, as shown in Figure 1B.
In the pre-disaster prevention stage, the proposed model aims to dispatch various flexibility resources, including DERs, energy storage systems, MPSs, etc., for minimizing the scheduling cost of diversified flexibility resources and the interaction cost with the main grid. The fault-prone sites are identified based on the fragility model, and MPSs should be proactively pre-located to optimum sites with high potential of failures for survivability enhancement (Wang et al., 2021). DERs should also keep sufficient reserved capacity to cope with voltage fluctuations in the event of power imbalance (Wu et al., 2023). The objective function of pre-disaster preparation model is formulated as Eq. 1 follows,
where T1 refers to the set of pre-disaster scheduling periods;
In the post-disaster restoration stage, the proposed model is to coordinate network reconfiguration, DERs and repair crew for minimizing the cost of load shedding. The network topology is reconstructed for critical load restoration by dynamically controlling status of sectionalizing and tie switches (Chen et al., 2017b; Shi et al., 2021). Diversified DERs are served as reserved generators to support the power supply within islands for reducing load curtailment (Chen et al., 2023). Moreover, the route and sequence of repair crew is optimized to facilitate the recovery of fault power devices (Arif et al., 2020). The objective function of post-disaster restoration model is presented as Eq. 2,
where T2 refers to the set of post-disaster scheduling periods;
In order to verify the resilient operation capability of cellular ADNs with the two-stage model, the comparison of per unit of average voltage is shown in Figure 2A. It can be found that per unit of average voltage in cellular ADNs is more stable compared to conventional ADNs.
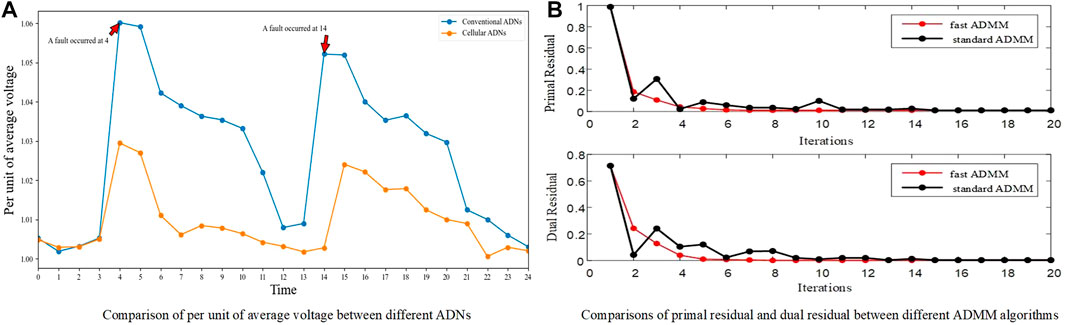
FIGURE 2. Comparison between different ADNs and comparisons different ADMM algorithms. (A) Comparison of per unit of average voltage between different ADNs (B) Comparisons of primal residual and dual residual between different ADMM algorithms.
