- 1MIIT Key Laboratory of Thermal Control of Electronic Equipment, School of Energy and Power Engineering, Nanjing University of Science and Technology, Nanjing, China
- 2Advanced Combustion Laboratory, School of Energy and Power Engineering, Nanjing University of Science and Technology, Nanjing, China
The effects of the upward-increasing gradient magnetic field on soot properties are experimentally investigated in ethylene inverse diffusion flames with different oxygen concentrations. The soot morphology, nanostructure, graphitization degree, and oxidation reactivity are obtained by high-resolution transmission electron spectroscopy (HRTEM), X-ray diffractometer (XRD), and thermogravimetric analyzer (TGA), respectively. The upward-increasing gradient magnetic field is induced by two Nd-Fe-B permanent magnets with different thicknesses. The results show that the magnetic field influences the soot properties mainly by affecting the distributions of paramagnetic O2 and OH radicals in the flames. The soot samples are more graphitized in the flame with higher O2 concentration, which contains a longer fringe length and smaller fringe tortuosity. Fullerene-like structures are more apparent with increasing oxygen content. The soot fringe length decreases and fringe tortuosity increases when the upward-increasing gradient magnetic field is applied. The application of the magnetic field enhances the soot oxidation reactivity, and it shows the greatest effect on the oxidation reactivity of soot produced in the flame with 21% O2 content.
Introduction
With the rapid growth of the world’s population and industrialization, global energy demand continues to grow in the coming decades. Large-scale development and use of fossil energy will do great harm to the climate and environment. In addition to greenhouse gases, there are hazardous pollutants such as nitrogen oxides, unburned hydrocarbons, soot, etc., (Wang and Chung, 2019; Li et al., 2021; Falco et al., 2022). Soot, emitted from the pyrolysis and incomplete burning of hydrocarbon fuels, may cause cardiac arrest, asthma, and pulmonary diseases (Arif et al., 2018; Ali et al., 2021). Recent studies have shown that the transmission rate and incidence of novel coronavirus pneumonia (COVID-19) are closely related to local atmospheric particulate matter concentration (Mehmood et al., 2020; Chakrabarty et al., 2021).
The physical factors affecting soot formation mainly include temperature, pressure, magnetic field, etc. A detailed study of the physical parameters contributes to a better design of the burner structure and reduces the generation and emission of soot. The utilization of a magnetic field is one of the potential strategies for combustion control (Agarwal et al., 2014; Agarwal et al., 2015). The magnetic field can affect the flame temperature, flame structure, and free radical distribution, which has been widely studied (Takashi, 1990; Gilard et al., 2008). During the combustion process, charged ions or ion clusters are generated, and the applied magnetic field produces the Lorentz force, which changes the direction of motion of the charged particles, thereby affecting the chemical reaction rate caused by the concentration change. In addition to the Lorentz force for ionic species, the non-conductive and paramagnetic substances are subjected to the magnetic force, which contributes to a significant effect on chemical reactions in flames (Shinoda, 2005).
In recent years, researchers have conducted studies on the change of gradient magnetic field on the combustion characteristics of gas fuel. The magnetic force increases the concentration of OH radicals toward the flame center, which is attributed to the effect of the magnetic field on paramagnetic O2 (Yamada et al., 2002; Yamada et al., 2003a). The paramagnetic force does not directly affect OH radicals, instead, the paramagnetic force acts on O2 with higher magnetic susceptibility and density, leading to the convective motion of O2 and changing the distribution of OH radicals. The combustion characteristics of butane diffusion flames are investigated under different kinds of magnetic fields, and it is observed that the flame temperature increases in an upward-decreasing gradient of magnetic field and decreases with an upward-increasing magnetic field gradient (Aoki, 1990; Kumar et al., 2015). Experimental investigations are conducted to assess the effects of the gradient of the square of the magnetic flux density (∇(B2)) on the sooting tendency in non-premixed flames (Jocher et al., 2015) and partially premixed flames (Jocher et al., 2017). In non-premixed flames, increasing the magnetic gradient promotes soot production, while the upward (∇(B2)) contributes to a reduction of soot for some partially premixed flames. Moreover, the magnetic field enhances the soot production by extending the residence time in flames with different oxidizer mixtures and increases the heat radiation of soot (Jocher et al., 2019).
