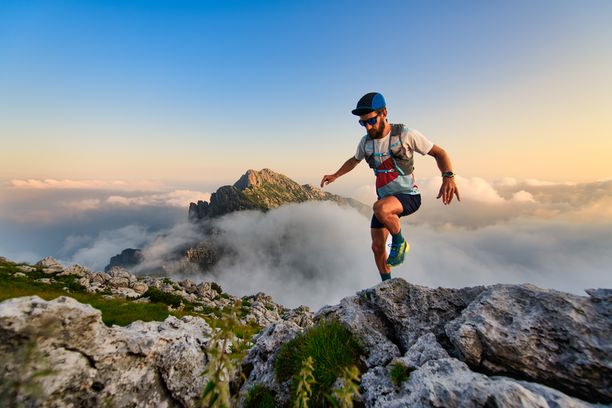
94% of researchers rate our articles as excellent or good
Learn more about the work of our research integrity team to safeguard the quality of each article we publish.
Find out more
REVIEW article
Front. Energy Res., 09 April 2024
Sec. Process and Energy Systems Engineering
Volume 12 - 2024 | https://doi.org/10.3389/fenrg.2024.1251188
Photo-thermal-catalysts (PTC) exceptional features and ability to concurrently solve sustainable energy requirements and environmental problems have drawn scientific interest. The photo-thermal effect is the process by which photo-thermal conversion materials transform radiant energy from light into thermal energy. The photo-thermal therapy of energy and environmental treatment faces the urgent task of developing effective photo-thermal agents to address complicated problem demands. In this study, we take a look at the fundamental principles and underlying process of photo-thermal material catalysis. In addition, this review provides a concise summary of the most up-to-date findings concerning photo-thermal catalysis materials, which have been aggressively researched for a wide-spread sustainable energy and environment treatment uses. In order to motivate the creation of a high-efficiency photo-thermal catalytic pathway, the criteria and techniques for designing photo-thermal catalysts are presented. This will make it possible for a better absorption of the whole spectrum of solar energy as well as a greater conversion of solar energy to heat. Theoretical direction is offered for the continued development of this technology, and the promise of the practical use of photo-thermal catalysis for different solar-driven interfacial photo-thermal conversion technologies is anticipated. This paper offers a unique perspective on photo-thermal catalysis focussing on the synergistic effects and suggesting a potential route for future applications.
Energy scarcity and environmental degradation have emerged as two of the main problems endangering the survival and advancement of mankind in light of the expanding population and the swiftly developing global economy. Intern, environmental restoration and renewable energy are urgent problems that necessitate efficient, long-term, and environmentally friendly solutions (Kho et al., 2017). Most of the world’s energy needs are still met by burning conventional fossil fuels like oil, natural gas, and coal (Govorov and Richardson, 2007). However, significant consumption of fossil fuels can cause serious environmental problems and massive volumes of carbon emissions (An et al., 2020). Among all renewable energy resources, solar energy stands out from other renewable options (such as tidal, wind, or geothermal power) because of its abundance, global sustainability, and ease of access and cleanliness (Mekhilef et al., 2011). Many young researchers have found that there are primarily three ways to put solar energy to use: through thermal conversion, electrical conversion, and chemical conversion. Considerable published research papers have recently addressed solar-to-thermal conversion in the fields of solar heat collecting, photo-thermal saltwater evaporation, and photo-thermal catalysis (Campos et al., 2019; Shan et al., 2020; Li et al., 2021a; Luo et al., 2021a). Most commonly, solar cells and photoelectric catalysis make use of solar-to-electricity conversion. Nevertheless, research into solar-to-chemical conversion has focused mostly on three up-hill reactions: photocatalytic water splitting, photocatalytic carbon dioxide reduction, and photocatalytic nitrogen fixation (Zhang et al., 2021).
Recently, photo-thermal catalysis has been proposed as a new way to drive chemical reactions. Photo-thermal conversion is one of these technologies that has achieved the best conversion exhibit efficiency possible through a direct conversion process. Photo-thermal-catalysis exhibits the ability to improve reaction rate and alter selection patterns, even at mild operating considerations, due to Sun’s synergistic photo- and thermo-chemical effects. The sunlight spectrum and local reaction temperature of photo-thermal catalysts are often substantially larger than those of room-temperature-operating photo-catalysts (Ghoussoub et al., 2019a). In addition, unlike thermal catalysis, which is driven by fossil fuels, photo-thermal catalysis shows clear evidence of cheap cost and cleanliness utilizing solar energy as a cleaner heat source (Li et al., 2020). The foregoing encourages the widespread use of photo-thermal materials in several fields, particularly those involving the environment and catalysis. The process of absorbing sunlight and converting it to heat for evaporation depends heavily on photo-thermal materials (Gelle et al., 2019). Author Ozin and co-workers found that hot carriers and the thermal influence work together to increase total reaction speed in photo-thermal catalysis, with the relative importance of the two mechanisms dependent on the operating environment (Ghoussoub et al., 2019b). The photo-thermal effect, for instance, occurs in the case of plasmonic materials as a result of the interaction of the thermal as well as photo-chemical donations of absence of radiative plasmon decay. However, it should be stressed that plasmonic nanoparticles are not the only objects capable of experiencing the photo-thermal effect. Even while the operating mechanisms for photo-thermal effectiveness in semiconducting oxides, chalcogenides, metal-organic-frameworks (MOF), and various materials made from carbon based graphene and carbon nanotubes (CNTs) have received less attention, these materials can also exhibit photo-thermal accomplishments (Zhao et al., 2019a).
In order to collect sunlight and transform it into heat for evaporation, photo-thermal materials are essential. Over time, solar-driven evaporation has progressed from relying on widespread nanomaterial heating to relying on pinpoint heating at the surface. In recent years, there has been significant development in this area, including the creation of novel photo-thermal materials with high light absorption and high light-to-heat conversion, as well as improvements in thermal management, which have led to greater heat utilization, more efficient evaporation, and the prevention of salt scaling, all of which are essential for the reliable long-term operation of these systems. Researchers are also looking beyond solar thermal power plants at additional energy and environmental uses made possible by photo-thermal materials and systems (Wu et al., 2020). For instance, during oil spill clean-up or petroleum transportation, the thermal energy from light-to-heat conversion can be employed to reduce the viscosity of crude oil (Kuang et al., 2019). Also, sterilization can be accomplished using the hot steam produced by solar heating (Li et al., 2018). However, it has been observed that photo-thermal evaporation can be combined with other techniques for more long-term applications. For instance, in wastewater treatment, a photo-thermal evaporator embellished with a photo-catalyst can be used for both evaporation and contaminant degradation (Yang et al., 2020). Meanwhile, when used in conjunction with hygroscopic materials, a photo-thermal evaporator can harvest ambient moisture for use in a condensation-evaporation cycle that yields potable water (Yang et al., 2020). Given the recent surge of interest in photo-thermal catalysis for green energy and chemical synthesis, we felt it was time for a comprehensive overview of the topic. The purpose of this article is to give a foundational understanding of photo-thermal catalysis and to generate a reference that may elucidate important considerations for future designers of photo-thermal materials for use in sustainable energy and environmental remediation. In this study, we take a fresh look at photo-thermal catalysis, with an emphasis on its synergistic effects and a proposed direction for future applications.
Full utilization of spectrum is gaining traction thanks to the present development of PTC, which relies on the combination of solar thermal and photochemical activities. The catalytic reactions are effectively driven at comparatively mild circumstances by the synergistic interaction of the thermochemical and photo-chemical activities. To produce an effective photo-thermal conversion, it is crucial to take into account a material’s optical and thermal properties. Material choice and the optimization of the light-to-heat conversion depend heavily on optical parameters like absorption-coefficient, selectivity and fitting of a material’s absorption spectrum to the incident light, also loss and transfer of heat. For real-world applications, it is important to consider not just the materials’ intrinsic qualities, but also their thermal stability, mechanical integrity, and cost. In the last decade, there has been a growing movement toward the methodical examination of the combined effects of thermal and photo-excitation. Photo-thermal catalysis was coined by Tang et al. as an umbrella word for both photocatalytic reactions that are sped up by heat and thermos-catalytic reactions that are boosted by light (Tang et al., 2017). The Colmenares group, however (Nair et al.), distinguished between the two and categorized them as photo-thermo-catalysis and thermo-photo-catalysis, wherein the corresponding descriptors relate to a photo-effect on a thermal catalyst or to a thermal-effect on a photo-catalyst, respectively (Nair et al., 2019a). In fact, there has been much discussion on what exactly is meant by the word “photo-catalysis.” At the extreme end, it might only be understood to refer to redox reactions in adsorbed species that are the result of optical (photonic) acceleration of electronic transitions in bulk semiconductor particles (electron/hole generation), followed by surface movement, etc.
Generally speaking, the term “photo-thermal” describes the process by which specific materials or structures transform incoming light into thermal energy. In spite of this, the “photo-thermal” character of PTC means that it cannot be reduced to a straightforward “light-to-heat conversion.” Overall, irradiation impact allows for a simple categorization of PTC into two distinct categories. One variety of PTC is wholly reliant on light-to-heat conversion, and it stands out in that the catalytic outcomes are same at the same temperature in the light and the dark. One should shorthand this process as PTC-T (for “photo-thermal chemistry with a thermal dominance”). Thermochemistry is used to explain the mechanism of the chemical processes that power PTC-T, in accordance with the light heat chemistry (L-H-C) approach. Conventional L-H-C activities may differ primarily in that it is possible to distinguish between the heat collecting and chemical reaction techniques, whereby a high concentration ratio (CR) concentrator and receiver are often used to achieve the high temperatures required by the solar reactor. PTC’s ability to generate heat locally when subjected to irradiation is due in large part to the material’s unusual ability to absorb light. The PTC-T system proposes the use of solar energy in place of more conventional fuels for use in thermochemistry (Villafan-Vidales et al., 2017). The second form of PTC features both thermal effects (either from light-to-heat conversions within the device or from external auxiliary heat supplies) and non-thermal impacts. The role of irradiation in this situation cannot be entirely attributed to the rise in temperature, and the intrusion of light will alter the outcomes even when the temperature stays unchanged. So one can refer this as Synergistic-photo-thermal-chemistry, (PTC-S). The interplay of thermal and non-thermal factors can make the PTC-S process even more difficult. PTC-S has the ability to produce superior reaction outcomes than thermochemical procedures precisely because of this, making it feasible to increase reaction pace and energy efficiency while maintaining fairly low temperatures. However, it is possible that these two effects will sometimes counteract one another. Understanding how the thermal and non-thermal effects operate in chemical processes is crucial to realize the lovely picture.
PTC typically has two heat sources: one that derives its energy from irradiation, and another that derives its heat from outside. In both semiconductors and plasmonic materials, the creation of energetic carriers is quickly followed by their radiative and/or non-radiative decay. Photons, the kind of energy emitted after radiative decay, are detectable using photoluminescence spectroscopy. Electron-electron scattering is followed by electron-phonon scattering, and the electrons’ energy is converted to heat through this process and the non-radiative relaxation of energetic carriers. To put it more simply, the lattice vibration will become more intense as the energy of the particles gets transmitted to the phonons, and this will be seen macroscopically as a rise in temperature (Meng et al., 2016). It is more common for plasmonic nanoparticles to undergo local thermalization. One on the other hand, hot carriers have lives on the order of femtoseconds, which is much smaller than the timeframes (on the order of milliseconds to seconds) necessary for reactions and comparable to the lifetimes of ordinary semiconductors (on the range of picoseconds to microseconds) (Brongersma et al., 2015). Employing plasmonic nanoparticles as heat sources has received increasing attention, despite the fact that heat production was once seen as a nuisance that needed to be mitigated (Baffou et al., 2020). Furthermore, many organic materials may absorb light and transform it into heat through lattice vibration, particularly when the structure has numerous linkages. The abundance of conjugated π bonds in graphene-like allotropes, which can produce different π-π* transitions, can boost light absorption at practically every wavelength of solar irradiation. As a result, they often have dark hues. A rise in temperature results from the excited electrons relaxing after the π-π* transitions due to electron-phonon coupling (Gao et al., 2019).
The photothermal effect refers to the process by which incident radiation energy is transformed into thermal energy, resulting in a measurable rise in temperature. Photoexcitation of matter makes it, which leads to the creation of heat energy. Scientists have extensive experience with the photothermal action of sunshine, which is used in solar water heaters. Also, there’s the matter of designing devices for photothermal power generation and photothermal energy storage. The photothermal effect has been seen in many photothermal materials, including inorganic materials (plasmonic metals and semiconductors) and biological materials (polymers). These materials change light into heat when they are exposed to it. The energy of the hot charge carriers created by the decay of localised surface plasmon resonance (LSPR) is greater than that of the charge carriers created by direct photoexcitation. In addition, these energetic carriers can de-excite on the inside, releasing their heat to the surrounding area and warming the material. Numerous fields have made use of the photo-thermal effect, including cancer treatment, pollution destruction, desalination of seawater, and evaporation of water. Furthermore, modifying the geometrical configuration and dielectric constant of metals permits enhancement in the photothermal effect exhibited by plasmonic and non-plasmonic structures. Solar desalination, photo-catalysis, and phototherapy are just a few of the many uses for the exceptional photothermal effect caused by surface Plasmon’s (SPs). One common strategy for increasing the effectiveness of designed metallic nanostructures in photothermal conversion is to take advantage of their thermos-plasmonic characteristics. Using spatial plasmon resonance in the metallic nanostructure, thermo-plasmonic behaviour enables fine-tuned heat regulation on the nano-scale. Both the creation of the evanescent wave through the interaction of radiation with metallic nanostructures and the absorption of the evanescent wave’s dissipation within the nanostructures play significant roles in shaping this behaviour. The thermos-plasmonic effect is thus affected by the nanostructure’s composition and shape. Radiation-induced Joule heating in these metallic nanoparticles is followed by localised surface plasmon resonance (LSPR). Nanostructures exhibiting thermo-plasmonic behaviours have traditionally been fabricated from precious metals including gold, silver, and aluminium. The aforementioned substances are fragile and thermally unstable. Titanium nitride (TiN) is an option that has been found to be both refractory and stable (Reddy et al., 2017). When exposed to both visible and near-infrared light, TiN reveals its exceptional thermoplasmonic capabilities. The substantial photothermal impact of nanostructured TiN has also been shown in prior studies (Yu et al., 2021).
Plasmonic structures consist of both microstructures and nanostructures, with their thermos-plasmonic characteristics being influenced by the appropriate optical reflection. Interest in plasmon-mediated processes is second only to that in charge separation upon light excitation. The manipulation of the surface topography of plasmonic devices enables the characterization of optical reflectance and the enhancement of thermos-plasmonic characteristics. A decrease in optical reflectance signifies the presence of a greater number of non-radiative electromagnetic waves within the plasmonic structures. Ultra-small gold nanoparticles reported to improve photothermal effects when attached to huge graphene sheets. However, specific nanoparticles with clear boundaries are needed for practical photothermal therapy, as opposed to broad sheets. In addition, the Hierarchical plasmonic structures (HPSs) can transform the energy of an electromagnetic wave that does not radiate into heat. Microstructure and nanostructure in hierarchical plasmonic structures were fabricated utilising a conventional semiconductor technique. Using deep reactive ion etching and the MACE technique, the surface topography of HPSs can be modified in two steps. This two-step process for the HPSs can be used to find out where the nanostructured TiN is distributed in space. The suggested HPSs has a lot of promise as a photothermal substrate that can be tuned for thermos-plasmonic properties. When plasmonic excitation happened, NPs created hot charge carriers by switching between electronic bands and/or within bands without using radiation to dampen them (Qian et al., 2019). Excited electrons with energies greater than the Fermi level (EF + hv) are accelerated by photons with hn energy, and these electrons can be driven by metallic nanoparticles to other species that are electron-deficient. The kinetic energy of the excited hot electrons was redirected via plasmon-decay to the adsorbates, which were then chemically activated by the changes in electrical and vibrational motion. Subsequent chemical reactions were set in motion when the higher-energy electrons migrated to the antibonding orbitals of interfacial species, causing a split in the molecular bonds. Based on whether the plasmonic photo-catalyst is a homogeneous or heterogeneous structure, four distinct pathways exist for using these hot charge carriers in various catalytic applications. Hot charge carriers from isolated plasmonic NPs interact with adsorbates either directly or indirectly in the first two plasmon-based photocatalytic materials. In the alternative pathways, plasmonic nanostructures that were assisted were directly elevated to the conduction band by an indirect insertion mechanism, hence facilitating their interaction with semiconductors and carriers or acceptor species.
Non-plasmonic components, such as those with direct intraband and/or interband electronic transitions, can also exhibit the photo-thermal effect, just as plasmonic structures. Intriguingly, it was discovered that, under visible light irradiation, the intrinsic catalytic activity of non-plasmonic metals can be improved for a variety of processes, even at elevated temperatures. Insight into the possibility of co-use of thermal energy and photon flux in non-plasmonic metals is provided by this finding. While the increased photocatalytic activity over non-plasmonic metals has been seen, the fundamental basis is still poorly understood. Collective behaviour is more common among plasmonic particles than among non-plasmonic ones. Maximum energy absorption or transfer occurs, and this phenomenon is called resonance, when a beam of radiation with the right wavelength collides with an object. In addition, unlike most non-plasmonic materials, plasmonic ones exhibit features like scattering, absorbance, and unique coupling. Many essential industrial reactions can be catalysed by non-plasmonic metals such Pd, Pt, Ru, Rh, and Ni. Furthermore, they can absorb visible light by a weaker light absorption mode than s LSPR called interband electronic transitions (electrons in the d-band of the metal nanoparticles are driven to high energy levels of their s-band). Visible light can also be used to efficiently drive organic changes when a nanoparticle is non-plasmonic and is made from a metal in the Pt group. Creating photo-catalysts from nanoparticles that non-plasmonic or made from precious metals is important for a number of reasons. For instance, Sarina et al. showed that cross-coupling reactions may be catalysed at room temperature using visible light and non-plasmonic metal NPs supported on ZrO2 (Sarina et al., 2014). When exposed to UV light, the electrons, like those in plasmonic metal NPs, can undergo interband transitions to higher energy levels, and only those with adequate energy can move to the LUMO of adsorbed molecules. To some extent, the increased reaction rate due to temperature effects can be attributed to the inability of electrons generated by low-energy visible-IR light to inject into adsorbate states. To get the most out of the photochemical process, it is crucial to have an efficient charge separation in metal oxide non-plasmonic semiconductors.
