- 1School of Economics and Management, Northwest Agricultural and Forestry University, Yangling, China
- 2Professional School of Agroindustrial Engineering, National University of Huancavelica, Huancavelicau, Peru
- 3Professional School of Economic Engineering and Business, Universidad Científica del Sur, Lima, Peru
- 4Universidad César Vallejo, Trujillo, Peru
- 5Professional School of Management, Universidad San Ignacio de Loyola, La Molina, Peru
- 6Professional School of Management, Universidad Privada del Norte, Trujillo, Peru
- 7Department of Applied Social Sciences, Faculty of Organization and Management, Silesian University of Technology, Za-brze, Poland
Natural disasters, characterized by their escalating frequency and intensification, fueled relentlessly by the inexorable forces of climate change, unambiguously underscore the urgent need to reassess our built environments and meticulously revise infrastructure design to withstand the unforgiving onslaught of these extreme events, thereby demanding concerted attention and unwavering dedication from policymakers and academic researchers to navigate this formidable predicament. The current study delves into the exploration of how natural disaster impact the course of energy technology evolution. To achieve this, This investigation uses panel data from 1990–2020 and sophisticated econometric analyses to model the effect of calamities on energy technology advancement across BRICS countries. Rigorous empirical methodologies lend credibility regarding the observed detrimental impact of disasters on innovation. The preliminary findings of the investigation suggest that natural disasters potentially exert a markedly detrimental effect on energy technology innovation. This influence is not merely confined to the year of the disaster occurrence but persistently lingers for the subsequent 4-year period. Moreover, the adverse impacts of such environmental upheavals on energy evolution seem to be mitigated by various factors. These include robust economic growth, government stability, interpersonal globalization, financial openness, trade openness, and the expansion of social globalisation. Adding further dimension to this research is the understanding that epidemics have the most pronounced negative implications on energy innovation. Concluding on a nuanced note, the study underscores a significant inverse relationship between natural disasters and low-carbon energy innovation. Interestingly, however, there is no discernible correlation when it comes to non-low carbon energy innovation. In a nutshell, this research presents a potential reference guide for policymakers, illuminating pathways to enhance the degree of energy innovation while tackling natural disasters and ensuring environmental sustainability.
1 Introduction
Natural disaster frequency and intensity, fueled by climate change, underscore the pressing need to reassess our built environments and revise infrastructure design to withstand such extreme events, demanding concerted attention from both policymakers and academic researchers. A discernible rise in their occurrence has been witnessed, leading to growing disruptions in societal operations and impacting human lives. The profound implications of these events on societal production and livelihoods underscore the escalating urgency to address this global concern. Data presented by Chen, Li (Chen et al., 2021) reveal a 13-fold escalation in global natural catastrophes between 1960 and 2019, paralleled by a greater than 35-fold surge in affected human populace. In a pinnacle year, natural disasters rose to 900, leaving an impact on approximately 660 million individuals. It is thus imperative that patterns and consequences of disaster occurrences be studied from socio-economic vantage points for both theoretical insight and pragmatic application.
Contemporary research on natural disasters has predominantly concentrated on evaluating their impact across various sectors such as political arenas, social spheres, and economic growth. To elucidate, in the economic context, natural disasters influence crucial variables like international reserves, investor response, the insurance market, fiscal revenue, and GDP growth rate, as indicated in numerous studies (Cavallo and Noy, 2011; Strobl, 2012; Chang et al., 2013; Hood et al., 2013; Felbermayr and Gröschl, 2014; Strobl et al., 2020). Social ramifications include escalated conflict levels and population out-migration trends that are intrinsically tied to disaster incidents (Boustan et al., 2020). Politically, a positive correlation exists between the severity of disasters and an upsurge in terrorist activities and political instability (Drury and Olson, 1998; Berrebi and Ostwald, 2011).
Nevertheless, it is noteworthy that a substantial gap persists in empirical research exploring the relationship between natural disasters and their effect on technological and scientific advancements. To our best knowledge, a pioneering empirical study by Chen, Li (Chen et al., 2021) has initiated investigation into the interplay between natural disasters and technological innovation. In this study, an unbalanced panel dataset comprising multiple countries was utilized, and the system GMM estimator was employed for analysis.
Their results provide compelling evidence of natural disasters substantially impeding innovation. However, their research scope was primarily country-level analysis, thereby not delving into the impact of disasters on specific industry-based technological advancements. In addition, potential endogeneity problems arising from omitted variables were not addressed. It is crucial to recognize that energy serves as the foundation and the catalyst for economic growth, experiences an increase in consumption during natural disasters (Doytch and Klein, 2017), while simultaneously witnessing a decrease in the extent of technological transfer (Cuaresma et al., 2008). In light of these findings, our study seeks to augment the pioneering work by Chen, Li (Chen et al., 2021), focusing specifically on the relationship between advancements in energy innovation and natural disasters, utilizing patent data pertaining to energy technology.
Albeit not the sole determinant, technological innovation significantly shapes the future of clean energy, offering substantial societal benefits such as mitigating climate change, improving air quality, and bolstering energy security (Sagar and van der Zwaan, 2006; Feng et al., 2021). These benefits are particularly critical in nations susceptible to frequent natural disasters (Lee and Law, 2017).
The introduction of innovation on a national scale in the energy sector has the potential to strengthen energy security. This could be achieved by boosting the modest domestic energy production and by curtailing reliance on imported energy. In the sphere of energy financing, the role of technological innovation is pivotal in making investment and financing decisions (Johnstone et al., 2009; Wen et al., 2021a; Wen et al., 2021b; Wen et al., 2022). As a case in point, clean energy technology has been a critical venture capital component since the late 1990s (Rai et al., 2015). The intricacy and profoundness of technological innovation within the energy sector call for substantial financial backing. The determinants of energy innovation are multifaceted, involving investment in technological innovation, technician communication, and a stable external environment among others (Costa-Campi et al., 2014; Lin and Chen, 2019; Bai et al., 2020; Ren et al., 2021). These factors may also serve as conduits through which natural disasters indirectly affect technological innovation outcomes and prospects.
The discipline of disaster economics underscores that, while disasters’ detrimental effects are inextricably linked with societal and economic development, the concept of turning adversity into advantage posits that transforming disaster damage into advantages can serve as a measure of disaster management, embodying a positive perspective towards disaster response (Tang et al., 2021). By examining the influence of natural disasters on energy innovation, our study aims to identify strategies to not only minimize the inflicted damage but also potentially convert these adversities into long-term positive impacts. In light of the escalating occurrence of natural disasters and the supreme significance of innovation in the energy sector, a deeper probe into their interrelation is crucial and warrants considerable focus and debate. This area has been largely overlooked in existing scholarly works, and our study aims to fill this research gap. Figures 1, 2 illustrate the escalating human and economic impacts of natural disasters from 1900 to 2020. Figure 1 shows the dramatic increase in global population affected by catastrophes over this period. Figure 2 quantifies the rise in financial costs of disasters, representing the tremendous economic losses that amplify the humanitarian toll.
This study employs a two-way fixed effect model to examine the BRICS region during the time span from 1990 to 2020. In this empirical research, patents associated with energy technology are considered as the dependent variable, whereas the proportion of the population affected by natural disasters is the independent variable. Our preliminary findings suggest a significant negative relationship between natural disasters and energy innovation, an effect observed not only in the year of the disaster but persisting for the subsequent 4 years. Validating the robustness of our results, we conducted multiple tests using alternative dependent variables, applying varied quantitative methodologies, and introducing additional influencing factors.
Delving into the underlying mechanisms, our empirical analysis indicates that natural disasters impede energy innovation via various channels, including economic development level, government stability, interpersonal globalization, financial openness, and trade openness. Additional insights reveal that energy innovation is significantly negative effect is exerted by pandemics. Furthermore, natural disasters exhibit no correlation with non-low carbon energy innovation but are observed to be negatively associated with low-carbon energy innovation. Our research thus extends valuable insights into the complex nexus between natural disasters and energy innovation.
The present manuscript offers seminal contributions to scholarly literature, drawing from distinct aspects. Initially, to the best of our understanding, the extant body of work is devoid of studies exploring the direct correlation of natural calamities and innovation in energy sector, with the existing studies providing tangential evidence regarding this relationship. Controversies persist surrounding the positive or negative interplay of natural disasters on energy innovation. Hence, we embark on pioneering empirical research elucidating this direct relationship, utilizing data from the Emergency Events Database. Our findings underscore the detrimental effect of natural disasters on energy innovation, thereby providing empirical confirmation of their relationship and potentially resolving the contentious academic discourse on this subject.
Secondarily, our study primarily hinges upon the two-way panel fixed effects model for the empirical analysis. Aiming to rectify potential endogeneity issues and dynamic specification issues inherent in variables, we additionally utilize the system Generalized Method of Moments (GMM) estimator to verify the robustness of our primary empirical result, an effective tool for handling autocorrelation and heteroscedasticity. We employ other robustness test methods such as adding omitted variables and alternative dependent variable specification.
Finally, this study extend our analysis beyond merely the global impact of natural disasters on energy innovation and scrutinize the effects of assorted disaster types including storms, floods, extreme temperatures, epidemics, earthquakes, and droughts. Our additional concern lies in the duration of the impact caused by disasters on energy innovation, as it is the time lag effect that we primarily focus on. The long-term effects may persist for a period of up to 4 years. The presence of distinct factors exert varying influences on the innovation of low-carbon energy and non-low-carbon energy, constituting an intriguing discovery. Our study provides supplementary evidence that fills a gap in the existing energy sector literature by examining the impacts of natural disasters. We posit that our findings will assist governments and businesses in revitalizing energy innovation output post unforeseen disasters.
The significance of this study is threefold. First, it address a gap in existing literature by providing empirical evidence on the relationship between natural disasters and innovation in energy sector. Second, the combination of panel data with utlization of the rigorous econometrics analysis for endogeneity problem represent methodological contribution. Lastly, the granular analysis of disaster types, time lags, and differences between low-carbon and non-low-carbon technologies provides novel insights.
