- 1Sichuan College of Architectural Technology, Chengdu, China
- 2Post-Doctoral Research Center, Southwest Petroleum University, Chengdu, China
- 3Shale Gas Evaluation and Exploitation Key Laboratory of Sichuan Province, Chengdu, China
- 4Natural Gas Geology Key Laboratory of Sichuan Province, Chengdu, China
In complex geological areas, the chronology of tectonic fracture formation is pivotal for the conservation and enhancement of shale gas reservoirs. These fractures, evolving over different geologic epochs, critically influence the modifications in hydraulic fracturing. The review sheds light on an integrated methodology that bridges conventional geological evaluations with experimental diagnostics to decipher the intricate evolution of such fractures in complex geological areas. Shale tectonic fractures, predominantly shear-induced, are delineated into four distinct levels (I, II, III, IV) based on observational scales. Understanding the geometric interplay across these scales provides insight into fracture distribution. Recognizing the constraints of isolated approaches, this study amalgamates macroscopic geological assessments, such as structural evolution and fault analysis, with microscopic techniques, including fluid inclusion studies, isotopic testing, rock AE experiments (U-Th)/He thermochronology, and AFT analysis, etc. This combined approach aids in accurately determining the tectonic fracture’s genesis and its geological time. Future research endeavors should refine this framework, with an emphasis on enhanced geochemical profiling of fracture fillings.
1 Introduction
Shale gas, recognized as a premier clean energy resource, has witnessed significant advancements in the Sichuan Basin, underpinned by a constellation of geological theories and innovative exploration and extraction methodologies (Wang et al., 2016a; Li et al., 2022; Li, 2022; 2023). The marine shale strata within the Wufeng-Longmaxi Formation are particularly noteworthy due to their elevated Total Organic Carbon (TOC) concentration, pronounced stratigraphic thickness, advanced maturity, inherent brittleness, and abundant gas content, solidifying their status as the prime locus for shale gas prospecting and extraction (Wang et al., 2018; 2022a). A testament to nearly a decade of relentless exploratory and developmental endeavors, successful shale gas fields have been commissioned in locales such as Fuling, Changning, Zhaotong, Weiyuan-Rongchang, and Luzhou (Fan et al., 2020a; Xu et al., 2020a; He et al., 2022a; He et al., 2022b). The marine shale sequences in the Sichuan Basin have been subjected to myriad tectonic episodes, culminating in the genesis of intricate fracture network systems spanning various scales—tectonic fractures being particularly prevalent (Li J. et al., 2022; 2023; Zhu et al., 2023). The evolution and architecture of these fractures and faults are pivotal in shaping the efficacy of shale gas reservoirs (Fan et al., 2020b). Serving dual roles, they act as reservoir spaces and enhance reservoir connectivity by bridging pores and microfractures, thereby ameliorating pore-permeability dynamics (Hou et al., 2020; Fan et al., 2022; Zhu et al., 2023).
Additionally, the unique adsorption and storage mechanisms of shale gas within rocks dictate that the development of these reservoirs relies on large-scale hydraulic fracturing to achieve industrial gas production (Hui et al., 2022). The construction of tectonic fracture networks plays an important role in the formation and expansion of hydraulic fractures (Wang et al., 2016b; Xia and Lu, 2022; Huang et al., 2023; Tan et al., 2023). Over the years, scholars have made significant progress in understanding various aspects of shale fractures, including their development characteristics, quantitative characterization, identification methods, distribution patterns, controlling factors, and impact on gas content, etc (Gale et al., 2014; Wu et al., 2019; Xu et al., 2019; 2020b; Gong et al., 2021; Meng et al., 2021; Shan et al., 2021; Tan et al., 2021). The formation period of tectonic fractures is considered a challenging and crucial aspect in this research field. It is generally believed that tectonic fractures are controlled by regional or local stress in the geological mechanical environment. Determining the formation period reflects the evolution periods of shale gas preservation conditions and contributes to the coupled study of shale gas enrichment and migration (Feng et al., 2023). Additionally, it can indicate the formation periods of geological structures, such as folds and faults.
Various methods have been developed to determine the formation period of tectonic fracture. These methods include structural analysis, multi-scale fracture cutting relationships, fracture fillings analysis, and rock AE analysis. This paper summarizes the latest developments and the potential challenges it may face. It provides a systematic and intuitive perspective on fracture formation, development, and evolution in “sweet spot” shale layers. It is expected to be a reference and guide for research and practices on exploring shale fracturing mechanisms, optimizing hydraulic fracturing processes, and increasing and stabilizing shale gas production.
2 Characterization of types and development characteristics parameters of shale tectonic fractures
Over the years, many research efforts have yielded a comprehensive understanding of the development characteristics of tectonic fractures. This understanding is based on the outcrop, core, and thin slice observation, focusing on parameters such as fracture types, length, aperture, density, and fillings, etc. Shale tectonic fractures are primarily dominated by shear fractures, followed by tensile fractures (Li et al., 2019a; Li et al., 2021a). In field outcrops, shear fractures are particularly prevalent, characterized by straight and stable orientations, often occurring in groups, and exhibiting prominent crosscutting relationships. Based on the mutual relationship between shear fractures and rock layers, they can be further classified into plane and profile shear fractures (Figure 1A). Plane shear fractures typically form early, primarily when the rock layers have not undergone significant deformation (Figure 1A). These fractures have relatively flat fracture surfaces and often intersect with the rock layers at high angles, ranging from 45° to 75° or even vertically (75°–90°) (Figures 1B,C). Plane shear fractures often exhibit a multi-set “X" type conjugate relationship on the plane of the rock layer. In cross-section, they can result in a stepped blocky exposure of the rock. In contrast, profile shear fractures form later, typically after significant deformation of the rock layers has occurred (Figure 1A). The fracture surfaces may display scratch marks resulting from shear action and intersect the rock layer at low-angle obliquities, ranging from 15° to 45° (Figure 1D). Tensile fractures, on the other hand, are less developed, but due to surface weathering and erosion in exposed outcrops, their recognition can be challenging. They tend to have unstable orientations, and are often filled with calcite (Figure 1E).
