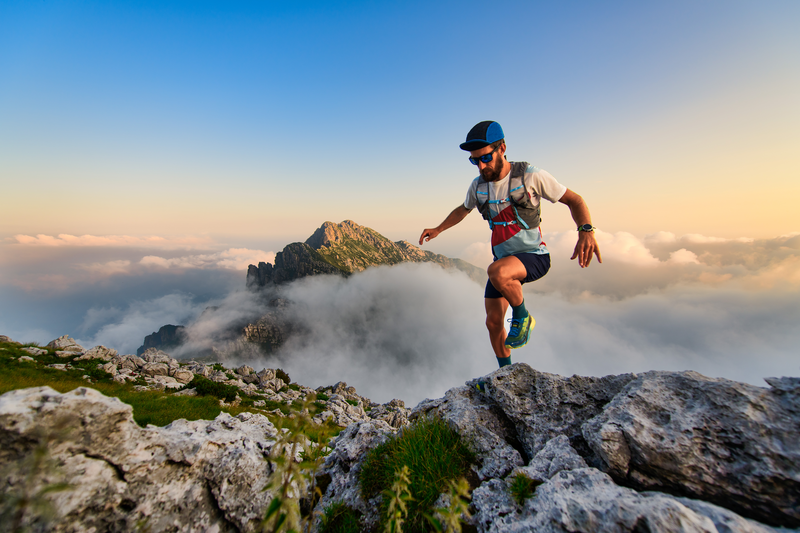
94% of researchers rate our articles as excellent or good
Learn more about the work of our research integrity team to safeguard the quality of each article we publish.
Find out more
ORIGINAL RESEARCH article
Front. Energy Res. , 28 February 2023
Sec. Nuclear Energy
Volume 11 - 2023 | https://doi.org/10.3389/fenrg.2023.1085788
This article is part of the Research Topic Benchmark Experiments, Development and Needs in Support of Advanced Reactor Design View all 12 articles
The International Criticality Safety Benchmark Evaluation Project, a sanctioned program under the auspices of the Nuclear Energy Agency of the Organisation for Economic Co-operation and Development, has been a highly successful and productive collaboration, now encompassing over 5,000 evaluated experimental benchmarks trusted and relied upon throughout the international nuclear communities. The success of this project led to the development of the International Reactor Physics Experiment Evaluation Project, which is dedicated to the evaluation of benchmark experiment data to sustain current and future reactor physics validation needs. These exemplary programs, and their widely utilized handbooks, serve as gold standards to which other databases strive to emulate. The purpose of the two projects is to preserve modern and legacy experimental data and evaluate it in a standardized handbook format to provide quality benchmarks to support modern and future criticality safety and reactor physics validation. These two projects have often served as the mechanism through which historic and modern neutronics experiments are evaluated and shared across international borders, to best provide unique, high-quality peer-reviewed, and often otherwise unavailable, benchmark data. The contents of these handbooks are utilized not only in validating criticality safety, reactor physics, and advanced reactor calculations, but are used to validate neutronics calculations and nuclear data for most other nuclear applications. This manuscript discusses both international programs and available content to enable advanced reactor design validation.
What began as an emblematic effort to bolster best practices in the 1990s for nuclear criticality safety validation has evolved into the backbone testing suite for contemporary neutronics methodologies, simulation, and nuclear data (Palmiotti, et al., 2014). The International Criticality Safety Benchmark Evaluation Project (ICSBEP) (Briggs, et al., 2003), a sanctioned program under the auspices of the Nuclear Energy Agency (NEA) of the Organisation for Economic Co-operation and Development (OECD), has been a highly successful and productive collaboration, now encompassing over 5,000 evaluated experimental benchmarks trusted and relied upon throughout the international nuclear communities. The success of the ICSBEP led to the development of the International Reactor Physics Experiment Evaluation Project (IRPhEP) (Briggs and Gulliford, 2014), which is dedicated to the evaluation of benchmark experiment data to sustain current and future reactor physics validation needs. The benchmark experiments, covering a wide range of applications, from thermal light water reactors (LWRs) and high temperature reactors (HTRs) to fast reactors of various designs or even special applications, are most often obtained via zero power reactors, many of which have been shut down or are nearing the end of their expected lifetimes. These two exemplary benchmark programs, and their widely utilized handbooks, serve as gold standards to which other databases strive to emulate, such as the OECD NEA Spent Fuel Composition (SFCOMPO) database (Michel-Sendis, et al., 2017), the OECD/IAEA (International Atomic Energy Agency) co-sponsored International Fuel Performance (IFPE) collection (Menut, et al., 2000), the International Experimental Thermal Hydraulics Systems (TIETHYS) database (Rohatgi, et al., 2018), the collection of multi-physics experiments being created by the NEA Expert Group on Reactor Systems Multi-Physics (EGMUP) (Valentine, et al., 2018), and the OECD-NEA/RSICC (Radiation Safety Information Computational Center) co-sponsored Shielding Integral Benchmark Archive and Database (SINBAD) (Kodeli and Sartori, 2021).
From the inception of the ICSBEP until very recently, both the ICSBEP and IRPhEP have been managed by a single chair, nominated and endorsed by the technical review group (TRG), whom has historically been from the United States (US). Most recently, the management of these projects has been divided into two separate chair and vice chair positions and expanded to include non-US representatives. The purpose of the two projects is to preserve both legacy and current generation experiment data and evaluate it in a standardized handbook format to provide quality benchmarks to support modern and future criticality safety and reactor physics validation. These two projects have often served as the mechanism through which historic and modern neutronics experiments are evaluated and shared across international borders, to best provide unique, high-quality peer-reviewed, and often otherwise unavailable, benchmark data. The handbooks provide a consolidated, maintained repository for benchmark data, instead of a landscape of fragmented information spread throughout various scientific journals, laboratory reports, and conference proceedings (Bess and Ivanova, 2020). Furthermore, detailed neutron spectral characteristics are calculated and provided in the handbook for most benchmark configuration to enable users to clearly understand the range of their applicability (Rozhikhin, 1999).