Our objective is to uncover the mechanisms through which natural disasters impact innovation in energy technology, by deriving valuable insights from the extant studies. The prevalent body of currently establishes a connection between natural disasters and various economic indices, implying a well-developed body of research on the financial ramifications of such events. It is unequivocal that powerful natural disasters precipitate significant direct economic damage, a metric frequently utilized to quantify disaster intensity. Furthermore, the economic detriments incurred from catastrophic natural events have potential ripple effects on economic expansion (Khan et al., 2019). Economic losses from severe natural disasters can also have ripple effects on economic growth (Skidmore and Toya, 2002; Khan et al., 2020). Inevitably, following a disaster, governments increase their expenditures to rebuild affected regions and provide assistance to victims. This has a direct impact on government spending allocated to economic development. Nevertheless, it is important to note that energy technology innovation relies heavily on a favorable economic condition (Raghupathi and Raghupathi, 2017; Kochmańska, 2019). A robust economy is capable of providing more public investments and government funds, thereby strengthening energy innovation efforts (Wen et al., 2020). Therefore, the level of economic development plays a significant role in linking natural disasters to energy innovation. Furthermore, natural disasters could debilitate economic development levels by heightening aid expenditure and inducing economic losses. These factors do not contribute positively to the performance of energy innovation.
The economic consequences of calamitous events are apparent not just evident in the gross domestic product but also affect trade. Governments often increase border security and trade expenses after natural disasters, resulting in reduced cross-country trade flow (Mochizuki and Chang, 2017). The adverse effects of natural disaster have a less severe and lasting impact on merchandise trade than on service trade. This discrepancy is particularly evident in sectors characterized by capital-intensive services (Xu and Kouwoaye, 2019). Trade openness serves as a significant conduit in mediating the impact of natural disasters on energy innovation, given the close association between energy innovation and service trade. Through engaging innovative talents from other countries for their energy innovation endeavors, firms can harness the benefits of trade openness. Furthermore, empirical studies have indicated that regions characterized by higher levels of trade openness exhibit greater resilience in the face of natural disasters, thereby mitigating potential macroeconomic consequences (Noy, 2009). In the long-term, negative economic impacts from natural disasters can extend to FDI and government finances (Khan et al., 2020). Natural disasters can pose a significant obstacle to FDI inflows (Skidmore and Toya, 2002; Kukułka, 2014). Moreover, fiscal policies may require adjustment to aid in disaster recovery. This unpredictability, stemming from fiscal policy changes, can substantially decrease investment in new energy innovation (Wen et al., 2022).
Investments, particularly in the form of FDI, serve as key drivers for energy research and development (R&D), given their inherent association with innovation and supportive role in innovative endeavors as highlighted by (Antonietti et al., 2014). Consequently, financial openness is a reflection of energy R&D initiatives and outcomes, allowing us to identify the impact of natural disasters on energy innovation through financial openness. Interpersonal globalization can also serve as a channel through which natural disasters influence energy innovation. The chaotic aftermath of such disasters can deter scientific professionals from focusing on technological innovation projects (Haddad and de Alcântara Teixeira, 2015; Gualandri and Kuzior, 2023; Kuzior et al., 2023). Beyond responding to unexpected domestic shocks, Innovators might also take action based on experiences with natural disasters in other regions, such as suspending technology exchange programs due to unexpected disasters, or ceasing planned overseas exchanges (Miao et al., 2019).
Natural disasters typically lead to significant damage to infrastructure (Robinson et al., 2015), including both ICT and transportation networks. Such damage can obstruct the sharing of innovative information and hinder collaboration between innovators, thereby impeding technological advancement (J et al., 2016; Lee and Lee, 2015). Consequently, natural disasters can adversely impact energy innovation by limiting communication and learning opportunities among technological innovators globally, reducing the extent of interpersonal globalization.
Lastly, in the context of this study, it is important to recognize the influential role of political stability in the association between natural disasters and energy innovation. Major disasters often lead to increased political turmoil, as they expose societal vulnerabilities (Drury and Olson, 1998). Natural disasters can exacerbate social disagreements and resource deficits, disrupt everyday routines and societal structures, which may trigger social instability and conflict (Bhavnani, 2006). Significantly, in nations with mixed political systems and lower-middle incomes, natural disasters may significantly amplify the likelihood of domestic conflicts (Nel and Righarts, 2008).
Such societal challenges can lead to skepticism regarding governmental competence, potentially undermining its stability. More critically, natural disasters may incite fear by highlighting societal and governmental weaknesses that could be exploited by terrorist groups (Berrebi and Ostwald, 2011). Nevertheless, political conditions play a crucial role in energy transition, which influences energy innovation as delineated in (Normann, 2015). Specifically, a transition towards a more sustainable economic growth pathway can be facilitated by government-imposed environmental regulations, which can stimulate the consumption of clean energy (Wang and Lee, 2022). Intriguingly, the vested interests of governmental actors and internal governmental conflicts also emerge as crucial factors in energy transitions.
Government instability often leads to corruption, and a governance system filled with blatant corruption and poor bureaucracy generally results in substandard innovation outcomes (Wen et al., 2021b; Wen et al., 2022). Therefore, the presence of top-tier governmental institutions is crucial in sparking levels of innovation (Lee and Law, 2017; Kuzior et al., 2021; Ober and Kochmańska, 2021). In its core, natural calamities can unsettle the steadiness of a government, a state that is necessary for the progression of energy technology innovation.
In summation, this study’s contributive aspects to the corpus of literature are threefold. Primarily, to the best of our knowledge, it is the inaugural empirical investigation to utilize the Emergency Events Database (EM-DAT). The aim is to analyze the intricate interplay between natural disasters and the advancements in energy innovation, shedding new light on this vital relationship. Moreover, this study effectively bridges a gap in literature and offers solid evidence to pacify the persistent scholarly surrounding this phenomenon.
Secondly, the two-way panel fixed effect model, complemented by the system GMM estimator, is employed as the research approach in the empirical analysis. This methodology enables a thorough examination of the initial empirical results, addressing concerns related to dynamic specification and endogeneity issue in the variables. Furthermore, it proves to be effective in handling issues such as autocorrelation and heteroscedasticity, ensuring robustness in the analysis.
Thirdly, we provide an exhaustive analysis of the overall effect of natural disasters on energy innovation and take into consideration the impacts of different disaster types and their potential long-term effects. An intriguing finding pertains to the differential influences on low- and non-low carbon energy innovation.
Hypothesis 1:. The perturbations induced by natural calamities impact energy advancement through conduits such as economic evolution level, trade openness, financial openness, interpersonal globalization, and governmental stability.
Academic discourses exhibit conflicting perspectives concerning the temporal impact of natural calamities. Conventional wisdom posits a negative repercussion on economic growth ensuing from natural calamities in the short term (Noy, 2009; Cavallo and Noy, 2011). However, empirical investigation of the investment-producing destruction hypothesis unveils scenarios where calamities resulting in substantial property and capital losses paradoxically propel short-term economic evolution (Noy and Vu, 2010). Mid-term examination of natural calamities’ influence by Porfiriev (Porfiriev, 2016) reveals a decline in the GDP growth rate, with subsequent economic expansion unable to entirely mitigate the losses. This underscores the temporal lag effect inherent to the impact of natural calamities. Besides economic repercussions, energy consequences of natural calamities also manifest temporal lag effects. Preceding research validates that half a decade post a calamity-induced shock, positive ramifications on energy consumption become evident (Doytch and Klein, 2017; Chiu and Lee, 2020; Wang et al., 2022). Contrastingly, the long-term consequences of natural calamities maintain an aura of uncertainty. Evidence from Akao and Sakamoto (Akao and Sakamoto, 2018) suggests that efficient resource allocation can prevent adverse effects on long-term growth rates due to natural calamities. In cases where a calamity predominantly wreaks havoc on physical capital, economic growth might even experience a positive stimulation. Through an examination of the temporal effects of natural calamities, we establish our next postulate.
Hypothesis 2:. Natural disasters’ considerable impact on energy innovation manifests a certain temporal lag effect.
The multiplicity and magnitude of natural disasters lead to a diverse range of impacts. Some academicians advocate for a nuanced approach towards discussing the ramifications of natural disasters, suggesting that the effects should be examined separately for different disaster types. The majority of studies validate the adverse effects of various natural disasters, focusing primarily on contrasting their magnitude and duration. The collated GeoMet data by Felbermayr and Gröschl (Felbermayr and Gröschl, 2014) contributes to the substantiation of the significant and consistently negative influence of catastrophic natural disasters, such as massive meteorological and earthquakes calamities, on economic growth. Concerning technological advancement, effects of climatic disasters on technology transfer differ from those brought on by geological disasters (Cuaresma et al., 2008). Chen, Li (Chen et al., 2021) investigates the differentiation in impacts on innovation caused by various types of natural disasters and their findings reveal that technological innovation is most significantly affected by epidemics, displaying a pronounced adverse effect. Additionally, in addition to assessing the detrimental consequences of diverse disasters, several research works emphasize the favorable outcomes associated with specific disasters.
For instance, events like floods exhibit a limited positive impact on GDP growth (Cunado and Ferreira, 2011). Moreover, climatic disasters have demonstrated a positive correlation with economic growth, as highlighted in reference (Skidmore and Toya, 2002). Hence, we propose categorizing natural disasters and formulating subsequent hypotheses.
Hypothesis 3:. Depending on their types, natural disasters instigate varied effects on energy innovation.
Research regarding energy intensity unveils a varied landscape across industries, and a dissimilar connection between renewable and non-renewable energy, prompting us to explore the varying impacts of natural disasters on an array of energy technologies (Wen et al., 2017; Wen et al., 2021a; Wen et al., 2021b). It is understood that a surge in renewable energy demand spurs energy innovation output (Aflaki et al., 2015). Evidence also suggests that the renewable energy sector in wealthy countries (Chen et al., 2019), the residential energy sector in middle-income countries, and the industrial energy sector in low-income countries will experience positive effects on energy consumption (Doytch and Klein, 2017). Different types of energy consumption are distinctly affected by natural disasters, and likewise, have diverse impacts on energy technology innovations. Pressure from external events on governmental structures provides prospects for innovative renewable energy technology. Furthermore, there is a growing emphasis on low-carbon energy technology to achieve environmental conservation goals, emissions reduction, and energy saving (McJeon et al., 2011; Howell et al., 2014), steering energy innovation investments towards this sector. However, financial investments in low-carbon energy innovation may be displaced by governmental spending on rebuilding after natural disasters. Consequently, the impacts of natural disasters on low- and non low carbon energy technologies can differ significantly. Drawing upon the discussed literature, we formulate our primary hypothesis.
Hypothesis 4:. Different types of energy technology innovations are affected differently by natural disasters.