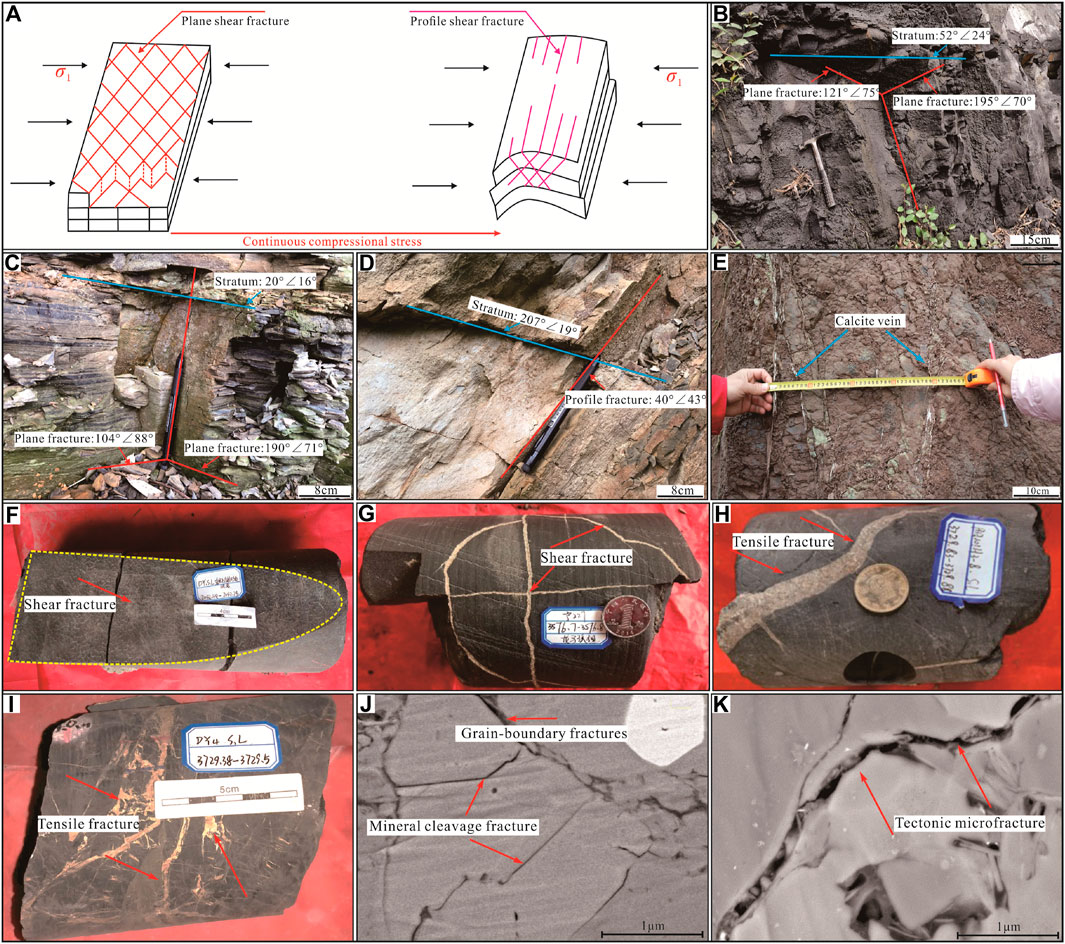
FIGURE 1. Tectonic fracture types and characteristics of the Longmaxi Formation shale in the Sichuan Basin (Zhong, 2019; Fan et al., 2020b). (A) Schematic diagram of the formation of plane shear fractures and profile shear fractures. (B,C) Plane shear fractures in Changning area (Fan et al., 2020b); (D) Profile shear fractures in Changning area; (E). Tensile fractures in Dingshan area; (F) DY1, 2042.45–2042.75 m, shear fracture; (G) N227, 3,576.70–3,576.80 m, shear fracture; (H) Y101H3-8, 3,729.83–3,729.86 m, tensile fracture; (I) DY4, 3,729.38–3,729.50 m, multiple tensile fractures forming a network; (J) DY1, grain-boundary fractures and mineral cleavage fractures; (K) DY1, tectonic fracture.
Fractures in core samples are still primarily characterized by shear fractures, which exhibit features such as flat fracture surfaces, long extents, and penetrating the entire core (Li et al., 2021a; Li et al., 2021b). On one hand, shear fractures can cut across the entire core, resulting in relatively smooth fracture surfaces after the core is split (Figure 1F). On the other hand, in the cross-section of the core, multiple sets of fractures can be observed intersecting each other at certain angles (Figure 1G). Tensile fractures, in contrast, are characterized by rough fracture surfaces, shorter extents, and uneven apertures. They often intersect, forming a network-like pattern, and most are partial to fully filled by calcite (Figures 1H,I).
Tectonic microfractures encompass grain-boundary fractures (intergranular fractures) and mineral cleavage fractures (intragranular fractures). Among them, grain-boundary fractures primarily develop along mineral grain boundaries, exhibiting shapes and patterns that are in harmony with the morphology of mineral boundaries. Their orientations and dip angles are often variable, and the fracture apertures can vary significantly (Figure 1J). Mineral cleavage fractures, on the other hand, develop within mineral grains. They feature straight fracture surfaces, minimal curvature, and show no evidence of cement fillings. The grain size of the mineral particles controls the length of these microfractures. In addition to undergoing deformation and displacement, the mineral grains may exhibit internal lattice dislocation or failure phenomena (Figure 1K).
3 Determining the formation period of shale tectonic fractures
3.1 Geological analysis
Based on the fracture orientation data measured from field outcrops resembling the target geological formation, applying structural geological theories to invert the ancient tectonic stress field is essential for establishing stress-matching relationships. In general, the intersection lines of conjugate shear fractures are parallel to the intermediate principal stress axis σ2. The maximum principal stress axis σ1 and the minimum principal stress axis σ3 are oriented along the angle bisectors of their acute and obtuse angles, respectively (Batayneh et al., 2012). This approach determines the maximum principal stress direction in the ancient tectonic stress regime. Utilizing image logging data for fracture orientation identification is currently one of the most accurate methods, particularly in areas where oriented core samples are unavailable. Based on the interpretation of fracture orientations from the imaging logging in the study area, generating a rose diagram of fracture orientations and analyzing the dominant orientations, combined with understanding the formation mechanisms of conjugate shear fractures, enables the accurate determination of the sequence of fracture formation.
Determining the sequence of fractures based on the crosscutting relationships observed between fractures in the outcrops, cores, thin slices, and other geological materials is one of the most fundamental and direct methods. In general, when determining the relative timing of fracture formation, the following principles are typically followed: If another fracture cuts a fracture, it forms earlier; If a fracture is truncated or terminated by another fracture, it forms later; If a fracture is not filled, it generally formed later. However, it is important to note that determining fracture sequences based on crosscutting relationships often represents only a portion of multiple-stage fractures in a single image. Comprehensive analysis is required to make accurate judgments.