A key fact to note is that these benchmarks preserve and evaluate experiments performed to support past, current, and future nuclear research needs. There is constant variability in programmatic needs amongst and between various international entities. The contents of these handbooks have enabled the development of nuclear data and codes now used to design the next-generation of nuclear reactors. Various numerous benchmarks can assist in the advanced reactor development, although no doubt additional benchmarks will be needed.
A historic summary of investment costs with conservative estimates of the monetary and intrinsic values of these two programs has been independently assessed:
“The total cost of the IRPhEP and ICSBEP over the past 2 decades is approximately $50 million, while the data obtained from those two programs can easily be valued at over $1.5 billion (estimate conservatively based on 5,000 experiments at $300,000 per experiment). If one new reactor or reprocessing facility is designed and built using advanced simulation methods validated only by legacy data without the construction of a separate critical facility, the entire cost of the two programs will be offset by many times. Furthermore, the IRPhEP and ICSBEP activities have helped pass knowledge from those involved in these legacy experiments to future generations and have influenced the development of future experimental programs.” (Palmiotti, et al., 2014)
Although benchmarks represent a cost investment, they very nearly always cost significantly less than the costs associated with construction and performance of the actual experiments themselves. The benchmarking process not just ensures that all data are collated, but more importantly, the quality of the experimental data are evaluated and preserved.
The Criticality Safety Benchmark Evaluation Project (CSBEP) was initiated in 1992 by the US Department of Energy (US DOE) under the Idaho National Engineering and Environmental Laboratory (INEEL). In 1995, the CSBEP became an official activity of the NEA Nuclear Science Committee at which time the name was changed to the ICSBEP. Historically, the management of the projects was funded in the US via the Nuclear Criticality Safety Program (NCSP) under the National Nuclear Security Administration (NNSA), but for approximately the past decade the management has been funded under the Nuclear Engineering Advanced Modeling and Simulation (NEAMS) program within the US Department of Energy, Office of Nuclear Energy (DOE-NE). Initial funding to support the establishment of the IRPhEP within the OECD NEA was graciously provided by the Japan Atomic Energy Agency (JAEA). Management of these projects includes coordination of benchmark evaluation and reviews, organizing TRG meetings, publication of the handbooks, and user engagement via international conferences and meetings, in close cooperation with NEA. Typically, additional funding has been provided to universities in the US via annual Nuclear Energy University Program (NEUP) MS-NE-1 awards to contribute to the handbooks. Funding for the evaluation and review of benchmarks, worldwide, has often been contributed via government, corporate, or even individual entities from 28 countries and OECD NEA seeking to improve upon the available content found within these handbooks.
DOE-NE has previously utilized the MS-NE-1 award to direct US reactor physics benchmark development. Prioritization and funding of criticality safety benchmarks in the US are currently managed by the NCSP. Prioritization of other reactor physics benchmarks in the US are provided by individual projects, organizations, and/or companies. A historic example includes Next-Generation Nuclear Plant (NGNP) funding of eight benchmark evaluations of graphite-moderated reactor systems with tristructural-isotropic (TRISO) fuel. International entities fund and prioritize their own benchmark development depending upon their respective needs, international collaborative activities, and/or university-driven research interests. However, through the ICSBEP and IRPhEP, independent technical review is coordinated and provided to ensure the utmost quality in modern benchmark development for these handbooks, at a peer-review standard greater than most, if not all, published scientific and engineering journals.
The International Handbook of Evaluated Criticality Safety Benchmark Experiments (ICSBEP Handbook) (NEA, 2022a) is primarily utilized in criticality safety, neutronics code validation, and nuclear data validation. We would not have, nor continue to maintain, the quality of neutronics codes implemented today without this handbook, as many of the ICSBEP evaluations are established key benchmarks in neutronics software validation suites. The International Handbook of Evaluated Reactor Physics Benchmark Experiments (IRPhEP Handbook) (NEA, 2022b) is used by reactor physicists that need further validation for specific reactor design and experiment characterization; it also supports specific nuclear data validation needs that cannot be provided via eigenvalue benchmark evaluations alone. International nuclear regulatory agencies, government facilities, industrial, and commercial entities utilize these handbooks to validate their codes and data to support neutronics modeling and simulation of the current power and research reactor fleets. Universities use them primarily for training and education purposes world-wide. These benchmark handbooks are known as the quality standard for international benchmarking activities. They are implemented internationally in advanced modeling and simulation; analytical and computational methods development, validation, and verification; reactor design and licensing; training; criticality and reactor safety analyses; fuel cycle and related activities; range of applicability and experiment design; and nuclear data refinement. Without modern integral benchmarks, we would be unable to reduce uncertainties in nuclear data, which are fundamental in sustaining practical, reliable, and realistic computational analyses.
