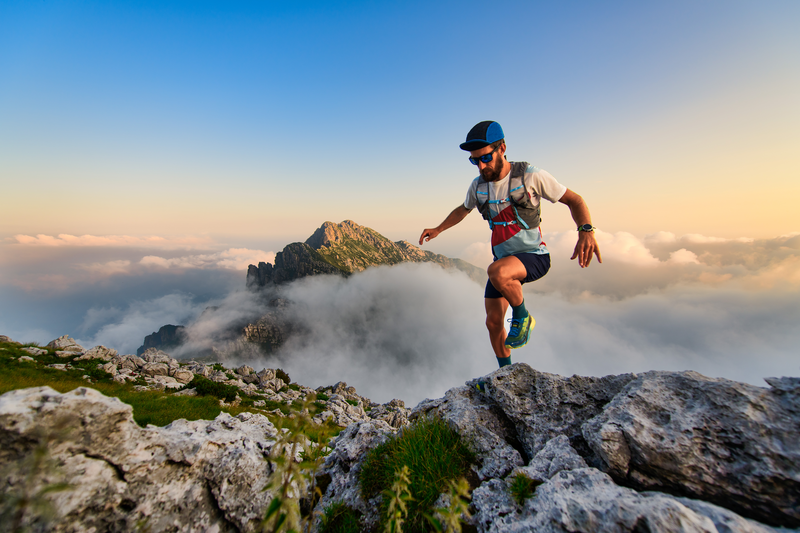
94% of researchers rate our articles as excellent or good
Learn more about the work of our research integrity team to safeguard the quality of each article we publish.
Find out more
ORIGINAL RESEARCH article
Front. Energy Res. , 01 November 2023
Sec. Advanced Clean Fuel Technologies
Volume 11 - 2023 | https://doi.org/10.3389/fenrg.2023.1297738
This article is part of the Research Topic Advances in Geomechanics Research and Application for Deep Unconventional Reservoirs View all 35 articles
Surfactant imbibition can effectively improve the development effect of fracturing technique. In order to enhance oil recovery, a temperature resistant surfactant was selected using interfacial tension and contact angle testers based on the offshore reservoir conditions of high temperature and low permeability. Experiments on spontaneous and forced imbibition with artificial columnar cores were performed to optimize parameters of imbibition processes during fracturing in high temperature and low permeability reservoirs. Results show that among the three surfactants, amphoteric Gemini surfactant had a stable molecular structure with non-compressible hydrophobic groups which significantly reduced oil-water interfacial tension, enhanced rock wettability, and demonstrated good resistance to high temperatures The maximum recovery increase during amphoteric Gemini surfactant imbibition is 16.22%. The higher the core permeability, the higher the core inside pressure, and the longer the well shut-in time, the greater the forced imbibition effect. A proper well shut-in time and injection volume should be considered when using surfactant solution as fracturing liquid.
China’s demand for oil and gas resources increases annually and offshore oilfields occupy an important position in the oil and gas supply field. Continuous development in China’s offshore oilfields has resulted in a greater proportion of crude oil reserves in low-permeability reservoirs, as well as increasing development difficulties (Cao et al., 2022; Han et al., 2022; Lin et al., 2022). Block Z of the Bohai Oilfield is characterized by high-temperature and low-permeability. Its oil has low density, low viscosity, low sulfur content, high wax content, and a high solidification point (Wei and Liu, 2019; Zhong et al., 2020; Xu et al., 2021). Besides, this reservoir is a structural layered reservoir with a complex oil-water relationship influenced by fault cutting, along with poor inter-well connectivity (Abbassi et al., 2022). During the initial stage of oil production, crude oil has good properties, a strong self-jet ability, a high production ability, and a long period of low water-cut (Dontsov, 2022; Ma et al., 2022). But, when a well encounters water, its production capacity falls off significantly and quickly. A fracturing technique is an effective way to decrease the seepage resistance (Tan et al., 2023; Huang et al., 2023a), and then increase the production of low permeability oil wells (Tan et al., 2021; Huang et al., 2023b). However, hundreds of cubic meters of guar gum or gel fracturing fluid can not only supply the formation energy, but also reduce the matrix permeability near the fracture due to its wall building property. Therefore, the current offshore fracturing technique has limited oil-increasing effects because of reservoir physical properties. The method to use surfactant solution as fracturing liquid to enhance imbibition function has shown to be an effective strategy to further improve oil recovery.