Despite the absence of explicit evidence linking disasters and energy innovation, numerous studies confirm the influence of natural disasters on financial openness, social globalization, trade liberalization, governmental stability, and economic growth. These factors are considered crucial in understanding the relationship between natural disasters and energy innovation. Although literature have examined the factors influencing energy innovation, none have accounted for the impact of natural disasters. Therefore, we propose a comprehensive model that integrates various types of disasters as potential drivers of energy innovation, enabling empirical research to be conducted.
The remaining sections of this manuscript are structured as follows. Section 2 outlines the variables, its data and sources and empirical approaches employed for estimation utilized in our study. Section 3 presents the empirical results, analysis of heterogeneity, mechanism tests, and robustness checks. Finally, in Section 4, we conclude based on the results obtained and provide policy recommendations.
2 Materials and methods
2.1 Data and variables
The EM-DAT database is the source of data on natural disasters, encompassing information from BRICS countries since 1990. This database is extensively employed in empirical research and holds a prominent position due to its rigorous inclusion criteria, distinguishing it from other natural disaster databases (Raddatz, 2007; Barredo, 2009; Busby et al., 2018). Data pertaining to energy innovation variables, encompassing energy technology, is procured from the BRICS energy report. This study serves as a repository of country-specific data concerning technology pertaining to energy and the environment (Panwar and Sen, 2019). Sourcing data from the BRICS energy report, which encompasses information at the national level, enables the inclusion of energy technology within the ambit of energy innovation variables related to the environment. For this study, we select the following variables explained below.
2.1.1 GINI framework
The dichotomy between the price effect and scale effect is ascribed as the principal mechanism underlying the influence of income inequality on innovation (Bertola et al., 2005). On one side, expanding market demand reduces industry monopoly. Companies that are adaptable not only survive but also operate optimally, thus motivating an increasing number of companies to engage in innovative endeavors in a competitive environment (Cook and Uchida, 2008). To demonstrate that the income gap stimulates innovation, we highlight the price effect, indicating that a widening income gap leads to an increased number of high-income individuals purchasing innovative products. Consequently, we monitor the Gini coefficient and its data is sourced from the World Income Inequality Database, represented by GINI.
2.1.2 Urban
A substantial degree of urbanization has been determined to be a salient catalyst in the amplification of technological innovation (Wang et al., 2021). This assertion equally holds true in regards to the influence on energy technological innovation. In light of this, we utilize the ratio of the urban population to the overall population as a means of gauging the urbanization rate, as represented by the proxy variable ‘Urban’.
2.1.3 Industry
There exists a reciprocal relationship between industry and innovation. Rapid industrial productivity growth results from technological overhauls, while capital accumulation lays the groundwork for technological progress. While technological innovation triggers industry change by replacing obsolete sectors, it also promotes the birth of fresh industries and the transformation of traditional ones. Simultaneously, the industry structure also encourages technological innovation. As such, following Hao, Chen (Hao et al., 2016), we account for industrial structure by using the industry’s added value as a percentage of GDP, symbolized as Industry.
2.1.4 Education
The contribution of human capital to the results of innovation is significant (Marvel and Lumpkin, 2007; Kato et al., 2014). Human capital aids in transforming resources into innovative processes. In particular, a nation’s skilled workforce greatly enhances the innovative output and competitiveness of companies (Chang et al., 2016; Ramachandran, 2017). Consequently, we control for human capital (represented by Education) using the gross secondary school enrollment rate. Hence, we account for human capital using the comprehensive secondary school enrollment rate, symbolized as Education.
2.1.5 POP
The impact of a country’s population size on innovation remains a contentious topic in academic circles. There are diverging opinions among scholars regarding the role of population size in facilitating innovation. To illustrate, Galor and Weil (Galor and Weil, 2000) in his examination of the post-Malthusian era, ascribes technological and economic progress to the exponential growth of population, thereby presenting an endogenous growth model. However, some scholars challenge this view, arguing that population growth does not invariably foster innovation and economic development. Nevertheless, an opposing stance is taken by some scholars who contest this perspective, contending that population growth does not uniformly promote innovation and the advancement of economies. Therefore, in line with Coccia (Coccia, 2014), this study adopt the use of total population (referred to as POP) as a control variable to account for its impact on energy innovation.
2.1.6 GDP
Gross Domestic Product (GDP) is recognized as a significant driver of national energy technology innovation, acting from both demand and supply perspectives (Zheng et al., 2021). From the demand perspective, GDP growth also creates significant societal demand for new products and technologies (Di Stefano et al., 2012). Conversely, on the supply side, GDP growth can augment human, material, and financial resources for innovation across all societal sectors (Kang and Park, 2012). Thus, following the method used by Chang, Wen (Chang et al., 2018), measure of economic development we utilize per capita GDP, denoted by GDP.
2.1.7 Affected
Our empirical analysis and hypothesis testing focus on the most prevalent natural disasters: floods, storms, extreme temperatures, epidemics, earthquakes, and droughts. We measure the magnitude of each natural disaster, following the methodology of (Lee et al., 2021), by the number of affected individuals normalized by the total population, denoted as Affected.
In addition to natural disaster, the control variables include urbanization level (Urban), income inequality (GINI), industrial structure (Industry), human capital (Education), total population (POP), and economic development level (GDP). The data for the majority of these variables is derived from the World Bank database. Below, you will find additional information are provided regarding these variables.
2.1.8 Patent
In the context of technological innovation, patents are frequently considered effective instruments to gauge a nation’s innovation despite certain limitations (Jaffe et al., 2000; Popp, 2005; Pradhan et al., 2018; Wang et al., 2019; Wen et al., 2021a; Wen et al., 2021b; Wang et al., 2021; Wen et al., 2022). An invention or technology that receives legal protection from the state is known as a patent. To measure energy innovation, we adopt the approach of previous research by utilizing patent data specifically related to energy technology (Albino et al., 2014; Li and Lin, 2016), which is approximated by Patent. Therefore, we predict a significant negative correlation between occurrences of natural disasters and the level of energy innovation.
2.2 Data synopsis
Upon examining Table 1, we observed marked disparities in energy technology-related patents across the sampled nations. To address this, it is imperative to understand the underlying factors contributing to this variance. Furthermore, the descriptive statistics associated with the ‘Affected’ variable elucidate significant differences in the populations impacted by natural disasters across these countries. Within the study, the correlation matrix serves as a snapshot, delineating both the magnitude and direction of linear associations among the selected variables. A primary objective of our analysis is to ascertain the presence of multicollinearity, a phenomenon observed when independent variables exhibit high correlation. Such multicollinearity can potentially compromise the accuracy and clarity of regression model interpretations. To address this concern, Table 2 provides an assessment, adopting conventional criteria: a VIF value below 5 and a tolerance value greater than 0.2 (Craney and Surles, 2002). Our findings confirm the absence of multicollinearity, with all VIF values falling below the stipulated threshold.
2.3 Estimation technique
Enlarging the sample size augments the estimation precision and substantially mitigates the repercussions of multicollinearity (Hsiao, 2022; Yang et al., 2022), which incentivizes our selection of panel data for conducting empirical research on mentioned title. The fixed effect model possesses several advantages. It incorporates the impacts of both observable and unobservable variables that do not change over time, thereby considerably reducing the model’s endogeneity (Long et al., 2022; Yang et al., 2022). Therefore, we account for country-specific, time-invariant effects like geographical attributes, ethnic traits, climate conditions, and legal origins.
Moreover, incorporating time fixed effects allows for the regulation of unchanging characteristics within the current year (Hu et al., 2021), limiting control to current year economic characteristics, like the repercussions of the economic crisis that occurred in 2008. Given these benefits, this research employs a two-way fixed effects model that controls for country-specific effects, which are constant over time but vary by country, and yearly fixed effects that are constant across countries but change over time. The proposed benchmark model for our study is as follows:
In this study, the dependent variable encapsulating energy innovation, designated as
2.4 Data sources
In this study, data from 2000 to 2020 was meticulously gathered through an assortment of reputable sources, such as the BP Statistical Review (Statistical Review of World Energy, 2020), US Energy Information Administration (U.S.Energy Information Administration, 2020), International Energy Agency (Agency I.E., 2020), World Bank (Worldbank, 2020), and Global Carbon Project (G.C Project, 2020). In addition, several national data repositories were tapped into, including Brazilian Institute of Geography and Statistics (Globaledge, 2020), Russian Federal State Statistics Service (F.S.A Agencies, 2020), Ministry of Statistics and Programme Implementation (G.OI, 2020), National Bureau of Statistics of China (National Bureau of Statistics of China, 2020), Statistics South Africa (Statistics South Africa, 2020). In Table 1, The descriptive statistics provide a summary of the key variables. Affected has a small mean (0.023) and low standard deviation (0.231), indicating that it varies narrowly around its mean. The GINI index ranges from 15.984 to 50.688 with an average value of 29.303, showing moderate variability. Urbanization levels have a mean of 66.318 and standard deviation of 9.9, suggesting a fairly consistent level of urbanization across the dataset. Industry shows a large standard deviation (42.424) relative to its mean (14.79), indicating high variability. Education ranges widely from 26.001 to 147.541, with a moderate standard deviation (18.351). Population (POP) has a small mean (0.329) but a larger standard deviation (0.486), indicating greater spread. GDP has a mean of 3.044 and a moderate spread (1.777). Lastly, the variable Patent varies significantly with a mean of 1.386 and a standard deviation of 4.069. Overall, the data suggests varying degrees of spread and central tendency across different variables.
The correlation matrix in Table 2 provides insight into the relationships between various variables. Firstly, the ‘Affected’ variable appears to have negligible linear relationships with ‘GINI’, ‘Industry’, and ‘POP’, indicated by their values being close to zero. However, there’s a slight positive association with ‘Urban’ and ‘GDP’. Interestingly, the GINI coefficient, which measures income inequality, has a strong inverse relationship with ‘Education’, suggesting that as education levels rise, income inequality might decrease. There’s also a noticeable positive association between ‘GINI’ and ‘POP’. It is worth noting that the Variance Inflation Factor (VIF) values for all variables are below the common threshold of 10. This suggests that multicollinearity, which refers to high intercorrelations among independent variables, is not a significant concern in this dataset. Specifically, ‘GDP’ has the highest VIF of 2.169 but is still well below the threshold, ensuring the reliability of the regression estimates.
3 Empirical findings
3.1 Nexus between natural disasters and energy innovation
Table 3 displays our primary findings. In column (A), we introduce the variable of natural disasters as the sole addition, devoid of any control variables. Our observations indicate a significant and negative impact of the Affected variable on energy innovation. Subsequently, we progressively incorporate additional control variables, namely, GINI, Urban, Industry, Education, GDP, and POP, in columns (B) to (F). Hence, we focus our discussion on the results obtained in column (F) due to its inclusion of all control variables, thereby enhancing the reliability of the regression outcomes.