It can be found that the application of a gradient magnetic field can affect the flame temperature, flame structure, free radical distributions, and sooting tendency. The influence of magnetic field on flame characteristics has been studied, but the effects of gradient magnetic field on the soot properties needs further investigation. This study aims to analyze the effects of the upward-increasing gradient magnetic field on soot properties in inverse diffusion ethylene flames with different oxygen concentrations. The soot properties including morphology, nanostructure, graphitization degree, and oxidation activity of the soot are obtained by high-resolution transmission electron spectroscopy (HRTEM), X-ray diffractometer (XRD), and thermogravimetric analyzer (TGA), respectively.
Experimental
An inverse diffusion flame burner is used in this study as in the previous work (Ying and Liu, 2017; Ying and Liu, 2021). The upward-increasing gradient magnetic field is induced by two Nd-Fe-B permanent magnets with different thicknesses, where the specific dimensions are × 50 mm × 50 mm × 10 mm and 50 mm × 50 mm × 30 mm. The Nd-Fe-B permanent magnet is placed above the cooling plate as Figure 1A shows. The quartz glass plate under the cooling plate is 40 mm away from the burner outlet. The magnetic field distributions of the two magnets are measured by taking the center of the magnet as the base point and arranging a measurement point every 6.25 mm along with the horizontal and vertical directions. The yellow circles in Figure 1B present the test positions. The magnetic field distribution is measured along the burner axis in units of 5 mm to ensure that the measurement area can completely cover the flame. The magnetic field gradient increases with the increasing height along the flame axis. For the magnet with a size of × 50 mm × 50 mm × 30 mm, the average magnetic field strength at 40 mm from the burner is 248.67 and 37.65 mT at the burner surface. While for the magnet with a size of × 50 mm × 50 mm × 10 mm, the average magnetic field strength at the same positions is 119.07 and 16.78 mT, respectively. The detailed magnetic field gradient distributions are shown in Figures 1C,D.
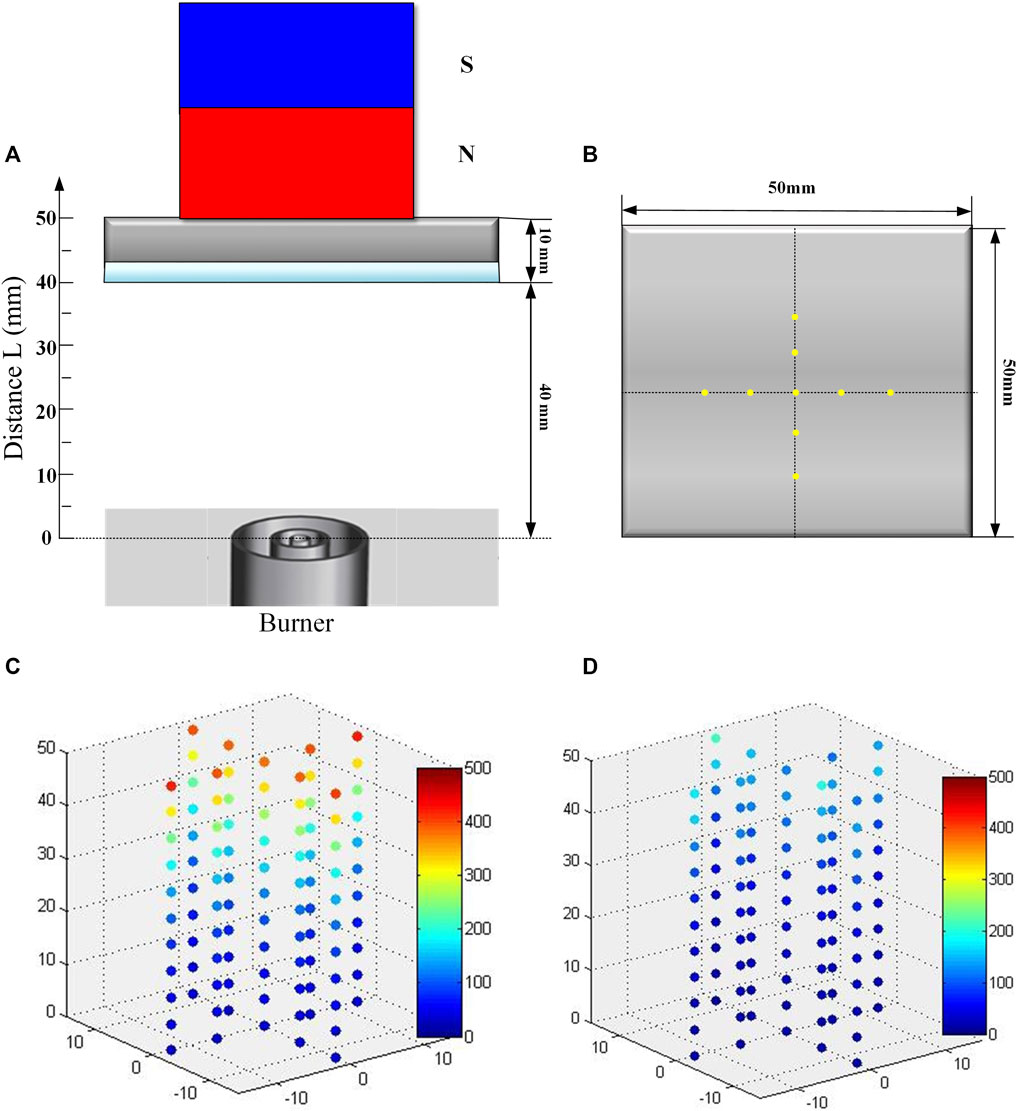
FIGURE 1. (A) Magnet arrangement, (B) test location of magnetic field, and spatial gradient distribution of (C) × 50 mm × 50 mm ×30 mm, (D) 50 mm × 50 mm × 10 mm magnet.
