- 1School of Engineering and Technology, Central Queensland University, Rockhampton, QLD, Australia
- 2Centre for Technology in Water and Wastewater, School of Civil and Environmental Engineering, University of Technology Sydney, Ultimo, NSW, Australia
- 3Mechanical Engineering Department, Prince Mohammad Bin Fahd University, Al Khobar, Saudi Arabia
- 4Science and Math Program, Asian University for Women, Chattogram, Bangladesh
- 5School of Environmental and Life Sciences, University of Newcastle, Newcastle Upon Tyne, VA, Australia
- 6Department of Chemical Engineering, Qatar University, Doha, Qatar
Recent years have seen a sharp rise in CO2 emissions into the atmosphere, which has contributed to the issue of global warming. In response to this several technologies have been developed to convert CO2 into fuel. It is discovered that the employment of a solar-driven thermochemical process (S-DTCP) that transforms CO2 into fuels can increase the efficiency of the production of sustainable fuels. The process involves the reduction of metal oxide (MO) and oxidizing it with CO2 in a two-step process using concentrated solar power (CSP) at higher and lower temperatures, respectively. This study summarizes current advancements in CO2 conversion methods based on MO thermochemical cycles (ThCy), including their operating parameters, types of cycles, and working principles. It was revealed that the efficiency of the solar conversion of CO2 to fuel is not only influenced by the composition of the MO, but also by its morphology as well as the available surface area for solid/gas reactions and the diffusion length. The conversion mechanism is governed by surface reaction, which is influenced by these two parameters (diffusion length and specific surface area). Solar energy contributes to the reduction and oxidation steps by promoting reaction kinetics and heat and mass transport in the material. The information on recent advances in metal oxide-based carbon dioxide conversion into fuels will be beneficial to both the industrial and academic sectors of the economy.
1 Introduction
Fossil fuels provide a significant amount of energy (Mofijur et al., 2013a), but their ongoing usage for industrial purposes is threatening the atmosphere because of the high amounts of greenhouse gas emissions i.e. CO2 (Mofijur et al., 2013b). The impacts of greenhouse gases are widely acknowledged to be one of the fundamental causes of climate change (Langford, 2005). In order to mitigate climate change, it is necessary to minimize the emission of CO2 (Jacobson et al., 2019). Carbon dioxide is a colorless, naturally occurring gas that is made up of molecules that each have one carbon atom covalently double bonded to two oxygen. Carbon dioxide, in addition to other greenhouse gases, is a significant contributor to the ability of the earth to sustain a temperature that is suitable for human habitation (Garba et al., 2021). Carbon dioxide at low concentrations has less toxicological effect. It causes the development of hypercapnia and respiratory acidosis when more than 5% are present in the atmosphere (Kettner et al., 2013). As a result of the increased effects of parasympathetic nerve activity, which is thought to be caused by interfering with the breakdown of acetylcholine by acetylcholinesterase, severe acidosis can result in a decrease in the rate of respiration (Permentier et al., 2017).
More than 10% of carbon dioxide concentrations have been shown to produce convulsions, coma, and even death (Żaba et al., 2011). Increased CO2 levels of more than 30% act quickly and can cause loss of consciousness in a matter of seconds. This might explain why sufferers of unintentional intoxication frequently do not act to fix the problem. Capturing carbon dioxide emissions at the site of emission is an appealing concept that has gained popularity in recent years and converting it into useful products such as synthetic fuels (Helal et al., 2020), with the entire cycle being powered by renewable resources such as solar irradiation (Glenk and Reichelstein, 2019). Figure 1 presents the different S-DTCPs used to produce industrial items and fuels. In general, these processes can be divided into two categories: the creation of industrial items and the generation of fuels (Mustafa et al., 2020). Solar fuels are a particularly tempting alternative to non-renewable fossil fuels because of the availability of solar energy and the fact that the production cycle produces almost no emissions (Shahabuddin et al., 2021). The method of manufacturing solar fuels generally comprises (i) thermochemical reduction-oxidation (redox) splitting of plentiful CO2/H2O into a CO/H2 known as syngas; and (ii) hydrocarbon fuel synthesis by some well-established gas to liquid processes (e.g. Fischer–Tropsch). In contrast, the commercialisation of solar-powered syngas has not yet been realised, mostly as a result of the process’s low solar-to-fuel efficiency (Boretti, 2021).
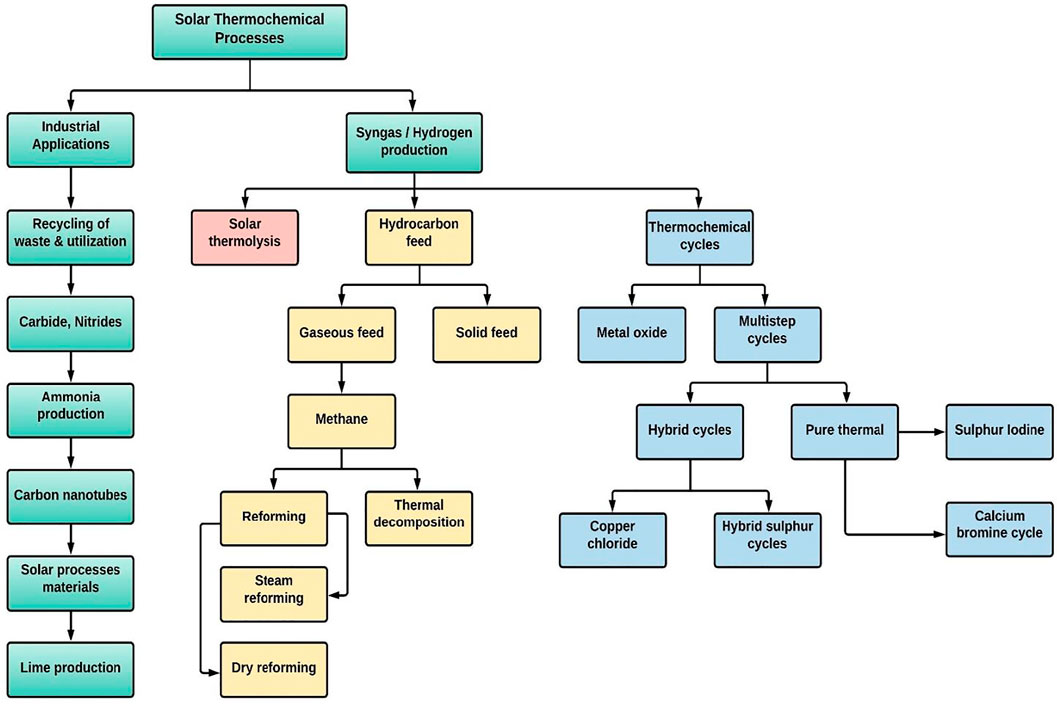
FIGURE 1. List of thermochemical processes that use solar energy to produce industry items and fuels (Mustafa et al., 2020).
The development of redox materials has received increased attention in recent years, intending to increase the efficiency of the technology while also making it more financially viable (Scheffe and Steinfeld, 2014). The ability of a redox material to exchange oxygen (release/absorption of O2) has a direct and proportional impact on the amount per mass of material. The use of redox materials in solar fuel production processes has proven successful in the past. Oxide minerals such as ferrites (Kodama et al., 2005; Scheffe et al., 2013a) and hercynite (Arifin et al., 2012; Muhich et al., 2013), and more recently ceria (Chueh et al., 2010; Furler et al., 2012a) and perovskites (Cooper et al., 2015; Dey et al., 2015a; Ezbiri et al., 2017a; Takacs et al., 2016), have been used successfully as redox materials (Boretti et al., 2021). It is evidenced that many pieces of researches are published on thermochemical splitting of CO2 but few researchers reviewed and analysed them.
Based on the aforementioned literature review, this min review aimed at summarizing the most recent works that are focused on MO-based solar thermochemical CO2 splitting to fuel. This condensed review will provide researchers updated news about the research directions in this topic and discuss it’s the technology readiness level as well as provide recent updates about scientific and industrial aspect of this technology.