Due to the effectiveness of imbibition of surfactant in the fracturing fluid to develop a low permeability reservoir, petroleum technology professionals have studied spontaneous imbibition extensively under the condition of unconnected wells. Wang et al. developed a static imbibition mathematical model for the numerical simulation of spontaneous imbibition, which considered the influence of imbibition driving forces such as capillary force and gravity on imbibition oil recovery (Wang et al., 2022; Marzhan et al., 2023). Through spontaneous imbibition experiments, Xu et al. investigated the mechanism of core imbibition oil displacement under different permeabilities, and concluded that better pore structure can enhance spontaneous imbibition (Xu and Ma, 2015). Li et al. used numerical modeling to explore the influence of fracturing fluid interfacial tension on spontaneous imbibition in low permeability reservoirs (Li et al., 2018). Based on low field nuclear magnetic resonance testing, Wang et al. carried out on-line scanning of core nuclear magnetic resonance under five boundary conditions. Zhu et al. studied the effects of different hydrophilic and hydrophobic structures on spontaneous imbibition in high temperature, high salinity and low permeability reservoirs (Zhu et al., 2021). Cai et al. analyzed the discriminant parameters of spontaneous imbibition mechanism including interfacial tension and wetting angle (Cai and Yu, 2012).
Currently, spontaneous imbibition is the main experimental technique used to explore imbibition in low-permeability cores, and the experiment temperature is often not more than 100°C. There are few studies considering external fluid pressure on the imbibition effect in high-temperature and low-permeability reservoirs (Gushchin et al., 2018; Bai et al., 2021; Liu et al., 2023). Therefore, in view of the characteristics of high temperature, insufficient productivity and disconnection between wells in Block Z of Bohai Oilfield, this paper studied the imbibition development effect of high temperature resistant surfactant through spontaneous and forced imbibition experiments. The type and concentration of imbibition agent suitable for target reservoir were optimized by static imbibition and forced imbibition physical simulation experiments. The influence mechanism of factors such as shut-in pressure, shut-in time and core permeability on the forced imbibition effect. The related study can provide theoretical basis for the practical construction in Block Z.
Experimental agents include: lauryl alcohol sodium sulfate (anionic), effective content of 30%; lauric acid (anionic), effective content of 40%; Gemini surfactant (amphoteric), content of 40%; heavy water (D2O), deuterium element could effectively shield nuclear magnetic signal, effective content of 99.9%.
The water used in spontaneous imbibition and forced imbibition is simulated injection water, and is prepared by using distilled water and heavy water, respectively. The ionic composition of injection water is shown in Table 1. The experimental oil was prepared by mixing degassed crude oil and light hydrocarbon in Bohai oilfield, with a viscosity of 5.0 mPa s at 138°C.
In this study, the effects of the surfactant imbibition ratio on spontaneous imbibition and forced imbibition were investigated using artificial hydrophilic columnar cores cemented by quartz sands (Ø2.5 × 5.0 cm) (Han et al., 2016; Xie et al., 2018). The permeabilities of these cores were approximately 20mD, 60mD, respectively, and their pore structures and permeabilities were comparable to reservoir cores in the Bohai oilfields. The procedures to prepare the artificial cores can be found in the Chinese patent “Quartz sand epoxy resin cementation heterogeneity model making method”.
The IFT between the oil and water was measured at 138 °C using a TX500HP high temperature interfacial tension meter. The contact angle was measured at 138 °C with an OCA20 optical contact angle measuring instrument using a sessile drop method. Additionally, spontaneous imbibition recovery was measured using an imbibition cell made of high-temperature and pressure-resistant glass (as shown in Figure 1). The smallest division values of this imbibition cell were 0.005 mL. The forced imbibition test equipment mainly includes ISCO injection pump, confining pressure pump, intermediate vessels, pressure sensor and core online displacement nuclear magnetic resonance analyzer (MacroMr12-150H–I, Niumag Analytical Instrument, Suzhou, China), with the experimental temperature of 138 °C. The nuclear magnetic pictures of T2 spectrum before and after forced imbibition were compared. Changes in T2 spectrum are used to determine the relative content change of crude oil at different pores scales in the core. The experimental equipment and procedures are shown in Figure 2.
1) The dried cores were weighed before and after being saturated with simulated injection water prepared by distilled water. The pore volume was calculated through measuring the weight difference of the core sample.
