- 1College of Engineering, China Agricultural University, Beijing, China
- 2School of Mechanical Engineering and Automation, Beihang University, Beijing, China
- 3School of Ecology and Environment, Zhengzhou University, Zhengzhou, China
Silicone rubber is a widely used functional material whose lifetime and safety properties can be improved by achieving superhydrophobicity on the surface. However, there are few studies on the use of superhydrophobic surfaces as nano-energy devices. In this work, laser etching was used to achieve continuous regulation of the superhydrophobic to superhydrophilic state of the surface on silicone rubber. The modulation of wettability is attributed to laser manufactured micro-nanostructures, while the laser can be used to recover the damaged structures. Meanwhile, a triboelectric nanogenerator (TENG) is fabricated using superhydrophobic silicone rubber and drives the LED to light up. In short, this study provides a simple solution for TENG and its recycling.
Introduction
Silicone rubber has the advantages of good insulation, wear resistance, high elasticity, low toxicity, and weather resistance (Shit and Shah, 2013; Emelyanenko et al., 2017; Chen et al., 2019). As a versatile functional material, it is usually applied in biomedicine, aerospace, moulds, power transmission, etc., (Hu et al., 2007; Song et al., 2015; Wu et al., 2017; Ma et al., 2022). When silicone rubber is used in insulators and roof waterproof coating for high-voltage transmission systems, it is easily affected by environmental factors such as high temperature, rainwater acid corrosion, snowfall and freezing, sand and dust, and ultraviolet irradiation during long-term service (Momen and Farzaneh, 2011; Momen et al., 2011; Liu et al., 2015). These attacks will affect its mechanical and electrical properties, including the hardening and brittleness of the silicone rubber surface, and local aging, which lead to cracking and safety accidents such as leakage of electricity and water (Kumosa et al., 2002; Velásquez and Lara, 2018). Superhydrophobic surfaces are surfaces where the contact angle (CA) of water droplets on the object is greater than 150° and the roll angle (RA) is less than 10° and has great anti-dirt, anti-ice, and self-cleaning properties (Ma et al., 2021). Due to the poor hydrophobic property of silicone rubber itself, it can obtain excellent hydrophobicity by preparing a superhydrophobic layer on the surface of silicone rubber without being invaded by rain, ice, wind and sand, waste liquid, etc. It can significantly improve the property of safety and service life of silicone products. Triboelectric nanogenerator (TENG) are considered to be an effective methods of harvesting energy from the environment. A class of TENG that can collect raindrop energy is widely used in outdoor scenarios, such as agricultural greenhouses and plant sensors (Zhang et al., 2021; Lan et al., 2021). Superhydrophobic surfaces have been shown to improve the output performance of TENG and to improve the anti-fouling properties of TENG surfaces to enhance their durability (Jiang et al., 2021).
Nowadays, two routes are used to achieve superhydrophobic surfaces: one is to build micro-nanoscale rough structures on the surface of hydrophobic materials with low surface energy, and the other is to modify the micro-nanoscale rough structures with low surface energy substances (Davis et al., 2014; Cao et al., 2017). In terms of the preparation process, the main methods include electrodeposition (Wang et al., 2016), polymer imprinting (Wen et al., 2018), electrospinning (Hou et al., 2018), self-assembly (Jiang et al., 2017), spray-coating (Li et al., 2015), plasma treatment (Yung et al., 2018), laser etching (Li et al., 2022), etc. Among these methods, laser etching has been widely used for surface microstructure processing due to its high efficiency, non-pollution, easy patterning, and diverse processing materials (Long et al., 2015; Ta et al., 2015; Samanta et al., 2019). Jalil et al. (2020) constructed different micro-nano structures on the gold (Au) surface by femtosecond laser and investigated the effect of different micro-nano structures on the hydrophobic properties of the surface. Using ultrafast lasers to fabricate special micro-nano structure superhydrophobic surfaces, Wang et al. found that liquid droplets can achieve a spontaneous transition from the Wenzel state to the Cassie state during freezing and melting cycles (Wang et al., 2022). It can be seen that the application of laser for surface micro-nanostructure processing is an effective technology for preparing superhydrophobic surfaces. However, the practical application of superhydrophobic surfaces is still problematic because of their poor durability and susceptibility to surface microstructure failure due to frictional wear during use. To improve their durability, efforts have been devoted to rationally enhancing the hierarchical structure of superhydrophobic surfaces, and these attempts include covalently cross-linked layered textures and wrapping superhydrophobic textures with polymers or rigid shells (Liu et al., 2017; Li C. et al., 2020). However, it is difficult for those methods to recover the superhydrophobic properties after the surface is subjected to severe wear such as scratches and scuffs.
In this paper, we investigated the preparation of superhydrophobic microstructures on silicone rubber surfaces using the CO2 lasers processing and discovered the optimal power for laser etching to achieve superhydrophobic surfaces. Also, it has been founded that the surface wettability of silicone rubber could be modulated by changing the laser etching parameters, which can achieve changes in superhydrophobic and wettable states, and these surface wettability properties can be quickly repaired in situ by laser after mechanical or chemical damage. The low-power laser used for restoration enables handheld laser processing to effectively restore the macroscopic configuration, hierarchical texture, mechanical properties, and wettability of the surface within minutes. In addition, due to the anti-fouling properties and strong repairability of the superhydrophobic silicon rubber surface, this study utilizes this superhydrophobic silicon rubber film as a Triboelectric nanogenerator (TENG), which significantly enhances the flexibility and self-cleaning ability of the device.
Materials and methods
Preparation of silicone rubber surface and recovery
The formation of a superhydrophobic surface is a very simple process (Figure 1A). First, commercially available standard silicone rubber can be used to prepare silicone films without any special treatment. To prepare the silicone rubber film, the silicone rubber (PartA/PartB = 1:1, w/w, Hong Ye Jie Technology Co.,.Ltd.) components were mixed. Spin coater (KW-4A) was used with a spin speed of 500 r/min. Then, the sample was heated on the heating stage (LC-DMS-H) at 65°C for 1 h. After that, the CO2 laser cutting tool (maximum power is 50 W, Huibang Laser5000) was applied to the entire substrate surface. The laser was automatically scanned at a pitch of 50 μm to produce a surface structure with micro-wall-like structures (width ≈25 μm). The height can be controlled by adjusting the laser power while scanning at a constant speed (≈100 mm/s). During the laser etching process, the laser power, the laser focal length and the angle of the two etchings were changed.
