- 1MIIT Key Laboratory of Thermal Control of Electronic Equipment, School of Energy and Power Engineering, Nanjing University of Science and Technology, Nanjing, China
- 2Advanced Combustion Laboratory, School of Energy and Power Engineering, Nanjing University of Science and Technology, Nanjing, China
The flash ignition as a new ignition method has attracted lots of interest from researchers. The flash ignition can successfully achieve distributed ignition in a short time. To study the flash ignition and combustion characteristics of titanium dioxide mixed with iron nanoparticles and aluminum nanoparticles, an appropriate amount of titanium dioxide was added to the iron nanoparticles and aluminum nanoparticles to form the composite material. The ignition phenomenon of mixture materials was recorded by the high-speed camera and the temperature distribution of ignited materials was calculated by using the two-color method. The minimum ignition energy of mixture materials with different content of titanium dioxide and total mass was measured to analyze the method to decrease the minimum ignition energy. The results showed that the effect of the added titanium dioxide was insignificant on the combustion phenomenon of the iron nanoparticles. The temperature was still maintained at approximately 850 K compared with the pure iron nanoparticles. The minimum ignition energy of the mixture materials increased with the increasing content of titanium dioxide. As for the aluminum nanoparticles, titanium dioxide can enhance the explosion phenomenon occurring at the beginning of the flash ignition. In the exposure process. With the content of titanium dioxide in the range of 0%–20%, the minimum ignition energy of the mixture materials decreased greatly. The content increased to the range of 20%–40%, the minimum ignition energy was neglected. When the content was further increased to higher than 60%, the minimum ignition energy gradually increased until it gets the saturation condition.
1 Introduction
In recent years, it has been found some materials can be successfully ignited by the normal camera flash. Different from spark ignition, plasma ignition, and laser ignition, the flash ignition can successfully achieve distributed ignition in hundreds or even tens of milliseconds. Most materials with higher energy density can be ignited by flash, such as aluminum particles (Abboud et al., 2013), carbon nanotubes (Xu et al., 2012; Malec et al., 2013), graphene oxide (Liu D. et al., 2018), and porous silicon (Ohkura et al., 2013a).
However, flash ignition of these materials is only achieved in loose powders or low densities. Consequently, these formulations may not be useful in practical energetic systems where density is critical for an energetic performance. Based on the problems mentioned above, scholars added suitable additives to metallic materials and used flash ignition to analyze the effect of the additives on the flash ignition of metallic materials. The tungsten trioxide reduced the minimum ignition energy of aluminum microparticles significantly (Ohkura et al., 2013b). The optical ignition and combustion properties of aluminum microparticles were greatly enhanced by adding 20 wt% of graphene oxide. The results showed that graphene oxide was an efficient additive to improve the energetic performance of aluminum microparticles, enabling aluminum microparticles to be ignited by optical activation and promoting the combustion of aluminum in the air (Jiang et al., 2018). Subsequently, the study found that the interaction between graphene oxide and graphene fluoride provided heat and free radicals to improve the combustion properties of micro-aluminum (Jiang et al., 2020).
The investigations on the ignition and combustion characteristics of metal microparticles have been performed extensively. However, the combustion rates of nanoparticles had significantly increased over similar microparticles (Yetter et al., 2009; Li et al., 2014). It was due to the higher specific surface area, lower particle spacing, and smaller size of nanoparticles (Mench et al., 1998; Sundaram et al., 2016). As a result, the nanoparticles can be ignited below their own melting point, increasing the combustion rates and efficiency of nanoparticle.
Recently, research on metal nanoparticles has attracted much attention. The oxidation and ignition of the aluminum nanoparticles in a simultaneous TGA–DSC system were divided into three-stage oxidation scenario and the early ignition was responsible for the co-existence of different polymorphs of alumina (Noor et al., 2013). In the a simultaneous TGA–DSC system, the general exothermic characteristics of aluminum–copper nanoalloys were very similar to that of aluminum nanoparticles but the nanoalloy was more reactive. An early ignition was found for both nanomaterials, and the eutectic melting of the nano-alloy was believed to be mainly responsible for its early ignition (Noor et al., 2015). The addition of porous silicon facilitates both the ignition and combustion of aluminum/copper oxide mixtures over a wide range of experimental conditions. The enhancement effects were attributed to the easy ignition and fast-burning properties of porous silicon (Parimi et al., 2016). The addition of nano-ferrocene facilitates the flash ignition process of multi-walled carbon nanotubes by the pulsed xenon lamp. CNT-based photo-induced combustion evolves more rapidly with shorter ignition delays, higher peak pressure values, and a higher fuel burning rate (Carlucci et al., 2017). The effect of molybdenum trioxide as additives on the peak pressure and the linear combustion rate was investigated in aluminum nanoparticles (Zakiyyan et al., 2018). The results showed that the peak pressure and the linear combustion rate were increased. The incorporation of mono-layer carbon nanotubes reduced the minimum ignition energy of aluminum nanoparticles and iron nanoparticles (Liu G. N et al., 2018).