2) Each core was placed into the core holder, saturated with crude oil, and aged with crude oil at 138 °C for 24 h to prepare it for use.
3) For spontaneous imbibition, an oil-aged core was placed inside an imbibition cell at 138 °C. The imbibition cell was filled with a surfactant solution to a desired height.
4) The volume of produced oil was monitored and recorded as often as appropriate. The imbibition recovery was calculated based on the volume of produced oil.
The specific experimental procedures of forced imbibition are as follows:
1) The dried core was weighed before and after being saturated with simulated water prepared by heavy water. The pore volume could be calculated by measuring the weight difference.
2) Core was placed into a core holder and was saturated with oil at reservoir temperature until it comes out at the outlet. The crude oil injection speed was 0.02 mL/min. After the oil saturation process, the core was aged with the crude oil at 138 °C for 24 h to prepare it for use. The core was placed into the core holder of NMR analyzer, and then the initial T2 spectrum was tested;
3) The experimental surfactant solution prepared by heavy water was heated to the temperature of 80 °C in advance.
4) The surfactant solution was injected at 0.2 mL/min into the core holder, the outlet was shut at the same time. When the injection pressure reaches the set pressure, close the inlet for a designed shut-in time to simulate forced imbibition during well shut;
5) Outlet valve was opened until no liquid flows out after the shut-in time reached the designed time, the T2 spectrum after forced imbibition was tested.
6) The forced imbibition effect of core was analyzed by comparing the change of T2 spectrum in different size pore-throat.
During NMR testing, the signal amplitude of transverse relaxation time T2 spectrum reflects the relative saturation of residual oil in pores of different sizes. Additionally, the decreasing amplitude of the T2 spectrum at various time intervals is used to quantify crude oil recovery. . . Regions with T2 relaxation times less than 10 m are defined as small pores, and regions with T2 relaxation times greater than 10 m are defined as large pores (Xie et al., 2022). The T2 spectrum of the initial stage and stage after well shut was entered into a computer image recognition program. The relative movable oil of different size pores was obtained by comparing the area of T2 spectrum between initial stage and stage after well shutting. According to Figure 3, the oil recovery can be calculated using following formula:
where R1, R2, R refers to the oil recovery of small pore, large pore, and total pore. S1, S1’ refers to the final area of T2 spectrum and differential area between beginning and ending time in small pore. S2, S2’ refers to the final area of T2 spectrum and differential area between beginning and ending time in small pore.
Three surfactants at concentrations of 0.1%, 0.2% and 0.3% were prepared using simulated injection water at 138°C reservoir temperature. Surfactant molecular structures and interfacial tension test results are shown in Figure 4 and Table 2 respectively.
As shown in Figure 4 and Table 2, the concentrations of the surfactant solutions and the interfacial tension are negatively correlated. At a concentration of 0.2%, the interfacial tension follows the trend: sodium lauryl alcohol sulfate > lauric acid > amphoteric Gemini surfactant. A greater oil-water interface layer results from a change in the structure of diffusion electric double layers due to high temperature, which also causes the hydrophobic group of chain structures to curl. As a result of the surfactants’ low temperature tolerance, the interfacial tension rises. In contrast with the previous two surfactants, the amphoteric surfactant has a higher degree of hydrophobic chain branching and a more stable bond energy (C-C bond 332 kJ/mol). It also has a temperature-resistant sulfonic acid group (-SO32-) in its hydrophobic chain. The surfactant’s hydrophobic chain is capable of maintaining a stretch state at high temperatures due to its stable molecular structure. Additionally, the hydrophobic group is difficult to be compressed which is good for high temperature resistance.
The standing time of different surfactants of 0.3% concentration under the temperature of 138 °C was changed, and then the interfacial tension between them and crude oil was tested by interfacial tension meter can be used in high temperature and high pressure. The test results are shown in Table 3.
As shown in Table 3, the standing time of the surfactant solutions at high temperature has an effect on the oil-water interfacial tension. After standing for 5 days, the interfacial tension of amphoteric Gemini surfactant can still obtain an oil-water interfacial tension of 10−3 mN/m, showing the best temperature resistance.
Columnar cores with a permeability of 20 mD were cut into several thin slices of 2 mm thickness using a mechanical cutting machine, and the initial contact angle is 65.2° which means a weaker water-wet. The cores were immersed in three surfactants of 0.1%, 0.2% and 0.3% concentrations for 24 h at 138 °C. The variation of contact angle on the core surface in relation to the type and concentration of surfactant is shown in Table 4.