An emerging and quickly expanding trend over the past decade has been the methodical examination of the combined effects of thermal and photo-excitation. As a result, there is no standardised’ vocabulary or agreed-upon singular definition. As a result, there is some consternation over reports that appear to describe the same phenomenon when, in fact, they do not. Two recent reviews, for instance, both use the word “photothermal,” but their meanings are slightly different: for Ghoussoub et al., it refers to (synergies in) simultaneous illumination under heating, and for Wang et al., it refers to the localised heating effect arising from light absorption and non-radiative relaxation (Ghoussoub et al., 2019a; Wang et al., 2020a). Instead, it is essential to thoroughly examine every paper that introduces terminology that can be broadly classified as “photo-thermal” in order to determine the precise methodology or phenomena to which they relate. Differentiating numerous synergistic effects that may occur under combined excitation from basic principles should be doable through kinetics experiments. In the same basic order, photo-excitation triggers a reaction via a different low-energy transition state, while thermal excitation operates on a different rds of the dark type. The Eapp, or apparent activation energy, is predicted to decrease. The steady-state equilibrium of intermediate coverage of (active) catalytic surfaces is shifted favourably by photo-excitation. The rate of a reaction can be increased in one of two ways: by increasing access to the limiting reactant(s) or by preventing the build-up or diffusion of poisonous by-products in the catalyst. It is possible that a shift in reaction sequence might accompany this concentration impact, there should be no shift in Eapp. To supplement a thermal process operating at a predetermined base (bulk) temperature, photo-excitation can be used to transfer localised heat (by non-radiative relaxation). Eapp is not likely to undergo any changes. On the other hand, the Colmenares group (Nair et al.) distinguished between photo-thermo-catalysis and thermo-photo-catalysis, where the former describes a photo-effect on a thermal catalyst and the latter describes a thermal-effect on a photo-catalyst (Nair et al., 2019b). While it is obvious that a catalytic system powered by heat enhancement (a higher temperature that crosses an activation energy barrier) requires the thermo prefix, the photo prefix is less clear and relies heavily on the reaction mechanisms, which is often poorly understood. One interpretation of this term would be that it only applies to redox processes in adsorbed species that are triggered by optical (photonic) stimulation of electronic transitions in the bulk semiconductor particles (electron/hole generation), followed by surface migration, etc. Overcoming activation energy limitations via thermal and photo-excitation techniques were formerly seen as two distinct options. A photo-catalyst may provide an alternative pathway at room temperature to the same product(s) via a new transition state with lower potential energy, reducing the amount of energy needed to carry out the process. When the rate determining step (rds) is overcome by catalytic methods, such as photo-excitation, the re-established kinetics are governed by a different rds further along in the mechanistic sequence, albeit at a quicker rate. It is possible that heat activation plays a secondary but crucial function in the photo-process, even if this is of the ‘dark’ variety. The idea that “a chain (reaction) is only as strong (fast) as its weakest (slowest) link” can be shown with this basic concept, which shows how many excitation modes can complement one another.
One of the most prevalent types of catalysis is thermal catalysis, which is used in classic industrial procedures like ammonia synthesis and reforming to counteract the endo-thermicity of the reactions involved. The catalyst models may also be utilized as a photo-thermal transferring agent to raise temperature of the reaction by converting solar light energy to thermal heat energy, therefore activating the process or accelerating its kinetics, similar to what happens in thermos-catalysis (Hu et al., 2022). Certain semiconducting and plasmonic catalysts, (energized electrons and holes with semiconductors and heated electrons with plasmonic nano structures), have a synergistic impact of produced carriers of charges by photon excitation in addition to the photo-thermal impact. Wherein even has a preponderant function at times in enhancing catalytic performance (Wang et al., 2020b). The photo-electro-catalysis technique, which primarily relies on effectively photo generated electron-hole segregation for driving chemical responses, is distinct from these methods. It also differs from thermal catalysis, which usually takes place in the absence of sunlight and is characterized by temperature-driven chemical processes (Zhao et al., 2019b). Solar light may be utilized to break down chemical bonds during the photo-thermal catalysis methods to breakdown organic contaminants. Additionally, it can create new chemical bonds for storing of energy in substances like methane (CH4), carbon dioxide (CO), and hydrogen (H2) (Fu et al., 2021a; Wu et al., 2021). As a result, photo-thermal catalysis may be used in place of or in addition to thermal catalysis and photo-electro-catalysis, offering a viable and practical solution to the world’s energy crises and environmental issues.
Rapid growth in photocatalytic research can be traced back to the pioneering research conducted by Honda and Fujishima on the application of a titanium dioxide semiconductor to the process of water splitting (Fujishima and Honda, 1972). Although the idea of directly converting light into chemical energy is appealing. Inadequate conversions severely limit the usefulness of photocatalytic systems in the real world. Particular for large-scale industrial applications, where the efficiency gap is often several orders of magnitude lower than what can be achieved using heat catalysis (Agrafiotis et al., 2014). As a result, the idea of using light to generate heat for catalysis emerged as an attractive replacement for current systems that rely on fossil fuels. The use of both the light and heat aspects of solar radiation, with heat being either derived directly from or triggered by the light absorption, sets photo-thermal catalysis apart from more conventional thermal- or photo-catalysis. This paves the way for the use of a renewable energy source (photo-catalysis) while yet achieving the efficient catalytic reactions usually associated with thermal catalysis. Figure 1A presents the photo-thermal catalysis (Song et al., 2022). Has studied the photo-thermal catalysis for CO2 conversion. Large amounts of attention have been paid to photo-thermal catalysis for CO2 conversion as a means to reach carbon emission peak and carbon neutrality shown in (Figure 1B) (Zhou et al., 2023).
Figure 1. (A) Photo-thermal catalysis, different mechanisms of photo-thermal effect, reproduced with permission from reference (Song et al., 2022) ________________ Elsevier. (B) Photo-thermal catalysis for CO2 conversion reproduced with permission from reference (Zhou et al., 2023) ________________ Elsevier.
Author, Xintong Zhang et al. have conducted research on Bi4TaO8Cl/Bi heterojunction enabled highly selective photo-thermal catalytic changes occurred in carbon dioxide to water (CO2-H2O) flow to alcohol (Li et al., 2022). Authors showed catalyst triggered charge detachment occurred on the Bi/Bi4TaO8Cl interfaces, also Bi-nanoparticles offered highest photo-thermal transformation observed through SPR influence. The CO2 transformation range and liq. alcohol property of Bi-Ov-Bi4TaO8Cl catalyst, which had an ideal Bi-nanoparticles load capacity around 9.11 Wt %, were both dramatically increased. Under broadband light irradiation, the maximal range of liq. alcohol synthesis (CH4 and CH2OH) was around 7.45 mol−1 g−1 h−1, with a selectivity of 92%. At the end, they confirmed that the work overcame the slow CO2 transformation value and high level CO generation derived from pure Bi4TaO8Cl catalyst in photo-thermal catalytic processes, this offers a new approach to generate valuable liquid fuels from CO2. Photo-thermal Catalysis of CO2 reduction efficiency of Bi-Ov-Bi4TaO8Cl compounds for liquid CH3OH and CH3CH2OH products is shown in Figure 2. The mechanism proposed to explain this result is depicted in the lower panel. Furthermore, Liqun Ye and his co-workers done research on photo-thermal synergetic catalytic CO2 conversion via photo-thermal material coated with TIO2. Experiment showed the synergistic influence of solar radiation whereas photocatalytic properties (2% CuS/TiO2) led to significantly greater CO2 reduction efficiency. Because CuS can absorb infrared light and convert it to thermal energy, it expands the solar spectrum usable for CO2 conversion (Deng et al., 2022).
Figure 2. Projected mechanism of photo-thermal catalysis for CO2 reduction activity of Bi-Ov-Bi4TaO8Cl compounds obtained for liq. CH3OH and CH3CH2OH compounds, reproduced with permission from reference (Li et al., 2022) ________________ Elsevier.
Another research group conducted the study on solar driven photo-thermal catalysis used for C-C coupling from CO2 reduction over WO3-x. For photo-thermal CO2 transferred to C2 hydrocarbons in occupancy of sunshine, the WO3 nano sheets demonstrate remarkable catalytic activity. By introducing oxygen vacancies, the band gap of WO3 may be shrunk, expanding the material’s absorption range to the plenoptic region and resulting in a greater photo-thermal synergy. Overall, according to the study, oxygen vacancies can greatly increase photocatalytic effectiveness by promoting C-C coupling to C2 hydrocarbons using a photo-thermal catalytic CO2 reduction process over WO3 (Deng et al., 2022).
Over the past several years, many photo-thermal materials, including as semiconductors, plasmonic metals, carbon and polymeric materials, have been produced. Speaking on ideal properties, high photo-thermal efficiency and long-term stability are desirable qualities in a photo-thermal material, and they are in turn dictated by the material’s inherent structures and the methods by which it converts light into heat. Increases in photo-thermal efficiency can be obtained via measures such as increase in the proportion of total light absorption, decrease in light reflection, transmission, and preventing unanticipated energy transformations. The proposed photo-thermal materials must to ideally possess the following qualities: a) wide bandwidth spectrum absorption, b) highly appreciated photo-thermal conversion efficiency, c) Physicochemical as well as processing stability, and d) economic feasibility. Apart from above characteristics, in terms of applications of photo-thermal materials will be well appreciated if material possesses working characteristics such as, a) High solar absorption is achieved when the solar absorber material is capable of efficiently absorbing light from the whole solar spectrum range, b) High light-to-heat conversion occurs when a solar absorbent material effectively transforms the energy it receives from light into heat without losing any of that heat’s original thermal energy in the process, c) Efficient transfers of heat for evaporation, where the produced heat is used to overcome the latent heat of evaporation of water while reducing loss of heat, is the result of well-managed thermal systems, and d) Materials for the substrate should have good water drainage, thermal insulation, and mechanical strength (Gao et al., 2021).
For instance, important components of the water purification procedure, especially the membrane distillation (MD) process, include photo-thermal substances that operate as Sun absorbers and the supporting structure, which works as thermal-insulating and water-transporting material (Ahmed et al., 2020). It is envisaged that photo-thermal materials would have a wide range of absorption across the Sun spectrum. Regardless of whether or not this component is a semiconductor, light sensitivity is a necessary component of a so-called photo-thermo-catalyst (Guo et al., 2015). However, much time and energy has been spent on research into promising nanomaterials for photo-thermal conversions; these include noble metals, transition metals, carbon, and non-metallic materials (Ni et al., 2008; Tong et al., 2015; Hoch et al., 2016; Chen et al., 2018; Han et al., 2018). Additionally, several modulation mechanisms have been explored to further improve photo-thermal effectiveness for various classes of photo-thermal materials. Nanomaterials that can produce customized photo-thermal impacts are constantly being created to fulfil the particular application specifications for photo-thermal treatment and solar vapour generating equipment’s (Wu et al., 2019). Nanoscale metallic structures, carbon-based light absorbers, inorganic semiconductors, and polymeric materials all play a role in these photo-thermal technologies.
Carbon compounds have significant light absorption, extensive sources, and good chemical stability, making them one of the most commonly utilized for photo-thermal purposes in various solar-driven operations. Furthermore, carbonized organic materials, such as carbonized wood, mushrooms, sucrose, sunflower, bamboo, lotus seedpods, and melamine sponge, are additional significant sources of carbon (Ghasemi et al., 2014; Yang et al., 2018; Singh et al., 2019). In most cases, the uses of carbonized materials benefit from the porous structure that was present in the materials before they were carbonized. For instance, using activated carbon (AC), a vacuum triggered filtration process, and an industry based spray coating technology, Sungchan Nam et al. developed a low-cost, straightforward, and scalable solar-to-steam generator. Light absorption, water uptake characteristics, and mechanical robustness were all significantly enhanced, which they attributed to the PEI-adsorbed on AC photo-thermal layers. Under simulated irradiation of 1 Sun with an increase of 3.5, the produced photo-thermal layers attained an evaporation speed of 1.27 kg m−2/h. Outdoor field tests utilizing A4-sized photo-thermal layers under roughly 1 Sun light showed a significantly reduced rate of 0.85 kg m−2 h−1, corresponding to an enhancement factor of 4.1. The solar-driven steam production employing the AC-based photo-thermal layers produced water with a salinity two orders of magnitude lower than the WHO’s requirement for potable water (Mnoyan et al., 2020).
Commercial applications of engineered carbon-based nanomaterials are expanding rapidly in the areas of cutting-edge technology, medicine, the environment, and agriculture. Researchers and businesspeople alike have been focusing intently on carbon-based nanomaterials because of their unique properties, which has fuelled the development of new methods of mass production. Carbon nanotubes (CNTs), fullerenes, graphene, and other novel components have brought considerable awareness from the scientific world, which, because to their idiosyncratic belongings, have been often dubbed “wonder materials” as a source of optimism for a wide range of potential uses (O'Brien et al., 2014). Carbon nanotubes (CNTs) are the most well-known type of nanomaterial that is based on carbon. Catalytic activity in organic chemical reactions has been shown to be significantly enhanced by carbon nanocomposites, which are generally metal nanostructures or carbon compounds comprised of graphene and carbon nanotubes and whose shape-dependent physical and chemical characteristics and thicknesses have led to their success (Zang et al., 2015). Industry and academics have taken notice of dye-sensitized solar cells (DSSCs), a novel form of solar cell based on nanocrystalline TiO2 electrodes (Ansari et al., 2021). The production costs of DSSCs are lower than those of commercial silicon-based solar cells while offering equivalent benefits to those of amorphous silicon solar cells. As a consequence of its high biocompatibility, the carbon cloth (CC) modified MWCNT-MnO2/PPy nanocomposite electrode has become a popular choice as a moderator for the generation of bioelectricity in wastewater using minimal amounts of MFCs (Fu et al., 2021b). Carbon nanotubes are categorized in Figure 3 depending on their structural configurations (Yuan et al., 2019).
Figure 3. Structure-based categorization of carbon nanotubes, reproduced with permission from reference (Yuan et al., 2019) ________________ Springer Nature.
Most catalytic reactions, including isomerization, alkylation, hydration, oxidation, halogenation, polymerization, and carbonylation can take place via thermal catalytic processes, that utilizes common sources such as light and heat to conduct reactions especially in heterogeneous catalysis (Li et al., 2020). Perhaps, self-heating photo-thermal impact, which may control the catalytic conversion through the thermochemical or photochemical route, is the basis of photo-thermal catalysis shown in Figure 4 (Mateo et al., 2021). The photo-thermal catalytic mechanism in the thermochemical pathway interacts with the incident light to absorb photon energy and subsequently dissipates as thermal energy (heat), which facilitates charge carriers mobility by enhancing the catalysis process. The photo-chemical route allow to generate photo-excited “hot” carriers, including electrons or holes, which are subsequently involved in catalytic processes (Wang et al., 2020a). It should be emphasized that occasionally, during a photo-thermal catalytic process, it might be challenging to completely separate these two entangled catalytic routes.
Figure 4. Classification and operating tenets of photo-thermal catalysis, reproduced with permission from reference (Song et al., 2022) ________________ Royal Society of Chemistry.
Consequently, a noteworthy aspect of photo-thermal catalysis is the ability to tailor the selective material employed towards certain desired products by combining thermal and photo-chemical pathways. Speaking in simple terms, photo-thermal catalysis may complete some “unattainable” chemical processes and produce desired products that are not achievable with thermally powered catalysis. Additionally, during the photo-thermal transformation process, heat may be generated locally surrounding active sites as a consequence of direct local heating at the nanoscale, eliminating the need to supply too much heat to warm up a whole reaction systems (Zhu et al., 2018a).
Light and heat can both play a role in photo-thermal catalysis, but they can also operate independently. Therefore, photo-thermal catalysis may be broken down into three distinct classes of reactions, distinguished by whether they include thermochemical or photochemical mechanisms. The first category involves a photocatalytic reaction where heat is used to speed up the process. The catalyst alone is insufficient for heat-driven reactions (Xu et al., 2019). Involvement of the photo-catalyst’s excited electronic state or hot carrier is possible. Adding heat helps reduce the apparent activating energy of photo-catalysis, which speeds up the reaction by increasing the ability to move and mass transfer rate of the photo-generated carriers. The second kind is thermal catalysis with photo assisted reaction, in which the thermochemical route serves as the primary mechanism of action (Zhang et al., 2016). In this scenario, the photo-catalyst’s ground electronic state plays a significant role in the reaction. By increasing the local temperature through the photo-thermal effect, light plays a role in stimulating vibrational modes, and a minor contributes to the photochemical process. Consequently, the process requires a photo-catalyst that can operate in an optimal temperature range and has a high capacity for light absorption (Feng et al., 2021). Photo driven thermos catalysis is the most severe example of this kind since light is exclusively used to supply heat. This subclass of photo-catalyst requires high levels of both light absorption capacity and light-to-thermal efficiency in the conversion process. By heating catalysts and reactants using the Sun’s rays, we may escape the normally severe conditions required for thermal reactions (Li et al., 2018).
This third category, known as photo-thermal co-catalysis, is the outcome of a synergistic effect between the thermochemical and photochemical reactions. The reaction cycle can be accelerated through the thermochemical route by the heat produced by the photo-thermal impact. The apparent activity is also greatly influenced by the photo-chemical impact, creating a synergistic influence triggered by the thermal and photo-chemical processes which is totally distinct from mere addition of two channels shown in Figure 5 (Song et al., 2020a). Excited vibrational modes of photo-catalysts (caused by thermal energy) may couple with excited electronic levels to catalyse the process.
Figure 5. Photo-thermal co-catalysis, reproduced with permission from reference (Fu et al., 2021b) ________________ ACS Publications.
Photo-thermal catalysis has grown rapidly over the past decade, and it has proven to be a more efficient and active catalyst than either traditional photocatalytic techniques or thermos-catalytic processes. In fact, the construction of effective photo-thermal catalysts has relied heavily on nanoscale materials and structural engineering. Nanomaterials’ size and quantum effects give them special optical and catalytic capabilities. The nanoscale scale enables the observation of novel features such as a variable effective refractive index, a semi-continuous band gap for semiconductors, and local field enhancement effects. Consequently, the nanomaterials can control the bandwidth and the local field light-heat transformation, all of which improve the effectiveness with which light is absorbed. Multiple additional heterogeneous catalytic processes have demonstrated size impacts, which may govern product activity or selectivity and even modify the entire catalytic process (Lin et al., 2019). While low-energy infrared light is typically squandered in traditional CO2 photo-catalysis, the photo-thermal route admits for more efficacious utilizations of the solar-spectrum (Wang et al., 2017).
Numerous attempts have been undertaken to create catalysts for photo-thermal CO2 transformations after the ground breaking work by (Meng et al., 2014). However, metal nanoparticles frequently have issues with insufficient use of photons over the whole solar spectrum. A number of techniques were devised to make the most of the Sun’s spectrum, such as the use of highly absorbent supporting and the development of plasmonic superstructures. Although there is still a great deal of work to be done to boost the activity of the resultant photo-thermal catalysts (Jelle et al., 2018). To improve the efficiency of photo-thermal CO2 catalysis, it is becoming increasingly important to investigate potential materials with outstanding photo-thermal characteristics. MXenes are a class of 2-dimensional transition-metal carbides and nitrides that have shown promise in a wide variety of fields, including energy conversion and storage, biomedicine, and wearable electronics, thanks to recent breakthroughs in the field of research. Here, we look at the feasibility of using MXene materials for photo-thermal CO2 catalysis because of their unique characteristics. In many cases, MXene materials (particularly carbides) have an advantageous photo-thermal conversion characteristic (Xu et al., 2020). Xiaohong Zhang and co-workers worked on Niobium and Titanium carbid3 based MXenes for photo-thermal supports for CO2 catalysis (Wu et al., 2021). They showed that Ni nanoparticles’ photo-thermal the catalytic function might be improved by combining them with Nb2C and Ti3C2, two common MXene materials. Under strong light, Ni nanoparticles anchored on Nb2C nano-sheets achieved great height of CO2 transformation rate around 8.50 mol.gNi−1.h−1 shown in Figure 6. This research not only stimulates the attention towards MXene based metal doped nanoparticles for various heterogeneous catalytic processes, but it also fills a gap among photo-thermal MXene materials and photo-thermal CO2 catalysis, paving the way for more effective solar-to-chemical energy conversions. Recent research has shown that in photo-thermal CO2 hydrogenation, Fe3C has more light absorption than Fe3O4 and greater selectivity toward CH4 than CO (Song et al., 2020b).