2 Solar thermochemical splitting of carbon dioxide via MO-based reaction
It is possible to operate at moderate temperatures and avoid separation difficulties by dissociating CO2 in many steps, each of which makes use of metal oxides during the redox processes (Snoeckx and Bogaerts, 2017). The first phase is endothermic, and it involves the reduction of oxidized MO (MOox) to metal (M) or reduced MO (MOred) using solar thermal energy. In the second phase, the MOred is oxidised with CO2, resulting in the generation of CO. The MOox can be used again for the first step (Mustafa et al., 2020). Multiple redox cycles are known to exist that can be followed in order to separate H2O from CO2. Theoretically, these redox cycles have an efficiency of greater than 40%, according to the researchers. It is generally accepted that two-cycle classes of metal oxides, both volatile and non-volatile, should be considered (Scheffe and Steinfeld, 2014). Doping techniques are used in both types of cycles to improve the thermodynamic, kinetic, and physical properties.
The basic equations for this cycle are:
Deepak and Banerjee presented literature that analysis the thermal efficiency of the ThCy on iron and Ceria under different conditions. It was concluded that the thermal efficiency
2.1 Solar thermochemical splitting of carbon dioxide via ceria-based redox reactions
Ceria (CeO2)-based ThCy may be able to store intermittent and diluted solar energy by generating chemical fuels. Many researchers have experimented with and reported the performance of ceria-based ThCy. For example, Hathaway, Bala Chandran, Gladen, Chase, Davidson (Hathaway et al., 2016) et al. studied a 4.4 kW solar receiver/reactor’s ability to split CO2 through the isothermal CeO2 thermochemical redox cycle in the course of steady-state operation within a high-flux solar simulator. At 1750K, a steady-periodic operation took place where 360 ml min−1 of CO generated more than 45 redox cycles in a continuous flow. A 95% of the sensible heat derived from process gases was recovered. Without considering the energy costs of N2 production, the solar-to-fuel efficiency was 1.64%. N2 is used as a sweep gas for reduction. Including the solar energy needed for N2 production through cryogenic separation, the solar-to-fuel efficiency was found to be 0.72%. A conclusion was reached that splitting CO2 or water, following the isothermal approach, via a thermochemical MO redox cycle does not show prospects for development. There are certain thermodynamic limitations in this cycle and other issues like the inability to increase reactor efficiency beyond 2%.
Lin, Samson, Wismer, Grolimund, Alxneit, Wokaun (Lin et al., 2016) investigated the dual-phase Zn that was modified by ceria synthesized via coprecipitation to be used as a redox material to thermochemically split H2O and CO2. In the first few cycles, it was observed that the materials showed a significant increase in the productivity of H2 and CO. There was a correlation between the increased productivity in the initial cycles and a considerable loss of Zn during the sublimation of ZnO. His observation suggests that the secondary ZnO phase in Zn-modified ceria expressed a negative effect on its thermochemical activity.
Nair, Abanades (Nair and Abanades, 2016a) studied the combination of methane being partially oxidized with the splitting of H2O and CO2 set in solar thermal conditions. CO2 and H2O were split by CeO2 by utilizing concentrated solar energy. The reaction temperatures were varying from 900 to 1100 °C. This experiment took place inside a solar-powered thermogravimetric system and results showed close reaction orders of both CO2-induced oxidation of CeO2−δ and CH4-induced reduction with corresponding activation energies, 36 and 109 kJ mol−1. A comparison of the outcomes was noted down with the ones acquired from surfactant-induced self-assembly and hydrothermal templating. The material synthesis that occurred via hydrothermal and self-assembly methods had high reaction rates and stability on cycling. MgO promoted CeO2 resulted in a higher rate of reduction and highest nonstoichiometric (δ = 0.431) when reduction occurred at a temperature of 1000°C. Results about the amount of evolved CO implied that reoxidation is almost complete as it came out to be the highest (δ = 0.402). For thermal reduction of ceria, the obtained nonstoichiometric and consequent fuel effectivity were 10 times more than the values that were reported. Some studies were performed in solar reactor prototypes to enable partial ceria reduction in the presence of methane. Subsequently, oxidation with ceria was promoted by H2O/CO2. MgO and Al2O3 took place and were experimented with under packed bed conditions. This was put into comparison with commercial ceria based on the production of syngas. MgO promoted CeO2 showed remarkable augmentation in the system efficiency.
Haeussler, Abanades, Julbe, Jouannaux, Cartoixa (Haeussler et al., 2020) designed, constructed and experimented with a new solar reactor that is monolithic and has compatibility with ceria redox reactions when the solar radiation is concentrated. The ceria redox material is built as structures that are porous and reticulated with controlled cell sizes and gradients, 10–60 pores per inch (ppi). These features allow effective volumetric solar radiation absorption and microscale interconnected porosity. This system helps increase efficiency in solid-gas reactions. The effect of functioning conditions like the type of oxidizing gas, pressure and reduction and oxidation temperatures on reactor performance was examined. When the temperature for reduction was increased or the pressure was decreased, the yields of fuel production and ceria reduction extent improved (up to 341 µmol/g). Decreasing CO: CO2, by raising the total inlet gas flow rate, or increasing inlet CO2 concentration resulted in an improved rate of oxidation by as far as 9.3 ml/g/min. The observed fuel production rates surpassed the maximum of the previously recorded values 8 times more utilizing the ceria porous foams that were manufactured to be highly reactive that underwent cycling between 900°C and 1400°C. In 100% of CO2, oxidation took place upon dynamic cooling. A mean H2/CO production of ∼280 Ncm (Langford, 2005)/cycle was attained where 64 cycles were performed, where solar-to-fuel efficiency reached ∼7.5% with significant performance stability of the material.
Riaz, Ali, Enge, Tsuzuki, Lowe, Lipiski (Riaz et al., 2020) investigated the effects of the concentrations of two elements, V and Ce, in vanadia–ceria multiphase systems for the generation of synthesis gas through the splitting of CO2 and H2O following thermochemical redox cycles of splitting that had methane partial oxidation reactions integrated with it. The concentration range varied from 0 to 100% each. Prepared oxygen carriers’ oxidation is executed by separate and sequential splitting reactions of CO2 and H2O. Pure CeO2 showed the lowest oxygen exchange capacities while pure V2O5 showed the highest. Pure CeO2 also exhibited the lowest performance of syngas production whereas pure V2O5 showed the highest. The systems involving mixed-oxide showed a balanced neutral performance where the oxygen exchange capacity was recorded to be 5 times higher than what pure CeO2 shows when the length of methane cracking was decreased. Having 25% V added to CeO2 resulted in a CeVO4 and CeO2 optimum mixture for the improved splitting of CO2 and H2O. When the concentration of V is high, the formation of cyclic carbide and oxidation are consequent to a syngas yield that is greater than pure CeO2. The synthesis methods used in preparation of ceria based material is presented in Table 1.
