- 1Wuppertal Institute for Climate, Environment and Energy, Wuppertal, Germany
- 2Institute of Mathematics, Osnabrück University, Osnabrück, Germany
Transponder-based Aircraft Detection Lighting Systems (ADLS) are increasingly used in wind turbines to limit beacon operation times, reduce light emissions, and increase wind energy acceptance. The systems use digital technologies such as receivers of digital transponder signals, LTE/5G, and other information and communication technology. The use of ADLS will be mandatory in Germany both for new and existing wind turbines with a height of >100 m from 2023 (onshore) and 2024 (offshore), so a nationwide rollout is expected to start during 2022. To fully realize the benefits while avoiding risks and bottlenecks, a thorough and holistic understanding of the efforts required and the impacts caused along the life cycle of an ADLS is essential. Therefore, this study presents the first multi-aspect holistic evaluation of an ADLS. A framework for evaluating digital applications in the energy sector, previously developed by the authors, is refined and applied. The framework is based on multi-criteria analysis (MCA), life cycle assessment (LCA), and expert interviews. On an aggregated level, the MCA results show an overall positive impact from all stakeholders’ perspectives. Most positive impacts are found in the society and politics category, while most negative impacts are of technical nature. The LCA of the ADLS reveals a slightly negative impact, but this impact is negligible when compared to the total life cycle impact of the wind turbines of which the ADLS is a part. Besides the aggregated evaluation, detailed information on potential implementation risks, bottlenecks, and levers for life cycle improvement are presented. In particular, the worldwide scarcity of the required semiconductors, in combination with the general lack of technicians in Germany, lead to the authors’ recommendation for a limited prolongation of the planned rollout period. This period should be used by decision-makers to ensure the availability of technical components and installation capacities. A pooling of ADLS installations in larger regions could improve plannability for manufacturers and installers. Furthermore, an ADLS implementation in other countries could be supported by an early holistic evaluation using the presented framework.
1 Introduction
Wind turbines (WTs) are potential obstructions to air traffic and must therefore be equipped with obstruction lights (flashing red beacons) if their total height exceeds 100 m (outside of urban areas), as defined by the International Civil Aviation Organization (ICAO, 2018) and specified for Germany in the “General Administrative Regulation on the Marking of Aviation Obstructions” (BMDV, 2020). Obstruction lights can cause annoyance and thus reduce the acceptance of wind energy (Pohl et al., 2021). As modern turbines become taller and more turbines are installed, more people may be affected, leading to an increasing acceptance problem. Social acceptance of wind energy projects, however, is of great importance to avoid local opposition. Such opposition can delay or impede the construction of new WTs and even slow down the overall transition to renewable energies (Ellis and Ferraro, 2016).
In order to reduce the light emissions caused by the flashing red beacons and thereby increase the social acceptance of WTs, the German regulatory authority has specified in the Renewable Energy Act (German Federal Ministry for Economic Affairs and Energy, 2021) the obligation to equip WTs with Aircraft Detection Lighting Systems (ADLS). ADLS allow WTs’ beacons to remain off during nighttime hours when no aircraft is detected in the vicinity. Under the aforementioned law, ADLS are mandatory for all onshore WTs that require aviation obstruction lighting and all offshore WTs located near the coast and in certain offshore areas. The obligation will take effect on 1 January 2023 for onshore and 1 January 2024 for offshore turbines. It is estimated that approximately 17,500 onshore turbines (Roscher, 2019) and all of the 1,500 offshore turbines (Deutsche WindGuard GmbH, 2021) will need to be retrofitted by the start of the obligation. However, due to a technological dispute that was not resolved until 2019, only a minority of turbines have already been equipped. Therefore, a large rollout is expected during the year 2022.
Given this nationwide rollout, a thorough understanding of its impacts is necessary for all stakeholders involved to be able to weigh positive and negative impacts against each other and to avoid otherwise unforeseen potential negative impacts or implementation bottlenecks. Several studies analyzed the impact of ADLS on WT acceptance. An early study (Hübner and Pohl, 2010; Pohl et al., 2012), funded by the German Federal Ministry for the Environment, Nature Conservation, Nuclear Safety, and Consumer Protection, found that nighttime obstruction lighting is less of a cause for annoyance compared to changes in the landscape and emitted noise, but is perceived as similarly annoying compared to shadow flicker. The authors recommend the use of ADLS as a measure to improve acceptance. Further, in (Rudolph et al., 2017), the authors confirmed previous studies and identified a perceived annoyance associated with obstruction lights from WTs. A comparison of annoyance between Europe and the United States (Hübner et al., 2019) shows that obstruction lights cause slightly higher annoyance among Europeans, but overall annoyance levels are relatively low. A more recent study found that annoyance from obstruction lighting is generally low but on average higher than noise annoyance and more geographically widespread. The authors again recommend the use of ADLS (Pohl et al., 2021). Overall, evidence suggests that ADLS will indeed have a positive impact on the social acceptance of wind turbines. In addition, a technical risk assessment focusing on aviation was conducted for the transponder-based technological options in comparison to radar-based options (Behrend, 2019). The author concludes that the risk of a system failure with consequences for aviation safety is very low and the same for all technological options.
According to the authors’ knowledge, societal impacts beyond acceptance, non-aviation-risk-related technical impacts, and environmental as well as economic impacts have not yet been analyzed. In particular, neither a life cycle assessment nor an environmental study, or a holistic assessment incorporating multiple perspectives, involving relevant stakeholders, and considering all relevant impact areas has been conducted so far. In order to close this gap, in this paper, a multi-method framework for evaluating digital applications in the energy sector, previously developed by the authors (Weigel et al., 2021), is refined and applied to conduct a holistic evaluation of ADLS. The main novelty of the study is that it presents the first holistic evaluation of ADLS, in contrast to existing publications, which focus on single evaluation aspects. A secondary minor novelty is the refinement of the evaluation framework and its application.
The remainder of the article is organized as follows: the refinement of the evaluation framework and its adaption to ADLS is described in Section 2. While Section 3 shortly describes the ADLS technology and the chosen assumptions, Section 4 shows the results of the holistic evaluation. After discussing the results in Section 5, the conclusions and an outlook are given in Section 6.
2 Methodology
There are a variety of sustainability and multi-criteria evaluation methods and combinations of methods, many of which have recently been used for high-level assessments at the country level, e.g. (Sun et al., 2022), (D’Adamo et al., 2022), for more specific assessments in the energy sector, e.g., (Kluczek and Gladysz, 2022), (Naegler et al., 2021), and for assessments of digital topics, e.g., (Gährs et al., 2021), (Zhang et al., 2021). A review of methods applied in the energy sector (energy planning) and each method’s appropriateness in the decision problem’s context is provided in (Cajot et al., 2017). In (Weigel et al., 2021) the authors thoroughly discuss a variety of current evaluation and assessment methods and identify a gap regarding an approach to transparently and holistically evaluate digital applications in the energy sector. To fill this gap, a combination of three well-established methods is suggested, and its use is demonstrated. The novelty of the framework consists of the specific combination of methods and its adaption to digital applications in the energy sector.
The objective of the framework is to provide a structured basis for the holistic evaluation of digital applications. To achieve this holistic view, multiple criteria covering the impact areas technology, ecology, economy and society and politics are evaluated in a multi-criteria analysis (MCA), and the perspectives of relevant stakeholders are considered in the form of weighting profiles. While most ecological criteria are assessed by performing a life cycle assessment (LCA), all other criteria are assessed by conducting expert interviews (EI) with relevant stakeholder representatives. Furthermore, due to the dynamic development of digital applications, a flexible approach is applied that can be adapted to the practitioner, the application, and the availability of information. In this way, a wide range of digital applications, including future developments, can be evaluated. Last but not least, the applied approach provides detailed insights as well as aggregated results with a high level of transparency on each step of the evaluation.
The framework applied in this study is a refined version of the framework originally presented by the authors in (Weigel et al., 2021) and consists of the following steps (Figure 1):
1) Definition of application, functional unit, reference, assumptions, and boundary conditions
2) Selection of criteria
3) Development of weighting profiles
4) Assessment of the criteria
a) Environmental criteria based on LCA
b) All other criteria based on EIs
5) Evaluation of application based on criteria assessments and weighting profiles within MCA
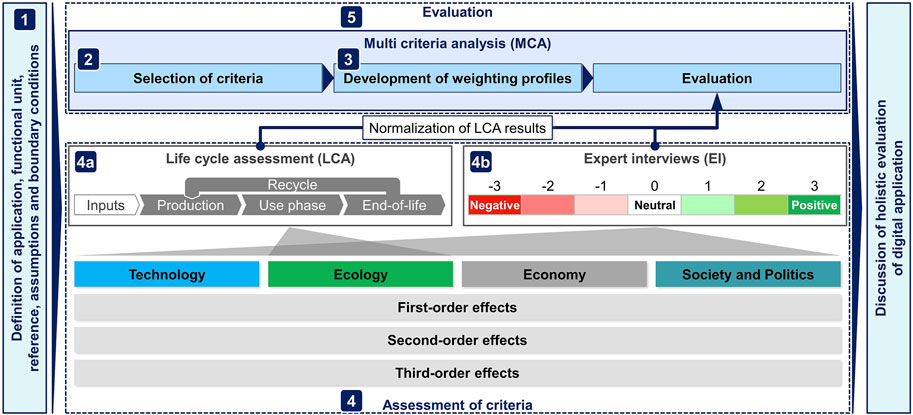
FIGURE 1. Modified multi-method framework for evaluating digital applications in the energy sector, based on (Weigel et al., 2021).