TABLE 4. Relationship between contact angle, contact angle difference and surfactant concentration (°).
As shown in Table 4, the contact angle of the rock surface decreases with the increase of surfactant concentration. When the surfactant concentration is 0.2%, the contact angle shows the following trend: sodium lauryl sulfate > lauric acid > amphoteric Gemini surfactant. Compared with the two anionic surfactants sodium lauryl sulfate and lauric acid, amphoteric Gemini surfactant can effectively improve the wettability of the core surface and make the core surface more water-wet. .It is challenging to determine the imbibition effectiveness solely based on physicochemical properties since the influencing factors during the surfactant imbibition process are complex. Therefore, spontaneous imbibition experiments are used to further compare and optimize the types and concentrations of surfactants for offshore oilfields.
Columnar cores were immersed in the surfactant solution in the high temperature imbibition bottle placed in the temperature of 138°C. The results of core spontaneous imbibition recovery experiments are shown in Table 5.
In static spontaneous imbibition, amphoteric Gemini surfactant had the maximum oil recovery among the three surfactants, as indicated in Table 5. When the surfactant concentration was 0.2%, the oil recovery of the amphoteric Gemini surfactant solution was 16.22%. A mechanism study shows that improving imbibition oil recovery can be achieved by properly increasing the surfactant concentration and reducing interfacial tension. However, too low interfacial tension will limit the capillary force, which is detrimental for imbibition. In addition, when the surfactant concentration exceeds the critical micelle concentration, the effective concentration decreases and the imbibition effect deteriorates. Further analysis revealed that surfactants can change the wettability of the rock pore surface to more water-wet resulting in the reduction of the adhesion of oil phase on the rock surface. This causes an even force field to form around the oil droplets in the pore-throat, which facilitates the oil film’s easier separation from the rock’s surface and increases imbibition.On the other hand, surfactants have the ability to significantly lower the capillary resistance and adherence of the rock surface to oil droplets by lowering the interfacial tension between oil and water. This increases the number of capillaries, enhancing the deformability of oil droplets, improving the flow ability of the oil phase, and thus increasing the imbibition oil recovery. Based on the above results, 0.2% amphoteric Gemini surfactant is recommended for subsequent experimental studies taking into account the technical and economic factors.
1) Influence of core permeability
0.2% of amphoteric Gemini surfactant was prepared by simulated formation water using heavy water. The inlet was closed when the core inside pressure reached a pressure of 15MPa, and then outlet was opened after a shut-in time of 24 h. When the outlet has no fluid to flow, the T2 spectrum was tested. The effect of core permeability on the forced imbibition and corresponding T 2 spectrum are shown in Table 6 and Figure 5.
As shown in Table 6 and Figure 5, as core permeability increases, the pore throat radius increases, oil has a lower flow resistance, more oil can be produced through imbibition under the function of capillary and displacement, and the forced imbibition recovery rate increases. According to the analysis, oil in large pore areas is primarily dependent on the displacement of surfactant, so the small pore area has a higher oil recovery than large pore areas, and the oil recovery in large pore areas decreases with the decrease of core permeability. The analysis also shows that small pore areas have a stronger capillary force, which is helpful to enhance imbibition function. Because the initial saturated oil volume is larger and the large pore area has a larger pore volume, oil recovery from the large area accounts for the majority of overall oil recovery.
2) Influence of core inside pressure
The reservoir inside pressure has an obvious effect on imbibition for it will influence the crossflow between large pore area and small pore area. Different volumes of fracturing fluid will lead to a different reservoir inside pressure. The core inside pressure was changed in the experiments to study the proper injection volume of fracturing fluid. A concentration of 0.2% amphoteric Gemini surfactant was prepared by simulated formation water using heavy water. The inlet was closed when the core inside pressure reached a pressure of 5, 10 and 15MPa, and the outlet was opened after a shut-in time of 24 h. When the outlet had no fluid flowing, the T2 spectrum was tested. The effects of core inside pressure on forced imbibition and corresponding T 2 spectrum are shown in Table 7 and Figure 6.
