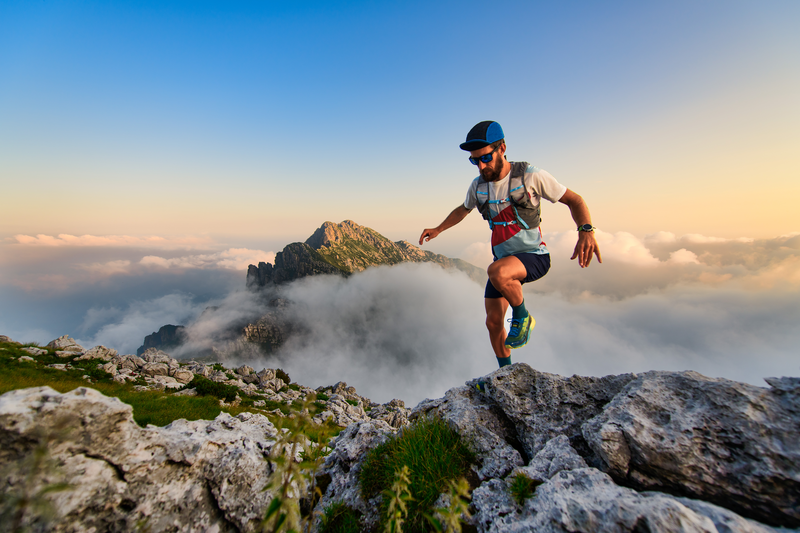
95% of researchers rate our articles as excellent or good
Learn more about the work of our research integrity team to safeguard the quality of each article we publish.
Find out more
ORIGINAL RESEARCH article
Front. Energy Res. , 29 January 2024
Sec. Smart Grids
Volume 12 - 2024 | https://doi.org/10.3389/fenrg.2024.1363198
This article is part of the Research Topic Power System Operation and Optimization Considering High Penetration of Renewable Energy View all 26 articles
With the rapid development of photovoltaic and wind power, the penetration rate of renewable energy in power system is gradually increasing. This escalation poses a challenge as it results in a continuous reduction in the inertia and damping of the power grid, accentuating the issue of frequency stability in power systems. It is one of the effective measures to deal with this risk that renewable energy sources such as wind turbines actively provide frequency support for power grid. This paper carries out research into the examination of wind turbines’ capacity to contribute to system frequency support, considering two aspects: inertia support and primary frequency regulation capabilities. Subsequently, the frequency control method of the wind turbine support system is analyzed, emphasizing the roles of rotor kinetic energy control and power reserve control in facilitating frequency support. The evaluation of the transient frequency support capability of wind turbines is introduced, incorporating factors such as control methods, controller parameters, and the duration of transient frequency support. Key metrics, including accumulated energy and frequency change rate indices during the transient frequency support stage, are proposed to quantitatively assess the transient frequency support capability of wind turbines. These indices provide a comprehensive framework for the quantitative evaluation of wind turbine transient frequency support capabilities.
In recent years, new energy power generation and voltage source converter-high voltage direct current (VSC-HVDC) transmission technology have developed rapidly (Xu, 2020). More and more new energy sources such as wind power and photovoltaic are connected to the power grid through grid-connected converters, which makes the penetration rate of new energy in the power grid continue to increase. The power grid presents the characteristics of high proportion of new energy access and high proportion of power electronic equipment (Wang et al., 2020; Xiao et al., 2023a). Unlike synchronous generators, grid-connected converters are static components without rotational inertia, and cannot respond to frequency changes of the system through mechanical rotors (Wang et al., 2020). Therefore, the inertia and damping of the power grid continue to decline with the increase of new energy penetration (Zhang et al., 2018; Xiao et al., 2023b).