Generally, the first step is the definition of the application under investigation, the functional unit, the reference for the evaluation, and key assumptions. This step ensures a consistent and efficient assessment and evaluation process.
The selection of criteria (step 2) can be moved up and down in the sequence within certain limits. In this study, it is performed beforehand based on the general requirements for digital applications in the energy sector, following a thorough literature review and discussions with experts.
In step 3, weighting profiles are developed. To some extent, this could also be done beforehand, based on general requirements for digital applications. However, case-specific adaptations are likely to be required, as different applications may involve different stakeholder roles. The study-specific stakeholder profiles are derived from expert opinions following the expert interviews in step 4b. The point allocation method is applied. Experts are asked to assign 100 points sequentially to categories and then to criteria. The 100 points represent 100% importance, a concept that is intuitively understood by the interviewees.
The LCA approach (step 4a) is based on the standard defined in (ISO/TC 207/SC 5, 2006). It is carried out using the software openLCA (v1.10.1), the ecoinvent (v3.3) database, and the CML2001 impact calculation method, from which the environmental impact criteria global warming potential 100a (GWP), adiabatic resource depletion (ARD), human toxicity 100a (HT), and ecotoxicity 100a (ET—as the average of different ecotoxicity aspects) are selected, extended by the cumulative energy demand (CED). The LCA results are normalized to the MCA evaluation scale by comparison with a reference value. This reference value is a quantification of the reference defined in step 1.
The expert interviews (step 4b) are semi-standardized, which ensures comparable results across different EIs while providing the flexibility to capture additional detailed information. The list of criteria is used as the interview structure.
The MCA (step 5) uses a direct ordinal rating scale ranging from -3 (strongly negative impact) via 0 (no/neutral impact) to +3 (strongly positive impact). The scale is intuitive for experts, and the evaluation can be broken down into two questions: 1. Is the impact positive, negative or neutral (+ or—range); 2. how positive or negative is the impact (±1, 2, or 3). The simple additive weighting (SAW) method, also known as the weighted sum method (WSM) is used to aggregate the criteria evaluations into category and total results. The SAW method is commonly applied due to its popularity and simplicity (Cajot et al., 2017). It provides a high level of transparency on how results are aggregated from detailed criteria evaluations.
Compared to the originally provided version of the framework (Weigel et al., 2021), three main improvements to the method are made in this paper:
1) The list of criteria is modified in order to reduce the complexity and eliminate overlaps between criteria. In particular, the number of responses per criterion, the weighting of each criterion, as well as direct expert feedback were evaluated to identify required adaptions. Based on the findings, the former sub-criteria level is eliminated, the total number of criteria is reduced, and the number of criteria is more evenly distributed among the categories. Some sub-criteria are upgraded to criteria, and a few new criteria are added. The updated criteria used in this study can be found in the presentation of the results in Section 4.4.
2) In addition, the expert interview approach is adjusted. The weighting (formerly being the first part of each expert interview) is done independently by the experts after the interview. The adapted approach meets the experts’ expectations to talk directly about the application itself, shortens the interview, and reduces the interviewer’s influence on the weighting. However, a good explanation of how to perform the weighting is necessary, and not all experts provide (useful) weighting results on their own.
3) Last but not least, an indication of the uncertainty and data robustness of the expert interview results is assessed using the standard deviation and the number of received evaluations. The standard deviation
where
3 ADLS technology, system boundaries, and assumptions
3.1 Description of the technology
Different technologies to equip WTs with an ADLS are permitted. Three technological approaches can be distinguished based on active radar, passive radar, and transponders (secondary radar). Each technology may have its own use case due to its inherent advantages and disadvantages. There is evidence, however, that transponder-based ADLS technology will be predominantly deployed. Most of the consulted experts expected this trend, and two organizations are already in the process of covering two entire German states with this technology—North Rhine-Westphalia (Bode and Klümper, 2021) and Saarland (BNK, 2022). Therefore, this publication focuses on transponder-based ADLS.
Transponders have been widely used in commercial aviation for several decades. They are considered part of secondary surveillance radar (SSR). The term transponder is a hybrid of transmitter and responder. After receiving a signal from a secondary radar antenna on 1,030 MHz, the transponder actively sends a response signal on 1,090 MHz. The response signal contains a four-digit identity code as a minimum (Mode A) or a unique 24-bit aircraft identity number, altitude, speed, and flight path, as well as GPS coordinates for ADS-B (Automatic Dependent Surveillance - Broadcast) as a maximum (Mode S enhanced). Transponder responses are triggered by air traffic control (ATC) and other aircraft’s traffic alert and collision avoidance systems (TCAS), see Figure 2A.
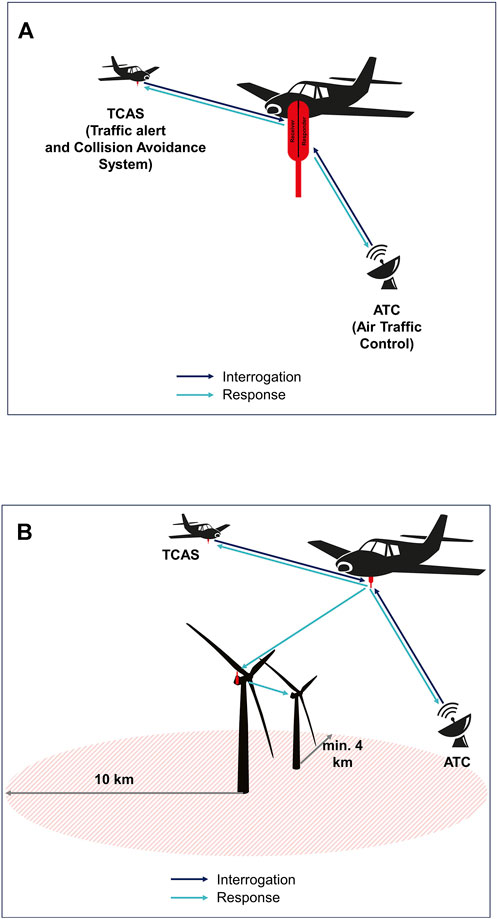
FIGURE 2. Illustrative functionality of (A) a transponder in aviation; (B) a transponder-based ADLS.
If airborne transponders are not triggered, they broadcast one signal per second by default (Mode S only, not Mode A). All aircraft flying at night within or outside of air traffic-controlled areas must use a Mode S transponder (BMDV, 2018). Some exceptions exist for military, police, and rescue aircrafts. The signals sent out by the aircrafts’ transponders are used by the transponder-based ADLS. The ADLS passively receive the transponder signals without sending any interrogation signal themselves. The minimum information received is the aircraft identity code (from Mode A transponders). In this case, the distance is calculated based on the signal intensity. In most cases, more information such as altitude, speed, flight path, and GPS coordinates are received (Mode S and ADS-B), and the exact position can be determined. An ADLS receiver covers a minimum radius of 10 km. Based on the defined impact area of a horizontal 4 km radius around each WT (BMDV, 2020), all WTs within a 6 km radius can be covered by one ADLS, see Figure 2B. In practice, the ADLS receives transponder signals of well beyond 10 km, however, this does not change the evaluation since the technical setup (how many turbines are covered by one ADLS) is defined by the 10 km minimum radius.
3.2 Functional unit and system boundaries
The functional unit of the evaluation is one single transponder-based ADLS. The reference system used to evaluate the magnitude of the impact is the wind turbines covered by the ADLS. The selection of the reference is therefore aligned with the subjective perception of the relevance of ADLS’s impact on the system they are part of. Since the number of covered turbines and the technical configuration of the ADLS may vary, a base case is defined, and sensitivity analysis for different setups are conducted. Theoretically, for modern wind farms, there is no limit to how many WTs can be covered by one single ADLS. In practice, however, there are technical, topological, and ownership structure limitations. In this paper, a setup with eight turbines is chosen as the base case based on the average number of turbines covered per ADLS in the German State of North Rhine-Westphalia (Bode and Klümper, 2021). If all covered turbines have a single central communication unit, which is usually the case for modern wind farms, the central ADLS can directly operate the obstruction lights of all turbines, as depicted in Figure 2B. However, if a central communication interface is not available, additional communication modules must be installed. For this study, the case of only one communication unit is chosen due to a lack of information on a realistic average number of communication units per ADLS. Therefore, the base case is defined as one ADLS with one signal receiver and one communication unit covering eight WTs.
3.3 Life cycle assessment key assumptions
For the life cycle inventory (LCI), the material and energy flows of one single ADLS are modeled. One analyzed base case ADLS consists of the following hardware components: one signal receiver, one communication module, mounting, cabling, antennas, and additional infrared (IR) beacons required per each of the eight turbines. As depicted in Figure 3, the model covers the production, the use, and the end-of-life phases. In addition to the directly attributable effects on the mass and energy flow, two effects caused by the reduced operating time of the beacons are additionally allocated to the LCI. Based on real-world data from a test site supplied by an ADLS supplier, it is assumed that the beacons remain off during 98% of the nighttime. Besides a reduced beacon electricity consumption, the reduced operating hours lead to an increased lifetime of the beacons’ LEDs and thus to a reduced need for replacement. A reduction in LED replacement of 1.29 units per beacon over the analysis period is calculated based on an expected LED operating life of 50,000 h and a correction factor based on manufacturers’ knowledge of the probability of failure modes leading to replacement. It is assumed that the ADLS hardware has a technical lifetime of more than 25 years, but the hardware is decommissioned together with the turbine so that the effective ADLS lifetime depends on the lifetime of the turbine. Here, a turbine and ADLS lifetime of 20 years is assumed. Therefore, the analysis period is also set at 20 years.