The generation of improved combustion efficiency of metal nanoparticles still needs further investigation. This study aims to investigate the effect of titanium dioxide additions on the ignition and combustion characteristics of aluminum and iron nanoparticles ignited by the flash method, expecting to provide experimental reference and theoretical support for the practical application of metal nanoparticles. Based on the flash ignition system, a series of experiments were conducted to explore the effect of titanium dioxide additions based on the combustion process images, combustion temperature distributions, and minimum ignition energy.
2 Experimental materials and methods
2.1 Materials
The aluminum particles (average diameters of 20–200 nm with the oxide layers), the iron particles (average diameters of 50 nm with the oxide layers), and the titanium dioxide (average diameters of 20 nm) used in experiments were from Shanghai Aladdin Co. Ltd. Figures 1A, B shows the high resolution transmission electron microscope (HRTEM) images of aluminum particles and iron particles. The oxide layer of aluminum particles is 2–3 nm, while that of iron particles is 3–4 nm. These oxide layers can prevent particles from being further oxidized in the air. It is worth noting that all the materials before the experiment were stored in the glovebox filled with argon gas to prevent oxidation.
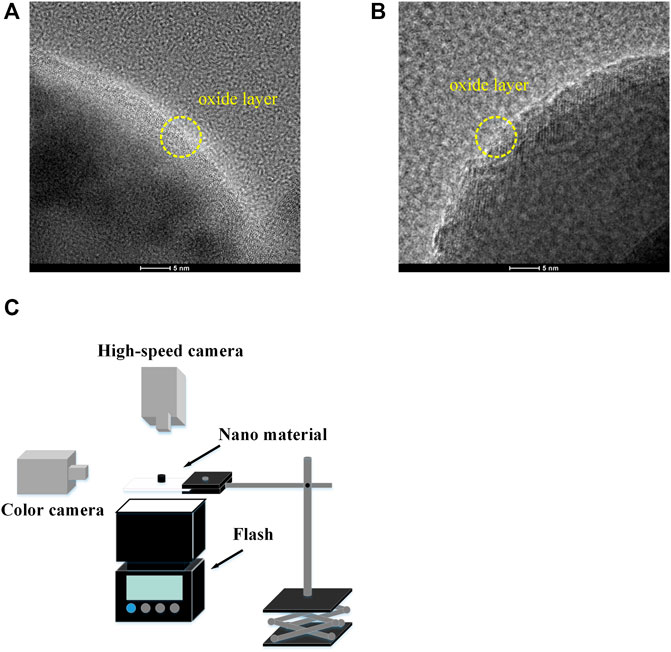
FIGURE 1. (A) The HRTEM image of the aluminum nanoparticles, (B) the HRTEM image of the iron nanoparticles, (C) experiment schematic.
2.2 Flash ignition experiments
2.2.1 Experimental setup
A commercial camera flash (Tripo-690N) was used for ignition and combustion of aluminum-titanium dioxide and the iron-titanium dioxide mixture particles. The duration of the flash was about 7.8 ms. The schematic of flash ignition experiment is shown in Figure 1C. The mixture particles were made into a cylinder and placed on a quartz glass sheet. The quartz glass sheet was fixed on the movable displacement table and placed above the xenon flash tube. For each experiment, a color camera (Nikon D7100) recorded the combustion transient process diagram and a high-speed camera (MIKROTRON Eosens mini1 champion) analyzed the temperature distributions from the top of the sample. Because the flash ignition phenomenon of pure iron nanoparticles was not obvious, a black-white high speed camera (IDT-Y4) was used instead of a color camera.