In order to cope with the above risks, new energy stations such as wind power stations need to have the ability to participate in frequency modulation (Ruttledge et al., 2012). In countries with rapid development of new energy, power grid companies have issued relevant regulations, which have made rigid requirements on the frequency of active support systems for energy stations (Yao et al., 2021). The regulations “Technical rule for connecting wind farm into power grid: Q/GDW 1392—2015” issued by the State Grid of China point out that the wind farm should have the ability to participate in system frequency modulation, peak shaving and power reserve. When the output active power of the wind farm is more than 20% of its rated output, all the wind turbines in the wind farm should be able to achieve smooth adjustment of power and participate in the active power control of the system (Wu and Sun, 2018). Germany company TenneT requires that the grid-connected wind farm must have the ability to participate in frequency regulation, and the power capacity of the wind farm participating in frequency regulation is not less than 2% of its installed capacity (Tennet, 2023). The grid-connected regulations of the Danish power grid require that the wind farm needs to reduce the load according to its own operation (Energinet En, 2016). The UK power system operation ESO also requires the frequency modulation capability of the wind farm (ESO, 2021).
Considering the frequency support of the system from the wind turbine level, it is mainly through control that the output active power of the wind turbine can respond to the frequency of the system and participate in the frequency modulation of the system. By introducing virtual inertia and damping into the controller of the wind turbine, the wind turbine has the external characteristics similar to that of the synchronous generator, and has the inertia and primary frequency modulation ability to meet the requirements of the grid for the frequency modulation ability of the grid-connected wind farm (Zhao et al., 2022). At the same time, the frequency response characteristics of the system are improved, and the inertia and damping of the system are increased. The wind turbine can also reserve frequency modulation power for the system through over-speeding control or pitch angle control, providing continuous energy support for the system during the frequency response process (Ouyang et al., 2021). When the wind turbine provides transient frequency support for the power grid, the corresponding evaluation methods and evaluation indexes are needed to evaluate and judge the transient frequency support ability of the wind turbine, so as to facilitate the quantitative analysis of the transient frequency support ability of the wind turbine. However, the existing evaluation indexes of transient frequency support capability, such as frequency change rate and inertia time constant are usually used to evaluate the transient frequency support capability of traditional synchronous generators (Zhang et al., 2022), while the generalized inertia time constant (Sun et al., 2020), equivalent inertia (Li et al., 2020) and equivalent inertia promotion factor (Xu, 2022) are the evaluation indexes of transient frequency support capability for power grid. The transient frequency support capability of the wind turbine is closely related to its control methods and controller parameters. Therefore, the wind turbine needs a set of transient frequency support capability evaluation indicators that can take into account its participation in frequency support control methods and controller parameters.
Therefore, this paper first analyzes the function of wind turbine participating in system frequency support and the role of wind turbine frequency support function in system frequency. Then the specific control method of wind turbine participating in system frequency support is introduced. Secondly, considering the transient frequency support control methods and the controller parameters of the wind turbine, the duration of the transient frequency support, the evaluation index of the transient frequency support capability of the wind turbine is proposed on the basis of the original transient frequency support capability index. Finally, a summary of the full text is given.
The frequency variation of the power grid is often caused by the mismatch between the active power output by the power supply and the active power required by the load. For synchronous generators, the change of system frequency will change the rotor speed. When the speed of the rotor changes, the kinetic energy released by the rotor changes, and the energy absorbed or released by the rotor is manifested as the increase or decrease of the electromagnetic power. The changed electromagnetic power balances the power difference of the system. The wind turbine adjusts its active power output by simulating the controller as a synchronous generator swing equation (Huang et al., 2020), thereby imitating the frequency response process of the synchronous machine and participating in the frequency adjustment of the system (Zhong and Georage, 2011), The frequency response of the wind turbine is shown in Figure 1. The swing equation of the synchronous machine is shown in Eqs 1, 2:
Where,
The inertia support of wind turbine is the function of the output power of wind turbine to respond to the frequency change rate of the system (Morren et al., 2006). When the system frequency changes and the frequency change rate is not 0, the inertia support of the wind turbine begins to work, releasing or absorbing power, and slowing down the speed of frequency change. When the frequency is stable at a new stable operating point, the inertia support also stops working (Milano et al., 2018). The characteristics of inertia support are summarized as follows:
1) The inertia support is a differential feedback control of the system frequency, compared with the primary frequency control, the inertia support has the characteristic of overshooting, and the response speed is faster.