Since the electricity consumption of digital applications with a long expected lifetime tends to have a great effect on the life cycle impact, special consideration is given to the electricity mix. Three different electricity mixes are defined for this study based on the expected development of greenhouse gas (GHG) intensity of the German electricity mix over time (measured in CO2 equivalents, CO2 eq.). The three mixes are defined as described in (Weigel et al., 2021), based on historical CO2 emissions and energy consumption, reference prognosis, and trend scenarios for future energy generation and the 2019 developed coal exit path. Different shares of electricity generation technologies are modeled for each mix, however, the underlying unit processes for these technologies in the LCA database remain unchanged as no prospective datasets are available. The 2022 mix is used for the production phase, the expected 2032 mix for the use phase, and the expected 2042 mix for the end-of-life phase. The ADLS’s energy self-consumption during the use phase is covered to 19% by electricity from the German electricity mix and to 81% by electricity from the wind turbine itself. The life cycle impact of the electricity generated by the wind turbine is based on a 3.25 MW turbine currently in operation. Effects that are not considered in the LCI are the need for maintenance and spare parts (no data available and the impact is likely to be very small), the server operation and data transmission (there is no data available), and the transport of materials for production, installation, and end-of-life steps (the impact is likely to be very small as most steps take place within Germany). Relevant assumptions and sources are listed in Appendix Table 2 in the Annex.
3.4 Expert interviews assumptions
The aim for the selection of experts is to cover all stakeholder roles and identify experts with a high level of expertise. In a first step governmental, scientific, business and journalistic publications were analyzed to identify relevant stakeholder roles and experts. Following the initial identification, further experts were identified by asking each expert at the end of the interview to identify further stakeholder roles and name experts.
The group of twelve interviewed experts includes: four representatives of wind farm operators or operator associations in charge of implementing ADLS, two environmental NGO experts for wind energy, two ADLS-specific policy advisors to the involved German Federal Ministries, one employee of a wind turbine manufacturer tasked with equipping future turbines with ADLS, one ADLS manufacturer (from the same company that supplied the data for the LCA), and two scientists with expertise in social acceptance of WTs, including lighting induced stress. No affected neighbors of WTs were directly interviewed for this study, but rather the two mentioned scientists researching social acceptance were interviewed to present their insights of the perspective of this group. All experts are based in Germany and are native German speakers. Age and sex were not documented as deemed not relevant.
The individual online expert interviews were conducted between September 2021 and January 2022 and each took about 90 min. No material was sent out beforehand, the interviews were not recorded or transcribed, and the results were captured as the evaluation per criterion, including qualitative remarks. Furthermore, following the expert interviews, the experts were asked to assign weights to the criteria and to submit the weighting within 2 weeks. Based on the responses of nine experts (one wind farm operator, the turbine manufacturer, and the ADLS manufacturer did not submit weighting), five (non-representative) weighting profiles reflecting key stakeholders’ perspectives were derived.
4 Results
In this section, the results of the LCA are presented first, followed by the results of the MCA, which integrates LCA and expert interviews. The LCA results are presented separately from the MCA results because detailed conclusions about life cycle impacts can be drawn.
4.1 LCA results
4.1.1 Impacts of the base case
In Figure 4, the breakdown of the calculated life cycle impacts over 20 years for the base case is depicted. In total, all five impacts increase with the application of an ADLS. The shares of the three life cycle phases on the total impact, however, are very different. Each step of the production phase leads to an increase in all five impacts. In the use phase, the energy consumption of the ADLS components leads to an increase, while the beacon electricity savings and the LED replacement reduction cause an impact decrease. Relative to the production and the use phase, the end-of-life phase causes a small impact decrease. The effort for disassembly and landfilling is more than compensated by credits given due to recycling.
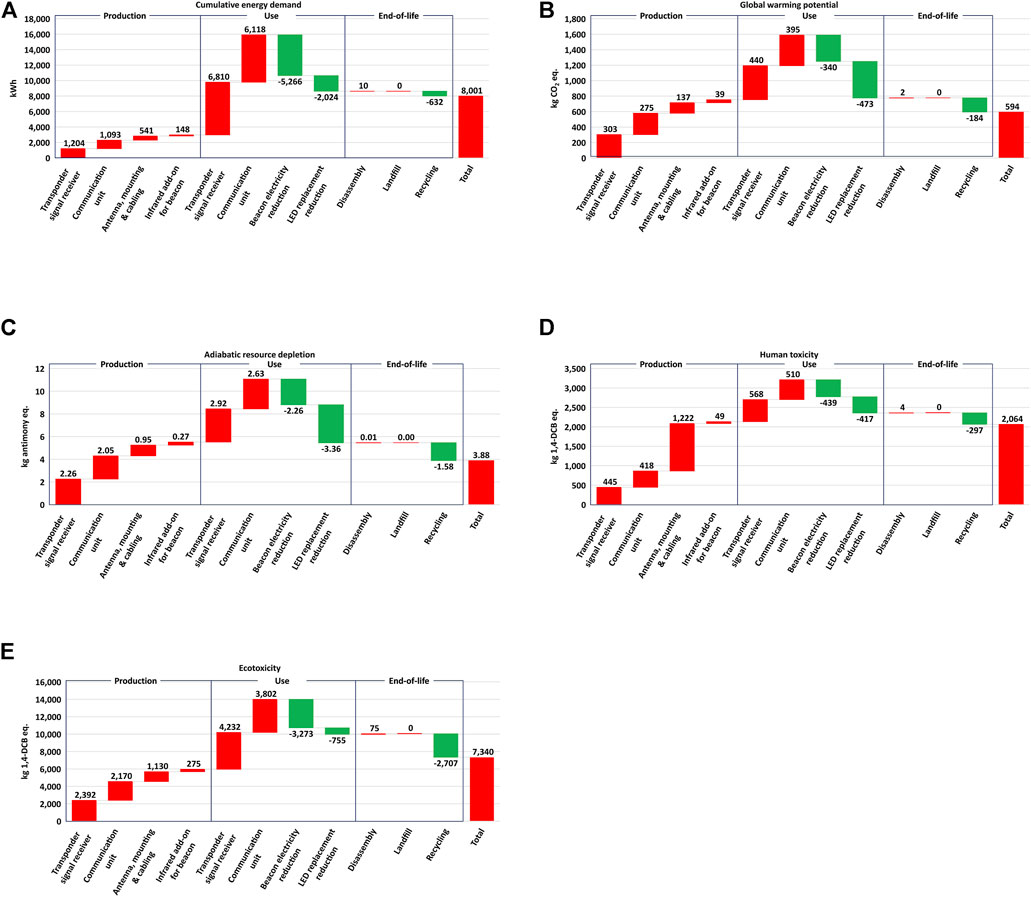
FIGURE 4. Life cycle impact assessment for the base case. Functional unit: one ADLS in the base case. (A) Cumulative energy demand; (B) Global warming potential; (C) Adiabatic resource depletion; (D) Human toxicity; (E) Ecotoxicity.
The cumulative energy demand (CED) is depicted in Figure 4A. The ADLS use phase’s energy consumption is 4.3 times higher than the production’s energy consumption. The decisive impact on the CED during the 20-years use phase is caused in particular by the electricity consumption of the ADLS hardware (approx. 13,000 kWh) and the saved electricity consumption of the beacons (approx. -5,200 kWh).
Figure 4B shows the breakdown of the global warming potential (GWP). The ADLS operation’s energy consumption emissions (835 kg CO2 eq.) over 20 years are about 10% higher than the production-related emissions (754 kg CO2 eq.). Similar to the CED, the GWP is mainly caused by the ADLS’s electricity consumption. Since 81% of the consumed electricity is assumed to be generated by the turbine itself (with a low impact on GWP), the GWP is mainly driven by the remaining 19% taken from the grid (although an increasingly decarbonized future German electricity mix is assumed). During the production phase, the signal receiver is the largest GWP contributor with 303 kg CO2 eq., followed by the communication unit with 275 kg CO2 eq. The mounting, cabling, and antenna (137 kg CO2 eq.) and the required infrared beacons (39 kg CO2 eq.) cause a relatively small GWP. For these hardware units, the components with the highest GWP are the printed circuit boards, including the electronic components mounted on them such as integrated circuits, resistors and capacitors, other electronic components such as power supplies, heaters, surge suppressors, and circuit breakers, and the mounting material (mainly steel). The savings of -814 kg CO2 eq. (caused by lower beacon energy consumption and reduced replacement of LEDs) offset 97% of the ADLS’s electricity consumption emissions. The reduction in LED replacement causes a larger GWP effect (-473 kg CO2 eq.) than the reduced beacon electricity consumption (-340 kg CO2 eq.). The main driver for the LED replacement reduction’s GWP effect is the saved energy consumption of the avoided production of diodes and PCBs. Again, the beacon energy consumption reduction’s GWP effect is driven by the 19% CO2 intensive electricity taken from the German energy mix. The end-of-life steps, especially disassembly and landfilling of the non-recyclable parts, do not cause significant GWP impacts. Recycling, however, can avoid emissions of -184 kg CO2 eq.