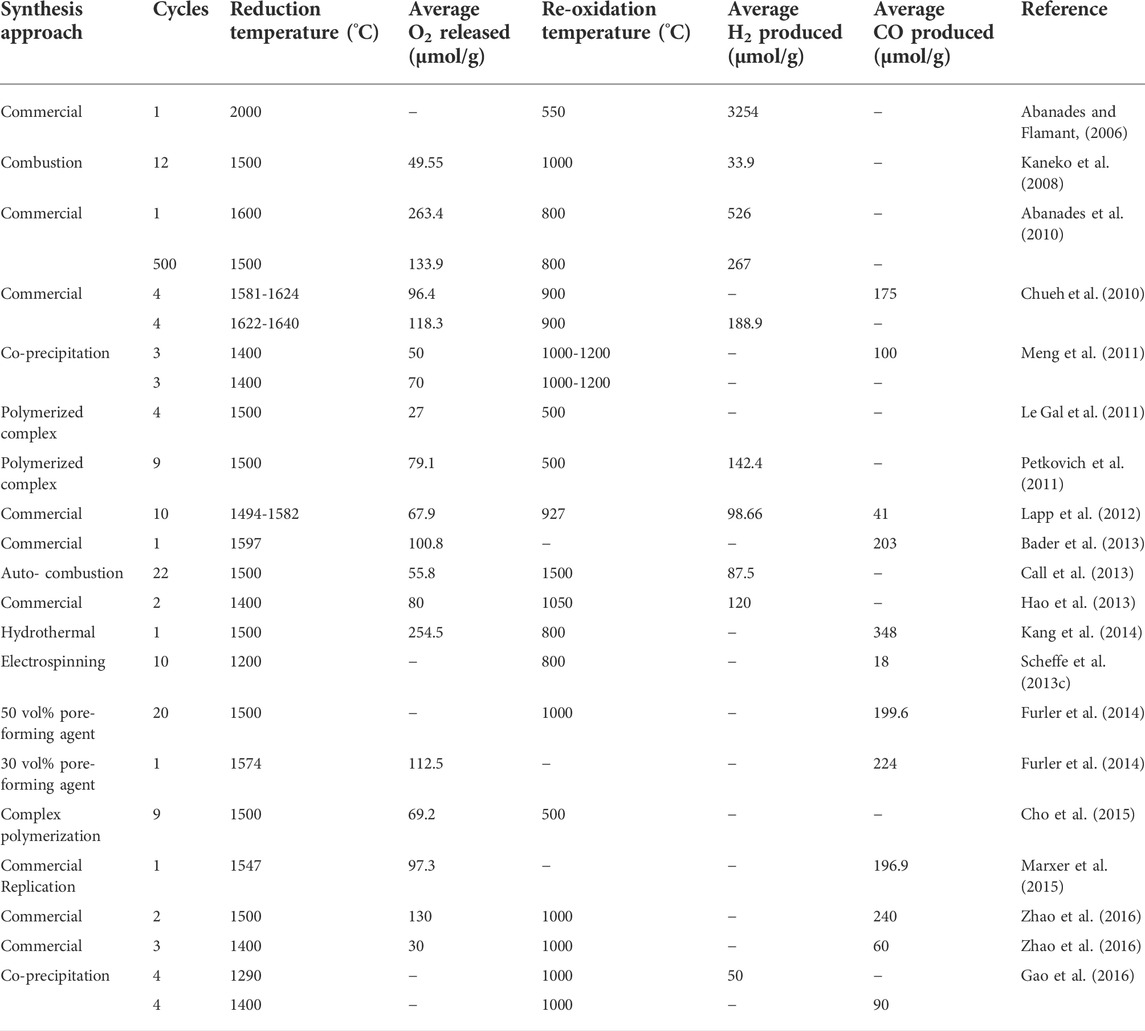
TABLE 1. Experimental findings reported in case of CeO2 based solar thermochemical H2O/CO2 splitting cycles. Reprinted with permission.
It can be seen that both binary and ternary MOs containing Ce and other metals at different compositions can be utilized in ThCy. Table 2 illustrates the O2 and H2/CO productivities of binary and ternary ceria-based composites in multiple solar ThCys. It was discovered that adding some dopants increased the ceria-based material’s reactivity and thermal stability through a number of ThCyas.
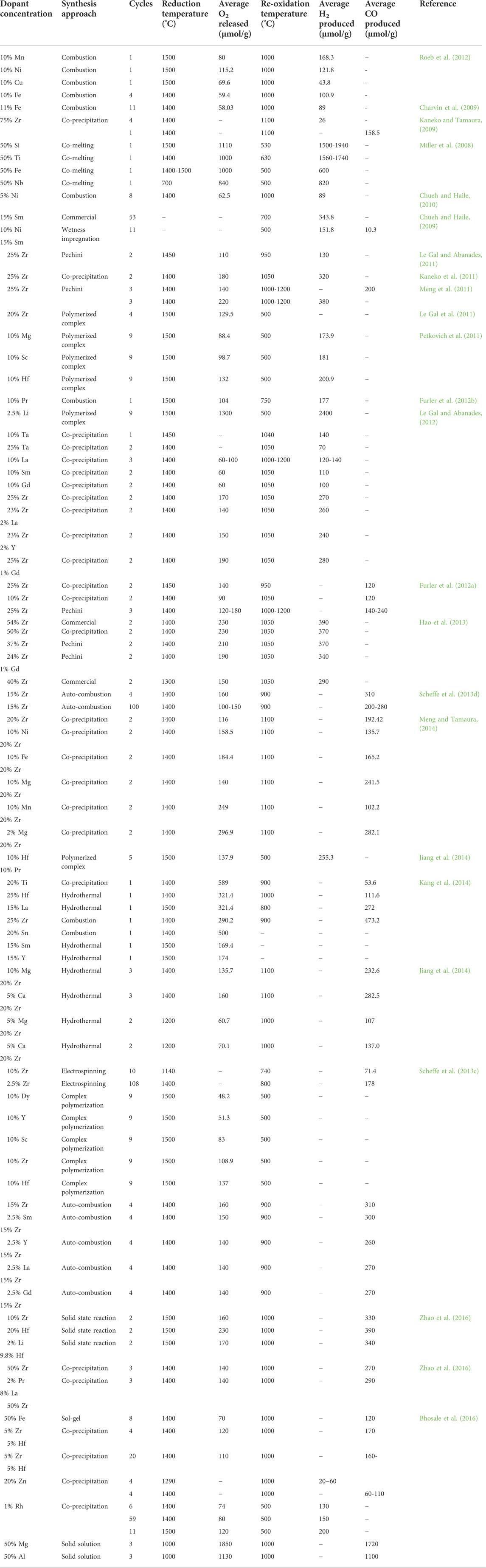
TABLE 2. Experimental findings reported in case of doped ceria based solar thermochemical H2O/CO2 splitting cycles. Reprinted with permission from Bhosale et al. (2019)
2.2 Solar thermochemical splitting of carbon dioxide via perovskite-based redox reactions
Perovskites have the capability of providing high production of O2 at temperatures that are comparatively high (Demont et al., 2014). Additionally, they can integrate reduction oxidation that demands energy (Sastre et al., 2017a). The cation found in the M site determines the redox properties of perovskites. It is denoted by ABO3−δ and falls in the category of nonstoichiometric oxides (Mustafa et al., 2020). There has not been much investigation into these oxides to reduce metals in thermochemical cycles (Arshad et al., 2021). The cation sites, A and B, are where the replacement of dopants can take place. This makes material configurations notably larger inside perovskites relative to ceria. Research related to thermodynamics that involved oxygen non-stoichiometry data evaluation and extracting entropies and enthalpies have proven lanthanum strontium manganite perovskites’ (indicated by La1-xSrxMnO3−δ) capability in augmenting oxygen exchange capacity relative to pure ceria (Scheffe et al., 2013b). It has been experimentally confirmed that even though the extent of reduction is notably higher, oxidation is thermodynamically less viable and this causes oxidation to be incomplete. Nevertheless, total CO generation from the decomposition of CO2 continues to be significantly higher in contrast to ceria.
Perovskites are considered to be potential redox materials for fuel synthesis via thermo-electrochemical means Ezbiri, Takacs, Stolz, Lungthok, Steinfeld, Michalsky (Ezbiri et al., 2017b). For designing perovskites that constitute balanced redox energetics for the thermochemical splitting of CO2, electronic structure computations predict lattice oxygen vacancy activities as well as the stability of a representative range of perovskites against phase changes of crystals and deleterious carbonate formation (Rafique et al., 2022). The range of free energy calculated for isothermal and temperature-swing redox cycles is used for illustrating systematic changes in the characteristics of these materials when they have specific metal cations doped with them.
Mulmi, Chen, Hassan, Marco, Berry, Sharif, Slater, Roberts, Adams, Thangadurai (Mulmi et al., 2017) examined the usage of perovskite oxides that are nonstoichiometric, (Ba2Ca0.66Nb1.34−xFexO6−δ (BCNF) (0 ≤ x ≤ 1)), for the purpose of splitting CO2 into C, CO, and O2 at increased temperatures. Double perovskite-type BCNF’s chemical stability is exhibited by powder X-ray diffraction after exposure to CO2 of 2000 ppm in Ar at a temperature of 700°C. All members of x ≤ 0.66 BCNF express great chemical stability despite being in conditions at 700 °C temperature and pure CO2. The creation of solid carbon upon being exposed to CO2 was confirmed through scanning electron microscopy along with Raman spectroscopy, DFT analyses, temperature-programmed oxidation (TPO) and mass spectroscopy (MS), and energy-dispersive X-ray. As Fe increases, more solid carbon is produced in BCNF. In the Mössbauer spectroscopy of the as-prepared BCNF, Fe3+, Fe4+ and Fe5+ were found. When exposed to Ar, a constituent Fe that has higher valence undergoes reduction to Fe3+ followed by Fe3+ enhanced CO2 reduction being oxidized. The overall outcomes of BCNFs displayed redox activity at much lesser temperatures if put into comparison with state-of-the-art ceria for reducing CO2. Thus, it can be seen that there is a possibility of putting them into use in fuel technologies that are powered by renewable sources.