2) In the initial stage of system frequency change, the frequency change is small and the frequency change rate is large, so the inertia support has a large output in the transient frequency support stage.
3) The system frequency will be over to a new stable operating point after a short transient frequency support process. At this time, the inertia support no longer works, so the inertia support lasts for a short time and the accumulated energy is very limited.
4) Since the inertia support only responds to the frequency change rate, its main role is to prevent the system frequency from changing rapidly, and cannot suppress the amplitude of frequency rise or fall.
The primary frequency regulation of the wind turbine is the function of the output power of the wind turbine to respond to the frequency change of the system, and its essence is the active power-frequency droop control of the wind turbine. When the actual operating frequency of the system deviates from the rated frequency, the primary frequency modulation begins to work, continuously releasing or absorbing power, so that the frequency of the system does not continue to fall or continue to rise (Qin et al., 2018). The characteristics of primary frequency modulation are summarized as follows:
1) The primary frequency modulation is a proportional feedback control of the system frequency. Compared with the inertia support control, its response speed is slower.
2) In the early stage of system frequency change, the frequency deviation of the system is small, so the output of primary frequency modulation in the transient frequency support stage is small.
3) Primary frequency modulation is a continuous power support. As long as there is a deviation in the system frequency, the power of the primary frequency modulation support will always exist. Therefore, the primary frequency modulation lasts for a long time, and the accumulated energy is very considerable.
4) The primary frequency modulation is to provide power support when there is a deviation in the frequency to prevent the system frequency from falling or rising continuously, but it cannot limit the rate of change of the frequency.
It is also necessary to point out that neither inertia support nor primary frequency modulation can achieve error-free adjustment of system frequency. Only by introducing the secondary frequency modulation function into the controller can the wind turbine realize the error-free adjustment of the system frequency.
Due to the decoupling of the VSC-HVDC transmission system, the wind turbine cannot directly respond to the frequency change of the AC system to participate in the frequency support (Yao et al., 2021). It is necessary to transmit the frequency signal of the AC system to each wind turbine. The wind turbine adopts the frequency support control method to respond to the frequency change of the AC system (Huang et al., 2017). According to the different principles of frequency modulation, the methods of wind turbines participating in frequency support mainly include: rotor kinetic energy control, power reserve control.
In order to maximize the use of wind energy, the maximum power point tracking (MPPT) method is used when the wind turbine is working normally. In the MPPT mode, the wind turbine supports the frequency of the current system through additional control (Hurtado et al., 2002), including additional inertia control, additional droop control, and the combination of additional inertia control and droop control. Rotor kinetic energy control is to release the kinetic energy stored in the rotor by changing the speed of the wind turbine, and carry out inertia support, so there is no need to retain power reserve.