2.2.2 Temperature distribution measurement
To reveal the effect of titanium dioxide addition on the ignition and combustion characteristics of iron and aluminum particles, experimental conditions were shown in Table 1. For the experiment, the samples were mixed uniformly and directly without significant color difference. Then, the sample was gently packed into a cylinder with a bottom diameter of 5 mm. Three thermal analysis tests were carried out for each condition to ensure accuracy and reliability of the experimental data.
The flash ignition of the samples was multi-point and distributed, so most contact temperature measurement methods were not applicable. In this paper, a modified two-color method was used to determine the combustion temperature distribution of the samples (Ma et al., 2022). Moreover, the images without the flash exposure were used to determine the combustion phenomenons and temperatures of the mixture material particles.
2.2.3 Minimum ignition energy
The combustion of nanoparticles had high deflagration characteristics, which can be transmitted to the whole material region after single-point ignition. Therefore, it can be determined that this position was the minimum ignition distance that can make the material burn. Then the total light energy was measured by a pulse energy meter (GenTec-EO, Solo 2 Series) and the energy density per unit area was calculated. In this process, the exposure energy control of the flash was constant, and the exposure time was constant as 1/128 s.
In order to determine the effect of titanium dioxide addition on the minimum ignition energy of aluminum and iron particles, the experimental conditions were set as shown in Table 2 and Table 3. During the experiment, the distance between the glass piece and the flash lamp was gradually reduced until the sample can be ignited by the flash lamp. Again, to ensure accuracy and reliability, the experiments were repeated three times for each condition and 10 times for the minimum ignition distance.
In this study, X wt% (Al/TiO2) and X wt% (Fe/TiO2) represent different mixture material particles, where the X is the additive content of titanium dioxide. Moreover, a preliminary experimental test was carried out to optimize the experimental conditions.
3 Results and discussion
3.1 Ignition and combustion process
