- 1Pacific Northwest National Laboratory, College Park, MD, United States
- 2Thammasat University Research Unit in Sustainable Energy and Built Environment, Klong Nueng, Thailand
- 3Thammasat Design School, Faculty of Architecture and Planning, Thammasat University, Klong Nueng, Thailand
- 4Center for Global Sustainability, School of Public Policy, University of Maryland, College Park, MD, United States
Thailand has established a target of carbon neutrality by 2050. Reaching this goal will require coordination and collaboration between stakeholders spanning sectors and scales, including energy system decision makers, land managers, and city planners. Robust decarbonization scenarios incorporating current plans and targets, additional measures needed, and trade-offs between strategies can help stakeholders make informed decisions in the face of uncertainty. Through iterative engagement with decision makers at the city and national levels, we develop and analyze carbon neutral scenarios for Thailand that incorporate Bangkok’s role using a global integrated assessment model. We find that Thailand can reach carbon neutrality through power sector decarbonization, energy efficiency improvements, widespread electrification, and advanced technologies including carbon capture and storage and hydrogen. Negative emissions technologies will also be needed to offset Thailand and Bangkok’s hardest-to-abate CO2 emissions. Bangkok, as a major population and economic center, contributes significantly to Thailand’s energy demand and emissions and can therefore play an important role in climate change mitigation. Accordingly, our results underscore the importance of subnational climate action in meeting Thailand’s carbon neutral goal. Our analysis also indicates that without sustained land-based carbon sequestration, much more mitigation effort will be needed in Thailand’s energy sector, including at the subnational scale, to reach carbon neutrality. These insights can help stakeholders identify priorities, consider tradeoffs, and make decisions that will impact Bangkok and Thailand’s long-term climate change mitigation potential. This analysis demonstrates how stakeholder engagement in integrated assessment modeling can facilitate and inform multilevel climate governance.
1 Introduction
As countries continue to make and revise their commitments to reducing greenhouse gas emissions, there is a growing need for holistic, multi-sector modeling to design and compare possible integrated decarbonization pathways. A common tool to do so is integrated assessment models (IAMs), which have been employed in numerous global and regional decarbonization assessments including many countries’ long-term greenhouse gas emissions reduction strategies (KLHK, 2021; ONEP, 2022; U.S. Department of State, 2021). However, when employed without stakeholder participation, IAMs can have limited utility to decision making and may lack credibility from stakeholders’ perspectives (O’Neill et al., 2020). Involving stakeholders in the IAM scenario design process can build trust between decision makers and researchers and helps to ensure that the modeled scenarios and outcomes are relevant to stakeholders’ planning needs. With the COVID-19 pandemic limiting opportunities for in-person interactions, flexible and creative processes are needed to maintain productive stakeholder engagement (Jäger et al., 2023). Through a combination of virtual and in-person events, we engaged numerous stakeholders across sectors and scales to design and analyze robust decarbonization pathways for Thailand and Bangkok.
Thailand has adopted numerous climate change mitigation targets and policy roadmaps developed by stakeholders across sectors and scales. At the 2021 Conference of Parties to the United Nations Framework Convention on Climate Change (COP26), Thailand’s prime minister announced the country’s goal to reach carbon neutrality by 2050 and net-zero GHG emissions by 2065. Thailand has since released their updated Long-term Low Greenhouse Gas Emission Development Strategy (LT-LEDS) (ONEP, 2022), which lays out pathways to reach these goals, as well as their second updated Nationally Determined Contribution to the Paris Agreement. The country has also adopted a suite of medium-term policy documents setting plans and targets for the energy system; these include the Power Development Plan (PDP) 2018–2037 (EPPO, 2020), the Alternative Energy Development Plan (AEDP) 2018–2037 (DEDE, 2018b), the Energy Efficiency Plan (EEP) 2018–2037 (DEDE, 2018a), the Thailand Smart Grid Development Master Plan 2015–2036 (MoE, 2015), and the Sustainable Transport Master Plan 2013–2030 (OTP, 2013).
Subnational climate action is essential to achieving national climate change mitigation goals, particularly in cities, as urban areas are responsible for up to 72% of GHG emissions globally (Lwasa et al., 2022). In Southeast Asia’s megacities, including Bangkok, rapid urbanization has occurred since the 1990s (Murakami et al., 2005), resulting in increased fossil fuel demand (Ho et al., 2021) and posing challenges for managing greenhouse gas emissions. The Bangkok Metropolitan Authority (BMA) has recognized these challenges and adopted a Master Plan on Climate Change covering 2013 to 2023 (BMA, 2013). The city has plans to develop an updated, longer-term roadmap which will serve as a foundation for their vision of net-zero CO2 emissions by 2050 in line with the national goal (BMA, 2023). Achieving city-level decarbonization targets can be challenging given that cities may not always have the jurisdictional authority to establish and enforce energy system policies (Jaccard et al., 2019). Therefore, vertical integration, or coordination and collaboration between city- and national-level climate action plans, could help to strengthen both Bangkok and Thailand’s decarbonization strategies.
Several studies have used the Asia-Pacific Integrated Assessment Model (AIM) to analyze carbon neutral pathways for Thailand (Limmeechokchai et al., 2023; Pradhan et al., 2022) and to assess impacts of carbon pricing on Thailand’s greenhouse gas emissions (Chaichaloempreecha et al., 2022). There have been some assessments of decarbonization potential in Bangkok (Ali et al., 2017), but none to our knowledge that integrate both city- and national-level measures into combined decarbonization pathways. We address this gap by modeling Bangkok’s options for CO2 emissions mitigation and the city’s role within Thailand’s carbon neutral trajectory. This approach, particularly when informed by stakeholder engagement, can provide valuable insights to both city- and national-level stakeholders. For example, renewable energy penetration in Bangkok’s electricity grids depends in part on Thailand’s national PDP; understanding both city- and national-scale electricity dynamics in a single carbon neutral scenario could help ensure that the PDP is consistent with both Bangkok and Thailand’s decarbonization goals. Integrating city scale dynamics in carbon neutral modeling could also help both city planners and regional to national natural resource managers understand the level of carbon sequestration that may be needed to offset Bangkok’s residual emissions in a decarbonized future.
Thailand’s LT-LEDS includes a target of 120 Mt CO2 sequestered by the land use, land use change, and forestry (LULUCF) sector by 2050. The LT-LEDS describes several specific goals and initiatives related to LULUCF sequestration, including forest protection, economic forest incentives, and urban green space projects. However, the LT-LEDS does not quantify the abatement potential for each strategy, which leaves room for major uncertainty; additionally, Thailand faces several barriers to these goals including population growth, illegal logging, and volatile prices of agricultural products (DNP, 2021). One study using the Agriculture, Forestry, and Other Land Use Bottom-up (AFOLUB) model found that LULUCF sequestration in 2050 could reach a maximum of only 67.7 Mt CO2, just over half of the LTS target, with a carbon price of at least $10/t CO2eq (Pradhan et al., 2019). Therefore, when modeling carbon neutral scenarios it is important to consider uncertainty in sequestration potential, including the possibility of failing to meet the LT-LEDS target, rather than assuming a single fixed LULUCF sequestration amount based on the target (Pongthanaisawan et al., 2023). One study compared Thailand’s GHG emissions under carbon price scenarios with and without an assumed carbon land sink (Chaichaloempreecha et al., 2022); however, these scenarios used a fixed range of carbon prices and therefore did not highlight the differences in effort needed to reach carbon neutrality. We address this gap by comparing modeled pathways to carbon neutrality with and without the achievement of Thailand’s ambitious LULUCF sequestration target. This approach highlights potential trade-offs between climate change mitigation efforts in the energy and land systems and provides stakeholders with information needed to address the key uncertainty of Thailand’s LULUCF sequestration potential.
In the methods section, we explain our process of stakeholder engagement, introduce our modeling framework including the subnational modeling capabilities we have developed for this analysis, and describe the scenarios that we developed in collaboration with stakeholders. The results section provides an overview of our findings including decarbonization efforts needed to reach Thailand’s national carbon neutrality goal, CO2 emissions mitigation potential in Bangkok and the city’s contribution to the national pathway, and implications of uncertainty in Thailand’s LULUCF sequestration potential. We then discuss the implications of our findings and provide corresponding policy recommendations considering stakeholders’ needs and interests.
2 Methods
2.1 Stakeholder engagement process
Our scenario development, modeling, and analysis processes were informed by iterative engagement with stakeholders at both the national and city levels (Table 1) including a combination of virtual and in-person events. We conducted a series of three virtual stakeholder workshops between September 2022 and June 2023. During the first workshop, we introduced stakeholders to our modeling framework; stakeholders identified their policy priorities and planning needs and provided feedback on scenario design. The second and third workshops each included two separate sessions with city and national stakeholders, facilitating detailed discussions of scenario components and initial model results. Stakeholders expressed their feedback and concerns and identified areas for additional scenario development to better meet their planning needs. We also conducted lengthier in-person meetings with each individual stakeholder organization in January 2023 (following the first workshop) and August 2023 (following the third workshop). These meetings strengthened our connections with the stakeholders and allowed for additional detailed discussion of scenario development and model results. See Supplementary Table S1 for a more details on the main outcomes and example discussion topics from each meeting and Section 2.4.1 for more information on how stakeholders informed scenario development.
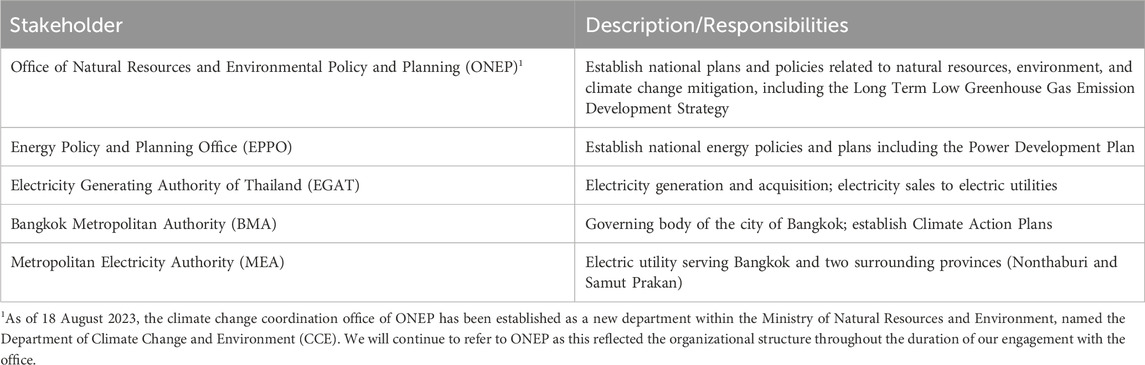
TABLE 1. Names and descriptions of the stakeholders engaged in this research, including some of their powers and responsibilities related to the energy system and climate change mitigation.