4 Multi-level distributed control strategy for diversified flexibility resources
Considering the individual autonomy and privacy preservation requirements of multi-microgrids in the cellular ADNs, a multi-level distributed control strategy is proposed for solving the two-stage resilient operation model to minimize overall load curtailment cost. At the upper level, SIESs serve as decision-makers to coordinate the energy exchange among multi-microgrids for maintaining power balance. At the lower level, each microgrid achieves autonomous operation through the coordinated scheduling of diversified flexibility resources (Wang et al., 2022). The proposed distributed control problem can be solved by ADMM algorithm, which combines the advantage of decomposability of dual ascent and excellent convergence properties of multiplier method (Rui et al., 2020; Liu et al., 2018). Taking the pre-event preparation model as an example, the resilient optimization problem of cellular ADNs can be decomposed into subproblems for microgrids and SIESs as Eq. 3. The respective optimization objectives are as Eqs 4, 5,
where
The optimization variables of hierarchical distributed scheduling strategy for cellular ADNs include diversified flexibility resources regulation power comprised of distributed generation, energy storage and MPSs for microgrids, and the power purchased from or sold to the main grid for SIESs. In addition, the power interaction between interconnected microgrids and SIESs needs to be optimized as coupling variables. Hence, the expected interaction power for microgrid i and SIES j are proposed as coupling variables
A Lagrange penalty function is added to the objective functions for microgrids and SIESs, as follows,
where
where
It can be shown from Eqs 7, 8 that both the Lagrange multipliers and iteration step have a dominated influence on convergence properties of the algorithm. The existing conventional method always keeps the iteration step size as a fixed parameter, which restricts the convergence properties owing to the imbalance of primal and dual residuals (Li Z. et al., 2022b; Mhanna et al., 2019). In order to accelerate the convergence speed, an adaptive step-size mechanism can be used to update the iteration step and promote the synchronous convergence of primal and dual residuals as shown in Eq. 12 (Gao et al., 2020; Ghadimi et al., 2015),
when
The iterative process and acceleration principle of multi-level distributed control strategy for cellular ADNs based on adaptive ADMM algorithm are shown in Figure 1C. Comparisons of primal residuals and dual residuals between different ADMM algorithms are shown in Figure 2B. It can be found that the model convergence speed can be enhanced by 18.7% compared to conventional ADMM algorithms through the adaptive correction of step sizes.
5 Discussion and conclusion
A survey on resilient operations of cellular ADNs with flexibility resources under extreme events is presented in this paper. The following are the key findings of this study: 1) The proposed two-stage model utilizes the cellular ADN architecture and diversified flexibility resources to alleviate the damage caused by extreme climate events. 2) A multi-level distributed control strategy based on adaptive ADMM is proposed to solve the two-stage resilient model for the purpose of reducing overall load curtailment cost, and the convergence speed can enhance by 18.7% with the adaptive step-size mechanism. 3) The further research will focus on diversified flexibility resources integration into active distribution networks.
Author contributions
XL: Conceptualization, Writing–original draft. HZ: Data curation, Formal Analysis, Writing–review and editing. ZL: Visualization, Writing–review and editing. QW: Writing–original draft. HX: Writing–original draft.
Funding
The author(s) declare financial support was received for the research, authorship, and/or publication of this article. This work is supported by the Science and Technology Project of China Southern Power Grid (090000KK52222143/SZKJXM20222116).
Conflict of interest
Authors XL, HZ, ZL, QW, and HX were employed by Shenzhen Power Supply Co., Ltd.
The authors declare that this study received funding from the Science and Technology Project of China Southern Power Grid. The funder had the following involvement in the study: conceptualization, data curation and formal analysis, visualization, writing the original draft and editing.