The sample of 10 wt% (Fe/TiO2) as shown in Figure 2C was taken as an example to illustrate the ignition and combustion process in details. The sample cannot be ignited immediately after the flash exposure. At 0.325 s, the particles located on the outer edge of the upper surface started to burn accompanied by a faint red light. Subsequently, the flame propagated along the exterior of the sample in all directions. After 2.26 s, significant combustion phenomenon appeared on the outer edge of the sample. Finally, most of the ignited particles burned out and extinguished. Compared to previous studies (Liu et al., 2017) and the Figure 2A, the addition of titanium dioxide had little effect on the ignition and combustion of iron particles.
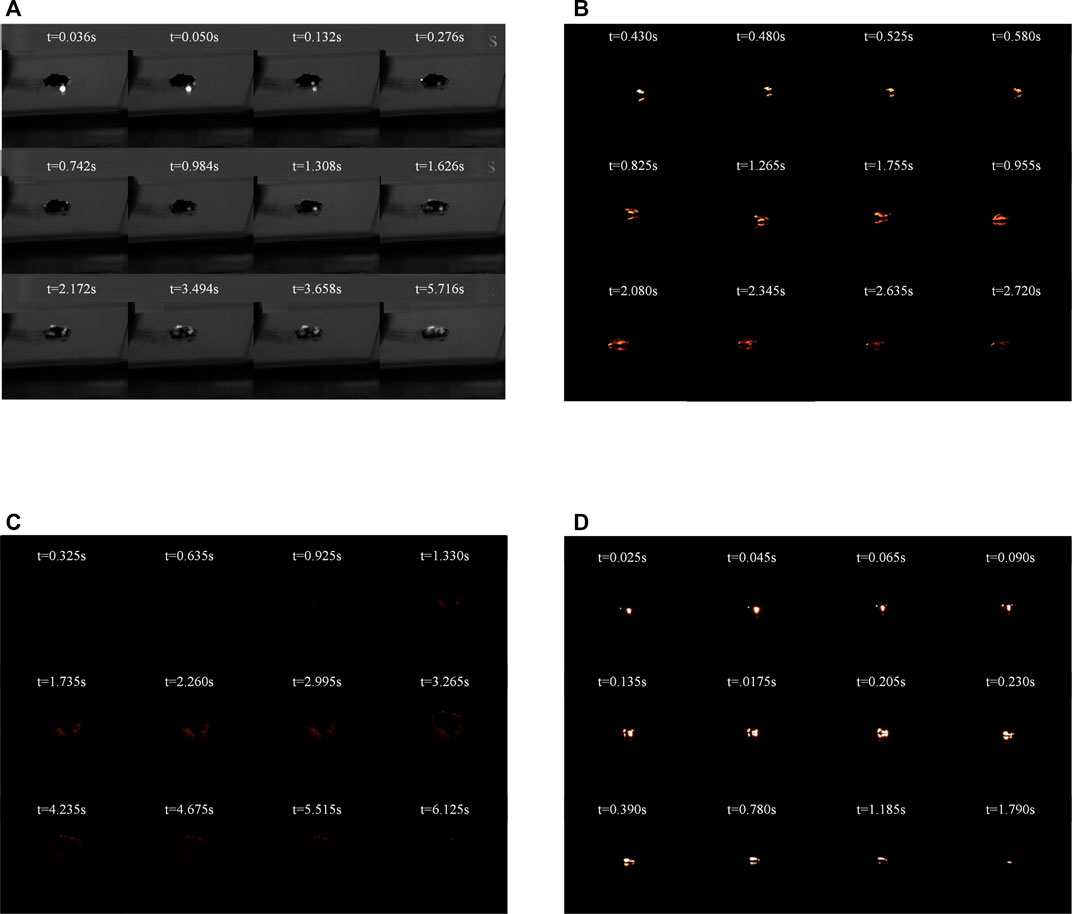
FIGURE 2. The images of the ignition and combustion process (A) pure iron nanoparticles, (B) pure aluminum nanoparticles, (C) 10 wt% (Fe/TiO2), (D) 10 wt% (Al/TiO2).
The aluminum and titanium dioxide mixture materials presented relatively intense combustion phenomenon. Figure 2D showed the ignition and combustion process of the sample of 10 wt% (Al/TiO2). At the moment of flash exposure, the sample started to burn rapidly and emitted a blinding white light. It was observed that the flame initially existed on the outer edge of the upper surface and gradually propagated around within a few tens of milliseconds. 0.2 s later, the flame gathered close to the center and burned until extinguished. Compared with the 10 mg of pure aluminum nanoparticles in Figure 2B need 3–4 s for the flame to disappear, the same mass of sample burned completely in about 2 s and emitted a brighter white light. On the one hand, titanium dioxide as a photocatalyst can enhance the ability of aluminum particles to absorb light energy. On the other hand, titanium dioxide can react with aluminum at high temperature as follows, which further promoteed the ignition and combustion process of aluminum particles.
3.2 Combustion temperature distributions
The combustion temperature distributions of iron and titanium dioxide mixture material shown in Figures 3A–D. The combustion spots were mainly distributed in the external edge region of the sample. In this stage, the average temperature was maintained at approximately 850 K. Moreover, the temperatures of the ignited particles were approximately the same and did not emerge a sequential heating process. As the flash exposure was completed in a few milliseconds, the sample instantly absorbed the light energy and ignited. Afterward, the combusti he high temperature caused the reaction between titanium dioxide and aluminumon of the sample depended to their own released heat. From the results above, it can be concluded that under the different titanium dioxide addition, the ignition and combustion process was quite similar. And the maximum temperature and average temperature in the ignition and combustion process were about 1,050 K and 850 K.