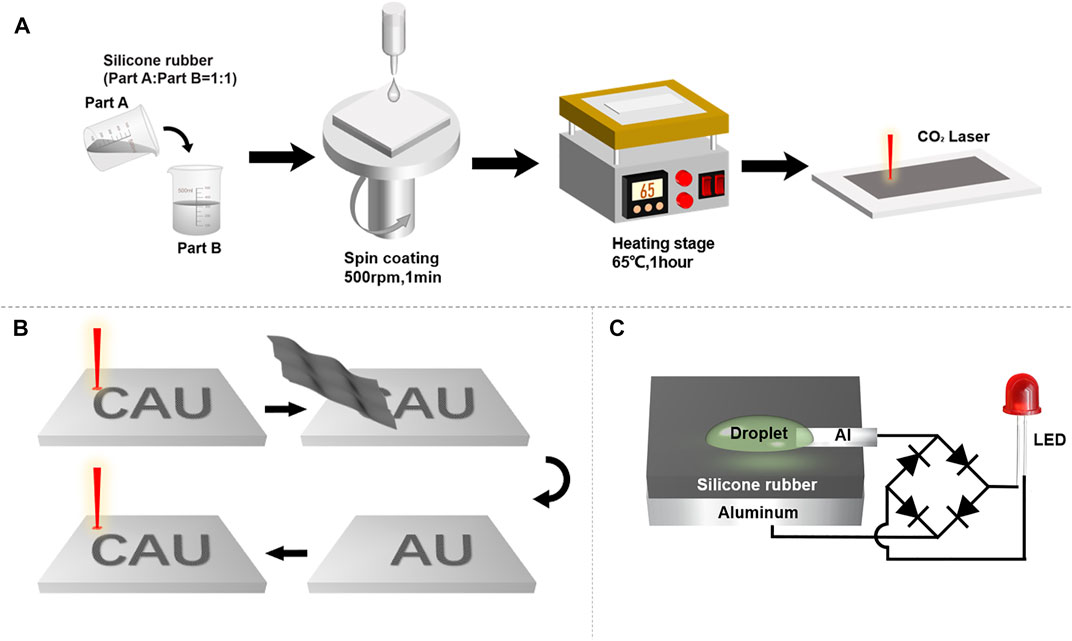
FIGURE 1. (A) Schematic illustration of the whole experiment methods. (B) Laser recovery of worn-out superhydrophobic letter “C.” (C) The structure of the TENG device.
Recovery process: First, the superhydrophobic surface (letter “CAU”) of the silicone rubber was prepared by laser at a certain power (2.0 W) and a certain focal length (9 mm), then 400# sandpaper was used to erase the letter “C". Finally, the letter “C" was erased by 400# sandpaper and repaired by laser in the same position with the same parameters (Figure 1B).
Characterizations of surface wettability and micro-nanostructure
Characterization of the wettability of laser-processed silicone rubber surfaces was performed by a video contact angle meter (Dataphysics OCA25) with a droplet volume of 9 μl used to measure contact and roll angles. A scanning electron microscope (SEM) system (ZEISS GeminiSEM 500) was used to observe the morphology of the laser-etched surfaces.
Triboelectric nanogenerator development and characterization
The surface of the silicone rubber film was irradiated with a laser to fabricate the superhydrophobic microstructure. Next, this surface was attached to a thin aluminum plate. The aluminum tape was used as an electrode for the TENG, which was placed under a device designed to simulate rain drops for measurement (Figure 1C). The open-circuit voltage of the TENG was measured by an electrostatic meter (6514, Keithley, United States).
Results and discussion
Silicone rubber surface wettability modulation
In this work, the parameters of the laser (power, defocus level, rotation angle) are considered as the crucial parameters to modify the micro-nano structures and the wettability of the silicone rubber surface. The etching depth and surface morphology of the silicone rubber were firstly investigated. For each laser power, the CA of the surface was measured. As shown in Figures 2A,B, fixing other conditions (i.e., scan rate = 100 mm/s, focal length 9 mm, pulse resolution = 500 pulses per inch), the laser power of 1.0 W could not form a clear structure, but the micro-nano surface roughness of the silicone rubber was still present, at which time CA = 129°, showing certain hydrophobicity. With the increase of laser power, the CA begined to increase from the original value of 102°. When the laser power reached 2.0 W, CA increased to 158° and SA decreased to 1.5°. After the laser power reached 2.5 W, the shape of the water droplet was found to be continuously tuned from the spherical state (CA = 158°) to the ellipsoidal or partially ellipsoidal state (10° < CA < 150°) until the completely flat state (CA < 10°) with increasing power, i.e., from superhydrophobic to superhydrophilic (Supplementary Figure S1). Simiarly, the focal length was changed at fixed power = 2.0 W, scan rate = 100 mm/s, and pulse resolution = 500 pulses/inch, respectively. As shown in Figures 2C,D, increasing the defocus level from the original focus [i.e., Defocus Level (DL) = 0.0 mm–DL = 4.0 mm] was allowed to gradually control the properties of the etched silicone rubber surface from a high surface roughness to a lower level. As the defocusing level increased, the CA gradually decreased from 158° until the superhydrophobic properties were lost, indicating that continuous modulation of the hydrophobicity of the silicone rubber surface droplets can be achieved by defocusing. Finally, a second etching was performed after one etching by rotating a different angle (0°–90°). Both times the power was 2 W. The CA and SA of the silicone rubber surface did not change significantly after etching. It indicates that the rotation angle is not a parameter used to change the immersion performance (Supplementary Figure S1).