The 2022 edition of the ICSBEP Handbook, when published, will include 592 evaluations containing acceptable benchmark specifications for 5,144 critical, subcritical, or near-critical configurations, representing contributions from 26 countries. An additional 838 configurations deemed unacceptable to support criticality safety requirements, yet present historical and legacy value, are also preserved within the handbook. There are eight criticality alarm placement/shielding evaluations containing a total of 46 benchmark configurations, and 11 fundamental physics evaluations containing a total of 246 measurements relevant to criticality safety applications. A summary of the ICSBEP Handbook contents in provided in Table 1. The 2022 edition of the IRPhEP Handbook will include data for 57 unique nuclear facilities with evaluations containing benchmark specifications for 170 experimental series, of which four are draft benchmark specifications yet to be formally adopted, with contributions from 25 countries. A summary of the IRPhEP Handbook contents is shown in Table 2. Many reactor physics benchmark evaluations include additional data besides criticality measurements, e.g., buckling and extrapolation length, spectral characteristics, reactivity effects, reactivity coefficients, kinetics measurements, reaction-rate distributions, power distributions, isotopic composition, and other miscellaneous types of measurements. Full technical details can be found for the various benchmark evaluations within the latest editions of these handbooks. The following countries have contributed towards the success of these two benchmark projects: Argentina, Austria, Belarus, Belgium, Brazil, Canada, the People’s Republic of China, the Czech Republic, Denmark, France, Germany, Hungary, India, Israel, Italy, Japan, Kazakhstan, Poland, Republic of Korea, the Russian Federation, Serbia, Slovenia, South Africa, Spain, Sweden, Switzerland, United Kingdom, and United States of America.
TABLE 2. Contents summary for the 2022 edition of the IRPhEP handbook.b
The already extensive and yearly increasing handbooks’ content required development of an evolving database structure to collate, organize, and better facilitate their use. The Database for the International Handbook of Evaluated Criticality Safety Benchmark Experiments (DICE) (Nouri, et al., 2003) was developed specifically for the ICSBEP Handbook. This relational database is loaded with pre-selected information from each of the benchmark evaluations. A users’ interface enables querying for specific parameters to identify benchmarks suitable for their respective needs. DICE also includes the ability to plot and compare neutron spectra and sensitivity coefficients for many evaluations. The IRPhEP Database and Analysis Tool (IDAT) (Hill, et al., 2014) was similarly developed for use with the IRPhEP Handbook. This database tool dramatically simplifies the identification of validation cases from the various reactor types and their respective benchmark measurements. The database also contains calculated quantities of the reactor systems such as neutron flux, capture, and fission spectrum data; neutron balance data; and sensitivity data. The ability to visualize these datasets is also included in IDAT.
The benchmark and experiment design community benefits from the development of uncertainty guides for both criticality safety and reactor physics measurements. Participants in these international projects contribute their expertise to enable the preservation of best practices, both historically and at present, for the benefit of current and future benchmark evaluation. Characterization and quantification of the typical uncertainties encountered for the varying measurements in criticality safety and reactor physics systems further contributes towards activities extended beyond typical benchmark evaluation and validation such as training, licensing, and design.
The ICSBEP Guide to the Expression of Uncertainties (Dean and Blackwood, 2008) was developed primarily to address uncertainties in the evaluation and characterization of critical experiments, i.e., measurement of keff. The International Reactor Physics Experiments Evaluation Project (IRPhEP) Guide to the Expression of Uncertainty (Dos Santos, et al., 2018) was more recently developed to support evaluation of uncertainties in the evaluation and characterization of other types of measurements aside from criticality, such as, buckling, spectral characteristics, reactivity effects/coefficients, kinetics, and reaction-rate/power distributions. These uncertainty guides are included in the ICSBEP and IRPhEP Handbooks and publicly available on-line.
The fundamentally cross-cutting nature of the handbooks serves to enable neutronics simulation to support nuclear safety and design through all aspects of the nuclear fuel cycle. Furthermore, the framework is established such that new benchmark evaluation data can be prepared, reviewed, and published for current and future handbook users. An overview of the benchmark evaluation process for these two international programs is shown in Figure 1. Brief examples of benchmarks relevant to contemporary research and development needs are provided in the subsections below. Explanations regarding the nomenclature for the report identifiers are located within the ICSBEP and IRPhEP Handbooks; some benchmark evaluations are cross listed within both handbooks.
The BFS-1 critical facility (shown in Figure 2) of the Institute of Physics and Power Engineering (IPPE) in Obninsk, Russia, was utilized to provide full-scale mockups of fast reactor cores including additional neutron physics measurements. The BFS-61 experiments included three core designs with lateral reflectors of depleted UO2, lead, and steel pellets contained within stainless steel tubes. The core itself contained Pu metal fuel with additional pellets of Pb, depleted U metal, and graphite. Additional spectral and reaction rate measurements have also been evaluated. Benchmark identifiers: BFS1-LMFR-EXP-002 (IRPhEP)/MIX-MET-FAST-006 (ICSBEP).
The Experimental Breeder Reactor-II (EBR-II) was a 62.5 MWth test reactor operated by Argonne National Laboratory (ANL) between 1964 and 1994, and initially used to demonstrate operability of a SFR, but later to support a myriad of testing needs. One of the most important experiments was the Shutdown Heat Removal Tests (SHRT) to test a liquid metal reactor during catastrophic failures of heat removal at full power. The critical configuration for the most extreme of these tests, SHRT 45, was evaluated. Benchmark identifier: EBR2-LMFR-RESR-001 (IRPhEP).
JOYO is Japan’s first experimental fast reactor and was constructed at the Oarai Engineering Center. Its purpose was to acquire data for fast reactor performance including nuclear characteristics, thermal hydraulics, and safety-related features. This benchmark includes evaluation of the first and second critical core loadings, six control rod worth measurements, six sodium void reactivities, six fuel replacement reactivities, one isothermal temperature coefficient, and five burnup reactivity coefficients. Benchmark identifier: JOYO-LMFR-RESR-001 (IRPhEP).
ZPR-3 (Zero Power Reactor) was the first of four fast critical facilities constructed by ANL. While constructed to support fast reactor development, data from some of the ZPR assemblies were also well suited for nuclear data validation needs. Assemblies 58 and 59 were constructed to test the worth of replacing a depleted U reflector with Pb. Benchmark identifier: PU-MET-INTER-004 (ICSBEP).