The gas molecules and activated groups in the flame can be divided into paramagnetic substances and diamagnetic substances, especially the two substances O2 and OH radicals. The ratio of O2 on the oxidative side is set to 21, 26, and 31% to study the effect of oxygen concentration on soot formation under a magnetic field. The flames with three different oxygen concentrations are abbreviated as F1, F2, and F3, respectively, and the following numbers indicate the thickness of the permanent magnet. The detailed flame conditions are shown in Table 1.
The soot is collected by quartz plate sampling method in the post-flame area at HAB = 40 mm for 15 min. The soot mass is weighted for each condition at least three times. The characterization methods and settings are the same as the previous studies (Ying et al., 2017; Ying and Liu, 2018). Briefly, the soot morphology and nanostructure are observed by a Tecnai G2 F30 HRTEM. To obtain the soot fringe characteristic parameters, a homemade MATLAB procedure is used (Ying and Liu, 2017). A Bruker D8 Advance XRD is applied to assess the graphitization degree of soot, and the soot oxidation reactivity is tested by a Netzsch STA449 F3 Jupiter TGA.
Results and discussion
O2 concentration distribution
The magnetically induced variation in the spatial distribution of paramagnetic O2 is measured at 21% oxygen content as a representative. The gas is assessed on the centerline and boundary of the flame at the height above the burner HAB = 15, 25, and 35 mm by an online detection system. The O2 concentration increases along with the flame centerline with the upward-increasing gradient magnetic field, especially at HAB = 15 mm, the oxygen content is significantly higher than that of the flame without the magnetic field. The gradient magnetic field causes the O2 concentration to drop significantly along the flame boundary. The change in O2 concentration along the flame centerline and boundary at different flame conditions are shown in Supplementary Figure S1. It is found that in the upward-increasing gradient magnetic field, O2 moves toward the flame center due to its paramagnetic susceptibility. Therefore, it can be inferred that paramagnetic OH radicals also shift to the flame center with the magnetic field.
Soot mass
Table 2 lists the soot mass in the flames collected by the quartz plate at HAB = 40 mm. The flame with a 21% O2 concentration and no magnetic field has the lowest soot production of 9.2 mg, while the flame with an oxygen concentration of 31% under the influence of a strong magnetic field produces the highest soot mass, 29.5 mg. It is found that increasing the oxygen concentration leads to increased soot formation. Under the same O2 concentration conditions, the soot mass also increases with the increasing magnetic field. This may be because the fuel generates charged particles during the pyrolysis process and is in the form of ions or ion clusters. When a certain magnetic field is applied, these ions or ion clusters are affected by the Lorentz force and changed from the original disordered Brownian diffusion motion to an ordered upward motion (Yamada et al., 2003b). As a result, the enhanced combustion increases the maximum flame temperature and promotes soot formation. After HAB = 10 mm, the soot mass increase is dominated by the agglomeration between soot particles.
Soot morphology
Figure 2 shows the typical TEM images of soot under different flame conditions. The soot particles generated by the ethylene inverse diffusion flame with a 21% O2 concentration have irregular liquid-like protrusions, which are mainly caused by the condensation of soot precursors like polycyclic aromatic hydrocarbons (PAHs) on the surface of solid particles (Alfè et al., 2009). When the upward-increasing gradient magnetic field is applied, the liquid-like substance increases and the protrusions transform into a sheet-like structure. The particle size of the soot decreases without an external magnetic field when the oxygen content increases to 26%. The particles cluster highly together and individual particles are difficult to distinguish from one another. The solid properties of the particles are more evident than those with low oxygen content. For F2-10 and F2-30 conditions, the clustered soot is wrapped in liquid-like material, and the larger coverage area of the liquid material can be observed with the larger magnetic field.