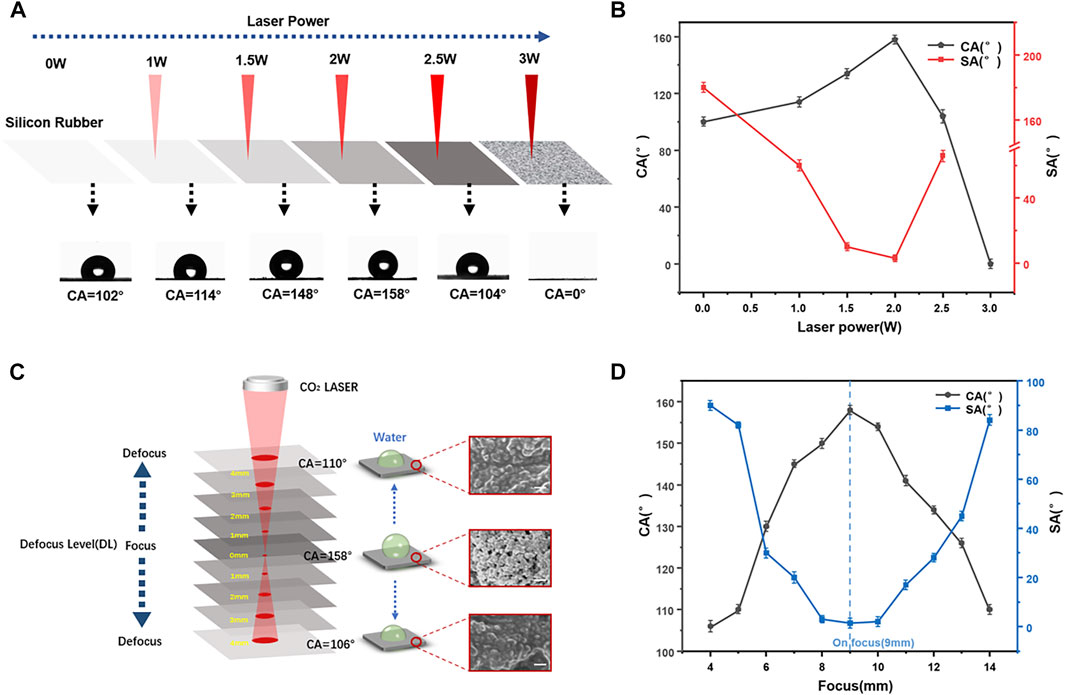
FIGURE 2. (A,B) Continuous regulation of silicone rubber surface wettability by controlling laser power. (C,D) Continuous regulation of silicone rubber surface wettability by adjusting the defocus level (DL). (Scale bar, 400 nm).
In the superhydrophilic state, the infiltration properties are not usually easy to be characterized. In this paper, the infiltration time was ragard as an index to evaluate the superhydrophobic properties. Under fixed conditions (i.e., scan rate = 100 mm/s, focal length = 9 mm, pulse resolution = 500 pulses per inch), the laser-etched silicone rubber surface would exhibit continuously superhydrophilic properties when the laser power exceeded 2.5 W. Droplets of the same volume (9 μl) was dropped at the same height (h = 3 mm) and the infiltration process was recorded with a video recording system. The faster the infiltration rate, the better the superhydrophilic properties. It is clearly observed that the increase in power leads directly the increase in superhydrophilicity (Figure 3). This proves that the superhydrophilic property of silicone rubber surface can be achieved by continuously adjusting the etching power of laser.
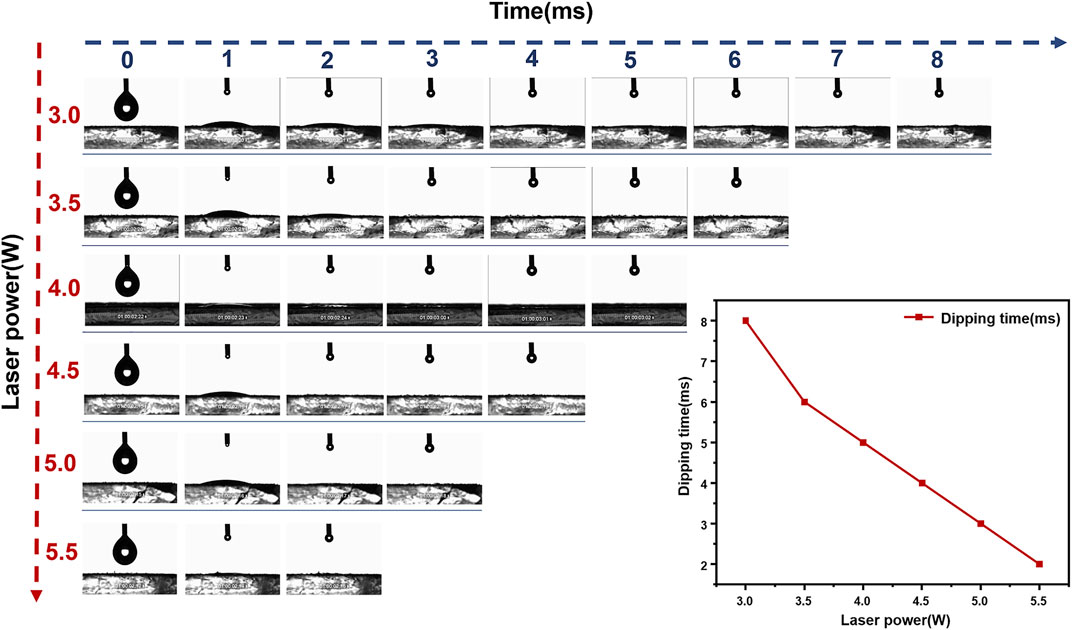
FIGURE 3. Preparation of silicon rubber surface by varying laser power and its continuously adjustable wettability to water.