Sastre, Carrillo, Serrano, Pizarro, Coronado (Sastre et al., 2017b) proposed mixed oxides with perovskite structure as an alternate material for solar fuel generation through thermochemical redox cycles. The system La0.6Sr0.4Mn1−xAlxO3 (x = 0–0.8) was chosen for this study as it has the required features: thermal stability that is high and rapid oxidation kinetics. The ratio of Al/Mn and its impact on the redox properties were also looked into. After characterization through thermogravimetric analysis, the five oxides samples having varying Al amounts verified the high redox capacity. They also verified the favourable behaviour of these materials in consecutive cycles. According to the results, consequent of reduction at temperature 1300 °C in the inert atmosphere, there is a release of up to 0.32 mmol g−1 of O2. A reaction test of 10 cycles confirmed that for long-term operations, perovskites are viable. Based on observations, when the content of Al was increased, the reduction extent became enhanced. However, the oxidation degree is maximal for constitutions that are close to x = 0.5 and showed 0.318 mmol g−1 O2 delivery (δ = 0.132). After the selection of the constitutions having potentially better redox properties, further reactions were operated in a fixed bed reactor that is of lab scale. For CO formation, CO2 was injected in the oxidation step at 900°C. Perovskite La0.6Sr0.4Mn0.6Al0.4O3 showed interesting results where the reduction extent was 0.266 mmol g−1. However, CO generation is comparatively notably lower with the value being 0.114 mmol g−1.
Ramos, Maiti, Daza, Kuhn, Bhethanabotla (Ramos et al., 2019) assert that perovskite oxides in the category of type ABO3 have proven significant potential for thermochemical CO2 conversion at low temperature using the reverse water gas shift chemical looping (RWGS-CL) process. Transition metals on the ‘B’ site of these perovskite oxides are responsible for adjusting the material properties necessary for the effective conversion of CO2. The functions of Fe, Mn and Co in LaBO3 were explored using an integrated approach of both theory and experiment. Ab-initio density functional theory (DFT) simulations were used for investigating the intrinsic oxygen vacancy generation features and electronic charge distribution of these materials. Properties that are microscale including conversion yield of CO2 and crystallite size were explored by experiments. A comparative analysis is performed to differentiate the material properties influencing the stability and improved CO2 conversion process using perovskites that are plentiful in Fe, in contrast to Mn and Co-rich phases.
Parvanian, Salimijazi, Shabaninejad, Troitzsch, Kreider, Lipiński, Saadatfar (Parvanian et al., 2020) explored the redox functioning ability of porous ceramics having a coating of perovskite designing different forms of architecture. Fabrication of reticulated porous ceramics (RPCs) in three varying pore sizes was done for representing structures and pore sizes of a wide range. The pore sizes were 5, 12, and 75 ppi. The perovskite matter is composed of lanthanum manganite. After its synthesis, the perovskite material underwent Ca and Al doping via Pechini method. Implementing a method involving deep coating, the surface of RPC substrates underwent modifications and got a thin-film coating with ∼15 μm thickness. The CO2 conversion performance of the materials that were formed was evaluated in a gold-image IR furnace. For an in-depth investigation of features pertaining to bulk and surface, an X-ray micro-computed tomography along with SEM/EDX was employed during the investigation. According to findings, 12 ppi, which is the intermediate pore size, reaches the highest perovskite loading and shows a high level of coating uniformity and connectivity. The highest CO generation for 75 ppi was observed after CO2 conversion tests. Inside the furnace, the severe conditions, along with the flow of gaseous phases make the RPCs shrink to 23% of their original length. This alters the pore phase and eliminates minute pores thus decreasing the overall specific surface area. The findings also revealed a major mechanism that causes the CO2 conversion to stop where the coating layer of perovskite shifts its position into the matrix of the RPC frame.
Takalkar, Bhosale, AlMomani, Rashid, Qiblawey, Saleh Saad, Khraisheh, Kumar, Gupta, Shende (Takalkar et al., 2021) investigated La (1-x) SrxMnO3 (LSM) perovskites and their redox reactivity in the context of splitting CO2. Synthesis of LSM perovskites took place following the solution combustion synthesis (SCS) technique. This technique used glycine as a reducing agent. Many types of analytical approaches are used to characterize the structural properties of LSM perovskites. In three sets, thermogravimetric thermal reduction (TR) and CS cycles are conducted to acquire an estimation of the amount of O2 released (nO2) and CO yielded (nCO) by an individual lanthanum strontium manganite perovskite. The sets are in one, three and ten cycles. Higher nO2 by each LSM perovskite is released than the nCO that releases nO2 during the first cycle. The nO2 is lessened, and each LSM perovskite’s re-oxidation capacity is enhanced from cycle one to cycle three. Considering the average nO2 and nCOfrom cycle 2-cycle 10, the perovskites La0.30Sr0.70Mn0.99O2.982 (342.1 μmol of CO/g. cycle) and La0.60Sr0.41Mn0.99O2.993 (214.8 μmol of O2/g·cycle) have been found to reach the top highest redox reactivity. LSM perovskites, excluding La0.88Sr0.11Mn1.00O2.980, have a record of having a very high redox activity than the CeO2 material.
There are a large number of studies that are based on perovskite-based redox reactions as presented in Table 3 in the supplementary documents. It was concluded that although perovskite-based redox reactions can accommodate a wide range of oxygen, the high vacancy formation associated with re-oxidation stem imped the reaction and generated low ratio of CO/H2. There for a compromises between maximal achievable oxygen and fuel generation yield should be optimized, which can be controlled by perovskite formulation.