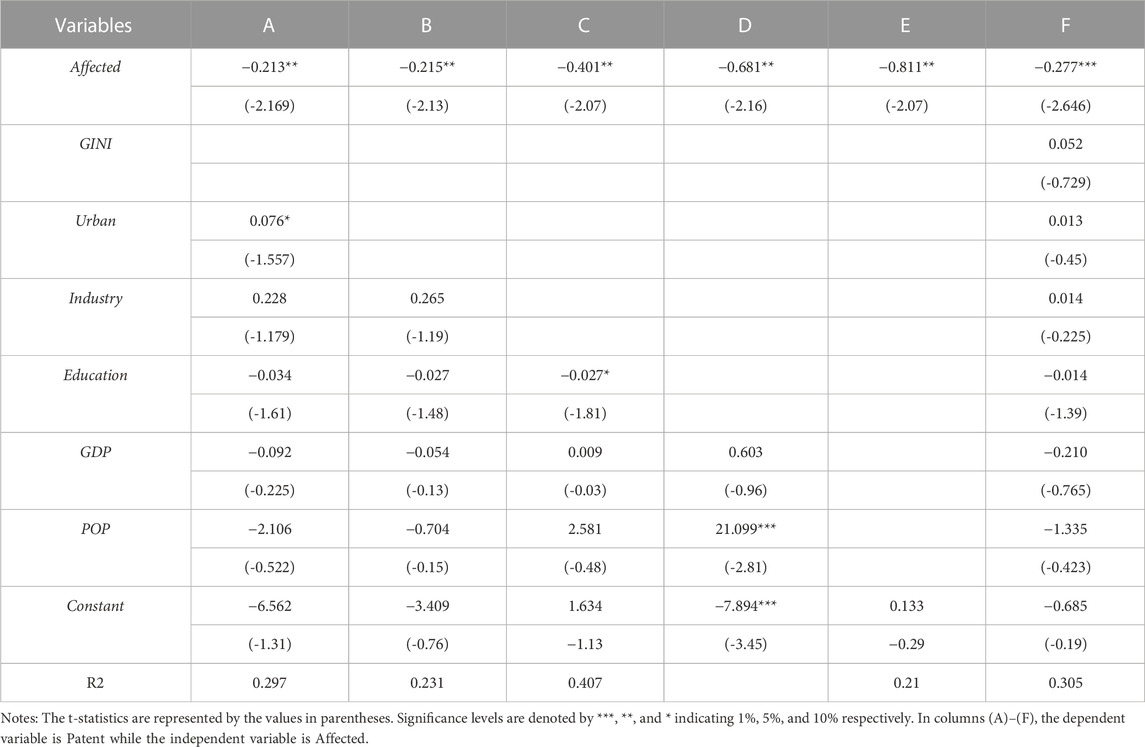
TABLE 3. Assessing the Influence of Natural Disasters on Innovation in Energy Technology using a Two-Way Fixed Effect Model.
Analysis of data from column (F) implies that natural disasters induce negative repercussions on energy technology innovation. To evaluate its economic significance, we calculate the result of multiplying the ‘Affected’ coefficient and the standard deviation of ‘Affected’, then dividing by the standard deviation of energy technology patent applications (Patent). An increase of one standard deviation in ‘Affected’ corresponds to a reduction of 0.015 percentage of a standard deviation in the patent applications related to energy technology [(−0.277*0.231)/4.069]. This result align with those of Lee, Wang (Lee et al., 2021), asserting significant disruptions to both nuclear and renewable energy consumption due to natural disasters. Rising energy demands pressurize energy companies to enhance efficiency, optimize operations, develop new products, and engage in increased innovative efforts to withstand intense competition. However, a decrease in energy consumption obstructs the technological innovation of energy companies.
There are various potential reasons that can be attributed to the inhibitory effect of natural disasters on energy innovation. First, the economic analysis of natural disasters, which began to gain attention in the 1950s and 1960s, was initially concentrated on the economic effects of such events. Traditional neoclassical growth theory suggests that a natural disaster has the potential to diminish per capita total output. This reduction arises from the destruction caused by the natural disaster to a portion of a country’s capital stock, thereby limiting the capacity for production. Similarly, natural disaster can also reduce per capita total output, leading to a decline in the average productivity of productive assets (Felbermayr and Gröschl, 2014). Post-disaster, government revenue shrinks due to dwindling tax exemptions and economic output, whereas financial expenditure escalates in efforts to restore basic living conditions for the affected population, bolster the economy of the affected regions, and rebuild the affected infrastructure (Debelle, 2020; Song et al., 2020; Fu and Chang, 2021). Consequently, the government reduces investment in activities related to energy technological innovation, limiting innovation performance since technological progress depends on investment in basic and applied research (Levi et al., 2020). Second, natural disasters have proven to have a negative effect on technological innovation. Scientific investigations have demonstrated that technological innovation may be adversely affected by natural disasters, primarily due to funding deficiencies, shifts in companies’ research and development (R&D) strategies, and the physical devastation of objective conditions (Chen et al., 2021). Considering the surge in technological innovation in recent years and its recognition as a key determinant in securing a competitive edge (Lee and Lee, 2013), it is postulated that the inflicted natural disasters damage on the wider technological innovation landscape will similarly permeate the energy sector. Third, Lee, Wang (Lee et al., 2021) reported that natural disasters significantly dent energy consumption. Energy security and energy efficiency constitute the objectives of technological advancement and innovation (Wang and Lee, 2022). The primary challenge confronting technological innovation in the energy sector is to ensure the provision of sufficient energy services at a minimal cost (Sagar and Holdren, 2002). The decrease in energy demand and consumption due to natural disasters slows down the pace of energy innovation. Thus, innovative activities in the energy sector are hindered after a natural disaster. Fourth, natural disasters can potentially spread terror, increasing conflict and create a turbulent external environment (Bhavnani, 2006; Berrebi and Ostwald, 2011). Consequently, the diminishing opportunities for communication and collaborative learning among technological innovation talents from various countries have a detrimental effect on energy innovation.
3.2 Time lag effect
The progression of economic and society landscapes has stimulated an increased interest in scholarly literature regarding the protracted implications of natural perturbations. The lingering consequences of environmental calamities are escalating in severity (Skidmore and Toya, 2002). Consequently, the ramifications of natural disturbances upon energy technology innovation could manifest in a delayed, or lagged, manner. Initially, these natural disasters assail the fundamental backdrop of the economy, prompting subsequent responsive actions by government bodies and corporate entities. These mitigative measures exert an impact upon the innovation of energy technology, each of which necessitates a certain span of time for execution. Therefore, the substantial repercussions of natural disasters on innovation in energy technology may manifest as a protracted effect.
To further scrutinize the effect of energy innovation lagging by 1–5 years, we conduct a detailed examination. The impact of natural disasters in the current year on energy innovation in the subsequent 1–5 years is presented in Table 4. The corresponding influence is listed in columns (A)-(E) accordingly. The findings demonstrate that the anticipated relationship between natural disasters and energy innovation remains intact in the subsequent 1–4 years. Specifically, the lag effect analysis reveals that natural shocks have a notable detrimental impact on energy technology innovation. The enduring effect of this phenomenon is not limited to the current year but extends over a span of 4 years. Additionally, the anticipated outcomes are reflected in the coefficients and significance of the primary explanatory variables across columns (A) to (E). These findings are in line with past research (Hallegatte and Dumas, 2009), who accentuated the inability of technological progress to convert a disaster into a long-term favorable event. Moreover, the influence of natural disasters on energy innovation gradually diminishes year by year to the point where it becomes indistinguishable, as evidenced by the comparison of coefficients in columns (A) to (E).
3.3 Rigorous validation
Further exploration into the validity of our findings is performed through robustness checks encompassing the employment of alternate dependent variables, the incorporation of additional variables influencing energy innovation, and the application of diverse quantitative methodologies.
3.3.1 Cross-sectional dependence considerations
With the growing use of panel data, concerns over cross-sectional dependence in panel data models have gained attention. It is not uncommon to observe correlations between individual entities, owing to shared exposure to specific shocks. Within the scope of this study, such correlations are discernible amongst sampled nations. However, given that the non-contemporaneous dependence across different data cross-sections does not addresseed by PCSE estimators, In order to enhance the credibility of our findings, this study employed the (Driscoll and Kraay, 1998) estimator, which employs a non-parametric approach to ensure a reliable variance estimation. This additional step supports the authenticity of our results. Column (A) of Table 5 displays the results of this model, confirming the durability of our primary findings, even when considering cross-sectional dependence. (Beck and Katz, 1995). proposed the Panel-Corrected Standard Errors (PCSE) estimation method, an effective solution to the problem of cross-sectional dependence. Accordingly, our initial step involves conducting a panel PCSE model, taking into consideration cross-sectional dependence, with empirical outcomes detailed in column (B) of Table 5.
3.3.2 Alternative dependent variables
To evaluate technological innovation capabilities, we predominantly rely on measures of patents and R&D investment as indicators of innovation (Wadhwa and Basu, 2013; Wen et al., 2017; Wen et al., 2022). When considering patent applications relevant to energy technology, we also incorporate total R&D investment expenses associated with energy (denoted as R&D) to reflect energy innovation. Data was sourced from IEA Energy Technology Research, Development and Demonstration (RD&D) Statistics. Energy technology-related research and development (R&D) is significantly impeded by natural disasters, as evident from the confirmed coefficient of the disaster variable in column (D) of Table 5. This estimate substantiates the substantial hindrance caused by natural disasters to energy innovation. This could be due to the redirection of government funds to disaster mitigation, unavoidably reducing R&D expenditure in energy technology.
Furthermore, we utilize innovation efficiency as a metric for assessing the degree of innovative energy technology. Following the methodology adopted by (Hirshleifer et al., 2013), we employ the ratio of patent applications to total R&D investment associated with energy to gauge energy innovation efficiency. This indicator serves as a better reflection of a nation’s innovation level compared to patent application alone, aiding energy sector companies in their transition to a more intensive development model. Table 5 column (C) reveals that natural disasters significantly thwart energy innovation, a finding consistent across varying dependent variable indicators.