As shown in Table 7, the forced imbibition oil recovery increases with increasing core insidepressure. The forced imbibition recovery can reach 27.42% at a core inside pressure of 15 MPa. The analysis presents that the surfactant injection volume increases with the increase in core inside pressure. On the one hand, more pore volume is displaced and water-wet degree of more area is improved, more pores will happen imbibition, oil recovery in small pore area and large pore area becomes higher. On the other hand, a larger core inside pressure can supply more energy for fluid after shut-in time, which is helpful for overcoming flow resistance.
As shown in Figure 6, the T2 spectrum following forced imbibition exhibits a declining tendency when compared to the T2spectrum of the first stage. The decreasing amplitude becomes larger as the core inside pressure increases and as core inside pressure increases, surfactant solution volume injected into the core increases. Due to the strong connection between different pores in the large pore area and the displacement of the larger pore volume, the oil recovery from the large pore area is increased. Besides, as the core inside pressure rises, the imbibition area between the large and small pore throats will be stronger due to a higher core inside pressure, increasing the likelihood that oil will be recovered from the small pore area. Therefore, a proper reservoir pressure should be considered when designing surfactant solution injection volume.
3) Influence of shut-in time
When employing surfactant solution as the fracturing fluid, the well shut-in time affects the reservoir interior pressure, hence the shut-in period should be optimised. A concentration of 0.2% amphoteric Gemini surfactant was prepared by simulated formation water using heavy water. The inlet was closed when the core inside pressure reached a pressure of 15MPa, and the outlet was opened after a shut-in time of 24h, 48 and 72h, respectively. When the outlet had no fluid flowing, the T2 spectrum was tested. The effects of core inside pressure on forced imbibition and the corresponding T2 spectrum are shown in Table 8 and Figure 7.
The oil recovery via forced imbibition is obviously influenced by the well shut-in duration, as illustrated in Table 8 and Figure 7, respectively. As a well’s shut-in duration increases, the rate of oil recovery rises. After a 72-h shut-in period, the forced imbibition oil recovery can reach 50.88%, which is higher than the forced imbibition recovery of 27.42% after a 24-h shut-in time. As the pressure diffuses during shut-in, surfactant solution can flow into more pore volume and displace the oil, causing crossflow between small and large pore areas. The oil recovery increasing amplitude of the big pore area is greater than that of the small pore area because the oil produced by displacement is more than the oil produced by imbibition. Therefore, a proper shut-in time should be considered when designing a well shut-in time.
The surfactant used in fracturing liquid can obviously improve the effect of increasing oil in offshore oilfields with low permeability and high temperature, the surfactant type, construction, spontaneous imbibition effect and forced imbibition effect should be considered at the same time.
1) As the concentration of the three surfactant increases, the IFT decreases, and the water-wet ability becomes stronger. Amphoteric Gemini surfactant has superior temperature resistance compared to sodium lauryl alcohol sulphate and lauric acid because of its stable molecular structure and the difficulty compressing its hydrophobic group.
2) In spontaneous imbibition, it was found that amphoteric Gemini surfactant showed by far the highest oil recovery of the three surfactants tested. At a concentration of 0.2% of the surfactant, amphoteric Gemini can achieve spontaneous oil recovery of 16.22% of the oil.
3) Imbibition oil recovery can be improved by appropriately increasing the surfactant concentration and decreasing interfacial tension, however, too low interfacial tension will limit the capillary force, which is undesirable for imbibition.
4) The forced imbibition effect is influenced by the core permeability, the core inside pressure and the well shut-in time. The high core permeabilities, high core inside pressures, and long well shut-in times results into better forced imbibition effect. When employing surfactant solutions as a fracturing liquid, proper well shut-in time and injection volume should be taken into account.
The original contributions presented in the study are included in the article/supplementary material, further inquiries can be directed to the corresponding author.
YL: Conceptualization, Formal Analysis, Investigation, Methodology, Writing–original draft. JZ: Project administration, Supervision, Writing–review and editing. XL: Conceptualization, Formal Analysis, Writing–original draft, Writing–review and editing. SG: Methodology, Writing–review and editing. LZ: Writing–review and editing. XH: Writing–review and editing.
The author(s) declare financial support was received for the research, authorship, and/or publication of this article. Project of Exploration and Research on Fracturing Technology of Enhancing Energy and Improving Efficiency in Bohai Oilfield (CCL2021TJT0NST2278). This work was supported by Tianjin Branch Research Institute.
The authors declare that the research was conducted in the absence of any commercial or financial relationships that could be construed as a potential conflict of interest.