The Zero Power Physics Reactor (ZPPR) was the last fast critical facility constructed by ANL. ZPPR-11, -12, and -13 were constructed to perform engineering mockup tests for the Clinch River Breeder Reactor (CRBR). The measurements in ZPPR-12 were designed specifically to address sodium void worth, cell heterogeneity, and the effects of changes in neutron streaming upon reactivity worth. Benchmark identifier: ZPPR-LMFR-EXP-010 (IRPhEP).
The zero-power reactor LR-0 at Research Centre Řež in the Czech Republic is utilized to mockup various tests for material testing and vodo-vodyanoi enyergeticheskiy reactors (VVERs). Tests were performed in 2014–2015 to support MSR and FHR development with material insertions in the center of the reactor containing various quantities of graphite, FLINA (LiF and NaF mixture), or FLIBE (LiF and BeF2 mixture), as shown in Figure 3. Benchmark identifier: LR (0)-VVER-RESR-003 (IRPhEP).
FIGURE 3. Schematic of the VVER core loading (Top) with various arrangements of central module testing materials (Bottom).
The MSRE was built and operated at Oak Ridge National Laboratory from 1965 to 1969 with the explicit purpose to demonstrate key features of MSR technology. The initial zero-power nuclear experiments were to establish the basic nuclear characteristics as a baseline for evaluating system performance. The initial critical core has been evaluated. There are a total of seventeen critical configurations with neutron spectra measurements for three cores: void center, graphite center, and FLINA center. Benchmark identifier: MSRE-MSR-RESR-001 (IRPhEP).
The versatile PROTEUS facility from the Paul Scherrer Institute (PSI) in Villigen, Switzerland was utilized to support measurements for various reactor concepts. The modular HTR series investigated 11 core loadings with TRISO-laden graphite pebbles from 1992 to 1996. Core four represented a randomly packed core of moderator and fuel pebbles with a 1:1 ratio (as demonstrated in Figure 4). The critical configuration and control rod worths are evaluated as benchmark experiment data. Benchmark identifier: PROTEUS-GCR-EXP-002 (IRPhEP).
The aforementioned PROTEUS facility also supported GCFR research from 1972 to 1979. Core 11 served as a reference configuration to test reproducibility and evaluate infinitely dilute cross sections of 232Th, 233U, and 237Np with a Pu-fueled core driven by a UO2-fueled annulus within a D2O shield annular tank and radial graphite reflector. Nine spectral indices were evaluated for this configuration. Benchmark identifier: PROTEUS-GCFR-EXP-001 (IRPhEP).
On the third fast critical facility by ANL, loadings 28 through 30 on ZPR-9 represented three phases supporting GCFR research in the US in 1975–1976. Criticality, spectral measurements, and reactivity measurements were evaluated for Phase II of this series, which was an unreflected core with ∼43% void/gas fraction. Benchmark identifier: ZPR-GCFR-EXP-001 (IRPhEP).
The High Temperature Engineering Test Reactor (HTTR) of the Japan Atomic Energy Agency (JAEA) is a 30 MWth, graphite-moderated, helium-cooled reactor constructed to establish and upgrade the technological bases for advanced high-temperature gas-cooled reactors (HTGRs). It is fueled with TRISO within graphite blocks. The fully loaded start-up core was operated in 1998 and evaluated as a benchmark. Evaluated measurements include a critical and subcritical core, excess reactivity, shutdown margin, and an axial reaction-rate distribution. Benchmark identifier: HTTR-GCFR-RESR-001 (IRPhEP).
Cores 9 and 10 from the HTR-PROTEUS experimental program contained hand-stacked TRISO-fueled pebbles with columnar hexagonal point-on-point packing and a moderator-to-fuel pebble ratio of 1:1. Criticality and rod worth measurements were evaluated for each core. These two cores are nearly identical, with Core 10 containing a shorter stack of pebbles and 654 polyethylene rods to simulate water ingress. Benchmark identifier: PROTEUS-GCR-EXP-004 (IRPhEP).
The Very High Temperature Reactor Critical Assembly (VHTRC), as shown in Figure 5, is another JAEA facility dedicated to the verification of calculation accuracy to support neutronic design of the HTTR. Seven critical configurations and ten temperature coefficients were evaluated for this BISO-fueled graphite reactor mockup facility. Benchmark identifier: VHTRC-GCR-EXP-001 (IRPhEP).
The Kilopower Reactor Using Stirling Technology (KRUSTY) demonstration was performed in 2017–2018 at the National Criticality Experiments Research Center (NCERC) in the US. Five of the sixty critical configurations were evaluated for this 93.1 wt% 235U U-Mo annular fuel reflected by BeO and shielded by stainless steel. The entire series was designed to test power generation from a nuclear reactor via heat pipes connected to Stirling engines. Benchmark identifiers: KRUSTY-SPACE-EXP-001 (IRPhEP)/HEU-MET-FAST-101 (ICSBEP).
During 1992 to 1993, an experiment was performed at the Kurchatov Institute in Russia to investigate accidental water immersion of a TOPAZ space reactor. Six configurations were evaluated representing various stages of water ingress and complete water immersion. Benchmark identifiers: TOPAZ-SPACE-RESR-001 (IRPhEP)/HEU-COMP-MIXED-003 (ICSBEP).
The ZPPR-20 experimental series provided data for development of the SP-100 nuclear power source for space applications. Phase C represents the reference flight configuration. Phases D and E represented water immersion and earth burial accidents and are also available as benchmarks. Benchmark identifiers: ZPPR-SPACE-EXP-001 (IRPhEP)/HEU-MET-FAST-075 (ICSBEP).