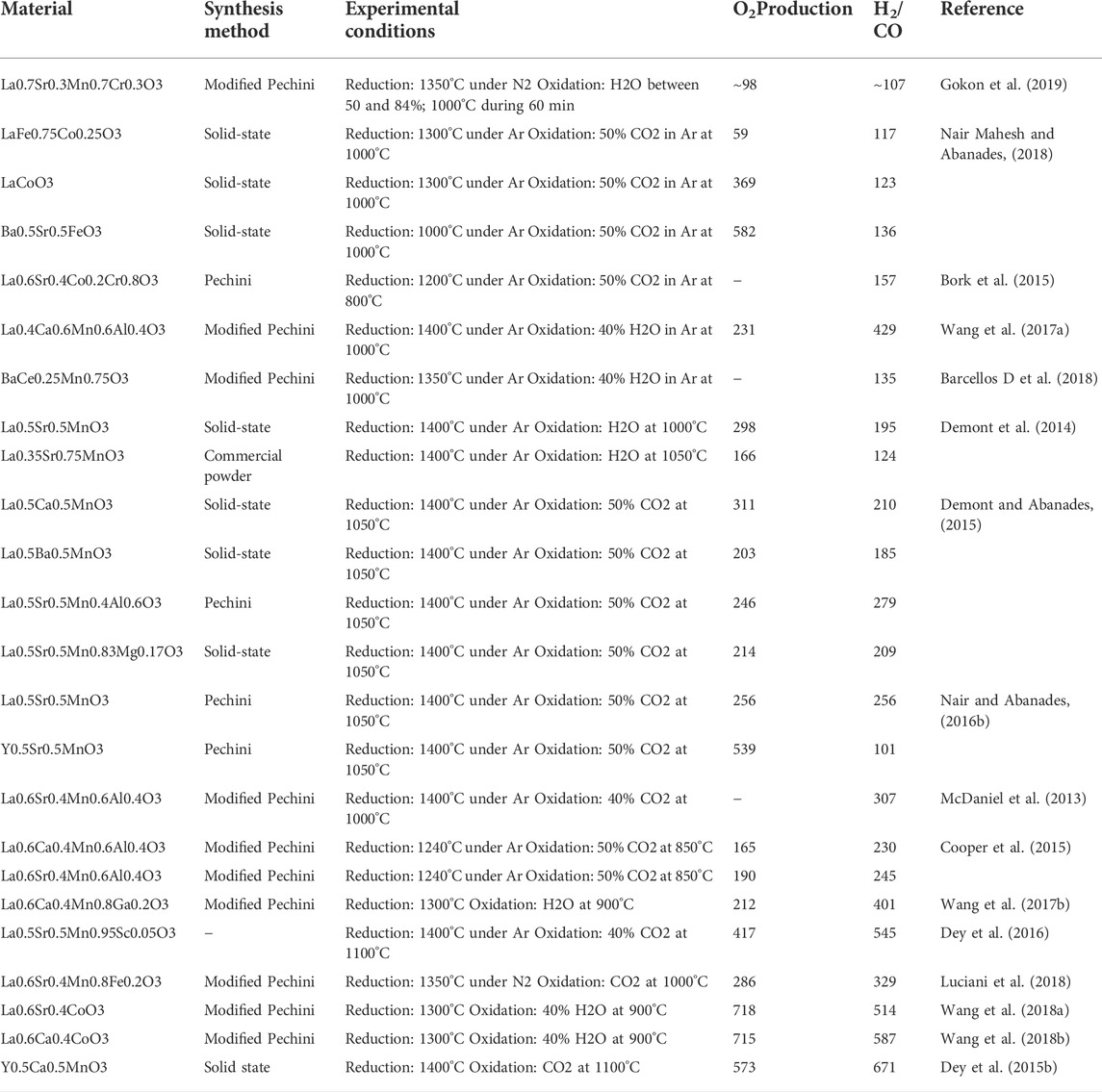
TABLE 3. Summary of the thermochemical cycles based on perovskites studies. Reprinted with permission from Bhosale et al. (2019)
Challenges for solar thermochemical processes
The challenges connected to various solar thermochemical processes can be summarized as follow:
1 The primary disadvantage of using perovskites is typically the insufficient re-oxidation yield brought on by low kinetics and low thermodynamic driving forces. Tuning the redox properties to maximize fuel production is possible thanks to the wide range of perovskite formulations that are possible and the discovery of novel materials.
2 It is crucial to continue making progress in the discovery and characterization of new, higher-performing redox materials that also meet the criteria for desirable thermodynamics, quick reaction kinetics, and crystallographic stability under thermochemical cycling.]
3 The cost projections for ThCy vary greatly and future cost projections are very difficult to make because they depend on a lot of arbitrary and incorrect assumptions. Therefore, well defined cost analysis is required
4 When producing fuel using a flammable redox material like zinc oxide, the dissociation reaction necessitates a very high temperature, which poses problems for the reactor’s materials. It has been noted that a sizable amount of zinc recombines with oxygen during the quench process to form zinc oxide, reducing the process’ overall effectiveness. To avoid zinc recombination with oxygen, rapid quenching with fine zinc particles is recommended.
5 Recombination is dealt with by non-volatile redox materials like ceria and iron. However, sintering, where the particle size increases after a few cycles of operation and lowers overall hydrogen yield, is a problem with ferrite-based cycles.
3 Conclusion
The conversion of CO2 into fuels and chemicals is a potential alternative strategy for addressing both energy and climate change issues simultaneously. There are several technologies available for converting CO2 into fuel, each of which faces its own set of obstacles when it comes to implementation in the field. A great deal has been accomplished in solar thermochemical technology, and it has emerged as a viable option for harnessing concentrated solar power. Because it makes direct use of solar energy, this technique is both highly beneficial and advantageous when compared to other energy utilisation methods. In industrial applications (i.e. electricity generation), CO2 and H2O reduction, concentrated solar energy is increasingly being used. However, even though the thermochemical splitting of CO2 and H2O through metal oxides is thermodynamically feasible, further research on achieving higher efficiency is still recommended.
Author contributions
ZR: Writing original draft; MM: Writing original draft; SA: Writing original draft; AC: Review and editing; FA: Review and editing.
Conflict of interest
The authors declare that the research was conducted in the absence of any commercial or financial relationships that could be construed as a potential conflict of interest.
Publisher’s note
All claims expressed in this article are solely those of the authors and do not necessarily represent those of their affiliated organizations, or those of the publisher, the editors and the reviewers. Any product that may be evaluated in this article, or claim that may be made by its manufacturer, is not guaranteed or endorsed by the publisher.
Abbreviations
CO2, Carbon Dioxide; CO, Carbon monoxide; H2, Hydrogen; ZnO, Zinc oxide; CeO2, Ceria Oxide; TPO, Temperature-Programmed Oxidation; MS, MassSpectroscopy; SCS, Solution Combustion Synthesis; TR, Thermal Reduction.
References
Abanades, S., and Flamant, G. (2006). Solar hydrogen production from the thermal splitting of methane in a high temperature solar chemical reactor. Sol. Energy 80 (10), 1321–1332. doi:10.1016/j.solener.2005.11.004
Abanades, S., Legal, A., Cordier, A., Peraudeau, G., Flamant, G., and Julbe, A. (2010). Investigation of reactive cerium-based oxides for H2 production by thermochemical two-step water-splitting. J. Mat. Sci. 45 (15), 4163–4173. doi:10.1007/s10853-010-4506-4
Arifin, D., Aston, V. J., Liang, X., McDaniel, A. H., and Weimer, A. W. (2012). CoFe2O4 on a porous Al2O3 nanostructure for solar thermochemical CO2 splitting. Energy Environ. Sci. 5, 9438–9443. doi:10.1039/c2ee22090c
Arshad, Z., Khoja, A. H., Shakir, S., Afzal, A., Mujtaba, M. A., Soudagar, M. E. M., et al. (2021). Magnesium doped TiO2 as an efficient electron transport layer in perovskite solar cells. Case Stud. Therm. Eng. 26, 101101. doi:10.1016/j.csite.2021.101101
Bader, R., Venstrom, L. J., Davidson, J. H., and Lipiński, W. (2013). Thermodynamic analysis of isothermal redox cycling of ceria for solar fuel production. Energy fuels. 27 (9), 5533–5544. doi:10.1021/ef400132d
Barcellos D, R., Sanders, M. D., Tong, J., McDaniel, A. H., and O’Hayre, R. P. (2018). BaCe0.25Mn0.75O3−δ—A promising perovskite-type oxide for solar thermochemical hydrogen production. Energy Environ. Sci. 11 (11), 3256–3265. doi:10.1039/c8ee01989d
Bhosale, R. R., Kumar, A., AlMomani, F., et al. (2016). Assessment of CexZryHfzO2 based oxides as potential solar thermochemical CO2 splitting materials. Ceram. Int. 42 (8), 9354. doi:10.1016/j.ceramint.2016.02.100
Bhosale, R. R., Takalkar, G., Sutar, P., Kumar, A., AlMomani, F., and Khraisheh, M. (2019). A decade of ceria based solar thermochemical H2O/CO2 splitting cycle. Int. J. Hydrog. Energy 44 (1), 34–60. doi:10.1016/j.ijhydene.2018.04.080
Boretti, A., Castelletto, S., and De Angelis, F. (2021). A solar concentrator/receiver/storage/reactor system for thermochemical splitting cycles based on perovskites. Int. J. Hydrogen Energy 47, 4970–4975. doi:10.1016/j.ijhydene.2021.11.094
Boretti, A. (2021). Concentrated solar energy-driven carbon black catalytic thermal decomposition of methane. Int. J. Energy Res. 45, 21497–21508. doi:10.1002/er.7142
Bork, A. H., Kubicek, M., Struzik, M., and Rupp, J. L. M. (2015). Perovskite La0.6Sr0.4Cr1−xCoxO3−δ solid solutions for solar-thermochemical fuel production: Strategies to lower the operation temperature. J. Mat. Chem. A Mat. 3 (30), 15546–15557. doi:10.1039/c5ta02519b
Call, F., Roeb, M., Schmücker, M., Bru, H., Curulla-Ferre, D., Sattler, C., et al. (2013). Thermogravimetric analysis of zirconia-doped ceria for thermochemical production of solar fuel. Am. J. Anal. Chem. 2013, 37–45. doi:10.4236/ajac.2013.410a1005