The breakdown of adiabatic resource depletion (ARD) impacts given in Figure 4C shows a high correlation with the GWP. The main driver in both cases is the use of fossil fuels for energy (electricity and heat) generation during production, use, and end-of-life. However, compared to GWP, production and recycling have a slightly higher proportional impact on ARD, as physical production materials contribute directly to ARD, while only their CO2 eq. footprint contributes to GWP.
The main difference in the breakdown of human toxicity (HT) impacts in Figure 4D compared to GWP and ARD is the high impact of the antenna, mounting, and cabling component production. With 1,222 kg 1,4-DCB eq., these parts cause more than half of the total HT production impact, compared to only 17–18% of the production impact for GWP and ARD. This high impact is driven by the exploration of copper, which is mainly used for cabling. The HT production impact is significantly higher than the impact caused by the ADLS’s electricity consumption during the use phase. Furthermore, it is evident that both savings in the use phase have a similar impact on the HT, while for GWP and ARD, the saving due to the reduction of LED replacement is larger.
The ecotoxicity (ET) impact in Figure 4E shows a relatively high impact of the ADLS energy consumption compared to the production. Moreover, the saved electricity consumption of the beacons causes a much higher ET impact than the reduction of the LED replacement. It is evident that ET is also driven by energy consumption. However, unlike GWP and ARD, it is not driven by the use of fossil fuels but rather by the production of the required power infrastructure such as power plants, wind turbines, and grids. Therefore, the advantage of using wind energy over fossil fuel energy is smaller for ET than for GWP/ARD. During production, ET is driven by the use of gold, brass, silver, and other precious metals.
In the following, the sensitivity of the results regarding the most relevant factors is presented. These factors are, in particular, the number of turbines covered by one ADLS, the required number of communication units, and the achieved beacon operation reduction rate.
4.1.2 Variation in the number of wind turbines
Figure 5A depicts the life cycle impact depending on the number of WTs covered by the ADLS. The technical setup of the base case is applied, i.e., the ADLS contains one signal receiver and one communication unit independently of the number of covered turbines. On the one hand, the required efforts for the production and operation of the ADLS hardware remain the same regardless of the number of turbines. In theory, there is no limit to how many turbines can be covered by one ADLS as long as they are within the defined spatial range of the system. On the other hand, the savings, i.e., the reduction of the beacon energy consumption and the LED replacements, occur per turbine. Therefore, the life cycle impacts decrease linearly the more turbines are covered by one ADLS. Depending on the impact criteria, the break-even point (BEP), i.e., the number of turbines, for which the savings outweigh the impacts of ADLS production and operation, is different. While for less than 15 turbines, all indicators show an increased impact (as also analyzed above for the base case with 8 WT), they all decrease if more than 30 turbines are covered. The BEP for both the GWP and the ARD, appears at about 15 WTs. In the case of CED, ET, and HT, it occurs only at larger numbers of turbines of about 18, 27, and 30, respectively. The setup of one single turbine covered by one ADLS is not displayed in Figure 5 since, in this case, the ADLS hardware can be reduced, making this a special case with a non-linear effect, which, however, in reality, is rather rare.
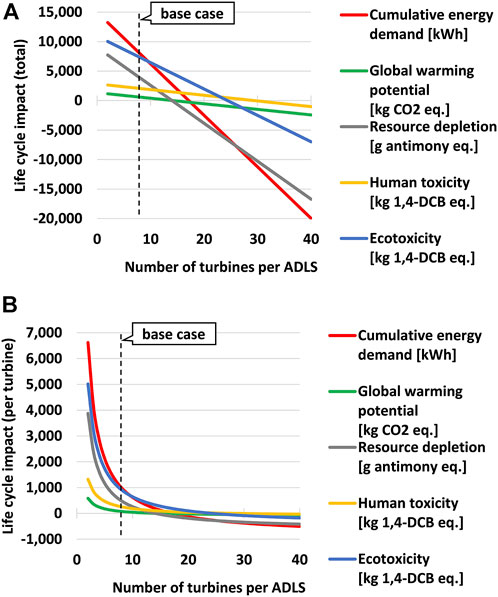
FIGURE 5. Life cycle impact of one ADLS for different numbers of covered WTs. Functional unit: one ADLS in the base case with varying numbers of WT. (A) per ADLS; (B) per turbine.
If the results are normalized to one turbine, the impact per turbine decreases and follows a hyperbola (Figure 5B). The family of curves can be described by
where
In order to understand the different BEP per impact criteria, the findings of Figure 5 and Figure 4 need to be combined. GWP and ARD are both driven by the use of fossil fuels. Both savings, beacon electricity consumption reduction and LED replacement reduction, increase directly with the number of turbines and have a proportionally large effect on fossil fuel use and thus on GWP and ARD, causing a BEP at already 15 turbines. The CED is directly driven by energy demand, regardless of its source. Therefore, while the reduction in LED replacement has a proportionally large impact (compared to the energy consumption of the ADLS and the saved energy consumption of the beacons) on GWP and ARD, it does not have a strong impact on CED. Consequently, the CED savings achieved per turbine are proportionally smaller than for GWP and ARD, so more turbines are required to achieve a net-zero balance. The HT has the same proportional reduction impact per turbine as the GWP (the lines are close to parallel), but because of the higher production effect, mainly driven by the use of copper, more turbines are needed to balance the production impact, i.e., the BEP shifts to the right. The ET has a rather similar BEP as the HT. However, the main reason why more turbines are needed to offset the ET impact of the production is that the reduction in LED replacement has a proportionally small impact on ET since, for the given quantity of LEDs, neither the total energy consumption for production nor the demand for precious metals is exceptionally high.
4.1.3 Variation of the required number of communication units
The base case contains one communication unit. Depending on the technical and legal setup of the wind farm, however, between one communication unit for all turbines and one unit for each turbine may be necessary. The additional communication units cause impacts both through their production and their energy consumption during the use phase. The results for different numbers of communication units are depicted in Figure 6: One unit as the base case (all turbines are part of the same wind farm with one central communication infrastructure) and seven units as the worst case (each turbine is a single wind farm, and/or no central communication infrastructure exists). It is evident that the requirement to install more than one communication unit could drastically increase all analyzed life cycle impacts. Between the best and the worst case, the identified impacts increase by a factor of about 3–7, depending on the impact criterion. The highest increase is observed for ARD, GWP, and CED.
4.1.4 Variation of the beacon operation reduction rate
Furthermore, the life cycle impact depends on the beacon operation reduction rate, i.e., the percentage of nighttime that the lights remain off. The beacons are assumed to remain off during 98% of the nighttime in the base case. The reduction rate could realistically be as low as 75% near airports with more nighttime air traffic. Figure 6 shows the impact of a low reduction rate, leading to a smaller reduction in beacon power consumption as well as a smaller reduction in LED replacement. The impact on the outcome compared to the base case is an increase of 50% on GWP and ARD, 30% on CED, and approximately 15% on human and ecotoxicity.
4.2 LCA result normalization
Since the MCA evaluation scale ranges from -3 to +3, the LCA results must be normalized to this scale in order to include them in the MCA. The reference chosen in Section 3.2 for this study are the WTs, which are covered by the ADLS, thus, the reference value is the life cycle impact of these WTs. By comparing the ADLS’s impact to the reference value, the normalized evaluation
based on the ratio between the assessed ADLS’s and WTs’ life cycle impact,
It can be seen that all criteria are evaluated very close to zero, i.e., with a negligible or neutral impact. In the context of the life cycle impact of the WTs covered by the ADLS, the ADLS life cycle impact is less than 0.006% and thus negligible. This finding also holds true for the technical scenarios with the highest increase in life cycle impact (two turbines, one receiver, one communication unit) and the highest (realistic) decrease in life cycle impact (40 turbines, one receiver, one communication unit). It can be concluded that environmental life cycle impacts do not play a significant role in the holistic evaluation of the application in this case.
4.3 MCA weighting profiles
Before combining the LCA and the EI assessment results in the MCA, weighting profiles for the criteria must be defined. As described earlier, the responses of nine experts are used to derive five weighting profiles, as depicted in Figure 7. The wind farm operator perspective is based on the three wind farm operator representatives, the consumer perspective on the two scientists with wind energy acceptance expertise, the environmental NGO perspective on the two NGO members, and the political perspective on the two political advisors. The national economy perspective is calculated as the average of the preceding weighting profiles.
For wind farm operators, i.e., the users of ADLS, the ecological impact is the most important impact, followed by societal, technical, and economic aspects, all of which are about equally important. From the consumers’ point of view, technical and economic aspects have low relevance, while aspects concerning society and politics have the highest importance. From the environmental NGO’s perspective, ecological aspects are by far the most important ones. The political perspective is the most balanced weighting profile, and the national economy represents the average view across all interviewed experts. Overall, ecological aspects are considered as most important, while the economic aspects are considered the least relevant.
4.4 MCA results integrating LCA and EIs
Subsequently, the normalized LCA and the EI results are combined in the MCA and aggregated based on the weighting profiles. First, the results calculated based on the “national economy” weighting profile are presented (Figure 8 and highlighted lines in Figure 9), followed by an overview of the results based on the different weighting profiles (Figure 10).