Figure 6. (A) Schematic diagram of the preparation of Nb2C MXene nanosheets, (B) XRD micrographs of the Nb2AlC precursor, (C) TEM, (D) AFM images obtained for Nb2C nanosheets, (E) CO2 is photo-thermally converted over time in a batch reactor using a few-layer Nb2C nano sheets, (F) The thermocatalytic performance graph of few-layer Nb2C under different temperature gradient conditions Point 2: MXene carrier improves the photothermal catalytic activity of nickel nanoparticles. Considering the excellent light absorption and photothermal conversion performance of Nb2C MXene, we tried to use it as a carrier to support Ni nanoparticles to build a photothermal catalyst. Under the same conditions, compared with Nb2O5 loaded Ni nanoparticles (Ni/Nb2O5), the photothermal catalytic activity of Nb2C loaded Ni nanoparticles (Ni/Nb2C) increased by 5.3 times reproduced with permission from reference (Wu et al., 2021) ACS Nano Publications.
Many scientists are currently focused on photo-thermal-derived applications in an attempt to broaden their scope and improve the conversion effectiveness of solar light energy (Tao et al., 2018). Since fresh water may be directly generated by solely utilizing solar light energy, and since it will be answer to freshwater scarcity dilemma for many countries, photo-thermal water evaporation for desalinization and water treatment has drawn greatest interest among all the uses (Wu et al., 2021; Cheng et al., 2021). In order to maximize the use of solar energy, more emphasis needs to be paid to research into alternative applications outside photo-thermal water evaporation. High concentrated Sun intensity allows for the use of photo-thermal catalysis for H2 production and CO2 reduction to transform solar energy into chemical energy; however, current efforts are insufficient. Under high concentration solar intensity, such as 38 Sun, photo-thermal electric power generation may exhibit a rate of conversion of solar to electricity greater than 7%; but, during low solar energy, like 1 Sun or nearly close circumstances, the process’s conversion efficiency stays very low (Kraemer et al., 2016). This highlights the need for future studies to take into account the trade-off between effectiveness, cost, and viability. Furthermore, photo-thermal bacterial killing technology has demonstrated its efficacy in killing bacteria in the presence of solar light irradiation; nevertheless, its potential for application in the treatment of wounds in humans and other animals remains debatable (Zou et al., 2021). Photo-thermal sensing relies on the reversible alteration of the physical characteristics of objects that are thermally sensitive to sunlight (Tian et al., 2021).
Solar power has the potential to displace traditional fossil fuels in a variety of energy-hungry settings due to its low environmental impact. Since few applications can make direct use of solar energy, it is often converted into other sources of energy. Photovoltaic modules, that utilize solar radiation to generate energy by separating charges at the semiconductor surface, are a prototypical implementation of this concept (Zhang et al., 2020). Light-to-heat conversion is a simple and effective method of harnessing solar energy, notwithstanding the low quality of heat as compared to electricity. As an example, the solar thermal collector is one of the most widely used photo-thermal systems with real-world applications. Steam created by solar heating may be utilized to spin a turbine, resulting in electric power generation via photo-thermoelectric transformation (Zhu et al., 2018b). In order to transform solar energy into usable heat for evaporation, photo-thermal materials are essential. The photo-thermal energy conversion technique is widely used in solar collectors because it has the best conversion rate. In order to increase the overall efficiency of solar thermal collecting devices, scientists have recently begun integrating nanotechnology to this field. Currently, nanoparticles increases the thermal characteristics of the functioning fluid associated with the solar-thermal-collector-system (STCS), hence increasing total thermal efficiency. The nanofluid receives its thermal energy through surface-absorbing-thermal-collector framework (SATCF), whereas thermal conducting surfaces will allow to efficient absorption of solar heat energy. According to the photo-thermal transformation of energy method is classified as plasmonic-localized-heating, absence of radiative relaxation observed in semiconductors, and thermal vibration observed in molecules relying on their matching light absorption spectrum (Gao et al., 2019). When metallic nanoparticles are bombarded with their resonance frequencies, the plasmonic photo-thermal energy conversion might be witnessed (as seen in Figure 7). Transmission is attenuated when photons or electromagnetic waves contact with a fluid containing nanoparticles because the nanoparticles absorb or scatter the photons or waves (Hogan et al., 2014). The percentage of light that is able to pass through a given sample of nanofluid is known as its optical transmittance. According to (Taylor et al., 2011), water is an excellent absorber and emitter at similar long wavelengths, making it an ideal base fluid. Vijayanandan et al. (2020) investigated optical as well as photo-thermal energy transformation of a Co3O4 nano-fluids produced by Aspergillus nidulans (endophytic fungus). Authors reported Rayleigh scattering occurred when light was shone on nanoparticles with radii lower than the incident wavelengths.
Figure 7. Various photo-thermal effect mechanisms and the wavelengths at which they are activated, reproduced with permission from reference (Gao et al., 2019) ________________ Royal Society of Chemistry.
The efficiency of photo-thermal conversion was investigated by Jia-Lin Sun et al. using CNT films. A bolometric photodetector based on a CNT film with the greatest photo-thermal conversion efficiency was made using this technology, and its high performance (2 A W−1 at 532 nm) verified the efficacy of the approach (Liu et al., 2022). Furthermore, Graphene nanocomposites have remarkable photocatalytic activity. Graphene-based nanocomposites are shown to have a vital role in the improved photocatalytic performance due to the photo-thermal effect. Author Xinglong Wu, prepared graphene based nanocomposites and verified the photocatalytic performance (Gan et al., 2014). They validated the enhanced photo-thermal performance of graphene-based nanocomposites as a viable technique to achieve highly efficient photocatalytic activity.
Nanoparticles made of precious metals including gold, silver, aluminum, and platinum are known as plasmonic nanoparticles because of the abundance of free electrons they contain (Wang et al., 2020). The wide cross section of absorption of nanoparticles and nanostructures facilitates their large-scale interaction with light. Therefore, significantly localized surface plasmon resonance effect metallic plasmonic nanoparticles are desirable for photo-thermal solar energy conversion. Zhou et al. described the fabrication of a self-assembled gold-plasmonic absorber using a physical-vapor-deposition (PVD) technique in an anodized aluminum oxide mask (Zhou et al., 2016). This plasmonic light absorber shows over 99% light absorption in the range from 400 nm to 10 m, turning the previously transparent AAO mask black and allowing for potent photo-thermal conversion. As a consequence, the gold plasmonic light absorber allows solar steam production efficiencies greater than 90% at Sun intensities of 4 kW m−2. Nevertheless, gold is a noble metal, and its high cost makes it impractical for use in solar steam generators. In order to reduce the cost of the light absorber, Xu et al. created a nickel (Ni) nanostructure (Xu et al., 2019). The plasmonic light absorber has been shown to have an absorption efficiency of up to ≈95% from 200 to 1,500 nm, making it ideal for usage in solar steam generators. The evaporation rate was increased by an astounding ≈2.3 times when the Ni-plasmonic absorption was used for solar-steam production instead of control experiment.
Some small organic molecules and polymers have ability to convert photon energy to heat energy via lattice relaxation (Huang et al., 2022). Such organic compounds mechanisms resemble semiconductors’ in terms of operation. In the solid state, the organic-small-molecule photo-thermal material (CR-TPE-T) developed by Chen et al. exhibited robust—stacking and exhibited broadband absorption from 300 to 1,600 nm (Chen et al., 2020). Through lattice relaxation, the substances performed admirably in a photo-thermal energy conversion experiment employing a laser with an 808 nm wavelength and an intensity of 800 mW cm−1. Additionally, under 1 Sun’s intensity, a solar steam evaporator using CR-TPE-T with porous polyurethane demonstrated an impressive solar-to-vapor efficiency of 87.2%. Double-shell textiles made of polypropylene, polyethylene dioxythiophene, and polypyrrole (PP-PEDOT-PPy) were created by Zhang et al., for the purpose of solar and thermal energy capturing (Zhang et al., 2020). The flexible photo-thermoelectric gadget was able to generate 536.47 µV of voltage when exposed to infrared light. All of this demonstrates a possibility for a source of energy in intelligent wearable electronics.
It is expected that the use of H2, a clean energy source that produces no polluting gases or “greenhouse” gases, will have a positive effect on the environment. Hydrogen is the most plentiful element, however unlike oxygen, it is not found in the form of free molecules. Hydrogen gas has a high energy density (143 MJ kg−1) and produces no harmful by-products when burned (Voiry et al., 2018). This highlights the critical need for a fast and effective photocatalytic hydrogen generation technology. Particle photo-catalysis, photovoltaic-assisted electrolysis, and photo-electrochemical cells are the three most common solar-powered hydrogen generation technologies (Chen et al., 2017), where it is expected that the cost-effectiveness of particulate photo-catalysis will exceed that of the other two systems. Using solar energy and the right catalysts, a process called photo-thermal catalysis may efficiently create hydrogen gas (H2). While particle photo-catalysis systems have shown promise, they still have a long way to go before they can efficiently produce hydrogen from water. Author Shaohui Guo et al. researched on photocatalytic triggered hydrogen generation from water via photo-thermally induced biphase models (Guo et al., 2021). In order to produce water steam by photo-thermal transpiration, the authors of this study expertly designed and executed A photo-thermal-photocatalytic system using natural wood under the light illumination simulated by a solar simulator at AM 1.5 G illumination (100 mW cm−2). A wood slice was cut from a tree perpendicular to its progress direction and served as a base for the photocatalytic reaction, with the surface of the wood slice being carbonized through a simple heating procedure to increase the steam generation effectiveness from solar energy from 46.7 percent to 90.5% shown in Figure 8A. To create the wood/photo-catalyst photo-thermal-photocatalytic system, CoO nanoparticles (NPs), a common photo-catalyst, were spin-coated on carbonized wood slices. The uniform CoO NPs have a size of 50 nm 5 nm, and the 0.24 nm d-spaced CoO lattice fringes are located on the (111) lattice planes. CoO NPs have a peak in their light absorption at around 550 nm. Raman spectra taken at varying distances from the top reveal that the CoO NPs are spread out along the walls of the wood microchannels by a factor of around 2 mm (Figure 8B). Raman spectra collected at a depth of 500 m reveal the typical CoO Raman peaks at 473.6 and 540.9 cm−1. Although the wood/CoO system floats on water, the photocatalysts are not completely submerged since the wood only submerges to a depth of around 2 mm (Figure 8C). By covering the wood with CoO NPs, as shown in Figure 8D, the wood/CoO system exhibits strong light absorption from 300 to 1,000 nm, suggesting that the wood/CoO system may efficiently harness solar energy. The photocatalysts adhering to the wood surface get blanketed in steam from photothermal transpiration within the wood when the surface temperature of the wood/CoO system rises to around 325 K (Figure 8E). It is worth noting that the potential energy surface gives a local temperature estimate of 346 K for the CoO NP. Considering the potential, we may infer that the local temperature of the CoO NP is 346 K, which is greater than the global temperature (325 K, in Figure 8F) due to the nanoscale effect. The effectiveness of the photocatalytic process may be improved, it is hypothesized, by increasing the temperature locally.
Figure 8. Biphase photo-thermal-photocatalytic system for wood and catalyst, (A) Diagram showing how the wood/photo-catalyst complex was made. It produces steam from water and catalyses its splitting to produce hydrogen, (B) Raman spectra recorded every 500 μm along the cross section of wood/CoO microchannels, (C) Image of a wood and CoO structure suspended in liquid, (D) Wood and wood/CoO reflectance spectra, (E) Light-illuminated thermal infrared picture of a wood/CoO system, (F) Wood/CoO’s potential when exposed to 100 mW cm−2, (G) CoO NPs’ calculated local temperature from the observed potential, reproduced with permission from reference (Guo et al., 2021) ________________ Nature Communications.
To facilitate the photocatalytic and photo-thermo-catalytic H2O splitting process when exposed to full-spectrum light, Zebao Rui et al. produced Pt nanoparticles (NP) supported by indium zinc sulphide (or ZnIn2S4). Without any additional heat source, a photo-thermo-catalytic environment was created on the Pt/ZnIn2S4 surface thanks to the infrared photo-thermal effect and localized surface plasma resonance (LSPR) impact of Pt nanoparticles. Accelerating transfer, separation, and redox reactions of photo-generated charges is made possible by the infrared photo-thermal influence as well as plasmon-thermal impact when the reaction proceeds, which together raises the surface temperature of the Pt/ZnIn2S4 compound as high as −45°C. Apart from this when 1% Pt/ZnIn2S4 taken which showed nearly twice increased value compared to the typical photo-catalytic procedure, photo-thermal interactive/synergetic catalytic H2 generation with an highest H2 production observed (19.4 mmol/g/h) shown in Figure 9) (Guo et al., 2022). Octahedral-shaped nanoparticle Cu2O/TiO2 heterojunction photo-catalysts achieved remarkable photocatalytic hydrogen production capability, 24.83 mmol g−1 h−1, via water splitting (Li et al., 2019). The maximum hydrogen yield was achieved with a catalyst containing 1.9% copper, which is over three orders of magnitude more than that achieved with pure TiO2 (0.03 mmol g−1 h−1). The inclusion of Cu2O broadens the light absorption from the ultraviolet area to roughly 515 nm, which, according to the authors, improves the photocatalytic efficacy of the TiO2 catalyst. The authors performed 10 cycles of reuse on the most effective catalyst to demonstrate its excellent photocatalytic stability. In addition, XRD examination showed no discernible changes in the structure of the employed catalysts after 10 h of operation, indicating their strong chemical stability. Another impressive experimental accomplishment is described in, where hydrothermal preparation of plasmonic-Au@ZnxCd1-xS photo-catalyst is described (Yu et al., 2019).
Figure 9. Charge separation and hydrogen generation in Pt/ZnIn2S4 under full spectrum light irradiation are depicted schematically, reproduced with permission from reference (Guo et al., 2022) ________________ Elsevier Publications.
In the realm of carbon dioxide (CO2) conversion, photo-thermal catalysis has garnered a lot of interest as novel catalytic approaches and a present attention focus of photo-thermal assisted research. The term “photo-thermal catalytic CO2 reduction” refers to a process in which photons are used to boost temperatures during thermos-catalysis. Carbon dioxide (CO2) content associated with the air has grown over the past few decades due to increased worldwide usage of fossil fuels, which has resulted in a number of environmental issues, including global warming and severe weather (Li et al., 2022). In order to reach carbon emission peak and carbon neutrality, it is crucial that carbon dioxide be converted into usable fuels or chemical feedstocks. Sustainable and ecologically friendly energy for human production and life may be obtained through the collection and conversion of solar energy. In addition to helping reduce global CO2 emissions, which are having a negative impact on the climate, the photo-catalytic transformation of CO2-H2O mixtures to affordable, high value and valuable compounds like fuels is an extremely assuring source for such chemicals (Yu et al., 2020). To solve the aforementioned problem, researchers have been shifting their attention to photo-thermal catalysis techniques, which utilise the energy from incoming photons to produce high temperatures in the catalyst (Zhang et al., 2021). The establishment of several TMOs, metal nanomaterials, and composites of the 2 has validated possibility of such methods, and the higher level temperatures associated in the catalyst resulting from above have shown to substantially boost the CO2 conversion value by 1–3 requests of magnitude (Cai et al., 2021). Additionally, it has been shown that the composites’ optimum choice of the TMOs and metal doped nanomaterials increased photo-thermal efficiency, catalytic selection along with C-C coupling capability of photo-thermal-catalysts (Li et al., 2021b). Such early investigations have made a lot of significant advancements. However, research on photo-thermal catalysts for turning CO2 into liquid alcohol is still in its early stages. Numerous layered inorganic compounds are being investigated as photo-catalysts at the moment including Bi2O2CO3, Mg-Fe-Al-LDH, Bi2MoO6, Ni-Al-LDH, PbBiO2Br, BiMO8X (M = Nb, Ta, Cl, Br, I), BiOX (X = halogens), LiFeO2 (Huo et al., 2020; Liu et al., 2020; Li et al., 2021b; Chen et al., 2021; Li et al., 2021c; Huo et al., 2021). There, a few stacked semiconductor oxide photo-catalysts have come under more and more scrutiny for potential application as photo-thermal catalysts. It is often accomplished by forming heterojunctions by adding metal doped nanomaterial substances to semiconducting oxide substrates. The surface-plasmon-resonance (SPR) influence of metal doped nanomaterials when exposed to light makes these heterojunction topologies very well-suited to producing higher photon to thermal conversion (Luo et al., 2021b). Moreover, “greenhouse” effect has lately inspired a new type of nanostructure for a supra-photo-thermal catalyst, which Cai and his team have disclosed (Al-Ashouri et al., 2020). The produced photo-thermal catalyst outperformed conventional methods of CO2 reduction. It was believed that the catalyst’s inclusion of nickel nanocrystals enveloped in porous silica (Ni@p-SiO2) would be useful in the process of CO2 reduction. When exposed to 2.8 W cm−2 of light, the Ni@p-SiO2 catalyst outperforms the other two in terms of photo-thermal activity. According to the researchers, silica’s superior heat insulation and infrared shielding effect are responsible for its impressive supra-photo-thermal CO2 reduction efficiency. Therefore, under 2.8 W cm-2 lighting, the Ni@p-SiO2 PTC is capable of converting CO2 at a rate of up to 0.344 mol g−1 min−1. In addition to its high CO selectivity of up to 90% and good stability after a decade of cycling, the device also functioned well. By using a simple reduction procedure and carbonization treatment on Fe3O4 as a precursor, Song and co-workers demonstrated a variety of Fe based PTC (Fe, Fe3C, Fe3O4, C@Fe3C, and Fe@Fe3C) (Song et al., 2020c). After 30 min of exposure to 2.05 W cm−2 of light, Fe3C surface temperature increased to 310°C, whereas Fe3O4 had reached 350°C. Intriguingly, Fe3O4 displayed nearly 100% selectivity for CO production over the entire 12 h of photo-thermal catalysis, whereas Fe3C’s selectivity for hydrocarbons formation was raised from ≈38% to 97.5% over the course of many years.