Before installation of a second core into the German nuclear-powered merchant ship, Otto Hahn, a series of zero-power experiments were performed to test the fuel loading, start up, and subcriticality safety issues such as a stuck control rod. These experiments validated theoretical calculations before the core was successfully installed and operated. Currently only the critical configuration (see Figure 6) of the zero-power tests has been evaluated as a benchmark. Benchmark identifiers: OTTOHAHN-PWR-RESR-001 (IRPhEP)/LEU-COMP-THERM-081 (ICSBEP).
FIGURE 6. Quarter-core Top view (Top) and side view (Bottom) of otto hahn reactor core benchmark model.
A series of small, compact critical assembly (SCCA) experiments were performed at Oak Ridge National Laboratory (ORNL) in the US from 1962 to 1965 to support the Medium-Power Reactor Experiments (MPRE) program, which was a stainless-steel system with 1 MWth boiling potassium, or 140 kWe. Initial SCCA experiments were graphite reflected. The third benchmark in this series was Be-reflected; two critical configurations, nine cadmium ratio measurements, and various fuel and material reactivity measurements were evaluated. Benchmark identifiers: SCCA-SPACE-EXP-003 (IRPhEP)/HEU-COMP-FAST-004 (ICSBEP).
The initial isothermal physics tests of the Fast Flux Test Facility (FFTF) have been evaluated: criticality, spectral, reactivity worth, reactivity coefficient, and additional miscellaneous measurements. The FFTF was a 400 MWth SFR located at the Hanford Site near Richland, Washington. This reactor was operated from 1982 to 1992 as a prototype liquid metal fast breeder reactor and a fast test reactor for mixed oxide (MOX) and metal fuels. Benchmark identifier: FFTF-LMFR-RESR-001 (IRPhEP).
This ZPR assembly was a part of a series of critical experiments performed to support design of the FFTF. The simplistic geometry of this experiment consisted of primarily Pu-U-Mo alloy fuel, sodium, iron, and nickel components. Benchmark identifiers: ZPR-LMFR-EXP-004 (IRPhEP)/MIX-COMP-FAST-004 (ICSBEP).
The Advanced Test Reactor (ATR) is a 250 MWth high flux test reactor located at Idaho National Laboratory (INL) in the US. This reactor is currently in operation. The fresh core internals changeout (CIC) from 1994 (Cycle 103A-2) critical loading is evaluated as a benchmark to support irradiation testing and experiment design, including tests necessary for advanced reactor development. Benchmark identifiers: ATR-FUND-RESR-001 (IRPhEP)/HEU-MET-THERM-022 (ICSBEP).
The French RHF is 58.3 MWth, located in Grenoble, and was refurbished in 1993–1995. It consists of a single fuel element with curved plates, very similar to the High Flux Isotope Reactor (HFIR) at ORNL. Currently only the critical configuration is evaluated. Benchmark identifier: RHF-FUND-RESR-001 (IRPhEP).
The Impulse Graphite Reactor (IGR) is located at the Atomic Energy Institute of the National Nuclear Center of Kazakhstan Republic. It is a large graphite stack with very low 235U content to support high-temperature transient testing (the core loading is provided in Figure 7). Benchmark identifiers: IGR-FUND-RESR-001 (IRPhEP)/HEU-COMP-THERM-016 (ICSBEP).
The TREAT facility at INL also serves to provide transient testing capabilities for a variety of fuels, materials, and instrumentation needs. The historic critical core loading from 1994 is evaluated and is most representative of the core loading currently in operation. Many modern experiments are proposed to enable advanced reactor development. Figure 8 shows a model of a more modern experiment test. Benchmark identifier: TREAT-FUND-RESR-002 (IRPhEP).
FIGURE 8. Serpent model of TREAT for SIRIUS calibration experiment: Vertical view of core (Top) and horizontal view of test fuel (Bottom).
There are ∼50 ICSBEP evaluations containing fissile material with 235U enrichments between 5% and 10%.
Five core loadings are evaluated for the Kyoto University Critical Assembly (KUCA) in Japan. The goal of these experiments was to assess the basic neutronic properties of erbia-loaded, low-enriched thermal spectrum cores. Three of the cores had an average U enrichment of 5.4 wt% 235U, and the other two cores 9.6 wt%. Erbia content was increased from 0 wt% up to 1.12 wt%. These experiments support development of higher burnup fuel, which is a necessity with the increase in fuel enrichment above ∼5 wt%. Benchmark identifiers: KUCA-FUND-RESR-001 (IRPhEP)/LEU-MET-THERM-005 (ICSBEP).
The Seven Percent Critical Experiment (7uPCX) is located at Sandia National Laboratories (SNL) in Albuquerque, New Mexico. It was designed to investigate critical systems with fuel for light water reactors in the enrichment range above 5 wt% 235U. The current fuel is 6.90 wt% enriched. Twenty-four different critical core arrangements are evaluated implementing fuel rods, some with empty grid positions, titanium, and/or aluminum interstitial rods. Benchmark identifier: LEU-COMP-THERM-097 (ICSBEP).
There are currently several benchmarks using the 7uPCX facility. This benchmark evaluates 22 critical configurations of varying designs: rectangular, cylindrical, and split rectangular arrays. Benchmark identifier: LEU-COMP-THERM-101 (ICSBEP).
There are ∼20 ICSBEP evaluations containing fissile material with 235U enrichments between 10% and 20%.
The IPEN/MB-01 research reactor center is located in São Paulo, Brazil. Of the numerous contributed benchmarks from this reactor facility, this series of experiments performed in 2016 investigates the reactivity worth of HALEU U-Mo plates placed within the core center (see Figure 9). Benchmark identifiers: IPEN (MB01)-LWR-RESR-020 (IRPhEP)/LEU-COMP-THERM-103 (ICSBEP).
FIGURE 9. Schematic of IPEN MB-01 reactor core loading (Left) and X-Ray of a UMo fuel plate (Right).