In addition to the above stakeholder engagement activities, our research process also involved two-way exchanges of knowledge and skills between the U.S.-based and Thailand-based coauthors. The Thailand-based coauthors established and strengthened the team’s connections with the stakeholders; they also provided local knowledge, data, and contextual information to validate the input data and assumptions to the Global Change Analysis Model (GCAM) and to critique intermediate model results. The U.S.-based coauthors developed and ran GCAM for the analysis; they also hosted several training sessions to help the Thailand-based coauthors build their capacity to run the model. Both groups provided and discussed iterative feedback on the scenario design and model results throughout the research process.
2.2 Global change analysis model (GCAM)
We conducted our analysis using GCAM-SEA, a version of the Global Change Analysis Model (GCAM) with added detail in the Southeast Asia region (Khan et al., 2023). GCAM is a global, multi-sector model that integrates dynamics within and between the socioeconomic, energy, land, water, and climate systems (Calvin et al., 2019). GCAM’s energy system encompasses primary energy resource production, energy transformation sectors including electricity generation, and energy demand for end-uses in the buildings, industry, and transportation sectors. Emissions of 24 greenhouse gases are represented, but for this analysis we focused on CO2. GCAM is calibrated using 2015 data and makes projections in 5-year time steps through 2100; for this analysis, we ran the model up to 2050 since this is Thailand’s target year for carbon neutrality (ONEP, 2022). The model solves for a market equilibrium solution in each period, balancing supplies and demands of dozens of global and regional markets for energy commodities and services, land, and water.
GCAM represents 32 global energy-economy regions that can trade with one another (including an aggregate Southeast Asia region), 235 river basins, and 384 land regions. For this analysis, we added additional spatial resolution to GCAM-SEA by disaggregating Thailand into an independent energy-economy region separate from the rest of Southeast Asia. Like other energy-economy regions, Thailand’s energy system was calibrated using data from the International Energy Agency, the Community Emissions Data System, and several other sources (see https://jgcri.github.io/gcam-doc/for more details).
2.3 Subnational modeling approach
To integrate both city- and national-level dynamics in our scenarios, we further disaggregated Thailand into four subregions in GCAM-SEA: Bangkok, Nonthaburi, Samut Prakan, and the rest of Thailand. Bangkok, Nonthaburi, and Samut Prakan are the provinces served by the MEA, but we focus primarily on Bangkok for the city-level analysis presented here. Figure 1 shows the components of the energy sector that were disaggregated to the subnational level and those that were modeled only at the national level. Primary energy supply and energy transformation processes, including electricity generation, refining, and hydrogen production, were modeled nationally. Of the energy consuming end use sectors, only GCAM’s detailed industry sectors were modeled at the national level, while ‘other industry’ and all buildings and transportation services were modeled sub-nationally. We also added detailed representations of building energy consumption in the subregions, disaggregating GCAM’s buildings sector into detailed services such as cooking, lighting, air conditioning, ventilation, and others. Dynamics outside of the energy system, including land use, land use change, agriculture, and water consumption, were modeled at the national level.
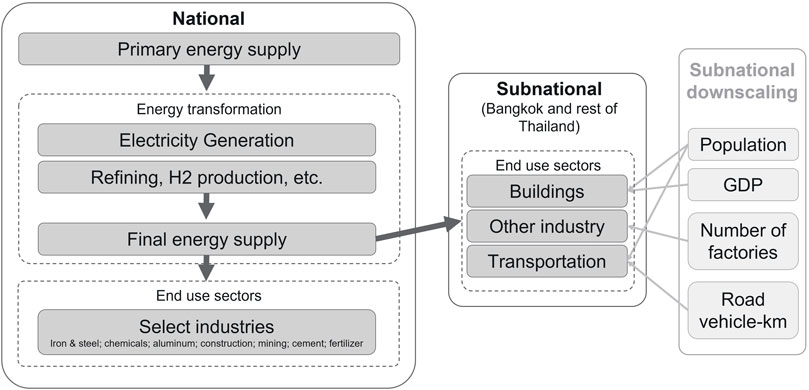
FIGURE 1. Components of the GCAM energy system that are modeled at the national level (Thailand) and the subnational level (Bangkok and the rest of Thailand). The proxies used to downscale national historical data for calibrating energy demands and GHG emissions are shown on the right.
We used various proxies to downscale model calibration data from the national to subnational levels, as these data were not available sub-nationally (Figure 1). In the buildings sector, the population of each subnational region was used to estimate historical floorspace, while GDP was used to downscale historical energy service demand. In the transportation sector, we disaggregated historical demand for road transport, including buses, motorcycles, light duty vehicles, and freight trucks, using provincial road vehicle-km data (Pansiri and Sriisaree, 2018); we downscaled demand for other transportation modes based on population. The number of registered factories in each province was used as a proxy to downscale calibration data for the industrial sector since industrial energy consumption data were not available at the subnational scale. See Supplementary Table S2 for more details on energy system calibration and proxies used for downscaling.
2.4 Scenario design
We collaborated with stakeholders to develop four scenarios that assess the impacts of Thailand’s current policies and goals, explore additional measures needed to reach carbon neutrality, and address uncertainty regarding the carbon sequestration potential of Thailand’s LULUCF sector (Table 2). The Business as Usual (BAU) scenario is GCAM’s reference scenario, which is calibrated to historical data and uses baseline assumptions about socioeconomic growth and technology development (Calvin et al., 2019) but does not impose additional constraints on technology adoption, emissions, or other factors considered in the subsequent scenarios. The Policies (POL) scenario incorporates policies in the power, buildings, industry, and transportation sectors based on Thailand’s current plans and targets (Supplementary Table S3). The Carbon Neutral 2050 (CN2050) scenario explores additional measures needed to reach Thailand’s 2050 carbon neutrality goal by imposing a constraint on CO2 emissions from fossil fuels and industry (FFI) in addition to the policies in POL. Lastly, the Carbon Neutral 2050 + LUC (CN2050+LUC) scenario is a modified version of CN2050 that incorporates Thailand’s target of 120 Mt CO2 sequestered by the LULUCF sector in 2050. Other scenarios do not take into account positive or negative LULUCF emissions.

TABLE 2. Description of the policies, emissions constraints, and LUC emission assumptions included in the five scenarios.
The policies included in all scenarios except BAU are aligned with Thailand’s current plans and targets; they also reflect stakeholders’ feedback on Thailand and Bangkok’s priorities and realistic levels of ambition (Supplementary Table S3). Power sector policies reflect the renewable energy expansion and coal phaseout goals in Thailand’s LT-LEDS. Building sector policies are aligned with Thailand’s Building Energy Code and LT-LEDS, targeting energy efficiency by improving average building envelope efficiency, phasing out inefficient lighting technologies, and increasing the use of high-efficiency air conditioners. In the industry sector, one policy increases overall energy efficiency to reflect the emphasis on industry in Thailand’s Energy Efficiency Plan; another policy incentivizes increasing use of hydrogen fuels as targeted by the LT-LEDS. In the transportation sector, policies target electrification by decreasing EV costs and eventually phasing out new internal combustion engine (ICE) vehicles; these measures are consistent with Thailand’s plan to scale up EV production through financial incentives (30@30 EV policy) and to phase down ICEs (LTS-LEDS).
The CO2 emissions constraints in CN2050 and CN2050+LUC linearly decrease from Thailand’s 2020 emissions level to net-zero CO2 emissions in 2050 in line with Thailand’s carbon neutrality target. Constraints are applied to the whole country, i.e., emissions in Bangkok and the rest of Thailand combined must reach the target emissions in each model period. GCAM identifies the carbon price needed to reach each model period’s constraint and applies this price across the economy. In effect, this carbon price method allows GCAM to identify the most cost-effective way to accomplish the target CO2 emissions mitigation. In CN2050+LUC, we exogenously set an assumed LULUCF carbon sink of 120 Mt CO2 in 2050 which aligns with the target in Thailand’s LT-LEDS.
2.4.1 Stakeholder input to scenario design
Stakeholder input played an important role in our scenario design process. The final policies chosen for inclusion in the Policies scenario reflect Thailand’s highest priority planned climate change mitigation efforts as identified by stakeholders during the workshops and meetings. As well as identifying and confirming the included policies, stakeholders also helped to inform their details, particularly in cases where official policy documents did not specify quantitative targets or prescribe timing. For example, stakeholders expressed that our initial ICE vehicle phase-out policy, which phased out new ICE vehicles by 2050, was not ambitious enough given that the Thai government is pushing to propose banning new ICEs in an earlier timeframe; in response, we modified the policy to assume an earlier phase-out. On the other hand, stakeholders expressed concerns that our original non-LED lighting phase-out policy in the buildings sector was too rapid and did not account for the challenges of enforcing building energy codes; we therefore modified this policy to represent a more delayed phase-out.
Additionally, Thai environmental stakeholders expressed concern over the uncertainty of Thailand’s potential LULUCF sink, highlighting the need to explore tradeoffs between energy and land system mitigation strategies. In response to this concern, we chose to compare carbon neutral pathways with and without Thailand’s LULUCF sequestration target (the Carbon Neutral 2050 + LUC and Carbon Neutral 2050 scenarios, respectively).
2.5 Indirect emissions accounting
While we did not model electricity generation at the subnational level, and therefore did not consider GHG emissions from power plants within Bangkok, cities contribute indirectly to GHG emissions in the power sector through demand for grid-supplied electricity; these emissions may be generated within or outside of city boundaries. According to the Global Protocol for Community-Scale Greenhouse Gas Emission Inventories, these “indirect emissions” from electricity consumption make up a city’s Scope 2 emissions, which is one of the protocols’ three major emissions inventory categories (Fong et al., 2021). In the absence of data detailing the individual power plants supplying Bangkok’s electricity grid, we used a simple approach to estimate indirect emissions for Bangkok and the other subnational regions based on the regions’ electricity consumption combined with Thailand’s national power sector emissions intensity. We calculated indirect emissions from electricity consumption in each end use sector and subnational region for a given model period using the following equation:
Where
3 Results
3.1 CO2 emissions mitigation needed for carbon neutrality
Based on our analysis, in the Business as Usual scenario, Thailand’s national emissions rise from just under 300 Mt CO2 to about 350 Mt CO2 by 2050 (Figure 2). The Policies scenario results in 227 Mt residual CO2 emissions in 2050 and contributes only 36% of the emissions mitigation needed to reach carbon neutrality (Figure 3). Most CO2 emissions mitigated in the Policies scenario are indirect emissions from electricity consumption, which can be attributed to power sector decarbonization as well as increasing efficiency of electricity consumption by the end use sectors. Most additional mitigation needed to reach carbon neutrality comes from mitigating direct emissions from the buildings, industry, and transportation sectors, which can be achieved primarily through electrification (Figure 3).