Publisher’s note
All claims expressed in this article are solely those of the authors and do not necessarily represent those of their affiliated organizations, or those of the publisher, the editors and the reviewers. Any product that may be evaluated in this article, or claim that may be made by its manufacturer, is not guaranteed or endorsed by the publisher.
References
Arif, A., Wang, Z., Chen, C., and Wang, J. (2020). Repair and resource scheduling in unbalanced distribution systems using neighborhood search. IEEE Trans. Smart Grid 11, 673–685. doi:10.1109/TSG.2019.2927739
Awad, A. S. A., Turcotte, D., and El-Fouly, T. H. M. (2021). Impact assessment and mitigation techniques for high penetration levels of renewable energy sources in distribution networks: voltage-control perspective. J. Mod. Power Syst. Clean Energy 10, 450. doi:10.35833/MPCE.2020.000177
Cao, Y., Zhou, B., Chung, C. Y., Wu, T., Zheng, L., and Shuai, Z. (2024). A coordinated emergency response scheme for electricity and watershed networks considering spatio-temporal heterogeneity and volatility of rainstorm disasters. IEEE Trans. Smart Grid, 1. doi:10.1109/TSG.2024.3362344
Cao, Y., Zhou, B., Or, S., Chan, K., Liu, N., and Zhang, K. (2021). An interactive tri-level multi-energy management strategy for heterogeneous multi-microgrids. J. Clean. Prod. 319, 128716. doi:10.1016/j.jclepro.2021.128716
Chen, C., Guo, C., Huang, G., Qi, J., and Wang, J. (2017b). Integration of preventive and emergency responses for power grid resilience enhancement. IEEE Trans. Power Syst. 32, 4451–4463. doi:10.1109/TPWRS.2017.2685640
Chen, C., Guo, C., Huang, G., Zhu, B., and Wang, J. (2017a). System resilience enhancement: smart grid and beyond. Front. Eng. Manag. 4, 271. doi:10.15302/J-FEM-2017030
Chen, H., Wang, J., Zhu, J., Xiong, X., Wang, W., and Yang, H. (2023). A two-stage stochastic mixed-integer programming model for resilience enhancement of active distribution networks. J. Mod. Power Syst. Clean Energy 11, 94–106. doi:10.35833/MPCE.2022.000467
Fang, R., Wang, H., Qin, B., Wu, D., and Zhu, Y. (2023). Underground energy storage system supported resilience enhancement for power system in high penetration of renewable energy. Front. Energy Res. 11. doi:10.3389/fenrg.2023.1138318
Gao, H., Wang, J., Liu, Y., Wang, L., and Liu, J. (2020). An improved ADMM-based distributed optimal operation model of AC/DC hybrid distribution network considering wind power uncertainties. IEEE Syst. J. 15, 2201–2211. doi:10.1109/JSYST.2020.2994336
Ghadimi, E., Teixeira, A., Shames, I., and Johansson, M. (2015). Optimal parameter selection for the alternating direction method of multipliers (ADMM): quadratic problems. IEEE Trans. Automatic Control 60, 644–658. doi:10.1109/TAC.2014.2354892
Hua, Z., Zhou, B., Or, S., Zhang, J., Li, C., and Wei, J. (2023). Robust emergency preparedness planning for resilience enhancement of energy-transportation nexus against extreme rainfalls. IEEE Trans. Industry Appl. 60, 1196–1207. doi:10.1109/TIA.2023.3274615
Khatami, R., Parvania, M., and Oikonomou, K. (2020). Deliverable energy flexibility scheduling for active distribution networks. IEEE Trans. Smart Grid 11, 655–664. doi:10.1109/TSG.2019.2927604
Khodayar, M., Che, L., and Shahidehpour, M. (2014). Adaptive protection system for microgrids: protection practices of a functional microgrid system. IEEE Electrification Mag. 2, 66–80. doi:10.1109/MELE.2013.2297031
Li, C., Xi, Y., Lu, Y., Liu, N., Chen, L., Ju, L., et al. (2022a). Resilient outage recovery of a distribution system: co-optimizing mobile power sources with network structure. Prot. Control Mod. Power Syst. 7, 32. doi:10.1186/s41601-022-00256-9
Li, Z., Li, P., Yuan, Z., Xia, J., and Tian, D. (2022b). Optimized utilization of distributed renewable energies for island microgrid clusters considering solar-wind correlation. Electr. Power Syst. Res. 206, 107822. doi:10.1016/j.epsr.2022.107822
Liu, H., Shi, W., and Zhu, H. (2018). Distributed voltage control in distribution networks: online and robust implementations. IEEE Trans. Smart Grid 9, 6106–6117. doi:10.1109/TSG.2017.2703642