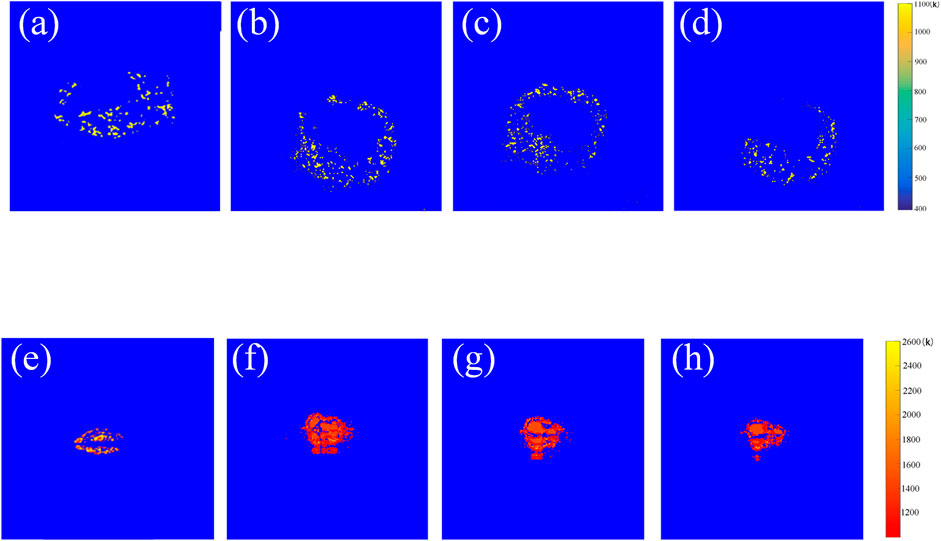
FIGURE 3. Combustion temperature distributions (A) pure iron nanoparticles, (B) 10 wt% (Fe/TiO2), (C) 20 wt% (Fe/TiO2), (D) 30 wt% (Fe/TiO2), (E) pure aluminum nanoparticles, (F) 10 wt% (Al/TiO2), (G) 20 wt% (Al/TiO2), (H) 30 wt% (Al/TiO2).
From Figures 3E–H, it can be found that the combustion spots for the aluminum and titanium dioxide mixture materials were much more than the iron and titanium dioxide mixture materials during the combustion. The maximum temperature at about 2,400 K. Moreover, the temperature distribution showed an significant gradient. At the outer edge of the sample, the average combustion temperature was around 1,200 K, while the average combustion temperature gradually increased to 1,500 K at the center of the sample. Compared with the combustion distribution of aluminum nanoparticles, the addition of titanium dioxide significantly increased the combustion temperature and intensified the combustion intensity of the samples (Ohkura et al., 2011). This is in fair agreement with the result of Figure 2D. Under the conditions in this study, the combustion distribution was not significant difference when changing the content of titanium dioxide. It showed that the addition of titanium dioxide with lower content can significantly promote the ignition and combustion of aluminum. Figure 4 showed the X-rays Diffraction (XRD) of the combustion product of 10 wt% (Al/TiO2). It can be seen that the product contains of titanium. The reason was that the sample instantly absorbed the large energy after the flash exposure and converted it into heat, leading to a rapid rise in temperature. The high temperature caused the reaction between titanium dioxide and aluminum. The reaction provided more oxygen and released more heat. More aluminum ignited at the same time and more energy increased in the mixture materials, which caused the higher temperature.
3.3 Minimum ignition energy
In order to further explore the effect of titanium dioxide on metal particles, the experiment of minimum ignition energy was carried out. Figures 5A–D showed the minimum ignition energy of iron and titanium dioxide mixture materials. The effect of the content of titanium dioxide addition on the total amount of different samples was about the same. When the content of titanium dioxide addition increased from 0% to 40%, the minimum ignition energy was almost constant, but from 40% to 80%, the minimum ignition energy increased with the content of titanium dioxide. In addition, under the same content of titanium dioxide, the minimum ignition energy of the sample in the low mass sample was bigger than that in the high mass sample. This indicated that the addition of titanium dioxide had no significant effect on the ignition and combustion of iron. It was the iron that absorbed light energy in the iron and titanium dioxide mixture materials. After the flash exposure, part of the light energy was absorbed through the blackbody effect of iron, and the temperature of the mixture materials increased. However, the oxidation activity of iron was not as high as that of titanium dioxide, resulting in the inability to react at high temperatures, and titanium dioxide itself cannot be ignited, which leads to relatively moderate combustion phenomenon. With the addition of less titanium dioxide, iron can still absorb enough light energy to be ignited, and the minimum ignition energy did not change significantly. When the content of titanium dioxide increased, the light energy absorbed by iron decreased at the same time to increase the minimum ignition energy.