Inertia control is added to the wind turbine to simulate the inertial response process of the synchronous generator. When the system frequency decreases and the frequency change rate is not 0, the wind turbine releases the kinetic energy of the rotor by changing the speed to support the inertia. The inertial control of the wind turbine can be expressed as Eq. 4:
Where,
Adding droop control to the wind turbine can simulate the governor response of the synchronous generator and realize the function of primary frequency modulation. When the AC system frequency decreases and the frequency deviates from the rated frequency, the wind turbine changes the speed to release the rotor kinetic energy for primary frequency modulation energy support. The droop control of the wind turbine can be expressed as Eq. 5:
Where,
The combination of additional inertia control and droop control of rotor kinetic energy control can make the wind turbine have both inertia support function and primary frequency modulation function, so as to better support the system frequency. The additional inertia control and droop control of the fan can be expressed as Eq. 6:
Where,
Rotor kinetic energy control is to use the kinetic energy of the rotor for power support, but because it does not reserve power reserve, the power and time that the wind turbine can support under this control are very limited. When encountering large power fluctuations, the rotor kinetic energy cannot provide sufficient power support. Therefore, some scholars have proposed the use of power reserve control, so that the wind turbine has a certain frequency modulation reserve power. Power reserve control mainly includes two types of control: over-speeding control and de-loading operation by pitch angle control (Dreidy et al., 2017; Trovato et al., 2020). The schematic diagram of over-speeding control is shown in Figure 2A. Increasing or decreasing the speed of the wind turbine can reduce the output of the wind turbine, but it is easy to cause the small-signal stability of the wind turbine when the speed is reduced. Therefore, overspeed control is generally used to reduce the load of the wind turbine. Let the speed of the wind turbine work at a speed greater than the MPPT state, and the wind turbine runs at a working point less than the maximum output power, leaving a certain power reserve for the frequency support. When the system frequency drops, the wind turbine improves the active power output by reducing the speed. The over-speeding control can be expressed as Eq. 7:
FIGURE 2. Schematic diagram of over-speeding control and de-loading operation by pitch angle control.
Where, PMPPT is the output power of the wind turbine running at the MPPT operating point, d% is the load shedding rate. By adjusting the load shedding rate, the reserve power output of the wind turbine can be adjusted to support the system frequency to varying degrees.
The schematic diagram of the de-loading operation by pitch angle control is shown in Figure 2B. When the speed of the wind turbine is constant, the larger the pitch angle is, the smaller the output active power is. By adjusting the pitch angle
The control block diagram of the control method of the wind turbine participating in the system frequency support is shown in Figure 3. Rotor kinetic energy control can increase the inertia and damping of the system, and power reserve can provide frequency support for the system. Combining the two controls can further improve the frequency stability of the system (Liu et al., 2016), so that the wind turbine can have good frequency response characteristics under various operating conditions. In response to different operating conditions, some scholars have proposed control mode switching methods, so that the wind turbine adopts different frequency support control methods under different operating conditions, thereby improving the applicability of the wind turbine (Attya and Thomas, 2014). Another method to improve the frequency support effect of wind turbines is to optimize the controller parameters of different control methods on the basis of joint control to improve the frequency response characteristics of the system (Pradhan and Chandrasekhar, 2016).
The existing evaluation index of transient frequency support capability usually adopts inertia or frequency change rate at the moment of frequency change (Xu, 2022). However, these two evaluation indexes only consider the frequency support ability of the power supply at the moment when the system frequency changes, and cannot reflect the frequency support ability of the wind turbine in the whole transient frequency support stage. Moreover, these two indexes do not take into account the control method and controller parameters of the frequency support of the wind turbine, and do not reflect the characteristics of the frequency support of the wind turbine. Therefore, considering the control method, controller parameters and the duration of transient frequency support, this paper proposes the cumulative energy in the transient frequency support stage and the average frequency change rate index in the transient frequency support stage to evaluate the frequency support capacity provided by the wind turbine during the transient process.
Energy is the integral of power to time, so this paper considers using energy to represent the support ability of wind turbines in the transient frequency support stage. The power provided by the wind turbine for the frequency support during the whole transient process is integrated to obtain the accumulated energy of the wind turbine in the transient frequency support stage. The specific calculation expression is shown as Eq. 8:
Where,
Where,
When the wind turbine adopts the rotor kinetic energy control with additional droop control,
When the wind turbine adopts the over-speeding control in the power reserve control,
When the wind turbine adopts the de-loading operation by pitch angle control in the power reserve control, due to its slow adjustment speed, it cannot provide transient frequency support for the wind turbine. Therefore, the cumulative energy in the transient frequency support stage of the wind turbine is approximately 0 when this control is adopted.