Researchers Fu et al., used a broadband Rh/Al nano antenna photo-thermal catalyst and highly focused solar radiation to reduce CO2 to CH4. Rh/Al crystal structure was seen after the catalyst was synthesized using a straightforward solvothermal technique. Light-induced thermo-catalysis under varying Sun intensities at 50°C, CO2 methanation may exhibit selectivity of about 100% (Fu et al., 2021a). The Reaction of Light and Heat Selectivity for CO2 methanation at 50 °C might reach almost 100% depending on the Sun intensity. Furthermore, photo-thermal CO2 catalysis, the group led by Zhang et al. presented a silicon nanowire array (SNA@Co@SiO2) that absorbed nearly all of the Sun’s light (Zhang et al., 2021). Under 25 kW m−2 illumination, the highest surface temperature of 250°C was achieved by SNAs@Co 5 min, thus demonstrating the best photo-thermal conversion capability. The experimental findings showed that the selectivity for CO generation was somewhat reduced while the stability of SNA@Co@SiO2 was improved. Changhua Wang et al. conducted research on photo-thermal catalytic reduction of CO2. Using thermally coupled photo-catalysis, the author hydrogenated ST-01 TiO2 and found it to have improved catalytic activity (Li et al., 2020). Dequan Xiao and co-workers studied photo-thermal transformation of CO2 using tuneable property using Fe-based catalysts. They confirmed that absence of thermal effects has crucial role to enhance catalytic efficiency of Fe-based catalysts during photo-thermal transformation of CO2 (Song et al., 2020). The detailed review on reduction of CO2 as hydrocarbons solar-fuels via synergistic PTC was done by (Tian and Guo, 2022).
One of the greatest problems in the chemical world in recent years has been the development of innovative catalytic methods for methane conversion to improved fuels and commercial chemicals under gentler circumstances. Methane’s unique chemical structure makes it a very stable and innocuous molecule (Olivos-Suarez et al., 2016). Several low-temperature methane conversion methods have been put into use so far. Several benefits may be gained from converting CH4 using solar energy. To begin, solar energy is an alternative energy source for methane conversion, which may cut down on both fossil fuel use and carbon dioxide emissions (Chen et al., 2018). Second, chemical obstacles (such C-H bond activation) may be readily surmounted by employing photo-energy and suitable photo-catalysts, allowing methane conversion to be accomplished at lower temperatures or even room temperature. Third, reducing the temperature of the reaction would have several positive effects on the environment and the procedure, including a greater availability of catalysts, less stringent stability standards for the catalysts, a decreased cost for the reactor, and increased safety.
In photo-thermal catalysis for methane conversion, metals are the most researched and widely utilized catalysts because of their inherent characteristics for selectively and effectively catalysing a wide range of chemical processes (Zhang et al., 2017). Moreover, several metal nanostructures (including Cu, Ag, Au, Rh, and Pt) demonstrate significant and tuneable light absorption capabilities via the well-known LSPR (Linic et al., 2011). In photo-thermo-catalytic processes, plasmonic metal nanoparticles are often utilized as catalytically active sites and/or light-absorbing materials, wherein traditional thermos-catalytic processes can occur without heat energy input or at lower reaction temperatures, resulting in decreased energy consumption, prolonged catalyst stability, and lower activation energy. By combining Au nanoparticles and ZnO with exposed (001) facets, Long et al. were able to create a photo-catalyst with a high Non-Oxidative Coupling of Methane (NOCM) conversion rate when subjected to artificial solar radiation (Meng et al., 2018). After 4 h of exposure to sunlight, the optimized Au/ZnO photo-catalyst produced C2H6 at a rate of about 11 μmol g−1 h−1 and evolved H2 at a rate of about 10 mmol g−1 h−1. According to the investigators, ZnO’s inherent electric field and high degree of polarization are crucial to its photocatalytic NOCM performance (Jelle et al., 2018). It is claimed here that CO2 may be photo-methanated over extensively distributed nanostructured RuO2 catalysts on 3D silicon photonic crystal supports at ambient temperatures and high-intensity solar-simulated irradiation, with conversion rates as high as 4.4 mmol gcat−1 h−1. Photocatalytic Methane (CH4) manufacture functioning ability observed for TiO2 PCs compound is comparatively larger than commercial available TiO2 as well as TiO2 nanotubes. This might be due to their outstanding physicochemical characteristic and excellent light consumption (Low et al., 2018). The photo conversion applications of this photo-thermal phenomenon, which originates from the special structure of photonic crystals, are extremely promising. For the visible-light-driven photo-thermal conversion of CO2 to methan, Jorge Gascon et al. created nickel nanoparticles mounted on Barium Titanate (Mateo et al., 2020). They found that the photo-catalyst’s extraordinary catalytic activity results from a confluence of elements, including efficient use of light and outstanding photo-thermal performance. Prepared the Rh/TiO2 catalysts for methane activation using visible light under mild conditions (Hui et al., 2018). To generate syngas from concentrated solar energy, Ying Li et al. presented a novel method called photo-thermal chemical carbon dioxide (CO2) (dry) reformation of CH4 (PTC-DRM) (Pan et al., 2017). By incorporating noble metals (Rh, Pd, Ru, and Pt) into a molecular sieve, Miyauchi et al. showed that methane could be oxidized more efficiently (POM) to create syngas under UV irradiation (Jiang et al., 2019).
Over the last few decades, pollution including organic pollutants, heavy metals, inorganic compounds, and many more complex substances has been identified in surface, ground, sewage, and drinking water supplies everywhere (Gómez-Pastora et al., 2017). One of the main classes of pollutants discharged into water from industrial production, agricultural activity, and other human activities is refractory organic contaminants, which contribute to the aforementioned contamination (Moradi et al., 2020). Additionally, saline organic wastewater (SOW), such as wastewater from dyeing, is typically harder to manage and will pollute the environment more than regular wastewater. Several methods are used to remove contaminants from wastewater, the most common of which being electrodialysis, membrane filtration, precipitation, adsorption, electrochemical reduction and electrodeionization. Above said wastewater treatment operations often need a great deal of energy and can get more difficult as contaminants are transferred between fluids, wastes, and by-products. Finding more gentle reaction conditions and efficient catalysts to remove different contaminants from wastewater is vital for economic and social growth (Li et al., 2019). In adequate conditions, the simple process and environmentally friendly technique known as photo-catalysis may convert the organic contaminants found in wastewater into innocuous compounds like water, carbon dioxide, or other tiny molecules through oxidation. However, photo-thermal catalysis often results in self-etching of the catalyst due to the inherent instability of inactive photo-catalysts.
Xiaoyong, studied photo-thermal synergy to boost photo-fenton activity with rGO-ZnFe2O4 and tried to elaborate photo-activation process and mechanism towards environment treatment (Yang et al., 2021). In order to achieve photocatalytic goals in the environmental field, it is necessary to combine the photo-Fenton effect with the photo-thermal effect. A probable photo-thermal Fenton degradation pathway of 7%rGO-ZnFe2O4 toward refractory CIP was postulated based on the previous data and discussions, and is depicted in Figure 10A.
Figure 10. Degradation of CIP by a photo-thermal Fenton process on rGO/ZnFe2O4, reproduced with permission from reference (Yang et al., 2021) ________________ Elsevier Publications.
Minjia Meng et al. were able to create a foam with a high evaporation rate of 2.63 kg m2 h1 at one Sun’s worth of illumination via in situ development of a rough CoNi2S4 nano needle array in a PVDF framework (SCoNi-pDA/PVDF foam) (Figure 11) (Li et al., 2021). In order to convert CO2 to CO, Deren Yang et al. presented a method for upcycling waste Ni from electroplating waste water into a photo-thermal catalyst (Wang et al., 2022).
Figure 11. (A) A schematic depicting the steps required to fabricate SCoNi-pDA/PVDF foams, (B) Foams and their size, (C) cut foams, (D) foams with different shapes, (E, F) SEM micrographs SCoNi-pDA/PVDF, (G, H) TEM image and its diagram, (I) elemental mapping, reproduced with permission from reference (Li et al., 2021) ________________ Royal Society of Chemistry Publications.
Although they play a vital role in metabolism, heavy metal ions become hazardous at extremely high quantities. Large quantities of poisonous heavy metal ions are created with the development of metallurgy, mining, nuclear energy, and chemical manufacture, posing a serious danger to both surface and subsurface water supplies. Heavy metal ions are lethal to living things because they attach to nucleic acids, proteins, and tiny metabolites, which they then use to degrade organic cells. Heavy metal ions cannot be broken down by living organisms, hence they will accumulate in humans and other animals through their consumption of contaminated food and water. Therefore, before releasing wastewater into the ecosystem, it is required to remove potentially harmful heavy metals, such as Cr, Hg, Cd, Ni, Zn, and Mn ions. Toxic high-valence heavy metal ions in water can be removed by photo-catalysis by being converted into less dangerous low-valence ions or non-existent metals. Chromium in water has been treated using photo-catalysis. Heavy metal ions may be removed using TiO2-ZrO2, which displays strong chemical stability and great sorption properties. The high removal rates of Cr (VI) (100%) and Cu(II) (91%) after 4 cycles of use are consistent with reports that this material may degrade both of these elements quickly in a single step (Yan et al., 2020). The removal rate for Cr (VI) was 98.7% when using the organic-inorganic hybrid PW12/CN@Bi2WO6 composite, which showed increased photon absorbance and facilitated charge transfer (Yang et al., 2020). In order to achieve 94% efficiency in the photocatalytic elimination of hexavalent Chromium (VI), Zhang et al. employed nanocomposites made from freeze-dried carbon quantum dots and CdS nano sheet precursors (Zhang et al., 2020). For Cr(VI) elimination, the ZnIn2S4/CdS heterostructure showed both enhanced visible photocatalytic activity and endurance (Zhang et al., 2018). The decrease of chromium (VI) across a 3-dimensional ZnIn2S4.CdS compound when illuminated by visible-light is seen schematically in Figure 12.
Figure 12. A schematic of the reaction pathway for the photocatalytic reduction of Cr(VI) over a 3D ZnIn2S4 CdS composite, reproduced with permission from reference (Zhang et al., 2018) ________________ Elsevier Publications.
The superparamagnetic NiFe2O4-Pd nanohybrid has been developed effectively by Alexander’s group. When compared to bare NiFe2O4, the removal efficiencies of NiFe2O4-Pd against Pb2+ and Cd2+ ions were 98% and 97%, respectively (Thomas and Alexander, 2020). Author Kanakaraju et al. used multifunctional TiO2/Alg/FeNPs magnetic beads as catalyst to removal of mixed heavy metals, including Cr(III), Cu(II), and Pb(II) ions, and observed nearly complete at removal (>98.4%) for all three ions within 72 min (Kanakaraju et al., 2019). Mesoporous -Fe2O3/g-C3N4 nanocomposites have shown 4.6- and 6.8-fold increases in photo-catalysis activity over pure -Fe2O3 NPs and g-C3N4 nano sheets, respectively, making them a promising candidate for Hg (II) photo-catalysis applications (Kadi et al., 2020). For the effective treatment of sewage, Isari et al. synthesized N-Cu co-doped TiO2@CNTs and coupled it with visible light and ultrasonic radiography (Isari et al., 2020). Sulfamethoxazole, chemical oxygen demand (COD), and total organic carbon elimination efficiencies of 100%, 93%, and 89% were achieved under ideal circumstances. Kim et al. pretreated wastewater with a Co-doped BiVO4 method. This technique can be used to kill Escherichia coli (81.3%, 5 h) and Chlamydomonas pulsatilla (65.6%, 1 h) (Regmi et al., 2017). Under visible light irradiation Si et al. that, g-C3N4@Co-TiO2 nano-fibrous was shown to photo-catalytically inactivate E. coli (Sun et al., 2021). After 90 min, the number of bacterial cells had decreased by 6 logs, indicating that E. coli had been inactivated.
Organic contaminants in water come in a wide variety of forms (Song et al., 2020). Dye chemicals, phenolic compounds, surfactants, organohalides, hydrocarbons, plasticizers, and so on are all examples of pollutants. These organic contaminants are difficult to break down in water, are chemically stable, poisonous, and in some cases carcinogenic. The breakdown of harmful organic compounds is one area where catalytic technology shows great promise for use in environmental clean-up (Zhang et al., 2018). In addition to their widespread usage in manufacturing, construction, and vehicle emissions, volatile organic compounds (VOCs) are also a major contributor to air pollution. The impacts of volatile organic compounds (VOCs) on the environment and biology are often permanent, including teratogenicity, carcinogenicity, and mutagenicity. Presented evidence for the production of ZnFe2O4 and its use as a photo-thermal catalytic material (Sun et al., 2021). Thermal catalytic performance of oxygen vacancy-rich ZnFe2O4 driven by high-intensity light is high and steady, as demonstrated by degradation of the gaseous organic pollutant isopropanol, not only that, but there is a noticeable improvement. Photo-thermal synergistic catalysis with BiVO4/TiO2 was utilized by Fang et al. to convert benzene fully at low temperatures (Fang et al., 2017). Although potent metal based fundamental interaction in reducing oxide triggered novel metal-nanoparticles (NPs) which is well acknowledged in thermos-catalytic models, its role in photo-catalytic as well in photo-thermo-catalytic modes has received much less attention.
Prepared Au–Pd/g-C3N4 nano-photo-catalyst through low-ramping pyrolysis in an argon atmosphere (Hosseini et al., 2018). The outcomes showed that the Au-Pd-containing catalyst outperformed its monometallic nanoparticle-containing competitors in terms of performance. The Au-Pd/g-C3N4 photo-catalyst demonstrated benzene conversion of 26% at a phenol selectivity of 100% while producing no dihydroxylated by-products under optimal circumstances. As a result of the catalyst’s great stability and recyclability, straight benzene to phenol conversion appeared to be a promising application. Dandan Zheng and co-worker investigated the very hard metal based support influence of Pt/g-C3N4 photo-catalyst to enhance photo-thermal interactive wearing of Benzene (Huang et al., 2023). The experimental results obtained for Pt/g-C3N4 were shown in Figure 13, synthesized the copper modified graphite carbon nitride nano-sheets (Cu-g-C3N4) for hydroxylation of benzene at mild reaction conditions using H2O2 as a green oxidant. The catalyst’s performance is remarkable; 74.1% of the benzene is converted into phenol and only p-benzoquinone is produced as a by-product. After being recycled many times, the catalyst showed no discernible loss of phenol selectivity (Muniandya et al., 2017).
Figure 13. (A) Rate of Conversion, (B) At 150°C and solar light condition total rate of mineralization observed in benzene by PUR, PMA PCA and PDA, (C) rate of conversion, (D) only under heat, visible-light photo-thermal conditions rate of mineralization rate of benzene for PDA, (E) conversion speed and mineralization of benzene for PDA under solar light photo-thermal conditions, (F) 4 cycle experimental rate of conversion notice for PDA under solar-light medium (at 162°C), reproduced with permission from reference (Huang et al., 2023) ________________ MDPI Publications.
Another group Ping Yang et al., used a two-step thermal polymerization procedure to incorporate Copper clusters inculcated in graphitic-carbon-nitride (g-C3N4), creating an outstanding thin black coloured g-C3N4 nano-sheets cantered copper-cluster-composite (Cu-g-C3N4) exhibiting high influenced transformation of charge features as well as improved photo-catalytic capacity. In addition, when using visible light to drive benzene oxidation, an excellent conversion rate of 90.4% and good selectivity to phenol (99.1%) are produced (Zhang et al., 2022). In order to make ZnO/g-C3N4 composites, Majeda Khraisheh and co-workers utilized a one-pot paralysis method to fuse hexagonal ZnO lotus buds with graphitic carbon nitride (g-C3N4) nano-sheets. The Z-scheme was used to describe the combination photo-catalyst and degradation process that was presented (Mamari et al., 2023).
Oil is a crucial component in the industrial creation of humans. Over the course of the last several decades, there have been numerous crude oil spills, which have resulted in serious harm to the marine ecosystem as well as tremendous economic loss. In order to lessen the negative impact that oil spills have on the surrounding ecosystem, a speedy clean-up method that relies on absorption materials has been created. Oil spills have consequences that persist for generations on the environment. Not only do the many poisonous components of hydrocarbons pose a hazard to marine life, but also to human people. Also Heavy crude oils may be discovered and extracted in a large number of locations in more than 70 nations, and the total amount of heavy oil resources is estimated to be 5.6 trillion barrels throughout the globe (Hein and Pet, 2016). In addition, the oil sector is being forced to boost its extraction of heavy oils as a result of the falling output of medium and light crude oil. The petrochemical sector is also responsible for the huge production of heavy oil in the form of heavy fuel oil and bitumen, which are respectively used in the transportation and construction industries (Martinez-Palou et al., 2011). In addition, because of the quickening pace of industrialization and the growing number of people who use an excessive amount of oil, the number of mishaps involving oil spills that occur during offshore oil extraction and transportation has skyrocketed in recent years. The prevalence of such behaviour has not only posed a risk to the continued existence of marine life and human health, but it has also led to catastrophic harm to resources (Cally, 2018). Perhaps, marine oil pollution is caused by a variety of human activities, including shipping, unlawful bilge water discharges, surface runoff, and accidents involving vessels and pipelines, as well as offshore petroleum exploration and production activities (Kostianoy et al., 2018). Traditional clean-up methods for oil spills have focused on oil adsorption. Clean and efficient technique for desalination and purification of polluted water, solar-driven evaporation of water by heat localization at the surface has been used (Chen et al., 2019). Highly effective solar-driven evaporation relies on the development of photo-thermal materials that can regulate water supply, steam escape, and thermal insulation in addition to efficiently absorbing solar light and converting it to thermal energy (Lin et al., 2019). Metal nanostructures that have a surface plasmonic effect and carbon nanomaterials that have a black-body effect are the types of materials that have been utilized most frequently for photo-thermal applications (Liu et al., 2018).
As an alternative to using electricity, photo-thermal materials can be used to heat crude oil. Using polyurethane with carbon nanotube (CNT) modifications, Chang et al. produced a photo-thermal sponge (Chang et al., 2018). In just 6 min of exposure to 1 Sun, the sponge’s surface temperature increased to roughly 40°C, and the viscosity of crude oil dropped by a factor of two, to 4,100 mPa s. Hu’s team also presented research showing how hydrophobic carbonized wood with aligned channels can rapidly absorb crude oil. Oil absorption rates of up to 1,550 mL/m2 in 30 s under 1 Sun illumination have been achieved because to the combination of straight pores and effective light-to-heat conversion (Kuang et al., 2019). A self-pumping photo-thermal oil-collection system was created by Wu et al. that makes use of the capillary and siphonic effects shown in Figure 14 (Wu et al., 2019). Kyubock Lee et al. proposed a new idea for effective oil-spill restoration using solar-driven evaporation of light oil components and simultaneous adsorption of heavy oil components (Ku et al., 2021).
Figure 14. Solar-heating siphon-capillary oil skimmer, reproduced with permission from reference (Wu et al., 2019) ________________ ACS Nano Publications.
In order to create a photo-thermal sorbent, Peng Wang et al. modified a polyurethane sponge using carbon nanotubes (CNTs). This sponge demonstrated super hydrophobicity, super oleophilicity, and an exceptional ability to absorb heavy oil (Chang et al., 2018). Gong et al. combined Joule heating and solar heating into one sponge-based device to increase viscosity reduction synergistically and function in all weather conditions. They used conductive Ti3C2TX MXene as the photo-thermal material (Gong et al., 2020). In addition, Yang’s team developed a Ti3C2Tx/cellulose membrane by using the dip-coating technique and investigated the material’s photo-thermal seawater evaporation capabilities. The MXenes/cellulose membrane’s ability to maximize evaporation efficiency by up to 85.6% with only a single solar irradiation is significant (Zha et al., 2019). The MXenes/cellulose membranes also showed excellent durability in the face of ultrasonic treatment and vigorous mechanical agitation.