Additionally, fault evolution and fracture development are controlled by the same regional structural stress field in the same geological region (Meier et al., 2015). They exhibit inheritance and consistency in terms of their origin and characteristics. Therefore, combining the fault characteristic can assist in analyzing the timing of tectonic fracture development. Generally, there is good correspondence between field outcrop analysis of similar exposures, multi-scale fracture crosscutting relationships, imaging log orientation analysis, and fault structural analysis.
3.2 Experimental testing analysis
In recent years, experimental testing has played a significant role in determining the timing of fracture formation. These experiments encompass a range of methods, including fluid inclusions, isotopes, AE, low-temperature thermochronology, and thermal history simulation, etc.
3.2.1 Experimental testing of fracture fillings
The minerals, such as calcite and quartz, found filling fractures in sedimentary rocks within oil and gas basins are the products of fluid migration, material transport, energy and substance exchange, and fluid-rock interactions during diagenesis. They represent the diagenetic response of these fractures to factors such as the geochemistry of diagenetic fluids, temperature-pressure conditions, and sources of materials at the time of their formation. Therefore, conducting experimental tests on fracture fillings can be utilized to analyze the timing of fracture formation. Additionally, it can provide insights into the dynamic evolution of temperature-pressure conditions and fluid redox environments during the burial and uplift processes of sedimentary strata within oil and gas basins.
3.2.1.1 Fluid inclusion testing
Fluid inclusions are portions of diagenetic or ore-forming fluids trapped within the lattice defects or cavities of minerals during their crystallization process. These inclusions remain sealed within the main mineral and have boundaries with the host mineral. They preserve geochemical information about the geological environment during their entrapment, including pressure, temperature, salinity, and more. Therefore, analyzing fluid inclusions can be employed to determine the timing of fracture formation, as they serve as valuable repositories of geological information related to the formation period (Fall et al., 2012).
Fluid inclusion temperature measurement is currently the most widely used non-destructive analysis method. Saltwater inclusions coexisting with oil and gas inclusions of different ages often have uniform temperatures, which can approximate the formation temperature of the reservoir at the time of inclusion trapping. Freeze-thaw analysis (freezing point depression) can be used to determine the original geological fluid type and salinity data preserved within these inclusions. Using the measured temperatures and referencing established fluid freezing point-salinity relationships, initial dissolution temperatures, and fluid type data, one can roughly infer the salinity and fluid type stored within the fluid inclusions. This information reflects different periods of fracture formation (Chen and Liu, 2021). However, it is important to note that there are limitations in this application, such as the difficulty in observing phase changes in some fluid inclusions during testing and the limited applicability range of empirical formulas for salinity-freezing point relationships.
3.2.1.2 Carbon and oxygen isotope testing
Fractures and faults in sedimentary rocks are crucial pathways for fluid movement in oil and gas basins. Under burial conditions, the formation of fractures allows underground saturated aquifer water to enter them first and flow along the fracture surfaces, resulting in the precipitation of mineral crystals on these surfaces. Different diagenetic environments and properties of diagenetic fluids during geological evolution lead to noticeable differences in the precipitated crystals’ carbon and oxygen stable isotope values. After identifying the stages and sequences of fractures within rock cores and assigning them to different periods, carbon and oxygen isotope experiments can be conducted on the corresponding fracture fillings to determine the distribution range of isotope values (Zhang et al., 2016; Kontakiotis et al., 2020; 2021). Combining this data with oxygen isotope thermometry and burial history analysis allows for determining the timing of fracture formation.
3.2.2 Rock acoustic emission experiment analysis
AE phenomena occur during the process of material stress and deformation. The essence of rocks’ AE phenomenon is derived from the expansion of internal micro-defects. Each time rocks are subjected to stress, their internal micro-fracture system, known as Griffith Microfracture, adapts to the magnitude and direction of the applied stress (Becker et al., 2014; Cai, 2020; Wang et al., 2022b). When rocks are subjected to stress below the threshold stress required to propagate pre-existing fractures or defects, there will be no further fracture development, and thus, no acoustic emission occurs. However, when the applied stress reaches or exceeds the threshold, the pre-existing fractures or defects will extend further, resulting in AE phenomena, known as the Kaiser effect. At this point, the stress value represents the paleo stress conditions during the previous fracture extension. Analyzing the number of Kaiser effect points on the AE response curve makes it possible to infer the stress-induced fracture periods and the paleo stress field strength that the rocks have experienced. In recent years, this method has been widely applied in studying fracture evolution history, fracture staging and correlation, and determining the stress field strength.
3.2.3 Low-temperature thermochronology analysis
In recent years, low-temperature thermochronology has been an important branch and cutting-edge field of geological dating research. It mainly involves two dating techniques: AFT and (U-Th)/He dating. The target minerals for this technique are primarily apatite, zircon, and titanite, with the first two being the most common. In sedimentary basins, the depth at which organic matter generates hydrocarbons corresponds to the partial annealing temperature zones of AFT and AHe systems. These systems exhibit a clear consistency, and a specific functional relationship (annealing sensitivity) exists between the length and density of mineral tracks and the sample’s history of uplift, erosion, and thermal events (Deng et al., 2016). Therefore, low-temperature thermochronology can effectively quantify a basin’s thermal history and the uplift and erosion processes. Based on low-temperature thermochronology measurements, it is possible to determine the sequence of tectonic movements and geological time, which can assist in identifying the timing of tectonic fracture formation.
The fission track dating method is based on the spontaneous fission of 238U in minerals and the resulting radiation damage to the host mineral. It involves analyzing the isotope ages that develop in minerals based on the spontaneous track density and the 238U content. Unlike conventional organic geochemical analysis methods, fission track dating can effectively record paleotemperatures and the time spent at these temperatures during the geological evolution of a geological body. It can provide insights into the paleotemperature evolution process at depths of 3–5 km near the Earth’s surface. (U-Th)/He dating, on the other hand, relies on the production of He through the decay of radioactive elements. In minerals, particularly apatite, the He primarily comes from the decay of 238U, 235U, and 232Th.
4 Discussion
4.1 Comprehensive determination of the fracture formation period
As previously mentioned, there are generally three methods for studying the formation period of tectonic fractures. However, individual methods for determining the fracture formation period have their limitations. Therefore, a comprehensive method for determining the fracture formation period based on macroscopic geological analysis and experimental testing has been established (Li et al., 2020; Li et al., 2021a; Hu et al., 2023).