The neutron radiography (NRAD) reactor is a 250 kWt TRIGA® (Training, Research, Isotopes, General Atomics) Mark II tank-type research reactor located at INL. This reactor was converted to HALEU UErZrH fuel in 2010 and upgraded to include more fuel in 2013. Two critical loadings and rod worth measurements are evaluated in this benchmark. Benchmark identifier: NRAD-FUND-RESR-002 (IRPhEP).
The Power Burst Facility (PBF) operated at INL from 1972 to 1985. It was designed to provide experimental data to define failure thresholds under postulated LWR accident conditions. This transient test facility used HALEU “inert-matrix” ternary oxide fuel. Two similar critical loadings from the startup tests were evaluated. Benchmark identifiers: PBF-FUND-RESR-001 (IRPhEP)/IEU-COMP-THERM-009 (ICSBEP).
The ERASME/S undermoderated MOX experiment was performed in 1985 as part of a 3-year program dedicated to high conversion PWRs. The experiment was performed in the EOLE facility at Cadarache in France. A single critical configuration was evaluated. Benchmark identifiers: EOLE-PWR-EXP-001 (IRPhEP)/MIX-COMP-INTER-005 (ICSBEP).
The Vulcain Experimental Nuclear Study (VENUS) zero power reactor has been used for a variety of experiments at the SCK-CEN complex in Mol, Belgium. The Plutonium Recycle Programme (PRP) was between 1967 and 1975. Configurations 9 and 9/1, the core loading of the latter provided in Figure 10, were performed to study boundary effects between zones with different plutonium content and the effective influence of perturbations at the boundary. Power distribution measurements were evaluated as benchmark experiment data. Benchmark identifier: VENUS-PWR-EXP-005 (IRPhEP).
FIGURE 10. Horizontal Historical Schematic of Configuration 9/1 Core Loading from VENUS-PRP (Region I represents MOX fuel pins; Regions II and III represent UO2 fuel pins).
Core 12 of the GCFR-PROTEUS experimental program contained a homogeneous mixed arrangement of (U-Pu)O2 and ThO2 fuel pins in a similar core design as the previously described Core 11. Six spectral indices were evaluated for this configuration to enable evaluation of the 232Th/233U fuel cycle. Benchmark identifier: PROTEUS-GCFR-EXP-002 (IRPhEP).
The benchmark summarizes a 1960 study by Babcock and Wilcox (B&W) for a Spectral Shift Control Reactor (SSCR) concept using rod lattices moderated by D2O-H2O mixtures. This evaluation includes a critical eigenvalue and thermal disadvantage factor. Benchmark identifier: SSCR-PWR-EXP-002 (IRPhEP).
Critical experiments were conducted at the High Temperature Test Facility of the Bettis Atomic Power Laboratory (BAPL) in West Mifflin, Pennsylvania, US to support a Light Water Breeder Reactor (LWBR) program. Benchmark identifier: U233-COMP-THERM-001 (ICSBEP).
The KAMINI reactor (see Figure 11) is a zero-power reactor with 233U fuel, light water moderation, and BeO reflector. It is located at the Indira Gandhi Centre for Atomic Research in Kalpakkam, India. A single critical configuration from 1996 has been evaluated as a benchmark. Benchmark identifier: U233-MET-THERM-001 (ICSBEP).
Another series of experiments with BFS-1 include MOX fast-neutron critical assemblies simulating damp MOX powders. The spectral characteristics were measured via fission chambers to obtain fission ratios for 238U, 237Np, 239Pu, 240Pu, 241Am, 243Am, 244Cm, and 245Cm relative to 235U and/or 239Pu, and the capture-to-fission ratio of 238U–235U. Benchmark identifiers: BFS1-FUND-EXP-001 (IRPhEP)/MIX-MISC-MIXED-001 (ICSBEP).
Another BFS-1 critical experiment of a sodium-cooled fast reactor included spectral and reactivity worth measurements for isotopes of Np, Pu, and Am. Benchmark Identifier: BFS1-LMFR-EXP-001 (IRPhEP).
A high-purity sample worth for 244Cm was evaluated for a replacement measurement performed with the Jezebel plutonium-240 sphere (PU-MET-FAST-002 in ICSBEP). Benchmark identifier: SPEC-MET-FAST-001 (ICSBEP).
A high-purity sample worth for 237Np was evaluated for a replacement measurement performed with the Flattop uranium sphere (MIX-MET-FAST-002 in ICSBEP). Benchmark identifier: SPEC-MET-FAST-003 (ICSBEP).
A neptunium sphere was encased within matching pairs of HEU shells to decrease the uncertainty in the critical mass of 237Np for criticality safety and non-proliferation issues (see Figure 12). Benchmark identifier: SPEC-MET-FAST-008 (ICSBEP).
The Benchmark for Evaluation And Validation of Reactor Simulations (BEAVRS) is a multi-cycle full-core PWR depletion benchmark based upon measurement data from two operational cycles of a commercial nuclear plant. This benchmark is excellent for testing computational capabilities. However, it remains as a draft evaluation on the IRPhEP Handbook as much of the original data is proprietary, significantly limiting bias and uncertainty analyses requisite of a high-quality benchmark experiment. Benchmark identifier: BEAVRS-PWR-POWER-001 (IRPhEP).
Core-wide fission rate distribution measurements from 44 cycles of PWR operation at Duke Power Company’s McGuire and Catawba nuclear power plants were utilized to infer fuel assembly reactivity due to nuclide burnup. The results are then used to create benchmarks for core-averaged fuel assembly depletion up to 60 GW d/t. Benchmark identifier: DUKE-PWR-POWER-001 (IRPhEP).