Polysodium methacrylate (pNaMMA) was UV-initially crosslinked by Ding et al., who later reported that Na+ ions were substituted with Ag+ and the material was photo-thermally in situ reduced to silver nanoparticles (AgNPs) (Ding et al., 2019). Sun et al. created graphene aerogel-CuFeSe2 (GA-CuFeSe2) by assembling CuFeSe2 nanoparticles made by wet chemistry with graphene aerogels (Sun et al., 2020). At a certain wavelength (808 nm), it possesses a high adsorption capacity and an extremely fast adsorption rate. Niu et al. implanted melamine sponges (MSs) with CuS nanoparticles (Niu et al., 2021). By employing an aqueous deposition approach to layer PDA and polydimethylsiloxane, created a self-heating hydrophobic/lipophilic sponge while taking use of the photo-thermal conversion effect of PDA coating (Zhang et al., 2018; Zhu et al., 2018a) created a polydimethylsiloxane (PDMS)/polyaniline (PANI)-modified MS using straightforward polymerization and dip coating. In just 2 minutes, the special photo-thermal coating causes the surface equilibrium temperature to quickly increase to 81.80°C, demonstrating an outstanding adsorption capacity of 1.17 × 106 g/m3 (Li et al., 2021). By using the PPy deposition approach, Zeng and his colleagues were able to create a super hydrophobic cotton fabric that also had a photo-thermal conversion effect (Zeng et al., 2022).
Pharmaceutical compounds (PCs) and other developing pollutants have been under increased scrutiny in recent years because of their detrimental effects on the environment (Kay et al., 2017). Reverse osmosis, flocculation, air stripping, and other traditional treatment techniques have limitations when it comes to treating such chemicals. Heterogeneous photo-catalysis is one of the procedures that is shown to be the most effective at removing harmful chemicals, such as antibiotics (Verma and Haritash, 2020).
In the treatment of non-insulin-dependent diabetes, also known as type 2 diabetes, metformin is the most widely used hypoglycemic medicine among lipid regulators. The human body does not digest metformin, therefore it is eliminated entirely after administration. Polluting marine environments, these chemicals reach water supplies from many different entry points. The photocatalytic degradation of amoxicillin and metformin using titanium dioxide was investigated by Chinnaiyan and co-workers (Chinnaiyan et al., 2018). Photocatalysis has been shown to be a successful method for removing metformin from water by Carbuloni et al. (2020), who employed TiO2 and a synthetic TiO2-ZrO2 catalyst to remove the drug from sewage. The maximum removal rates for amoxicillin (90%) and metformin (98%) were seen at a pH of 7.6, a TiO2 concentration of 563 mg/L, an initial pollutant concentration of 10 mg/L, and a reaction duration of 150 min.
Antibiotics are a growing concern for PCs because of their potential impact on aquatic ecosystems and on human health. Antibiotics in large quantities are a major contributor to the degradation of water quality that results from the discharge of sewage and animal waste. Consequently, investigating efficient means of removing these chemicals from wastewater is of utmost importance. Tetracycline (TC) is a widely used antibiotic and one of the most important medicinal chemicals. There is widespread evidence that tetracycline, which is present in soil, groundwater, surface water, and even drinking water, contributes to the development of antibiotic-resistant genes and eco-toxicity in aquatic systems (Wang et al., 2020). There is concern that it could pose a threat to aquatic ecosystems and human health. Bare TiO2 and multiple CuO(x)-TiO2/MCM-41 nanocomposites of varying CuO concentrations were produced using the hydrothermal/impregnation approach by Morteza et al. (Khanmohammadi et al., 2021). These catalysts were employed to break down TC in the presence of UV radiation. It was mentioned that h+ can oxidize TC directly, but that O2− and OH were more efficient oxidizers. In order to oxidize the pharmaceutical molecule levofloxacin under visible light, the MoS2/Ag2Mo2O7 photocatalyst was produced by (Adhikari et al., 2019). Incorporating 30 wt.% MoS2/Ag2Mo2O7 resulted in increased catalytic activity (efficiency of 97%) and excellent stability. This is due to the heterojunction structure’s ability to significantly enhance light absorption, electron-hole separation, and the efficiency of charge transfer across interfaces to adsorption substrates (Ji et al., 2020). Similarly, by creating various graphene oxide loaded Ag3PO4/GO film catalysts, Yang’s research team discovered a solution to the recycling problem. The breakdown rate of norfloxacin was approximately 83.68% in 100 min, and the reaction rate constant k was 1.9 times that of pure Ag3PO4 (Kay et al., 2017).
Pesticides are used to control growth, defoliate, desiccate, thin fruit, regulate ripening, and guard against degradation during storage or transportation. Pesticides, however, are also one of the main causes of water contamination. All pesticides are cancer-causing and have negative side effects (Hamza et al., 2016). The biological resistance of pesticides is harmful. Even minute amounts of pesticides can linger and have a profound effect on both human health and the ecosystem. Pesticide degradation has also benefited from the application of semiconductor photo-catalysis technology, which is chemically stable and resistant to biological degradation (AbuKhadra et al., 2020; Shawky et al., 2020). The semiconductor components for the photocatalytic breakdown of pesticides are predominantly focused in different metal oxides (such TiO2 and ZnO) (Taghizade Firozjaee et al., 2018). Under visible light, mesoporous Ag/Ag2O-TiO2 p-n heterojunction achieved photocatalytic destruction of dimethenamid-P herbicide. After 180 min, the photo-activity findings showed that imazapyr had completely disappeared (Table 1) (Mkhalid et al., 2020). Shawky used Ag/LaTiO3 nanowire to study the photocatalytic breakdown of the pesticide atrazine (Jamal Sisi et al., 2020). The findings showed that 2.5 weight percent of Ag loading was used to achieve complete photo degradation of herbicide with photo-catalyst after 40 min of visible light.
Significant progress has been made in the field of photo-thermal catalysis in recent years. Benefiting from both the thermal effects of light on the catalyst’s surface and the photo-induced charge carrier effects, which are not directly related to temperature. In comparison to conventional thermal catalysis, photo-thermal catalysis may be more cost- and energy-effective. Consequently aiding in the clean and efficient generation of renewable energy sources like hydrogen, syngas, methanol, and the elimination of waste products. Researchers have been devoting a lot of time and effort to developing sustainable technologies that are efficient, environmentally friendly, and cost-effective for Sustainable Energy Production and Environmental Treatment because of the critical nature of this issue. As a result, many nations now choose wastewater treatment systems based mostly on cost and ecological impact. Indicators for measuring the United Nations’ (UN) success in achieving SDG6 were evaluated by (Malik et al., 2015; Bhaduri et al., 2016). Both reports highlighted the fact that SDG6 will be difficult to achieve in low-income areas due to monetary constraints. In addition, the techno-economic viability of incorporating this technique into wastewater treatment facilities (WWTFs) has been the subject of multiple research (Mahmoud et al., 2021). looked at aggregation and absorption by Fe/Cu nanoparticles in textile WWTFs. Capital costs for coagulation and adsorption facilities were calculated to be 0.0208 USD/m3 annually, while energy costs were calculated to be 0.00054 USD/m3 and the material costs for coagulant and adsorbent materials were calculated to be 0.15 USD/m3 and 6.1 USD/m3. Authors also calculated 0.1 USD/m3 for labour expenses and 0.0037 USD/m3 for maintenance costs associated with the system. As a result, it was calculated that the operating costs would amount to 6.35 USD/m3. This operating cost exceeds the 5.8 USD/m3 estimate of operating expenses for the combination of electrocoagulation and ozonation methods for textile water treatment given by Yin et al. (2019). Therefore, rather than utilising chemically synthesised nanoparticles (Mahmoud et al., 2021), proposed that employing greenly synthesised nanoparticles can lower the operational expenses. Proanthocyanidins, complex flavonoid polymers found in fruits, legume seeds, and cereals, have also been studied for their potential to be used in the environmentally friendly production of pro-anthocyanidin-functionalized Fe3O4 nanoparticles for the removal of heavy metals (Shi et al., 2020).
Nonetheless, there is still a dearth of research on the use of recycled nanoparticles for large-scale elimination of heavy metals in treatment processes, as well as techno-economic evaluations of the potential financial benefits of recycling nanoparticles for wastewater treatment. Numerous investigations have effectively demonstrated the recyclable nature of nanoparticles. Nonetheless, there is still a dearth of research on the use of recycled nanoparticles for large-scale heavy metal removal in treatment processes, as well as techno-economic evaluations of the potential financial benefits of recycling nanoparticles for wastewater treatment. Furthermore, Cu/Zn nanoparticles were studied by (Singh et al., 2019) as a bacterial disinfectant for sewage treatment (Noman et al., 2019). The study’s findings showed that designing a suitable treatment plant with a 1,000 m3 capacity would require fixed capital of USD 950,000, while the treatment plant’s operating and working capital costs were projected to be USD 475,000 and USD 61,750, respectively. It is observed that the cost of nanoparticle raw materials varies according on the nation in which the investigation is carried out. Raw materials for producing 375,000 kg of magnesium oxide cost 483,750 USD, according to a techno-economic study conducted in Indonesia by (Meramo-Hurtado and González-Delgado, 2020). The market prices of magnesium nitrate, sodium bicarbonate, and sodium hydroxide were 2.00 USD/kg, 0.25 USD/kg, and 0.90 USD/kg. The price of machinery to manufacture magnesium oxide was calculated at USD 44,943. The author also said that the cost of this production over a year was 41,271 USD/kWh and that the cost of salaries was about 10 USD/day (Febriani et al., 2020), who reported the cost of making ZnO NPs in Malaysia, came up with a slightly higher than the estimation. As stated by the writer, the total cost of the orange peels and Zinc Acetate Dehydrate was 00.00 USD/kg and 259.00 USD/kg. It has been demonstrated that using agricultural waste as an absorbent for heavy metals is a less expensive option. A technological and economic investigation on the use of rice husk for copper (II) adsorption was carried out in Egypt by (Abd Elhafez et al., 2017). For a 1,200 t/y production, the anticipated expenses of operations, energy, and labour (five people) were 50,400 €/y, 32,040 €/y, and 46,900 €/y, respectively, whereas the market price of rice husk raw materials was 42 €/t. Keep in mind that the present economic status in various parts of the world may cause these estimates to fluctuate. It is worth noting that the varying state of economies around the world could cause these projections to shift. Table 2 summarizes the levelized cost of energy of photo-thermal catalysis in different applications.
The photocatalytic reaction of titanium dioxide, which breaks down water into hydrogen and oxygen when exposed to light, was initially observed by Fujishima and Honda in 1976 (Hashimoto et al., 2005). Since then, this discovery has prompted a large number of global photo-catalysis research projects. When it comes to cost savings and an effective process, this photo-catalysis approach has both significant benefits and drawbacks.
Strong chemical reactions can be facilitated by combining the advantages of photo-thermal heating with catalysis in the exciting field of photo-thermal catalysis. Due to its excellent efficiency and mild reaction conditions, it has the potential to revolutionise a variety of industries in a way that is both environmentally and fiscally sustainable, thereby resolving many of the issues that the modern world faces.
Since secondary water treatment is incapable of eliminating organic pollutants like pesticides, herbicides, and micro-pollutants like endocrine disrupting substances, employing heterogeneous photo-catalysis is a great alternative. Photocatalytic reaction is an effective method for cleaning oil from water. Photo-catalysis allows for quick oxidation, often taking only a few hours to complete. This technology is very feasible because to the availability of inexpensive, highly active catalysts that can be tailored to specific reactor systems. Moreover, no consumable chemicals are needed, and the catalyst itself remains unaltered throughout the procedure. As a result, there are significant savings and the equipment involved operates more easily. Furthermore, the catalyst’s photo-excitation energy is derived directly from the Sun, while the atmosphere provides the oxygen required for the reaction. This allows for an infinite and free supply of both sunlight and oxygen (Malato et al., 2009). Furthermore, as the contaminated molecules are gradually broken down by the process, no original material is left behind, meaning that no sludge that needs to be disposed of in a landfill is created. Furthermore, the global market for photo-catalysts is expected to rise at a compound annual growth rate (CAGR) of 10.5% from 2021 to 2026, from $3.1 billion in 2021 to $5.1 billion. During the forecast period of 2021–2026, the construction segment of the global photo-catalysts market is estimated to increase at a compound annual growth rate (CAGR) of 10.3%, from $2.7 billion in 2021 to $4.5 billion in 2026. With a compound annual growth rate (CAGR) of 12.1%, the consumer products segment of the worldwide photo-catalysts market is anticipated to increase from $238.7 million in 2021 to $421.9 million in 2026. The primary drivers of this expansion, which translates to a compound annual growth rate (CAGR) of Redacted for the years 2020–2026, are increased use in the consumer products and construction sectors as well as an overall improvement in global economic conditions. Since 2018, a notable decline in raw material unit prices has mostly countered a substantial increase in product demand, resulting in lower revenues. Over the course of the forecast period, the global market for products based on photo-catalysis is anticipated to rise at a CAGR of redacted, from redacted in 2020 to redacted in 2026 (Kumari et al., 2023).
Notwithstanding the benefits indicated above, photo-catalysis has many disadvantages as well. One major drawback is that, unlike conventional catalysis, the rate of surface reaction on photo-catalysts under irradiation is limited by electron-hole recombination, reduced surface areas, and smaller adsorptive and diffusive characteristics of substrates. Setting up semiconductor photo-catalysis has thus far only been effectively applied to wastewater treatment, where low concentrations of contaminants such as persistent toxic compounds and cyanide are degraded using solar photo-catalysis. In waste water treatment, the cost of production for the preparation of the membranes and the modules, a short lifespan for the membrane. When compared to inorganic or metallic membranes, polymeric membranes have a lower resistance to high temperatures and chemicals that are hostile. Similarly, Nano films can experience long lasting deposition, and higher fabrication cost. Even various catalysts used in photo-catalysis also has disadvantages when exposed to the air in the environment, catalyst can cause poisoning. Product contamination is a risk that arises from the leaching of catalyst active sites. It is hard to separate the catalyst from the result. The cost of catalysts is pretty high if enzymes can’t be retrieved and used again.
The developing subject of photo-thermal catalysis uses light-induced local heating to propel chemical processes, just like photo-catalysis does. This novel strategy has received a lot of interest because of its potential to improve reaction speeds, selectivity, and energy efficiency across a wide range of catalytic processes. While photo-thermal catalysis has a lot of potential, certain issues and questions about the future still need to be worked out:
a) Catalyst Design and Stability: Stable and effective photo-thermal catalysts that can operate in extreme environments, including high temperatures and reactive intermediates, remain a design problem. To find catalysts with high activity and stability over time, scientists need to look at new materials and designs.
b) Optimal Light Absorption: For photo-thermal catalysis to be successful, it is essential to achieve effective light absorption throughout a broad range of the electromagnetic spectrum. Creating catalysts that can absorb light in the visible and near-infrared spectrums simultaneously increases their usefulness.
c) Heat Dissipation and Temperature Control: To prevent undesirable side reactions or catalyst deactivation, accurate oversight of the localized heating impact is important. To guarantee optimum catalytic performance, it is necessary to devise strategies for efficient heat dissipation and temperature management within the reaction environment.
d) Scalability and Cost: To be useful in the real world, photo-thermal catalysis has to be both scalable and cost-effective. The broad use of photo-thermal catalysis in an extensive variety of chemical reactions and energy conversion activities is contingent on overcoming obstacles associated with catalyst design, stability, optimum light absorption, temperature control, scalability, and cost.
As our civilization progresses, pressing issues like energy scarcity and environmental damage have come to the fore. In order to make better use of renewable resources (Solar energy), scientists have created photo-thermal catalytic chemistry. Excellent qualities and the ability to tackle energy requirements and environmental problems at the same time have made photo-thermal catalysts a prominent topic of study. To present, a variety of materials with higher solar absorption for effective light to heat transformation have been produced, includes novel metals, semiconductors, carbon, nanocomposites, MXenes, polymers, and an organic-polymer substances. The goal of this research is to demonstrate the widespread nature of photo-thermo-catalysis synergies and their promising potential for increasing operational efficiency and adaptability in sustainable and environmental pollution control. Although here it is reviewed in a specific context and illustrated with examples based on the advantageous of renewable solar driven photo-thermal catalysis resources, the new phenomenology is clearly fundamental in origin and provides the basis of a new paradigm in applied photo-thermal catalysis. Although this analysis focuses on sustainable energy applications, similarly outstanding findings from other domains such as H2, CO2 Methane conversion, waste water, organic pollutant treatment, and oil spill clean-up have emerged even as this review was being written.
All authors listed have made a substantial, direct, and intellectual contribution to the work and approved it for publication.
The contributions of all authors to the preparation of this manuscripts is acknowledged.
The authors declare that the research was conducted in the absence of any commercial or financial relationships that could be construed as a potential conflict of interest.
All claims expressed in this article are solely those of the authors and do not necessarily represent those of their affiliated organizations, or those of the publisher, the editors and the reviewers. Any product that may be evaluated in this article, or claim that may be made by its manufacturer, is not guaranteed or endorsed by the publisher.