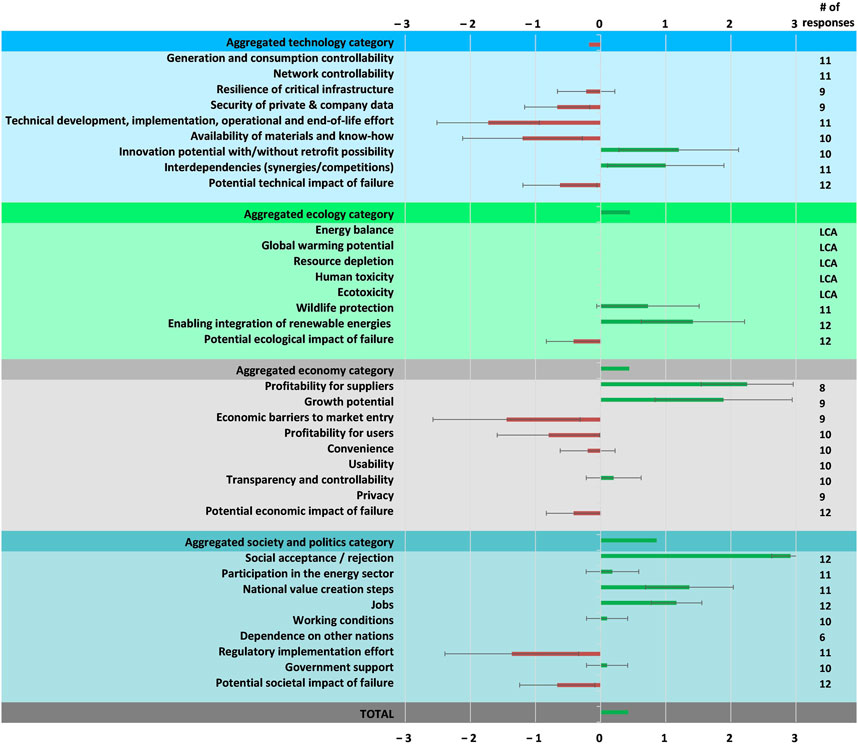
FIGURE 9. Criteria level MCA results from a national economy perspective, including standard deviation and number of data points.

FIGURE 10. Aggregated MCA results from different stakeholders’ perspectives: (A) Wind farm operator; (B) Consumer (Prosumer); (C) Environmental NGO; (D) Politics; (E) National economy.
Figure 8 illustrates the aggregated results at the MCA category level. The overall evaluation is slightly positive. The largest positive impacts are in the society and politics category. The technology category is evaluated slightly negatively. The ecology and economy categories are both slightly positive.
The next level of detail, i.e., the results per criterion, is depicted in Figure 9. Together with each result, the standard deviation of the EI responses and the number of responses received are given as an indication of the reliability of the data per criterion. Since the values of the five LCA-based criteria are derived from the normalization in Section 4.2, no standard deviation is provided. Further sources of uncertainty are qualitatively discussed in Section 5.3.
Within the slightly negatively evaluated technology category, the main negative drivers are the required technical effort and the availability of materials and know-how. The greatest technical effort is required for development and implementation, while operation does not cause any relevant additional effort. In particular, the implementation effort for existing turbines is high, especially for old turbines without appropriate communication infrastructure. For newly built turbines, the additional implementation effort will be low. Regarding the availability of materials and know-how, a major bottleneck for the rollout is identified. In particular, the production bottleneck is a shortage of ICT equipment on the world market, especially semiconductor-based integrated circuits. A bottleneck for the implementation is a shortage of technicians with the necessary know-how due to a general shortage of technicians in Germany. Although these shortages are generally expected to persist for several years, they are most likely no long-term barrier to further ADLS deployment after the initial rollout due to the small quantities needed in comparison to the total capacities of semiconductors and trained technicians. Minor negative effects are expected on the resilience of the critical infrastructure and data security, as there are additional potential points of attack, especially for systems with internet access. However, since the information processed is not critical, the risk of cyberattacks is low. Nevertheless, there is an additional dependency on the availability of correct data. The main positive factors are seen in the potential for innovation, such as improved accuracy of aviation data, integration of other data sources, improved monitoring of beacons, and synergies with other applications. These synergies could include, for example, more effective management of increasing air traffic and control of, for example, unmanned aerial vehicles (UAVs) based on detailed traffic data over lower airspace. Others are the identification of noise sources by matching air traffic data with sound pressure levels and the application of ADLS to other obstacles such as buildings. No impacts on generation, consumption, and grid controllability are identified.
Although the five normalized LCA results do not show significant impacts on the ecology category, the overall category reveals a positive result due to the anticipated positive impacts on enabling more renewable energies and protecting wildlife. The integration of more renewable energies could be positively influenced as approval processes could proceed more quickly due to a reduced number of arguments of lawsuits against new WTs, and neighbors could accept more turbines in their vicinity because they are perceived as less stressful. Although a positive impact on wildlife protection is expected by most experts, the standard deviation of responses is very high. On the one hand, the impact on wildlife could be reduced as nighttime light emissions are reduced. On the other hand, IR light could have a new impact, possibly on other species. Plants will most likely not be affected.
In the economy category, high profits and growth potential are seen for ADLS providers. After an initial spike during the rollout, long-term demand to equip new turbines and offer operational services is likely. Since Germany is the first country to introduce a nationwide ADLS obligation that also allows the use of transponder technology, there could be significant export potential, giving German providers a first-mover advantage. In addition, as mentioned, further growth potential exists through the application of the technology to other obstacles such as buildings, bridges, chimneys, etc. However, the standard deviation of responses regarding growth potential is high, indicating significantly divergent views. Furthermore, market entry barriers are estimated to be relatively high. The main obstacles for new suppliers are patents and the complex type examination certification procedure. For the user’s side, the profitability is evaluated slightly negatively, with a high standard deviation in responses. No significant impact on non-monetary user benefits, such as transparency, controllability, usability, etc., is found. Monitoring and control of beacons could be slightly improved, which would lead to more transparency.
The most positively evaluated category, society and politics, is primarily driven by the very strong positive evaluation of the impact of ADLS on social acceptance. Reduced lighting reduces lighting-related stress and thus increases acceptability. The acceptance evaluation shows a very low standard deviation and a maximum number of responses, indicating low uncertainty. In addition, positive effects on the value creation steps in Germany and on the number of jobs are also expected. New value creation steps for the production, installation, and opertion of ADLS will be implemented, creating some jobs in the medium to high qualification range. No significant impacts are seen on participation in the energy sector, labor conditions, dependence on other nations, or need for government support. However, the regulatory implementation effort is evaluated relatively strongly negatively. The effort required to create the necessary regulatory framework in advance of the ADLS obligation was quite high, several laws and regulations had to be amended. Some final procedural clarifications are still needed, in particular, a definition of who is authorized to test and certify the proper installation and operation of ADLS.
The potential impact of a failure is a cross-category set of criteria. These criteria are therefore analyzed jointly. The impact of a failure is a combination of its probability and its potential magnitude. The overall potential impact of a failure is found to be low. The probability of a failure, such as a collision between an aircraft and a turbine, is judged to be virtually zero. Systems must be designed with an engineered fallback option to ensure that beacons remain on if the system fails to operate properly. In addition, always-on IR lights will be added for pilots conducting low-level night flights without transponders, e.g., military and police. The technical and social/political impacts if a failure (collision) actually occurs are somewhat higher compared to the environmental and economic impacts. The technical impact of such a failure could, in the worst case, be the loss of the aircraft, the turbine, and even loss of life. Socially/politically, such a failure could lead to a reduction in the acceptance of wind energy in general.
While the weighting profile “national economy” was analyzed above, the MCA results can also be analyzed with weighting profiles representing the perspectives of the different stakeholders. The aggregated results for different weighting profiles are displayed in Figure 10. The evaluations with all weighting profiles show a positive total result. Overall, the result of the consumer perspective (B) is the most positive, which is mainly due to the high weighting of the society and politics category and in particular the social acceptance criterion. Wind farm operators (A), on the other hand, evaluate the application almost neutrally overall, which is mainly due to the high weighting of profitability for the user. The technology category is evaluated slightly negatively and very similarly across all weighting profiles. The ecology category is evaluated the most positively from the consumer perspective (B), even more positively than from the environmental NGO perspective (C). This is due to the high importance consumers place on enabling renewable energies, while environmental NGOs distribute the weighting more evenly across all ecology criteria. The evaluations for the economics category range from very slightly negative for wind farm operators (A) to positive from a political perspective (D). This evaluation range is based on the different prioritization of economic impacts on the provider side compared to the user side. All weighting profiles lead to a positive evaluation of the category society and politics. From a political perspective (D), not only positive impacts in the society and politics category but also economic benefits are expected. However, from a national economy perspective (E), these are less relevant.
5 Discussion
Following the structure of the result section, the LCA results are discussed first, followed by the MCA results, which integrate LCA and EI results. The section concludes with a discussion of the possible sources of error.
5.1 LCA result discussion
Based on the LCA results given in Figure 4, it is evident that the five considered impact criteria increase by the use of ADLS in the base case. The analysis of the breakdown of each of the impact criteria reveals the impact drivers and thus aspects with potentially high improvement levers. ADLS hardware energy consumption emerges as one of the most important impact drivers for CED, GWP, ARD, and ET. This underscores the importance of paying attention to energy-efficient design in engineering development. The impact of GWP and ARD can be further reduced by decreasing the CO2 intensity of the energy mix used for production. To reduce the HT and ET impact, the design of the hardware needs to be analyzed regarding the presence and possible substitution of certain materials such as copper, gold, silver, brass, and other precious metals. The finding that GWP and ARD are driven by the CO2 intensity of the energy mix, while HT and ET are driven by the underlying infrastructure, is consistent with other LCAs on energy systems, e.g., (Baumgärtner et al., 2021). Recycling, as modeled in the presented study, results in only a small reduction in all impact criteria (compared to the production impacts). However, an improved recycling process could improve the overall result.