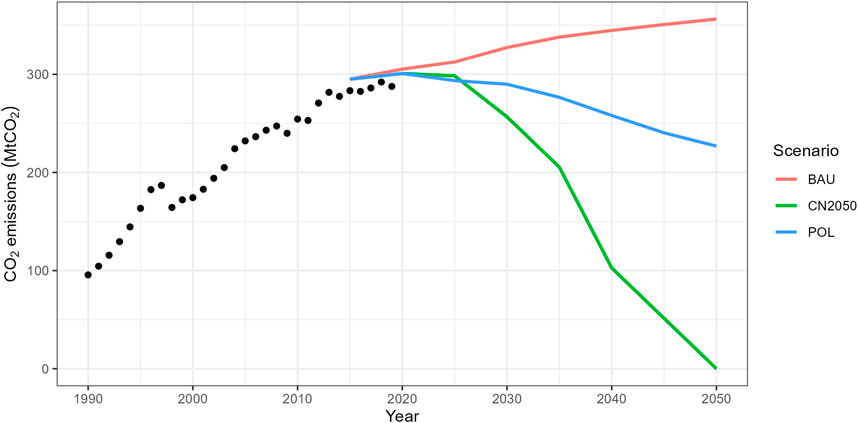
FIGURE 2. Historical national CO2 emissions (O’Rourke et al., 2021) and projected CO2 emissions in the Business as Usual (BAU), Policies (POL), and Carbon Neutral (CN2050) scenarios.
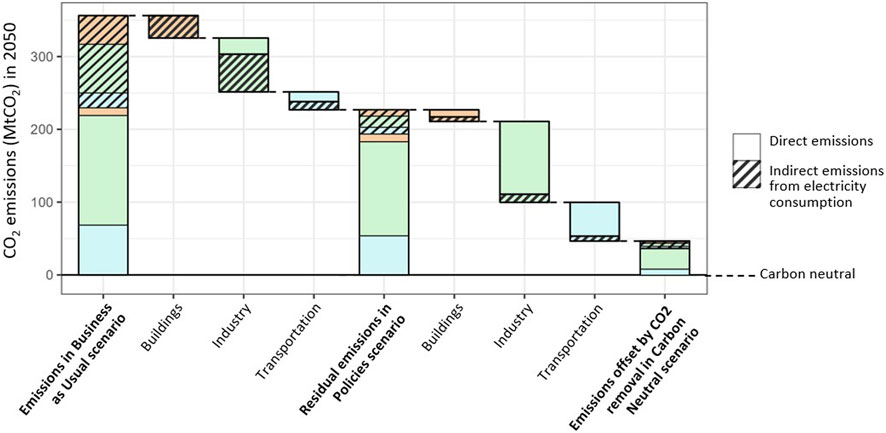
FIGURE 3. Summary of 2050 CO2 emissions in the BAU, POL, and CN2050 scenarios; direct and indirect emissions mitigation between scenarios are shown for each sector.
3.2 Power sector transformation
The power sector was responsible for 30% of Thailand’s CO2 emissions in 2015, making it the country’s greatest emitting sector. We find that the power sector also has the highest potential for mitigation, contributing 72% and 33% of total CO2 emissions mitigation by 2050 in the Policies and Carbon Neutral scenarios, respectively, compared to Business as Usual. Power sector decarbonization requires a rapid expansion of renewables, particularly solar PV. In the Policies scenario, wind and solar power reach 74% of total electricity generation by 2050 in line with the target in Thailand’s LT-LEDS (Figure 4). While the Policies scenario includes some electrification, total electricity demand decreases compared to the Business as Usual due to energy efficiency policies; this results in 394 TWh total electricity generation in 2050, down 14% from Business as Usual.
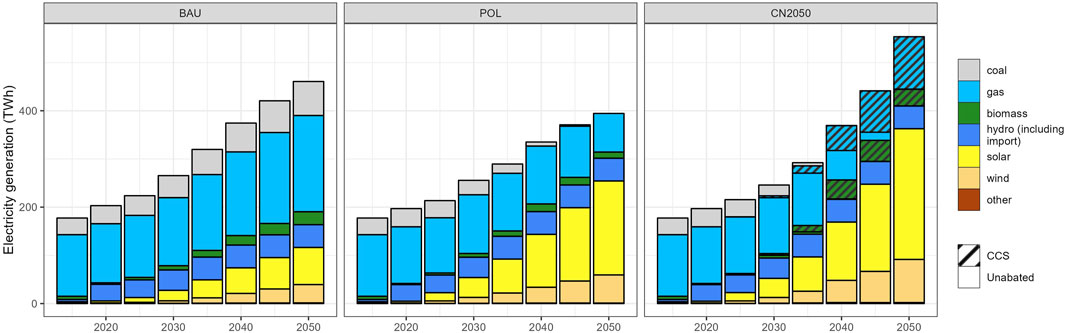
FIGURE 4. Thailand’s total electricity generation (TWh) by fuel over time in the Business as Usual (BAU), Policies (POL), and Carbon Neutral (CN 2050) scenarios.
Electricity demand and generation mix in the Carbon Neutral scenario stay relatively consistent with the Policies scenario until 2035, when electricity consumption begins to increase due to fuel switching and CCS technologies begin to play a role. By 2050, the share of renewables in the Carbon Neutral scenario remains similar to the Policies scenario, but substantially higher electricity demand due to electrification necessitates greater solar PV and wind capacity, whose combined electricity generation reaches 360 TWh. More natural gas capacity is maintained in the Carbon Neutral scenario compared to the Policies scenario due to the greater need for backup capacity to accommodate intermittent renewables; however, by 2050, all natural gas generation in the Carbon Neutral is paired with CCS. Additionally, BECCS comprises 6% of electricity generation by 2050 in the Carbon Neutral scenario (Figure 4).
3.3 End-use sector electrification and energy efficiency
CO2 emissions mitigation in the Policies and Carbon Neutral scenarios also requires electrification and increased energy efficiency in the buildings, industry, and transportation sectors. Total final energy consumption grows in the Business as Usual scenario, increasing by 29% in 2050 compared to 2015. This growth is dominated by electricity, which reaches a 35% share of total energy consumption by 2050. Energy consumption grows more slowly in the Policies scenario; however, the fuel mix remains similar to that of the Business as Usual scenario. In the Carbon Neutral scenario, final energy consumption peaks in 2025 then declines to 10% below the 2015 level by 2050. There is a major fuel mix shift in the Carbon Neutral scenario, with electricity displacing fossil fuels and biomass to reach a 61% share of total final energy by 2050 (Figure 5).
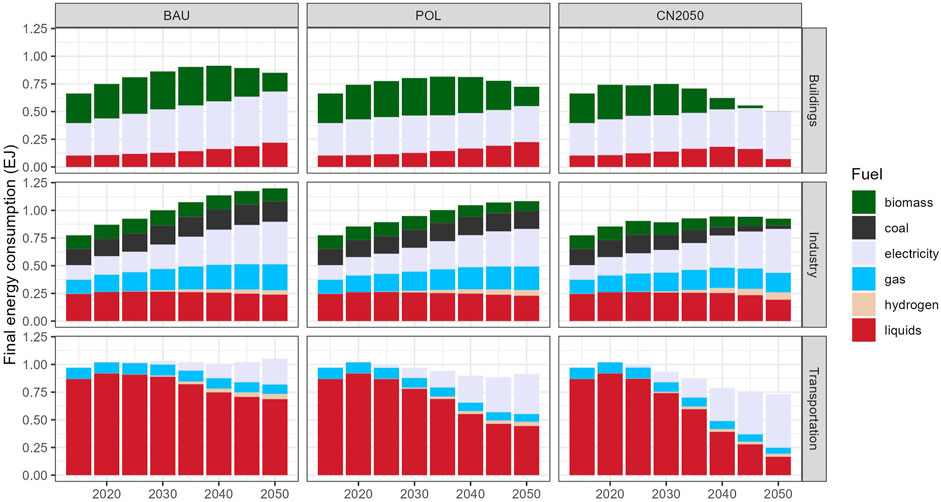
FIGURE 5. Thailand’s total final energy consumption (EJ) by sector in the Business as Usual (BAU), Policies (POL), and Carbon Neutral (CN 2050) scenarios. Industrial feedstocks are not included.
Measures to improve building energy efficiency in the Policies scenario reduce building electricity consumption by 30% in 2050 compared to Business as Usual. About half of these electricity savings are from increased efficiency of commercial air conditioning and mechanical ventilation (ACMV), while the remainder are split between residential ACMV and residential lighting. The main additional building sector measure required in the Carbon Neutral scenario is rapid electrification of cooking, with the share of electricity in cooking energy consumption rising from only 8% in 2015 to 78% in 2050, displacing biomass and LPG.
Similarly, the Policies scenario results in lower energy consumption in the industrial sector, while electrification is the main additional measure needed to reach carbon neutrality. Industrial energy consumption grows more slowly in the Policies scenario compared to Business as Usual, with 10% lower total energy consumption by 2050. The fuel mix remains similar to Business as Usual other than a slight increase in the share of hydrogen. In the Carbon Neutral scenario, energy efficiency improvements are paired with electrification, with electricity displacing fossil fuels and biomass to reach 43% of total industrial energy consumption by 2050.
Electrification is central to decarbonization in the transport sector across scenarios. There is some electrification in the Policies scenario, with electricity comprising 40% of total energy consumption, respectively, by 2050, compared to only 22% in the Business as Usual scenario. However, more aggressive electrification is needed to reach carbon neutrality, with electricity reaching 66% of energy consumption by 2050 in the Carbon Neutral scenario. This includes a more than threefold increase in EVs for personal passenger transport and a 67% increase in electric freight trucks compared to Business as Usual.