When the oxygen ratio is 31%, the agglomerated soot particles contain an increased number of primary soot particles compared to low oxygen concentrations. After adding an upward-increasing gradient magnetic field, the morphology is still a cluster of hundreds or thousands of nearly spherical elementary particles, but the particle edges are slightly blurred. From the above TEM images, it can be found that with increasing oxygen concentration, the content of liquid-like substances decreases and the agglomeration degree increases. When an upward-increasing gradient magnetic field is applied, the surface of the particles grows slowly due to the low flame temperature in the primary region. Moreover, collisions and condensation occur between the particles, and the PAHs covered on the surface increase.
Soot nanostructure
To further obtain the nanostructure of the soot, the yellow circle areas shown in Figure 2 are magnified by HRTEM. Figure 3 presents the typical HRTEM images of soot. Those with long, straight, ordered, and regularly arranged fringes represent a higher degree of carbonization, while the curved, disordered, and randomly distributed fringes stand for a lower degree of maturity (Ying and Liu, 2017). The soot particles in the F1 flame show a disordered structure, in which no clear center is found and the direction of the fringes is random. The degree of the fringe disorder increases with the increasing magnetic field.
For the soot in the F2 flame, the arrangement of the inner fringes becomes more ordered, and different-sized outer shells are formed, which shows a typical fullerene-like structure. This is mainly because the total flame temperature increases with increasing oxygen ratio. The oxidation reaction causes the PAH layers stacked on the particle surface to be oxidized and aggregate several particles to form new condensed soot particles. However, for F2-10 and F2-30 conditions, the inner part also exhibits a fullerene-like structure consisting of partially curved fringes, but the outer shells are relatively disordered. This variation is mainly because the paramagnetic force acting on the O2 and OH radicals changes their movements after the application of an upward-increasing gradient magnetic field. While the oxidant side of the inverse diffusion burner is located in the center, the oxygen diffusion to the fuel side becomes poor. Therefore, the surface growth rate of the soot is slowed down and the degree of carbonization is relatively low. Compared with the soot in the F2 flame, the fullerene-like nanostructure of the soot particles in the F3 flame is more obvious and frequent. The center is more defined and the outer shell is composed of longer and more ordered fringes. This is because the soot particles grow faster in the high-temperature reaction region with 31% O2 content and the fringes are relatively long and straight. However, even when an external magnetic field is applied, the nanostructure observed by the naked eye is not significantly changed.
To further compare the differences in the soot nanostructure quantitatively, the skeletonized images of the typical HRTEM images are shown in Figure 4. The extracted fringe length and tortuosity are calculated and the average values are listed in Table 3. The detailed distribution of fringe length and tortuosity and mean value of soot particles under different flame conditions are presented in Supplementary Figure S2. For the F1 flame, the average fringe length is 0.93 nm, and 61% of the fringes are less than 1 nm. When an upward-increasing gradient magnetic field is arranged, the mean fringe length decreases with the increasing magnetic field, and the minimum average fringe length is 0.91 nm for F1-30 soot. The average fringe length of the soot produced by the flame with 26% oxygen concentration is longer and the fringes are mainly concentrated in the range of 0.8–1.0 nm. In the magnetic field flame, the fringe length decreases slightly and is mainly distributed between 0.7 and 0.9 nm. The mean fringe length of the soot reaches the maximum value of 1.01 nm in flame with 31% oxygen content. The fringe distribution range is wide, with 47.6% fringes longer than 1 nm. With the increasing magnetic field, the average fringe length decreases at the same oxygen content, and the shortest fringe length is 0.97 nm for the soot in the F3-30 flame. In an environment with sufficient oxygen, the fringes are susceptible to oxidation and the arrangement is more regular, so the average fringe length increases. However, with an upward-increasing gradient magnetic field, the paramagnetic force inhibits the diffusion and convection movement of O2 to the flame side (Yamada et al., 2003), thus retarding the nucleation and surface growth of soot particles. The lower degree of carbonization further influence the fringe arrangement and length.