1) Quantitative characterization of fracture parameters: The study begins with the quantitative characterization of fundamental parameters associated with the development of fractures at various scales. This quantification is achieved through a combination of methods, including seismic interpretation, field outcrop investigations, core observations, thin slices, scanning electron microscopy, and CT scanning, etc. Geometric quantitative relationships for characteristic parameters of tectonic fractures at different scales (I-II-III-IV) are established. 2) Integration of macroscopic and microscopic analyses: Following this, a fusion of macroscopic geological analysis is undertaken, which encompasses tectonic evolution analysis, the evaluation of fault and fold structures, and the examination of matching and crosscutting relationships among multi-scale fracture orientations. This macroscopic analysis is complemented by microscopic experimental techniques, including fluid inclusion and carbon-oxygen isotope testing of fracture fillings, rock AE experiments, (U-Th)/He low-temperature thermochronology, and AFT analysis, etc. 3) Cross-Verification and comprehensive determination: Through the combination of these diverse methods, the study cross-verifies and comprehensively determines the formation periods and geological times of fractures formed under multi-stage tectonic stress conditions. This integrative approach overcomes the limitations of individual techniques, providing a more holistic understanding of the timing of tectonic fracture formation.
Figure 2 provides a good example of a comprehensive method for determining the formation periods of tectonic fractures of Dingshan area in Sichuan Basin. Tectonic fractures (faults) are the result of stress and can generally be divided into four stages, which we define as four different scales (I, II, III, IV) ranging from micro to macro. The matching and crosscutting relationships of multi-scale fracture orientations indicate the presence of two formation periods. The AE experiments reveal five periods, after removing the influence of current stress, it can be determined as four periods. In addition, the Indosinian movement in the study area is mainly characterized by ascending and descending movements, with almost no fracture formation. Therefore, the AE experiment can be determined as third stage periods (Xie et al., 2019a; 2019b). The fluid inclusion homogenization temperatures show three periods, and the carbon and oxygen isotope tests indicate three periods. By combining the analysis of tectonic evolution and thermal history, we ultimately determined three formation periods for tectonic fractures in this area (Figure 2).
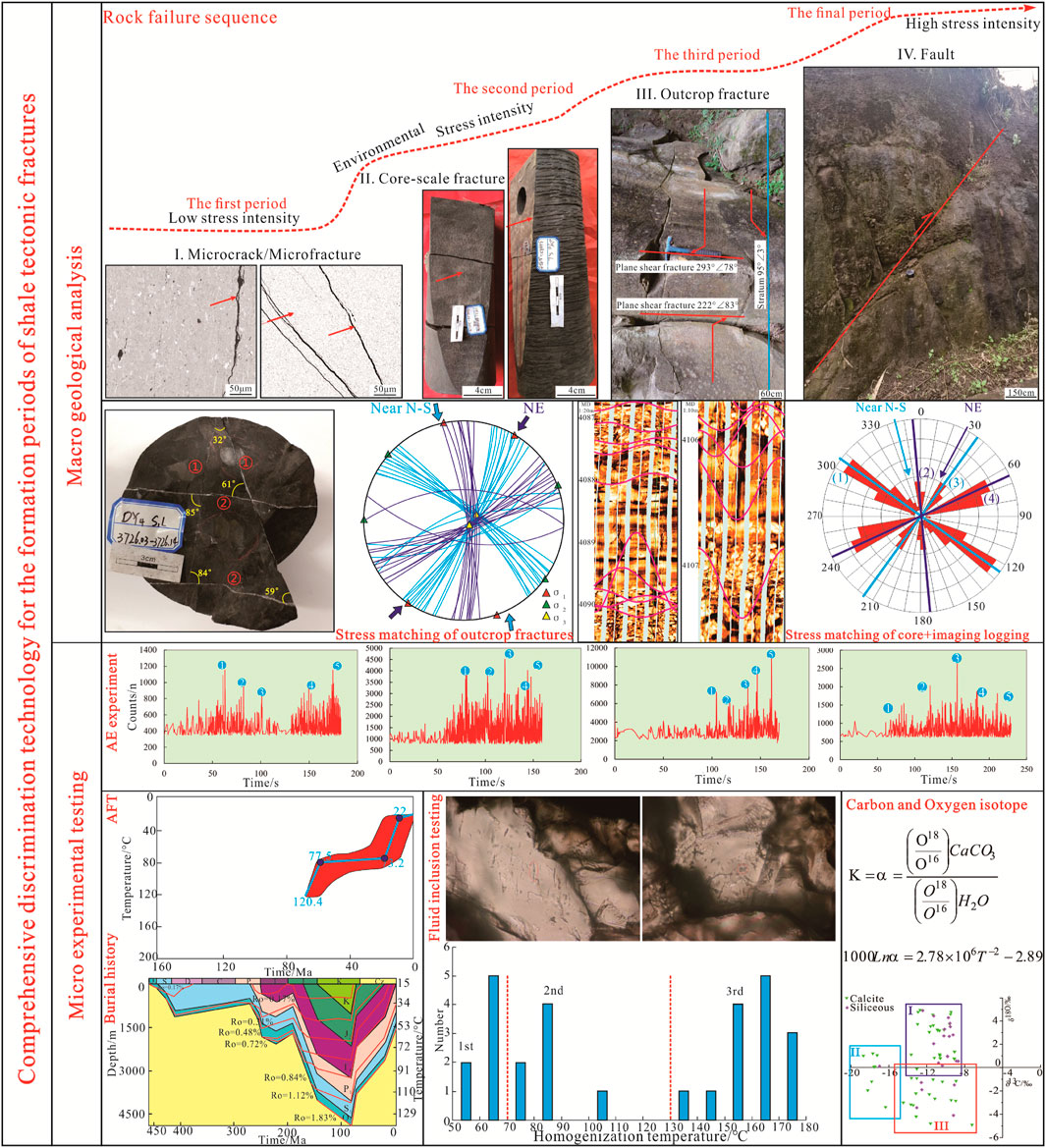
FIGURE 2. A comprehensive method for determining the formation periods of tectonic fractures in shale (Data sourced from Xie et al., 2019a; 2019b; Zhong, 2019; Xie, 2020).