Llaria, A., Curea, O., Jiméne, J., and Camblong, H. (2011). Survey on microgrids: unplanned islanding and related inverter control techniques. Renew. energy 36, 2052–2061. doi:10.1016/j.renene.2011.01.010
Ma, S., Wang, Z., and Chen, B. (2018). Resilience enhancement strategy for distribution systems under extreme weather events. IEEE Trans. Smart Grid 9, 1442–1451. doi:10.1109/TSG.2016.2591885
Mhanna, S., Verbic, G., and Chapman, A. (2019). Adaptive ADMM for distributed AC optimal power flow. IEEE Trans. Power Syst. 34, 2025–2035. doi:10.1109/TPWRS.2018.2886344
Rui, P., Ricardo, J., Jean, S., and Manuel, A. (2020). Distributed multi-period three-phase optimal power flow using temporal neighbors. Electr. Power Syst. Res. 182, 106228. doi:10.1016/j.epsr.2020.106228
Shi, Q., Li, F., Olama, M., Dong, J., Xue, Y., Starke, M., et al. (2021). Network reconfiguration and distributed energy resource scheduling for improved distribution system resilience. Int. J. Electr. Power & Energy Syst. 124, 106355. doi:10.1016/j.ijepes.2020.106355
Shi, Q., Zeng, B., Hui, H., Li, F., and Liu, W. (2022). Enhancing distribution system resilience against extreme weather events: concept review, algorithm summary, and future vision. Int. J. Electr. Power & Energy Syst. 138, 107860. doi:10.1016/j.ijepes.2021.107860
Vijay, A., Doolla, S., and Chandorkar, M. (2021). Varying negative sequence virtual impedance adaptively for enhanced unbalanced power sharing among DGs in islanded AC microgrids. IEEE Trans. Energy Convers. 36, 3271–3281. doi:10.1109/TEC.2021.3073150
Wang, D., Zhang, N., Lei, Y., Wang, C., and Zhang, Z. (2019). Prospects for key technologies of new-type urban integrated energy system. Glob. Energy Interconnect. 2, 402–412. doi:10.1016/j.gloei.2019.11.015
Wang, L., Zu, G., Xu, W., Zhu, W., and Luo, F. (2022). Honeycomb distribution networks: concept and central features. 2022 5th International Conference on Energy, Electrical and Power Engineering (CEEPE), April 2022, Chongqing, China. IEEE. doi:10.1109/CEEPE55110.2022.9783369
Wang, Z., Ma, S., Arif, A., and Zhang, Q. (2021). Stochastic pre-event preparation for enhancing resilience of distribution systems. Renew. Sustain. Energy Rev. 152, 111636. doi:10.1016/j.rser.2021.111636
Wu, J., Gu, J., Jin, Z., and Liu, S. (2023). Strategies for improving resilience of regional integrated energy systems in the prevention–resistance phase of integration. Prot. Control Mod. Power Syst. 8, 29. doi:10.1186/s41601-023-00299-6
Xiao, H., He, H., Zhang, L., and Liu, T. (2023). Adaptive grid synchronization based grid-forming control for voltage source converters. IEEE Trans. Power Syst. 39, 4763–4766. doi:10.1109/TPWRS.2023.3338967
Yang, X., Zhou, Z., Zhang, Y., Liu, J., Wen, J., Wu, Q., et al. (2022). Resilience-oriented co-deployment of remote-controlled switches and soft open points in distribution networks. IEEE Trans. Power Syst. 38, 1350–1365. doi:10.1109/TPWRS.2022.3176024
Zhou, B., Zou, J., Chung, C. Y., Wang, H., Liu, N., Voropai, N., et al. (2021a). Multi-microgrid energy management systems: architecture, communication, and scheduling strategies. J. Mod. Power Syst. Clean Energy 9, 463–476. doi:10.35833/MPCE.2019.000237
Keywords: active distribution networks, cellular architecture, distributed algorithm, flexibility resources, resilience enhancement
Citation: Liang X, Zhang H, Liu Z, Wang Q and Xie H (2024) A survey on resilient operations of active distribution networks with diversified flexibility resources. Front. Energy Res. 12:1378325. doi: 10.3389/fenrg.2024.1378325
Received: 29 January 2024; Accepted: 04 March 2024;
Published: 15 March 2024.
Edited by:
Shuai Yao, Cardiff University, United KingdomReviewed by:
Cao Yingping, Hong Kong Polytechnic University, Hong Kong SAR, ChinaXi Lu, Southeast University, China
Copyright © 2024 Liang, Zhang, Liu, Wang and Xie. This is an open-access article distributed under the terms of the Creative Commons Attribution License (CC BY). The use, distribution or reproduction in other forums is permitted, provided the original author(s) and the copyright owner(s) are credited and that the original publication in this journal is cited, in accordance with accepted academic practice. No use, distribution or reproduction is permitted which does not comply with these terms.
*Correspondence: Xiaorui Liang, xrliang@mail.tsinghua.edu.cn