The value of the transient frequency change rate at a time point cannot represent its frequency support ability over a period of time, while the average value can take into account the value of multiple time points over a period of time, which can better characterize the support ability of the wind turbine in the transient frequency support process. Therefore, this paper considers using the average frequency change rate in the transient frequency support stage to represent the frequency support capacity of the wind turbine in the transient frequency support stage. The average frequency change rate
The average frequency change rate in the transient frequency support stage takes into account the frequency change rate of the wind turbine at five time points from the frequency change to the end of the transient frequency support process, and takes its average to represent the transient frequency support capability of the wind turbine, which can more accurately reflect the frequency support capability of the wind turbine in the whole transient support process. The smaller the value is, the smaller the change rate of the system frequency during the transient frequency support stage is, indicating that the frequency support ability of the wind turbine to the system is stronger.
In this paper, the frequency support capability of wind turbines and the control method of wind turbines participating in frequency support are analyzed, and two evaluation indexes, the cumulative energy in the transient frequency support stage and the average frequency change rate in the transient frequency support stage, are proposed to evaluate the transient frequency support capability of wind turbines. Among them, the cumulative energy in the transient frequency support stage takes into account the control method and controller parameters of the wind turbine participating in the frequency support, and the index can be obtained by integrating the support power provided by the wind turbine in the transient frequency support stage; the average frequency change rate in the transient frequency support stage takes into account the five frequency change rates from the system frequency change to the end of the transient frequency support, and takes the average of the five values to represent the transient frequency support capability of the wind turbine. Summarizing the full text, the following conclusion are obtained:
1) Different from the synchronous generator, which supports the frequency of the AC system by absorbing or releasing power from the mechanical rotor, the wind turbine adjusts its output power through the controller to support the system frequency.
2) The transient frequency support ability of wind turbine is closely related to the frequency support control method and controller parameters.
3) The cumulative energy in the transient frequency support stage and the average frequency change rate in the transient frequency support stage proposed in this paper take into account the entire frequency support stage, which can more accurately characterize the strength of the transient frequency support capability of the wind turbine.
The original contributions presented in the study are included in the article/Supplementary material, further inquiries can be directed to the corresponding author.
HH: Writing–original draft. HX: Formal Analysis, Writing–review and editing. PY: Funding acquisition, Writing–review and editing.
The author(s) declare financial support was received for the research, authorship, and/or publication of this article. This work was supported in part by National Key R&D Program of China under Grant 2023YFB4203102, and in part by Guangzhou Science and Technology Plan Project under Grant 202201010694.
The authors declare that the research was conducted in the absence of any commercial or financial relationships that could be construed as a potential conflict of interest.
All claims expressed in this article are solely those of the authors and do not necessarily represent those of their affiliated organizations, or those of the publisher, the editors and the reviewers. Any product that may be evaluated in this article, or claim that may be made by its manufacturer, is not guaranteed or endorsed by the publisher.
Almeida, R., and Pecas Lopes, J. A. (2007). Participation of doubly fed induction wind generators in system frequency regulation. IEEE Trans. Power Syst. 22, 944–950. doi:10.1109/TPWRS.2007.901096
Attya, A., and Thomas, H. (2014). Wind turbine contribution in frequency drop mitigation – modified operation and estimating released supportive energy. IET Generation, Transm. Distribution 8, 862–872. doi:10.1049/iet-gtd.2013.0512
Dreidy, M., Mokhlis, H., and Saad, M. (2017). Inertia response and frequency control techniques for renewable energy sources: a review. Renew. Sustain. Energy Rev. 69, 144–155. doi:10.1016/j.rser.2016.11.170
Energinet En (2016). Regulations for grid connection. Avaliable at: https://en.energinet.dk/electricity/rules-and-regulations/regulations-for-grid-connection/.
ESO (2021). Grid code documents. Avaliable at: https://www.nationalgrideso.com/industry-information/codes/grid-code-gc/grid-code-documents.