Given the results shown in Figure 5 and Figure 6, it is evident that the ADLS life cycle impacts depend heavily on the number of turbines covered by one ADLS and the number of communication units required per ADLS. Although it may be difficult to influence the layout for existing wind farms, both of these issues can be considered for newly built WTs. Based on the experience from the ADLS planning in North Rhine-Westphalia, the realistic range of onshore turbines covered by one ADLS is between 1 and 33. Therefore, in some cases, the ADLS may lead to an impact reduction of some or all of the analyzed ecological criteria, at least if only one communication unit is required. Since offshore wind farms typically consist of significantly more turbines (currently 12–80 turbines per operational German offshore wind farm) with a central communication unit and are less likely to be located in high air traffic zones, a decreased life cycle impact of using ADLS can be expected here.
5.2 MCA result discussion
The MCA provides a holistic overview of all relevant criteria from relevant stakeholders’ perspectives. The main objective of the ADLS obligation, to increase social acceptance of wind energy, is likely to be achieved. The evaluation from the consumers’ point of view is the most positive, with the highest evaluation in the society and politics category. No fundamental opposition to the obligation needs to be expected, as all stakeholders come to an overall positive evaluation. The stakeholders most affected by the obligation, the wind farm operators, are the ones with the least positive evaluation. Compensation, i.e., a lower regulatory burden, could improve their perception of the application. From a political perspective, not only the positive impact on the society but also on the economy is relevant. Furthermore, it is evident that due to the identified bottlenecks for the rollout (global scarcity of semiconductors and lack of technicians in Germany) and the regulatory process clarifications still needed, the feasibility of a full rollout by the end of 2022 is questionable. The authors recommend a limited extension of the rollout period until these challenges are likely to be mitigated to an acceptable degree. With a very similar argumentation, the German authorities intend to postpone the deadline by 1 year, according to the first draft of a future version of the German Renewable Energy Law (BMWK, 2022).
Such a limited extension of the rollout period would also allow for analyzing the pre- and post-implementation situation of two important aspects, which still require investigation:
1) No studies have yet been conducted on the effects of ADLS on wildlife. Therefore, the uncertainty of expert responses for this criterion is very high (reflected in the high standard deviation shown in Figure 9). Further studies are recommended, e.g., by monitoring finds of dead birds in the area.
2) Several studies demonstrate the relevance of WT lighting on stress and acceptance. Even though lighting is a less relevant cause of stress than noise and shadows flicker, it is visible in a larger surrounding and thus affects more people (Rudolph et al., 2017). However, no study has been conducted that directly measured the change in acceptance in the vicinity of a wind farm before and after the implementation of an ADLS. Therefore, such a study is recommended.
5.3 Validity of the results
The evaluation shown here is valid for currently installed ADLS. It is assumed that changing conditions within a short time frame of a few years will not significantly change the evaluation, but changes occurring after more than a decade might do so. Although LCA results, particularly the GWP and ARD impact, might become more positive due to reductions in GHG intensity of the energy used and advances in recycling, the LCA impact as part of the MCA will still be insignificant. However, as the size and number of WTs increase, more people might be affected by lighting-related stress without ADLS. Therefore, it is likely that more and more countries will approve the application of transponder-based ADLS, which will increase the market size and growth potential. At the same time, this will lead to more international competition, which will likely reduce profitability for the first movers.
Furthermore, a critical look at potential sources of error and uncertainty is needed. Some sources of error are inherent to the design and methodology of the framework, while others depend on the evaluation practitioner, the availability of data, and the functionality of the application being evaluated. The most important source of error in MCA is the selection of evaluation criteria. If the criteria do not cover the relevant impacts, the evaluation cannot produce a meaningful result. Therefore, considerable effort has been put into the development of the criteria, which have been updated for this study based on a previous study (Weigel et al., 2021). Furthermore, the MCA and weighting methods may have an impact on the result. The simple additive weighting method (SAW, also known as the weighted sum method), which is commonly applied due to its popularity and simplicity (Cajot et al., 2017), makes it intuitive for decision makers and thereby reduces the probability of user error. Furthermore, the aggregation method allows for a high level of transparency on how aggregated results are derived from criteria evaluations (Wilkens, 2012). The downside of the simplicity is that the compensatory aggregation might reduce the clarity of specific effects for complex problems (Marler and Arora, 2010), (Chu et al., 2007). This disadvantage is mitigated in this study by discussing results on aggregated levels as well as on the detailed criteria level. In (Terrapon-Pfaff, 2014) and (Daugavietis et al., 2022) the authors compare results obtained with different methods, including SAW, and conclude that results using the SAW method do not differ significantly from the results obtained with more complex methods. Furthermore, the SAW method is suitable to be applied with fuzzy numbers for uncertain input data such as subjective expert evaluations. The use of fuzzy sets is described in (Greco et al., 2016, 637), and an application is presented, for example, in (Ziemba, 2021). The integration of fuzzy SAW presents a future improvement possibility for the evaluation framework.
Further uncertainties may arise from the criteria assessment in the LCA and the EIs. The accuracy of the LCA result depends largely on the life cycle model created, including system boundaries, assumptions, input data, databases used, and the inclusion or exclusion of effects. A structured approach to assessing LCA data quality is the pedigree matrix (Weidema, 1998). Since the quality of the data used in this study varies significantly by data point, an overall evaluation of data quality according to the pedigree matrix does not appear feasible. Therefore, rather than assessing the quality of the input information, the effect of uncertainties on the results is estimated using sensitivity analysis. In this study, the sensitivity of the results is shown regarding several parameters, namely the number of turbines per ADLS, the number of communication units per ADLS, and the beacon reduction rate. No other parameters are identified as having a significant impact on the ADLS result. It should be noted that some ADLS use cloud data management, but the required data transfer and server operation are not included in the LCA due to a lack of information (Malmodin et al., 2014). find that the end-user applications cause a significantly higher GHG impact than the data transfer and servers. Therefore, it is unlikely that including both aspects would drastically change the outcome. Yet, a more accurate assessment could increase certainty. Furthermore, the LCA impact method used may have an influence on the results. Within the limited options of methods which include the required impact criteria (CML2001 and ReCiPe) the (midpoint) methods provide largely consistent results for the analyzed impacts (Bueno et al., 2016).
The choice of the reference for normalizing the LCA might have the greatest impact on how the LCA result affects the MCA result. In this study, the life cycle impact of the WTs, which are covered by the ADLS, is used as the reference. The WTs’ life cycle impacts assessed with the Ecoinvent database (data source from 2001) result in a GHG intensity of 17.6 g CO2 eq./kWh, while (Hengstler et al., 2021) finds a lower intensity of 10.6 g CO2 eq./kWh for modern onshore low wind turbines. However, even a very large deviation of ±50% from the reference values would not significantly change the impact of the LCA on the MCA outcome.
The EIs are mainly influenced by the selection of experts, i.e., the number of experts, the area and level of their expertise, and their self-perception of knowledgeability to evaluate criteria. Unless a large number of interviews are conducted, which would require a great effort, the interview results cannot be considered representative. Nevertheless, expert knowledge can provide very valuable insights into the subject. Therefore, even a smaller number of experts is acceptable as long as their expertise covers the perspectives of the relevant stakeholders. A practical approach used in this study to ensure that the relevant stakeholder representatives are included is to ask each expert to identify relevant stakeholders. It is highly unlikely that a relevant stakeholder role would not be identified by any of the 12 experts interviewed, so good coverage of the relevant perspectives can be expected. However, as mentioned, no affected neighbors of WTs are directly included in the EIs of this study, but the insights of the two scientists researching the social acceptance of WTs is seen as a good proxy for the perspective of this group. The level of expertise of the experts can be difficult to assess for a non-expert, but information about professional positions, publications, or involvements in reputable organizations can be used as quality control. In addition, each expert is explicitly advised to evaluate only criteria regarding which he or she feels sufficiently knowledgeable. Thus, it can be expected that most answers are based on sound expertise and that different answers are mainly an indication of uncertainty due to the range of possible impacts. As an improvement of the framework in this study, the standard deviation of the EI results is used in combination with the number of responses as an indicator of uncertainty, which revealed high uncertainties in particular for the criteria innovation potential, growth potential, economic barriers, availability of materials and know-how and regulatory implementation effort. Last but not least, the EI result may also be influenced by the interviewer during the interview. Here, a minimal intervention approach was applied, i.e., besides an initial introduction and some necessary criteria clarifications, the interviewer only passively documented the experts’ evaluation.
5.4 Suitability of the framework
Since, in this study, an adapted version of the previously developed framework is applied, the adapted version’s suitability is reviewed. The intended characteristics of the framework are that meaningful and useful results can be obtained, that it is easily usable for researchers and representatives of companies and organizations, and that it can be applied to the variety of current and future digital applications. Therefore, the suitability should be evaluated based on the conclusiveness of results, the feasibility of use, and the adaptability of the framework. The conclusiveness of the results includes both correctness and potential for deriving action. Feasibility of use is based on the effort required for each evaluation as well as the inherent complexity and thus the level of expertise required by the practitioner. Adaptability of the framework is required regarding different types of digital applications, the level of data availability, and practitioners’ preferences. These suitability criteria are specific to the evaluation of this framework and can only be evaluated qualitatively.