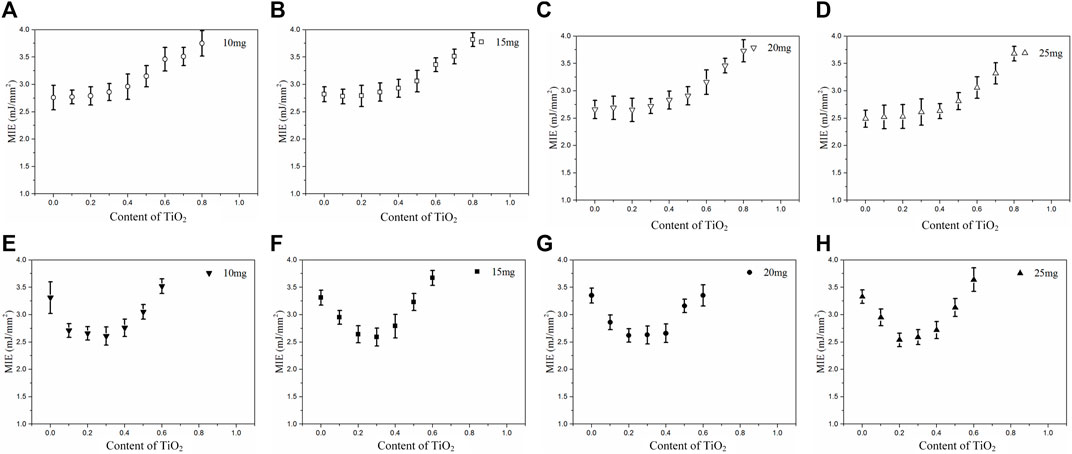
FIGURE 5. The minimum ignition energy. (A–D) different mass of (Fe/TiO2), (E–H) different mass of (Al/TiO2).
The minimum ignition energy for all the aluminum and titanium dioxide mixture materials was summarized in Figures 5E–H. When content of titanium dioxide addition increased from 0%–20%, the minimum ignition energy of the mixture materials was significantly reduced. The contact area between aluminum and titanium dioxide in the mixture was much larger than that between aluminum and air (Chernenko et al., 1988; Yang et al., 2003). After reaching the ignition temperature, aluminum reacted with titanium dioxide to release more heat. At the same time, titanium dioxide provided more oxygen for the ignition and combustion of aluminum. On the other hand, the titanium dioxide produced photogenerated holes (h+) and photogenerated electrons (e−) with strong oxidation promotion, which made aluminum easily to oxidize under the action of light. Moreover, aluminum nanoparticles can enhance the local photothermal energy deposition as active photothermal media to attribute to localized surface plasmon resonance-enhanced photothermal effects (Chong et al., 2013; Uhlenhake et al., 2021). When the content of titanium dioxide was 20%–40%, the minimum ignition energy of the mixture materials remained essentially constant. Although the titanium dioxide promoted the oxidation of aluminum, excessive content of titanium dioxide caused aluminum not to absorb enough energy. When the content of titanium dioxide was above 40%, the minimum ignition energy of the mixture increased rapidly until it was impossible to ignite. At this stage, the content of titanium dioxide played a dominant role. As a result of excessive content of titanium dioxide, the sample required greater optical energy density to rise to the ignition point. Compared with Figures 5E–H, it was found that the effect of titanium dioxide on the minimum ignition energy was basically the same, which showed that the effect of the total mass of the sample on the minimum ignition energy was much less than that of titanium dioxide.
4 Conclusion
The influences of the added titanium dioxide on the flash ignition and combustion processes of the iron and aluminum were investigated in this study. Titanium dioxide had no significant effect on the flash ignition of iron nanoparticles. It neither improved the macroscopic phenomenon during combustion nor increased the combustion temperature. The average temperature of the iron and titanium dioxide mixture materials was still maintained at approximately 850 K determined by the two-color pyrometry method. When the content of titanium dioxide exceeded 30%, excessive content of titanium dioxide even reduced the minimum ignition energy of iron. On the contrary, aluminum and titanium dioxide mixture materials presented relatively intense combustion phenomenon and the combustion time was significantly shortened. The maximum temperature of the ignited particles was as high as 2,400 K. The minimum ignition energy results showed that an appropriate amount of titanium dioxide can significantly reduce the minimum ignition energy of aluminum.
Data availability statement
The raw data supporting the conclusions of this article will be made available by the authors, without undue reservation.
Author contributions
RY and YL: investigation, data curation, formal analysis, validation and writing-original draft; GL: investigation, formal analysis, writing-review and editing; YY and TL: writing-review and editing; DL: methodology, investigation, formal analysis, writing-review and editing, supervision, project administration, and funding acquisition.