4.2 Geological significance of the formation periods of shale tectonic fractures
The degree of fracture development is a crucial factor in the quality of shale gas reservoirs. Well-developed fractures can improve porosity and permeability by providing pathways for gas migration and storage near them in shale gas reservoirs that are not disrupted. The timing of tectonic fracture formation is of great significance for preserving and enriching shale gas. It forms the basis for analyzing the timing and evolution of shale gas accumulation (Li et al., 2019a; Li et al., 2019b).
Shale gas reservoir preservation is an intricate interplay of geological processes and is independent of the structural morphology. The post-formation dynamics of shale gas, particularly the timing and magnitude of subsequent structural uplifts, play a pivotal role in determining the reservoir’s integrity and gas content. Intricately linked to this is the timing of shale tectonic fracture formation. The interrelation between the emergence of these fractures and the extent of structural uplift underscores the notion that fracture formation timing profoundly influences gas content (Ma et al., 2022; Wood, 2022). Delving into the historical geology of China’s southern marine shale regions, two distinct phases emerge: an early phase dominated by continuous burial and a later phase characterized by consistent uplift (Li et al., 2019a; Wang et al., 2020). The extended deep burial in the initial phase acted as a catalyst for shale gas genesis. Contrarily, the subsequent structural evolution dictated the intensity and scope of shale gas migration. A notable consequence of stratigraphic uplift is the cessation of the hydrocarbon generation process within source rocks. Thus, the moment of this uplift is a key determinant in gauging potential gas losses from the reservoir. To elucidate, precocious uplifts would mean an extended exposure of shale gas reservoirs to late-stage geological alterations, culminating in a more intricate fracture network. This nuanced understanding of the temporal dynamics of uplift and burial offers invaluable insights for exploration strategies and reservoir management.
Post-uplift weathering and erosion decrease overlying strata pressure, upsetting the reservoir’s formation-pore pressure balance. This shift can lead to the opening of deep underground fractures (Li et al., 2019c; Oliveira et al., 2021; Yuan et al., 2021). Reduced temperature and pressure increase rock brittleness, making them prone to complex fractures under tectonic compression. Consequently, areas with significant strata uplift have enhanced fracture development and increased shale gas dissipation. In contrast, regions with minimal uplift exhibit limited fractures, preserving the shale gas reservoir’s overpressure (Li et al., 2019b). Tectonic fractures from different periods vary in orientation due to distinct tectonic stresses. The angle between fracture direction and the current maximum principal stress influences gas content. Large angles keep fractures mostly closed, preventing gas escape, while smaller angles or parallel alignments create open pathways for gas migration.
5 Conclusion and prospection
Shale tectonic fractures play a crucial role in the preservation and enrichment of shale gas, with fractures from different formation periods carrying distinct geological implications. Based on bibliometrics, this paper reviews the research techniques and methods associated with the formation periods of shale tectonic fractures. It establishes a comprehensive discrimination method for determining the formation period of these fractures, leading to the following conclusions and prospects.
(1) Tectonic fractures are the most important type of fractures in organic-rich shale, with predominant shear fractures. In field outcrops, they can be further divided into plane and profile shear fractures. Under microscopic examination, they mainly consist of grain-boundary fractures (intergranular fractures) and mineral joint fractures (intramineral fractures). Depending on the precision of observation, tectonic fractures can be categorized into four scales ranging from micro to macro, and establishing geometric quantitative relationships for I-II-III-IV level multi-scale fracture characteristics is of great significance for evaluating the distribution patterns of fractures.
(2) The analysis methods for determining the formation periods of tectonic fractures in shale primarily include field outcrop observations, core examinations, thin slice analysis, filling material analysis, and AE methods. However, each method has its own advantages and limitations when assessing the timing of fracture formation. Establishing a comprehensive determination approach based on macro-geological analysis and experimental testing often yields better results and is currently a mainstream method for studying the timing of tectonic fracture formation.
(3) The fracture formation period is paramount for preserving, enriching, and adjusting shale gas reservoirs. The formation of tectonic fractures often corresponds to tectonic uplift. Moderate uplift can promote the opening of I-II level fractures, improving the reservoir space and facilitating the desorption of adsorbed gas. However, excessive uplift can induce the formation and opening of III-IV level fractures, leading to shale gas loss and reducing the gas content of shale reservoirs. Additionally, the differences in fracture orientation at different periods, matching with the present maximum principal stress, can also impact the gas content of shale formations.
(4) Tectonic fractures are the smallest-scale features resulting from tectonic movements. Therefore, a comprehensive determination of the fracture formation period must rely on regional tectonic evolution as the fundamental constraint. Structural analysis plays a pivotal role in establishing when fractures form. This can be achieved through various methods, including field exposures, detailed seismic interpretation, low-temperature thermochronology, and the analysis of thermal evolution history (burial history and hydrocarbon generation history). These analyses help accurately assess the tectonic evolution history of the study area and determine the magnitude and direction of tectonic stress.
Data availability statement
The original contributions presented in the study are included in the article/Supplementary Material, further inquiries can be directed to the corresponding author.
Author contributions
HL: Conceptualization, Data curation, Formal Analysis, Funding acquisition, Investigation, Methodology, Project administration, Resources, Supervision, Visualization, Writing–original draft, Writing–review and editing.
Funding
The authors declare financial support was received for the research, authorship, and/or publication of this article. This study was financially supported by the Open funds of Shale Gas Evaluation and Exploitation Key Laboratory of Sichuan Province (Nos. YSK2023001 and YSK2022002) and Natural Gas Geology Key Laboratory of Sichuan Province (No. 2021trqdz05), the key R&D projects of the Deyang Science and Technology Plan (No. 2022SZ049), and the research project of Sichuan College of Architectural Technology (No. 2023KJ14).
Conflict of interest
The author declares that the research was conducted in the absence of any commercial or financial relationships that could be construed as a potential conflict of interest.
Publisher’s note
All claims expressed in this article are solely those of the authors and do not necessarily represent those of their affiliated organizations, or those of the publisher, the editors and the reviewers. Any product that may be evaluated in this article, or claim that may be made by its manufacturer, is not guaranteed or endorsed by the publisher.