3.3.3 Endogeneity considerations
We aim to address potential endogeneity concerns by adopting several strategies, inclusive of incorporating more omitted variables that could influence energy innovation, utilizing the system Generalized Method of Moments (GMM) and the Instrumental Variables (IV) method.
(a) Implementing the Method of Instrumental Variables (IV)
Despite the effectiveness of prior robustness test methods in addressing potential endogeneity issues associated with model, certain unobserved factors continue to influence natural disaster occurrences and energy technology innovation. Thus, we have implemented the instrumental variable (IV) method to enhance robustness testing. To elaborate, we employ (Lewbel, 2012) technique to create instrumental variables utilizing exogenous variables and heteroscedasticity. To establish the instrumental variable within the econometric model, a two-step procedure is employed. Firstly, the endogenous variables are regressed on the exogenous variables, yielding the residuals. Secondly, the instrumental variable is constructed by multiplying the mean-subtracted exogenous variables with the aforementioned residuals.
A review of Table 6 column (A) displays a Wald F statistic value over 10, proving the legitimacy of the created instrumental variable. Moreover, the core findings confirm that the estimated coefficient of the variable associated with natural disasters is in accordance, affirming that natural disasters indeed obstruct energy technology innovation, despite addressing potential endogeneity issues through the instrumental variables method.
(b) Exploring Various Estimation Techniques.
Initially, (Bai, 2009), introduced the concept of incorporating interactive effects between individuals and time in the linear panel model framework. The objective of this methodology is to effectively capture the varying influences of common factors on different individuals, thus addressing the limitations of the conventional panel fixed-effects model. In contrast, the conventional panel fixed-effects model inadequately represents the complexities associated with specific issues. On the other hand, the panel interactive fixed-effects model presents a more realistic depiction, overcoming these limitations. It duly considers the multidimensional disturbances prevailing in the real economy and the heterogeneous responses of individuals to these disturbances. Column (C) of Table 6 presents the outcomes obtained from the model incorporating fixed effects in a panel setting, corroborating the primary findings.
Furthermore, to address potential endogeneity concerns and ensure result consistency, we further employ the dynamic Generalized Method of Moments (GMM) panel estimator, as expounded upon by (Arellano and Bover, 1995). The instrumental variables (IVs) employed in this study consist of the internal lags of the endogenous variables. To estimate the model, we apply the system generalized method of moments (GMM) approach, considering both the dependent variables and other exogenous variables as endogenous. Furthermore, we adopt a two-step estimation procedure that addresses for biases inherent in small sample sizes and remains robust against panel autocorrelation and heteroscedasticity. The validity of the instrumental variables and the absence of second-order serial correlation in the random error term of the first-order difference equation are confirmed through an examination of the lower test statistics in column (B) of Table 6. Furthermore, we verify the robustness of the core regression findings.
(c) Pondering Omitted Variables
When scrutinizing the multitude of determinants that sway energy innovation within the fundamental regression, one must take into account trade openness and energy consumption. Overlooking such elements invites the risk of endogeneity, as established by (Feng et al., 2021). The prudent inclusion of other contributory factors impacting a nation’s energy innovation can significantly attenuate the adverse repercussions of omitted variable bias on empirical analysis.
In the era of global economic integration, a nation’s prowess in technological advancement is shaped not solely by internal determinants, but also by external, global influences. Notably, among these extraneous variables, the degree of trade liberalization emerges as a paramount influencer of innovation, as underscored by (Laursen and Salter, 2005; Love et al., 2013). Empirical data suggests that nations embracing elevated levels of trade liberalization tend to garner a richer influx of ideas and resources from the global ecosystem, thereby enhancing their innovative capabilities (Dahlander and Gann, 2010). Thus, to meticulously factor in this crucial determinant, we incorporate an index—denoted as ‘Openness'—that encapsulates the quotient of international commerce relative to GDP, representing the extent of trade liberalization. Furthermore, to adeptly adjust for the ramifications of Foreign Direct Investment (FDI), we incorporate it into our analytical framework, quantified by the net influx of foreign investment as a percentage of GDP, herein represented by the moniker ‘FDI'.
In Table 7, the second column elucidates the computed outcomes subsequent to the inclusion of the control determinant, Foreign Direct Investment (FDI), premised upon the prior variables. A third observation illuminates the pronounced influence of governmental ideological leanings on innovative endeavors, an assertion echoed in the empirical analysis of (Wang et al., 2019). This notable study discerned an augmentation in innovation under the governance of right-leaning political entities, contrasting with a perceivable diminution in innovative prowess under left-leaning establishments. Thus, this investigation incorporates an ideological classification (represented by ‘Ideology’) to augment its analytical depth, and pursues the execution of robustness assays. Such endeavors are manifested in the tabulation’s third column, post the annexation of the said ideological parameter.
As a fourth proposition, one cannot neglect the crucial correlation between energy supply-demand dynamics and energy pricing as evidenced by (Wang et al., 2011). Moreover, a myriad of intricate factors—including recurrent epidemic ramifications, exacerbated climatic aberrations, the brisk pace of ecological reformation, and an inundation of fiscal liquidity—have precipitated a pronounced disequilibrium within the global energetic spectrum. This has culminated in a formidable escalation in energy valuations, thus amplifying the inherent fragility of the energy marketplace, as delineated by (Wen et al., 2021a; Wen et al., 2021b; Wen et al., 2021c; Wen et al., 2021d). As an imperative, the intricacies of energy provision and requisition warrant rigorous scrutiny. This manuscript employs a metric of primary energy provision normalized by Gross Domestic Product (GDP)—symbolized as ‘Supply’. Concurrently, drawing parallels with the methodology espoused by (Brenton, 1997), the consumption of primary energy normalized by GDP offers a measure for energy requisition (denoted as ‘Demand’). Within the sixth tabulation, the fourth column unveils computed findings subsequent to the integration of these vital determinants—Supply and Demand—based upon preceding parameters. An analytical perusal post the adjustment for the aforementioned triad of potential confounders ratifies the tenacity of the conclusions drawn herein.
3.4 Unraveling potential mechanisms
Emerging from our comprehensive analysis of the extant literature and conjectural development section, we have deduced that natural disasters present a significant impediment to energy innovation through the following conduits: socioeconomic progress, commercial globalism, financial integration, interhuman globalization, and governmental stability. To portray commercial globalism, we introduce the international trade to GDP ratio (indicated by Openness). Financial openness is reflected through an index representing the de facto financial globalization of our sample nations, denoted as Finance, deriving from the 2020 KOF Globalization Index. Equally, the de facto interpersonal globalization index of the examined nations, represented by Personnel, is sourced from the same database as the financial openness index. Our final potential pathway is government stability (implied by Stability), where a score nearing 0 signifies heightened risk and a score nearing 4 implies decreased risk, originating from the ICRG database. Our proposed estimation model is:
In this model, all variables remain consistent, with
3.5 Delving into heterogeneity
3.5.1 Dissecting heterogeneity in different types of natural disasters
Distinct in their nature, mechanism, and unfolding process, different types of natural disasters exert varying influences on energy technology (Loayza et al., 2012). Hence, we scrutinize the ramifications of individual natural disasters on energy innovation. We establish variables to represent various types of natural disasters, including Earthquake, Drought, Epidemic, Temperature, Flood, and Storm. The results of these variables are displayed in Table 9. In column (G), we incorporate all variables related to natural disasters to assess their individual impact on energy technology innovation.
3.5.2 Findings of heterogeneity across diverse energy technology types
As humanity stands at the threshold of a pivotal shift in energy paradigms, transitioning from conventional to carbon-conscious energy systems, it is essential to appreciate the diversity encompassed within the energy technological domain. Conventional carbon-intensive energy systems are being supplanted by more sustainable and environmentally friendly substitutes such as biomass, solar, and wind energies, collectively designated as low-carbon energy. Implementation of low-carbon energy technology not only alleviates climatic transformation and attenuates carbon dioxide emissions, but also actualizes goals of ecological protection, emission reduction, and energy conservation through the deployment and amplification of highly efficient technology systems (McJeon et al., 2011; Howell et al., 2014). The creativity intrinsic to low-carbon energy technology transcends a handful of technical methodologies aiming at carbon neutrality, decarbonization, and reduction, enveloping a fundamental metamorphosis in the knowledge matrices, foundational principles, and regulations, that underpin technological progression.
The ascension of low-carbon energy technology necessitates considerable capital infusion. In the wake of cataclysmic events, governmental entities might disproportionately allocate their finite resources towards post-calamity reconstruction, inevitably impinging upon the human, material, and fiscal investments earmarked for fostering low-carbon energy innovation. Consequently, the implications of cataclysmic events on low-carbon and conventional energy technologies might manifest discernible discrepancies. The aggregate R&D expenditure pertaining to energy technology is bifurcated into low-carbon and conventional energy outlays. We investigate the differential ramifications of cataclysmic events on low-carbon and conventional energy technologies through standardizing the quantity of patents with R&D expenditure associated with low-carbon and conventional energy technology. Empirical outcomes, demonstrated in Table 10 (columns A and B), substantiate that cataclysmic events levy a significantly deleterious effect on low-carbon energy innovation, while conventional energy innovation remains unscathed.
4 Conclusion and policy implication
Our primary investigation scrutinizes the ramifications of environmental shocks on advancements in energy as reflected by patent filings in the domain of energy technologies within five BRICS nations over the temporal span from 1990 to 2020. Our data-driven findings corroborate the hypothesis that climatic calamities pose a significant obstacle to innovation in the energy sector, manifested by a decrease in patent filings pertinent to energy technology, a decline in total R&D expenditure associated with energy technology, and a deterioration of energy innovation efficacy. These outcomes withstand rigorous validity checks including the use of alternative outcome variables symbolizing energy innovation, the integration of extra variables affecting energy innovation, and the employment of diverse statistical approaches. Furthermore, the detrimental effect on energy innovation lingers for up to 4 years following the incident, with pandemics exhibiting the gravest negative impact on energy technology innovation. Interestingly, climatic catastrophes significantly hinder low-carbon energy innovation, while the innovation within non-low-carbon energy remains unaffected.
Our results enrich the existing studies within the area of disaster economics, and innovation and energy economics. The scarcity of empirical analyses examining the influence of energy innovation and natural disasters on highlights the significance of our contribution. We weave together the concepts of natural disaster and energy innovation to address an existing research lacuna in disaster economics from a technological innovation viewpoint.