Analysis of superhydrophobic to superhydrophilic continuous transition process
Based on the Cassie-Baxter model, a rough surface always enhances the hydrophobicity of a solid surface, regardless of its hydrophobicity or hydrophilicity. In addition, the hydrophobicity of a solid surface increases as the solid-liquid contact area decreases. When the surface roughness of hydrophobic substrates increases, the water contact behavior may shift from the Wenzel model to the Cassie-Baxter model (Yoshimitsu et al., 2002). Thus, this shift in the model is usually accompanied by an increase in CA, and the substrate seems to change from hydrophobic to superhydrophobic. In addition, the SA is subsequently reduced from high to low adhesion.
The surface morphology of each specimen was observed by SEM. As the laser energy was accumulated to 2W and focused, a new surface micro-nano structures was formed on the sample surface, which consisted of numerous papillary structures about 150 nm in size (Figure 4A). The contact behavior between the water droplets and the sample surface changed from the Wenzel state to the Cassie state, that is, the sample, and the wettability of the surface was transformed into a superhydrophobic state. The superhydrophobic surface of the sample had a layered nano/micro structure similar to pine cones, which consist of many irregular petal-like structures with sizes ranging from tens to hundreds of nanometers. Many grooves can be generated from these pinecone-like layered structures, resulting in higher contact angles (Figure 4D). It can be considered that the laser power of 2 W is the critical energy required to etch the superhydrophobic silicone rubber. When the laser energy increased to 2.5 W, some micro-wrinkles started to appear on the surface, and when the arching papillae on the surface were ablated by the laser energy again, the size was reduced to about 100 nm, the microporous structure appeared on the surface, and the superhydrophobicity disappeared (Figures 4B,E). This state means the transition from superhydrophobic to superhydrophilic. As shown in Figures 4C,F, continuing to increase the laser power, when the laser power is 3 W, micro lamellar structures appeard on the surface, just as the three-dimensional pore micro structure observed in the SEM images. It is precisely because of this porous micro structure of surface that it achieves superhydrophilic properties. The surface can be achieved to continuously modulate from superhydrophobic to superhydrophilic by varying the laser power because of the ablation of specific laser power. After a short period of high temperature treatment of silicone rubber, a complex micro/nano three-dimensional pore structure appears on the surface. The pores enlarge the spacing of nano-papillae and put them in Cassie state, and the pores originally formed by the papillae no longer exist. Further, the pores will absorb liquid droplets and exhibit a macroscopic superhydrophilic state.
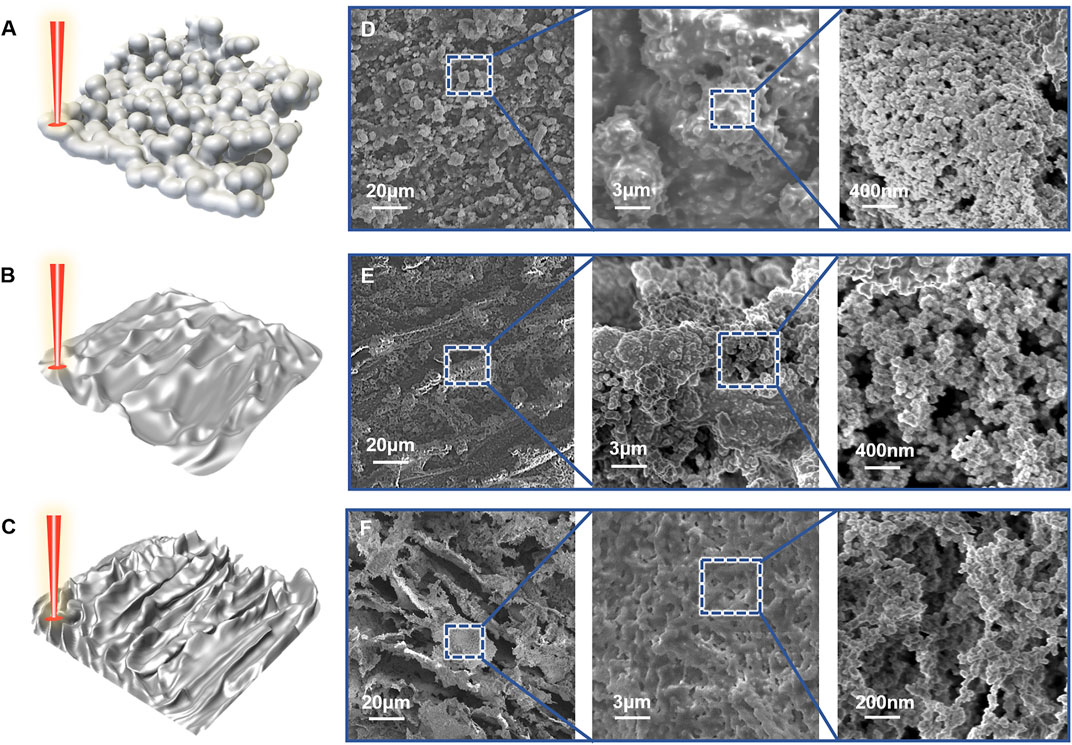
FIGURE 4. (A) Schematic view of superhydrophobic surface structure. (B) Schematic view of the surface structure of the transition from superhydrophobic to superhydrophilic. (C) Schematic diagram of super hydrophilic structure surface structure. (D,E,F) Irregular micro-nanostructure by laser etching. SEM images with different magnifications E of intermediate state, F of superhydrophilic surface, Scale bars are marked.
Recoverability of surface superhydrophobicity
The surface undergoes a series of mechanical damage by using a laser such as abrasion, and rapid recovery of the surface impregnation properties can be achieved. The letter “CAU” (Figure 5A) was written directly with the laser under certain parameters, as shown in (Figure 5B), the part of the letter has good water repellency. Sandpaper (400#) was sanded to remove the letter “C.” After writing the letter “C” in situ by a laser with the same parameters (Figure 5C), the letter “C” was produced in situ and the drip test showed that it had regained good hydrophobicity and could be rebuild (Figure 5D). The loss of microstructure after abrasion was observed by SEM images (Figure 5AE), but after laser etching, the papillary structure was recovered in situ on the surface (Figure 5F). This demonstrates the ability of the laser etching process to recover superhydrophobicity in situ. Laser-etched surfaces with mass wettability modulation properties are allowed to design and process the flexible surfaces with pattern. As mentioned above, silicone rubber treated with different laser etching parameters is capable to form any shape hydrophobic and hydrophilic arrays or channels on the superhydrophobic background, such as dot arrays, square arrays, star arrays, etc (Wang et al., 2021). As shown in Figure 6, a 14 × 14 square array was prepared by adjusting the power in one step, and the difference in hydrophobic properties was exploited to align the water droplets as the letter “CAU.”