The correctness of the results is difficult to assess due to the lack of other studies on ADLS. However, based on the possible comparisons of specific aspects with other publications and the relatively high consistency of expert opinions, the results are likely to be very realistic. Sources for uncertainties are identified and, where possible, analyzed via sensitivity analysis or statistical means. Furthermore, the potential to derive actions is high as direct measures and recommendations for further studies, life cycle improvement initiatives, as well as regulatory adjustments are identified. The overall conclusiveness of the result is therefore considered to be high.
The effort required to collect the necessary data for the LCA and to conduct the twelve expert interviews is relatively high. However, the framework improvement implemented in this study to let experts independently conduct the weighting after the interview decreased the interview effort and time requirement for the research team significantly. Due to the methods chosen, the complexity of the MCA and the EIs is rather low, such that this part of the framework can also be carried out by practitioners without a deep theoretical understanding of the methodology. The LCA, however, requires in-depth expertise. Therefore, the feasibility of use is evaluated as medium. This drawback could be mitigated if existing life cycle results could be integrated instead of conducting a separate LCA. In addition, the effort could be further reduced by decreasing the number of expert interviews. However, this could affect the correctness of the result.
The adaptability of the framework regarding different types of applications can be assessed by looking at the difference between the application evaluated in this study and the smart meter rollout evaluated in (Weigel et al., 2021). The two applications differ greatly in terms of the energy value stream step in which they are deployed, their function, and their effects. Furthermore, in this study, a single application is evaluated, while in (Weigel et al., 2021), a nationwide rollout of an application is evaluated. The adaptability regarding the type of application is therefore considered to be very high. The availability of information differs considerably between criteria, e.g., there are several studies on the social acceptance of ADLS, but none on the impact on wildlife. Nevertheless, all criteria can be evaluated in the expert interviews and discussed, including the standard deviation and the number of responses as a measure of the robustness of the result. This demonstrates the very high adaptability of the framework to different levels of data availability. Finally, the adaptability to practitioners’ preferences can only be evaluated once the framework has been applied by different practitioners, which is not the case at this stage. Therefore, the overall adaptability is considered to be very high, but the unevaluated adaptability to practitioners’ preferences has to be taken into account.
Considering the high conclusiveness of the results, the medium feasibility of use, and the very high adaptability, it is concluded that the evaluation framework is well suited for its purpose.
6 Conclusion and outlook
In this study, an updated version of a holistic evaluation framework previously developed by the authors was applied to evaluate the application of aircraft detection lighting systems for wind turbines. The framework is specifically designed for the holistic evaluation of digital applications in the energy sector. To achieve a holistic view, multiple criteria covering all relevant impact areas were evaluated, relevant stakeholders’ perspectives were considered as weighting profiles, and representatives of relevant stakeholders were involved in the process. A life cycle assessment was performed to assess several environmental criteria. The required data and information were provided by both an ADLS and a beacon manufacturer. Furthermore, twelve expert interviews were conducted to assess all other criteria that were not part of the LCA. The experts also weighted the criteria, and five weighting profiles were created. The study presents the first holistic evaluation of ADLS, in contrast to previous studies, which focus on single evaluation aspects such as aviation risks or social acceptance as well as a refined version of the evaluation framework.
The results of the LCA show a likely increase in the life cycle impact of all analyzed criteria if a realistic design of the system is assumed. The magnitude of the increase depends mainly on the number of WTs covered per ADLS and the need to install additional communication units. Due to the size of offshore wind farms, the ADLS could lead to a reduction in life cycle impacts here. The LCA results of this study can be used by ADLS manufacturers as a starting point for life cycle improvement activities. However, in the context of the life cycle impacts of the turbines covered by the ADLS, the impact of the ADLS is negligible, whether it is increased or decreased. The MCA based on LCA and EI results shows an overall slightly positive evaluation from all stakeholders’ perspectives. Therefore, a rollout is expected to be beneficial. The most significant benefits are seen in the increased social acceptance of wind turbines as well as the economic (international) growth potential for the providers of the technology and the resulting impact on the national economy. Two further studies are recommended with respect to 1) wildlife impacts to ensure that potential adverse impacts are identified and addressed and 2) social acceptance impacts to validate and measure wind energy acceptance before and after installing ADLS. Three main bottlenecks for the rollout were identified: the shortage of global semiconductor supply needed for production, the lack of trained technicians for installation, and remaining regulatory uncertainties regarding the approval process. Given these bottlenecks, an extension of the rollout period is recommended. The remaining time until the obligation becomes effective should be used by decision-makers to address the identified bottlenecks. Political decision-makers should drive the administrative process to eliminate the regulatory uncertainties. The issues of global semiconductor scarcity and lack of technicians in Germany go well beyond affecting only ADLS but hinder major developments, such as the transition towards renewable energies, and therefore, need to be counteracted on a broader economic-political level by, e.g., researching material substitutions, investing in new production capacities and supporting continuing professional development. However, smaller measures to mitigate the impact of these bottlenecks on the ADLS rollout can be taken by business decision-makers. For example, the pooling of ADLS installations for an entire region, as done by the association for renewable energies in the state of North Rhine-Westphalia, could improve the plannability for both ADLS manufacturers and installers. Furthermore, the implementation of ADLS in other countries could benefit from an early holistic evaluation using the presented framework.
Potential sources of uncertainty were identified, and, where possible, sensitivity analyses were performed. Given the limitations and uncertainties, the study provides a robust evaluation result with an aggregated overview and valuable insights into bottlenecks and potential for improvements at the criteria level.
The suitability of the updated framework was assessed based on three criteria: conclusiveness of results, feasibility of use, and adaptability of the framework. Overall, the framework was found to be highly suitable for its purpose. Two measures are suggested to further improve the feasibility of use. In addition, the possibility of applying fuzzy sets for the SAW aggregation method was pointed out to improve the handling of uncertainties.
A prospective future direction of the research might be for the framework to be applied to different digital applications in the energy sector by different practitioners. Additionally, the framework could be adapted for the evaluation of digital applications in other sectors. With increasing numbers of performed evaluations, the comparability of results becomes increasingly interesting and should be analyzed. The proposed integration of fuzzy logic may improve the comparability of applications with varying uncertainties. Another interesting future aspect could be the retrospective analysis of previous evaluations regarding the accuracy of results and conclusions.
Data availability statement
The datasets presented in this article are not readily available because due to confidentiality restrictions of the information supplying company, data on the technical setup of the application can only be shared on an aggregated level upon request to the corresponding author. Raw and anonymized expert interview results can be shared upon request. Requests to access the datasets should be directed to pweigel@uni-osnabrueck.de.
Ethics statement
Ethical review and approval was not required for the study on human participants in accordance with the local legislation and institutional requirements. Written informed consent from the (patients/participants OR patients/participants legal guardian/next of kin) was not required to participate in this study in accordance with the national legislation and the institutional requirements.
Author contributions
All authors contributed to the conception and design of the study. PW gathered and analyzed the data and conducted the assessments. The manuscript was prepared by PW, with frequent input and revisions from PV. All authors carefully read and approved the submitted manuscript.
Conflict of interest
The authors declare that the research was conducted in the absence of any commercial or financial relationships that could be construed as a potential conflict of interest.
Publisher’s note
All claims expressed in this article are solely those of the authors and do not necessarily represent those of their affiliated organizations, or those of the publisher, the editors and the reviewers. Any product that may be evaluated in this article, or claim that may be made by its manufacturer, is not guaranteed or endorsed by the publisher.
Acknowledgments
We acknowledge financial support by Wuppertal Institut für Klima, Umwelt, Energie gGmbH within the funding programme Open Access Publishing.
References
Baumgärtner, N., Deutz, S., Reinert, C., Nolzen, N., Kuepper, L. E., Hennen, M., et al. (2021). Life-cycle assessment of sector-coupled national energy systems: Environmental impacts of electricity, heat, and transportation in Germany till 2050. Front. Energy Res. 9. Available at: https://www.frontiersin.org/article/10.3389/fenrg.2021.621502 (Accessed April 26, 2022).
Behrend, F. (2019). Identifizierung und Bewertung der durch die Einführung der transponderbasierten bedarfsgesteuerten Nachtkennzeichnung (BNK) entstehenden flugbetrieblichen Risiken und Beschreibung von Risikominimierungsmaßnahmen. Rosengarten, Germany: German Federal Ministry for Economic Affairs and Energy (BMWi).
BMDV (2020). Allgemeine Verwaltungsvorschrift zur Kennzeichnung von Luftfahrthindernissen. Offenbach, Germany: German Federal Ministry for Digital and Transport (BMDV).
BMDV (2018). Verordnung über die Flugsicherungsausrüstung der Luftfahrzeuge. Berlin, Germany: German Federal Ministry for Digital and Transport (BMDV).
BMDV (2022). Wetter und Klima - deutscher Wetterdienst - EDDE Erfurt. Berlin, Germany: German Federal Ministry for Digital and Transport. Available at: https://www.dwd.de/DE/fachnutzer/luftfahrt/teaser/luftsportberichte/edde/node.html (Accessed May 2, 2022).