References
Batayneh, A., Ghrefat, H., and Diabat, A. (2012). Lineament characterization and their tectonic significance using gravity data and field studies in the Al-Jufr Area, southeastern Jordan Plateau. J. Earth Sci. 23 (6), 873–880. doi:10.1007/s12583-012-0298-6
Becker, D., Cailleau, B., Kaiser, D., and Dahm, D. (2014). Macroscopic failure processes at mines revealed by acoustic emission (AE) monitoring. Bull. Seismol. Soc. Am. 104 (4), 1785–1801. doi:10.1785/0120130286
Cai, M. F. (2020). Key theories and technonogies for surrounding rock stability and ground control in deep mining. J. Min. Strata Control Eng. 2 (3), 033037. doi:10.13532/j.jmsce.cn10-1638/td.20200506.001
Chen, S. W., and Liu, J. Z. (2021). Research progress and prospects of the stages, genesis and fluid evolution of micro-fracture veins in petroliferous basins. Bull. Geol. Sci. Tech. 40 (4), 81–92. doi:10.19509/j.cnki.dzkq.2021.0426
Deng, B., Yong, Z. Q., Liu, S. G., Li, Z. W., Zhao, G. P., Mise, Z. H., et al. (2016). Cenozoic mountain-building processes in the Daliangshan, southeastern margin of the Tibetan Plateau: evidence from low-temperature thermochronology and thermal modeling. Chin. J. Geophys.-CH. 59 (6), 2162–2175. doi:10.6038/cjg20160621
Fall, A., Eichhubl, P., Cumella, S. P., Bodnar, R. J., Laubach, S. E., and Becker, S. P. (2012). Testing the basin-centered gas accumulation model using fluid inclusion observations: southern Piceance Basin, Colorado. AAPG Bull. 96 (12), 2297–2318. doi:10.1306/05171211149
Fan, C. H., Li, H., Qin, Q. R., He, S., and Zhong, C. (2020a). Geological conditions and exploration potential of shale gas reservoir in Wufeng and Longmaxi Formation of southeastern Sichuan Basin, China. J. Petrol. Sci. Eng. 191, 107138. doi:10.1016/j.petrol.2020.107138
Fan, C. H., Li, H., Zhao, S. X., Qin, Q. R., Fan, Y., Wu, J. F., et al. (2020b). Formation stages and evolution patterns of structural fractures in marine shale: case study of the Lower Silurian Longmaxi Formation in the Changning area of the southern Sichuan Basin, China. Energy Fuel 34 (8), 9524–9539. doi:10.1021/acs.energyfuels.0c01748
Fan, C. H., Xie, H. B., Li, H., Zhao, S. X., Shi, X. C., Liu, J. F., et al. (2022). Complicated fault characterization and its influence on shale gas preservation in the southern margin of the Sichuan Basin, China. Lithosphere 2022 (S12), 8035106. doi:10.2113/2022/8035106
Feng, Q. Q., Qiu, N. S., Borjigin, T., Li, F., Ji, R. Y., Liu, X., et al. (2023). Effect of tectonic reworking on shale fracturing and gas preservation in the upper Yangtze block, south China. Mar. Petrol. Geo. 148, 106069. doi:10.1016/j.marpetgeo.2022.106069
Gale, J. F. W., Laubach, S. E., Olson, J. E., Eichhubl, P., and Fall, A. (2014). Natural fractures in shale: a review and new observations. AAPG Bull. 98 (11), 2165–2216. doi:10.1306/08121413151
Gong, L., Wang, J., Gao, S., Fu, X. F., Liu, B., Miao, F. B., et al. (2021). Characterization, controlling factors and evolution of fracture effectiveness in shale oil reservoirs. J. Petrol. Sci. Eng. 203, 108655. doi:10.1016/j.petrol.2021.108655
He, S., Qin, Q. R., Li, H., and Wang, S. L. (2022b). Deformation differences in complex structural areas in the southern Sichuan Basin and its influence on shale gas preservation: a case study of Changning and Luzhou area. Front. Earth Sci. 9, 818155. doi:10.3389/feart.2021.818155
He, S., Qin, Q. R., Li, H., and Zhao, S. X. (2022a). Deformation differences in complex structural areas in the southern Sichuan Basin and its influence on shale gas preservation: a case study of changning and Luzhou areas. Front. Earth Sci. 9, 818543. doi:10.3389/feart.2021.818534
Hou, E. K., Cong, T., Xie, X. S., and Wei, J. B. (2020). Ground surface fracture development characteristics of shallow double coal seam staggered mining based on particle flow. J. Min. Strata Control Eng. 2 (1), 013521. doi:10.13532/j.jmsce.cn10-1638/td.2020.01.002
Hu, X. F., Deng, H. C., He, J. H., Shen, Z. M., and Peng, X. F. (2023). Complex genesis of multiperiod fractures in the middle triassic leikoupo formation in the pengzhou gas field, western Sichuan Basin, China. Petro. Sci. 20 (1), 161–176. doi:10.1016/j.petsci.2022.10.001
Huang, L. K., Tan, J., Fu, H. F., Liu, J. J., Chen, X. Y., Liao, X. C., et al. (2023). The non-plane initiation and propagation mechanism of multiple hydraulic fractures in tight reservoirs considering stress shadow effects. Eng. Fract. Mech. 292, 109570. doi:10.1016/j.engfracmech.2023.109570
Hui, G., Chen, Z., Chen, S., and Gu, F. (2022). Hydraulic fracturing-induced seismicity characterization through coupled modeling of stress and fracture-fault systems. Adv. Geo-Energy Res. 6 (3), 269–270. doi:10.46690/ager.2022.03.11
Kontakiotis, G., Karakitsios, V., Cornée, J. J., Moissette, P., Zarkogiannis, S. D., Pasadakis, N., et al. (2020). Preliminary results based on geochemical sedimentary constraints on the hydrocarbon potential and depositional environment of a Messinian sub-salt mixed siliciclastic-carbonate succession onshore Crete (Plouti section, eastern Mediterranean). Med. Geosc. Rev. 2, 247–265. doi:10.1007/s42990-020-00033-6
Kontakiotis, G., Karakitsios, V., Maravelis, A. G., Zarkogiannis, S. D., Agiadi, K., Antonarakou, A., et al. (2021). Integrated isotopic and organic geochemical constraints on the depositional controls and source rock quality of the Neogene Kalamaki sedimentary successions (Zakynthos Island, Ionian Sea). Med. Geosc. Rev. 3, 193–217. doi:10.1007/s42990-020-00045-2