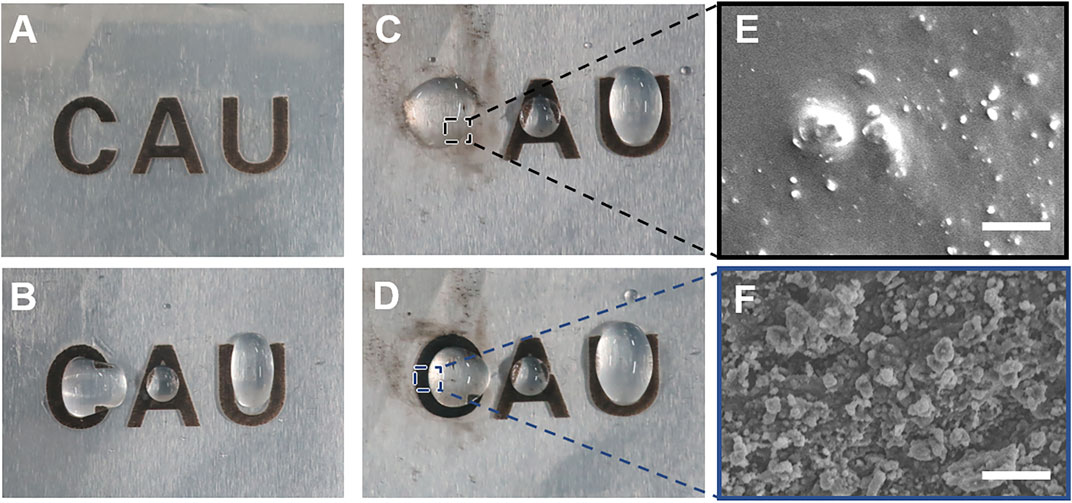
FIGURE 5. Silicone rubber superhydrophobic surface recover test. (A) Superhydrophobic CAU letters. (B) Water repellency of superhydrophobic letters. (C) Scratch off the surface letter C. (D) Laser etching method for in-situ recovery of letter C. (E) Silicone rubber SEM diagram after the destruction of surface structure. (F) SEM image of silicone rubber surface after in-situ recovery. (Scale bar, 20 μm).
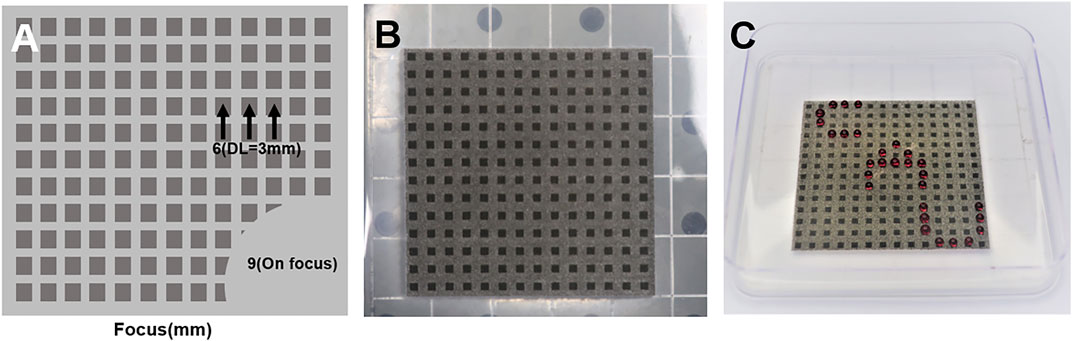
FIGURE 6. Laser etching of 14*14 square arrays for droplet modulation. (A) Superhydrophobic grid manufacture. (B) Superhydrophobic grid diagram on silicone. (C) Droplets on a superhydrophobic grid forming the letters “CAU.”
Silicone rubber/Al triboelectric nanogenerator
Silicone rubber with a superhydrophobic surface is used to fabricate single-electrode Triboelectric nanogenerator (TENG) that operate in a water-drop driven mode. As shown in Figure 7, the water droplet already carries a certain positive charge generated by the electrical effect of friction with air before it comes into contact with the TENG surface. When the water droplet hits the silicone rubber surface, the positive charge it carries creates a positive potential difference between the surface aluminum electrode and the back electrode, and electrons flow from the back electrode to the surface to balance this potential difference. When the water droplet rolls off the silicone rubber surface, this state is broken and the electrons flow in the opposite direction, forming an AC loop (Figure 7A).