3.4 Advanced technologies: CCS and hydrogen
In the Carbon Neutral scenario, CCS is used to mitigate some emissions from industry, as well as in power generation and other energy transformation sectors. CCS starts to play a role in 2030; by 2050, 57 Mt CO2 is captured from fossil fuel combustion, and an additional 47 Mt CO2 is sequestered via CO2 removal technologies, such as bioenergy with carbon capture and storage (BECCS) or direct air capture (DAC), offsetting Thailand’s residual CO2 emissions to reach carbon neutrality (Figure 6A). Residual emissions mostly come from industry, transportation, and backup natural gas generation in the power sector (Figure 6B). Most of Thailand’s CCS occurs at natural gas and biomass power plants in the Carbon Neutral scenario, with hydrogen production plants, refineries, and industries also playing a role (Figure 7).
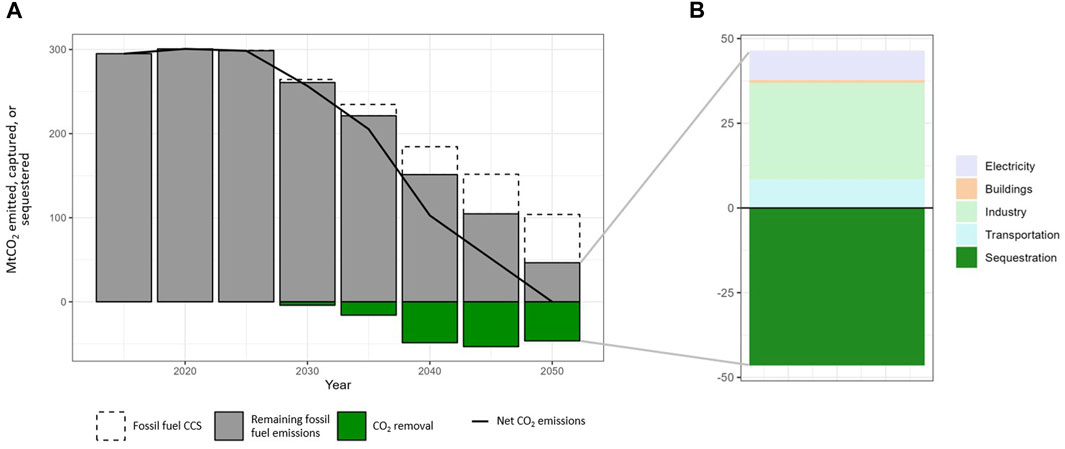
FIGURE 6. (A) Summary of the amount of CO2 emitted, captured via carbon capture and storage (CCS), and sequestered via CO2 removal technologies over time in the Carbon Neutral scenario; (B) residual CO2 emissions in 2050 that are offset by CO2 removal.
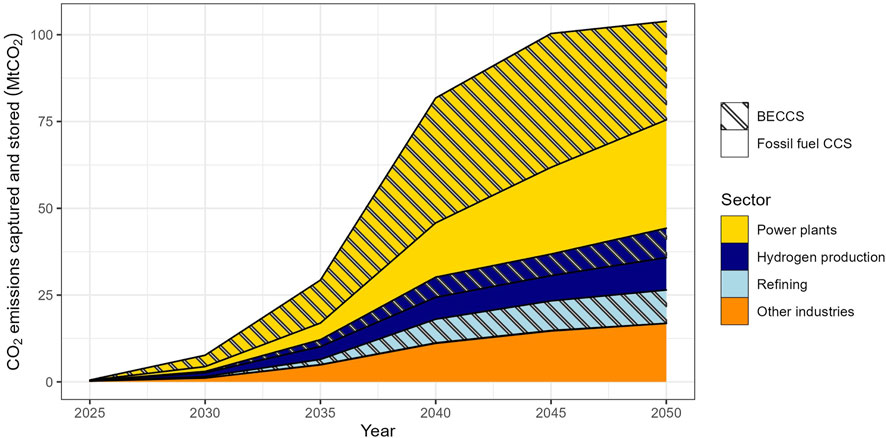
FIGURE 7. Amount of CO2 emissions abated via fossil fuel carbon capture and storage (CCS) and sequestered via bioenergy with CCS (BECCS) by sector in the Carbon Neutral Scenario.
Hydrogen is produced in all scenarios starting in 2025, but the Carbon Neutral scenario has the most growth of this fuel, with hydrogen comprising 4% of Thailand’s total final energy supply by 2050. While hydrogen produced using natural gas steam reforming without CCS dominates hydrogen supply in the Business as Usual and Policies scenarios, hydrogen in the Carbon Neutral scenario is produced from a mix of technologies including electrolysis using renewable energy, natural gas steam reforming with CCS, biomass gasification, and coal gasification (Figure 8).
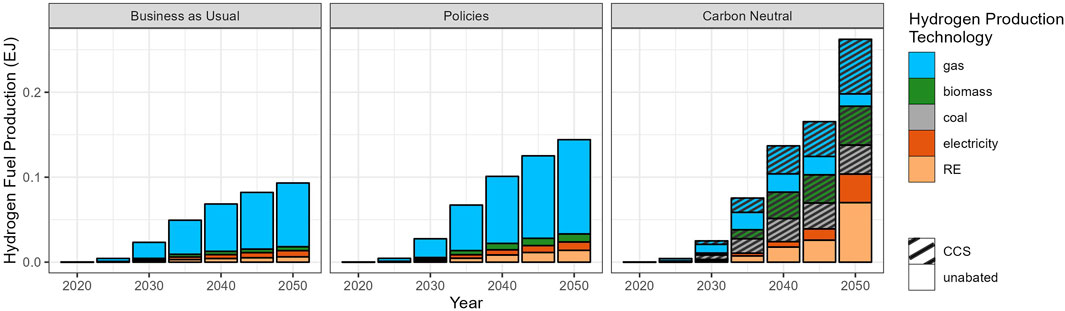
FIGURE 8. Hydrogen production by technology in the Business as Usual (BAU), Policies (POL), and Carbon Neutral (CN 2050) scenarios.
3.5 Bangkok’s role in carbon neutrality
As a major population and economic center of the country, Bangkok plays an important part in Thailand’s carbon neutrality pathway, particularly through the city’s significant electricity demand. Despite comprising less than 10% of Thailand’s population, Bangkok is responsible for almost a fifth of national electricity consumption. Bangkok contributed about 13 Mt of direct CO2 emissions in 2015 and an additional 17 Mt of indirect CO2 emissions from electricity consumption. In the Business as Usual scenario, Bangkok’s emissions increase moderately through 2030 and then decrease slightly below the 2015 level by 2050 (Figure 9). Emissions steadily decrease in the Policies and Carbon Neutral scenarios, reaching 13 and 3 Mt CO2, respectively, by 2050, or 43% and 11% of the 2015 level (Figure 9). In the Carbon Neutral scenario, Bangkok’s residual emissions in 2050, most of which come from the industrial sector, are offset by carbon sequestration modeled at the national level. Our analysis indicates that direct CO2 emissions mitigation in Bangkok contributes 3% of the total mitigation in the Carbon Neutral scenario by 2050 compared to Business as Usual, but this share increases to 7.1% when both direct emissions and indirect emissions from electricity consumption are considered (Supplementary Figure S1).
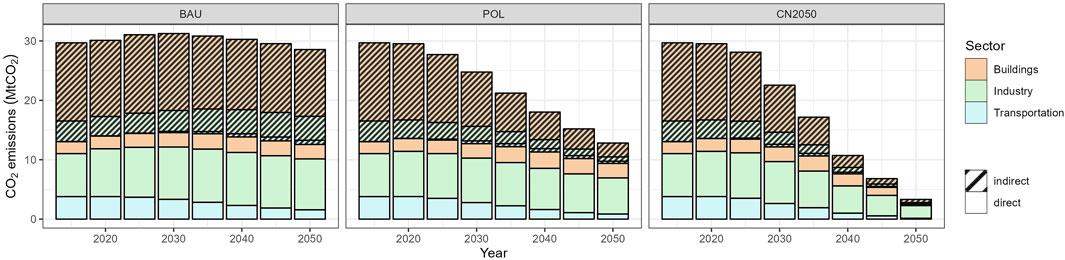
FIGURE 9. Direct CO2 emissions (solid color) and indirect CO2 emissions from electricity consumption (striped) over time in Bangkok in the Business as Usual (BAU), Policies (POL), and Carbon Neutral (CN2050) scenarios.
Bangkok’s buildings sector plays a particularly important role in the Carbon Neutral scenario, contributing 28% of total direct and indirect emissions mitigation needed in buildings nationally by 2050 (Supplementary Figure S1). Indirect emissions are mitigated through electricity savings in the Policies scenario (Figure 10A) as well as decreasing CO2 intensity of remaining electricity consumption due to power sector decarbonization in both the Policies and Carbon Neutral scenarios (Figure 10B). In the Carbon Neutral scenario, CO2 emissions from cooking, the main source of direct emissions in Bangkok’s buildings sector, are mitigated through the replacement of biomass and LPG stoves with electric ones (Figure 10C).
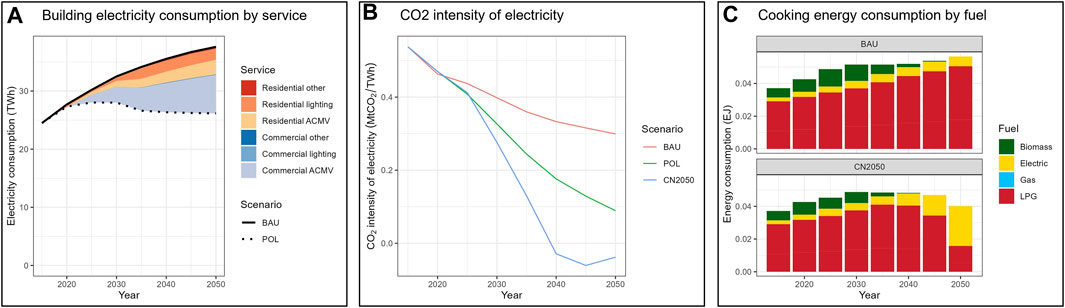
FIGURE 10. Components of CO2 emissions mitigation in Bangkok’s building sector including (A) electricity savings in the Policies (POL) scenario; (B) decreasing CO2 intensity of Thailand’s electricity supply; and (C) electrification of cooking.