Li, H. (2022). Research progress on evaluation methods and factors influencing shale brittleness: a review. Energy Rep. 8, 4344–4358. doi:10.1016/j.egyr.2022.03.120
Li, H. (2023). Coordinated development of shale gas benefit exploitation and ecological environmental conservation in China: a mini review. Front. Ecol. Evol. 11, 1232395. doi:10.3389/fevo.2023.1232395
Li, H., Qin, Q. R., Zhang, B. J., Ge, X. Y., Hu, X., Fan, C. H., et al. (2020). Tectonic fracture formation and distribution in ultradeep marine carbonate gas reservoirs: a case study of the Maokou Formation in the Jiulongshan Gas Field, Sichuan Basin, southwest China. Energy Fuel 34 (11), 14132–14146. doi:10.1021/acs.energyfuels.0c03327
Li, H., Tang, H. M., Qin, Q. R., Zhou, J. L., Qin, Z. J., Fan, C. H., et al. (2019a). Characteristics, formation periods and genetic mechanisms of tectonic fractures in the tight gas sandstones reservoir: a case study of Xujiahe Formation in YB area, Sichuan Basin, China. J. Petrol. Sci. Eng. 178, 723–735. doi:10.1016/j.petrol.2019.04.007
Li, H., Tang, H. M., and Zheng, M. J. (2019b). Micropore structural heterogeneity of siliceous shale reservoir of the Longmaxi Formation in the southern Sichuan Basin, China. Minerals 9, 548. doi:10.3390/min9090548
Li, H., Tang, H. M., Qin, Q. R., Wang, Q., and Zhong, C. (2019c). Effectiveness evaluation of natural fractures in Xujiahe Formation of Yuanba area, Sichuan basin, China. Arab. J. Geosci. 12 (6), 194. doi:10.1007/s12517-019-4292-5
Li, H., Wang, Q., Qin, Q. R., and Ge, X. Y. (2021a). Characteristics of natural fractures in an ultradeep marine carbonate gas reservoir and their impact on the reservoir: a case study of the Maokou Formation of the JLS Structure in the Sichuan Basin, China. Energy Fuel 35 (16), 13098–13108. doi:10.1021/acs.energyfuels.1c01581
Li, H., Zhou, J. L., Mou, X. Y., Guo, H. X., Wang, X. X., An, H. Y., et al. (2022a). Pore structure and fractal characteristics of the marine shale of the Longmaxi Formation in the changning area, southern Sichuan Basin, China. Front. Earth Sci. 10, 1018274. doi:10.3389/feart.2022.1018274
Li, H. T., Peng, R., Du, W. S., Li, X. P., and Zhang, N. B. (2021b). Experimental study on structural sensitivity and intervention mechanism of mechanical behavior of coal samples. J. Min. Strata Control Eng. 3 (4), 043012. doi:10.1638/td.20210820.001
Li, J., Li, H., Yang, C., Ren, X. H., and Li, Y. D. (2023). Geological characteristics of deep shale gas and their effects on shale fracability in the Wufeng–Longmaxi Formations of the southern Sichuan Basin, China. Lithosphere 2023 (1), 4936993. doi:10.2113/2023/4936993
Li, J., Li, H., Yang, C., Wu, Y. J., Gao, Z., and Jiang, S. L. (2022b). Geological characteristics and controlling factors of deep shale gas enrichment of the Wufeng-Longmaxi Formation in the southern Sichuan Basin, China. Lithosphere 2022 (S12), 4737801. doi:10.2113/2022/4737801
Ma, J., Fang, D. Z., Zhang, P. X., Gu, H. T., Hu, C. F., and Lu, B. (2022). Characteristics and genesis of shale fractures in Wufeng-Longmaxi formations of Yangchungou structural belt in Southeast Chongqing. Nat. Gas. Geosci. 33 (7), 1117–1131. doi:10.11764/j.issn.1672-1926.2022.03.001
Meier, S., Bauer, J. F., and Philipp, S. L. (2015). Fault zone characteristics, fracture systems and permeability implications of Middle Triassic Muschelkalk in Southwest Germany. J. Struct. Geo. 70, 170–189. doi:10.1016/j.jsg.2014.12.005
Meng, Q. F., Hao, F., and Tian, J. Q. (2021). Origins of non-tectonic fractures in shale. Earth Sci. Rev. 222, 103825. doi:10.1016/j.earscirev.2021.103825
Oliveira, G. P., Rodrigues, T. N. E., and Lie, K.-A. (2021). GAWPS: a MRST-based module for wellbore profiling and graphical analysis of flow units. Adv. Geo-Energy Res. 6 (1), 38–53. doi:10.46690/ager.2022.01.04
Shan, S. C., Wu, Y. Z., Fu, Y. K., and Zhou, P. H. (2021). Shear mechanical properties of anchored rock mass under impact load. J. Min. Strata Control Eng. 3 (4), 043034. doi:10.13532/j.jmsce.cn10-1638/td.20211014.001
Tan, P., Fu, S. H., Chen, Z. W., and Zhao, Q. (2023). Experimental investigation on fracture growth for integrated hydraulic fracturing in multiple gas bearing formations. Geoenergy Sci. Eng. 231, 212316. doi:10.1016/j.geoen.2023.212316
Tan, P., Jin, Y., and Pang, H. W. (2021). Hydraulic fracture vertical propagation behavior in transversely isotropic layered shale formation with transition zone using XFEM-based CZM method. Eng. Fract. Mech. 248, 107707. doi:10.1016/j.engfracmech.2021.107707
Wang, R. Y., Ding, W. L., Zhang, Y. Q., Wang, Z., Wang, X. H., He, J. H., et al. (2016b). Analysis of developmental characteristics and dominant factors of fractures in Lower Cambrian marine shale reservoirs: a case study of Niutitang formation in Cen'gong block, southern China. J. Petrol. Sci. Eng. 138, 31–49. doi:10.1016/j.petrol.2015.12.004
Wang, R. Y., Gu, Y., Ding, W. L., Gong, D. J., Yin, S., Wang, X. H., et al. (2016a). Characteristics and dominant controlling factors of organic-rich marine shales with high thermal maturity: a case study of the Lower Cambrian Niutitang Formation in the Cen'gong block, southern China. J. Nat. Gas. Sci. Eng. 33, 81–96. doi:10.1016/j.jngse.2016.05.009
Wang, R. Y., Hu, Z. Q., Sun, C. X., Liu, Z. B., Zhang, C. C., Gao, B., et al. (2018). Comparative analysis of shale reservoir characteristics in the Wufeng-Longmaxi (O3w-S1l) and Niutitang (Є1n) Formations: a case study of wells JY1 and TX1 in the southeastern Sichuan Basin and its neighboring areas, southwestern China. Interpretation-J. Sub. 6 (4), SN31–SN45. doi:10.1190/INT-2018-0024.1