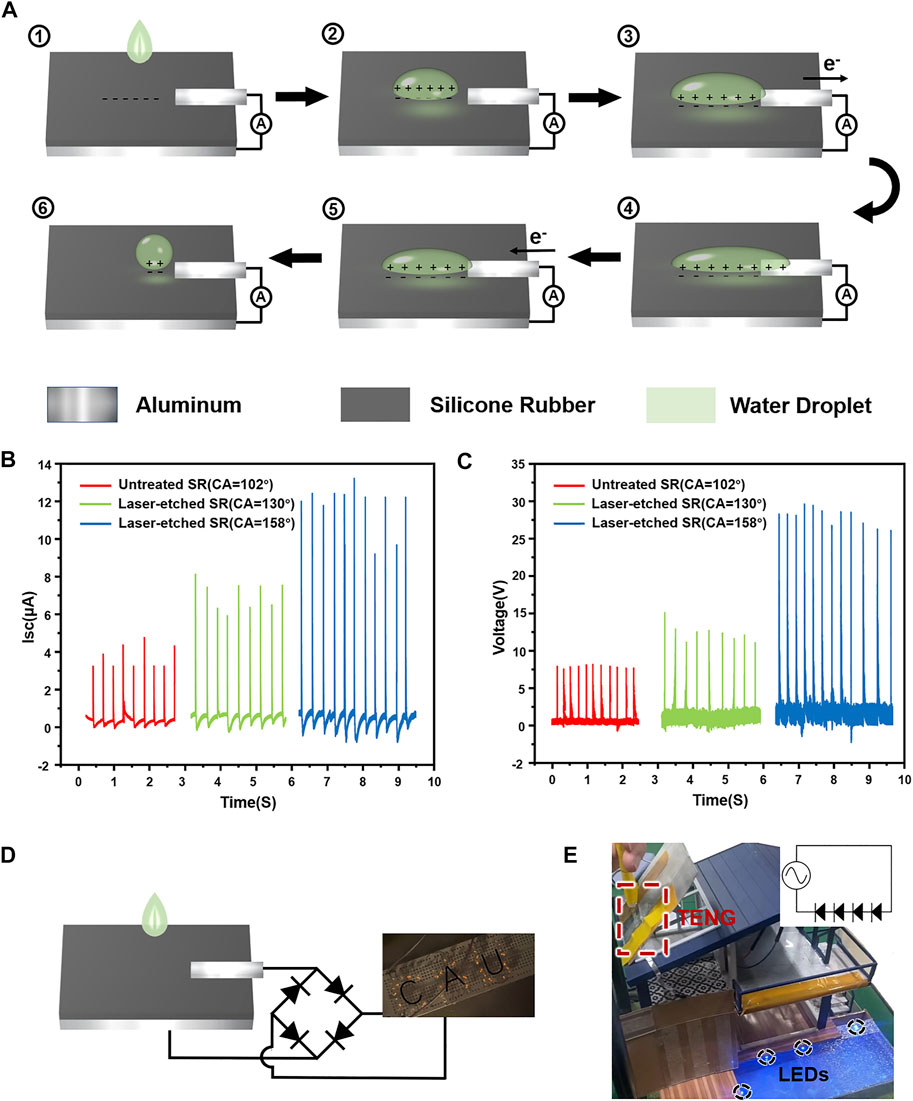
FIGURE 7. (A)Mechanism of the corresponding charge transfer. (B,C) Outputs of different droplets when they impinge on the friction layer with a 40° inclination of the TENG device. (D) Droplet lights up a “CAU” shaped LEDs through the TENG. (E) TENG lights up blue LEDs with rainwater power on the roof.
The use of outdoor scenarios requires long-term stable operation of TENG devices in contact with water. Superhydrophobic surfaces have excellent anti-fouling, anti-corrosion and self-cleaning performance properties. For this reason, superhydrophobic surfaces can enhance the stability of TENG in outdoor use. In addition, TENG with a superhydrophobic surface is more conducive to the rapid sliding of water droplets, which can transfer more induced charges. Reports have shown that maximum power output cannot be obtained if the droplets are not removed cleanly from the TENG surface (Li X. et al., 2020), which requires a good hydrophobicity of the TENG surface. This is because when a water drop falls on a poorly hydrophobic surface, it tends to bond with the previous drop, resulting in negative charge transfer. In our study, the contact angle of water droplets on the TENG surface was as high as 158° due to the presence of a superhydrophobic structure on the TENG surface, and the large contact angle resulted in a significant increase in the droplet departure velocity.
To demonstrate the feasibility of the TENG to harvest rain energy in a natural environment, we simulated rainfall with a flow rate of 10 ml/s and a height of about 20 cm with the device, and used a pump to automatically control the droplet velocity and utilized untreated tap water and artificial rain as water sources, respectively. When driven by water droplets, the maximum output voltage is 30 V and the maximum output current is 14 μA on the laser-etched silicone rubber (SR) surface; on the untreated silicone rubber surface, the maximum output voltage is 6 V and the maximum output current is 4.6 μA. When the laser power is 1.2 W(CA = 130°), the maximum output voltage is 15 V and the maximum output current is 8 μA (Figures 7B,C). There is a significant increase in the output of TENG as the contact angle increases. The main reason for this phenomenon is that the fixed volume of water droplets, and the charge contained in it remains essentially constant, and the amount of charge contained is related to the nature of the liquid. The surface of the TENG is hydrophobic, which makes the water droplets on the surface fall rapidly with a small tilt angle. The above results demonstrate that surface hydrophobicity enhancement can increase the output of TENG driven by liquid droplets.
Finally, to demonstrate the power supply performance of the device, the power generation performance of the TENG is used to light up the “CAU”-shaped LEDs (Figure 7D), and the ambient rainfall is simulated to power commercial LEDs by the Al/Superhydrophobic silicone rubber/Al TENG (Figure 7E; Supplementary Video S1). However, the droplet-driven TENG formed on the untreated silicone rubber surface can only light up one LED (Supplementary Video S2). Compared with other TENG solutions, this paper realizes the use of low-power lasers to build TENG directly on silicone rubber surfaces. Handheld lasers can directly create superhydrophobic surfaces on silicone rubber membranes in scenes such as rooftop and thus create TENG. The laser etching method is a green solution as it does not require additional surface materials in the process of creating TENG.
Conclusion
In summary, we have used laser etching to achieve continuous regulation of the silicone rubber surface from superhydrophobic to superhydrophilic, introduced the process of laser etching to regulate the surface wettability, and elaborated its mechanism. At the same time, the rapid in situ repair of the wettability of silicone rubber surface was realized by laser, and the fabrication of a selective hydrophobic array microstructure was realized. In addition, a droplet-driven TENG was fabricated from superhydrophobic silicone rubber using domestic water and rainwater drive. Compared with conventional methods, we highly anticipate that this efficient production and cost-effective method, which results in continuously tunable wettability of the surface with customizable patternable properties, can be innovatively applied to nano energy.