Following the building sector, the industrial sector has the next highest potential for CO2 emissions reduction in Bangkok. As in the rest of the country, Bangkok can accomplish these reductions through a combination of improved energy efficiency in line with national goals and widespread electrification. We also find that, in the Carbon Neutral scenario, the industrial sector is responsible for the majority of Bangkok’s residual emissions in 2050 which will need to be offset by LULUCF sequestration or other carbon removal technologies (Figure 9). This suggests that industrial emissions may be the hardest to abate in Bangkok. Conversely, the city has the potential to almost entirely eliminate CO2 emissions from its transportation sector through electrification. While our analysis suggests that transportation may not play as major a role in Bangkok’s decarbonization pathway as the buildings and industry sectors, mitigating emissions from transportation will have significant co-benefits for the city such as air pollution reduction. Rapid growth in EVs will be needed, with EVs comprising nearly 90% of cars and motorcycles in Bangkok by 2050, and a similar transition for freight trucks.
Decarbonization will have substantial impacts on electricity demand in Bangkok and the surrounding region, although these impacts differ from those projected for the rest of the country. Due to Bangkok’s prominent buildings sector, the energy efficiency policies modeled result in a proportionally larger reduction of electricity demand in the Policies scenario compared to the country as a whole. While the Carbon Neutral scenario results in increased electricity demand in all regions, demand stays below the Business as Usual level by 2050 in Bangkok, while it increases substantially beyond the Business as Usual level in the rest of Thailand (Figure 11). This suggests that energy efficiency policies, if implemented effectively, could offset electricity demand growth from electrification in Bangkok. As this is not feasible on a national scale, it represents a unique opportunity in the urban context.
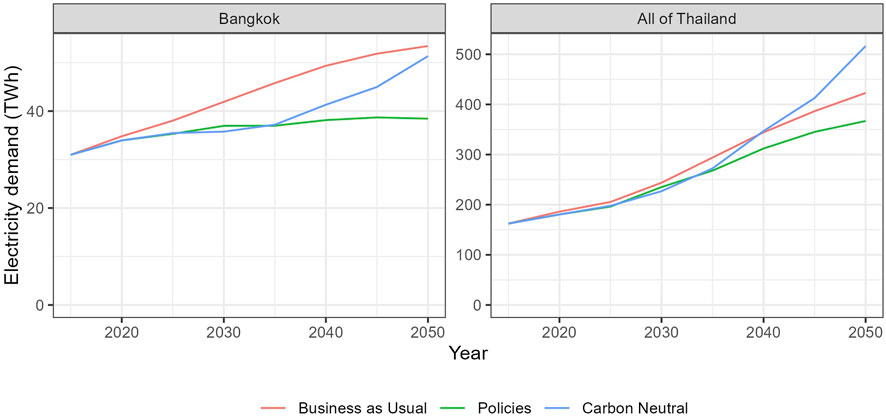
FIGURE 11. Electricity demand over time in Bangkok and all of Thailand under the Business as Usual (BAU), Policies (POL), and Carbon Neutral scenarios.
3.6 Implications of an ambitious LULUCF carbon sequestration target
Our analysis identifies tradeoffs between climate change mitigation efforts in the energy and LULUCF sectors. The level of effort needed in the energy system will be much higher if Thailand does not achieve their ambitious goal of 120 Mt CO2 LULUCF sequestration by 2050. In the Carbon Neutral scenario, which includes no LULUCF sequestration, there are 46 Mt of residual emissions in 2050 which are offset by CO2 removal. However, 2050 residual emissions are almost three times higher in the Carbon Neutral + LUC scenario, which assumes that Thailand will reach their 120 MtCO2 LULUCF sequestration goal (Figure 12). Most of these 137 Mt of residual CO2 emissions are offset by the LULUCF sequestration, with the remaining 17 MtCO2 offset by CO2 removal. LULUCF sequestration reduces the need for fossil fuel CCS, with 30% less CO2 captured from fossil fuel combustion in the Carbon Neutral + LUC scenario compared to the Carbon Neutral scenario (Figure 12). LULUCF sequestration uncertainty also has implications for Bangkok’s residual emissions, which are 2.5 times as high in the Carbon Neutral + LUC scenario as in the Carbon Neutral scenario (Figure 12).
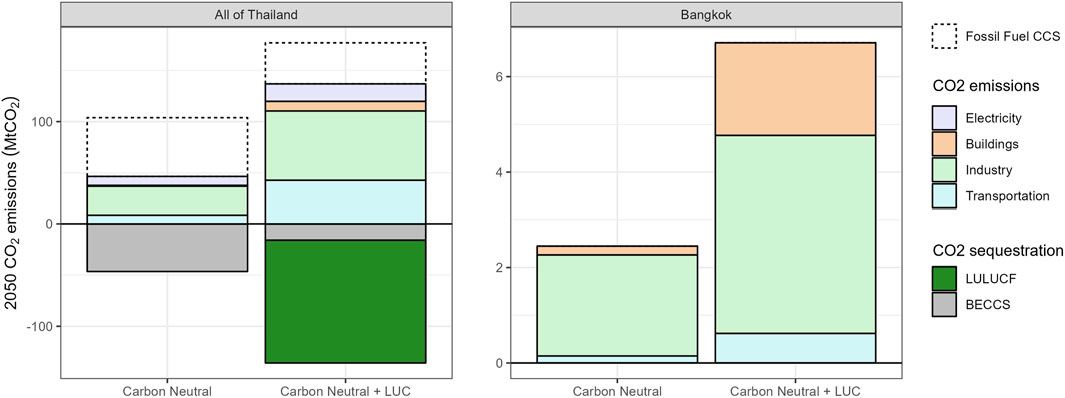
FIGURE 12. Summary of 2050 residual CO2 emissions, CCS, and sequestration in the Carbon Neutral (CN 2050) and Carbon Neutral + LUC (CN 2050 + LUC) scenarios, both in Thailand and Bangkok. Bangkok is assumed not to have the potential for significant LULUCF CO2 sequestration.
In the Carbon Neutral + LUC scenario, there is 20% less total electricity generation by 2050 compared to the Carbon Neutral scenario (Figure 13A) due to the reduced need for end use sector electrification (Figure 13B). This includes 17%, 12%, and 13% less electricity consumption in the buildings, industry, and transportation sectors by 2050, and corresponding higher levels of fossil fuel and biomass consumption. As well as less electricity generation, there is also less CCS deployment in the power sector in the Carbon Neutral + LUC scenario compared to the Carbon Neutral scenario; while all gas power plants are equipped with CCS by 2050 in the latter scenario, some electricity is still generated from gas without CCS in the former.
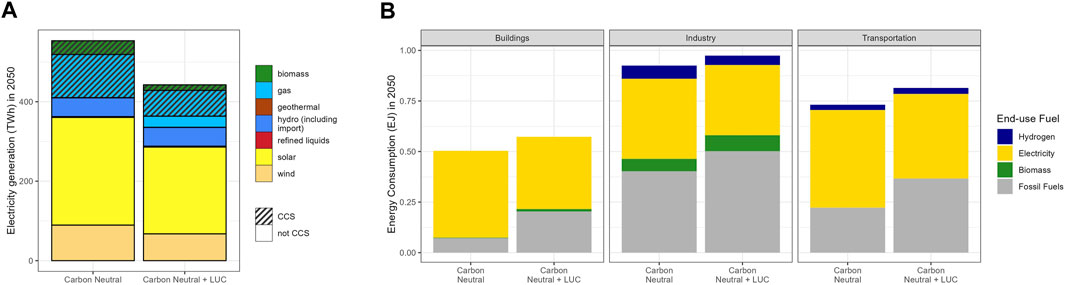
FIGURE 13. Comparison of (A) electricity generation by technology and (B) end-use sector energy consumption by fuel in 2050 in the Carbon Neutral (CN 2050) and Carbon Neutral + LUC (CN2050+LUC) scenarios.
Our analysis also suggests that Thailand’s targeted land sink, if achieved, could decrease the costs of CO2 emissions mitigation needed in the energy system to reach carbon neutrality. While estimating the abatement costs associated with the Policies scenario was not in the scope of this analysis, GCAM models CO2 emissions constraints by solving for an economy-wide1 CO2 price that achieves the target emissions reduction. This CO2 price is not intended to represent an explicit carbon tax policy, but it does provide a measure of relative abatement costs between scenarios. The CO2 prices are substantially higher in the Carbon Neutral scenario compared to the Carbon Neutral + LUC scenario (Supplementary Figure S2); this suggests that, with Thailand’s current policies in place, additional abatement needed in the energy system to reach carbon neutrality by 2050 may be less costly in the Carbon Neutral + LUC scenario.
4 Discussion and conclusions
4.1 Thailand’s decarbonization potential
We find that to reach carbon neutrality by 2050, Thailand will need to use robust mitigation measures across sectors. Thailand’s current sectoral plans and targets can contribute a substantial amount of CO2 emissions mitigation, but they will need to be supplemented with additional measures to reach net zero CO2 emissions. Our analysis highlights five major insights for Thailand’s carbon neutral pathway. The first is that end-use sector electrification, combined with robust decarbonization of the power sector, will be a key driver of CO2 emissions mitigation for the country. Second, demand-side measures such as energy efficiency and technology changes will play a major role. Third, Thailand will need to invest in advanced technologies including hydrogen, CCS, and CO2 removal technologies, to mitigate the hardest to abate emissions. Fourth, Bangkok can play an important part in Thailand’s decarbonization, particularly through its buildings sector. Finally, uncertainty regarding Thailand’s LULUCF carbon sequestration potential could substantially impact the extent of decarbonization measures needed in the energy system.
Thailand’s goal of 74% renewable electricity generation by 2050 (LT-LEDS) will account for most of the decarbonization needed in the power sector. However, to maintain this share of renewables in a carbon neutral pathway, wind and solar capacity will need to grow substantially to meet increasing electricity demand as the end-use sectors are electrified. Technical feasibility may be a limiting factor for wind and solar expansion; Thailand has about 91 TWh/year of wind potential (Lau, 2022), while solar PV potential estimates vary widely from 33 to 600 TWh/year (Siala and Stich, 2016; Lau, 2022). While maximum wind and solar generation in our carbon neutral scenario falls within these estimates at 89 and 271 TWh/year, respectively, the wide range of estimates for solar PV indicates that the technical potential for this technology may be uncertain.