Charvin, P., Abanades, S., Beche, E., Lemont, F., and Flamant, G. (2009). Hydrogen production from mixed cerium oxides via three-step water-splitting cycles. Solid State Ion. 14 (180), 1003–1010. doi:10.1016/j.ssi.2009.03.015
Cho, H., Gokon, N., Kodama, T., Kang, Y., and Lee, H. (2015). Improved operation of solar reactor for two-step water-splitting H2 production by ceria-coated ceramic foam device. Int. J. Hydrogen Energy 40 (1), 114–124. doi:10.1016/j.ijhydene.2014.10.084
Chueh, W. C., Falter, C., Abbott, M., Scipio, D., Furler, P., Haile, S. M., et al. (2010). High-flux solar-driven thermochemical dissociation of CO2 and H2O using nonstoichiometric ceria. Science 330, 1797–1801. doi:10.1126/science.1197834
Chueh, W. C., and Haile, S. M. (2010). A thermochemical study of ceria: Exploiting an old material for new modes of energy conversion and CO2 mitigation. Phil. Trans. R. Soc. A 368 (1923), 3269–3294. doi:10.1098/rsta.2010.0114
Chueh, W. C., and Haile, S. M. (2009). Ceria as a thermochemical reaction medium for selectively generating syngas or methane from H2O and CO2. ChemSusChem 2 (8), 735–739. doi:10.1002/cssc.200900138
Cooper, T., Scheffe, J. R., Galvez, M. E., Jacot, R., Patzke, G., and Steinfeld, A. (2015). Lanthanum manganite perovskites with Ca/Sr A-site and Al B-site doping as effective oxygen exchange materials for solar thermochemical fuel production. Energy Technol. 3, 1130–1142. doi:10.1002/ente.201500226
Demont, A., Abanades, S., and Beche, E. (2014). Investigation of perovskite structures as oxygen-exchange redox materials for hydrogen production from thermochemical two-step water-splitting cycles. J. Phys. Chem. C 118, 12682–12692. doi:10.1021/jp5034849
Demont, A., and Abanades, S. (2015). Solar thermochemical conversion of CO2 into fuel via two-step redox cycling of non-stoichiometric Mn-containing perovskite oxides. J. Mat. Chem. A Mat. 3 (7), 3536–3546. doi:10.1039/c4ta06655c
Dey, S., Naidu, B. S., Govindaraj, A., and Rao, C. N. R. (2015). Noteworthy performance of La1−xCaxMnO3 perovskites in generating H2 and CO by the thermochemical splitting of H2O and CO2. Phys. Chem. Chem. Phys. 17, 122–125. doi:10.1039/c4cp04578e
Dey, S., Naidu, B. S., and Rao, C. N. R. (2016). Beneficial effects of substituting trivalent ions in the B-site of La0.5Sr0.5Mn1−xAxO3 (A = Al, Ga, Sc) on the thermochemical generation of CO and H2 from CO2 and H2O. Dalton Trans. 45 (6), 2430–2435. doi:10.1039/c5dt04822b
Dey, S., Naidu, B. S., and Rao, C. N. R. (2015). Ln0.5A0.5MnO3 (Ln=Lanthanide, A= Ca, Sr) perovskites exhibiting remarkable performance in the thermochemical generation of CO and H2 from CO2 and H2O. Chem. Eur. J. 21 (19), 7077–7081. doi:10.1002/chem.201500442
Ezbiri, M., Takacs, M., Stolz, B., Lungthok, J., Steinfeld, A., and Michalsky, R. (2017). Design principles of perovskites for solar-driven thermochemical splitting of CO2. J. Mater. Chem. A 5, 15105–15115. doi:10.1039/c7ta02081c
Ezbiri, M., Takacs, M., Theiler, D., Michalsky, R., and Steinfeld, A. (2017). Tunable thermodynamic activity of LaxSr1−xMnyAl1−yO3−δ (0 ≤ x ≤ 1, 0 ≤ y ≤ 1) perovskites for solar thermochemical fuel synthesis. J. Mat. Chem. A Mat. 5, 4172–4182. doi:10.1039/c6ta06644e
Furler, P., Scheffe, J., Gorbar, M., Moes, L., Vogt, U., and Steinfeld, A. (2012). Solar thermochemical CO2 splitting utilizing a reticulated porous ceria redox system. Energy fuels. 26, 7051–7059. doi:10.1021/ef3013757
Furler, P., Scheffe, J., Marxer, D., Michal, G., Bonk, A., Ulrich, V., et al. (2014). Thermochemical CO2 splitting via redox cycling of ceria reticulated foam structures with dual-scale porosities. Phys. Chem. Chem. Phys. 16 (22), 10503–10511. doi:10.1039/C4CP01172D
Furler, P., Scheffe, J. R., and Steinfeld, A. (2012). Syngas production by simultaneous splitting of H2O and CO2 via ceria redox reactions in a high-temperature solar reactor. Energy & Environ. Sci. 5 (3), 6098–6103. doi:10.1039/C1EE02620H
Gao, X., Vidal, A., Bayon, A., Bader, R., Hinkley, J., Lipinski, W., et al. (2016). Efficient ceria nanostructures for enhanced solar fuel production via high-temperature thermochemical redox cycles. J. Mat. Chem. A Mat. 4 (24), 9614–9624. doi:10.1039/c6ta02187e
Garba, M. D., Usman, M., Khan, S., Shehzad, F., Galadima, A., Ehsan, M. F., et al. (2021). CO2 towards fuels: A review of catalytic conversion of carbon dioxide to hydrocarbons. J. Environ. Chem. Eng. 9, 104756. doi:10.1016/j.jece.2020.104756
Glenk, G., and Reichelstein, S. (2019). Economics of converting renewable power to hydrogen. Nat. Energy 4, 216–222. doi:10.1038/s41560-019-0326-1
Gokon, N., Hara, K., Sugiyama, Y., Bellan, S., Kodama, T., and Hyun-seok, C. (2019). Thermochemical two-step water splitting cycle using perovskite oxides based on LaSrMnO3 redox system for solar H2 production. Thermochim. Acta 680, 178374. doi:10.1016/j.tca.2019.178374
Haeussler, A., Abanades, S., Julbe, A., Jouannaux, J., and Cartoixa, B. (2020). Solar thermochemical fuel production from H2O and CO2 splitting via two-step redox cycling of reticulated porous ceria structures integrated in a monolithic cavity-type reactor. Energy 201, 117649. doi:10.1016/j.energy.2020.117649
Hao, Y., Yang, C-K., and Haile, S. M. (2013). High-temperature isothermal chemical cycling for solar-driven fuel production. Phys. Chem. Chem. Phys. 15 (40), 17084–17092. doi:10.1039/c3cp53270d
Hathaway, B. J., Bala Chandran, R., Gladen, A. C., Chase, T. R., and Davidson, J. H. (2016). Demonstration of a solar reactor for carbon dioxide splitting via the isothermal ceria redox cycle and practical implications. Energy fuels. 30, 6654–6661. doi:10.1021/acs.energyfuels.6b01265
Helal, A., Cordova, K. E., Arafat, M. E., Usman, M., and Yamani, Z. H. (2020). Defect-engineering a metal–organic framework for CO2 fixation in the synthesis of bioactive oxazolidinones. Inorg. Chem. Front. 7, 3571–3577. doi:10.1039/d0qi00496k
Jacobson, T. A., Kler, J. S., Hernke, M. T., Braun, R. K., Meyer, K. C., and Funk, W. E. (2019). Direct human health risks of increased atmospheric carbon dioxide. Nat. Sustain. 2, 691–701. doi:10.1038/s41893-019-0323-1
Jiang, Q., Zhou, G., Jiang, Z., and Li, C. (2014). Thermochemical CO2 splitting reaction with CexM1−xO2−δ (M=Ti4+, Sn4+, Hf4+, Zr4+, La3+, Y3+ and Sm3+) solid solutions. Sol. Energy 99, 55–66. doi:10.1016/j.solener.2013.10.021