Data availability statement
The original contributions presented in the study are included in the article/Supplementary Material, further inquiries can be directed to the corresponding author.
Author contributions
CL: Conception and design of the study, analysis of data, drafted the manuscript; LZ and NZ prepared the samples, was involved in discussions regarding interpretation of the results, and reviewed and edited the final manuscript; WZ and DX: Experimental design, experimental execution; YZ: Experimental execution; YH: Analysis of data; WZ: Analysis of data, editing the final manuscript.
Funding
The present study was supported by the National Natural Science Foundation of China (Grant No. 51975574) and The Fundamental Research Funds for the Central Universities (Grant No. 2020TC017).
Conflict of interest
The authors declare that the research was conducted in the absence of any commercial or financial relationships that could be construed as a potential conflict of interest.
Publisher’s note
All claims expressed in this article are solely those of the authors and do not necessarily represent those of their affiliated organizations, or those of the publisher, the editors and the reviewers. Any product that may be evaluated in this article, or claim that may be made by its manufacturer, is not guaranteed or endorsed by the publisher.
Supplementary material
The Supplementary Material for this article can be found online at: https://www.frontiersin.org/articles/10.3389/fenrg.2022.987181/full#supplementary-material
References
Cao, N., Yang, B., Barras, A., Szunerits, S., Boukherroub, R., et al. (2017). Polyurethane sponge functionalized with superhydrophobic nanodiamond particles for efficient oil/water separation. Chem. Eng. J. 307, 319–325. doi:10.1016/j.cej.2016.08.105
Chen, Q., Zhao, J., Ren, J., Rong, L., Cao, P. F., and Advincula, R. C. (2019). 3D printed multifunctional, hyperelastic silicone rubber foam. Adv. Funct. Mat. 29 (23), 1900469. doi:10.1002/adfm.201900469
Davis, A., Yeong, Y. H., Steele, A., Bayer, I. S., and Loth, E. (2014). Superhydrophobic nanocomposite surface topography and ice adhesion. ACS Appl. Mat. Interfaces 6 (12), 9272–9279. doi:10.1021/am501640h
Emelyanenko, A. M., Boinovich, L. B., Bezdomnikov, A. A., Chulkova, E. V., and Emelyanenko, K. A. (2017). Reinforced superhydrophobic coating on silicone rubber for longstanding anti-icing performance in severe conditions. ACS Appl. Mat. Interfaces 9 (28), 24210–24219. doi:10.1021/acsami.7b05549
Hou, L., Wang, N., Wu, J., Cui, Z., Jiang, L., and Zhao, Y. (2018). Bioinspired superwettability electrospun micro/nanofibers and their applications. Adv. Funct. Mat. 28 (49), 1801114. doi:10.1002/adfm.201801114
Hu, J., Sun, C., Jiang, X., Zhang, Z., and Shu, L. (2007). Flashover performance of pre-contaminated and ice-covered composite insulators to be used in 1000 kV UHV AC transmission lines. IEEE Trans. Dielectr. Electr. Insul. 14 (6), 1347–1356. doi:10.1109/TDEI.2007.4401217
Jalil, S. A., Akram, M., Bhat, J. A., Hayes, J. J., Singh, S. C., ElKabbash, M., et al. (2020). Creating superhydrophobic and antibacterial surfaces on gold by femtosecond laser pulses. Appl. Surf. Sci. 506, 144952. doi:10.1016/j.apsusc.2019.144952
Jiang, C., Li, X., Ying, Y., and Ping, J. (2021). Fluorinated graphene‐enabled durable triboelectric coating for water energy harvesting. Small 17 (8), 2007805. doi:10.1002/smll.202007805
Jiang, H., Zhang, L., Chen, J., and Liu, M. (2017). Hierarchical self-assembly of a porphyrin into chiral macroscopic flowers with superhydrophobic and enantioselective property. ACS Nano 11 (12), 12453–12460. doi:10.1021/acsnano.7b06484
Kumosa, M., Han, Y., and Kumosa, L. (2002). Analyses of composite insulators with crimped end-fittings: Part I—non linear finite element computations. Compos. Sci. Technol. 62 (9), 1191–1207. doi:10.1016/s0266-3538(02)00066-0
Lan, L., Xiong, J., Gao, D., Li, Y., Chen, J., Lv, J., et al. (2021). Breathable nanogenerators for an on-plant self-powered sustainable agriculture system. ACS Nano 15 (3), 5307–5315. doi:10.1021/acsnano.0c10817
Li, C., Lai, H., Cheng, Z., Yan, J., Xiao, L., Jiang, L., et al. (2020). Coating “nano-armor” for robust superwetting micro/nanostructure. Chem. Eng. J. 385, 123924. doi:10.1016/j.cej.2019.123924
Li, J., Wu, R., Jing, Z., Yan, L., Zha, F., and Lei, Z. (2015). One-step spray-coating process for the fabrication of colorful superhydrophobic coatings with excellent corrosion resistance. Langmuir 31 (39), 10702–10707. doi:10.1021/acs.langmuir.5b02734
Li, X., Jiang, C., Zhao, F., Shao, Y., Ying, Y., and Ping, J. (2020). A self-charging device with bionic self-cleaning interface for energy harvesting. Nano Energy 73, 104738. doi:10.1016/j.nanoen.2020.104738
Li, X., Jiang, Y., Tan, X., Zhang, Z., Jiang, Z., Lian, J., et al. (2022). Superhydrophobic brass surfaces with tunable water adhesion fabricated by laser texturing followed by heat treatment and their anti-corrosion ability. Appl. Surf. Sci. 575, 151596. doi:10.1016/j.apsusc.2021.151596
Liu, M., Li, J., Hou, Y., and Guo, Z. (2017). Inorganic adhesives for robust superwetting surfaces. ACS Nano 11 (1), 1113–1119. doi:10.1021/acsnano.6b08348