Additionally, a high share of intermittent renewable energy in the power system will increase the need for energy storage and backup capacity. While battery energy storage systems (BESS) are likely to become more economically feasible in the future (IRENA, 2017), their costs could still be significant, particularly if storage batteries are larger than needed (Deguenon et al., 2023); thus, studies assessing the optimal BESS size for Thailand’s power grids will be crucial (Klansupar and Chaitusaney, 2020; Yimprapai and Chaitusaney, 2022). In the Carbon Neutral scenario, natural gas plants equipped with CCS provide low-emissions backup capacity for grids powered by wind and solar, but high costs could be a barrier to CCS deployment.
Our analysis also emphasizes the role of energy end-use sectors in Thailand’s carbon neutrality pathway; while existing measures such as the Building Energy Code, Energy Efficiency Plan, and 30@30 EV policy can contribute substantial emissions mitigation, additional effort will be needed, particularly to achieve widespread electrification across sectors. Our buildings sector results agree with others’ findings that energy efficiency measures in Thailand’s buildings can significantly reduce electricity demand (Chaichaloempreecha et al., 2017); efficient air conditioners can be particularly impactful while offering low marginal abatement costs (Promjiraprawat et al., 2014). We provide stakeholders with useful benchmarks for the extent of energy efficiency improvement and electrification needed in the industrial sector to be in line with Thailand’s carbon neutrality goal. However, additional research is needed to identify appropriate strategies for specific industries such as cement (Chunark et al., 2021) and steel (Sodsai and Rachdawong, 2012). In the transportation sector, our analysis shows that EVs will need to grow substantially in both passenger and freight applications. A widespread transition to electric vehicles could offer co-benefits such as decreasing air pollution and reducing Thailand’s crude oil imports; however, major efforts to promote investment in EVs, develop the necessary charging infrastructure, and increase market demand will be needed for Thailand to accomplish this transition (Preedakorn et al., 2023). Growing the market for EVs in freight transportation could be particularly challenging given that freight transportation business owners do not currently see EVs as a feasible investment (Sattayathamrongthian and Vanpetch, 2023).
While electrification, energy efficiency, and power sector decarbonization can significantly reduce Thailand’s CO2 emissions, Thailand will need to deploy advanced technologies including CCS and hydrogen, and carbon removal technologies will be needed to offset the hardest-to-abate emissions. The feasibility of CCS as a mitigation strategy depends in part on the technical potential of carbon storage in Thailand. Studies have estimated Thailand’s total potential storage and mapped CO2 sources and sinks, matching major emitters with proximate storage sites to minimize potential transportation costs (ADB, 2013; Zhang et al., 2022). While Thailand has existing plans to initiate hydrogen pilot projects (Praiwan, 2023), our analysis informs how these efforts can be scaled up in the future. For example, our results inform stakeholders about how demand for hydrogen will evolve in the long-term as well as the most cost effective mix of grey, blue, green, and other hydrogen technologies to support Thailand’s carbon neutrality.
Our analysis indicates that Bangkok can play an important role in Thailand’s national decarbonization pathway, contributing 7% of the country’s direct and indirect CO2 emissions mitigation, while making significant progress toward the city’s own climate action goals. Our subnational modeling approach reveals the sectors and actions through which Bangkok can make the most CO2 emissions mitigation progress. We demonstrate that Bangkok’s role as a major electricity demand center, particularly in the buildings sector, results in significant potential for emissions mitigation through energy efficiency and clean power transitions. In fact, energy efficiency policies in buildings and industry could almost entirely offset increased electricity demand from electrification in Bangkok, which could help the city maintain grid stability throughout its energy transition. However, stakeholders expressed concern over Bangkok’s ability to enforce building energy codes at the city level; additionally, the extent to which Bangkok’s electricity grids can adopt renewable energy and CCS may depend on decisions made at the national level by organizations that inform Thailand’s Power Development Plan. While our analysis suggests that Bangkok has the potential to electrify most of its transportation sector, this will require rapid and extensive scaling up of EV charging infrastructure and could pose additional challenges to grid stability and peak electricity load management.
Stakeholders identified Thailand’s potential for LULUCF carbon sequestration to be a key uncertainty in climate change mitigation planning. Our analysis of two alternative carbon neutral pathways which assume that Thailand either does or does not achieve their ambitious sequestration target highlights significant tradeoffs between climate change mitigation efforts in the LULUCF sector and the energy system. For example, greater electrification and CCS deployment could serve as an alternative to relying on LULUCF sequestration; this agrees with findings from Latin America that identified electrification as a decarbonization strategy that limits the risk of exceeding sequestration potentials (Matamala et al., 2023). However, greater electrification could raise costs associated with electricity generation and storage and could increase the risk of exceeding Thailand’s technical renewable energy potential. Additionally, greater CCS deployment could be associated with significant costs; nature-based solutions, which include increasing LULUCF sequestration potential, could be a more cost-effective option with significant co-benefits (Griscom et al., 2017).
4.2 Policy recommendations and next steps
Iterative stakeholder engagement throughout the research process enabled us to validate our data and assumptions, to accurately represent Thailand’s current priorities and level of ambition across sectors, and to identify new priorities and benchmarks for climate change mitigation policy. To maximize the utility of this analysis for stakeholders, we developed a set of policy recommendations informed by the key insights outlined above combined with stakeholder input regarding key concerns, uncertainties, and priorities.
Power system digitalization and modernization will be crucial to support high shares of intermittent renewable energy sources in Thailand’s power grid, and to minimize costs associated with backup capacity and storage. Examples include load forecasting and monitoring, demand response to manage peak load, building energy management systems, and smart EV charging stations (Junlakarn et al., 2017; Charoen et al., 2022; Chanraksa and Singh, 2023). These measures could be particularly effective in the Bangkok metropolitan area as it is a major electricity demand center. Throughout the country, grid digitalization could play an important role in minimizing the growth of electricity prices during the energy transition, which was a major concern expressed during stakeholder meetings. Accordingly, Thai stakeholders have already developed a national Smart Grid Master plan and the Metropolitan Electricity Authority has established specialized Smart Grid plans for the Bangkok Metropolitan area (Phayomhom et al., 2015; Bangkok Post, 2020). While GCAM’s spatial and temporal resolution does not allow for assessing fine-scale grid dynamics or grid stability, our findings underscore the importance of these plans and justify the need to rapidly scale up Smart Grid pilot projects within and beyond Bangkok. Additionally, uncertainty in estimates of Thailand’s total solar PV potential underscores the importance of employing diverse solar technologies such as floating solar and rooftop PV, both of which were identified as priorities in our meetings with stakeholders in the power sector.
Strong policy support will also be needed to accomplish the extensive demand-side measures identified in our analysis, such as widespread EV adoption and significant energy efficiency improvement across sectors. Policies will need to minimize the costs of these transitions and provide incentives and finance opportunities such as tax credits and green finance schemes. Regulatory measures, such as building energy code and energy efficiency resource standard enforcement, may also need to be strengthened. While policy implementation at the city level may be particularly challenging, Bangkok could incentivize compliance with energy codes using strategies such as expedited permitting; the city could also consider implementing road pricing to incentivize EV adoption while investing in critical EV infrastructure, which the city has already identified as a priority.
While Thai stakeholders have recognized the need to invest in advanced technologies such as CCS and hydrogen, robust policies will be needed to scale up these efforts. Policies supporting CCS development could include establishing legal and regulatory frameworks in parallel with executing initial pilot projects, as well as securing international finance opportunities (IEA, 2021). Given that Thailand has existing plans for a hydrogen production pilot project, an impactful next step could be to develop a comprehensive national hydrogen strategy, such as that of Japan (TCMREH, 2017), to establish hydrogen markets and scale up production infrastructure.
Vertical integration could help enable Bangkok to realize their key role in Thailand’s decarbonization. Vertical integration encompasses strategies to align coordinate climate goals and policies across subnational and national governments (C40, 2020). This concept has been used to highlight integration between city and national climate strategies as an important step supporting city-level decarbonization success and enabling the scaling up of local climate action to achieve national to global climate change mitigation targets (Fuhr et al., 2018; Gordon, 2018). The Bangkok and Thai governments could practice vertical integration by collaborating on ways for Bangkok to enforce or incentivize compliance with policies set at the national level such as the building energy code. They could also mutually benefit from aligning their plans for renewable energy expansion and incorporating Bangkok’s opportunities for distributed energy resources in national power sector planning.
We also find that, in a national carbon neutral scenario, Bangkok will have residual emissions that will need to be offset by LULUCF sequestration or other carbon removal strategies. This suggests that these emissions, which occur mainly in the industrial sector, may be costlier to abate than to offset. Thus, while Bangkok has a vision of city-wide carbon neutrality by 2050, they may need to consider either deploying carbon removal technologies within the city or participating in a carbon market wherein carbon sequestered in other regions of the country is used to offset urban emissions.
Given that our analysis highlights significant tradeoffs between LULUCF and energy system measures, Thailand could increase the robustness of their climate change mitigation plans by further examining the country’s potential for land-based CO2 sequestration to reduce the uncertainty associated with current estimates. While we find that Thailand’s ambitious LULUCF sequestration target could substantially decrease required mitigation effort and associated costs in the energy system, our analysis does not account for the additional costs of expanding and maintaining the land sink. Economic and policy analysis will likely be needed to determine these costs and to evaluate tradeoffs with investment in energy system decarbonization measures. These tradeoffs also further underscore the importance of vertical integration, as the availability of CO2 emission offsets at the national level may impact Bangkok’s level of decarbonization effort in the energy system if the city decides to offset some of their emissions. Collaboration between city- and national-level stakeholders will also be needed to determine the extent to which Bangkok could contribute to LULUCF sequestration through urban green spaces.
Following the insights and policy recommendations from this analysis, stakeholders identified next steps and future research interests based on our existing modeling framework. Thai stakeholders are interested in expanding our analysis to additional cities and provinces to help inform detailed subnational climate change mitigation strategies. They are also interested in using our analysis to derive metrics of decarbonization co-benefits such as health and employment; air pollution reduction, for example, could be a particularly important co-benefit for Bangkok. Stakeholders at both the city and national level are also concerned about the potential costs associated with decarbonization, including investment costs and consumer impacts such as increasing electricity prices. Future work could make use of recent developments of GCAM’s macroeconomic representation, as well as additional analysis tools, to estimate transition costs for Thailand and Bangkok.