The reactivity worth of major fission products was measured in the MINERVE reactor in Cadarache, France as part of the CERES program between France and the United Kingdom to validate LWR fuel reactivity loss with burnup. The CERES Phase II measurements performed in MINERVE are evaluated in this benchmark for various isotopes including Sm, Nd, Gd, Eu, Rh, Cs, Mo, and Tc. Benchmark identifier: MINERVE-FUND-RESR-001 (IRPhEP).
The Thermal/Epithermal eXperiments (TEX) program was designed to provide a platform of similar experiments for testing materials and fuels across the neutron spectra. Historically experiments have focused upon neutron behavior in thermal or fast regimes. The TEX program includes experiments for testing fuels and materials across the intermediate neutron energy spectra. The TEX-HEU experiments evaluated in this benchmark provide five baseline cases. Benchmark identifier: HEU-MET-MIXED-021 (ICSBEP).
This benchmark contains the TEX baseline cases for plutonium experiments across the neutron spectra. Benchmark identifier: PU-MET-MIXED-002 (ICSBEP).
It is impossible to summarize the plethora of benchmarks available to support testing and validation of the nuclear data utilized in nuclear codes, methods, design, and applications worldwide. Only a snippet of examples is provided below, and many more examples can be found within the handbooks using the tools presented in Section 3.
A series of critical experiments were performed at ORNL in 1965–1966 to support the design of SORA, which was to be a U-Mo burst facility. Fifteen mockup critical configurations were evaluated for U-metal rod arrays reflected by high-purity (99.5 wt%) Fe and Be. Benchmark identifier: HEU-MET-FAST-096 (ICSBEP).
Thirty-five critical loadings were evaluated for the IPEN/MB-01 reactor. The core was reflected on the west side by increasing cumulative thicknesses of carbon steel or nickel laminates. This experiment tests neutron absorption and scattering in the reflecting material as the spectra hardens from thermal neutron absorption to fast neutron reflection. Benchmark identifiers: IPEN (MB01)-LWR-RESR-015 (IRPhEP)/LEU-COMP-THERM-088 (ICSBEP).
The Matériaux Interaction Réflexion Toutes Epaisseurs (MIRTE) program was carried out from 2008 to 2013 at the Commissariat à l’Energie Atomique (CEA) Valduc center in France. The purpose of this program was to measure integral reactivity characteristics of various structural materials, as demonstrated in Figure 13, to support validation of computer codes and nuclear data. A total of 28 benchmark configurations were evaluated from this experimental series. The experiments consisted of LEU rod arrays reflected or separated by screens of the test materials. Benchmark identifier: LEU-COMP-THERM-074 (ICSBEP).
FIGURE 13. Configurations of screens in MIRTE experiments: Reflected (Top), interacting with Thick screen (middle), and interacting with Thin cruciform screens (Bottom).
The ICSBEP and IRPhEP actively provide international preservation, evaluation, and dissemination of integral benchmark data to support computational validation of models, simulations, and nuclear data in support of criticality safety and reactor physics applications. Annual contributions to their respective handbooks provide an ever-growing resource of evaluated benchmark experiment data that has been assessed qualitatively and quantitatively to provide uncertainties, biases, and established benchmark models within a standardized handbook format. All benchmark evaluations undergo an intensive peer-review process with participants and contributions representing over 20 countries. Experiments were performed historically to support reactor operations, measurements, design, and nuclear safety. The extensive investments in infrastructure, expertise, and cost are not cheaply reproduced should the information from these legacy experiments become lost. The preservation and evaluation activities of the ICSBEP and IRPhEP provide a means to provide quality nuclear data for current and future needs in computational tools and nuclear data testing.
The needs of the nuclear modeling and simulation community continues to expand and evolve. The ICSBEP and IRPhEP provide well-established frameworks to continue to provide high-quality, peer-reviewed benchmark data to serve as the intrinsic backbone of contemporary neutronics methodologies, simulation, and nuclear data. The true value of their handbooks is priceless considering the impact they have in supporting, sustaining, and enabling modern nuclear safety, design, modeling, and simulation.
Future endeavors in advancement of nuclear reactor designs builds upon contemporary and historic efforts to understand and characterize our nuclear world via experimentation. The ICSBEP and IRPhEP serve as an international omnibus of curated and evaluated experimental data from around the world. The benchmarks contained within their handbooks serve as the backbone for validation testing of contemporary neutronics methodologies, simulation, and nuclear data. This manuscript serves as only a snapshot of the current culmination of international collaborations to utilize our nuclear heritage to enable our nuclear future. It does not include numerous evaluations that are currently being considered or that are in the initial phases of the evaluation process.
The data analyzed in this study is subject to the following licenses/restrictions: Data available to OECD NEA member countries and participating organizations. Requests to access these datasets should be directed to Julie-Fiona Martin (Julie-Fiona.MARTIN@oecd-nea.org) and Ian Hill (Ian.HILL@oecd-nea.org).
All authors listed have made a substantial, direct, and intellectual contribution to the work and approved it for publication.
Contributions, financial and otherwise, towards the success of the OECD NEA international benchmark projects have come from a myriad of national and international sources.
The ICSBEP and IRPhEP are collaborative efforts that involve numerous scientists, engineers, and administrative support personnel from 28 different countries. The authors would like to acknowledge the efforts of all these dedicated individuals without whom these technical working groups would not be possible. A listing of all contributors can be found in the introductory material of both ICSBEP and IRPhEP Handbooks.
JB is employed by JFoster & Associates, LLC.
The remaining authors declare that the research was conducted in the absence of any commercial or financial relationships that could be construed as a potential conflict of interest.
All claims expressed in this article are solely those of the authors and do not necessarily represent those of their affiliated organizations, or those of the publisher, the editors and the reviewers. Any product that may be evaluated in this article, or claim that may be made by its manufacturer, is not guaranteed or endorsed by the publisher.