The change of fringe tortuosity is exactly opposite to the fringe length. The average value of the fringe tortuosity is F1<F1-10 < F1-30 in the order from small to large with 21% O2 concentration. The stacking sequence of the fringes is disordered as shown in Figure 4. The fringes are short and curved. When 26% O2 is added, the mean fringe tortuosity decreases, and the average fringe curvatures corresponding to the conditions of F2, F2-10, and F2-30 are 1.26, 1.27, and 1.28, respectively. The fringe tortuosity of soot particles without a magnetic field at 31% oxygen concentration is the smallest, 1.20. The fringe tortuosity increases slightly with the upward-increasing gradient magnetic field. It is evident that the fringe length is negatively correlated with the fringe tortuosity: the longer the fringe length, the smaller the tortuosity.
Soot graphitization degree
Table 4 gives the (002) peak diffraction value of the soot. The soot all shows broadened diffraction peaks near 24° as the XRD spectra are shown in Figure 5. Since XRD is more sensitive to crystalline substances, it shows that all samples belong to an amorphous structure. The closer the diffraction angle of the (002) peak near 25°, the higher the degree of graphitization of soot particles. Combined with the offset of the (002) peak in Table 4, the peak position approaches 25° as the oxygen concentration increases, indicating that the graphitization degree of soot particles is enhanced and relatively more mature. At the same O2 ratio, when the upward-increasing gradient magnetic field increases, the overall peak position shifts to the smaller side. The results show that the effect of the magnetic field significantly reduces the graphitization degree of soot, which is consistent with the results of nanostructure analysis.
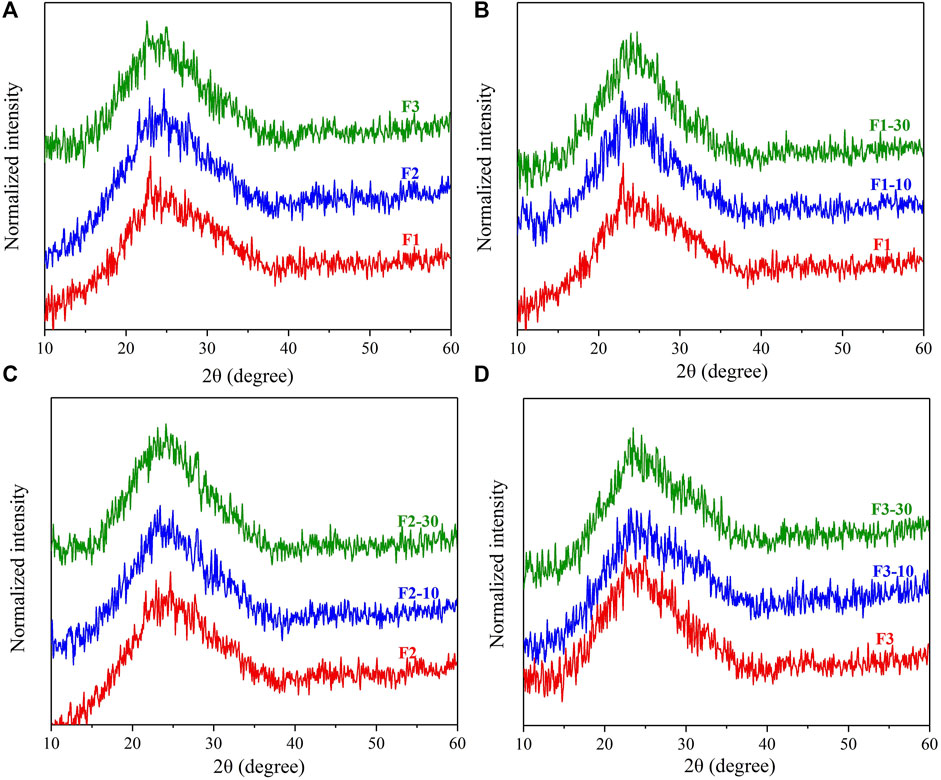
FIGURE 5. XRD spectra of soot in different magnetic fields. (A) Without magnetic field, (B) 21% O2, (C) 26% O2, (D) 31% O2.
Soot oxidation reactivity
Figure 6 shows the weight loss curves of the soot at a constant temperature of 500 °C. The greater the slope of the curve, the faster the oxidation rate. As O2 concentration increases in the flames, the oxidation rate of the soot gradually slows down shown in Figure 4A. Considering the weight loss curves in Figure 4B, the time required for soot generated in flames F1, F1-10, and F1-30 to consume 90% of their mass is 37.32, 32.30, and 30.82 min, respectively. The oxidation reactivity of soot is enhanced by the application of the upward-increasing gradient magnetic field. When the O2 ratio increases to 26 and 31%, the effects of the magnetic field on the oxidation reactivity of soot are relatively weakened. The time required for the soot produced in F3, F3-10, and F3-30 flames to oxidize to 90% of their mass is 51.34, 48.5, and 47.9 min, respectively.