Funding
This work was supported by the National Natural Science Foundation of China (52076110, 52106160), Jiangsu Provincial Natural Science Foundation of China (BK20200490, BK20220955) and the Fundamental Research Funds for the Central Universities (30920031103).
Conflict of interest
The authors declare that the research was conducted in the absence of any commercial or financial relationships that could be construed as a potential conflict of interest.
Publisher’s note
All claims expressed in this article are solely those of the authors and do not necessarily represent those of their affiliated organizations, or those of the publisher, the editors and the reviewers. Any product that may be evaluated in this article, or claim that may be made by its manufacturer, is not guaranteed or endorsed by the publisher.
References
Abboud, J. E., Chong, X. Y., Zhang, M. J., Zhang, Z. L., Jiang, N. B., Roy, S., et al. (2013). Photothermally activated motion and ignition using aluminum nanoparticles. Appl. Phys. Lett. 102, 023905. doi:10.1063/1.4776660
Carlucci, A. P., Visconti, P., Primiceri, P., Strafella, L., Ficarella, A., and Laforgia, D. (2017). Photo-induced ignition of different gaseous fuels using carbon nanotubes mixed with metal nanoparticles as ignitor agents. Combust. Sci. Technol. 189, 937–953. doi:10.1080/00102202.2016.1256880
Chernenko, E. V., Afanas'Eva, L. F., Lebedeva, V. A., and Rozenband, V. I. (1988). Inflammability of mixtures of metal oxides with aluminum. Combust. Explos. Shock Waves 24, 639–646. doi:10.1007/bf00740402
Chong, X. Y., Jiang, N. B., Zhang, Z. L., Roy, S., and Gord, J. R. (2013). Plasmonic resonance-enhanced local photothermal energy deposition by aluminum nanoparticles. J. Nanopart. Res. 15, 1678. doi:10.1007/s11051-013-1678-2
Jiang, Y., Deng, S. L., Hong, S., Tiwari, S., Chen, H. H., Nomura, K., et al. (2020). Synergistically chemical and thermal coupling between graphene oxide and graphene fluoride for enhancing aluminum combustion. ACS Appl. Mat. Interfaces 12, 7451–7458. doi:10.1021/acsami.9b20397
Jiang, Y., Deng, S. L., Hong, S., Zhao, J. H., Huang, S. D., Wu, C. C., et al. (2018). Energetic performance of optically activated aluminum/graphene oxide composites. ACS Nano 12, 11366–11375. doi:10.1021/acsnano.8b06217
Li, X., Zhao, F. Q., Hao, H. X., Luo, Y., Xu, S. Y., Yao, E. G., et al. (2014). Research on ignition and combustion properties of different micro/nano-aluminum powders. Acta Armamentarii 35, 640–647. doi:10.3969/j.issn.1000-1093.2014.05.010
Liu, D., Liu, Y. X., and Liu, G. N. (2018). Effects of carbon nanotubes additions on flash ignition characteristics of Fe and Al nanoparticles. Fullerenes, Nanotub. Carbon Nanostruct 26, 168–174. doi:10.1080/1536383x.2017.1420062
Liu, G. N., Liu, D., Zhu, J. W., Wei, J. L., Cui, W., and Li, S. Q. (2018). Energy conversion and ignition of fluffy graphene by flash light. Energy 14, 669–678. doi:10.1016/j.energy.2017.12.062
Liu, Y. X., Liu, D., and Liu, G. N. (2017). Energy conversion and ignition of iron nanoparticles by flash. Sci. China Technol. Sci. 60, 1878–1884. doi:10.1007/s11431-017-9144-6
Ma, M. M., Liu, G. N., Qin, Z., Zhang, R., Ying, Y. Y., Xu, L., et al. (2022). Effects of aluminum addition on flash ignition and combustion of boron nanoparticles. Combust. Flame 236, 111762. doi:10.1016/j.combustflame.2021.111762
Malec, C. D., Voelcker, N. H., Shapter, J. G., Joseph, G., and Ellis, A. V. (2013). Carbon nanotubes initiate the explosion of porous silicon. Mat. Lett. 64, 2517–2519. doi:10.1016/j.matlet.2010.08.021