Abd Elhafez, S. E., Hamad, H. A., Zaatout, A. A., and Malash, G. F. (2017). Management of agricultural waste for removal of heavy metals from aqueous solution: adsorption behaviors, adsorption mechanisms, environmental protection, and techno-economic analysis. Environ. Sci. Pollut. Res. 24, 1397–1415. doi:10.1007/s11356-016-7891-7
AbuKhadra, M. R., Mohamed, A. S., El-Sherbeeny, A. M., and Elmeligy, M. A. (2020). Enhanced photocatalytic degradation of acephate pesticide over MCM-41/Co3O4 nanocomposite synthesized from rice husk silica gel and Peach leaves. J. Hazard. Mat. 389, 122129. doi:10.1016/j.jhazmat.2020.122129
Adhikari, S., Mandal, S., and Kim, D.-H. (2019). Z-scheme 2D/1D MoS2 nanosheet-decorated Ag2Mo2O7 microrods for efficient catalytic oxidation of levofloxacin. Chem. Eng. J. 373, 31–43. doi:10.1016/j.cej.2019.05.017
Agrafiotis, C., von Storch, H., Roeb, M., and Sattler, C. (2014). Solar thermal reforming of methane feedstocks for hydrogen and syngas production—A review. Renew. Sustain. Energy Rev. 29, 656–682. doi:10.1016/j.rser.2013.08.050
Ahmed, F. E., Lalia, B. S., Hashaikeh, R., and Hilal, N. (2020). Alternative heating techniques in membrane distillation: a review. Desalination 496, 114713. doi:10.1016/j.desal.2020.114713
Al-Ashouri, A., Köhnen, E., Li, B., Magomedov, A., Hempel, H., Caprioglio, P., et al. (2020). Monolithic perovskite/silicon tandem solar cell with >29% efficiency by enhanced hole extraction. Science 370, 1300–1309. doi:10.1126/science.abd4016
An, H., Li, M., Liu, R., Gao, Z., and Yin, Z. (2020). Design of AgxAu1−x alloy/ZnIn2S4 system with tunable spectral response and Schottky barrier height for visible-light-driven hydrogen evolution. Chem. Eng. J. 382, 122953. doi:10.1016/j.cej.2019.122953
Ansari, A. A., Nazeeruddin, M. K., and Tavakoli, M. M. (2021). Organic-inorganic upconversion nanoparticles hybrid in dye-sensitized solar cells. Coord. Chem. Rev. 436, 213805. doi:10.1016/j.ccr.2021.213805
Baffou, G., Cichos, F., and Quidant, R. (2020). Applications and challenges of thermoplasmonics. Nat. Mat. 19, 946–958. doi:10.1038/s41563-020-0740-6
Bhaduri, A., Bogardi, J., Siddiqi, A., Voigt, H., Vörösmarty, C., Pahl-Wostl, C., et al. (2016). Achieving sustainable development goals from a water perspective. Front. Environ. Sci. 4, 64. doi:10.3389/fenvs.2016.00064
Brongersma, M. L., Halas, N. J., and Nordlander, P. (2015). Plasmon-induced hot carrier science and technology. Nat. Nanotechnol. 10, 25–34. doi:10.1038/nnano.2014.311
Cai, M., Wu, Z., Li, Z., Wang, L. U., Sun, W., Tountas, A. A., et al. (2021). Greenhouse-inspired supra-photothermal CO2 catalysis. Nat. Energy 6 (8), 807–814. doi:10.1038/s41560-021-00867-w
Cally, C. (2018). Unique oil spill in East China Sea frustrates scientists. Nature 554, 17–18. doi:10.1038/d41586-018-00976-9
Campos, C., Vasco, D., Angulo, C., Burdiles, P. A., Cardemil, J., and Palza, H. (2019). About the relevance of particle shape and graphene oxide on the behavior of direct absorption solar collectors using metal based nanofluids under different radiation intensities. Energy Convers. Manag. 181, 247–257. doi:10.1016/j.enconman.2018.12.007
Carbuloni, C. F., Savoia, J. E., Santos, J. S. P., Pereira, C. A. A., Marques, R. G., Ribeiro, V. A. S., et al. (2020). Degradation of metforminin water by TiO2-ZrO2 photocatalysis. J. Environ. Manag. 262, 110347. doi:10.1016/j.jenvman.2020.110347
Chang, J., Shi, Y., Tang, C., and Wang, P. (2018b). Solar-assisted fast cleanup of heavy oil spill by a photothermal sponge. J. Mater. Chem. A. doi:10.1039/C8TA00779A
Chang, J., Shi, Y., Wu, M., Li, R., Shi, L., Jin, Y., et al. (2018a). Solar-assisted fast cleanup of heavy oil spills using a photothermal sponge. J. Mat. Chem. A 6, 9192–9199. doi:10.1039/c8ta00779a
Chen, C., Kuang, Y., and Hu, L. (2019). Challenges and opportunities for solar evaporation. Joule 3, 683–718. doi:10.1016/j.joule.2018.12.023
Chen, G., Gao, R., Zhao, Y., Li, Z., Waterhouse, G. I. N., Shi, R., et al. (2018a). Alumina-supported CoFe alloy catalysts derived from layered-double-hydroxide nanosheets for efficient photothermal CO2 hydrogenation to hydrocarbons. Adv. Mat. 30, 1704663. doi:10.1002/adma.201704663
Chen, G., Sun, J., Peng, Q., Sun, Q., Wang, G., Cai, Y., et al. (2020). Biradical-featured stable organic-small-molecule photothermal materials for highly efficient solar-driven water evaporation. Adv. Mat. 32, 1908537. doi:10.1002/adma.201908537
Chen, H., Liu, F., Xiao, X., Hu, J., Gao, B., Zou, D., et al. (2021). Visible light driven photocatalysis of carbon dioxide and organic pollutants by MFeO2 (M=Li, Na, or K). J. Colloid Interface. Sci. 601, 758–772. doi:10.1016/j.jcis.2021.05.156
Chen, S., Qi, Y., Li, C., Domen, K., and Zhang, F. (2018b). Surface strategies for particulate photocatalysts toward artificial photosynthesis. Joule 2, 2260–2288. doi:10.1016/j.joule.2018.07.030
Chen, S., Takata, T., and Domen, K. (2017). Particulate photocatalysts for overall water splitting. Nat. Rev. Mat. 2, 17050. doi:10.1038/natrevmats.2017.50
Cheng, P., Wang, H., Müller, B., Müller, J., Wang, D., and Schaaf, P. (2021). Photo-thermoelectric conversion using black silicon with enhanced light trapping performance far beyond the band edge absorption. ACS Appl. Mat. Interfaces 13, 1818–1826. doi:10.1021/acsami.0c17279
Chinnaiyan, P., Thampi, S. G., Kumar, M., and Balachandran, M. (2018). Photocatalytic degradation of metformin and amoxicillin in synthetic hospital wastewater: effect of classical parameters. Int. J. Environ. Sci. Technol. 16, 5463–5474. doi:10.1007/s13762-018-1935-0
Deng, Y., Li, J., Zhang, R., Han, C., Chen, Y., Zhou, Y., et al. (2022). Solar-energy-driven photothermal catalytic C–C coupling from CO2 reduction over WO3–x. Chin. J. Catal. 43, 1230–1237. doi:10.1016/s1872-2067(21)63868-8
Ding, Y. J., Zhang, C., Cai, G. Q., Xu, K., Wu, J. D., Zheng, J. H., et al. (2019). A facile preparation of cotton fabric containing hybrid poly(sodium methacrylate)/silver nanoparticles for oil removal and water disinfection. Text. Res. J. 89, 5096–5107. doi:10.1177/0040517519849470
Fang, J., Chen, Z., Zheng, Q., and Li, D. (2017). Photocatalytic decomposition of benzene enhanced by the heating effect of light: improving solar energy utilization with photothermocatalytic synergy. Catal. Sci. Technol. 7, 3303–3311. doi:10.1039/c7cy00532f
Febriani, L. I., Nurhashiva, C., Veronica, J., Ragadhita, R., Nandiyanto, A. B. D., and Kurniawan, T. (2020). Computation application: TechnoEconomic analysis on the production of magnesium oxide nanoparticles by precipitation method. Int. J. Inf. Inf. Syst. Comput. Eng. 1, 117–128. doi:10.34010/injiiscom.v1i1.5462
Feng, X., Liu, D., Yan, B., Shao, M., Hao, Z., Yuan, G., et al. (2021). Highly active PdO/Mn3O4/CeO2 nanocomposites supported on one dimensional halloysite nanotubes for photo assisted thermal catalytic methane combustion. Angew. Chem. Int. Ed. 60, 18552–18556. doi:10.1002/anie.202107226
Fu, G., Cho, E. J., Luo, X., Cha, J., Kim, J. H., Lee, H. W., et al. (2021b). Enhanced light harvesting in panchromatic double dye-sensitized solar cells incorporated with bilayered TiO2 thin film-based photoelectrodes. Sol. Energy 218, 346–353. doi:10.1016/j.solener.2021.03.011
Fu, G., Jiang, M., Liu, J., Zhang, K., Hu, Y., Xiong, Y., et al. (2021a). Rh/Al nanoantenna photothermal catalyst for wide-spectrum solar-driven CO2 methanation with nearly 100% selectivity. Nano Lett. 21, 8824–8830. doi:10.1021/acs.nanolett.1c03215
Fujishima, A., and Honda, K. (1972). Electrochemical photolysis of water at a semiconductor electrode. Nature 238, 37–38. doi:10.1038/238037a0
Gan, Z., Wu, X., Meng, M., Zhu, X., Yang, L., and Chu, P. K. (2014). Photothermal contribution to enhanced photocatalytic performance of graphene-based nanocomposites. ACS Nano 8 (9), 9304–9310. doi:10.1021/nn503249c
Gao, M., Peh, C. K., Meng, F. L., and Ho, G. W. (2021). Photothermal membrane distillation toward solar water production. Small Methods 5, e2001200. doi:10.1002/smtd.202001200
Gao, M., Zhu, L., Peh, C. K. N., and Ho, G. W. (2019a). Solar absorber material and system designs for photothermal water vaporization towards clean water and energy production. Energy Environ. Sci. 12, 841–864. doi:10.1039/c8ee01146j
Gao, M. M., Zhu, L. L., Peh, C. K., and Ho, G. W. (2019b). Solar absorber material and system designs for photothermal water vaporization towards clean water and energy production. Energy Environ. Sci. 12, 841–864. doi:10.1039/c8ee01146j
Gelle, A., Jin, T., de la Garza, L., Price, G. D., Besteiro, L. V., and Moores, A. (2019). Applications of plasmon-enhanced nanocatalysis to organic transformations. Chem. Rev. 120, 986–1041. doi:10.1021/acs.chemrev.9b00187
Ghasemi, H., Ni, G., Marconnet, A. M., Loomis, J., Yerci, S., Miljkovic, N., et al. (2014). Solar steam generation by heat localization. Nat. Commun. 5, 4449. doi:10.1038/ncomms5449
Ghoussoub, M., Xia, M., Duchesne, P. N., Segal, D., and Ozin, G. (2019a). Principles of photothermal gas-phase heterogeneous CO2 catalysis. Energy Environ. Sci. 12, 1122–1142. doi:10.1039/c8ee02790k
Ghoussoub, M., Xia, M., Duchesne, P. N., Segal, D., and Ozin, G. (2019b). Principles of photothermal gas-phase heterogeneous CO2 catalysis. Energy Environ. Sci. 12, 1122–1142. doi:10.1039/c8ee02790k
Gómez-Pastora, J., Dominguez, S., Bringas, E., Rivero, M. J., Ortiz, I., and Dionysiou, D. D. (2017). Review and perspectives on the use of magnetic nanophotocatalysts (MNPCs) in water treatment. Chem. Eng. J. 310, 407–427. doi:10.1016/j.cej.2016.04.140
Gong, C., Lao, J., Wang, B., Li, X., Li, G., Gao, J., et al. (2020). Fast and all-weather cleanup of viscous crude-oil spills with Ti3C2TX MXene wrapped sponge. J. Mat. Chem. A 8, 20162–20167. doi:10.1039/d0ta06480g
Govorov, A. O., and Richardson, H. H. (2007). Generating heat with metal nanoparticles. Nanotoday 2 (1), 30–38. doi:10.1016/s1748-0132(07)70017-8
Guo, S., Li, X., Ju, L., and Wei, B. (2021). Boosting photocatalytic hydrogen production from water by photothermally induced biphase systems, Nat. Commun. 12, 1343. doi:10.1038/s41467-021-21526-4
Guo, X., Li, J., Wang, Y., and Rui, Z. (2022). Photothermocatalytic water splitting over Pt/ZnIn2S4 for hydrogen production without external heat. Catal. Today 402, 210–219. doi:10.1016/j.cattod.2022.04.001
Guo, X. Y. X. N., Jiao, Z. F., Jin, G. Q., and Guo, X. Y. X. N. (2015). Photocatalytic fischer-tropsch synthesis on graphene-supported worm-like ruthenium nanostructures. ACS Catal. 5, 3836–3840. doi:10.1021/acscatal.5b00697
Hamza, R. A., Iorhemen, O. T., and Tay, J. H. (2016). Occurrence, impacts and removal of emerging substances of concern from wastewater. Environ. Technol. Innov. 5, 161–175. doi:10.1016/j.eti.2016.02.003
Han, C., Li, J., Ma, Z., Xie, H., Waterhouse, G. I. N., Ye, L., et al. (2018). Black phosphorus quantum dot/g-C3N4 composites for enhanced CO2 photo-reduction to CO. Sci. China Mat. 61, 1159–1166. doi:10.1007/s40843-018-9245-y
Hashimoto, K., Irie, H., and Fujishima, A. (2005). TiO2 photocatalysis: a historical overview and future prospects. Jpn. J. Appl. Phys. 44, 8269. doi:10.1143/jjap.44.8269
Hein, F. J., and Pet, J. (2016). Geology of bitumen and heavy oil: an overview. Sci. Eng. 154, 551–563. doi:10.1016/j.petrol.2016.11.025
Hoch, L. B., O’Brien, P. G., Jelle, A., Sandhel, A., Perovic, D. D., Mims, C. A., et al. (2016). Nanostructured indium oxide coated silicon nanowire arrays: a hybrid photo-thermal/photochemical approach to solar fuels. ACS Nano 10, 9017–9025. doi:10.1021/acsnano.6b05416
Hogan, N. J., Urban, A. S., Ayala-Orozco, C., Pimpinelli, A., Nordlander, P., and Halas, N. J. (2014). Nanoparticles heat through light localization. Nano Lett. 14, 4640–4645. doi:10.1021/nl5016975
Hosseini, S. M., Ghiaci, M., Kulinich, S. A., Wunderlich, W., Farrokhpour, H., Saraji, M., et al. (2018). Au-Pd@g-C3N4 as an efficient photocatalyst for visible-light oxidation of benzene to phenol: experimental and mechanistic study. J. Phys. Chem. C 122 (48), 27477–27485. doi:10.1021/acs.jpcc.8b08788
Hu, S., Shi, J., Luo, B., Ai, C., and Jing, D. (2022). Significantly enhanced photothermal catalytic hydrogen evolution over Cu2O-rGO/TiO2 composite with full spectrum solar light. J. Colloid Interface Sci. 608, 2058–2065. doi:10.1016/j.jcis.2021.10.136
Huang, X., Liu, J., Zhou, P., Su, G., Zhou, T., Zhang, X., et al. (2022). Ultrarobust photothermal materials via dynamic crosslinking for solar harvesting. Small 18, 2104048. doi:10.1002/smll.202104048
Huang, Z., Cai, X., Zang, S., Zheng, D., Li, F., and Li, F. (2023). Strong metal support effect of Pt/g-C3N4 photocatalysts for boosting photothermal synergistic degradation of benzene. Int. J. Mol. Sci. 24, 6872. doi:10.3390/ijms24076872
Hui, S., Mengbc, X., Wangbf, Z. J., Wangg, Z., Cheng, H., Wenggh, Y., et al. (2018). Visible-light-mediated methane activation for steam methane reforming under mild conditions: a case study of Rh/TiO2 catalysts. ACS Catal. 8 (8), 7556–7565. doi:10.1021/acscatal.8b01787
Huo, W., Cao, T., Xu, W., Guo, Z., Liu, X., Yao, H., et al. (2020). Facile construction of Bi2Mo3O12@Bi2O2CO3 heterojunctions for enhanced photocatalytic efficiency toward NO removal and study of the conversion process. Chin. J. Catal. 41, 268–275. doi:10.1016/S1872-2067(19)63460-1
Huo, W., Xu, W., Guo, Z., Zhang, Y., and Dong, F. (2021). Motivated surface reaction thermodynamics on the bismuth oxyhalides with lattice strain for enhanced photocatalytic NO oxidation. Appl. Catal. B 284, 119694. doi:10.1016/j.apcatb.2020.119694
Isari, A. A., Hayati, F., Kakavandi, B., Rostami, M., Motevassel, M., and Dehghanifard, E. N. (2020). N, Cu co-doped TiO2@functionalized SWCNT photocatalyst coupled with ultrasound and visible-light: an effective sono-photocatalysis process for pharmaceutical wastewaters treatment. Chem. Eng. J. 392, 123685. doi:10.1016/j.cej.2019.123685
Jamal Sisi, A., Fathinia, M., Khataee, A., and Orooji, Y. (2020). Systematic activation of potassium peroxydisulfate with ZIF-8 via sono-assisted catalytic process: mechanism and ecotoxicological analysis. J. Mol. Liq. 308, 113018. doi:10.1016/j.molliq.2020.113018
Jelle, A. A., Ghuman, K. K., O’Brien, P. G., Hmadeh, M., Amit, S., Perovic, D. D., et al. (2018b). Highly efficient ambient temperature CO2 photomethanation catalyzed by nanostructured RuO2 on silicon photonic crystal support. Adv. Energy Mat. 8, 1870041. doi:10.1002/aenm.201870041
Jelle, A. A., Ghuman, K. K., O’Brien, P. G., Hmadeh, M., Sandhel, A., Perovic, D. D., et al. (2018a). Highly efficient ambient temperature CO2 photomethanation catalyzed by nanostructured RuO2 on silicon photonic crystal support. Adv. Energy Mat. 8, 1702277. doi:10.1002/aenm.201702277
Ji, B., Zhao, W., Duan, J., Fu, L., Ma, L., and Yang, Z. (2020). Immobilized Ag3PO4/GO on 3D nickel foam and its photocatalytic degradation of norfloxacin antibiotic under visible light. RSC Adv. 10, 4427–4435. doi:10.1039/c9ra08678a
Jiang, H. Y., Peng, X. B., Yamaguchi, A., Fujita, T., Abe, H., and Miyauchi, M. (2019). Synergistic photothermal and photochemical partial oxidation of methane over noble metals incorporated in mesoporous silica. Chem. Commun. 55, 13765–13768. doi:10.1039/c9cc06170c
Kadi, M. W., Mohamed, R. M., Ismail, A. A., and Bahnemann, D. W. (2020). Performance of mesoporous α-Fe2O3/g-C3N4 heterojunction for photoreduction of Hg(II) under visible light illumination. Ceram. Int. 46, 23098–23106. doi:10.1016/j.ceramint.2020.06.087
Kanakaraju, D., Rusydah bt Mohamad Shahdad, N., Lim, Y.-C., and Pace, A. (2019). Concurrent removal of Cr(III), Cu(II), and Pb(II) ions from water by multifunctional TiO2/Alg/FeNPs beads. Sustain. Chem. Pharm. 14, 100176. doi:10.1016/j.scp.2019.100176
Kay, P., Hughes, S. R., Ault, J. R., Ashcroft, A. E., and Brown, L. E. (2017). Widespread, routine occurrence of pharmaceuticals in sewage effluent, combined sewer overflows and receiving waters. Environ. Pollut. 220, 1447–1455. doi:10.1016/j.envpol.2016.10.087
Khanmohammadi, M., Shahrouzi, J. R., and Rahmani, F. (2021). Insights into mesoporous MCM-41-supported titania decorated with CuO nanoparticles for enhanced photodegradation of tetracycline antibiotic. Environ. Sci. Pollut. Res. 28, 862–879. doi:10.1007/s11356-020-10546-0
Kho, E. T., Tan, T. H., Lovell, E., Wong, R. J., Scott, J., and Amal, R. (2017). A review on photo-thermal catalytic conversion of carbon dioxide. Green Energy Environ. 2, 204–217. doi:10.1016/j.gee.2017.06.003