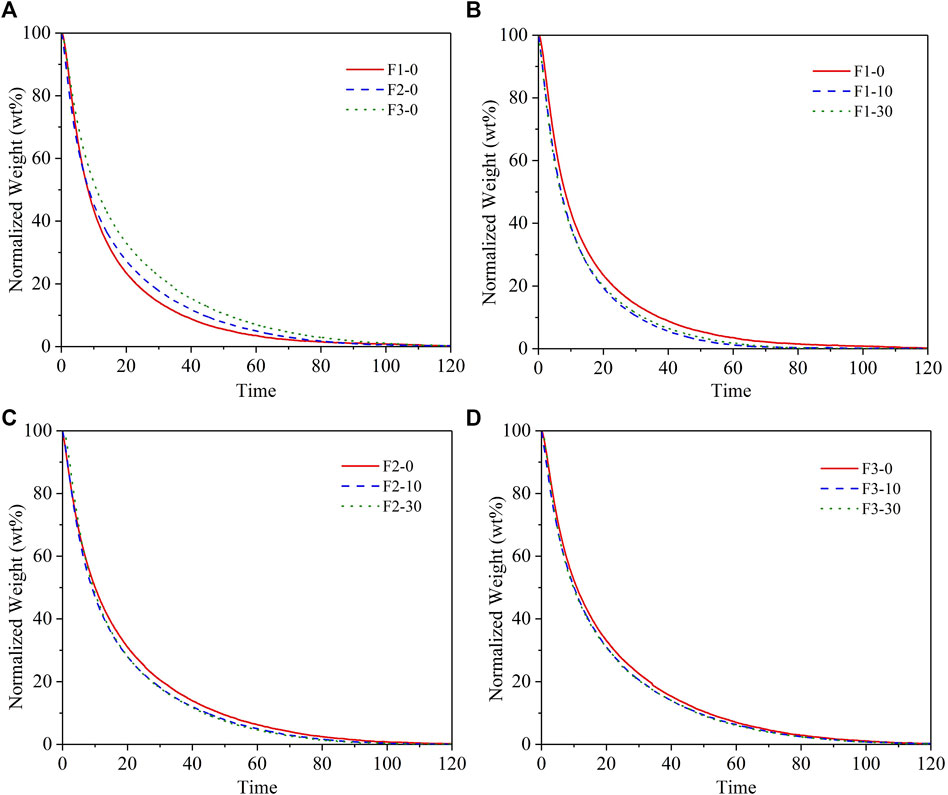
FIGURE 6. TGA results of soot in different magnetic fields, (A) without magnetic field, (B) 21% O2, (C) 26% O2, (D) 31% O2.
The oxidation results show that the higher the proportion of O2 added to the flames, the greater the influence in reactivity to the soot. When an upward-increasing gradient magnetic field is applied, the oxidation reactivity of soot is significantly enhanced at 21% O2 content, whereas the effect of the magnetic field is relatively weak when the O2 concentration is increased to 31%. This is because, at 21% O2 content, the effects of the magnetic field have a greater influence on the direction of movement of O2 and OH radicals, which lowers the flame temperature and inhibits nucleation and surface growth of soot. Therefore, the soot is nascent with the magnetic field, resulting in a low graphitization degree and high oxidation reactivity. When the O2 concentration continues to increase to 31%, the ability to diffuse is strong due to the high O2 content in the flame center, and the paramagnetic force generated by the magnetic field is relatively weak. The combined effects cause a slight change in soot reactivity.
Conclusion
This study investigates the effects of the upward-increasing gradient magnetic field on the soot properties in the ethylene inverse diffusion flames with different oxygen concentrations, including soot morphology, nanostructure, graphitization degree, and oxidation reactivity experimentally. The main results are summarized as follows:
1) The graphitization degree of soot with larger O2 content is higher. The soot shows an amorphous carbon structure at 21% O2 concentration, while fullerene-like structures appear with increasing oxygen content. The soot fringe length decreases and the fringe tortuosity increases with the upward-increasing gradient magnetic field.
2) The magnetic field has the greatest effect on the oxidation reactivity of soot produced in the flame with 21% O2 concentration, and the oxidation rate is significantly accelerated. The effect of the magnetic field is relatively weak for the soot from the flame with 31% O2 content.