This study derives specific policy recommendations for governments and policymakers. Governments should prudently weigh the potential advantages of diverse disaster mitigation and prevention strategies and distinguish the unique impacts of diverse natural shocks on the technological progress within the energy industry. Such discernment will assist in the crafting of finely honed disaster intervention measures. Additionally, BRICS nations ought to strive to formulate and polish regulations and systems nurturing an environment conducive to energy innovation, thereby enhancing their capacity to combat an array of natural disasters and mitigate the damage inflicted by such occurrences.
Notwithstanding its contributions, our research is not without limitations, underscoring the need for additional inquiries. Our study did not delve into more intricate domains within the energy or resources field. We recommend for future investigations to probe specific questions such as the impact of natural disasters on renewable energy technology or the effect of floods and droughts on technological innovation in water resources. Beyond energy innovation, researching the unique influences of natural disasters on energy finance and energy price risks in various countries would yield significant scientific contributions. The purview of our research was primarily confined to OECD countries due to data availability constraints, signaling the need for forthcoming studies to broaden their geographical scope. Finally, continued exploration of the socio-economic impacts of meteorological phenomena, i.e., effects on ecosystems and green growth, is encouraged.
4.1 Underlying mechanisms of natural disasters’ influence on energy innovation
In the wake of natural disasters, particularly those of significant intensity, a cascade of consequences unfolds that impedes energy innovation. For instance, the resultant devastation to infrastructure critically damages research facilities, laboratories, and educational institutions, which are the crucibles of energy innovation. This destruction not only demands considerable resources for reconstruction but siphons off potential investments from innovation. Concurrently, these calamities can severely disrupt supply chains, leaving manufacturers bereft of vital components, thereby stalling or elongating research and development timelines. The aftermath of such events also witnesses a discernible redirection of both financial and human resources toward immediate relief efforts, leading to diminished funds for long-term innovative endeavors. This post-disaster landscape often sees the migration or displacement of essential human capital, including experts and researchers, whose absence introduces chasms in the innovation continuum. Governments, responding to the exigencies, might recalibrate policy priorities, potentially sidelining energy innovation. This environment also breeds investment uncertainties, as the looming threat of recurrent disasters makes stakeholders circumspect about funding long-term projects. Moreover, a psychological shift can permeate society post-disaster, emphasizing immediate survival over future-oriented innovation. Finally, a not-so-apparent but significant impediment is the potential loss or corruption of ongoing research data, introducing further delays in the quest for energy innovation.
4.2 Future direction of the study
The initial analyses present numerous opportunities for more rigorous empirical investigation. Thus far, innovation in energy has been quantified primarily through patent filings. However, a more comprehensive conceptualization requires examining additional indices, such as private capital investment, emerging enterprises focused on disruptive energy technologies, and academic interest as seen in scholarly publications. Additionally, comparing countries with established disaster mitigation protocols to those without could reveal relationships between resilience to exogenous shocks and technological productivity. Investigating how different disaster types (e.g., earthquakes vs. storms) uniquely impact innovation represents an intriguing potential analysis. Given the heterogeneity within the energy sector, elucidating differential recovery rates following disruptions across technologies (e.g., photovoltaics vs. wind vs. nuclear) could provide actionable insights. Moreover, accurately modeling national innovation outcomes necessitates incorporating socioeconomic factors as key variables. As societal reliance on digital technologies increases, exploring how information systems may restore innovative momentum after unexpected equilibrium disruptions warrants prioritization.
Data availability statement
The datasets presented in this study can be found in online repositories. The names of the repository/repositories and accession number(s) can be found in the article/Supplementary material.
Author contributions
NA: Validation, Writing–original draft, Conceptualization, Data curation, Formal Analysis, Investigation, Project administration, Resources, Supervision. FO: Software, Validation, Visualization, Writing–original draft. ES: Methodology, Writing–review and editing. RC: Data curation, Methodology, Resources, Validation, Writing–original draft, Writing–review and editing. JJ-V: Investigation, Methodology, Writing–review and editing. SS-A: Software, Visualization, Writing–original draft. JB: Conceptualization, Data curation, Funding acquisition, Resources, Validation, Writing–original draft, Writing–review and editing. JO: Conceptualization, Validation, Resources, Data curation, Writing–original draft preparation, Writing–review and editing, Funding acquisition. AK: Data curation, Funding acquisition, Resources, Validation, Writing–original draft, Writing–review and editing.
Funding
The author(s) declare financial support was received for the research, authorship, and/or publication of this article. The research received funding under the research subsidy of the Faculty of Organization and Management of the Silesian University of Technology in Poland for the year 2023 (13/020/BK_23/0081).
Conflict of interest
The authors declare that the research was conducted in the absence of any commercial or financial relationships that could be construed as a potential conflict of interest.
Publisher’s note
All claims expressed in this article are solely those of the authors and do not necessarily represent those of their affiliated organizations, or those of the publisher, the editors and the reviewers. Any product that may be evaluated in this article, or claim that may be made by its manufacturer, is not guaranteed or endorsed by the publisher.
References
Aflaki, A., Mahyuddin, N., Al-Cheikh Mahmoud, Z., and Baharum, M. R. (2015). A review on natural ventilation applications through building façade components and ventilation openings in tropical climates. Energy Build. 101, 153–162. doi:10.1016/j.enbuild.2015.04.033
Akao, K.-I., and Sakamoto, H. (2018). A theory of disasters and long-run growth. J. Econ. Dyn. Control 95, 89–109. doi:10.1016/j.jedc.2018.08.006
Albino, V., Ardito, L., Dangelico, R. M., and Messeni Petruzzelli, A. (2014). Understanding the development trends of low-carbon energy technologies: A patent analysis. Appl. Energy 135, 836–854. doi:10.1016/j.apenergy.2014.08.012
Antonietti, R., Bronzini, R., and Cainelli, G. (2014). Inward greenfield FDI and innovation. Econ. Polit. Ind. 42 (1), 93–116. doi:10.1007/s40812-014-0007-9
Arellano, M., and Bover, O. (1995). Another look at the instrumental variable estimation of error-components models. J. Econ. 68 (1), 29–51. doi:10.1016/0304-4076(94)01642-d
Bai, C., Feng, C., Yan, H., Yi, X., Chen, Z., and Wei, W. (2020). Will income inequality influence the abatement effect of renewable energy technological innovation on carbon dioxide emissions. J. Environ. Manag. 264, 110482. doi:10.1016/j.jenvman.2020.110482
Barredo, J. I. (2009). Normalised flood losses in europe: 1970–2006. Nat. Hazards Earth Syst. Sci. 9 (1), 97–104. doi:10.5194/nhess-9-97-2009
Beck, N., and Katz, J. N. (1995). What to do (and not to do) with time-series cross-section data. Am. Political Sci. Rev. 89 (3), 634–647. doi:10.2307/2082979
Berrebi, C., and Ostwald, J. (2011). Earthquakes, hurricanes, and terrorism: do natural disasters incite terror? Public Choice 149 (3), 383–403. doi:10.1007/s11127-011-9868-x
Bertola, G., Foellmi, R., and Zweimüller, J. (2005). Income distribution in macroeconomic models. Princeton University Press.
Boustan, L. P., Kahn, M. E., Rhode, P. W., and Yanguas, M. L. (2020). The effect of natural disasters on economic activity in us counties: A century of data. J. Urban Econ. 118, 103257. doi:10.1016/j.jue.2020.103257
Brenton, P. (1997). Estimates of the demand for energy using cross-country consumption data. Appl. Econ. 29 (7), 851–859. doi:10.1080/000368497326507
Busby, J. W., Smith, T. G., Krishnan, N., Wight, C., and Vallejo-Gutierrez, S. (2018). In harm's way: climate security vulnerability in asia. World Dev. 112, 88–118. doi:10.1016/j.worlddev.2018.07.007
Cavallo, E. A., and Noy, I. (2011). Natural disasters and the economy — a survey. Int. Rev. Environ. Resour. Econ. 5 (1), 63–102. doi:10.1561/101.00000039
Chang, C.-P., and Berdiev, A. N. (2013). Natural disasters, political risk and insurance market development. Geneva Pap. Risk Insur. - Issues Pract. 38 (3), 406–448. doi:10.1057/gpp.2013.14
Chang, C.-P., Wen, J., Zheng, M., Dong, M., and Hao, Y. (2018). Is higher government efficiency conducive to improving energy use efficiency? Evidence from OECD countries. Econ. Model. 72, 65–77. doi:10.1016/j.econmod.2018.01.006
Chang, F., Min, W., Shi, Y., Kenny, K., and Loyalka, P. (2016). Educational expectations and dropout behavior among junior high students in rural China. China and World Econ. 24 (3), 67–85. doi:10.1111/cwe.12159
Chen, Y.-E., Chang, C. P., and Zheng, M. (2021). Identifying the influence of natural disasters on technological innovation. Econ. Analysis Policy 70, 22–36. doi:10.1016/j.eap.2021.01.016
Chen, Y. E., Fu, Q., Zhao, X., Yuan, X., and Chang, C. P. (2019). International sanctions’ impact on energy efficiency in target states. Econ. Model. 82, 21–34. doi:10.1016/j.econmod.2019.07.022
Chiu, Y.-B., and Lee, C.-C. (2020). Effects of financial development on energy consumption: the role of country risks. Energy Econ. 90, 104833. doi:10.1016/j.eneco.2020.104833
Coccia, M. (2014). Driving forces of technological change: the relation between population growth and technological innovation. Technol. Forecast. Soc. Change 82 (1), 52–65. doi:10.1016/j.techfore.2013.06.001
Cook, P., and Uchida, Y. (2008). Structural change, competition and income distribution. Q. Rev. Econ. Finance 48 (2), 274–286. doi:10.1016/j.qref.2006.12.017
Costa-Campi, M. T., Duch-Brown, N., and Garcia-Quevedo, J. (2014). R&D drivers and obstacles to innovation in the energy industry. Energy Econ. 46, 20–30. doi:10.1016/j.eneco.2014.09.003
Craney, T. A., and Surles, J. G. (2002). Model-dependent variance inflation factor cutoff values. Qual. Eng. 14 (3), 391–403. doi:10.1081/qen-120001878
Cuaresma, J. C., Hlouskova, J., and Obersteiner, M. (2008). Natural disasters as creative destruction? Evidence from developing countries. Econ. Inq. 46 (2), 214–226. doi:10.1111/j.1465-7295.2007.00063.x
Cunado, J., and Ferreira, S. (2011). The macroeconomic impacts of natural disasters: New evidence from floods.