Huang, L., Xin, H., Ju, P., and Hu, J. (2020). Synchronization stability analysis and unified synchronization control structure of grid-connected power electronic devices. Electr. Power Autom. Equip. 40, 10–25. doi:10.16081/j.epae.202009042
Huang, L., Xin, H., Zhang, L., Wang, Z., Wu, K., and Wang, H. (2017). Synchronization and frequency regulation of DFIG-based wind turbine generators with synchronized control. IEEE Trans. Energy Convers. 32, 1251–1262. doi:10.1109/TEC.2017.2675480
Hurtado, S., Gostales, G., de Lara, A., Moreno, N., Carrasco, J. M., Galvan, E., et al. (2002). “A new power stabilization control system based on making use of mechanical inertia of a variable-speed wind-turbine for stand-alone wind-diesel applications,” in IEEE 2002 28th Annual Conference of the Industrial Electronics Society, Seville, Spain, 05-08 November 2002 (IEEE).
Li, D., Zhang, J., Xu, B., Zhao, Y., and Yang, F. (2020). Equivalent inertia assessment in renewable power system considering frequency distribution properties. Power Syst. Technol. 44, 2913–2921. doi:10.13335/j.1000-3673.pst.2019.1935
Liu, Y., Lin, J., Wu, Q., and Zhou, X. (2016). Frequency control of DFIG based wind power penetrated power systems using switching angle controller and AGC. IEEE Trans. Power Syst. 32, 1–1567. doi:10.1109/TPWRS.2016.2587938
Milano, F., Florian, D., Gabriela, H., David, J., and Gregor, V. (2018). “Foundations and challenges of low-inertia systems (invited paper),” in 2018 Power Systems Computation Conference (PSCC), Dublin, Ireland, 11-15 June 2018 (IEEE), 11–15.
Morren, J., de Haan, S. W. H., Kling, W. L., and Ferreira, J. A. (2006). Wind turbines emulating inertia and supporting primary frequency control. IEEE Trans. Power Syst. 21, 433–434. doi:10.1109/TPWRS.2005.861956
Ouyang, J., Yuan, Y., Li, M., Pang, M., Jiang, H., and Zhong, L. (2021). Optimal dispatching method of high-proportion wind power systems considering wind power reserve for frequency adjustment. Power Syst. Technol. 45, 2192–2201. doi:10.13335/j.1000-3673.pst.2020.1049
Pradhan, C., and Chandrasekhar, N. (2016). Enhancement in primary frequency regulation of wind generator using fuzzy-based control. Electr. Power Components Syst. 44, 1669–1682. doi:10.1080/15325008.2016.1183153
Qin, X., Su, L., Cui, Y., Guo, Q., and Xu, X. (2018). Functional orientation discrimination of inertia support and primary frequency regulation of virtual synchronous generator in large power grid. Automation Electr. Power Syst. 42, 36–43. doi:10.7500/AEPS20171122007
Ruttledge, L., Nicholas, W. M., Jonathan, O., and Damian, F. (2012). Frequency response of power systems with variable speed wind turbines. IEEE Trans. Sustain. Energy 3, 683–691. doi:10.1109/TSTE.2012.2202928
Sun, H., Wang, B., Li, W., Yang, C., Wei, W., and Zhao, B. (2020). Research on inertia system of frequency response for power system with high penetration electronics. Proc. CSEE 40, 5179–5192. doi:10.13334/j.0258-8013.pcsee.200493
Tennet (2023). Grid connection regulations. Avaliable at: https://www.tennet.eu/grid-connection-regulations.
Trovato, V., Domenica, C., Maria, D., Giuseppe, F., and Michele, T. (2020). Economic and financial benefits for wind turbines providing frequency response exploiting the kinetic energy or operating part-loaded. IET Gener. Transm. &. 14, 4371–4387. doi:10.1049/iet-gtd.2020.0010
Wang, B., Yang, D., and Cai, G. (2020b). Review of research on power system inertia related issues in the context of high penetration of renewable power generation. Power Syst. Technol. 44, 2998–3007. doi:10.13335/j.1000-3673.pst.2020.0154
Wang, X., Mads, G. T., Wu, H., Liao, Y., Frede, B., and Lennart, H. (2020a). Grid-synchronization stability of converter-based resources—an overview. IEEE Open J. Industry Appl. 1, 115–134. doi:10.1109/OJIA.2020.3020392
Wu, Q., and Sun, Y. (2018). Modeling and modern control of wind power. Hoboken, NJ: John Wiley & Sons.