3) The upward-increasing gradient magnetic field influences the flame structures mainly by affecting the movement of the paramagnetic O2 and OH radicals, which subsequently changes the soot properties.
Data availability statement
The raw data supporting the conclusions of this article will be made available by the authors, without undue reservation.
Author contributions
YY: conceptualization, methodology, investigation, formal analysis; writing-original draft and editing, and funding acquisition; JD: investigation, data curation, and visualization; DL: supervision, project administration, and funding acquisition.
Funding
This work is supported by the Natural Science Foundation of Jiangsu Province (BK20200490), the National Natural Science Foundation of China (52106160, 52076110), and the Fundamental Research Funds for the Central Universities (30920031103).
Conflict of interest
The authors declare that the research was conducted in the absence of any commercial or financial relationships that could be construed as a potential conflict of interest.
Publisher’s note
All claims expressed in this article are solely those of the authors and do not necessarily represent those of their affiliated organizations, or those of the publisher, the editors and the reviewers. Any product that may be evaluated in this article, or claim that may be made by its manufacturer, is not guaranteed or endorsed by the publisher.
Supplementary material
The Supplementary Material for this article can be found online at: https://www.frontiersin.org/articles/10.3389/fenrg.2022.982391/full#supplementary-material
References
Agarwal, S., Kumar, M., and Shakher, C. (2015). Experimental investigation of the effect of magnetic field on temperature and temperature profile of diffusion flame using circular grating talbot interferometer. Opt. Lasers Eng. 68, 214–221. doi:10.1016/j.optlaseng.2015.01.004
Agarwal, S., Kumar, M., and Shakher, C. (2014). Temperature measurement of axisymmetric flames under the influence of magnetic field using talbot interferometry. AIP Conf. Proc. 1620, 485–491.
Alfè, M., Apicella, B., Barbella, R., Rouzaud, J. N., Tregrossi, A., and Ciajolo, A. (2009). Structure–property relationship in nanostructures of young and mature soot in premixed flames. Proc. Combust. Inst. 32, 697–704. doi:10.1016/j.proci.2008.06.193
Ali, M. U., Siyi, L., Yousaf, B., Abbas, Q., Hameed, R., Zheng, C., et al. (2021). Emission sources and full spectrum of health impacts of black carbon associated polycyclic aromatic hydrocarbons (PAHs) in urban environment: A review. Crit. Rev. Environ. Sci. Technol. 51, 857–896. doi:10.1080/10643389.2020.1738854
Aoki, T. (1990). Radical emissions and anomalous reverse flames appearing in upward-increasing magnetic fields. Jpn. J. Appl. Phys. 29, 181–190. doi:10.1143/jjap.29.181
Arif, M., Kumar, R., Kumar, R., Eric, Z., and Gourav, P. (2018). Ambient black carbon, PM2.5 and PM10 at patna: Influence of anthropogenic emissions and brick kilns. Sci. Total Environ. 624, 1387–1400. doi:10.1016/j.scitotenv.2017.12.227
Chakrabarty, R. K., Beeler, P., Liu, P., Goswami, S., Harvey, R. D., Pervez, S., et al. (2021). Ambient PM2.5 exposure and rapid spread of COVID-19 in the United States. Sci. Total Environ. 760, 143391. doi:10.1016/j.scitotenv.2020.143391
Falco, G. De., Bocchicchio, S., Commodo, M., Minutolo, P., and D’Anna, A. (2022). Raman spectroscopy of nascent soot oxidation: Structural analysis during heating. Front. Energy Res. 10, 878171. doi:10.3389/fenrg.2022.878171
Gilard, V., Gillon, P., Blanchard, J.-N., and Sarh, B. (2008). Influence of A Horizontal magnetic field on A Co-flow methane/air diffusion flame. Combust. Sci. Technol. 180, 1920–1935. doi:10.1080/00102200802261506
Jocher, A., Bonnety, J., Gomez, T., Pitsch, H., and Legros, G. (2019). Magnetic control of flame stability: Application to oxygen-enriched and carbon dioxide-diluted sooting flames. Proc. Combust. Inst. 37, 5637–5644. doi:10.1016/j.proci.2018.05.156