Kaneko, H., Ishihara, H., Taku, S., Naganuma, Y., Hasegawa, N., and Tamaura, Y. (2008). Cerium ion redox system in CeO2–xFe2O3 solid solution at high temperatures (1, 273–1, 673 K) in the two-step water-splitting reaction for solar H2 generation. J. Mat. Sci. 43 (9), 3153–3161. doi:10.1007/s10853-008-2499-z
Kaneko, H., Taku, S., and Tamaura, Y. (2011). Reduction reactivity of CeO2–ZrO2 oxide under high O2 partial pressure in two-step water splitting process. Sol. Energy 85 (9), 2321–2330. doi:10.1016/j.solener.2011.06.019
Kaneko, H., and Tamaura, Y. (2009). Reactivity and XAFS study on (1−x) CeO2–xNiO (x= 0.025–0.3) system in the two-step water-splitting reaction for solar H2 production. J. Phys. Chem. Solids 70 (6), 1008–1014. doi:10.1016/j.jpcs.2009.05.015
Kang, M., Wu, X., Zhang, J., Zhao, N., Wei, W., and Sun, Y. (2014). Enhanced thermochemical CO 2 splitting over Mg-and Ca-doped ceria/zirconia solid solutions. RSC Adv. 4 (11), 5583–5590. doi:10.1039/c3ra45595e
Kettner, M., Ramsthaler, F., Juhnke, C., Bux, R., and Schmidt, P. (2013). A fatal case of CO(2) intoxication in a fermentation tank. J. Forensic Sci. 58, 556–558. doi:10.1111/1556-4029.12058
Kodama, T., Kondoh, Y., Yamamoto, R., Andou, H., and Satou, N. (2005). Thermochemical hydrogen production by a redox system of ZrO2-supported Co(II)-ferrite. Sol. Energy 78, 623–631. doi:10.1016/j.solener.2004.04.008
Langford, N. J. (2005). Carbon dioxide poisoning. Toxicol. Rev. 24, 229–235. doi:10.2165/00139709-200524040-00003
Lapp, J., Davidson, J. H., and Lipiński, W. (2012). Efficiency of two-step solar thermochemical non-stoichiometric redox cycles with heat recovery. Energy 37 (1), 591–600. doi:10.1016/j.energy.2011.10.045
Le Gal, A., and Abanades, S. (2011). Catalytic investigation of ceria-zirconia solid solutions for solar hydrogen production. Int. J. Hydrogen Energy 36 (8), 4739–4748. doi:10.1016/j.ijhydene.2011.01.078
Le Gal, A., and Abanades, S. (2012). Dopant incorporation in ceria for enhanced water-splitting activity during solar thermochemical hydrogen generation. J. Phys. Chem. C 116 (25), 13516–13523. doi:10.1021/jp302146c
Le Gal, A., Abanades, S., and Flamant, G. (2011). CO2 and H2O splitting for thermochemical production of solar fuels using nonstoichiometric ceria and ceria/zirconia solid solutions. Energy fuels. 25 (10), 4836–4845. doi:10.1021/ef200972r
Lin, F., Samson, V. A., Wismer, A. O., Grolimund, D., Alxneit, I., and Wokaun, A. (2016). Zn-modified ceria as a redox material for thermochemical H2O and CO2 splitting: Effect of a secondary ZnO phase on its thermochemical activity. CrystEngComm 18, 2559–2569. doi:10.1039/c6ce00430j
Luciani, G., Landi, G., Aronne, A., and Di Benedetto, A. (2018). Partial substitution of B cation in La0.6Sr0.4MnO3 perovskites: A promising strategy to improve the redox properties useful for solar thermochemical water and carbon dioxide splitting. Sol. Energy 171, 1–7. doi:10.1016/j.solener.2018.06.058
Marxer, D., Furler, P., Scheffe, J., Geerlings, H., Falter, C., Batteiger, V., et al. (2015). Demonstration of the entire production chain to renewable kerosene via solar thermochemical splitting of H2O and CO2. Energy fuels. 29 (5), 3241–3250. doi:10.1021/acs.energyfuels.5b00351
McDaniel, A. H., Miller, E. C., Arifin, D., Ambrosini, A., Coker, E. N., O'Hayre, R., et al. (2013). Sr- and Mn-doped LaAlO3−δ for solar thermochemical H2 and CO production. Energy Environ. Sci. 6 (8), 2424–2428. doi:10.1039/c3ee41372a
Meng, Q., Lee, C., Ishihara, T., Kaneko, H., and Tamaura, Y. (2011). Reactivity of CeO2-based ceramics for solar hydrogen production via a two-step water-splitting cycle with concentrated solar energy. Int. J. Hydrogen Energy 36 (21), 13435–13441. doi:10.1016/j.ijhydene.2011.07.089
Meng, Q-L., and Tamaura, Y. (2014). Enhanced hydrogen production by doping Pr into Ce0. 9Hf0.1O2 for thermochemical two-step water-splitting cycle. J. Phys. Chem. Solids 75 (3), 328–333. doi:10.1016/j.jpcs.2013.07.023
Miller, J. E., Allendorf, M. D., Diver, R. B., Evans, L. R., Siegel, N. P., and Stuecker, J. N. (2008). Metal oxide composites and structures for ultra-high temperature solar thermochemical cycles. J. Mat. Sci. 43 (14), 4714–4728. doi:10.1007/s10853-007-2354-7
Mofijur, M., Atabani, A. E., Masjuki, H. H., Kalam, M. A., and Masum, B. M. (2013). A study on the effects of promising edible and non-edible biodiesel feedstocks on engine performance and emissions production: A comparative evaluation. Renew. Sustain. Energy Rev. 23, 391–404. doi:10.1016/j.rser.2013.03.009
Mofijur, M., Masjuki, H. H., Kalam, M. A., and Atabani, A. E. (2013). Evaluation of biodiesel blending, engine performance and emissions characteristics of Jatropha curcas methyl ester: Malaysian perspective. Energy 55, 879–887. doi:10.1016/j.energy.2013.02.059
Muhich, C. L., Evanko, B. W., Weston, K. C., Lichty, P., Liang, X., Martinek, J., et al. (2013). Efficient generation of H2 by splitting water with an isothermal redox cycle. Science 341, 540–542. doi:10.1126/science.1239454
Mulmi, S., Chen, H., Hassan, A., Marco, J. F., Berry, F. J., Sharif, F., et al. (2017). Thermochemical CO2 splitting using double perovskite-type Ba2Ca0.66Nb1.34-x. J. Mater. Chem. A 5, 6874–6883. doi:10.1039/C6TA10285A
Mustafa, A., Lougou, B. G., Shuai, Y., Wang, Z., and Tan, H. (2020). Current technology development for CO2 utilization into solar fuels and chemicals: A review. J. Energy Chem. 49, 96–123. doi:10.1016/j.jechem.2020.01.023
Nair Mahesh, M., and Abanades, S. (2018). Experimental screening of perovskite oxides as efficient redox materials for solar thermochemical CO2 conversion. Sustain. Energy Fuels 2 (4), 843–854. doi:10.1039/c7se00516d
Nair, M. M., and Abanades, S. (2016). Insights into the redox performance of non–stoichiometric lanthanum manganite perovskites for solar thermochemical CO2 splitting. ChemistrySelect 1 (15), 4449–4457. doi:10.1002/slct.201601171
Nair, M. M., and Abanades, S. (2016). Tailoring Hybrid nonstoichiometric ceria redox cycle for combined solar methane reforming and thermochemical conversion of H2O/CO2. Energy Fuels 30, 6050–6058. doi:10.1021/acs.energyfuels.6b01063
Parvanian, A. M., Salimijazi, H., Shabaninejad, M., Troitzsch, U., Kreider, P., Lipiński, W., et al. (2020). Thermochemical CO2 splitting performance of perovskite coated porous ceramics. RSC Adv. 10, 23049–23057. doi:10.1039/d0ra02353a
Permentier, K., Vercammen, S., Soetaert, S., and Schellemans, C. (2017). Carbon dioxide poisoning: A literature review of an often forgotten cause of intoxication in the emergency department. Int. J. Emerg. Med. 10, 14. doi:10.1186/s12245-017-0142-y