Xiao, H., Gan, H., Yang, P., Li, L., Li, D., Hao, Q., et al. (2023b). Robust submodule fault management in modular multilevel converters with nearest level modulation for uninterrupted power transmission. IEEE Trans. Power Deliv. 2023, 1–16. doi:10.1109/TPWRD.2023.3343693
Xiao, H., He, H., Zhang, L., and Liu, T. (2023a). Adaptive grid-synchronization based grid-forming control for voltage source converters. IEEE Trans. Power Syst. 2023, 1–4. doi:10.1109/TPWRS.2023.3338967
Xu, Z. (2020). Physical mechanism and research approach of generalized synchronous stability for power systems. Electr. Power Autom. Equip. 09, 3–9. doi:10.16081/j.epae.202008009
Xu, Z. (2022). Reasonable definition and calculation method of power grid strength under the background of new type power systems. High. Volt. Eng. 48, 3805–3819. doi:10.13336/j.1003-6520.hve.20221327
Yao, W., Xiong, Y., Yao, Y., Li, Y., Xin, H., and Wen, J. (2021a). Review of voltage source converter-based high voltage direct current integrated offshore wind farm on providing frequency support control. High. Volt. Eng. 10, 3397–3413.
Yao, Y., Yao, W., Xiong, Y., Zhang, J., and Wen, J. (2021b). Coordinated frequency support and wind turbine preset restoration scheme of VSC-mtdc integrated offshore wind farms. Power Syst. Technol. 47, 3537–3548. doi:10.13336/j.1003-6520.hve.20211122
Zhang, G., Liu, F., Wang, S., and Li, J. (2022). Inertia requirement analysis of frequency stability of renewable-dominant power system. Proc. CSU-EPSA. 34, 81–87. doi:10.19635/j.cnki.csu-epsa.000974
Zhang, X., Chen, Y., Yue, S., Zhu, X., Zhang, D., and Xue, L. (2018). Retrospect and prospect of research on frequency regulation technology of power system by wind power. Power Syst. Technol. 42, 1793–1803. doi:10.13335/j.1000-3673.pst.2018.0359
Zhao, W., Xie, Y., Pan, Y., Yang, X., Xu, P., and Li, F. (2022). Analysis and evaluation of frequency adaptability for wind farm: comprehensive indices system. Proc. CSU-EPSA. 34, 100–108. doi:10.19635/j.cnki.csu-epsa.000888
Keywords: frequency support capability, wind turbines, control strategy, system frequency, transient frequency support capability assessment
Citation: He H, Xiao H and Yang P (2024) Analysis and quantitative evaluation of wind turbine frequency support capabilities in power systems. Front. Energy Res. 12:1363198. doi: 10.3389/fenrg.2024.1363198
Received: 30 December 2023; Accepted: 15 January 2024;
Published: 29 January 2024.
Edited by:
Yuqing Dong, The University of Tennessee, Knoxville, United StatesCopyright © 2024 He, Xiao and Yang. This is an open-access article distributed under the terms of the Creative Commons Attribution License (CC BY). The use, distribution or reproduction in other forums is permitted, provided the original author(s) and the copyright owner(s) are credited and that the original publication in this journal is cited, in accordance with accepted academic practice. No use, distribution or reproduction is permitted which does not comply with these terms.
*Correspondence: Huangqing Xiao, eGlhb2hxQHNjdXQuZWR1LmNu
Disclaimer: All claims expressed in this article are solely those of the authors and do not necessarily represent those of their affiliated organizations, or those of the publisher, the editors and the reviewers. Any product that may be evaluated in this article or claim that may be made by its manufacturer is not guaranteed or endorsed by the publisher.
Research integrity at Frontiers
Learn more about the work of our research integrity team to safeguard the quality of each article we publish.