Dahlander, L., and Gann, D. (2010). How open is innovation. Res. Policy 39 (6), 699–709. doi:10.1016/j.respol.2010.01.013
Debelle, G. (2020). The Reserve Bank of Australia’s policy actions and balance sheet☆. Econ. analysis policy 68, 285–295. doi:10.1016/j.eap.2020.10.001
Di Stefano, G., Gambardella, A., and Verona, G. (2012). Technology push and demand pull perspectives in innovation studies: current findings and future research directions. Res. Policy 41 (8), 1283–1295. doi:10.1016/j.respol.2012.03.021
Doytch, N., and Klein, Y. L. (2017). The impact of natural disasters on energy consumption: an analysis of renewable and nonrenewable energy demand in the residential and industrial sectors. Environ. Prog. Sustain. Energy 37 (1), 37–45. doi:10.1002/ep.12640
Driscoll, J. C., and Kraay, A. (1998). Consistent covariance matrix estimation with spatially dependent panel data. Rev. Econ. Statistics 80 (4), 549–560. doi:10.1162/003465398557825
Drury, A. C., and Olson, R. S. (1998). Disasters and political unrest: an empirical investigation. J. Contingencies Crisis Manag. 6 (3), 153–161. doi:10.1111/1468-5973.00084
Felbermayr, G., and Gröschl, J. K. (2014). Naturally negative: the growth effects of natural disasters. J. Dev. Econ. 111, 92–106. doi:10.1016/j.jdeveco.2014.07.004
Feng, F., Yang, H. C., Gong, Q., and Chang, C. P. (2021). What is the exchange rate volatility response to COVID-19 and government interventions? Econ. Analysis Policy 69, 705–719. doi:10.1016/j.eap.2021.01.018
F.S.A Agencies (2020). The Russian government. Available at https://eng.rosstat.gov.ru/.
Fu, Q., and Chang, C.-P. (2021). How do pandemics affect government expenditures. Asian Econ. Lett. 2 (1), 21147. doi:10.46557/001c.21147
Galor, O., and Weil, D. N. (2000). Population, technology, and growth: from malthusian stagnation to the demographic transition and beyond. Am. Econ. Rev. 90 (4), 806–828. doi:10.1257/aer.90.4.806
G.C Project (2020). Global carbon project. Available at: https://www.globalcarbonproject.org/.
Globaledge (2020). Connect with globalEDGE. Available at: https://globaledge.msu.edu/.
G.OI (2020). Ministry of statistics and Programme implementation. Available at https://www.mospi.gov.in/.
Gualandri, F., and Kuzior, A. (2023). Home energy management systems adoption scenarios: the case of Italy. Energies 16 (13), 4946. doi:10.3390/en16134946
Haddad, E. A., and de Alcântara Teixeira, E. (2015). Economic impacts of natural disasters in megacities: the case of floods in são paulo, Brazil. Habitat Int. 45, 106–113. doi:10.1016/j.habitatint.2014.06.023
Hallegatte, S., and Dumas, P. (2009). Can natural disasters have positive consequences? Investigating the role of embodied technical change. Ecol. Econ. 68 (3), 777–786. doi:10.1016/j.ecolecon.2008.06.011
Hao, Y., Chen, H., and Zhang, Q. (2016). Will income inequality affect environmental quality? Analysis based on China's provincial panel data. Ecol. Indic. 67, 533–542. doi:10.1016/j.ecolind.2016.03.025
Hirshleifer, D., Hsu, P.-H., and Li, D. (2013). Innovative efficiency and stock returns. J. Financial Econ. 107 (3), 632–654. doi:10.1016/j.jfineco.2012.09.011
Hood, M., Kamesaka, A., Nofsinger, J., and Tamura, T. (2013). Investor response to a natural disaster: evidence from Japan's 2011 earthquake. Pacific-Basin Finance J. 25 (25), 240–252. doi:10.1016/j.pacfin.2013.09.006
Howell, R., Shackley, S., Mabon, L., Ashworth, P., and Jeanneret, T. (2014). Engaging the public with low-carbon energy technologies: results from a scottish large group process. Energy Policy 66, 496–506. doi:10.1016/j.enpol.2013.11.041
Hu, H., Chen, D., and Fu, Q. (2021). Does a government response to COVID-19 hurt the stock price of an energy enterprise? Emerg. Mark. Finance Trade 58 (1), 1–10. doi:10.1080/1540496x.2021.1911803
Jabbouri, N. I., Siron, R., Zahari, I., and Khalid, M. (2016). Impact of information technology infrastructure on innovation performance: an empirical study on private universities in Iraq. Procedia Econ. Finance 39, 861–869. doi:10.1016/s2212-5671(16)30250-7
Jaffe, A. B., Trajtenberg, M., and Fogarty, M. S. (2000). Knowledge spillovers and patent citations: evidence from a survey of inventors. Am. Econ. Rev. 90 (2), 215–218. doi:10.1257/aer.90.2.215
Johnstone, N., Haščič, I., and Popp, D. (2009). Renewable energy policies and technological innovation: evidence based on patent counts. Environ. Resour. Econ. 45 (1), 133–155. doi:10.1007/s10640-009-9309-1
Kang, K.-N., and Park, H. (2012). Influence of government R&D support and inter-firm collaborations on innovation in Korean biotechnology SMEs. Technovation 32 (1), 68–78. doi:10.1016/j.technovation.2011.08.004
Kato, M., Okamuro, H., and Honjo, Y. (2014). Does founders' human capital matter for innovation? Evidence from Japanese start-ups. J. Small Bus. Manag. 53 (1), 114–128. doi:10.1111/jsbm.12094
Khan, A., Chenggang, Y., Khan, G., and Muhammad, F. (2020). The dilemma of natural disasters: impact on economy, fiscal position, and foreign direct investment alongside belt and road initiative countries. Sci. total Environ. 743, 140578. doi:10.1016/j.scitotenv.2020.140578
Khan, K. A., Zaman, K., Shoukry, A. M., Sharkawy, A., Gani, S., Sasmoko, , et al. (2019). Natural disasters and economic losses: controlling external migration, energy and environmental resources, water demand, and financial development for global prosperity. Environ. Sci. Pollut. Res. Int. 26 (14), 14287–14299. doi:10.1007/s11356-019-04755-5
Kochmańska, A. (2019). The concept of CSR as one of the determinants of sustainable development. Zesz. Nauk. Organ. i Zarządzanie/Politechnika Śląska (135), 87–97.
Kukułka, A. (2014). Natural disasters and FDI inflow in the developing countries of South-Eastern Asia. Pr. Nauk. Uniw. Ekon. we Wrocławiu (370), 208–216. doi:10.15611/pn.2014.370.16
Kuzior, A., Kochmańska, A., and Marszałek-Kotzur, I. (2021). “Information and communication technologies as a tool of modern communication in organizations and society Innovation management and information technology impact on global economy in the era of pandemic,” in Proceedings of the 37th International Business Information Management Association Conference (IBIMA).
Kuzior, A., Samusevych, Y., Lyeonov, S., Krawczyk, D., and Grytsyshen, D. (2023). Applying energy taxes to promote a clean, sustainable and secure energy system: finding the preferable approaches. Energies 16 (10), 4203. doi:10.3390/en16104203
Laursen, K., and Salter, A. (2005). Open for innovation: the role of openness in explaining innovation performance among U.K. manufacturing firms. Strategic Manag. J. 27 (2), 131–150. doi:10.1002/smj.507
Lee, C.-C., Wang, C. W., Ho, S. J., and Wu, T. P. (2021). The impact of natural disaster on energy consumption: international evidence. Energy Econ. 97, 105021. doi:10.1016/j.eneco.2020.105021
Lee, I., and Lee, K. (2015). The internet of things (IoT): applications, investments, and challenges for enterprises. Bus. horizons 58 (4), 431–440. doi:10.1016/j.bushor.2015.03.008
Lee, K., and Lee, S. (2013). Patterns of technological innovation and evolution in the energy sector: A patent-based approach. Energy Policy 59, 415–432. doi:10.1016/j.enpol.2013.03.054
Lee, W. C., and Law, S. H. (2017). Roles of formal institutions and social capital in innovation activities: A cross-country analysis. Glob. Econ. Rev. 46 (3), 203–231. doi:10.1080/1226508x.2017.1292859
Levi, P., Pales, A. F., and Gül, T. (2020). Aligning investment and innovation in heavy industries to accelerate the transition to net-zero emissions.
Lewbel, A. (2012). Using heteroscedasticity to identify and estimate mismeasured and endogenous regressor models. J. Bus. Econ. Statistics 30 (1), 67–80. doi:10.1080/07350015.2012.643126
Li, K., and Lin, B. (2016). Impact of energy technology patents in China: evidence from a panel cointegration and error correction model. Energy Policy 89, 214–223. doi:10.1016/j.enpol.2015.11.034
Lin, B., and Chen, Y. (2019). Does electricity price matter for innovation in renewable energy technologies in China. Energy Econ. 78, 259–266. doi:10.1016/j.eneco.2018.11.014
Loayza, N. V., Olaberría, E., Rigolini, J., and Christiaensen, L. (2012). Natural disasters and growth: going beyond the averages. World Dev. 40 (7), 1317–1336. doi:10.1016/j.worlddev.2012.03.002
Long, H., Chang, C. P., Jegajeevan, S., and Tang, K. (2022). Can Central Bank mitigate the effects of the COVID-19 pandemic on the macroeconomy? Emerg. Mark. Finance Trade 58 (9), 2652–2669. doi:10.1080/1540496x.2021.2007880
Love, J. H., Roper, S., and Vahter, P. (2013). Learning from openness: the dynamics of breadth in external innovation linkages. Strategic Manag. J. 35 (11), 1703–1716. doi:10.1002/smj.2170
Marvel, M. R., and Lumpkin, G. T. (2007). Technology entrepreneurs' human capital and its effects on innovation radicalness. Entrepreneursh. Theory Pract. 31 (6), 807–828. doi:10.1111/j.1540-6520.2007.00209.x
McJeon, H., Clarke, L., Kyle, P., Wise, M., Hackbarth, A., Bryant, B. P., et al. (2011). Technology interactions among low-carbon energy technologies: what can we learn from a large number of scenarios? Energy Econ. 33 (4), 619–631. doi:10.1016/j.eneco.2010.10.007
Miao, Q., Chen, C., Lu, Y., and Abrigo, M. (2019). Natural disasters and financial implications for subnational governments: evidence from China. Public Finance Rev. 48 (1), 72–101. doi:10.1177/1091142119884710
Mochizuki, J., and Chang, S. E. (2017). Disasters as opportunity for change: tsunami recovery and energy transition in Japan. Int. J. disaster risk Reduct. 21, 331–339. doi:10.1016/j.ijdrr.2017.01.009
National Bureau of Statistics of China (2020). National Bureau of statistics of China. Available at http://www.stats.gov.cn/english/.