4.3 Limitations
Due to limited availability of subnational energy and emissions data, we used proxy data to calibrate historical energy and emissions for the subnational regions in GCAM-SEA; these proxies included population and GDP in the buildings sector, highway vehicle kilometer data in the transportation sector, and factory count data in the industry sector. These proxies may not fully capture the subnational distribution of historical energy demand and emissions. For example, we could be underestimating emissions from road transportation in Bangkok; downscaling emissions calibration data based on vehicle kilometer data may not account for the impact of congestion on vehicle emissions factors in urban settings (Li et al., 2023). However, the model dynamics and outputs resulting from our calibration method were validated qualitatively by stakeholders familiar with the national and subnational energy systems. It is also important to note that, while our analysis takes into account Bangkok’s scope 2 emissions (indirect emissions from electricity consumption) we do not consider scope 3 emissions (Fong et al., 2021). For example, our use of highway vehicle kilometer data to calibrate the subnational transportation sector only assigns transportation occurring within Bangkok’s boundaries to the city’s demand; freight vehicles on highways elsewhere in the country that are delivering goods to Bangkok are therefore not included in Bangkok’s freight demand.
Another limitation of our analysis is that we were unable to represent all of Thailand’s current emissions mitigation policies in the Policies scenario due to the qualitative nature of many policies. However, our iterative scenario design process allowed stakeholders to provide feedback on both our choice of policies to include and our methods of quantifying the measures presented qualitatively in policy documents. The final set of policies included in the Policies scenario therefore accurately represent Thailand and Bangkok’s main priorities and levels of ambition across sectors and measures.
4.4 Conclusion
Through iterative stakeholder engagement during scenario design and results analysis, we produced decision-relevant scenarios for carbon neutrality in Thailand including Bangkok’s contributions. Outcomes underscored the importance of existing policies such as MEA’s smart grid initiatives and EPPO’s hydrogen pilot projects. We also provided additional information and policy benchmarks such as projections of Bangkok and Thailand’s future renewable energy demand considering end-use electrification, analysis of the extent of energy efficiency and fuel switching needed in the end-use sectors, projected future demand for hydrogen, and the amount of CCS deployment and CO2 removal needed to reach carbon neutrality. These insights can inform Bangkok and Thailand’s continued decarbonization plans including updated national power development plans, energy efficiency plans, and building energy codes as well as Bangkok’s future climate action plans.
This analysis demonstrates how iterative stakeholder engagement, combined with international research partnerships, can produce integrated analysis relevant to policy and planning needs. We maximized the decision relevance of this analysis by working with stakeholders to identify key uncertainties in their planning processes such as LULUCF carbon sequestration potential and priorities for policy and investment. Stakeholder engagement and our U.S.- Thailand research partnership also helped us to overcome the limitations of data availability, particularly for city-level modeling, by consulting stakeholders for qualitative validation of our model calibration and results. While virtual engagement could be challenging, particularly due to constraints on meeting length, it was an effective way to maintain connections during the COVID-19 pandemic and between in-person visits; this allowed stakeholders to stay engaged throughout the entire research process. Our resulting integrated assessment modeling analysis reflects the priorities and perspectives of numerous Thai stakeholders at the city and national levels.
Data availability statement
The datasets presented in this study can be found in online repositories. The names of the repository/repositories and accession number(s) can be found below: GCAM-SEA (https://zenodo.org/records/10014560).
Author contributions
TW: Conceptualization, Investigation, Methodology, Visualization, Writing–original draft, Writing–review and editing. BP: Investigation, Validation, Writing–review and editing. PW: Investigation, Validation, Writing–review and editing. ZK: Conceptualization, Investigation, Methodology, Validation, Writing–review and editing. MW: Formal Analysis, Investigation, Methodology, Writing–review and editing. LP: Investigation, Methodology, Writing–review and editing, Formal Analysis. AC: Investigation, Validation, Writing–review and editing. SR: Investigation, Validation, Writing–review and editing. PP: Validation, Writing–review and editing, Investigation. MW: Investigation, Writing–review and editing. AJ: Writing–review and editing. DJ: Writing–review and editing. BL: Investigation, Validation, Writing–review and editing. ME: Conceptualization, Investigation, Methodology, Project administration, Supervision, Writing–review and editing.
Funding
The author(s) declare financial support was received for the research, authorship, and/or publication of this article. This research was supported by the U.S. Department of State, Bureau of Energy Resources, Power Sector Program, in support of the Clean EDGE Asia initiative and the U.S.-ASEAN Smart Cities Partnership. PNNL is operated by Battelle Memorial Institute for the U.S. Department of Energy under Contract DE-AC05-76RL01830.
Acknowledgments
The authors would like to thank Aine Shiozaki of the U.S. Department of State and Helen Santiago Fink of the U.S.-ASEAN Smart Cities Partnership. Special thanks to the many representatives from the following Thai authorities who met with our team to provide input data, discuss our results, and offer invaluable feedback and suggestions: the Bangkok Metropolitan Authority, the Metropolitan Electricity Authority, the Energy Policy and Planning Office, the Office of Natural Resources and Environmental Policy and Planning, and the Electricity Generating Authority of Thailand.
Conflict of interest
The authors declare that the research was conducted in the absence of any commercial or financial relationships that could be construed as a potential conflict of interest.
Publisher’s note
All claims expressed in this article are solely those of the authors and do not necessarily represent those of their affiliated organizations, or those of the publisher, the editors and the reviewers. Any product that may be evaluated in this article, or claim that may be made by its manufacturer, is not guaranteed or endorsed by the publisher.
Supplementary material
The Supplementary Material for this article can be found online at: https://www.frontiersin.org/articles/10.3389/fenrg.2024.1335290/full#supplementary-material
Footnotes
1CO2 prices are applied only to energy and industrial combustion and process CO2 emissions.
References
ADB (2013). Prospects for carbon capture and storage in Southeast Asia. Mandaluyong City, Philippines: Asian Development Bank. Available at: https://www.adb.org/sites/default/files/publication/31122/carbon-capture-storage-southeast-asia.pdf.
Ali, G., Pumijumnong, N., and Cui, S. (2017). Decarbonization action plans using hybrid modeling for a low-carbon society: the case of Bangkok Metropolitan Area. J. Clean. Prod. 168, 940–951. doi:10.1016/j.jclepro.2017.09.049
Bangkok Post (2020). MEA injects billions into Smart Metro Grid system. Available at: https://www.bangkokpost.com/thailand/pr/1839829/mea-injects-billions-into-smart-metro-grid-system.
C40 (2020). C40 climate action planning programme: vertical integration guide. Available at: https://www.c40knowledgehub.org/s/article/Climate-Action-Planning-Vertical-Integration-Guide?language=en_US.
Calvin, K., Patel, P., Clarke, L., Asrar, G., Bond-Lamberty, B., Cui, R. Y., et al. (2019). GCAM v5.1: representing the linkages between energy, water, land, climate, and economic systems. Geosci. Model. Dev. 12 (2), 677–698. doi:10.5194/gmd-12-677-2019
Chaichaloempreecha, A., Chunark, P., Hanaoka, T., and Limmeechokchai, B. (2022). Thailand’s mid-century greenhouse gas emission pathways to achieve the 2 degrees Celsius target. Energy, Sustain. Soc. 12 (1), 22. doi:10.1186/s13705-022-00349-1
Chaichaloempreecha, A., Winyuchakrit, P., and Limmeechokchai, B. (2017). “Long-term energy savings and GHG mitigations in Thailand’s building sector: impacts of energy efficiency plan,” in 2017 international conference on alternative energy in developing countries and emerging economies, 138, 847–852. doi:10.1016/j.egypro.2017.10.110
Chanraksa, T., and Singh, J. G. (2023). Benefits of demand response with electric vehicles in smart grid: a case study of pattaya city, Thailand. in 2023 international conference on power, instrumentation, energy and control (PIECON), 1–6. doi:10.1109/PIECON56912.2023.10085819
Charoen, P., Kitbutrawat, N., and Kudtongngam, J. (2022). A demand response implementation with building energy management system. Energies 15 (3), 1220. doi:10.3390/en15031220
Chunark, P., Hanaoka, T., and Limmeechokchai, B. (2021). Shared socioeconomic pathways and long-term GHG mitigation towards 2050 in Thailand cement industry. Clean. Responsible Consum. 2, 100006. doi:10.1016/j.clrc.2020.100006
DEDE (2018a). Energy efficiency plan 2018-2037 (EEP2018). Bangkok, Thailand: Department of Alternative Energy Development and Efficiency, Ministry of Energy.
DEDE (2018b). Alternative energy development plan 2018-2037 (AEDP 2018). Bangkok, Thailand: Department of Alternative Energy Development and Efficiency, Ministry of Energy.
Deguenon, L., Yamegueu, D., Moussa kadri, S., and Gomna, A. (2023). Overcoming the challenges of integrating variable renewable energy to the grid: a comprehensive review of electrochemical battery storage systems. J. Power Sources 580, 233343. doi:10.1016/j.jpowsour.2023.233343
DNP, (2021). Forest reference emission level and forest reference level Thailand. in Wildlife, and plant conservation (Bangkok, Thailand: Department of National Parks). Available at: https://redd.unfccc.int/media/modified_thailand_rl_july2021.pdf.
EPPO (2020). Thailand’s power development plan 2018-2037 revision 1. Energy policy and planning office. Ministry of Energy Available at: https://www.eppo.go.th/images/Infromation_service/public_relations/PDP2018/PDP2018Rev1.pdf.
Fong, W. K., Sotos, M., Doust, M., Schultz, S., Marques, A., and Deng-Beck, C. (2021). Global protocol for community-scale greenhouse gas Inventories: an accounting and reporting standard for cities version 1.1. World Resources Institute. https://ghgprotocol.org/sites/default/files/standards/GPC_Full_MASTER_RW_v7.pdf.
Fuhr, H., Hickmann, T., and Kern, K. (2018). The role of cities in multi-level climate governance: local climate policies and the 1.5 °C target. 1.5°C Clim. Change Urban Areas 30, 1–6. doi:10.1016/j.cosust.2017.10.006
Gordon, D. J. (2018). Global urban climate governance in three and a half parts: experimentation, coordination, integration (and contestation). WIREs Clim. Change 9 (6), e546. doi:10.1002/wcc.546
Griscom, B. W., Adams, J., Ellis, P. W., Houghton, R. A., Lomax, G., Miteva, D. A., et al. (2017). Natural climate solutions. Proc. Natl. Acad. Sci. 114 (44), 11645–11650. doi:10.1073/pnas.1710465114
Ho, C. M., Nguyen, L. T., Vo, A. T., and Vo, D. H. (2021). Urbanization and the consumption of fossil energy sources in the emerging Southeast asian countries. Environ. Urbanization ASIA 12 (1), 90–103. doi:10.1177/0975425321990378
IEA (2021). Carbon capture, utilisation and storage: the opportunity in Southeast Asia. Paris, France: International Energy Agency.