All claims expressed in this article are solely those of the authors and do not necessarily represent those of their affiliated organizations, or those of the publisher, the editors and the reviewers. Any product that may be evaluated in this article, or claim that may be made by its manufacturer, is not guaranteed or endorsed by the publisher.
Abbassi, F. T., Karrech, A., Islam, M. S., and Seibi, A. C. (2022). Poromechanics of fractured/faulted reservoirs during fluid injection based on continuum damage modeling and machine learning. Nat. Resour. Res. 32 (1), 413–430. doi:10.1007/s11053-022-10134-8
Bai, Y., Pu, C. S., and Liu, S. (2021). Carboxyl/alkyl composite silica-based amphiphilic nanoparticles enhanced imbibition of low spontaneous permeability sandstone at reservoir conditions. Colloids Surfaces A Physicochem. Eng. Aspects 2021, 629. doi:10.1016/j.colsurfa.2021.127504
Cai, J. C., and Yu, B. M. (2012). Advances in studies of spontaneous imbibition in porous media. Adv. Mech. 42 (6), 735–754. doi:10.6052/1000-0992-11-096
Cao, G. S., Wu, J. J., Zhang, N., Sui, Y., and Bai, Y. (2022). Study on migration characteristics of oil gas water three-phase interface and distribution law of remaining oil in gas cap edge water reservoir. Energy Sources, Part A Reserv. Util. Environ. Eff. 44 (3), 6362–6377. doi:10.1080/15567036.2022.2090642
Dontsov, E. V. (2022). Analysis of a constant height hydraulic fracture driven by a power-law fluid. Rock Mech. Bull. 1 (1), 100003. doi:10.1016/j.rockmb.2022.100003
Gushchin, P. A., AntonovS, V., Mishin, A. S., Khamidullina, V., Gushchina, Y. F., and Khlebnikov, V. N. (2018). Physicochemical mechanisms of light oil oxidation during extraction from high-temperature low-permeability oil reservoirs. Chem. Technol. Fuels Oils 54 (1), 64–71. doi:10.1007/s10553-018-0899-6
Han, D. W., Lu, X. G., Wang, T. T., et al. (2016). Difference of pore structure between artificial cores and natural cores and its influence on the seepage characteristics of oil displacement agent. Petroleum Geol. Effic. Recovery 23 (4), 82–87. doi:10.13673/j.cnki.cn37-1359/te.2016.04.013
Han, H. S., Chen, X. L., Ji, Z. M., Li, J., Lv, W., Zhang, Q., et al. (2022). Experimental characterization of oil/gas interface self-adjustment in CO2-assisted gravity drainage for reverse rhythm reservoir. Energies 15 (16), 5860. doi:10.3390/en15165860
Huang, L. K., He, R., Yang, Z. Z., Tan, P., Chen, W., Li, X., et al. (2023b). Exploring hydraulic fracture behavior in glutenite formation with strong heterogeneity and variable lithology based on DEM simulation. Eng. Fract. Mech. 278, 109020. doi:10.1016/j.engfracmech.2022.109020
Huang, L. K., Tan, J., Fu, H. F., Liu, J., Chen, X., Liao, X., et al. (2023a). The non-plane initiation and propagation mechanism of multiple hydraulic fractures in tight reservoirs considering stress shadow effects. Eng. Fract. Mech. 292, 109570. doi:10.1016/j.engfracmech.2023.109570
Li, A. F., He, B. Q., and Lei, Q. H. (2018). Influence of interfacial tension on water-imbition in low-wet permeability reservoirs. J. China Univ. Petroleum Ed. Nat. Sci. 42 (4), 67–74. doi:10.1016/j.engfracmech.2022.109020
Lin, R. Y., Jia, Y., and Sun, L. (2022). Experimental study on the seepage characteristics of multilayer commingled production and influencing factors of the development effect in low-permeability tight sandstone gas reservoirs. ACS omega 7 (38), 34080–34088. doi:10.1021/acsomega.2c03273
Liu, Y., Yang, D., Liang, C., Li, Y., He, M., Jia, J., et al. (2023). A scientometric review on imbibition in unconventional reservoir: a decade of review from 2010 to 2021. . Process. 11 (3), 845. doi:10.3390/pr11030845
Ma, X., Zhang, S., Zhang, X., Liu, J., Jin, J., Cheng, W., et al. (2022). Lithology-controlled stress variations of Longmaxi shale–Example of an appraisal wellbore in the Changning area. Rock Mech. Bull. 1 (1), 100002. doi:10.1016/j.rockmb.2022.100002