Jocher, A., Bonnety, J., Pitsch, H., Gomez, T., and Legros, G. (2017). Dual magnetic effects on soot production in partially premixed flames. Proc. Combust. Inst. 36, 1377–1385. doi:10.1016/j.proci.2016.05.017
Jocher, A., Pitsch, H., Gomez, T., and Legros, G. (2015). Modification of sooting tendency by magnetic effects. Proc. Combust. Inst. 35, 889–895. doi:10.1016/j.proci.2014.05.139
Kumar, M., Agarwal, S., Kumar, V., Khan, G. S., and Shakher, C. (2015). Experimental investigation on butane diffusion flames under the influence of magnetic field by using digital speckle pattern interferometry. Appl. Opt. 54, 2450–2460. doi:10.1364/ao.54.002450
Li, J., Lai, S., Chen, D., Wu, R., Kobayashi, N., Deng, L., et al. (2021). A review on combustion characteristics of ammonia as A carbon-free fuel. Front. Energy Res. 9, 760356. doi:10.3389/fenrg.2021.760356
Mehmood, K., Saifullah, M. I., and Abrar, M. M. (2020). Can exposure to PM2.5 particles increase the incidence of coronavirus disease 2019 (COVID-19)?[J]. Sci. Total Environ. 741, 140441. doi:10.1016/j.scitotenv.2020.140441
Shinoda, M., Yamada, E., Kajimoto, T., Yamashita, H., and Kitagawa, K. (2005). Mechanism of magnetic field effect on OH density distribution in A methane-air premixed jet flame. Proc. Combust. Inst. 30, 277–284. doi:10.1016/j.proci.2004.07.006
Takashi, A. (1990). A magnetically induced anomalous ring flame and quenching characteristics of butane flame. Jpn. J. Appl. Phys. 29, 864–867.
Wang, Y., and Chung, S. H. (2019). soot formation in laminar counterflow flames. Prog. Energy Combust. Sci. 74, 152–238. doi:10.1016/j.pecs.2019.05.003
Yamada, E., Kitagawa, K., Shinoda, M., and Yamashita, H. (2003b). Influence of four kinds of gradient magnetic fields on hydrogen-oxygen flame. AIAA J. 41, 1535–1541. doi:10.2514/2.2104
Yamada, E., Shinoda, M., Yamashita, H., and Kitagawa, K. (2003a). Experimental and numerical analyses of magnetic effect on OH radical distribution in A hydrogen-oxygen diffusion flame. Combust. Flame 135, 365–379. doi:10.1016/j.combustflame.2003.08.005
Yamada, E., Shinoda, M., Yamashita, H., and Kitagawa, K. (2002). Numerical analysis of a hydrogen-oxygen diffusion flame in vertical or horizontal gradient of magnetic field. Combust. Sci. Technol. 174, 149–164. doi:10.1080/713713079
Ying, Y., and Liu, D. (2017). Effects of butanol isomers additions on soot nanostructure and reactivity in normal and inverse ethylene diffusion flames. Fuel 205, 109–129. doi:10.1016/j.fuel.2017.05.064
Ying, Y., and Liu, D. (2018). Nanostructure evolution and reactivity of nascent soot from inverse diffusion flames in CO2, N2, and He atmospheres. Carbon 139, 172–180. doi:10.1016/j.carbon.2018.06.047
Ying, Y., and Liu, D. (2021). Soot properties in ethylene inverse diffusion flames blended with different carbon chain length Alcohols. Fuel 287, 119520. doi:10.1016/j.fuel.2020.119520
Keywords: soot properties, magnetic field, inverse diffusion flame, nanostructure, oxidation reactivity
Citation: Ying Y, Duan J and Liu D (2022) Effects of the upward-increasing gradient magnetic field on soot properties in ethylene inverse diffusion flames with different oxygen concentrations. Front. Energy Res. 10:982391. doi: 10.3389/fenrg.2022.982391
Received: 30 June 2022; Accepted: 20 July 2022;
Published: 11 August 2022.
Edited by:
Zhongyang Luo, Zhejiang University, ChinaReviewed by:
Gianluigi De Falco, DICMAPI—University of Naples Federico II, ItalyPatrick Mountapmbeme Kouotou, National Advanced School of Engineering of Maroua/ Researcher, Cameroon
Copyright © 2022 Ying, Duan and Liu. This is an open-access article distributed under the terms of the Creative Commons Attribution License (CC BY). The use, distribution or reproduction in other forums is permitted, provided the original author(s) and the copyright owner(s) are credited and that the original publication in this journal is cited, in accordance with accepted academic practice. No use, distribution or reproduction is permitted which does not comply with these terms.
*Correspondence: Yaoyao Ying, yingyaoyao@njust.edu.cn; Dong Liu, dongliu@njust.edu.cn