Petkovich, N. D., Rudisill, S. G., Venstrom, L. J., Boman, D. B., Davidson, J. H., and Stein, A. (2011). Control of heterogeneity in nanostructured ce1–x zr x o2 binary oxides for enhanced thermal stability and water splitting activity. J. Phys. Chem. C 115 (43), 21022–21033. doi:10.1021/jp2071315
Rafique, M., Safdar, N., Irshad, M., Usman, M., Akhtar, M., Saleem, M. W., et al. (2022). Influence of low sintering temperature on BaCe0.2Zr0.6Y0.2O3−δ IT-SOFC perovskite Electrolyte synthesized by Co-precipitation method. Materials 15, 3585. doi:10.3390/ma15103585
Ramos, A. E., Maiti, D., Daza, Y. A., Kuhn, J. N., and Bhethanabotla, V. R. (2019). Co, Fe, and Mn in La-perovskite oxides for low temperature thermochemical CO2 conversion. Catal. Today 338, 52–59. doi:10.1016/j.cattod.2019.04.028
Riaz, A., Ali, M. U., Enge, T. G., Tsuzuki, T., Lowe, A., and Lipiski, W. (2020). Concentration-dependent solar thermochemical CO2/H2O splitting performance by vanadia-ceria multiphase metal oxide systems. Research 2020, 3049534. doi:10.34133/2020/3049534
Roeb, M., Neises, M., Monnerie, N., Call, F., Simon, H., Sattler, C., et al. (2012). Materials-related aspects of thermochemical water and carbon dioxide splitting: A review. Materials 5 (11), 2015–2054. doi:10.3390/ma5112015
Sastre, D., Carrillo, A. J., Serrano, D. P., Pizarro, P., and Coronado, J. M. (2017). Exploring the redox behavior of La0.6Sr0.4 Mn1−xAlxO3 perovskites for CO2-splitting in thermochemical cycles. Top. Catal. 60, 1108–1118. doi:10.1007/s11244-017-0790-4
Sastre, D., Carrillo, A. J., Serrano, D. P., Pizarro, P., and Coronado, J. M. (2017). Exploring the redox behavior of La0.6Sr0.4Mn1−xAlxO3 perovskites for CO2-splitting in thermochemical cycles. Top. Catal. 60, 1108–1118. doi:10.1007/s11244-017-0790-4
Scheffe, J. R., Jacot, R., Patzke, G. R., and Steinfeld, A. (2013). Synthesis, characterization, and thermochemical redox performance of Hf4+, Zr4+, and Sc3+ doped ceria for splitting CO2. J. Phys. Chem. C 117 (46), 24104–24114. doi:10.1021/jp4050572
Scheffe, J. R., McDaniel, A. H., Allendorf, M. D., and Weimer, A. W. (2013). Kinetics and mechanism of solar-thermochemical H2 production by oxidation of a cobalt ferrite–zirconia composite. Energy Environ. Sci. 6, 963–973. doi:10.1039/c3ee23568h
Scheffe, J. R., and Steinfeld, A. (2014). Oxygen exchange materials for solar thermochemical splitting of H2O and CO2: A review. Mater. Today 17, 341–348. doi:10.1016/j.mattod.2014.04.025
Scheffe, J. R., Weibel, D., and Steinfeld, A. (2013). Lanthanum–strontium–manganese perovskites as redox materials for solar thermochemical splitting of H2O and CO2. Energy fuels. 27, 4250–4257. doi:10.1021/ef301923h
Scheffe, J. R., Welte, M., and Steinfeld, A. (2013). “Reduction of cerium dioxide in an aerosol tubular reactor for the thermal dissociation of CO2 and H2O,” in Paper presented at: Abstracts of Papers of the American Chemical Society, New Orleans, LA, April 7 - 11.
Shahabuddin, M., Alim, M. A., Alam, T., Mofijur, M., Ahmed, S. F., and Perkins, G. (2021). A critical review on the development and challenges of concentrated solar power technologies. Sustain. Energy Technol. Assessments 47, 101434. doi:10.1016/j.seta.2021.101434
Snoeckx, R., and Bogaerts, A. (2017). Plasma technology – A novel solution for CO2 conversion? Chem. Soc. Rev. 46, 5805–5863. doi:10.1039/c6cs00066e
Takacs, M., Hoes, M., Caduff, M., Cooper, T., Scheffe, J. R., and Steinfeld, A. (2016). Oxygen nonstoichiometry, defect equilibria, and thermodynamic characterization of LaMnO3 perovskites with Ca/Sr A-site and Al B-site doping. Acta Mater. 103, 700–710. doi:10.1016/j.actamat.2015.10.026
Takalkar, G., Bhosale, R. R., AlMomani, F., Rashid, S., Qiblawey, H., Saleh Saad, M. A., et al. (2021). Thermochemical splitting of CO2 using solution combustion synthesized lanthanum–strontium–manganese perovskites. Fuel (Lond). 285, 119154. doi:10.1016/j.fuel.2020.119154
Wang, L., Al-Mamun, M., Liu, P., Wang, Y., Gui Yang, H., and Zhao, H. (2017). La1-xCaxMn1-yAlyO3 perovskites as efficient catalysts for two-step thermochemical water splitting in conjunction with exceptional hydrogen yields. Chin. J. Catal. 38 (6), 1079–1086. doi:10.1016/S1872-2067(17)62820-1
Wang, L., Al-Mamun, M., Liu, P., Wang, Y., Yang, H. G., and Zhao, H. (2018). Notable hydrogen production on LaxCa1−xCoO3 perovskites via two-step thermochemical water splitting. J. Mat. Sci. 53 (9), 6796–6806. doi:10.1007/s10853-018-2004-2
Wang, L., Al-Mamun, M., Zhong, Y. L., Jiang, L., Liu, P., Wang, Y., et al. (2017). Ca2+ and Ga3+ doped LaMnO3 perovskite as a highly efficient and stable catalyst for two-step thermochemical water splitting. Sustain. Energy Fuels 1 (5), 1013–1017. doi:10.1039/c6se00097e
Wang, L., Al-Mamun, M., Zhong, Y. L., Liu, P., Wang, Y., Yang, H. G., et al. (2018). Enhanced thermochemical water splitting through formation of oxygen vacancy in La0.6Sr0.4BO3−δ (B=Cr, Mn, Fe, Co, and Ni) perovskites. Chempluschem 83 (10), 924–928. doi:10.1002/cplu.201800178
Żaba, C., Marcinkowski, J. T., Wojtyła, A., Tężyk, A., Tobolski, J., and Zaba, Z. (2011). Acute collective gas poisoning at work in a manure storage tank. Ann. Agric. Environ. Med. 18, 448
Keywords: thermochemical splitting, ceria, perovskites, carbon dioxide emission, climate change
Citation: Rony ZI, Mofijur M, Ahmed SF, Kabir Z, Chowdhury AA and Almomani F (2022) Recent advances in the solar thermochemical splitting of carbon dioxide into synthetic fuels. Front. Energy Res. 10:982269. doi: 10.3389/fenrg.2022.982269
Received: 30 June 2022; Accepted: 06 September 2022;
Published: 03 October 2022.
Edited by:
M.A. Mujtaba, University of Engineering and Technology, PakistanReviewed by:
Fahad Noor, University of Engineering and Technology, PakistanManzoore Elahi Soudagar, Chandigarh University, India
Muhammad Amjad, University of Engineering and Technology, Pakistan
Copyright © 2022 Rony, Mofijur, Ahmed, Kabir, Chowdhury and Almomani. This is an open-access article distributed under the terms of the Creative Commons Attribution License (CC BY). The use, distribution or reproduction in other forums is permitted, provided the original author(s) and the copyright owner(s) are credited and that the original publication in this journal is cited, in accordance with accepted academic practice. No use, distribution or reproduction is permitted which does not comply with these terms.
*Correspondence: Fares Almomani, ZmFsbW9tYW5pQHF1LmVkdS5xYQ==