Mench, M. M., Kuo, K. K., Yeh, C. L., and Lu, Y. C. (1998). Comparison of thermal behavior of regular and ultra-fine aluminum powders (alex) made from plasma explosion process. Combust. Sci. Technol. 135, 269–292. doi:10.1080/00102209808924161
Noor, F., Vorozhtsov, A., Lerner, M., and Wen, D. S. (2015). Exothermic characteristics of aluminum based nanomaterials. Powder Technol. 282, 19–24. doi:10.1016/j.powtec.2014.12.058
Noor, F., Zhang, H., Korakianitis, T., and Wen, D. S. (2013). Oxidation and ignition of aluminum nanomaterials. Phys. Chem. Chem. Phys. 15, 20176–20188. doi:10.1039/c3cp53171f
Ohkura, Y., Rao, P. M., Cho, I. S., and Zheng, X. L. (2013a). Reducing minimum flash ignition energy of Al microparticles by addition of WO3 nanoparticles. Appl. Phys. Lett. 102, 043108. doi:10.1063/1.4790152
Ohkura, Y., Rao, P. M., and Zheng, X. L. (2011). Flash ignition of Al nanoparticles: Mechanism and applications. Combust. Flame 158, 2544–2548. doi:10.1016/j.combustflame.2011.05.012
Ohkura, Y., Weisse, J. M., Cai, L. L., and Zheng, X. L. (2013b). Flash ignition of freestanding porous silicon films: Effects of film thickness and porosity. Nano Lett. 13, 5528–5533. doi:10.1021/nl403114g
Parimi, V. S., Huang, S., and Zheng, X. L. (2016). Enhancing ignition and combustion of micron-sized aluminum by adding porous silicon. Proc. Combust. Inst. 36, 2317–2324. doi:10.1016/j.proci.2016.06.185
Sundaram, D. S., Puri, P., and Yang, V. (2016). A general theory of ignition and combustion of nano- and micron-sized aluminum particles. Combust. Flame 169, 94–109. doi:10.1016/j.combustflame.2016.04.005
Uhlenhake, K. E., Olsen, D., Gomez, M., Ornek, M., Zhou, M., and Son, S. F. (2021). Photoflash and laser ignition of full density nano-aluminum PVDF films. Combust. Flame 233, 111570. doi:10.1016/j.combustflame.2021.111570
Xu, X., Xiang, S. B., Wang, Z., Wang, X., and Hua, G. (2012). Photo-responsive behaviors and structural evolution of carbon-nanotube-supported energetic materials under a photoflash. Mat. Lett. 88, 27–29. doi:10.1016/j.matlet.2012.08.049
Yang, Y. Q., Sun, Z. Y., Wang, S. F., and Dlott, D. D. (2003). Fast spectroscopy of laser-initiated nanoenergetic materials. J. Phys. Chem. B 107, 4485–4493. doi:10.1021/jp0269322
Yetter, R. A., Risha, G. A., and Son, S. F. (2009). Metal particle combustion and nanotechnology. Proc. Combust. Inst. 32, 1819–1838. doi:10.1016/j.proci.2008.08.013
Keywords: flash ignition, iron nanoparticles, aluminum particles, minimum ignition energy, titanium dioxide
Citation: Yu R, Liu Y, Liu G, Ying Y, Li T and Liu D (2023) Effects of titanium dioxide additions on flash ignition characteristics of aluminum and iron nanoparticles. Front. Energy Res. 11:1090217. doi: 10.3389/fenrg.2023.1090217
Received: 05 November 2022; Accepted: 23 January 2023;
Published: 07 February 2023.
Edited by:
Covadonga Pevida, National Coal Institute (CSIC), SpainReviewed by:
Fahad Noor, University of Engineering and Technology, Lahore, PakistanIbukun Oluwoye, Murdoch University, Australia
Copyright © 2023 Yu, Liu, Liu, Ying, Li and Liu. This is an open-access article distributed under the terms of the Creative Commons Attribution License (CC BY). The use, distribution or reproduction in other forums is permitted, provided the original author(s) and the copyright owner(s) are credited and that the original publication in this journal is cited, in accordance with accepted academic practice. No use, distribution or reproduction is permitted which does not comply with these terms.
*Correspondence: Guannan Liu, Z3Vhbm5hbi5saXUwOUBnbWFpbC5jb20mI3gwMjAwYTs=; Dong Liu, ZG9uZ2xpdUBuanVzdC5lZHUuY24=
†These authors have contributed equally to this work