Wang, R. Y., Nie, H. K., Hu, Z. Q., Liu, G. X., Xi, B. B., and Liu, W. X. (2020). Controlling effect of pressure evolution on shale gas reservoirs:A case study of the Wufeng–Longmaxi Formation in the Sichuan Basin. Nat. Gas. Ind. 40 (10), 1–11. doi:10.3787/j.issn.1000-0976.2020.10.001
Wang, X., Li, J., Jiang, W., Zhang, H., Feng, Y., and Yang, Z. (2022a). Characteristics, current exploration practices, and prospects of continental shale oil in China. Adv. Geo-Energy Res. 6 (6), 454–459. doi:10.46690/ager.2022.06.02
Wang, S. L., Li, H., Lin, L. F., and Yin, S. (2022b). Development characteristics and finite element simulation of fractures in tight oil sandstone reservoirs of Yanchang Formation in western Ordos Basin. Front. Earth Sci. 9, 823855. doi:10.3389/feart.2021.823855
Wood, D. A. (2022). Predicting brittleness indices of prospective shale formations from sparse well-log suites assisted by derivative and volatility attributes. Adv. Geo-Energy Res. 6 (4), 334–346. doi:10.46690/ager.2022.04.08
Wu, S., Li, T. T., Ge, H. K., Wang, X. Q., Li, N., and Zou, Y. S. (2019). Shear-tensile fractures in hydraulic fracturing network of layered shale. J. Petrol. Sci. Eng. 183, 106428. doi:10.1016/j.petrol.2019.106428
Xia, Y., and Lu, A. H. (2022). Analysis of the mechanical properties and damage characteristics of continuously graded high-strength cemented backfill. J. Min. Strata Control Eng. 5 (1), 013037. doi:10.13532/j.jmsce.cn10-1638/td.20221212.002
Xie, J. T. (2020). Shale fractures in complex tectonic zones and their effects on shale gas preservation - a case study of Longmaxi Formation in Dongxi-Songkan area of southeast Sichuan. PhD dissertation. Sichuan: Southwest Petroleum University. doi:10.27420/d.cnki.gxsyc.2020.000008
Xie, J. T., Qin, Q. R., and Fan, C. H. (2019a). Quantitative prediction of fracture distribution of the Longmaxi Formation in the Dingshan Area, China-using FEM numerical simulation. Acta Geol. sin.-eng. Ed. 93 (6), 1662–1672. doi:10.1111/1755-6724.13815
Xie, J. T., Qin, Q. R., and Fan, C. H. (2019b). Influencing factors of gas adsorption capacity of marine organic shale: a case study of Dingshan Area, southeast Sichuan. J. Coast. Res. 93, 507–511. doi:10.2112/SI93-066.1
Xu, S., Gou, Q. Y., Hao, F., Zhang, B. Q., Shu, Z. G., and Zhang, Y. Y. (2020b). Multiscale faults and fractures characterization and their effects on shale gas accumulation in the Jiaoshiba area, Sichuan Basin, China. J. Petrol. Sci. Eng. 189, 107026. doi:10.1016/j.petrol.2020.107026
Xu, S., Hao, F., Shu, Z. G., Zhang, A. H., and Yang, F. (2020a). Pore structures of different types of shales and shale gas exploration of the Ordovician Wufeng and Silurian Longmaxi successions in the eastern Sichuan Basin, South China. J. Asian Earth Sci. 193, 104271. doi:10.1016/j.jseaes.2020.104271
Xu, S., Liu, R., Hao, F., Engelder, T., Yi, J. Z., Zhang, B. Q., et al. (2019). Complex rotation of maximum horizontal stress in the Wufeng-Longmaxi Shale on the eastern margin of the Sichuan Basin, China: implications for predicting natural fractures. Mar. Petrol. Geol. 109, 519–529. doi:10.1016/j.marpetgeo.2019.06.008
Yuan, Y. Y., Li, H., Wang, Y., Xiang, H., Xu, G. H., and Bian, Y. Y. (2021). Study on the influence of lithofacies types on the formation and evolution of fracture: a case study of Longmaxi Formation in Northwest Guizhou. Chin. J. Geol. 56 (1), 82–97. doi:10.12017/dzkx.2021.006
Zhang, Q. Y., Liang, B., Qin, F. R., Cao, J. W., Dan, Y., and Li, J. R. (2016). Environmental and geochemical significance of carbon and oxygen isotopes of Ordovician carbonate paleokarst in Lunnan, Tarim Basin. Enviorn. Earth .Sci. 75 (14), 1074. doi:10.1007/s12665-016-5882-0
Zhong, C. (2019). Characteristics of shale fractures in the Longmaxi Formation in the Dingshan area of southeastern Sichuan and their relationship with gas bearing properties. MA Thesis. Sichuan: Southwest Petroleum University. doi:10.27420/d.cnki.gxsyc.2019.000349
Zhu, B. Y., Meng, J. H., Pan, R. F., Hu, H. Y., Song, C., Zhu, Z. P., et al. (2023). New insights into the evaluation criteria for high-quality deep marine shale gas reservoirs in the Longmaxi formation: evidence from organic matter pore development characteristics. Front. Ecol. Evol. 11, 1138991. doi:10.3389/fevo.2023.1138991
Keywords: tectonic fractures, formation timing, geochemistry, low-temperature thermochronology, fracture fillings, shale, complex structural areas
Citation: Li H (2023) Deciphering the formation period and geological implications of shale tectonic fractures: a mini review and forward-looking perspectives. Front. Energy Res. 11:1320366. doi: 10.3389/fenrg.2023.1320366
Received: 12 October 2023; Accepted: 02 November 2023;
Published: 10 November 2023.
Edited by:
Peng Tan, CNPC Engineering Technology R&D Company Limited, ChinaReviewed by:
Fuhua Shang, Inner Mongolia University of Technology, ChinaShang Xu, China University of Petroleum (East China), China
Ruyue Wang, SINOPEC Petroleum Exploration and Production Research Institute, China
Copyright © 2023 Li. This is an open-access article distributed under the terms of the Creative Commons Attribution License (CC BY). The use, distribution or reproduction in other forums is permitted, provided the original author(s) and the copyright owner(s) are credited and that the original publication in this journal is cited, in accordance with accepted academic practice. No use, distribution or reproduction is permitted which does not comply with these terms.
*Correspondence: Hu Li, bGlodTg2MDYyOEAxMjYuY29t