Kostianoy, A. G., and Carpenter, A. (2018). “History, sources and volumes of oil pollution in the mediterranean sea,” in Oil pollution in the mediterranean sea: Part I. Handbook of environmental chemistry. Editors A. Carpenter, and A. Kostianoy (Springer), 9. doi:10.1007/6982018369
Kraemer, D., Jie, Q., McEnaney, K., Cao, F., Liu, W., Weinstein, L. A., et al. (2016). Concentrating solar thermoelectric generators with a peak efficiency of 7.4%. Nat. Energy 1, 16153. doi:10.1038/nenergy.2016.153
Ku, B.-J., Mnoyan, A., Hong, S.-K., Mnoyan, A., Hong, S. K., Go, K. S., et al. (2021). Photothermal fabrics for efficient oil-spill remediation via solar-driven evaporation combined with adsorption. ACS Appl. Mat. Interfaces 13, 13106–13113. doi:10.1021/acsami.0c21656
Kuang, Y., Chen, C., Chen, G., Pei, Y., Pastel, G., Jia, C., et al. (2019). Bioinspired solar-heated carbon absorbent for efficient cleanup of highly viscous crude oil. Adv. Funct. Mat. 29, 1900162. doi:10.1002/adfm.201900162
Kumari, H., Sonia, S., Ranga, R., Chahal, S., Devi, S., Sharma, S., et al. (2023). A review on photocatalysis used for wastewater treatment: dye degradation. Water, Air, & Soil Pollut. 234, 349. doi:10.1007/s11270-023-06359-9
Li, B., Guo, Z., Feng, Y., and Meng, M. (2021d). A multi-functional photothermal-catalytic foam for cascade treatment of saline wastewater. J. Mat. Chem. A 9, 16510–16521. doi:10.1039/d1ta03376j
Li, D., Huang, Y., Li, S., Wang, C., Li, Y., Zhang, X., et al. (2020c). Thermal coupled photoconductivity as a tool to understand the photothermal catalytic reduction of CO2. Chin. J. Catal. 41, 154–160. doi:10.1016/s1872-2067(19)63475-3
Li, G., Huang, J., Chen, J., Deng, Z., Huang, Q., Liu, Z., et al. (2019a). Highly active photocatalyst of Cu 2 O/TiO 2 octahedron for hydrogen generation. ACS Omega 4, 3392–3397. doi:10.1021/acsomega.8b03404
Li, J., Du, M., Lv, G., Zhou, L., Li, X., Bertoluzzi, L., et al. (2018a). Interfacial solar steam generation enables fast-responsive, energy-efficient, and low-cost off-grid sterilization. Adv. Mat. 30, 1805159. doi:10.1002/adma.201805159
Li, M., Han, N., Zhang, X., Wang, S., Jiang, M., Bokhari, A., et al. (2022b). Perovskite oxide for emerging photo(electro)catalysis in energy and environment. Environ. Res. 205, 112544. doi:10.1016/j.envres.2021.112544
Li, R. Q., Zhang, G. L., Yang, L. X., and Zhou, C. Q. (2021e). Superhydrophobic polyaniline absorbent for solar-assisted adsorption of highly viscous crude oil. Sep. Purif. Technol. 276, 119372. doi:10.1016/j.seppur.2021.119372
Li, S., Liu, J., Yin, Z., Ren, P., Lin, L., Gong, Y., et al. (2020b). Impact of the coordination environment on atomically dispersed Pt catalysts for oxygen reduction reaction. ACS Catal. 10, 907–913. doi:10.1021/acscatal.9b04558
Li, S., Wang, C., Li, D., Xing, Y., Zhang, X., and Liu, Y. (2022a). Bi4TaO8Cl/Bi heterojunction enables high-selectivity photothermal catalytic conversion of CO2-H2O flow to liquid alcohol. Chem. Eng. J. 435, 135133. doi:10.1016/j.cej.2022.135133
Li, Y., Li, R., Li, Z., Wei, W., Ouyang, S., Yuan, H., et al. (2020a). Effect of support on catalytic performance of photothermal Fischer-Tropsch synthesis to produce lower olefins over Fe5C2-based catalysts. Chem. Res. Chin. Univ. 36, 1006–1012. doi:10.1007/s40242-020-0253-5
Li, Z., Liu, J., Shi, R., Waterhouse, G. I. N., Wen, X. D., and Zhang, T. (2021a). Fe-based catalysts for the direct photohydrogenation of CO2 to value-added hydrocarbons. Adv. Energy Mat. 11, 2002783. doi:10.1002/aenm.202002783
Li, Z., Liu, J., Shi, R., Waterhouse, G. I. N., Wen, X.-D., and Zhang, T. (2021b). Fe-Based catalysts for the direct photohydrogenation of CO2 to value-added hydrocarbons. Adv. Energy. Mater 11 (12), 2002783. doi:10.1002/aenm.202002783
Li, Z., Liu, J., Zhao, Y., Waterhouse, G. I. N., Chen, G., Shi, R., et al. (2018b). Co-based catalysts derived from layered-double-hydroxide nanosheets for the photothermal production of light olefins. Adv. Mat. 30, 1800527. doi:10.1002/adma.201800527
Li, Z., Meng, X., and Zhang, Z. (2019b). Fabrication of surface hydroxyl modified g-C3N4 with enhanced photocatalytic oxidation activity. Catal. Sci. Technol. 9, 3979–3993. doi:10.1039/c9cy00550a
Li, Z., Shi, R., Zhao, J., and Zhang, T. (2021c). Ni-based catalysts derived from layered-double-hydroxide nanosheets for efficient photothermal CO2 reduction under flow-type system. Nano Res. 14 (12), 4828–4832. doi:10.1007/s12274-021-3436-6
Lin, L., Yao, S., Gao, R., Liang, X., Yu, Q., Deng, Y., et al. (2019a). A highly CO-tolerant atomically dispersed Pt catalyst for chemoselective hydrogenation. Nat. Nanotechnol. 14, 354–361. doi:10.1038/s41565-019-0366-5
Lin, Y., Xu, H., Shan, X., Di, Y., Zhao, A., Hu, Y., et al. (2019b). Solar steam generation based on the photothermal effect: from designs to applications, and beyond. J. Mat. Chem. A 7, 19203–19227. doi:10.1039/c9ta05935k
Linic, S., Christopher, P., and Ingram, D. B. (2011). Plasmonic-metal nanostructures for efficient conversion of solar to chemical energy. Nat. Mat. 10, 911–921. doi:10.1038/nmat3151
Liu, F., Dai, Y., Chen, F., and Chen, C. (2020). Lead bismuth oxybromide/graphene oxide: synthesis, characterization, and photocatalytic activity for removal of carbon dioxide, crystal violetdye, and 2-hydroxybenzoic acid. J. Colloid Interface. Sci. 562, 112–124. doi:10.1016/j.jcis.2019.12.006
Liu, S., Huang, C., Luo, X., and Rao, Z. (2018). High-performance solar steam generation of a paper-based carbon particle system. Appl. Therm. Eng. 142, 566–572. doi:10.1016/j.applthermaleng.2018.07.032
Liu, Y., Lin, Z., Wang, P., Huang, F., and Sun, J.-L. (2022). Measurement of the photothermal conversion efficiency of CNT films utilizing a Raman spectrum. Nanomaterials 12, 1101. doi:10.3390/nano12071101
Low, J., Zhang, L., Zhu, B., Liu, Z., and Yu, J. (2018). TiO2 photonic crystals with localized surface photothermal effect and enhanced photocatalytic CO2 reduction activity. ACS Sustain. Chem. Eng. 6, 15653–15661. doi:10.1021/acssuschemeng.8b04150
Luo, S., Ren, X., Lin, H., Song, H., and Ye, J. (2021a). Plasmonic photothermal catalysis for solar-to-fuel conversion: current status and prospects. Chem. Sci. 12, 5701–5719. doi:10.1039/d1sc00064k
Luo, S., Ren, X., Lin, H., Song, H., and Ye, J. (2021b). Plasmonic photothermal catalysis for solar-to-fuel conversion: current status and prospects. Chem. Sci. 12 (16), 5701–5719. doi:10.1039/D1SC00064K
Mahmoud, A. S., Mostafa, M. K., and Peters, R. W. (2021). A prototype of textile wastewater treatment using coagulation and adsorption by Fe/Cu nanoparticles: techno-economic and scaling-up studies. Nanomater. Nanotechnol. 11, 184798042110411. doi:10.1177/18479804211041181
Malato, S., Fernandez-Ibanez, P., Maldonado, M. I., Blanco, J., and Gernjak, W. (2009). Decontamination and disinfection of water by solar photocatalysis: recent overview and trends. Catal. Today 147, 1–59. doi:10.1016/j.cattod.2009.06.018
Malik, O. A., Hsu, A., Johnson, L. A., and de Sherbinin, A. (2015). A Global indicator of wastewater treatment to inform the Sustainable Development Goals (SDGs). Environ. Sci. Policy 48, 172–185. doi:10.1016/j.envsci.2015.01.005
Mamari, S. A., Khudaish, E., Kim, Y., Khraisheh, M., and Selvaraj, R. (2023). Lotus-bud like hexagonal ZnO/g-C3N4 composites for the photodegradation of benzene present in aqueous solution. Inorg. Chem. Commun. 150, 110539. doi:10.1016/j.inoche.2023.110539
Martinez-Palou, R., Mosqueira, M. d. L., Zapata-Rendon, B., Mar-Juarez, E., Bernal Huicochea, C., de la Cruz Clavel-Lopez, J., et al. (2011). Transportation of heavy and extra-heavy crude oil by pipeline: a review. J. Pet. Sci. Eng. 75, 274–282. doi:10.1016/j.petrol.2010.11.020
Mateo, D., Cerrillo, J. L., Durini, S., and Gascon, J. (2021). Fundamentals and applications of photo-thermal catalysis. Chem. Soc. Rev. 50, 2173–2210. doi:10.1039/d0cs00357c
Mateo, D., Morlanes, N., Maity, P., Shterk, G., Mohammed, O. F., and Gascon, J. (2020). Efficient visible-light driven photo-thermal conversion of CO2 to methane by nickel nanoparticles supported on barium titanate. Adv. Funct. Mat. 31, 2008244. doi:10.1002/adfm.202008244
Mekhilef, S., Saidur, R., and Safari, A. (2011). A review on solar energy use in industries. Renew. Sust. Energy Rev. 15, 1777–1790. doi:10.1016/j.rser.2010.12.018
Meng, L., Chen, Z., Ma, Z., He, S., Hou, Y., Li, H.-H., et al. (2018). Gold plasmon-induced photocatalytic dehydrogenative coupling of methane to ethane on polar oxide surfaces. Energy Environ. Sci. 11, 294–298. doi:10.1039/c7ee02951a
Meng, X., Liu, L., Ouyang, S., Xu, H., Wang, D., Zhao, N., et al. (2016). Nanometals for solar-to-chemical energy conversion: from semiconductor-based photocatalysis to plasmon-mediated photocatalysis and photo-thermocatalysis. Adv. Mat. 28, 6781–6803. doi:10.1002/adma.201600305
Meng, X., Wang, T., Liu, L., Ouyang, S., Li, P., Hu, H., et al. (2014). Photothermal conversion of CO2 into CH4 with H2 over group VIII nanocatalysts: an alternative approach for solar fuel production. Angew. Chem. Int. Ed. 53, 11478–11482. doi:10.1002/anie.201404953
Meramo-Hurtado, S. I., and González-Delgado, A. D. (2020). Application of techno-economic and sensitivity analyses as decision-making tools for assessing emerging large-scale technologies for production of chitosan-based adsorbents. ACS Omega 5, 17601–17610. doi:10.1021/acsomega.0c02064
Mkhalid, I. A., Fierro, J. L. G., Mohamed, R. M., and Alshahri, A. A. (2020). Visible light driven photooxidation of imazapyr herbicide over highly efficient mesoporous Ag/Ag2O–TiO2 p-n heterojunction photocatalysts. Ceram. Int. 46, 25822–25832. doi:10.1016/j.ceramint.2020.07.064
Mnoyan, A., Choi, M., Dong, H. K., Ku, B.-J., Kim, H., Lee, K. J., et al. (2020). Cheap, facile, and up scalable activated carbon based photo-thermal layers for solar steam generation. RSC Adv. 10, 42432–42440. doi:10.1039/d0ra07746a
Moradi, M., Hasanvandian, F., Isari, A. A., Hayati, F., Kakavandi, B., and Setayesh, S. R. (2020). CuO and ZnO co-anchored on g-C3N4 nanosheets as an affordable double Z-scheme nanocomposite for photocatalytic decontamination of amoxicillin. Appl. Catal. B Environ. 285, 119838. doi:10.1016/j.apcatb.2020.119838
Muniandya, L., Adama, F., Abdul Rahman Mohamedb, A. I., Nur Ruzaina, A. R., and Rahman, N. R. A. (2017). Cu2+ coordinated graphitic carbon nitride (Cu-g-C3N4) nanosheets from melamine for the liquid phase hydroxylation of benzene and VOCs. Appl. Surf. Sci. 398, 43–55. doi:10.1016/j.apsusc.2016.11.103
Nair, V., MarioMunoz-Batista, J., Fernandez-Garcia, M., Luque, R., and Colmenares, J. C. (2019b). Thermo-photo-catalysis: environmental and energy applications. ChemSusChem 12 (10), 2098–2116. doi:10.1002/cssc.201900175
Nair, V., Mu˜noz-Batista, M. J., Fernandez-Garcia, M., Luque, R., and Colmenares, J. C. (2019a). Thermo-photo-catalysis: environmental and energy applications. Chem. Sus Chem. 12, 2098–2116. doi:10.1002/cssc.201900175
Ni, W., Kou, X., Yang, Z., and Wang, J. (2008). Tailoring longitudinal surface plasmon wavelengths, scattering and absorption cross sections of gold nanorods. ACS Nano 2, 677–686. doi:10.1021/nn7003603
Niu, H. F., Li, J. B., Wang, X. F., Luo, F. H., and Qiang, Z. (2021). Solar-assisted, fast, and in situ recovery of crude oil spill by a superhydrophobic and photothermal sponge. Acs Appl. Mater Interfaces 13, 21175–21185. doi:10.1021/acsami.1c00452
Noman, E., Al-Gheethi, A., Talip, B. A., Mohamed, R., and Kassim, A. H. (2019). Inactivating pathogenic bacteria in greywater by biosynthesized Cu/Zn nanoparticles from secondary metabolite of Aspergillus iizukae; optimization, mechanism and techno economic analysis. PLoS ONE 14, 0221522. doi:10.1371/journal.pone.0221522
O’Brien, P., Nuzzo, R., Kroto, H., and Rocha, J. (2014). Hierarchical nanostructures for energy devices. Royal Society of Chemistry.
Olivos-Suarez, A. I., Sze´ cse´ nyi, A. `., Hensen, E. J. M., Ruiz-Martinez, J., Pidko, E. A., and Gascon, J. (2016). Strategies for the direct catalytic valorization of methane using heterogeneous catalysis: challenges and opportunities. ACS Catal. 6, 2965–2981. doi:10.1021/acscatal.6b00428
Pan, F., Xiang, X., Deng, W., Zhao, H., Feng, X., and Li, Y. (2017). A novel photo-thermochemical approach for enhanced carbon dioxide reforming of methane. ChemCatChem 10, 940–945. doi:10.1002/cctc.201701565
Qian, K., Sweeny, B. C., Johnston-Peck, A. C., Niu, W., Graham, J. O., DuChene, J. S., et al. (2019). Surface plasmon-driven water reduction: gold nanoparticle size matters. J. Am. Chem. Soc. 136, 9842–9845. doi:10.1021/ja504097v
Reddy, H., Guler, U., Kudyshev, Z., Kildishev, A. V., Shalaev, V. M., and Boltasseva, A. (2017). Temperature-dependent optical properties of plasmonic titanium nitride thin films. ACS Photonics 4, 1413–1420. doi:10.1021/acsphotonics.7b00127
Regmi, C., Kim, T.-H., Ray, S. K., Yamaguchi, T., and Lee, S. W. (2017). Cobalt-doped BiVO4 (Co-BiVO4) as a visible-light-driven photocatalyst for the degradation of malachite green and inactivation of harmful microorganisms in wastewater. Res. Chem. Intermed. 43, 5203–5216. doi:10.1007/s11164-017-3036-y
Sarina, S., Zhu, H. Y., Xiao, Q., Jaatinen, E., Jia, J., Huang, Y., et al. (2014). Angew. Chem. 126, 2979–2984. doi:10.1002/ange.201308145
Shan, X., Zhao, A., Lin, Y., Hu, Y., Di, Y., Liu, C., et al. (2020). Low-cost, scalable, and reusable photothermal layers for highly efficient solar steam generation and versatile energy Conversion. Adv. Sustain. Syst. 4, 1900153. doi:10.1002/adsu.201900153
Shawky, A., Mohamed, R. M., Mkhalid, I. A., Youssef, M. A., and Awwad, N. S. (2020). Visible light-responsive Ag/LaTiO3 nanowire photocatalystsfor efficient elimination of atrazine herbicide in water. J. Mol. Liq. 299, 112163. doi:10.1016/j.molliq.2019.112163
Shi, Y., Xing, Y., Deng, S., Zhao, B., Fu, Y., and Liu, Z. (2020). Synthesis of proanthocyanidins-functionalized Fe3O4 magnetic nanoparticles with high solubility for removal of heavy-metal ions. Chem. Phys. Lett. 753, 137600. doi:10.1016/j.cplett.2020.137600
Singh, S., Shauloff, N., and Jelinek, R. (2019). Solar-enabled water remediation via recyclable carbon dot/hydrogel composites. ACS Sustain. Chem. Eng. 7, 13186–13194. doi:10.1021/acssuschemeng.9b02342
Song, C., Liu, X., Xu, M., Masi, D., Wang, Y., Deng, Y., et al. (2020a). Photothermal conversion of CO2 with tunable selectivity using Fe-based catalysts: from oxide to carbide. ACS Catal. 10, 10364–10374. doi:10.1021/acscatal.0c02244
Song, C., Liu, X., Xu, M., Masi, D., Wang, Y., Deng, Y., et al. (2020d). Photothermal conversion of CO2 with tunable selectivity using Fe-based catalysts: from oxide to carbide. ACS Catal. 10 (18), 10364–10374. doi:10.1021/acscatal.0c02244
Song, C., Liu, X., Xu, M., Masi, D., Wang, Y., Deng, Y., et al. (2020b). Photothermal conversion of CO2 with tunable selectivity using Fe-based catalysts: from oxide to carbide. ACS Catal. 10, 10364–10374. doi:10.1021/acscatal.0c02244
Song, C., Liu, X., Xu, M., Masi, D., Wang, Y., Deng, Y., et al. (2020c). Photothermal conversion of CO2 with tunable selectivity using Fe-based catalysts: from oxide to carbide. ACS Catal. 10, 10364–10374. doi:10.1021/acscatal.0c02244
Song, C., Wang, Z., Yin, Z., Xiao, D., and Ding, M. (2022). Principles and applications of photothermal catalysis. Princ. Appl. photo-thermal Catal. 2 (1), 52–83. doi:10.1016/j.checat.2021.10.005
Song, J., Wu, X., Zhang, M., Liu, C., Yu, J., Sun, G., et al. (2020e). Highly flexible, core-shell heterostructured, and visible-light-driven titania-based nanofibrous membranes for antibiotic removal and E. coil inactivation. Chem. Eng. J. 379, 122269. doi:10.1016/j.cej.2019.122269
Sun, A. Q., Hou, X. A., and Hu, X. G. (2020). Super-performance photothermal conversion of 3D macrostructure graphene-CuFeSe2 aerogel contributes to durable and fast clean-up of highly viscous crude oil in seawater. Nano Energy 70, 104511. doi:10.1016/j.nanoen.2020.104511
Sun, H., He, C., Li, L., Yuan, Y., Pan, H., Zhou, K., et al. (2021). Photothermal synergic catalytic degradation of the gaseous organic pollutant isopropanol in oxygen vacancies utilizing ZnFe2O4. J. Chem. Res. 45, 773–780. doi:10.1177/1747519821999335
Taghizade Firozjaee, T., Mehrdadi, N., Baghdadi, M., and Nabi Bidhendi, G. R. (2018). Application of nanotechnology in Pesticides removal from aqueous solutions-A review. Int. J. Nanotechnol. 15, 43–56.