Liu, Q., Liu, D., and Yang, Y. (2015). Research on superhydrophobic silicon rubber surface made by high voltage electrospray. High. Volt. Eng. 41 (8), 2818–2824. doi:10.13336/j.1003-6520.hve.2015.08.037
Long, J., Fan, P., Gong, D., Jiang, D., Zhang, H., Li, L., et al. (2015). Superhydrophobic surfaces fabricated by femtosecond laser with tunable water adhesion: From lotus leaf to rose petal. ACS Appl. Mat. Interfaces 7 (18), 9858–9865. doi:10.1021/acsami.5b01870
Ma, J., Porath, L. E., Haque, M. F., Sett, S., Rabbi, K. F., Nam, S., et al. (2021). Ultra-thin self-healing vitrimer coatings for durable hydrophobicity. Nat. Commun. 12 (1), 5210–10. doi:10.1038/s41467-021-25508-4
Ma, K., Jiang, Z., Gao, S., Cao, X., and Xu, F. (2022). Design and analysis of fiber-reinforced soft actuators for wearable hand rehabilitation device. IEEE Robot. Autom. Lett. 7 (3), 6115–6122. doi:10.1109/LRA.2022.3167063
Momen, G., Farzaneh, M., and Jafari, R. (2011). Wettability behaviour of RTV silicone rubber coated on nanostructured aluminium surface. Appl. Surf. Sci. 257 (15), 6489–6493. doi:10.1016/j.apsusc.2011.02.049
Momen, G., and Farzaneh, M. (2011). Survey of micro/nano filler use to improve silicone rubber for outdoor insulators. Rev. Adv. Mat. Sci. 27 (1), 1–13. doi:10.1007/s12274-010-0073-x
Samanta, A., Wang, Q., Shaw, S. K., and Ding, H. (2019). Nanostructuring of laser textured surface to achieve superhydrophobicity on engineering metal surface. J. Laser Appl. 31 (2), 022515. doi:10.2351/1.5096148
Shit, S. C., and Shah, P. (2013). A review on silicone rubber. Natl. Acad. Sci. Lett. 36 (4), 355–365. doi:10.1007/s40009-013-0150-2
Song, Y., Yu, J., Yu, L., Alam, F. E., Dai, W., Li, C., et al. (2015). Enhancing the thermal, electrical, and mechanical properties of silicone rubber by addition of graphene nanoplatelets. Mat. Des. 88, 950–957. doi:10.1016/j.matdes.2015.09.064
Ta, D. V., Dunn, A., Wasley, T. J., Kay, R. W., Stringer, J., Smith, P. J., et al. (2015). Nanosecond laser textured superhydrophobic metallic surfaces and their chemical sensing applications. Appl. Surf. Sci. 357, 248–254. doi:10.1016/j.apsusc.2015.09.027
Velásquez, R. M. A., and Lara, J. V. M. (2018). The need of creating a new nominal creepage distance in accordance with heaviest pollution 500 kV overhead line insulators. Eng. Fail. Anal. 86, 21–32. doi:10.1016/j.engfailanal.2017.12.018
Wang, H., Zhu, Y., Hu, Z., Zhang, X., Wu, S., Wang, R., et al. (2016). A novel electrodeposition route for fabrication of the superhydrophobic surface with unique self-cleaning, mechanical abrasion and corrosion resistance properties. Chem. Eng. J. 303, 37–47. doi:10.1016/j.cej.2016.05.133
Wang, L., Tian, Z., Jiang, G., Luo, X., Chen, C., Hu, X., et al. (2022). Spontaneous dewetting transitions of droplets during icing & melting cycle. Nat. Commun. 13 (1), 378–415. doi:10.1038/s41467-022-28036-x
Wang, Y., Wang, G., He, M., Liu, F., Han, M., Tang, T., et al. (2021). Multifunctional laser‐induced graphene papers with combined defocusing and grafting processes for patternable and continuously tunable wettability from superlyophilicity to superlyophobicity. Small 17 (42), 2103322. doi:10.1002/smll.202103322
Wen, G., Gao, X., and Guo, Z. (2018). Simple fabrication of a multifunctional inorganic paper with high-efficiency separations for both liquids and particles. J. Mat. Chem. A Mat. 6 (43), 21524–21531. doi:10.1039/C8TA08782B
Wu, J., Dong, J., Wang, Y., and Gond, B. K. (2017). Thermal oxidation ageing effects on silicone rubber sealing performance. Polym. Degrad. Stab. 135, 43–53. doi:10.1016/j.polymdegradstab.2016.11.017
Yoshimitsu, Z., Nakajima, A., Watanabe, T., and Hashimoto, K. (2002). Effects of surface structure on the hydrophobicity and sliding behavior of water droplets. Langmuir 18 (15), 5818–5822. doi:10.1021/la020088p
Yung, C. S., Tomlin, N. A., Heuerman, K., Keller, M. W., White, M. G., Stephens, M., et al. (2018). Plasma modification of vertically aligned carbon nanotubes: Superhydrophobic surfaces with ultra-low reflectance. Carbon 127, 195–201. doi:10.1016/j.carbon.2017.10.093
Keywords: wetting modulation, in-situ repair, laser etching, surface modification, silicone rubber, triboelectric nanogenerator
Citation: Liang C, Zhang L, Zhao N, Zhou W, Deng X, Zhou Y, Hou Y and Zhang W (2022) Laser etching-based surface wetting modulation of silicone rubber for triboelectric nanogenerator. Front. Energy Res. 10:987181. doi: 10.3389/fenrg.2022.987181
Received: 05 July 2022; Accepted: 26 August 2022;
Published: 09 September 2022.
Edited by:
Xianhua Liu, Tianjin University, ChinaCopyright © 2022 Liang, Zhang, Zhao, Zhou, Deng, Zhou, Hou and Zhang. This is an open-access article distributed under the terms of the Creative Commons Attribution License (CC BY). The use, distribution or reproduction in other forums is permitted, provided the original author(s) and the copyright owner(s) are credited and that the original publication in this journal is cited, in accordance with accepted academic practice. No use, distribution or reproduction is permitted which does not comply with these terms.
*Correspondence: Wenqiang Zhang, zhangwq@cau.edu.cn