BMWK (2022). Entwurf eines Gesetzes zu Sofortmaßnahmen für einen beschleunigten Ausbau der erneuerbaren Energien und weiteren Maßnahmen im Stromsektor. Berlin, Germany: German Federal Ministry for Economic Affairs and Climate Action. Available at: https://www.bmwk.de/Redaktion/DE/Artikel/Service/Gesetzesvorhaben/referentenentwurf-erneuerbaren-energien-und-weiteren-massnahmen-im-stromsektor.html (Accessed April 27, 2022).
BNK (2022). Saar GmbH BNK saar. Available at: https://bnk-saar.de/(Accessed May 25, 2022).
Bode, M., and Klümper, L. (2021). Das BNK Gemeinschaftsprojekt für NRW. Düsseldorf, Germany: Association for renewable energies in the state of North Rhine-Westphalia (LEE NRW GmbH).
Bueno, C., Hauschild, M. Z., Rossignolo, J. A., Ometto, A. R., and Mendes, N. C. (2016). Sensitivity analysis of the use of life cycle impact assessment methods: A case study on building materials. J. Clean. Prod. 112, 2208–2220. doi:10.1016/j.jclepro.2015.10.006
Cajot, S., Mirakyan, A., Koch, A., and Maréchal, F. (2017). Multicriteria decisions in urban energy system planning: A review. Front. Energy Res. 5, 10. doi:10.3389/fenrg.2017.00010
Chu, M.-T., Shyu, J., Tzeng, G.-H., and Khosla, R. (2007). Comparison among three analytical methods for knowledge communities group-decision analysis. Expert Syst. Appl. 33, 1011–1024. doi:10.1016/j.eswa.2006.08.026
D’Adamo, I., Gastaldi, M., and Morone, P. (2022). Economic sustainable development goals: Assessments and perspectives in Europe. J. Clean. Prod. 354, 131730. doi:10.1016/j.jclepro.2022.131730
Daugavietis, J. E., Soloha, R., Dace, E., and Ziemele, J. (2022). A comparison of multi-criteria decision analysis methods for sustainability assessment of district heating systems. Energies 15, 2411. doi:10.3390/en15072411
Deutsche WindGuard GmbH (2021). Status des Offshore-Windenergieausbaus in Deutschland. Varel, Germany: Deutsche WindGuarad GmbH.
Ellis, G., and Ferraro, G. (2016). The social acceptance of wind energy. LU: European commission. Joint research centre. Available at: https://data.europa.eu/doi/10.2789/696070 (Accessed April 28, 2022).
Gährs, S., Bluhm, H., Dunkelberg, E., Katner, J., Weiß, J., Henning, P., et al. (2021). Potenziale der Digitalisierung für die Minderung von Treibhausgasemissionen im Energiebereich. Umweltbundesamt. Available at: https://www.umweltbundesamt.de/publikationen/potenziale-der-digitalisierung-fuer-die-minderung (Accessed April 26, 2022).
German Corporation for International Cooperation GmbH (GIZ) (2022). Extractive industries transparency initiative - report 2019 for Germany. Available at: https://d-eiti.de/en/(Accessed May 4, 2022).
German Federal Ministry for Economic Affairs and Energy (2021). Gesetz für den Ausbau erneuerbarer Energien. Berlin, Germany: German Federal Ministry for Economic Affairs and Energy (BMWi).
S. Greco, M. Ehrgott, and J. R. Figueira (Editors) (2016). Multiple criteria decision analysis (New York, NY: Springer New York). doi:10.1007/978-1-4939-3094-4
Hengstler, J., Russ, M., Stoffregen, A., Hendrich, A., Weidner, S., Held, M., et al. (2021). Aktualisierung und Bewertung der Ökobilanzen von Windenergie- und Photovoltaikanlagen unter Berücksichtigung aktueller Technologieentwicklungen. Umweltbundesamt. Available at: https://www.umweltbundesamt.de/publikationen/aktualisierung-bewertung-der-oekobilanzen-von (Accessed April 28, 2022).
Hübner, G., and Pohl, J. (2010). Akzeptanz und Umweltverträglichkeit der Hinderniskennzeichnung von Windenergieanlagen. Halle (Saale): Martin-Luther-Universität Halle–Wittenberg Institut für Psychologie.
Hübner, G., Pohl, J., Hoen, B., Firestone, J., Rand, J., Elliott, D., et al. (2019). Monitoring annoyance and stress effects of wind turbines on nearby residents: A comparison of U.S. And European samples. Environ. Int. 132, 105090. doi:10.1016/j.envint.2019.105090
ICAO (2018). Aerodromes: International standards and recommended practices. Int. Civ. Aviat. Organ. Available at: https://store.icao.int/en/annex-14-aerodromes (Accessed March 22, 2022).
Kluczek, A., and Gladysz, B. (2022). Frontiers in energy research 10. Available at: https://www.frontiersin.org/article/10.3389/fenrg.2022.848584 (Accessed April 26, 2022).Energy sustainability performance index of biodigester using energy LCA-based indicators
Malmodin, J., Lundén, D., Moberg, Å., Andersson, G., and Nilsson, M. (2014). Life cycle assessment of ICT. J. Industrial Ecol. 18, 829–845. doi:10.1111/jiec.12145
Marler, R. T., and Arora, J. S. (2010). The weighted sum method for multi-objective optimization: New insights. Struct. Multidiscipl. Optim. 41, 853–862. doi:10.1007/s00158-009-0460-7
Naegler, T., Becker, L., Buchgeister, J., Hauser, W., Hottenroth, H., Junne, T., et al. (2021). Integrated multidimensional sustainability assessment of energy system transformation pathways. Sustainability 13, 5217. doi:10.3390/su13095217
Pohl, J., Hübner, G., and Mohs, A. (2012). Acceptance and stress effects of aircraft obstruction markings of wind turbines. Energy Policy 50, 592–600. doi:10.1016/j.enpol.2012.07.062
Pohl, J., Rudolph, D., Lyhne, I., Clausen, N.-E., Aaen, S. B., Hübner, G., et al. (2021). Annoyance of residents induced by wind turbine obstruction lights: A cross-country comparison of impact factors. Energy Policy 156, 112437. doi:10.1016/j.enpol.2021.112437
Rudolph, D., Kirkegaard, J., Lyhne, I., Clausen, N.-E., and Kørnøv, L. (2017). Spoiled darkness? Sense of place and annoyance over obstruction lights from the world’s largest wind turbine test centre in Denmark. Energy Res. Soc. Sci. 25, 80–90. doi:10.1016/j.erss.2016.12.024
Sun, J., Jin, H., Tsai, F.-S., and Jakovljevic, M. (2022). A global assessment of sustainable development: Integrating socioeconomic, resource and environmental dimensions. Front. Energy Res. 10, 816714. doi:10.3389/fenrg.2022.816714
Terrapon-Pfaff, J. (2014). Nachhaltige Bioenergieoptionen für Afrika: energetische Nutzung von Agrarreststoffen als Teil einer Transformationsstrategie hin zur nachhaltigeren Energieversorgung in Tansania: Potentialanalyse und multikriterielle Bewertung. Hamburg: Dr. Kovač GmbH.
Weidema, B. P. (1998). Multi-user test of the data quality matrix for product life cycle inventory data. Int. J. Life Cycle Assess. 3, 259–265. doi:10.1007/BF02979832
Weigel, P., Fischedick, M., and Viebahn, P. (2021). Holistic evaluation of digital applications in the energy sector—evaluation framework development and application to the use case smart meter roll-out. Sustainability 13, 6834. doi:10.3390/su13126834
Wilkens, I. (2012). Multikriterielle Analyse zur Nachhaltigkeitsbewertung von Energiesystemen - von der Theorie zur praktischen Anwendung. Available at: https://depositonce.tu-berlin.de//handle/11303/3682 (Accessed May 29, 2018).
Zhang, S., Ma, X., and Cui, Q. (2021). Assessing the impact of the digital economy on green total factor energy efficiency in the post-COVID-19 era. Front. Energy Res. 9, 798922. doi:10.3389/fenrg.2021.798922
Ziemba, P. (2021). Selection of electric vehicles for the needs of sustainable transport under conditions of uncertainty—a comparative study on fuzzy MCDA methods. Energies 14, 7786. doi:10.3390/en14227786
Appendix: Relevant assumptions for the LCA of the ADLS
Keywords: digitalization, cyber-physical systems, wind energy, sustainability, holistic evaluation, multi-criteria analysis, life cycle assessment, aircraft detection lighting systems
Citation: Weigel P, Viebahn P and Fischedick M (2022) Holistic evaluation of aircraft detection lighting systems for wind turbines in Germany using a multi-method evaluation framework. Front. Energy Res. 10:984003. doi: 10.3389/fenrg.2022.984003
Received: 01 July 2022; Accepted: 22 August 2022;
Published: 15 September 2022.
Edited by:
Nallapaneni Manoj Kumar, City University of Hong Kong, Hong Kong SAR, ChinaReviewed by:
Idiano D'Adamo, Sapienza University of Rome, ItalyUmar Muhammad Modibbo, Modibbo Adama University of Technology, Nigeria
Sandhya Thotakura, Gandhi Institute of Technology and Management (GITAM), India
Copyright © 2022 Weigel, Viebahn and Fischedick. This is an open-access article distributed under the terms of the Creative Commons Attribution License (CC BY). The use, distribution or reproduction in other forums is permitted, provided the original author(s) and the copyright owner(s) are credited and that the original publication in this journal is cited, in accordance with accepted academic practice. No use, distribution or reproduction is permitted which does not comply with these terms.
*Correspondence: Paul Weigel, pweigel@uni-osnabrueck.de