IRENA (2017). Electricity storage and renewables: costs and markets to 2030. Abu Dhabi, United Arab Emirates: International Renewable Energy Agency. Available at: https://www.irena.org/publications/2017/Oct/Electricity-storage-and-renewables-costs-and-markets.
Jaccard, M., Murphy, R., Zuehlke, B., and Braglewicz, M. (2019). Cities and greenhouse gas reduction: policy makers or policy takers? Energy Policy 134, 110875. doi:10.1016/j.enpol.2019.07.011
Jäger, J., Brutschin, E., Pianta, S., Omann, I., Kammerlander, M., Sudharmma Vishwanathan, S., et al. (2023). Stakeholder engagement and decarbonization pathways: meeting the challenges of the COVID-19 pandemic. Front. Sustain. 3. doi:10.3389/frsus.2022.1063719
Junlakarn, S., Hoonchareon, N., and Keskamol, K. (2017). “Interval pricing scheme of demand response to maximize benefits of utility and participants under a vertically integrated electric industry of Thailand,” in 2017 asian conference on energy, power and transportation electrification (ACEPT), 1–6. doi:10.1109/ACEPT.2017.8168586
Khan, Z., Waite, T., and Weber, M. (2023). gcamv6p0_seasia (Version v1). Zenodo. doi:10.5281/zenodo.10014560
Klansupar, C., and Chaitusaney, S. (2020). Optimal sizing of utility-scaled battery with consideration of battery installtion cost and system power generation cost. 2020 17th international Conference on electrical engineering/electronics, computer, Telecommunications and information technology (ECTI-CON), 498–501. doi:10.1109/ECTI-CON49241.2020.9158074
KLHK (2021). Indonesia long-term strategy for low carbon and climate resilience 2050. in Kementerian lingkungan hidup dan kehutanan. Available at: https://unfccc.int/sites/default/files/resource/Indonesia_LTS-LCCR_2021.pdf.
Lau, H. C. (2022). Decarbonizing Thailand’s economy: a proposal. Energies 15 (24), 9498. doi:10.3390/en15249498
Li, X., Gu, D., Hohenberger, T. L., Fung, Y. H., Fung, J. C. H., Lau, A. K. H., et al. (2023). Dynamic quantification of on-road emissions in Hong Kong: impact from traffic congestion and fleet composition variation. Atmos. Environ. 313, 120059. doi:10.1016/j.atmosenv.2023.120059
Limmeechokchai, B., Rajbhandari, S., Pradhan, B. B., Chunark, P., Chaichaloempreecha, A., Fujimori, S., et al. (2023). Scaling up climate ambition post-2030: a long-term GHG mitigation analysis for Thailand. Clim. Policy 23 (2), 168–183. doi:10.1080/14693062.2022.2126813
Lwasa, S., Seto, K. C., Bai, X., Blanco, K. R., Gurney, K. R., Kilkis, S., et al. (2022). “Urban systems and other settlements,” in Climate change 2022: mitigation of climate change. Contribution of working group III to the sixth assessment report of the intergovernmental panel on climate change. Editors P. R. Shukla, J. Skea, R. Slade, A. Al Khourdajie, R. van Diemen, D. McCollumet al. (Cambridge University Press). Available at: https://www.ipcc.ch/report/ar6/wg3/downloads/report/IPCC_AR6_WGIII_FullReport.pdf.
Matamala, Y., Flores, F., Arriet, A., Khan, Z., and Feijoo, F. (2023). Probabilistic feasibility assessment of sequestration reliance for climate targets. Energy 272, 127160. doi:10.1016/j.energy.2023.127160
MoE (2015). Smart grid development master plan 2016-2036. Bangkok, Thailand: Ministry of Energy. Available at: https://www.eppo.go.th/images/Power/pdf/smart_gridplan.pdf.
Murakami, A., Medrial Zain, A., Takeuchi, K., Tsunekawa, A., and Yokota, S. (2005). Trends in urbanization and patterns of land use in the Asian mega cities Jakarta, Bangkok, and Metro Manila. Ecol. Dyn. Urban Rural Landscapes East Asia 70 (3), 251–259. doi:10.1016/j.landurbplan.2003.10.021
O’Neill, B. C., Carter, T. R., Ebi, K., Harrison, P. A., Kemp-Benedict, E., Kok, K., et al. (2020). Achievements and needs for the climate change scenario framework. Nat. Clim. Change 10 (12), 1074–1084. doi:10.1038/s41558-020-00952-0
ONEP, (2022). Thailand’s long-term low greenhouse gas emission development strategy (revised version). in Office of natural resources and environmental policy and planning, Ministry of natural resources and environment. https://unfccc.int/sites/default/files/resource/Thailand%20LT-LEDS%20%28Revised%20Version%29_08Nov2022.pdf.
O’Rourke, P. R., Smith, S. J., Mott, A., Ahsan, H., McDuffie, E. E., Crippa, M., et al. (2021). CEDS v_2021_02_05 release emission data (v_2021_02_05). Zenodo. doi:10.5281/ZENODO.4509372
OTP, (2013). Sustainable transport master plan 2013-2030. Office of transport and traffic policy and planning. Bangkok, Thailand: Ministry of Transport.
Pansiri, K., and Sriisaree, P. (2018). Travelled vehicle-kilometers on highways report 2017. Bangkok, Thailand: Bureau of Highway Safety.
Phayomhom, A., Chotiheerunyasakaya, N., Kheawkham, M., and Songsit, S. (2015). “MEA Smart Grid roadmap and initiation of the smart district office building pilot project,” in 2015 IEEE innovative smart grid technologies - Asia (ISGT ASIA), 1–5. doi:10.1109/ISGT-Asia.2015.7387095
Pongthanaisawan, J., Wangjiraniran, W., and Nakapreecha, N. (2023). Thailand energy scenarios: pathways towards carbon neutrality 2050. Int. J. Energy Econ. Policy 13 (1), 489–500. doi:10.32479/ijeep.13884
Pradhan, B. B., Chaichaloempreecha, A., Chunark, P., Rajbhandari, S., Pita, P., and Limmeechokchai, B. (2022). Energy system transformation for attainability of net zero emissions in Thailand. Int. J. Sustain. Energy Plan. Manag. 35, 27–44. doi:10.54337/ijsepm.7116
Pradhan, B. B., Chaichaloempreecha, A., and Limmeechokchai, B. (2019). GHG mitigation in agriculture, forestry and other land use (AFOLU) sector in Thailand. Carbon Balance Manag. 14 (1), 3. doi:10.1186/s13021-019-0119-7
Praiwan, Y. (2023). Eppo prepping hydrogen pilot project. Bangkok Post Available at: https://www.bangkokpost.com/business/2603058/eppo-prepping-hydrogen-pilot-project.
Preedakorn, K., Butler, D., and Mehnen, J. (2023). Challenges for the adoption of electric vehicles in Thailand: potential impacts, barriers, and public policy recommendations. Sustainability 15 (12), 9470. doi:10.3390/su15129470
Promjiraprawat, K., Winyuchakrit, P., Limmeechokchai, B., Masui, T., Hanaoka, T., and Matsuoka, Y. (2014). CO2 mitigation potential and marginal abatement costs in Thai residential and building sectors. Energy Build. 80, 631–639. doi:10.1016/j.enbuild.2014.02.050
Sattayathamrongthian, M., and Vanpetch, Y. (2023). Road freight transportation business operator’s perception toward electric vehicle adoption in Nakhon Pathom, Thailand. E3S Web Conf 389, 05020. doi:10.1051/e3sconf/202338905020
Siala, K., and Stich, J. (2016). Estimation of the PV potential in ASEAN with a high spatial and temporal resolution. Renew. Energy 88, 445–456. doi:10.1016/j.renene.2015.11.061
Sodsai, P., and Rachdawong, P. (2012). The current situation on CO2 emissions from the steel industry in Thailand and mitigation options. Int. J. Greenh. Gas Control 6, 48–55. doi:10.1016/j.ijggc.2011.11.018
TCMREH (2017). Basic hydrogen strategy. Tokyo, Japan: The Council of Ministers on the Renewable Energy and Hydrogen. Available at: https://www.cas.go.jp/jp/seisaku/saisei_energy/pdf/hydrogen_basic_strategy.pdf.
U.S. Department of State (2021). The long-term strategy of the United States: pathways to net-zero greenhouse gas emissions by 2050. Available at: https://unfccc.int/sites/default/files/resource/US-LongTermStrategy-2021.pdf.
Yimprapai, A., and Chaitusaney, S. (2022). “Optimal sizing of BESS considering economic dispatch and VRE in Thailand generation system,” in 2022 IEEE international conference on cybernetics and computational intelligence (CyberneticsCom), 67–71. doi:10.1109/CyberneticsCom55287.2022.9865363
Keywords: integrated assessment model (IAM), stakeholder engagement, Thailand, Bangkok, cities, decarbonization
Citation: Waite T, Pradhan BB, Winyuchakrit P, Khan Z, Weber M, Pressburger L, Chaichaloempreecha A, Rajbhandari S, Pita P, Westphal MI, Jonvisait A, Jareemit D, Limmeechokchai B and Evans M (2024) Stakeholder-driven carbon neutral pathways for Thailand and Bangkok: integrated assessment modeling to inform multilevel climate governance. Front. Energy Res. 12:1335290. doi: 10.3389/fenrg.2024.1335290
Received: 08 November 2023; Accepted: 18 January 2024;
Published: 09 February 2024.
Edited by:
Douglas Jackson-Smith, The Ohio State University, United StatesReviewed by:
Puneet Kamboj, King Abdullah Petroleum Studies and Research Center (KAPSARC), Saudi ArabiaLu Liu, Iowa State University, United States
Copyright © 2024 Waite, Pradhan, Winyuchakrit, Khan, Weber, Pressburger, Chaichaloempreecha, Rajbhandari, Pita, Westphal, Jonvisait, Jareemit, Limmeechokchai and Evans. This is an open-access article distributed under the terms of the Creative Commons Attribution License (CC BY). The use, distribution or reproduction in other forums is permitted, provided the original author(s) and the copyright owner(s) are credited and that the original publication in this journal is cited, in accordance with accepted academic practice. No use, distribution or reproduction is permitted which does not comply with these terms.
*Correspondence: Taryn Waite, dGFyeW4ud2FpdGVAcG5ubC5nb3Y=