Bess, J. D., and Ivanova, T. (2020). Current overview of ICSBEP and IRPhEP benchmark evaluation practices. EPJ Web Conf. 239, 18002. doi:10.1051/epjconf/202023918002
Briggs, J. B., and Gulliford, J. (2014). An overview of the international reactor physics experiment evaluation project. Nucl. Sci. Eng. 178 (3), 269–279. doi:10.13182/NSE14-27
Briggs, J. B., Scott, L., and Nouri, A. (2003). The international criticality safety benchmark evaluation project. Nucl. Sci. Eng. 145 (1), 1–10. doi:10.13182/NSE03-14
Dean, V. F., and Blackwood, L. G. (2008). ICSBEP guide to the expression of uncertainties. Paris, France: OECD Nuclear Energy Agency.
Dos Santos, A., Briggs, J. B., Tsiboulia, A. M., Hill, I., Szatmary, Z., Snoj, L., et al. (2018). “Overview of the international reactor physics experiments evaluation project (IRPhEP) guide to the expression of uncertainty,” in Proceedings of International Conference on Physics of Reactors (PHYSOR 2018), Cancun, Mexico, April 22-26 2018.
Hill, I., Soppera, N., and Bossant, M. (2014). Idat: The international handbook of evaluated reactor physics benchmark experiments database and Analysis tool. Nucl. Sci. Eng. 178 (3), 280–294. doi:10.13182/NSE14-37
Kodeli, I. A., and Sartori, E. (2021). Sinbad – radiation shielding benchmark experiments. Ann. Nucl. Energy 159, 108254. doi:10.1016/j.anucene.2021.108254
Menut, P., Sartori, E., and Turnbull, J. A. (2000). “The public domain database on nuclear fuel performance experiments (IFPE) for the purpose of code development and validation,” in Proceedings of the 2000 International Topical Meeting on Light Water Reactor Fuel Performance, Park City, Utah, April 10-13 2000.
Michel-Sendis, F., Gauld, I., Martinez, J., Alejano, C., Bossant, M., Boulanger, D., et al. (2017). SFCOMPO-2.0: An OECD NEA database of spent nuclear fuel isotopic assays, reactor design specifications, and operating data. Ann. Nucl. Energy 110, 779–788. doi:10.1016/j.anucene.2017.07.022
NEA (2022a). International handbook of evaluated criticality safety benchmark experiments. Paris, France: OECD Nuclear Energy Agency.
NEA (2022b). International handbook of evaluated reactor physics benchmark experiments. Paris, France: OECD Nuclear Energy Agency.
NEA (2015). Review of integral experiments for minor actinide management. NEA No. 7222. Paris, France: OECD Nuclear Energy Agency.
Nouri, A., Nagel, P., Briggs, J. B., and Ivanova, T. (2003). Dice: Database for the international criticality safety benchmark evaluation program handbook. Nucl. Sci. Eng. 145 (1), 11–19. doi:10.13182/NSE03-15
Palmiotti, G., Briggs, J. B., Kugo, T., Trumble, E. F., Kahler, A. C. S., and Lancaster, D. (2014). Applications of integral benchmark data. Nucl. Sci. Eng. 178 (3), 295–310. doi:10.13182/nse14-33
Rohatgi, U., Dyrda, J., and Soppera, N. (2018). “The international experimental thermal hydraulic systems database (TIETHYS): A new NEA validation tool,” in Proceedings of the 26th International Conference on Nuclear Engineering, London, England, July 22-26 2018. doi:10.1115/ICONE26-82631
Rozhikhin, Y. (1999). “Detailed spectra data for the international handbook of evaluated criticality safety benchmark experiments,” in Proceedings of International Conference on Nuclear Criticality Safety (ICNC 1999), Versailles, France, Sept. 20-24 1999, 1167–1172. Volume III.
Valentine, T., Avramova, M., Fleming, M., Hursin, M., Ivanov, K., Petruzzi, A., et al. (2018). “OECD-NEA Expert group on multi-physics experimental data, benchmarks and validation,” in Proceedings of the 26th International Conference on Nuclear Engineering, London, England, July 22-26 2018. doi:10.1115/ICONE26-81571
Keywords: advanced reactors, benchmark, criticality safety, experiment, handbook, nuclear data, reactor physics, validation
Citation: Bess JD, Ivanova T, Hill I, Martin J-F, Briggs JB, Scott L, DeHart MD, Percher C, Marshall BJ and Blaise P (2023) Intrinsic value of the international benchmark projects, ICSBEP and IRPhEP, for advanced reactor development. Front. Energy Res. 11:1085788. doi: 10.3389/fenrg.2023.1085788
Received: 31 October 2022; Accepted: 09 February 2023;
Published: 28 February 2023.
Edited by:
Hitesh Bindra, Kansas State University, United StatesReviewed by:
Liangzhi Cao, Xi’an Jiaotong University, ChinaCopyright © 2023 Bess, Ivanova, Hill, Martin, Briggs, Scott, DeHart, Percher, Marshall and Blaise. This is an open-access article distributed under the terms of the Creative Commons Attribution License (CC BY). The use, distribution or reproduction in other forums is permitted, provided the original author(s) and the copyright owner(s) are credited and that the original publication in this journal is cited, in accordance with accepted academic practice. No use, distribution or reproduction is permitted which does not comply with these terms.
*Correspondence: John D. Bess, am9obi5iZXNzQGpmYWlkYWhvLmNvbQ==
Disclaimer: All claims expressed in this article are solely those of the authors and do not necessarily represent those of their affiliated organizations, or those of the publisher, the editors and the reviewers. Any product that may be evaluated in this article or claim that may be made by its manufacturer is not guaranteed or endorsed by the publisher.
Research integrity at Frontiers
Learn more about the work of our research integrity team to safeguard the quality of each article we publish.