Nel, P., and Righarts, M. (2008). Natural disasters and the risk of violent civil conflict. Int. Stud. Q. 52 (1), 159–185. doi:10.1111/j.1468-2478.2007.00495.x
Normann, H. E. (2015). The role of politics in sustainable transitions: the rise and decline of offshore wind in Norway. Environ. Innovation Soc. Transitions 15, 180–193. doi:10.1016/j.eist.2014.11.002
Noy, I. (2009). The macroeconomic consequences of disasters. J. Dev. Econ. 88 (2), 221–231. doi:10.1016/j.jdeveco.2008.02.005
Noy, I., and Vu, T. B. (2010). The economics of natural disasters in a developing country: the case of vietnam. J. Asian Econ. 21 (4), 345–354. doi:10.1016/j.asieco.2010.03.002
Ober, J., and Kochmańska, A. (2021). Adaptation of innovations in the IT industry in Poland: the impact of selected internal communication factors. Sustainability 14 (1), 140. doi:10.3390/su14010140
Panwar, V., and Sen, S. (2019). Disaster damage records of EM-DAT and DesInventar: A systematic comparison. Econ. Disasters Clim. Change 4 (2), 295–317. doi:10.1007/s41885-019-00052-0
Phan, D. H. B., and Narayan, P. K. (2020). Country responses and the reaction of the stock market to COVID-19—A preliminary exposition. Emerg. Mark. Finance Trade 56 (10), 2138–2150. doi:10.1080/1540496x.2020.1784719
Popp, D. (2005). Lessons from patents: using patents to measure technological change in environmental models. Ecol. Econ. 54 (2), 209–226. doi:10.1016/j.ecolecon.2005.01.001
Porfiriev, B. (2016). The economics of natural disasters. Her. Russ. Acad. Sci. 86 (1), 1–11. doi:10.1134/s1019331616010020
Pradhan, R. P., Arvin, M. B., Nair, M., Bennett, S. E., Bahmani, S., and Hall, J. H. (2018). Endogenous dynamics between innovation, financial markets, venture capital and economic growth: evidence from europe. J. Multinatl. Financial Manag. 45, 15–34. doi:10.1016/j.mulfin.2018.01.002
Raddatz, C. (2007). Are external shocks responsible for the instability of output in Low income countries? - are external shocks responsible for the instability of output in low income countries. J. Dev. Econ. 84 (1), 1–187.
Raghupathi, V., and Raghupathi, W. (2017). Innovation at country-level: association between economic development and patents. J. Innovation Entrepreneursh. 6 (1), 4. doi:10.1186/s13731-017-0065-0
Rai, V., Erik, F., and Trevor, U. (2015). Venture capital in clean energy innovation finance: insights from the US market during 2005-2014. SSRN Electron. J. Available at SSRN 2676216, 2015. doi:10.2139/ssrn.2676216
Ramachandran, R. (2017). Language use in education and human capital formation: evidence from the Ethiopian educational reform. World Dev. 98, 195–213. doi:10.1016/j.worlddev.2017.04.029
Ren, S., Hao, Y., and Wu, H. (2021). Government corruption, market segmentation and renewable energy technology innovation: evidence from China. J. Environ. Manag. 300, 113686. doi:10.1016/j.jenvman.2021.113686
Robinson, J., and Bangwayo-Skeete, P. (2015). “The financial impact of catastrophes and natural disasters: assessing the effect international terrorism and hurricanes on stock markets in the commonwealth caribbean,” in Central Bank of Barbados Annual Review Conference.
Sagar, A. D., and Holdren, J. P. (2002). Assessing the global energy innovation system: some key issues. Energy Policy 30 (6), 465–469. doi:10.1016/s0301-4215(01)00117-3
Sagar, A. D., and van der Zwaan, B. (2006). Technological innovation in the energy sector: R&D, deployment, and learning-by-doing. Energy Policy 34 (17), 2601–2608. doi:10.1016/j.enpol.2005.04.012
Skidmore, M., and Toya, H. (2002). DO natural disasters promote long-run growth? Econ. Inq. 40 (4), 664–687. doi:10.1093/ei/40.4.664
Song, P., Zhang, X., Zhao, Y., and Xu, L. (2020). Exogenous shocks on the dual-country industrial network: A simulation based on the policies during the COVID-19 pandemic. Emerg. Mark. Finance Trade 56 (15), 3554–3561. doi:10.1080/1540496x.2020.1854723
Statistics South Africa (2020). Statistics South Africa. Available at: https://www.statssa.gov.za/.
Strobl, E., Ouattara, B., and Kablan, S. (2020). Impact of hurricanes strikes on international reserves in the Caribbean. Appl. Econ. 52 (38), 4175–4185. doi:10.1080/00036846.2020.1731411
Strobl, E. (2012). The economic growth impact of natural disasters in developing countries: evidence from hurricane strikes in the central American and caribbean regions. J. Dev. Econ. 97 (1), 130–141. doi:10.1016/j.jdeveco.2010.12.002
Tang, C., Xu, Y., Hao, Y., Wu, H., and Xue, Y. (2021). What is the role of telecommunications infrastructure construction in green technology innovation? A firm-level analysis for China. Energy Econ. 103, 105576. doi:10.1016/j.eneco.2021.105576
Wadhwa, A., and Basu, S. (2013). Exploration and resource commitments in Unequal partnerships: an examination of corporate venture capital investments. J. Prod. Innovation Manag. 30 (5), 916–936. doi:10.1111/jpim.12037
Wang, E.-Z., Lee, C.-C., and Li, Y. (2022). Assessing the impact of industrial robots on manufacturing energy intensity in 38 countries. Energy Econ. 105, 105748. doi:10.1016/j.eneco.2021.105748
Wang, E.-Z., and Lee, C.-C. (2022). The impact of clean energy consumption on economic growth in China: is environmental regulation a curse or a blessing? Int. Rev. Econ. Finance 77, 39–58. doi:10.1016/j.iref.2021.09.008
Wang, Q.-J., Feng, G. F., Chen, Y. E., Wen, J., and Chang, C. P. (2019). The impacts of government ideology on innovation: what are the main implications? Res. Policy 48 (5), 1232–1247. doi:10.1016/j.respol.2018.12.009
Wang, Q.-J., Feng, G. F., Wang, H. J., and Chang, C. P. (2021). The impacts of democracy on innovation: revisited evidence. Technovation 108, 102333. doi:10.1016/j.technovation.2021.102333
Wang, Y., Gu, A., and Zhang, A. (2011). Recent development of energy supply and demand in China, and energy sector prospects through 2030. Energy Policy 39 (11), 6745–6759. doi:10.1016/j.enpol.2010.07.002
Wen, H., Lee, C.-C., and Zhou, F. (2021a). Green credit policy, credit allocation efficiency and upgrade of energy-intensive enterprises. Energy Econ. 94, 105099. doi:10.1016/j.eneco.2021.105099
Wen, H., Lee, C.-C., and Zhou, F. (2022). How does fiscal policy uncertainty affect corporate innovation investment? Evidence from China's new energy industry. Energy Econ. 105, 105767. doi:10.1016/j.eneco.2021.105767
Wen, H., Li, N., and Lee, C.-C. (2021b). Energy intensity of manufacturing enterprises under competitive pressure from the informal sector: evidence from developing and emerging countries. Energy Econ. 104, 105613. doi:10.1016/j.eneco.2021.105613
Wen, J., Deng, P., Zhang, Q., and Chang, C. P. (2021c). IS higher government efficiency bringing about higher innovation. Technol. Econ. Dev. Econ. 27 (3), 626–655. doi:10.3846/tede.2021.14269
Wen, J., Feng, G. F., Chang, C. P., and Feng, Z. Z. (2017). Stock liquidity and enterprise innovation: new evidence from China. Eur. J. Finance 24 (9), 683–713. doi:10.1080/1351847x.2017.1347573
Wen, J., Zhao, X.-X., and Chang, C.-P. (2021d). The impact of extreme events on energy price risk. Energy Econ. 99 (NA), 105308–NA. doi:10.1016/j.eneco.2021.105308
Wen, J., Zhao, X., Wang, Q. J., and Chang, C. P. (2020). The impact of international sanctions on energy security. Energy and Environ. 32 (3), 458–480. doi:10.1177/0958305x20937686
Worldbank (2020). World development indicators. Available at: https://databank.worldbank.org/source/world-development-indicators.
Xu, A., and Kouwoaye, A. R. (2019). WTO staff working paper.How do natural disasters affect services trade?
Yang, H.-C., Feng, G. F., Zhao, X. X., and Chang, C. P. (2022). The impacts of energy insecurity on green innovation: A multi-country study. Econ. Analysis Policy 74, 139–154. doi:10.1016/j.eap.2022.01.017
Keywords: climate change, natural disaster, environments and infrastructure design, energy technology evolution, BRICS countries and natural disasters, low-carbon energy innovation, environmental sustainability
Citation: Ahmed N, Ore Areche F, Saenz Arenas ER, Cosio Borda RF, Javier-Vidalón JL, Silvera-Arcos S, Ober J and Kochmańska A (2023) Natural disasters and energy innovation: unveiling the linkage from an environmental sustainability perspective. Front. Energy Res. 11:1256219. doi: 10.3389/fenrg.2023.1256219
Received: 10 July 2023; Accepted: 22 September 2023;
Published: 13 October 2023.
Edited by:
He Li, University of Lisbon, PortugalReviewed by:
Chen Jiang, Huazhong University of Science and Technology, ChinaAibo Zhang, University of Science and Technology Beijing, China
Copyright © 2023 Ahmed, Ore Areche, Saenz Arenas, Cosio Borda, Javier-Vidalón, Silvera-Arcos, Ober and Kochmańska. This is an open-access article distributed under the terms of the Creative Commons Attribution License (CC BY). The use, distribution or reproduction in other forums is permitted, provided the original author(s) and the copyright owner(s) are credited and that the original publication in this journal is cited, in accordance with accepted academic practice. No use, distribution or reproduction is permitted which does not comply with these terms.
*Correspondence: Józef Ober, jozef.ober@polsl.pl