Marzhan, K., Razieh, K., and Peyman, P. (2023). A review of wettability alteration by spontaneous imbibition using low-salinity water in naturally reservoirs. Energies 16 (5), 2373. doi:10.3390/en16052373
Tan, P., Fu, S. H., Chen, Z. W., and Zhao, Q. (2023). Experimental investigation on fracture growth for integrated hydraulic fracturing in multiple gas bearing formations. Geoenergy Sci. Eng. 2023, 212316. doi:10.1016/j.geoen.2023.212316
Tan, P., Jin, Y., and Pang, H. W. (2021). Hydraulic fracture vertical propagation behavior in transversely isotropic layered shale formation with transition zone using XFEM-based CZM method. Eng. Fract. Mech. 248, 107707. doi:10.1016/j.engfracmech.2021.107707
Wang, Q., Zhao, J. Z., and Hu, Y. Q. (2022). Numerical simulation of static spontaneous seepage at core scale. J. Petroleum 43 (6), 860–870. doi:10.7623/syxb202206010
Wei, R., and Liu, C. J. (2019). Techniques for low efficiency well management in complex formation of BZ34-5 oilfield. Prospect. Eng. Geotech. Drill. Eng. 46 (6), 47–52. doi:10.12143/j.tkgc.2019.06.009
Xie, K., Lu, X. G., Pan, H., Han, D., Hu, G., Zhang, J., et al. (2018). Analysis of dynamic imbibition effect of surfactant in microcracks of reservoir at high temperature and low permeability. SPE Prod. Operations 33 (03), 596–606. doi:10.2118/189970-pa
Xie, K., Mie, J., Cao, W. J., Cao, B., Yao, L., Zhang, B., et al. (2022). Improving oil mechanism of polymer gel fracturing fluid based on filtration displacement. J. petroleum Sci. &engineering 218, 111030. doi:10.1016/j.petrol.2022.111030
Xu, J. H., and Ma, L. L. (2015). Spontaneous imbibition in Efficitured low permeability reservoir. Petroleum Geol. Recovery Reserv. 22 (3), 111–114. doi:10.13673/j.cnki.cn37-1359/te.2015.03.020
Xu, K., Lu, Y. J., Chang, J., and Li, Y. (2021). Research progress of high temperature resistant fracturing fluid system. J. Phys. Conf. Ser. 2076 (1), 012039. doi:10.1088/1742-6596/2076/1/012039
Zhong, L. G., Zhu, T. Y., Hao, T. C., Zhang, X., Wang, X., and Zhang, L. (2020). Prediction method of formation pressure for the adjustment well in the reservoir with fault. J. Phys. Conf. Ser. 1707 (1), 012012. doi:10.1088/1742-6596/1707/1/012012
Keywords: surfactants, imbibition effect, offshore oilfields, high temperature, low permeability, physical simulation
Citation: Liu Y, Zou J, Lan X, Gao S, Zhang L and He X (2023) Research on imbibition effect of surfactant fracturing fluid in offshore reservoirs with low permeability and high temperature. Front. Energy Res. 11:1297738. doi: 10.3389/fenrg.2023.1297738
Received: 20 September 2023; Accepted: 02 October 2023;
Published: 01 November 2023.
Edited by:
Peng Tan, CNPC Engineering Technology R & D Company Limited, ChinaReviewed by:
Mengfei Zhou, The University of Texas at Austin, United StatesCopyright © 2023 Liu, Zou, Lan, Gao, Zhang and He. This is an open-access article distributed under the terms of the Creative Commons Attribution License (CC BY). The use, distribution or reproduction in other forums is permitted, provided the original author(s) and the copyright owner(s) are credited and that the original publication in this journal is cited, in accordance with accepted academic practice. No use, distribution or reproduction is permitted which does not comply with these terms.
*Correspondence: Xitang Lan, bGFueGl0YW5nNTIyQDE2My5jb20=
Disclaimer: All claims expressed in this article are solely those of the authors and do not necessarily represent those of their affiliated organizations, or those of the publisher, the editors and the reviewers. Any product that may be evaluated in this article or claim that may be made by its manufacturer is not guaranteed or endorsed by the publisher.
Research integrity at Frontiers
Learn more about the work of our research integrity team to safeguard the quality of each article we publish.