Tang, S., Sun, J., Hong, H., and Liu, Q. (2017). Solar fuel from photo-thermal catalytic reactions with spectrum-selectivity: a review. Front. Energy. 11, 437–451. doi:10.1007/s11708-017-0509-z
Tao, P., Ni, G., Song, C., Shang, W., Wu, J., Zhu, J., et al. (2018). Solar-driven interfacial evaporation. Nat. Energy 3, 1031–1041. doi:10.1038/s41560-018-0260-7
Taylor, R. A., Phelan, P. E., Otanicar, T. P., Adrian, R., and Prasher, R. (2011). Nanofluid optical property characterization: towards efficient direct absorption solar collectors. Nanoscale Res. Lett. 6, 225. doi:10.1186/1556-276x-6-225
Thomas, B., and Alexander, L. K. (2020). Removal of Pb2+ and Cd2+ toxic heavy metal ions driven by Fermi level modification in NiFe2O4-Pd nano hybrids. J. Solid State Chem. 288, 121417. doi:10.1016/j.jssc.2020.121417
Tian, J., and Guo, Q. (2022). Direct conversion of CO2 into hydrocarbon solar fuels by a synergistic photothermal catalysis. Catalysts 12, 612. doi:10.3390/catal12060612
Tian, L., Chen, R., Qiao, K., Xiong, W., Zhang, Y., Mao, H., Li, J., et al. (2021). Photothermal-assist enhanced high-performance self-powered photodetector with bioinspired temperature-autoregulation by passive radiative balance. Nano Energy 79, 105435. doi:10.1016/j.nanoen.2020.105435
Tong, Z., Yang, D., Shi, J., Nan, Y., Sun, Y., and Jiang, Z. (2015). Three-dimensional porous aerogel constructed by g-C3N4 and graphene oxide nanosheets with excellent visible-light photocatalytic performance. ACS Appl. Mat. Interfaces 7, 25693–25701. doi:10.1021/acsami.5b09503
Verma, M., and Haritash, A. K. (2020). Photocatalytic degradation of Amoxicillin in pharmaceutical wastewater: a potential tool to manage residual antibiotics. Environ. Technol. Innov. 20, 101072. doi:10.1016/j.eti.2020.101072
Vijayanandan, A. S., Valappil, R. S. K., and Balakrishnan, R. M. (2020). Evaluation of photothermal properties for absorption of solar energy by Co3O4 nanofluids synthesized using endophytic fungus Aspergillus nidulans. Sustain. Energy Technol. Assess. 37, 100598. doi:10.1016/j.seta.2019.100598
Villafan-Vidales, H. I., Arancibia-Bulnes, C. A., Riveros-Rosas, D., Romero-Paredes, H., and Estrada, C. A. (2017). An overview of the solar thermochemical processes for hydrogen and syngas production: reactors, and facilities. Renew. Sust. Energy Rev. 75, 894–908. doi:10.1016/j.rser.2016.11.070
Voiry, D., Shin, H. S., Loh, K. P., and Chhowalla, M. (2018). Low-dimensional catalysts for hydrogen evolution and CO2 reduction. Nat. Rev. Chem. 2, 0105. doi:10.1038/s41570-017-0105
Wang, J., Li, Y., Deng, L., Wei, N., Weng, Y., Dong, S., et al. (2017). High-performance photo-thermal conversion of narrow-bandgap Ti2O3 nanoparticles. Adv. Mat. 29, 1603730. doi:10.1002/adma.201603730
Wang, L., Hasanzadeh Kafshgari, M., and Meunier, M. (2020c). Optical properties and applications of plasmonic-metal nanoparticles. Adv. Funct. Mat. 30, 2005400. doi:10.1002/adfm.202005400
Wang, S., Zhang, D., Wu, W., Zhong, J., Feng, K., Wu, Z., et al. (2022). Grave-to-cradle upcycling of Ni from electroplating wastewater to photothermal CO2 catalysis. Nat. Commun. 13, 5305. doi:10.1038/s41467-022-33029-x
Wang, X., Jiang, L., Li, K., Wang, J., Fang, D., Zhang, Y., et al. (2020d). Fabrication of novel Z-scheme SrTiO3/MnFe2O4 system with double-response activity for simultaneous microwave-induced and photocatalytic degradation of tetracycline and mechanism insight. Chem. Eng. J. 400, 125981. doi:10.1016/j.cej.2020.125981
Wang, Z., Song, H., Liu, H., and Ye, J. (2020a). Coupling of solar energy and thermal energy for carbon dioxide reduction: status and prospects. Angew. Chem. Int. Ed. 59, 8016–8035. doi:10.1002/anie.201907443
Wang, Z., Song, H., Liu, H., and Ye, J. (2020b). Coupling of solar energy and thermal energy for carbon dioxide reduction: status and prospects. Angew. Chem. Int. Ed. 59, 8016–8035. doi:10.1002/anie.201907443
Wu, S., Yang, H., Xiong, G., Tian, Y., Gong, B., Luo, T., et al. (2019b). Spill-SOS: self-pumping siphon-capillary oil recovery. ACS Nano 13, 13027–13036. doi:10.1021/acsnano.9b05703
Wu, S.-L., Chen, H., Wang, H.-L., Chen, X., Yang, H.-C., and Darling, S. B. (2020). Solar-driven evaporators for water treatment: challenges and opportunities. Environ. Sci. Water Res. Technol. 7, 24–39. doi:10.1039/d0ew00725k
Wu, X., Chen, G. Y., Owens, G., Chu, D., and Xu, H. (2019a). Photo-thermal materials: a key platform enabling highly efficient water evaporation driven by solar energy. Mat. Today Energy. 12, 277–296. doi:10.1016/j.mtener.2019.02.001
Wu, Z., Li, C., Li, Z., Feng, K., Cai, M., Zhang, D., et al. (2021a). Niobium and titanium carbides (MXenes) as superior photothermal supports for CO2 photocatalysis. ACS Nano 15, 5696–5705. doi:10.1021/acsnano.1c00990
Wu, Z., Li, C., Zhao, L., Feng, K., Cai, M., Zhang, D., et al. (2021b). Niobium and titanium carbides (MXenes) as superior photothermal supports for CO2 photocatalysis. ACS Nano 15, 5696–5705. doi:10.1021/acsnano.1c00990
Xu, D., Li, Z., Li, L., and Wang, J. (2020). Insights into the photothermal conversion of 2D MXene nanomaterials: synthesis, mechanism, and applications. Adv. Funct. Mat. 30, 2000712. doi:10.1002/adfm.202000712
Xu, M., Hu, X., Wang, S., Yu, J., Zhu, D., and Wang, J. (2019a). Photothermal effect promoting CO2 conversion over composite photocatalyst with high graphene content. J. Catal. 377, 652–661. doi:10.1016/j.jcat.2019.08.010
Xu, R., Zhao, H., Jin, H., Wang, Z., Zhang, Z., Xu, S., et al. (2019b). Scalable fabrication of geometry-tunable self-aligned superlattice photonic crystals for spectrum-programmable light trapping. Nano Energy 58, 543–551. doi:10.1016/j.nanoen.2019.01.074
Yan, R., Luo, D., Fu, C., Tian, W., Wu, P., Wang, Y., et al. (2020). Simultaneous removal of Cu(II) and Cr(VI) ions from wastewater by photoreduction with TiO2–ZrO2. J. Water Process Eng. 33, 101052. doi:10.1016/j.jwpe.2019.101052
Yang, J., Zhang, X., Qu, H., Yu, Z. G., Zhang, Y., Eey, T. J., et al. (2020b). Water harvesting: a moisture-hungry copper complex harvesting air moisture for potable water and autonomous urban agriculture (adv. Mater. 39/2020). Adv. Mat. 32, 2002936. doi:10.1002/adma.202002936
Yang, L., Xiang, Y., Jia, F., Xia, L., Gao, C., Wu, X., et al. (2021). Photo-thermal synergy for boosting photo-Fenton activity with rGO-ZnFe2O4: novel photo-activation process and mechanism toward environment remediation. Appl. Catal. B Environ. 292, 120198. doi:10.1016/j.apcatb.2021.120198
Yang, M., Tan, C. F., Lu, W., Zeng, K., and Ho, G. W. (2020a). Spectrum tailored defective 2D semiconductor nanosheets aerogel for full-spectrum-driven photothermal water evaporation and photochemical degradation. Adv. Funct. Mat. 30, 2004460. doi:10.1002/adfm.202004460
Yang, R., Zhong, S., Zhang, L., and Liu, B. (2020c). PW12/CN@Bi2WO6 composite photocatalyst prepared based on organic-inorganic hybrid system for removing pollutants in water. Sep. Purif. Technol. 235, 116270. doi:10.1016/j.seppur.2019.116270
Yang, X., Yang, Y., Fu, L., Zou, M., Li, Z., Cao, A., et al. (2018). An ultrathin flexible 2D membrane based on single-walled nanotube–MoS2 hybrid film for high-performance solar steam generation. Adv. Funct. Mat. 28, 1704505. doi:10.1002/adfm.201704505
Yin, H., Qiu, P., Qian, Y., Kong, Z., Zheng, X., Tang, Z., et al. (2019). Textile wastewater treatment for water reuse: a case study. Processes 7, 34. doi:10.3390/pr7010034
Yu, F., Wang, C., Li, Y., Ma, H. E., Wang, R., Liu, Y., et al. (2020). Enhanced solar photothermal catalysis over solution plasma activated TiO2. Adv. Sci. 7 (16), 2000204. doi:10.1002/advs.202000204
Yu, G., Qian, J., Zhang, P., Zhang, B., Zhang, W., Yan, W., et al. (2019). Collective excitation of plasmon-coupled Au-nanochain boosts photocatalytic hydrogen evolution of semiconductor. Nat. Commun. 10, 4912–4918. doi:10.1038/s41467-019-12853-8
Yu, M. J., Chang, C. L., Lan, H. Y., Chiao, Z. Y., Chen, Y. C., Lee, H. W. H., et al. (2021). Plasmon-enhanced solar-driven hydrogen evolution using titanium nitride metasurface broadband absorbers. ACS Photonics 8, 3125–3132. doi:10.1021/acsphotonics.1c00927
Yuan, X., Zhang, X., Sun, L., Wei, Y., and Wei, X. (2019). Cellular toxicity and immunological effects of carbon-based, nanomaterials. Part. Fibre Toxicol. 16 (1), 18–27. doi:10.1186/s12989-019-0299-z
Zang, X., Zhou, Q., Chang, J., Liu, Y., and Lin, L. (2015). Graphene and carbon nanotube (CNT) in MEMS/NEMS applications. Microelectron. Eng. 132, 192–206. doi:10.1016/j.mee.2014.10.023
Zeng, H. Y., Wang, P., Liang, L. Z., Hu, H., Peng, Y., Li, X. L., et al. (2022). Facile preparation of superhydrophobic cotton fabric with a photothermal conversion effect via polypyrrole deposition for oil/water separation. J. Environ. Chem. Eng. 10, 106915. doi:10.1016/j.jece.2021.106915
Zha, X. J., Zhao, X., Pu, J. H., Tang, L. S., Ke, K., Bao, R. Y., et al. (2019). Flexible anti-biofouling MXene/cellulose fibrous membrane for sustainable solar-driven water purification. ACS Appl. Mat. Interfaces 11, 36589–36597. doi:10.1021/acsami.9b10606
Zhang, C., Wu, M. B., Wu, B. H., Yang, J., and Xu, Z. K. (2018c). Solar-driven selfheating sponges for highly efficient crude oil spill remediation. J. Mater Chem. A 6, 8880–8885. doi:10.1039/c8ta02336k
Zhang, D., Lv, K., Li, C., Fang, Y., Wang, S., Chen, Z., et al. (2021c). All-earth-abundant photothermal silicon platform for CO2 catalysis with nearly 100% sunlight harvesting ability. Sol. RRL 5, 2000387. doi:10.1002/solr.202000387
Zhang, D., Ren, Y., Fan, X., Zhai, J., and Jiang, L. (2020a). Photoassisted salt-concentration-biased electricity generation using cation-selective porphyrin-based nanochannels membrane. Nano Energy 76, 105086. doi:10.1016/j.nanoen.2020.105086
Zhang, F., Li, Y.-H., Qi, M.-Y., Yamada, Y. M. A., Anpo, M., Tang, Z.-R., et al. (2021b). Photothermal catalytic CO2 reduction over nanomaterials. Chem. Catal. 1 (2), 272–297. doi:10.1016/j.checat.2021.01.003
Zhang, G., Chen, D., Li, N., Xu, Q., Li, H., He, J., et al. (2018a). Preparation of ZnIn2S4 nanosheet-coated CdS nanorod heterostructures for efficient photocatalytic reduction of Cr(VI). Appl. Catal. B Environ. 232, 164–174. doi:10.1016/j.apcatb.2018.03.017
Zhang, H., Wang, T., Wang, J., Liu, H., Dao, T. D., Li, M., et al. (2016). Surface-plasmon enhanced photo driven CO2 reduction catalyzed by metal–organic-frame work derived iron nanoparticles encapsulated by ultrathin carbon layers. Adv. Mat. 28, 3703–3710. doi:10.1002/adma.201505187
Zhang, S., Zhao, Y., Shi, R., Zhou, C., Waterhouse, G. I. N., Wang, Z., et al. (2021a). Sub-3 nm ultrafine Cu2O for visible light driven nitrogen fixation. Angew. Chem. Int. Ed. 60, 2554–2560. doi:10.1002/anie.202013594
Zhang, X., Junhui, Y., Jing, Y., Ting, C., Bei, X., Zhe, L., et al. (2018b). Excellent low-temperature catalytic performance of nanosheet Co-Mn oxides for total benzene oxidation. Appl. Catal. A Gen. 566, 104–112. doi:10.1016/j.apcata.2018.05.039
Zhang, X., Li, T.-T., Ren, H.-T., Peng, H.-K., Shiu, B.-C., Wang, Y., et al. (2020b). Dual-shell photothermoelectric textile based on a PPy photothermal layer for solar thermal energy harvesting. ACS Appl. Mat. Interfaces 12, 55072–55082. doi:10.1021/acsami.0c16401
Zhang, X., Zhang, R., Yang, P., Chen, H. S., and Ping Jiang, S. (2022). Black magnetic Cu-g-C3N4 nanosheets towards efficient photocatalytic H2 generation and CO2/benzene conversion. Chem. Eng. J. 450, 138030. doi:10.1016/j.cej.2022.138030
Zhang, Y., He, S., Guo, W., Hu, Y., Huang, J., Mulcahy, J. R., et al. (2017). Surfaceplasmon-driven hot electron photochemistry. Chem. Rev. 118, 2927–2954. doi:10.1021/acs.chemrev.7b00430
Zhang, Y., Zhao, Y., Xu, Z., Su, H., Bian, X., Zhang, S., et al. (2020c). Carbon quantum dots implanted CdS nanosheets: efficient visible-light-driven photocatalytic reduction of Cr(VI) under saline conditions. Appl. Catal. B Environ. 262, 118306. doi:10.1016/j.apcatb.2019.118306
Zhao, Y., Dunn, A., Lin, J., and Shi, D. (2019a). Novel nanomaterials for biomedical, environmental and energy applications. Elsevier, 415–434.
Zhao, Y., Gao, W., Li, S., Williams, G. R., Mahadi, A. H., and Ma, D. (2019b). Solar-versus thermal-driven catalysis for energy conversion. Joule 3, 920–937. doi:10.1016/j.joule.2019.03.003
Zhou, J., Liu, H., and Wang, H. (2023). Photothermal catalysis for CO2 conversion. Chin. Chem. Lett. 34 (2), 107420. doi:10.1016/j.cclet.2022.04.018
Zhou, L., Tan, Y., Ji, D., Zhu, B., Zhang, P., Xu, J., et al. (2016). Self-assembly of highly efficient, broadband plasmonic absorbers for solar steam generation. Sci. Adv. 2, e1501227. doi:10.1126/sciadv.1501227
Zhu, L., Gao, M., Peh, C. K. N., and Ho, G. W. (2018a). Solar-driven photothermal nanostructured materials designs and prerequisites for evaporation and catalysis applications. Mat. Horiz. 5, 323–343. doi:10.1039/c7mh01064h
Zhu, L., Gao, M., Peh, C. K. N., Wang, X., and Ho, G. W. (2018b). Carbon sponges: self-contained monolithic carbon sponges for solar-driven interfacial water evaporation distillation and electricity generation (adv. Energy mater. 16/2018). Adv. Energy Mat. 8, 1702149. doi:10.1002/aenm.201870074
Keywords: photo-thermal catalysis, sustainable energy, environmental pollution, solar-driven conversions, and applications, synergistic effects
Citation: Darko DA, Sahu S, Rathore J, Kaur L, Jain B, Kanungo S and Rawat R (2024) Photo-thermal catalysis for sustainable energy production and environmental treatment. Front. Energy Res. 12:1251188. doi: 10.3389/fenrg.2024.1251188
Received: 01 July 2023; Accepted: 14 March 2024;
Published: 09 April 2024.
Edited by:
Ellen B. Stechel, Arizona State University, United StatesReviewed by:
Atul Sagade, Solar Energy Research Laboratory (SERL), IndiaCopyright © 2024 Darko, Sahu, Rathore, Kaur, Jain, Kanungo and Rawat. This is an open-access article distributed under the terms of the Creative Commons Attribution License (CC BY). The use, distribution or reproduction in other forums is permitted, provided the original author(s) and the copyright owner(s) are credited and that the original publication in this journal is cited, in accordance with accepted academic practice. No use, distribution or reproduction is permitted which does not comply with these terms.
*Correspondence: Daniel Amoako Darko, ZGFkYXJrb0B1Zy5lZHUuZ2g=; Sangeeta Sahu, ZHIuc2FuZ2VldGFzYWh1QGJpdHJhaXB1ci5hYy5pbg==
Disclaimer: All claims expressed in this article are solely those of the authors and do not necessarily represent those of their affiliated organizations, or those of the publisher, the editors and the reviewers. Any product that may be evaluated in this article or claim that may be made by its manufacturer is not guaranteed or endorsed by the publisher.
Research integrity at Frontiers
Learn more about the work of our research integrity team to safeguard the quality of each article we publish.